- 1Centre for the Research and Technology of Agro-Environmental and Biological Sciences (CITAB), University of Trás-os-Montes e Alto Douro (UTAD), Vila Real, Portugal
- 2Institute for Innovation, Capacity Building and Sustainability of Agri-Food Production (Inov4Agro), University of Trás-os-Montes e Alto Douro, Vila Real, Portugal
- 3Cermouros-Cerejas de São Martinho de Mouros, Lda., Resende, Portugal
- 4Department of Biotechnology and Biomedicine, Technical University of Denmark, Lyngby, Denmark
Sweet cherry is a high-value crop, and strategies to enhance production and sustainability are at the forefront of research linked to this crop. The improvement of plant status is key to achieving optimum yield. Biostimulants, such as glycine betaine (GB) or seaweed-based biostimulants [e.g., Ecklonia maxima (EM)], can represent a sustainable approach to improving plant conditions, even under adverse environmental circumstances. Despite their potential, few studies have focused on the effects of GB or EM exogenous application on sweet cherry tree physiology. To address this lack of research, a study was conducted in a Portuguese sweet cherry commercial orchard, using Lapins and Early Bigi cultivars. Trees were treated with products based on GB and EM at two different concentrations [GB 0.25% (v/v) and GB 0.40% (v/v); EM 0.30% (v/v) and EM 0.15% (v/v)], a combination of the lowest concentrations of both biostimulants (Mix —GB 0.25% and EM 0.15%), and a control group (C) treated with water. Applications were performed over three consecutive years (2019, 2020, and 2021) at three different phenological stages, according to the BBCH scale: 77, 81, and 86 BBCH. Results showed, in general, that the application of biostimulants led to improvements in water status as well as significantly lower values of electrolyte leakage and thiobarbituric acid reactive substances compared to C samples. Additionally, biostimulants reduced pigment loss in the leaves and enhanced their biosynthesis. The Chlorophylla/Chlorophyllb ratio, ranging from 2 to 4, indicated a greater capacity for light absorption and lower stress levels in treated leaves. Soluble sugar and starch content decreased during fruit development in both cultivars and years; however, biostimulants increased these contents, with increments of approximately 15% to 30% in leaves treated with EM. Soluble protein content also showed the same pattern for treated leaves. Biostimulants, especially EM, demonstrated a significant positive effect (p ≤ 0.001) on total phenolic content, with increases of approximately 25% to 50% in treated leaves. In conclusion, the application of biostimulants, especially algae-based, significantly improved tree performance by enhancing physiological parameters and stress resilience and could represent a novel approach in fruit production systems.
1 Introduction
Climate change, driven by human activities, is one of the greatest challenges of the current century, as it threatens agricultural production and human wellbeing (Bantis and Koukounaras, 2022). The latest Assessment Report of the Intergovernmental Panel on Climate Change (IPCC) projects a continued increase in global average surface temperatures and a decrease in annual precipitation, particularly affecting southern European countries such as Portugal (IPCC and Core Writing Team, 2023). This scenario, coupled with the rising global trade of plants and fruits, driven by population growth and consumer demand for a consistent supply of high-quality products, underscores the urgent need to adopt sustainable agricultural practices. Implementing resource-efficient strategies that align with the principles of the circular economy can help address these challenges (Afonso et al., 2022; Asif et al., 2023; Zulfiqar et al., 2024).
In this context, biostimulants are gaining recognition within the scientific community and businesses alike as a sustainable and eco-friendly alternative to conventional agrochemicals (du Jardin, 2015; Rouphael and Colla, 2020; Basile et al., 2021; Muhie, 2023). When used with good agricultural practices, biostimulants can enhance crop development and productivity and resilience against biotic and abiotic stresses (Moreno-Hernández et al., 2020). Among the seven categories of biostimulants identified by du Jardin (2015), glycine betaine (GB) and seaweed extracts are noteworthy, though their use in cherry tree cultivation remains relatively unexplored (Correia et al., 2020a; Correia et al., 2020b; Serapicos et al., 2022). Given the susceptibility of cherry trees to adverse weather conditions, studying these biostimulants offers a promising approach to improving resilience and productivity, even in challenging environmental circumstances (Rojas et al., 2021; Tudela et al., 2023; Salvadores and Bastías, 2023).
GB, a quaternary amine, plays a role in maintaining membrane integrity under abiotic stress, as it is recognized for its osmoregulatory and osmoprotective functions against drought, salinity, and extreme temperatures (Khalid et al., 2015; Rasheed et al., 2018; Dutta et al., 2019; Fedotova, 2019; Sharma et al., 2023). Its exogenous application in crops has been linked with increased yield potential and improved quality (Shan et al., 2016; Gonçalves et al., 2020; Khalid et al., 2022; Zulfiqar et al., 2022). Similarly, the foliar application of brown macroalgae Ascophyllum nodosum and Ecklonia maxima seaweed-based biostimulants has been shown to promote plant growth, increase yields, and enhance fruit protein and nutrient content (Jolayemi et al., 2023). Furthermore, it improves leaf health and enhances plant tolerance to abiotic and biotic stress (Parađiković et al., 2019; Ali et al., 2021), due to their complex composition, rich in plant hormones, proteins, amino acids, sugars, vitamins, and phenolic compounds (Gonçalves et al., 2020; Afonso et al., 2022). Despite extensive research on the application of biostimulants in various fruit tree species (Basile et al., 2020; Mones Sardrodi et al., 2022; Serapicos et al., 2022), scientific evidence on the effects of such application on cherry tree performance is scarce. To the best of our knowledge, there is no available information regarding studies on the effects of E. maxima seaweed-based biostimulants on cherry tree physiology. Additionally, limited studies are focusing on the application of GB.
In this regard, this study aims to fill this research gap by evaluating the effect of pre-harvest treatments with GB and E. maxima seaweed extracts on the physiological and biochemical responses of Early Bigi and Lapins cherry cultivars grown in northern Portugal.
2 Materials and methods
2.1 Experimental site and plant material
The study was carried out at Quinta da Alufinha, municipality of Resende, Portugal (latitude 41°06′ N and longitude 7°54′ W), on a 7-year-old sweet cherry commercial orchard, located at a low altitude (140 m above sea level), in the years of 2019, 2020, and 2021. Trees were trained under a vertical axis system with a spacing of 3.0 m between rows and 2.5 m in the row. Between March and August, trees were drip-irrigated daily, ensuring a uniform water supply. In addition, trees were also periodically fertilized. Standard meteorological variables [air temperature (°CC), rainfall (mm), and solar radiation (W m−2)] for the 3 years were recorded by an automatic weather station set up near the experimental site (Figure 1). In 2019, the average air temperature between March and May was approximately 1.42°C lower than that of 2020, and 1.09°C higher than that of 2021. Based on the annual precipitation data, the year 2019 recorded the highest annual rainfall (1,163 mm), followed by 2020 (1,091 mm), and 2021 (1,076 mm). Furthermore, the mean solar radiation until May was also higher in 2019 than in the other 2 years. Compared to climate normals available (1971–2000), all 3 years were drier (especially 2021, with 20% less rainfall), while, regarding temperature, all 3 years were all hotter than the 30-year average, namely, 2020 (average hotter by 1°C).
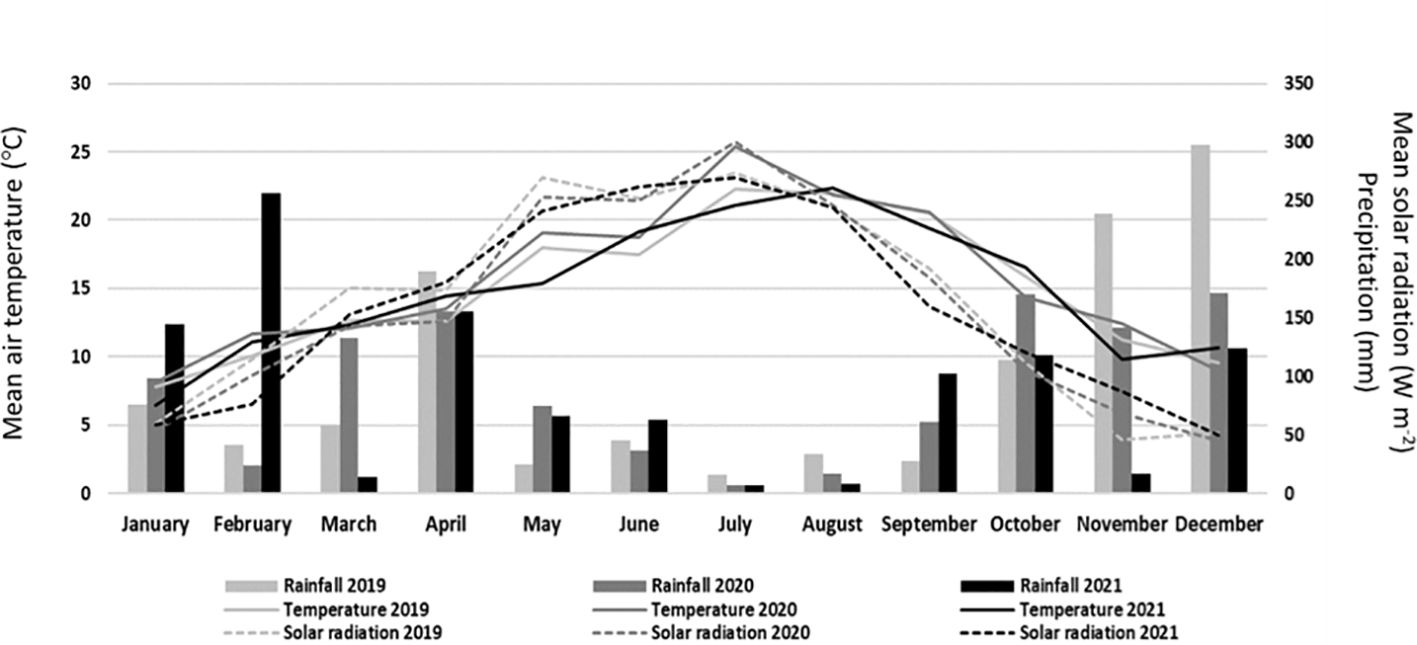
Figure 1. Monthly mean air temperature (°C), precipitation (mm), and mean solar radiation (W m−2) in 2019, 2020, and 2021.
The early cultivar (cv.) Early Bigi and the late cv. Lapins, both grafted onto “Santa Lucia 64”, were selected for this trial, due to its economic importance of this region.
2.2 Experimental design and treatments
Eight trees from each cultivar, homogeneous, and in good phytosanitary conditions, were chosen for each treatment, resulting in a total of 48 trees per cultivar. Six treatments (expressed as % of volume/volume) were foliar sprayed, using a mechanical sprayer, and they included two concentrations of GB (97%) (GB 0.25% and GB 0.40%), two concentrations of E. maxima seaweed-based biostimulants (EM 0.30% and EM 0.15%), a combination of the lowest concentrations of both biostimulants (Mix —GB 0.25 and EM 0.15), and a control (C) group (water). Foliar treatments were repeated at three different dates during the phenological stages of the cherry tree, according to the BBCH scale [Biologische Bundesantalt, Bundessortenamt und Chemische Industrie (Fadon et al., 2015)]: stage 77 (70% of final fruit size), stage 81 (beginning of fruit coloring), and stage 86 (coloring advanced, 3 days before fruit harvesting). To achieve more accurate results, treatments (Table 1) were applied for three consecutive years (2019, 2020, and 2021), in a windless morning (Table 2).
Healthy, fully expanded, sun-exposed, and mature leaves in a similar position were carefully chosen for the assessment of gas exchange, electrolyte leakage (EL), and relative water content (RWC). These assessments were carried out at solar noon in both cultivars, 3 days after the application of the biostimulants, for the years 2019 and 2021. Additionally, about seven leaves per tree and treatment were collected for biochemical analyses and were immediately frozen in liquid nitrogen, ground to a fine powder, and then stored at −80°C for further analysis. In 2020, despite the foliar applications of the treatments being carried out, it was impossible to conduct these assessments due to the COVID-19 pandemic.
2.3 Leaf gas exchange
Leaf gas exchange measurements were accomplished using a portable infrared gas analyzer system (LC Pro+, ADC, Hoddesdon, UK). The IRGA was equipped with a 2. 5-cm2 leaf chamber (ADC-PLC) and operated in open mode. Incident photosynthetic photon flux density (PPFD) on the leaves was always greater than 1,500 μmol m−2 s−1. The measurements were performed on cloudless days, under natural light conditions, and at solar noon. Through the formulas proposed by von Caemmerer and Farquhar (1981), the net photosynthetic rate (A, μmol m−2 s−1), transpiration rate (E, mmol m−2 s−1), stomatal conductance (gs, mmol m−2 s−1), and intercellular CO2 concentration (Ci, µmol mol−1) were calculated. The intrinsic water use efficiency was calculated as the ratio of A/gs (A/gs, µmol mol−1), to dismiss the potential effects of air humidity and temperature on transpiration (Iacono et al., 1998). Measurements were only performed in the year 2019 (for cv. Early Bigi on April 14, April 22, and May 3, and for cv. Lapins on April 14, May 19, and May 27), as the portable infrared gas analyzer system was unavailable in 2021. Results are expressed as the mean ± standard deviation (SD) of eight replicates.
2.4 Leaf water status
For water status analysis, detached sweet cherry leaves were immediately placed into airtight containers with the petiole facing downwards. Fresh weight (FW, g) was measured, and after immersing leaf petioles in demineralized water for 48 h in the dark at 4°C, turgid weight (TW, g) was measured. Dry weight (DW, g) was determined after drying at 70°C until a constant weight was achieved. Leaf area (LA, cm2) was also measured using the WinDias image analysis system (Delta-T Devices Ltd., Cambridge, UK). Furthermore, the RWC was calculated as:
Leaf mass per unit area (LMA) (g m−2) was calculated by the ratio between leaf area and DW. Results are expressed as the mean ± standard error (SE) of eight replicates.
2.5 Metabolite composition determination
2.5.1 Photosynthetic pigments
The chlorophylls and carotenoids were extracted with 80% acetone by weighing 25 mg into a screw tube and adding 4 mL of 80% acetone solution. The mixture was then homogenized using a vortex and sonicated for 5 min at 30 Hz. Subsequently, the mixture was centrifuged at 4,000 rpm at 4°C for 10 min. Next, 200 μL of each sample was transferred to a 96-well microplate, and absorbance readings were taken at 470, 645, and 663 nm against a blank. Chlorophyll a (Chla), chlorophyll b (Chlb), and total chlorophyll [Chl(a+b)] were determined according to Arnon (1949) and Sestak et al. (1971), and total carotenoids (Cartotal) were determined according to Lichtenthaler (1987). Additionally, ratios Chla/Chlb and Chl(a+b)/Cartotal were also determined. All procedures were performed under dim light to prevent photodegradation, isomerization, and structural changes of photosynthetic pigments, and results were expressed as mg g−1 DW, as the mean ± SD of eight replicates.
2.5.2 Total soluble sugars and starch
Total soluble sugars (SS) were quantified using the spectrophotometry method described by Irigoyen et al. (1992). Samples were heated in ethanol/water (80/20, v/v) for 1 h at 80°C. TSS were measured, at 625 nm, after the alcoholic extract reacted with fresh anthrone in a boiling water bath for 10 min. The soluble fractions were then separated from the solid fraction. Starch (St) was extracted from the same solid fraction by heating it in 30% perchloric acid for 1 h, at 60°C, following the method of Osaki et al. (1991). The St concentration was determined by the anthrone method, as previously described, and both SS and St are expressed as mg g−1 DW, using glucose as a standard, presented as the mean ± SD of eight replicates.
2.5.3 Soluble proteins
Total soluble protein (TP) quantification followed the Bradford (1976) method. Total soluble proteins were extracted using a phosphate buffer (pH 7.5) containing 0.1 mM ethylenediaminetetraacetic acid (EDTA), 100 mM phenyl-methylsulfonyl fluoride (PMSF), and 2% (w/v) polyvinylpyrrolidone (PVP), followed by centrifugation at 12,000×g at 4°C, for 30 min. Absorbance was measured at 595 nm using bovine serum albumin (BSA) as a standard. The results, expressed as mg g−1 DW, were the mean ± SD of eight replicates.
2.5.4 Total phenolics
For the quantification of total phenolics, a previous extraction was performed, as previously described by Serapicos et al. (2022): 40 mg of each sample (DW) was added to 1.5 mL of 70% (v/v) methanol, mixed thoroughly on a vortex for 30 min, and centrifuged at 5,000 rpm at 4°C for 15 min. The supernatant was collected into a 10-mL volumetric flask. This procedure was repeated three more times, with the final volume adjusted to 10 mL using methanol. Total phenolics were then determined using the method by Singleton and Rossi (1965) and Dewanto et al. (2002), with some modifications. In each well of a 96-well microplate, 20 µL of each leaf extract, 100 µL of Folin-Ciocalteu reagent (1:10 in bidistilled water), and 80 µL of 7.5% Na2CO3 were mixed. The microplate was incubated in the dark for 15 min at 45°C. Absorbance values were measured at 765 nm against a blank. The colorimetric response of total phenols measurements was compared to a standard curve based on gallic acid, and the results were expressed as mg gallic acid equivalents (GAE) g−1 DW, as the mean ± SD of eight replicates.
2.6 Cell membrane damage
2.6.1 Electrolyte leakage
Leaf EL was measured to assess cell membrane permeability, based on the method described by Mena-Petite et al. (2001) with modifications. After collecting the leaves, they were washed in deionized water to remove surface ions. Foliar discs with a diameter of 0.8 cm were then punched out from each leaf and placed in test tubes with 10 mL of deionized water. Incubation at 25°C was carried out for 24 h on a rotary shaker. After incubation, the electrical conductivity of the solution (CE1) was measured using a conductivity meter (Mettler Toledo). The samples were autoclaved at 120°C for 20 min, and a new reading of electrical conductivity (CE2) was taken, after cooling to 25°C. The EL (%) was calculated as follows:
The values are presented as the mean ± SD of eight replicates.
2.6.2 Lipid peroxidation
To assess cell membrane lipid peroxidation, thiobarbituric acid reactive substances (TBARS) were quantified according to Hodges et al. (1999), with some adaptations. Briefly, the lyophilized samples were frozen in liquid nitrogen and ground in 20% (w/v) trichloroacetic acid with mortar and pestle. The absorbance of the supernatant was measured at 532 and 600 nm. TBARS were calculated using the malondialdehyde (MDA) extinction coefficient of 155 mM cm−1. Lipid peroxidation was expressed in mmol MDA equivalents g−1 DW, as the mean ± SD of eight replicates.
2.7 Statistical analysis
The statistical analysis was conducted using the SPSS V.27 software (SPSS-IBM, Corp., Armonk, New York, USA). After testing for analysis of variance (ANOVA) assumptions, namely, the homogeneity of variances with Levene’s mean test and normality with the Shapiro–Wilk’s test, statistical differences among treatments within each variety and year were evaluated by one-way ANOVA, followed by the post-hoc Tukey’s test. To assess the effects of treatment, year, phenological stage, and their interactions, a multivariate analysis of variance (MANOVA) was performed using Pillai’s trace statistic in SPSS V.27 software. Differences were considered statistically significant at a significance level of p ≤ 0.05.
Correlations between measured gas exchange parameters were assessed using Pearson correlation coefficients.
Data are presented as the mean ± SD of eight replicates, and the results are presented by dry weight, for photosynthetic pigments. For leaf water status, total soluble sugars, starch and protein, total phenolics, and cell membrane damage, data are presented as the mean ± SD of eight replicates.
3 Results and discussion
3.1 Leaf gas exchange parameters
The application of biostimulants affected the two tested cultivars differently regarding leaf gas exchange parameters (Tables 3, 4). For cv. Early Bigi, the general trend in leaf gas exchange parameters showed an increase as the season progressed (Table 3). The effect of spraying biostimulants appears to have some influence, as C leaves always presented the lowest values, even though significant effects of treatments were not recorded on all sampling dates. Indeed, at the first sampling date, significant effects were not recorded for Ci and A/gs, while for other parameters, higher values were observed in trees treated with GB 0.25%, even though these values were similar to other treatments (for A, similar to GB 0.25% and Mix treatments; for E and gs, similar to EM 0.3% and Mix). In the second sampling moment, a different trend was found, with differences between treatments only recorded for Ci, and only when comparing EM 0.3% to C leaves. At fruit harvest, significant changes were only recorded for E and gs. For the former parameter, lower values were recorded for the C treatment, while the transpiration rate increased in all other treatments, namely, in the lower dosages of EM and GB. The effects recorded on stomatal conductance follow a similar pattern, although significant differences were only observed between the C and GB 0.25% treatments.
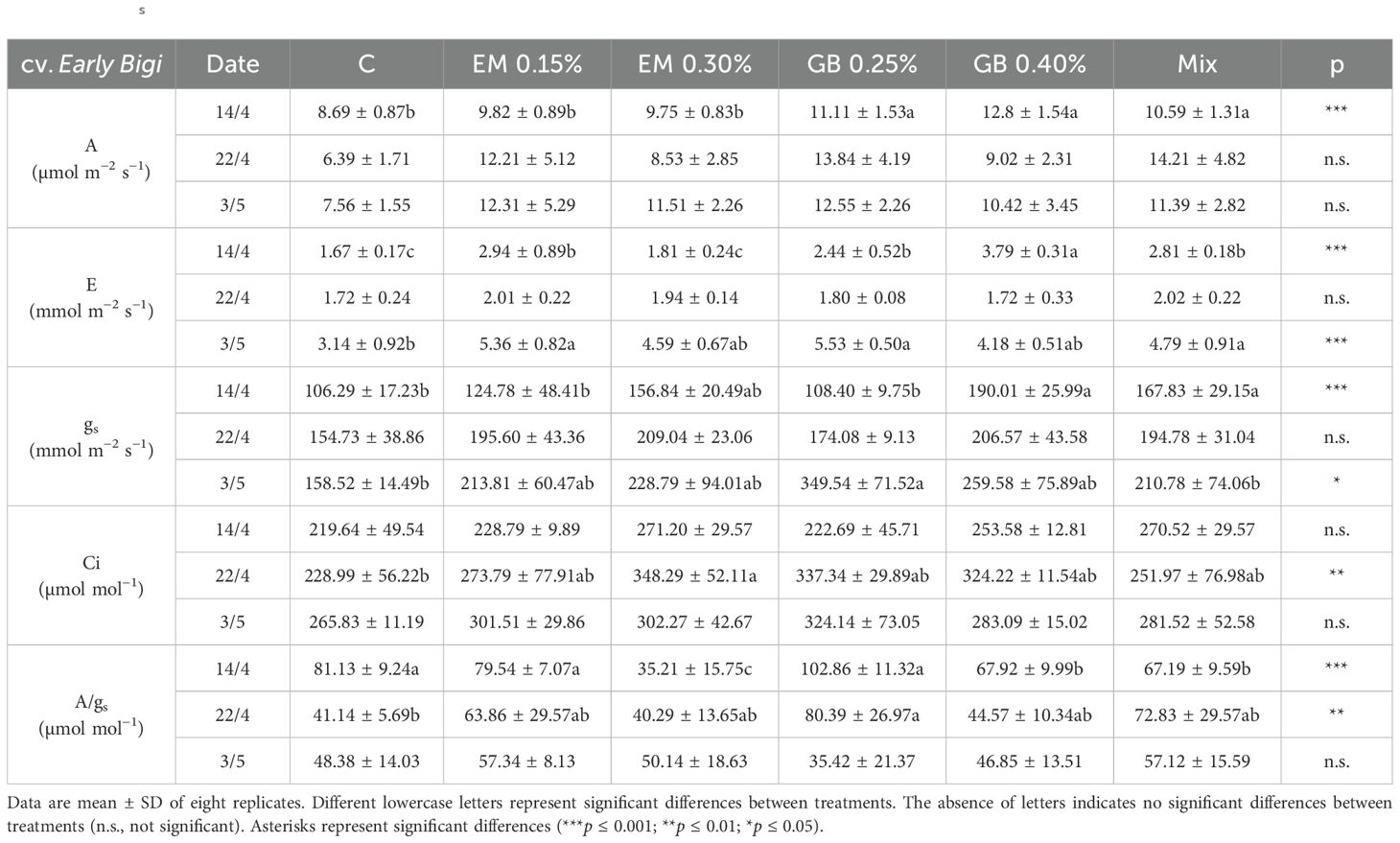
Table 3. Photosynthetic rate (A), transpiration rate (E), stomatal conductance (gs), intercellular CO2 concentration (Ci), and intrinsic water use efficiency (A/gs) recorded in leaves of cv. Early Bigi, recorded in 2019.
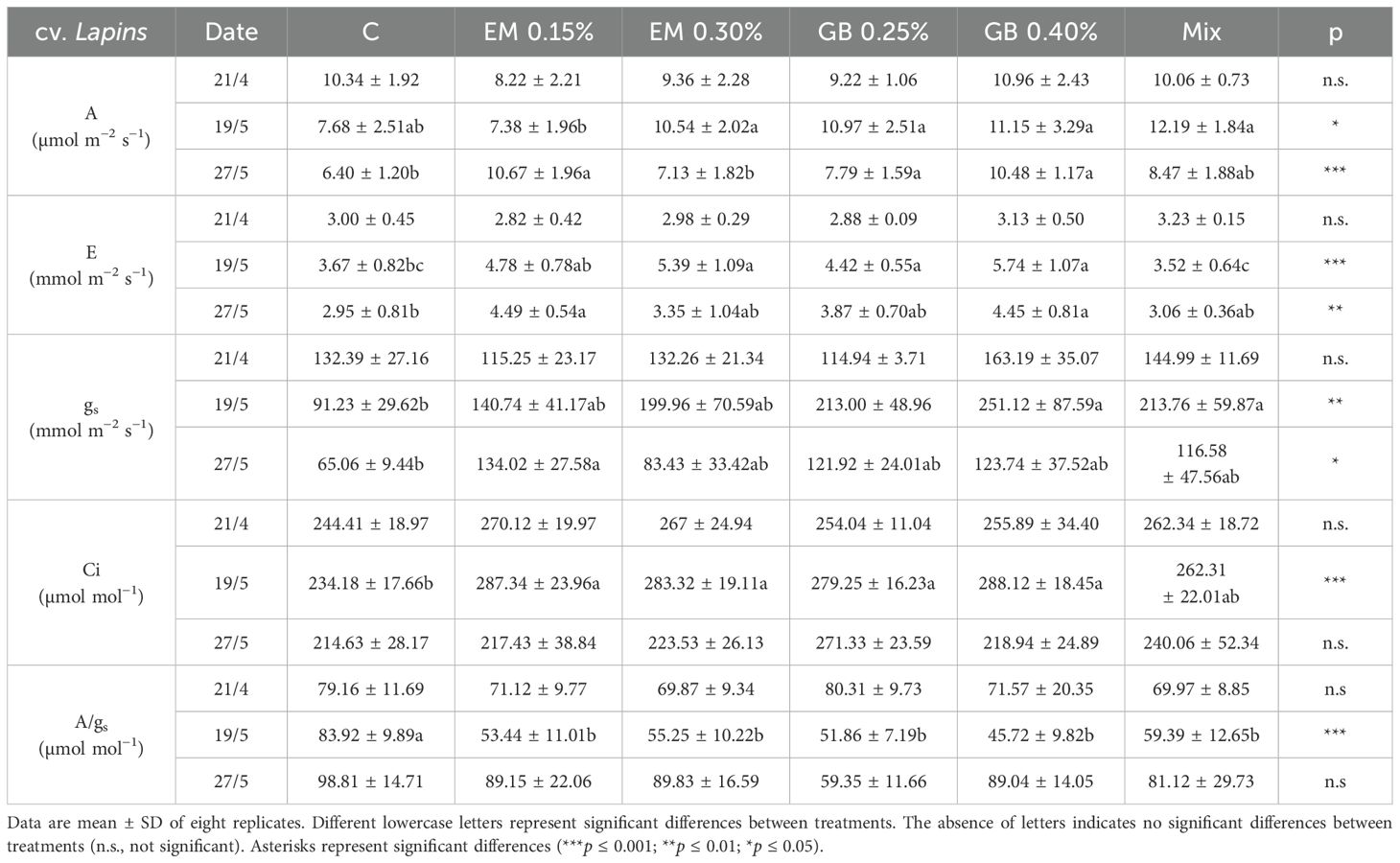
Table 4. Photosynthetic rate (A), transpiration rate (E), stomatal conductance (gs), intercellular CO2 concentration (Ci), and intrinsic water use efficiency (A/gs) recorded in leaves of cv. Lapins, recorded in 2019.
Regarding cv. Lapins, and in contrast to cv. Early Bigi, there is no noticeable major trend in leaf gas exchange as the season progresses. For instance, A is lower at harvest than during the first sampling, whereas E is higher (Table 4). Regarding the application of biostimulants, no significant effects were recorded on the first sampling date, as the data were similar among treatments for all analyzed parameters. On the second sampling date, significant differences were noted for all leaf gas exchange parameters, with lower values recorded for C in almost all of them, except for A/gs. The influence of the applied treatments was also evident at harvest, as only Ci and A/gs values were statistically similar across all samples (Table 4). Indeed, values of A, E, and gs were significantly different among treatments, with higher values recorded for EM 0.15% for A (significantly different from C and EM 0.30%), E (significantly different from C), and gs (also significantly different from C).
Some correlations were found between the measured gas exchange parameters. Indeed, for cv. Early Bigi, the photosynthetic rate (A) presented correlations with Ci (r = −0.595, p ≤ 0.001), gs (r = 0.220, p = 0.043), and E (r = 0.215, p = 0.048). Similarly, for cv. Lapins, correlations were also found, namely, for A and gs (r = 0.697, p ≤ 0.001) and between A and E (r = 0.433, p ≤ 0.001). These relationships have been previously reported in sweet cherries (Gonçalves et al., 2005, 2007; Correia et al., 2020b; Houghton et al., 2023). Furthermore, the positive effects on leaf gas exchange parameters due to the application of biostimulants have been documented in various crops. For example, the application of E. maxima has sowed beneficial effects in melon, cucumber, and tomato (Lefi et al., 2023); Cucurbita pepo L (Rouphael et al., 2017); or Vigna unguiculata (Gyogluu Wardjomto et al., 2023). The use of GB as a biostimulant has improved leaf gas exchange parameters in olive (Denaxa et al., 2020), Lactuca sativa (Lin et al., 2020) or Solanum lycopersicum (Annunziata et al., 2019).
This improvement in leaf gas exchange parameters can be linked to several factors. Enhanced photosynthesis may lead to increased dry matter accumulation (Carvalho et al., 2019; Mateus-Cagua and Rodríguez-Yzquierdo, 2019), by enhancement of mineral nutrient availability and uptake (Caruso et al., 2019), plant hormone regulation, and an increase in metabolites that benefit the electron transport chain (Sorrentino et al., 2022). Furthermore, biostimulants can also potentially improve water-use efficiency in plants (Burghardt and Riederer, 2006; Jiménez-Arias et al., 2022), increasing turgor pressure in leaf guard cells and enhancing gaseous exchange attributes.
3.2 Leaf water status
The leaf water status of both cultivars was affected by different factors (years, phenological stage, treatment, and their interaction), although in different ways (Supplementary Table S1). Regarding cv. Early Bigi, significant influence of the RWC was recorded for all factors, apart from the year and the interaction of treatment, year, and phenological stage, while for cv. Lapins, influence was found for all factors and their interaction. For cv. Early Bigi, the RWC trend was similar in both 2019 and 2021, with an increase in values during fruit development (Figure 2). However, in 2019, significant changes were more noticeable in the last sampling date (at fruit harvest), with differences in treatments C and Mix, both with lower values, while treatment GB 0.25% had higher values. In 2021, significant differences were observed in all sampling moments, with a clear difference recorded for the C treatment (always lower values), while higher values were observed when using the EM 0.15% treatment (even though it does not differ significantly from other treatments, except for the abovementioned C). For cv. Lapins, there is also a similar trend in both years, with an increase of RWC from the first to the second sampling date. However, the values recorded at harvest differed: in 2019, there was a reduction, while in 2021, the water content remained almost the same (Figure 2). In 2019, and at the first sampling date, no differences were observed between treatments, but in the following analyses, C samples recorded the lowest RWC, with leaves from EM 0.15% sprayed trees recording the higher values (even though statistically similar to other treatments). In 2021, C leaves recorded the lowest values even at the first sampling date, with leaves from the EM 0.30% treatment presenting increased water content. Water stress can influence RWC values, which can result in growth limitations and changes in physiological and metabolic processes (Irigoyen et al., 1992), with the benefits of high RWC arising from greater resistance of cell walls and their ability to endure tissue destruction or mechanical damage caused by dehydration. However, in the present study, no water stress situation appears to be occurring, as RWC values are usually above 90%, suggesting sufficient drip irrigation, except for those recorded for the C treatment, in 2021. Even so, the application of both biostimulants on either cultivar results in increased RWC values, with a more pronounced effect for cv. Early Bigi, in 2021, a year that was hotter and dryer than 2019. The use of GB or algae-based biostimulants has demonstrated positive effects on sweet cherry, as reviewed by Afonso et al. (2022), including an increase in RWC (Correia et al., 2020b; Serapicos et al., 2022), corroborating our findings.
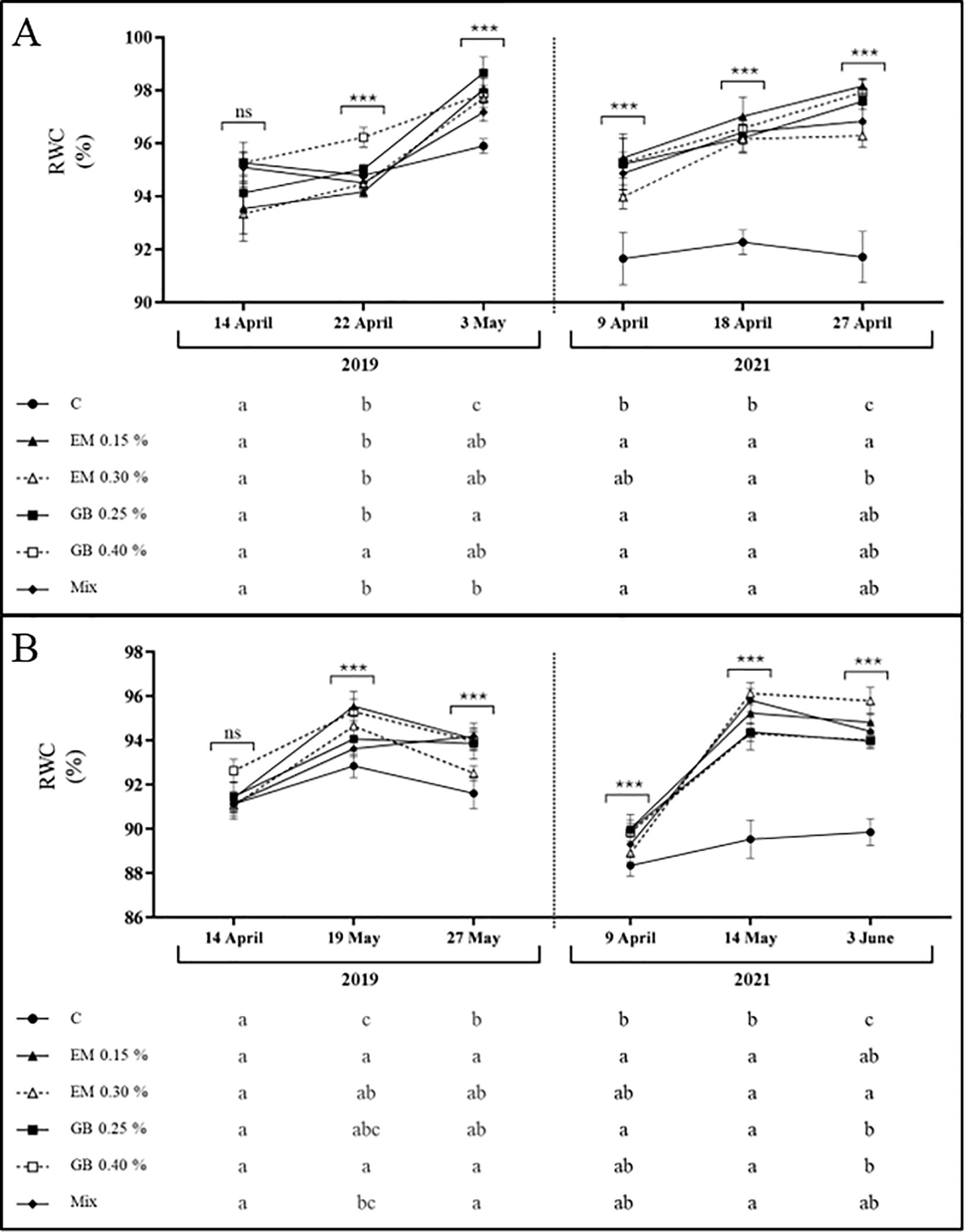
Figure 2. Relative water content (RWC, %) of cv. Early Bigi (A) and cv. Lapins (B) cherry leaves after spray treatment application in 2019 and 2021. Data are presented as mean ± SD of eight replicates. Different lowercase letters indicate significant differences between treatments. The absence of letters indicates no significant differences between treatments (n.s., not significant). Asterisks represent significant differences (***p ≤ 0.001).
Data for leaf mass per unit area (LMA) show, again, different behavior for the two cultivars under study (Figure 3). This variable was affected by all factors, with the exception of the interaction of the treatment, year, and phenological year for both cultivars, and the interaction of treatment and year was only recorded for cv. Lapins (Supplementary Table S1). Values recorded for cv. Early Bigi shows an overall increase of LMA with fruit development, in both years of study. Interestingly, no differences in LMA were recorded at the first sampling date in either year. However, at subsequent stages, C leaves exhibited lower LMA compared to the other treatments, particularly EM 0.15% and GB 0.40%. Regarding cv. Lapins, a similar trend was observed, with an increase in LMA with fruit development. Nonetheless, C leaves consistently had lower values, except for the first sampling date of 2019 and at harvest, in 2021. In contrast, algae-based biostimulants, specifically the EM 0.15% treatment, resulted in increased LMA throughout all sampling dates. High values of LMA have been associated with a higher density of mesophyll or thickness of total lamina, reflecting an increased tolerance to adverse conditions (Centritto, 2002; de la Riva et al., 2016), with improved photosynthetic capacity often related to higher LMA (Reich et al., 2000).
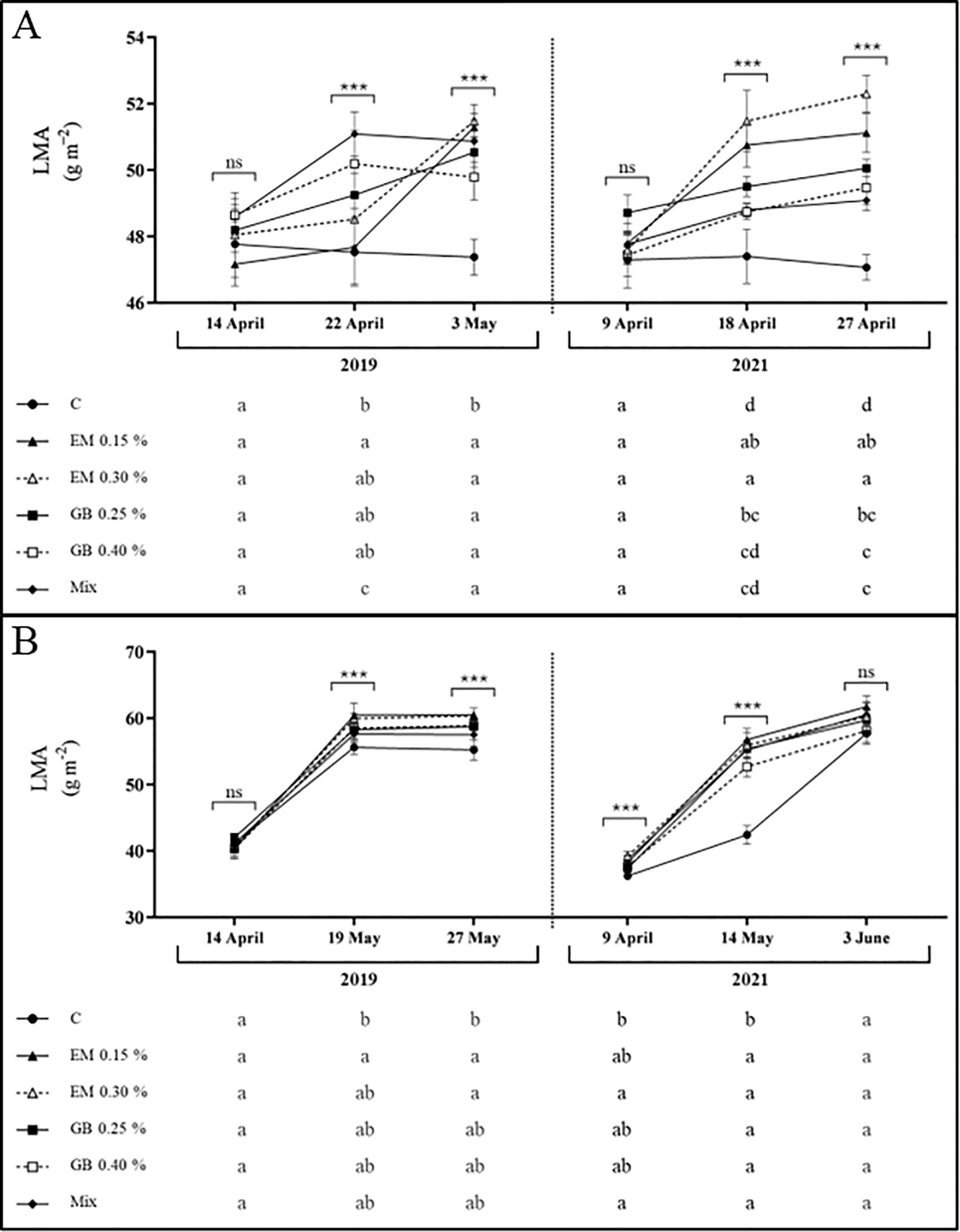
Figure 3. Leaf mass per unit area (LMA, g m−2) of cv. Early Bigi (A) and cv. Lapins (B) cherry leaves after spray treatment application in 2019 and 2021. Data are presented as mean ± SD of eight replicates. Different lowercase letters represent significant differences between treatments. The absence of letters indicates no significant differences between treatments (n.s., not significant). Asterisks represent significant differences (***p ≤ 0.001).
3.3 Metabolite composition determination
3.3.1 Photosynthetic pigments
Leaf photosynthetic pigment content was influenced by the application of either biostimulant (p ≤ 0.001) (Tables 5, 6, and Supplementary Table S1) yet remained within the range recorded for sweet cherry leaves (Gonçalves et al., 2006, 2008). Conversely, the effect of the factors (year, phenological stage, and treatment and their interactions) presented a complex behavior depending on the studied cultivar (Supplementary Table S1). Furthermore, chlorophyll content increased as the season progressed, a pattern previously recorded in sweet cherries (Flore and Layne, 1999; Sytykiewicz et al., 2013).
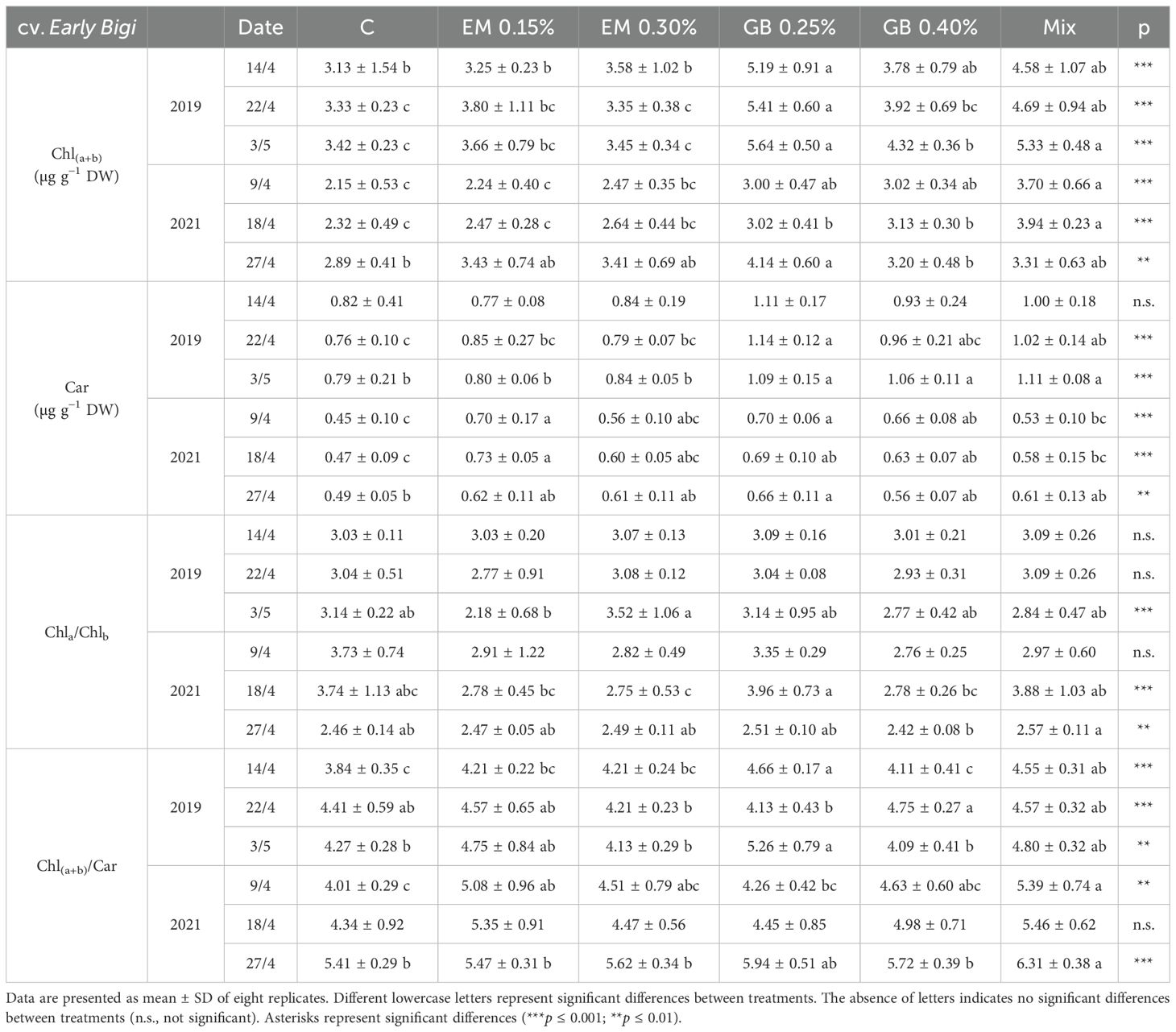
Table 5. Total chlorophyll [Chl(a+b)], carotenoids (Car), and related ratios [Chla/Chlb, Chl(a+b)/Car] of cv. Early Bigi leaves in 2019 and 2021.
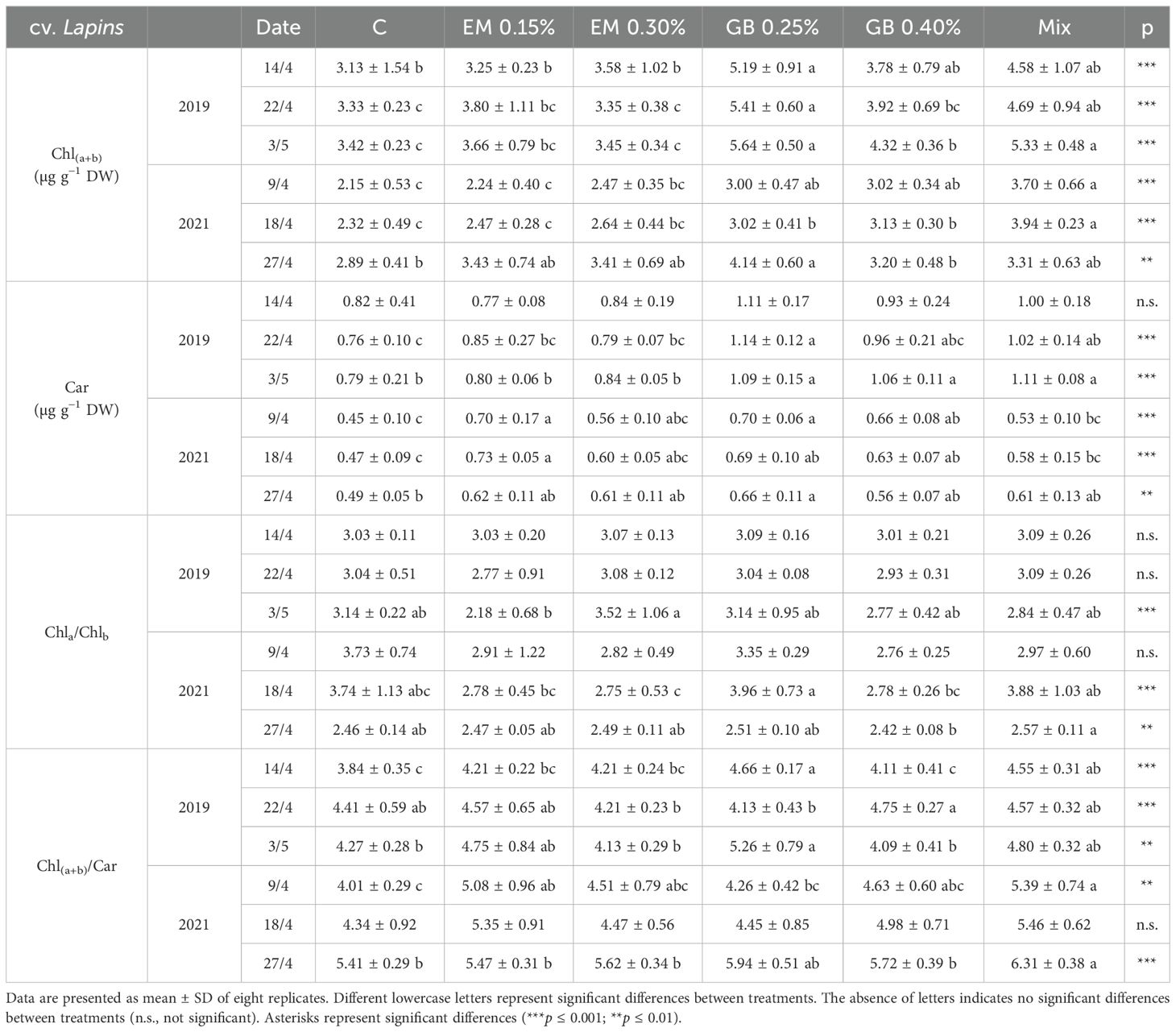
Table 6. Total chlorophyll [Chl(a+b)], carotenoids (Car), and related ratios [Chla/Chlb, Chl(a+b)/Car] of cv. Lapins leaves in 2019 and 2021.
In almost all situations, C leaves from cv. Early Bigi (Table 5) exhibited lower values of total chlorophyll and carotenoids, with an inverse behavior recorded mainly for GB 0.25% and Mix treatments. The Chla/Chlb and Chl(a+b)/Car ratios reflect the variations in the individual components. For cv. Lapins (Table 6), this pattern is also evident, even though other treatments present statistically similar values to those of the C treatment.
This increase in photosynthetic pigments after the application of biostimulants has been previously documented in sweet cherry (Correia et al., 2020b; Serapicos et al., 2022) and in other plants (Yakhin et al., 2017; Afonso et al., 2022). This increase may be attributed to biostimulants reducing the amount of pigment loss due to peroxidation and pigment decomposition by oxygen radicals or by boosting pigment biosynthesis (Navari-Izzo et al., 1990; Hojjati et al., 2023).
Other possible explanations for the observed effects include improved water and ion use efficiency, enhanced stomatal conductance, increased photosynthetic capacity, and the presence of bioactive compounds (Ali et al., 2020; Bahmani Jafarlou et al., 2022; Jafarlou et al., 2022). These factors contribute to inducing sink capability by facilitating the supply and translocation of photoassimilates from leaves to other parts of the plant (Arif et al., 2023).
The Chla/Chlb ratio has been reported to vary from 2 to 4, depending on the plant (Filimon et al., 2016), and serves as an indicator of functional pigment equipment and photosynthetic apparatus light adaptation (Lichtenthaler and Buschmann, 2005). For both studied cultivars, all recorded values were above 2, and higher Chla/Chlb values are often related to a greater capacity to absorb light and consequently higher photosynthetic rates. However, this ratio can be affected by the growth habit of trees, where low light microenvironment can point to an increase in light-harvesting complexes of photosystem II and consequently a decrease of Chla/Chlb (Demmig-Adams, 1998).
The chlorophyll - to-carotenoid ratio (Chltotal/Cartotal) was also influenced by the studied biostimulants, with a more pronounced effect observed for cv. Early Bigi (Table 5). Carotenoids not only are considered accessory pigments but also play an essential role in photoprotection by accepting energy from chlorophyll and dissipating it as heat, thus preventing chloroplast and tissue damage (Hashimoto et al., 2016). Their increase in the use of biostimulants is well-documented (Mones Sardrodi et al., 2022), including in sweet cherries, as recently demonstrated by Correia et al. (2020b). The lower values of Chltotal/Cartotal might indicate that trees were under higher stress, with samples showing higher values probably more able to be protected by carotenoids against photooxidation (Barthod et al., 2007; Loggini et al., 1999).
3.3.2 Total soluble sugars and starch
Soluble sugars and starch content present, for both cultivars and across both years, a very similar trend, with a decrease in their content as fruit development progressed (Figure 4). Moreover, their content was clearly influenced by the application of biostimulants, year, phenological stage, and the interactions between all the factors (apart from the interaction of treatment, year, and phenological stage in cv. Early Bigi and interaction of treatment and phenological stage of cv. Lapins, regarding starch content) (Supplementary Table S1). For soluble sugars (SS) (Figure 4), lower contents were observed in the control (C) treatment, a trend also recorded for starch content. These metabolites are generally associated with responses to different types of stress (Thalmann and Santelia, 2017), with soluble sugars being osmoprotectants (Chaves et al., 2002), while starch is mostly a reservoir for future use, depending on the source–sink dynamics (Brito et al., 2018). Overall, higher values were recorded for EM-treated leaves. These higher values might be linked to higher photosynthetic activity in leaves treated with biostimulants, leading to increased production of sugars and starch (Correia et al., 2020b; Serapicos et al., 2022). Additionally, biostimulant application can upregulate gene expression associated with carbohydrate metabolism (Contartese et al., 2016). Nonetheless, carbohydrate dynamics is very complex, influenced not only by plant growth conditions but also by genotype and even the specific types of sugars involved. Some sugars, such as sucrose and glucose, function as osmolytes or are involved in cellular respiration, while others (fructose) are crucial for secondary metabolite synthesis (Rosa et al., 2009). The key differences observed between C and treated samples are a lower amount of reduction (starch in 2019, in cv. Lapins, or sugars, in 2019, for both cultivars) or an increased initial content. However, the overall decreases in sugars and starch content in leaves during fruit growth have been previously recorded (Quentin et al., 2013), influenced by the presence of fruit, which indicates that sweet cherry fruits are strong sinks (Falchi et al., 2020).
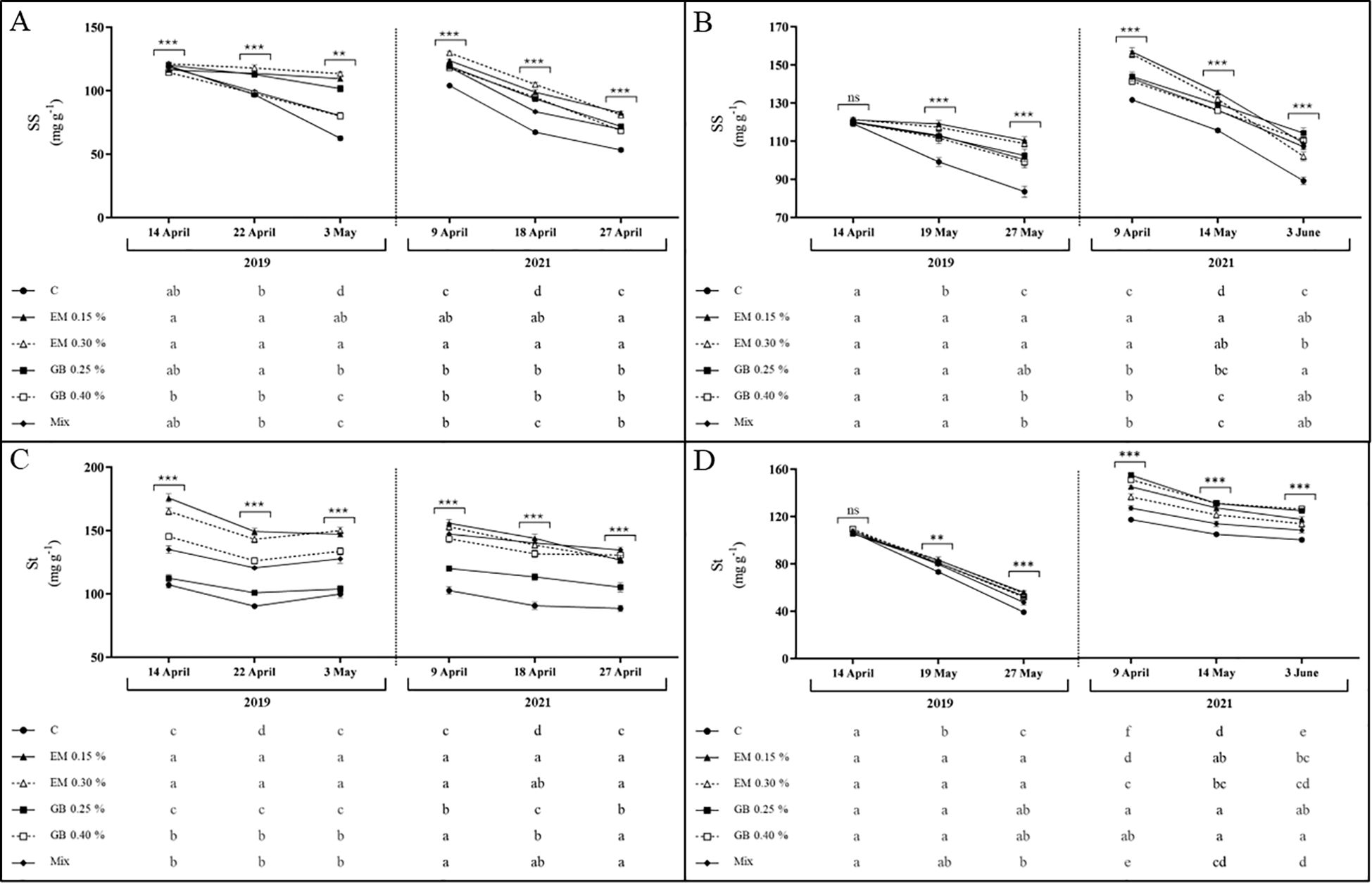
Figure 4. Soluble sugars (SS, mg g−1 DW) and starch contents (St, mg g−1 DW) of cvs. Early Bigi (A, C) and Lapins (B, D) leaf cherry after spray treatment application in 2019 and 2021. Data are mean ± SD of eight replicates. Different lowercase letters represent significant differences. The absence of letters indicates no significant differences between treatments (n.s., not significant). Asterisks represent significant differences (***p ≤ 0.001; **p ≤ 0.01).
3.3.3 Total soluble protein
Soluble protein content (SP) follows a similar pattern to that of carbohydrates, with lower values in C treatment across both years and cultivars (Figure 5). In leaves of both cultivars, the concentration of total soluble protein was affected by treatment (p ≤ 0.001), year (p ≤ 0.001), and phenological stage (p ≤ 0.001). Furthermore, the interaction between treatment and year (p ≤ 0.001) for cv. Early Bigi and the interaction of treatment and phenological stage (p ≤ 0.001) and the interaction year and phenological stage (p ≤ 0.001) for cv. Lapins also influenced the total soluble protein content (Supplementary Table S1).
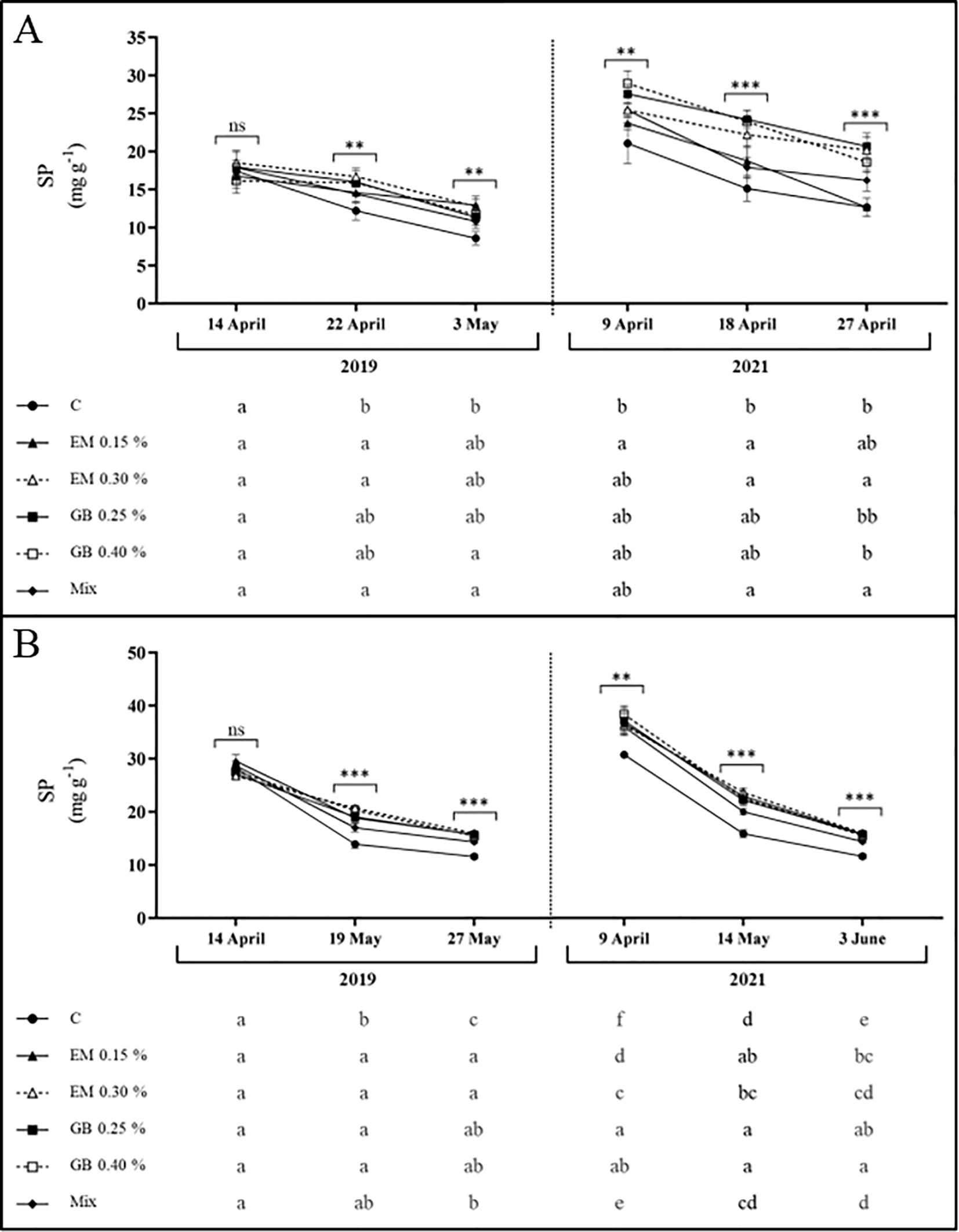
Figure 5. Total soluble protein content (SP, mg g−1 DW) of cvs. Early Bigi (A) and Lapins (B) leaf cherry after spray treatment application in 2019 and 2021. Data presented are mean ± SD of eight replicates. Different lowercase letters represent significant differences. The absence of letters indicates no significant differences between treatments (n.s., not significant). Asterisks represent significant differences (***p ≤ 0.001; **p ≤ 0.01).
The accumulation of proteins after the foliar application of biostimulants has been recorded elsewhere (Yakhin et al., 2017; Afonso et al., 2022). This increase may be associated with the rich bioactive endogenous profile of seaweed, which includes hormones, minerals, and vitamins that promote enzymatic actions responsible for protein synthesis (Aslam et al., 2016), and is linked to the increased expression of genes (Goñi et al., 2018; Baltazar et al., 2021) or the N input present in the biostimulants (Afonso et al., 2022). Additionally, the amino acid glycine, a reduced form of nitrogen, can be directly assimilated by leaves, thereby accelerating protein biosynthesis (Zargar Shooshtari et al., 2020). On the other hand, the reduction of protein content with fruit development may possibly be related to the onset of mobilization of foliar N towards other plant parts (Thimann, 1980).
3.3.4 Total phenolics
Data for total phenolic content show an increase in all situations as fruit development progresses (Figure 6). This pattern is commonly observed in plants (Colaric et al., 2006), including sweet cherries (Serapicos et al., 2022), and the average value is within the range found in sweet cherry leaves (Gonçalves et al., 2008; Dziadek et al., 2019; Nunes et al., 2021). In both cultivars, the effect of biostimulants was evident (p ≤ 0.001), as well as the effect of the remaining factors. However, only observed in cv. Early Bigi, the interactions between treatment and year (p > 0.05) and between treatment, year, and phenological stage (p > 0.05) did not significantly affect the total phenolic content (Supplementary Table S1). Overall, the greatest results were recorded with algae-based biostimulants, regarding cv. Early Bigi, although differences can be observed with all treatments. For cv. Lapins, algae-based biostimulants also led to increased phenolic content, but with reduced variations when compared to other treatments. The increased content of phenolic compounds in spray samples might be linked to the ability of biostimulants to enhance the activity of key enzymes, like phenylalanine ammonia lyase and tyrosine aminotransferase, that are involved in phenolics biosynthesis (Kulkarni et al., 2019; Afonso et al., 2022). On the other hand, it may be attributed to the improved activity of endogenous antioxidant enzymes, hence protecting existing phenolics form oxidation (Pylak et al., 2019; Afonso et al., 2022).
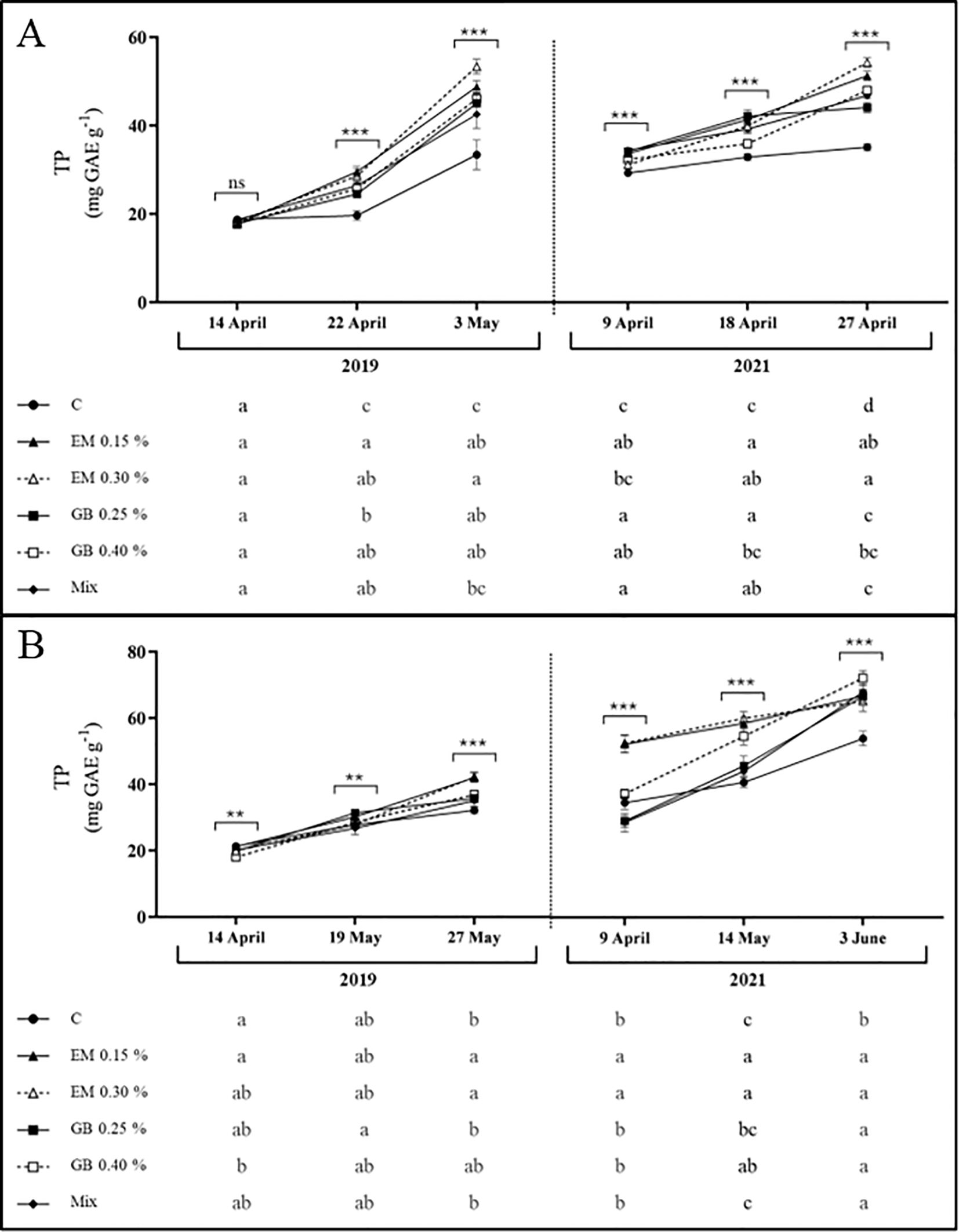
Figure 6. Total phenolics content (TP, mg GAE g−1 DW) of cvs. Early Bigi (A) and Lapins (B) leaf cherry after spray treatment application in 2019 and 2021. Data presented are mean ± SD of eight replicates. Different lowercase letters represent significant differences. The absence of letters indicates no significant differences between treatments (n.s., not significant). Asterisks represent significant differences (***p ≤ 0.001; **p ≤ 0.01).
3.4 Cell membrane damage
Biotic or abiotic stresses can cause the accumulation of reactive oxygen species, causing damage to cell membranes through lipid peroxidation, thereby changing their permeability (Sachdev et al., 2021). These changes in permeability can be monitored by measuring the EL, an indicator of cell membrane integrity (Bajji et al., 2001), or by detecting the presence of TBARS.
In the current study, both indexes of membrane damage exhibit a very similar tendency (Figure 7). In leaves of both cultivars, these indexes were affected by year (p ≤ 0.001), phenological stage (p ≤ 0.001), treatments (p ≤ 0.001), and the interaction of treatment and phenological stage (p ≤ 0.001). The EL was also influenced by the interaction between year and treatment (p ≤ 0.001) and the interaction between treatment, year, and phenological stage (p ≤ 0.01) only for cv. Early Bigi. The interaction between year and phenological stage affected the EL (p ≤ 0.001 for both cultivars) and TBARS (p ≤ 0.001 and p ≤ 0.01, for cv. Early Bigi and Lapins, respectively) (Supplementary Table S1).

Figure 7. Electrolyte leakage (EL, %) and thiobarbituric acid reactive substances concentration (TBARS, nmol MDAE g−1 DW) of cvs. Early Bigi (A, C) and Lapins (B, D) leaf cherry after spray treatment application in 2019 and 2021. Data are mean ± SD of eight replicates. Different lowercase letters represent significant differences. The absence of letters indicates no significant differences between treatments (n.s., not significant). Asterisks represent significant differences (***p ≤ 0.001; **p ≤ 0.01; *p ≤ 0.05).
Results for cv. Early Bigi (Figure 7) in both years show a similar trend, with an overall decrease in EL with the advance of fruit development, observed in both 2019 and 2021. The application of biostimulants appears to affect this specific parameter, as significantly higher values were recorded for C leaves in all sampling dates of both years (the only exception on the first sampling of 2019). This same pattern was recorded in leaves of cv. Lapins, with a decrease of EL with fruit development, and higher values were recorded for C treatment (with exceptions noted in the first sampling of both years).
The lower EL observed with the application of biostimulants might point out a positive effect with the use of these compounds in preserving cell membranes during dehydration (Savé et al., 1999), even in the absence of severe stress, as in the current work.
The evaluation of TBARS allows an overview regarding peroxidation of membrane lipids mediated by reactive oxygen species, which can lead to cellular damage (Sofo et al., 2004; Beis and Patakas, 2012), and typically, higher TBARS values indicate a higher exposure to stress. The results of the present work underscore the significant effect of the use of biostimulants in this specific parameter (Figure 7). The overall trend of TBARS is a decrease of their content with fruit development, in all situations. However, the use of biostimulants presented two different effects: firstly, in all samples, the decrease in TBARS content was significantly higher than that recorded in the C samples; in addition, in the 2021 dataset, the initial TBARS content was already considerably lower in leaves from sprayed trees. This pronounced effect of biostimulants on TBARS data has previously been recorded in sweet cherry leaves (Correia et al., 2020b).
3.5 Conclusion
This study evaluated the impact of biostimulants on leaf water status, photosynthetic pigments, soluble sugars, starch, soluble proteins, and leaf gas exchange parameters in two cultivars of sweet cherries.
Results revealed significant differences between cultivars and years, with notable effects observed in 2021, a hotter and dryer year compared to 2019. Despite variations, the application of biostimulants generally led to an improved leaf water status, enhanced photosynthetic pigment content, and increased photosynthetic activity (Jolayemi et al., 2023). Furthermore, the application of biostimulants also contributed to the preservation of cell membrane integrity.
These improvements were most evident after the application of both concentrations of the seaweed-based biostimulant E. maxima and treatment GB 0.40%, which positively influenced the performance of cherry trees.
These findings indicate the potential of biostimulants to mitigate the impact of environmental stressors and enhance physiological processes in sweet cherry cultivation, contributing to improved crop performance. Further research is warranted to elucidate the underlying mechanisms and optimize biostimulant applications for sustainable cherry production.
Data availability statement
The raw data supporting the conclusions of this article will be made available by the authors, without undue reservation.
Author contributions
SA: Conceptualization, Data curation, Formal Analysis, Funding acquisition, Investigation, Methodology, Writing – original draft, Writing – review & editing. IO: Conceptualization, Investigation, Validation, Writing – review & editing. FG: Methodology, Resources, Writing – review & editing. AM: Conceptualization, Funding acquisition, Supervision, Validation, Writing – review & editing. BG: Conceptualization, Funding acquisition, Supervision, Validation, Writing – review & editing.
Funding
The author(s) declare that financial support was received for the research, authorship, and/or publication of this article. SA is grateful to FCT, MCTES, and FSE for the PhD Fellowship SFRH/BD/139922/2018. This research was funded by “Fundo Europeu Agrícola de Desenvolvimento Rural (FEADER)” and by “Estado Português” in the context of “Ação 1.1 «Grupos Operacionais»“, integrated in “Medida 1. «Inovação» do PDR 2020—Programa de Desenvolvimento Rural do Continente—Grupo Operacional para a valorização da produção da Cereja de Resende e posicionamento da subfileira nos mercados (iniciativa no. 362)”. (https://doi.org/10.54499/UIDB/04033/2020 (accessed on 11 July 2024)) and LA/P/0126/2020, Inov4Agro (https://doi.org/10.54499/LA/P/0126/2020 (accessed on 11 July 2024)).
Conflict of interest
The authors declare that the research was conducted in the absence of any commercial or financial relationships that could be construed as a potential conflict of interest.
The author(s) declared that they were an editorial board member of Frontiers, at the time of submission. This had no impact on the peer review process and the final decision.
Publisher’s note
All claims expressed in this article are solely those of the authors and do not necessarily represent those of their affiliated organizations, or those of the publisher, the editors and the reviewers. Any product that may be evaluated in this article, or claim that may be made by its manufacturer, is not guaranteed or endorsed by the publisher.
Supplementary material
The Supplementary Material for this article can be found online at: https://www.frontiersin.org/articles/10.3389/fpls.2024.1467376/full#supplementary-material
References
Afonso, S., Oliveira, I., Meyer, A. S., Gonçalves, B. (2022). Biostimulants to improve tree physiology and fruit quality: a review with special focus on sweet cherry. Agronomy 12, 659. doi: 10.3390/agronomy12030659
Ali, Q., Perveen, R., El-Esawi, M. A., Ali, S., Hussain, S. M., Amber, M., et al. (2020). Low doses of Cuscuta reflexa extract act as natural biostimulants to improve the germination vigor, growth, and grain yield of wheat grown under water stress: Photosynthetic pigments, antioxidative defense mechanisms, and nutrient acquisition. Biomolecules 10, 1212. doi: 10.3390/biom10091212
Ali, O., Ramsubhag, A., Jayaraman, J. (2021). Biostimulant properties of seaweed extracts in plants: Implications towards sustainable crop production. Plants 10, 531. doi: 10.3390/plants10030531
Annunziata, M. G., Ciarmiello, L. F., Woodrow, P., Dell’Aversana, E., Carillo, P. (2019). Spatial and temporal profile of glycine betaine accumulation in plants under abiotic stresses. Front. Plant Sci. 10, 230. doi: 10.3389/fpls.2019.00230
Arif, Y., Bajguz, A., Hayat, S. (2023). Moringa oleifera extract as a natural plant biostimulant. J. Plant Growth Regul. 42, 1291–1306. doi: 10.1007/s00344-022-10630-4
Arnon, D. I. (1949). Copper enzymes in isolated chloroplasts. Polyphenoloxidase in Beta vulgaris. Plant Physiol. 24, 1–15. doi: 10.1104/pp.24.1.1
Asif, A., Ali, M., Qadir, M., Karthikeyan, R., Singh, Z., Khangura, R., et al. (2023). Enhancing crop resilience by harnessing the synergistic effects of biostimulants against abiotic stress. Front. Plant Sci. 14, 1276117. doi: 10.3389/fpls.2023.1276117
Aslam, M., Sultana, B., Anwar, F., Munir, H. (2016). Foliar spray of selected plant growth regulators affected the biochemical and antioxidant attributes of spinach in a field experiment. Turkish J. Agric. Forestry 40, 136–145. doi: 10.3906/tar-1412-56
Bahmani Jafarlou, M., Pilehvar, B., Modaresi, M., Mohammadi, M. (2022). Seaweed liquid extract as an alternative biostimulant for the amelioration of salt-stress effects in Calotropis procera (Aiton) WT. J. Plant Growth Regul. 42, 449–464. doi: 10.1007/s00344-021-10566-1
Bajji, M., Kinet, J. M., Lutts, S. (2001). The use of the electrolyte leakage method for assessing cell membrane stability as a water stress tolerance test in durum wheat. Plant Growth Regul. 36, 61–70.
Baltazar, M., Correia, S., Guinan, K. J., Sujeeth, N., Bragança, R., Gonçalves, B. (2021). Recent advances in the molecular effects of biostimulants in plants: An overview. Biomolecules 11 (8), 1096.
Bantis, F., Koukounaras, A. (2022). Ascophyllum nodosum and Silicon-Based Biostimulants differentially affect the physiology and growth of watermelon transplants under abiotic stress factors: The case of drought. Horticulturae 8, 1177. doi: 10.3390/horticulturae8121177
Barthod, S., Cerovic, Z., Epron, D. (2007). Can dual chlorophyll fluorescence excitation be used to assess the variation in the content of UV-absorbing phenolic compounds in leaves of temperate tree species along a light gradient? J. Exp. Bot. 58 (7), 1753–1760. doi: 10.1093/jxb/erm030
Basile, B., Brown, N., Valdes, J. M., Cardarelli, M., Scognamiglio, P., Mataffo, A., et al. (2021). Plant-based biostimulant as sustainable alternative to synthetic growth regulators in two sweet cherry cultivars. Plants 10, 619. doi: 10.3390/plants10040619
Basile, B., Rouphael, Y., Colla, G., Soppelsa, S., Andreotti, C. (2020). Appraisal of emerging crop management opportunities in fruit trees, grapevines and berry crops facilitated by the application of biostimulants. Scientia Hortic. 267, 109330. doi: 10.1016/j.scienta.2020.109330
Beis, A., Patakas, A. (2012). Relative contribution of photoprotection and anti-oxidative mechanisms to differential drought adaptation ability in grapevines. Environ. Exp. Bot. 78, 173–183. doi: 10.1016/j.envexpbot.2011.12.038
Bradford, M. M. (1976). A rapid and sensitive method for the quantitation of microgram quantities of protein utilizing the principle of protein-dye binding. Analytical Biochem. 72 (1-2), 248–254. doi: 10.1016/0003-2697(76)90527-3
Brito, C., Dinis, L. T., Ferreira, H., Rocha, L., Pavia, I., Moutinho-Pereira, J., et al. (2018). Kaolin particle film modulates morphological, physiological and biochemical olive tree responses to drought and rewatering. Plant Physiol. Biochem. 133, 29–39. doi: 10.1016/j.plaphy.2018.10.028
Burghardt, M., Riederer, M. (2006). “Cuticular transpiration,” in Biology of the plant cuticle. Eds. Riederer, M., Müller, C. (Blackwell, Oxford), 292–311.
Caruso, G., De Pascale, S., Cozzolino, E., Giordano, M., El-Nakhel, C., Cuciniello, A., et al. (2019). Protein hydrolysate or plant extract-based biostimulants enhanced yield and quality performances of greenhouse perennial wall rocket grown in different seasons. Plants 8, 208. doi: 10.3390/plants8070208
Carvalho, M., Castro, I., Moutinho-Pereira, J., Correia, C., Egea-Cortines, M., Matos, M., et al. (2019). Evaluating stress responses in cowpea under drought stress. J. Plant Physiol. 241, 153001. doi: 10.1016/j.jplph.2019.153001
Centritto, M. (2002). The effects of elevated [CO2] and water availability on growth and physiology of peach (Prunus persica) plants. Plant Biosyst. 136, 177–188. doi: 10.1080/11263500212331351079
Chaves, M. M., Pereira, J. S., Maroco, J., Rodrigues, M. L., Ricardo, C. P. P., Osório, M. L., et al. (2002). How plants cope with water stress in the field? Photosynthesis and growth. Ann. Bot. 89, 907–916. doi: 10.1093/aob/mcf105
Colaric, M., Stampar, F., Hudina, M. (2006). Changes in sugars and phenolics concentrations of Williams pear leaves during the growing season. Can. J. Plant Sci. 86, 1203–1208. doi: 10.4141/P05-195
Contartese, V., Garabello, C., Occhipinti, A., Barbero, F., Bertea, C. M. (2016). Effects of a new biostimulant on gene expression and metabolic responses of tomato plants. Acta Hortic. 1148, 35–42. doi: 10.17660/ActaHortic.2016.1148.4
Correia, S., Aires, A., Queirós, F., Carvalho, R., Schouten, R., Silva, A. P., et al. (2020a). Climate conditions and spray treatments induce shifts in health promoting compounds in cherry (Prunus avium L.) fruits. Scientia Hortic. 263, 109147. doi: 10.1016/j.scienta.2019.109147
Correia, S., Queirós, F., Ferreira, H., Morais, M. C., Afonso, S., Silva, A. P., et al. (2020b). Foliar application of calcium and growth regulators modulate sweet cherry (Prunus avium L) tree performance. Plants 9, 410. doi: 10.3390/plants9040410
de la Riva, E. G., Olmo, M., Poorter, H., Ubera, J. L., Villar, R. (2016). Leaf mass per area (LMA) and its relationship with leaf structure and anatomy in 34 Mediterranean woody species along a water availability gradient. PloS One 11, e0148788. doi: 10.1371/journal.pone.0148788
Demmig-Adams, B. (1998). Survey of thermal energy dissipation and pigment composition in sun and shade leaves. Plant Cell Physiol. 39, 474–482. doi: 10.1093/oxfordjournals.pcp.a029394
Denaxa, N. K., Damvakaris, T., Roussos, P. A. (2020). Antioxidant defense system in young olive plants against drought stress and mitigation of adverse effects through external application of alleviating products. Scientia Hortic. 259, 108812. doi: 10.1016/j.scienta.2019.108812
Dewanto, V., Wu, X., Adom, K. K., Liu, R. H. (2002). Thermal processing enhances the nutritional value of tomatoes by increasing total antioxidant activity. J. Agricul. Food Chem. 50 (10), 3010–3014. doi: 10.1021/jf0115589
du Jardin, P. (2015). Plant biostimulants: Definition, concept, main categories and regulation. Scientia Hortic. 196, 3–14. doi: 10.1016/j.scienta.2015.09.021
Dutta, T., Neelapu, N. R. R., Wani, S. H., Surekha, C. (2019). Role and regulation of osmolytes as signaling molecules to abiotic stress tolerance. Plant Signaling Molecules, 459–477. doi: 10.1016/B978-0-12-816451-8.00029-0
Dziadek, K., Kopeć, A., Tabaszewska, M. (2019). Potential of sweet cherry (Prunus avium L.) by-products: bioactive compounds and antioxidant activity of leaves and petioles. Eur. Food Res. Technol. 245, 763–772. doi: 10.1007/s00217-018-3198-x
Fadón, E., Herrero, M., Rodrigo, J. (2015). Flower development in sweet cherry framed in the BBCH scale. Scientia Hortic. 192, 141–147. doi: 10.1016/j.scienta.2015.05.027
Falchi, R., Bonghi, C., Drincovich, M. F., Famiani, F., Lara, M. V., Walker, R. P., et al. (2020). Sugar metabolism in stone fruit: Source-sink relationships and environmental and agronomical effects. Front. Plant Sci. 11, 573982. doi: 10.3389/fpls.2020.573982
Fedotova, M. V. (2019). Compatible osmolytes-bioprotectants: is there a common link between their hydration and their protective action under abiotic stresses? J. Mol. Liquids 292, 111339. doi: 10.1016/j.molliq.2019.111339
Filimon, R. V., Rotaru, L., Filimon, R. M. (2016). Quantitative investigation of leaf photosynthetic pigments during annual biological cycle of Vitis vinifera L. table grape cultivars. South Afr. J. Enology Viticulture 37, 1–14. doi: 10.21548/37-1-753
Flore, J. A., Layne, D. R. (1999). Photoassimilate production and distribution in cherry. HortScience 34, 1015–1019. doi: 10.21273/HORTSCI.34.6.1015
Gonçalves, B., Correia, C. M., Silva, A. P., Bacelar, E. A., Santos, A., Moutinho-Pereira, J. M. (2008). Leaf structure and function of sweet cherry tree (Prunus avium L.) cultivars with open and dense canopies. Scientia Hortic. 116, 381–387. doi: 10.1016/j.scienta.2008.02.013
Gonçalves, B., Correia, C. M., Silva, A. P., Bacelar, E. A., Santos, A., Ferreira, H., et al. (2007). Variation in xylem structure and function in roots and stems of scion–rootstock combinations of sweet cherry tree (Prunus avium L.). Trees 21, 121–130. doi: 10.1007/s00468-006-0102-2
Gonçalves, B., Morais, M. C., Sequeira, A., Ribeiro, C., Guedes, F., Silva, A. P., et al. (2020). Quality preservation of sweet cherry cv.’staccato’by using glycine-betaine or Ascophyllum nodosum. Food Chem. 322, 126713. doi: 10.1016/j.foodchem.2020.126713
Gonçalves, B., Moutinho-Pereira, J., Santos, A., Silva, A. P., Bacelar, E., Correia, C., et al. (2006). Scion–rootstock interaction affects the physiology and fruit quality of sweet cherry. Tree Physiol. 26 (1), 93–104. doi: 10.1093/treephys/26.1.93
Goncalves, B., Moutinho-Pereira, J., Bacelar, E., Correia, C., Silva, A. P., Santos, A. (2005). Relationships among sweet cherry leaf gas exchange, morphology and chemical composition. In V International Cherry Symposium 795, 633–638. doi: 10.17660/ActaHortic.2008.795.98
Goñi, O., Quille, P., O’Connell, S. (2018). Ascophyllum nodosum extract biostimulants and their role in enhancing tolerance to drought stress in tomato plants. Plant Physiol. Biochem. 126, 63–73. doi: 10.1016/j.plaphy.2018.02.024
Gyogluu Wardjomto, C., Mohammed, M., Ngmenzuma, T. Y., Mohale, K. C. (2023). Effect of rhizobia inoculation and seaweed extract (Ecklonia maxima) application on the growth, symbiotic performance and nutritional content of cowpea (Vigna unguiculata (L.) Walp.). Front. Agron. 5, 1138263. doi: 10.3389/fagro.2023.1138263
Hashimoto, H., Uragami, C., Cogdell, R. J. (2016). Carotenoids and photosynthesis. Subcell Biochem. 79, 111–139. doi: 10.1007/978-3-319-39126-7_4
Hodges, D. M., DeLong, J. M., Forney, C. F., Prange, R. K. (1999). Improving the thiobarbituric acid-reactive-substances assay for estimating lipid peroxidation in plant tissues containing anthocyanin and other interfering compounds. Planta 207, 604–611. doi: 10.1007/s004250050524
Hojjati, M., Jahromi, M. G., Abdossi, V., Torkashvand, A. M. (2023). Exogenous melatonin modulated drought stress by regulating physio-biochemical attributes and fatty acid profile of sweet cherry (Prunus avium L.). J. Plant Growth Regul. 43 (1), 299–313. doi: 10.1007/s00344-023-11085-x
Houghton, E., Bevandick, K., Neilsen, D., Hannam, K., Nelson, L. M. (2023). Effects of postharvest deficit irrigation on sweet cherry (Prunus avium) in five Okanagan Valley, Canada, orchards: I. Tree water status, photosynthesis, and growth. Can. J. Plant Sci. 103, 73–92. doi: 10.1139/cjps-2022-0201
Iacono, F., Buccella, A., Peterlunger, E. (1998). Water stress and rootstock influence on leaf gas exchange of grafted and ungrafted grapevines. Scientia Hortic. 75, 27–39. doi: 10.1016/S0304-4238(98)00113-7
IPCC, Core Writing Team (2023). Climate Change 2023: Synthesis Report. A Report of the Intergovernmental Panel on Climate Change. Contribution of Working Groups I, II and III to the Sixth Assessment Report of the Intergovernmental Panel on Climate Change. Eds. Lee, H., Romero, J. (Geneva, Switzerland: IPCC).
Irigoyen, J. J., Einerich, D. W., Sánchez-Díaz, M. (1992). Water stress induced changes in concentrations of proline and total soluble sugars in nodulated alfalfa (Medicago sativa) plants. Physiol. Plant 84, 55–60. doi: 10.1111/j.1399-3054.1992.tb08764.x
Jafarlou, M. B., Pilehvar, B., Modaresi, M., Mohammadi, M. (2022). Interactive effects of seaweed and microalga extract priming as a biostimulant agent on the seed germination indices and primary growth of milkweed (Calotropis procera ait.). Biologia, 1–11. doi: 10.1007/s11756-021-00996-3
Jiménez-Arias, D., Hernándiz, A. E., Morales-Sierra, S., García-García, A. L., García-MaChado, F. J., Luis, J. C., et al. (2022). Applying biostimulants to combat water deficit in crop plants: research and debate. Agronomy 12, 571. doi: 10.3390/agronomy12030571
Jolayemi, O. L., Malik, A. H., Vetukuri, R. R., Saripella, G. V., Kalyandurg, P. B., Ekblad, T., et al. (2023). Metabolic processes and biological macromolecules defined the positive effects of protein-rich biostimulants on sugar beet plant development. Int. J. Mol. Sci. 24, 9720. doi: 10.3390/ijms24119720
Khalid, M., Rehman, H. M., Ahmed, N., Nawaz, S., Saleem, F., Ahmad, S., et al. (2022). Using exogenous melatonin, glutathione, proline, and glycine betaine treatments to combat abiotic stresses in crops. Int. J. Mol. Sci. 23, 12913. doi: 10.3390/ijms232112913
Khalid, A., Zafar, Z. U., Akram, A., Hussain, K., Manzoor, H., Al-Qurainy, F., et al. (2015). Photosynthetic capacity of canola (Brassica napus L.) plants as affected byglycine betaine under the salt stress. J. Appl. Bot. Food Qual. 88, 78–86. doi: 10.5073/JABFQ.2015.088.011
Kulkarni, M. G., Rengasamy, K. R., Pendota, S. C., Gruz, J., Plačková, L., Novák, O., et al. (2019). Bioactive molecules derived from smoke and seaweed Ecklonia maxima showing phytohormone-like activity in Spinacia oleracea L. New Biotechnol. 48, 83–89. doi: 10.1016/j.nbt.2018.08.004
Lefi, E., Badri, M., Hamed, S. B., Talbi, S., Mnafgui, W., Ludidi, N., et al. (2023). Influence of brown seaweed (Ecklonia maxima) extract on the morpho-physiological parameters of melon, cucumber, and tomato plants. Agronomy 13, 2745. doi: 10.3390/agronomy13112745
Lichtenthaler, H. K. (1987). Chlorophylls and carotenoids: Pigments of photosynthetic biomembranes. Method Enzymol. 148, 350–382. doi: 10.1016/0076-6879(87)48036-1
Lichtenthaler, H. K., Buschmann, C. (2005). Chlorophylls and carotenoids: Measurement and characterization by UV-VIS spectroscopy. Handb. Food Analytical Chem. 2–2, 171–178. doi: 10.1002/0471142913.faf0403s01
Lin, F. W., Lin, K. H., Wu, C. W., Chang, Y. S. (2020). Effects of betaine and chitin on water use efficiency in lettuce (Lactuca sativa var. capitata). HortScience 55, 89–95. doi: 10.21273/HORTSCI14518-19
Loggini, B., Scartazza, A., Brugnoli, E., Navari-Izzo, F. (1999). Antioxidative defense system, pigment composition, and photosynthetic efficiency in two wheat cultivars subjected to drought. Plant Physiol. 119 (3), 1091–1100. doi: 10.1104/pp.119.3.1091
Mateus-Cagua, D., Rodríguez-Yzquierdo, G. (2019). Effect of biostimulants on dry matter accumulation and gas exchange in plantain plants (Musa AAB). Rev. Colombiana Cienc. Hortícolas 13, 151–160. doi: 10.17584/rcch.2019v13i2.8460
Mena-Petite, A., Ortega-Lasuen, U., González-Moro, M. B., Lacuesta, M., Muñoz-Rueda, A. (2001). Storage duration and temperature effect on the functional integrity of container and bare-root Pinus radiata D. Don stock-types. Trees 15, 289–296. doi: 10.1007/s004680100104
Mones Sardrodi, M., Biglari Quchan Atigh, Z., Dehghanian, Z., Balilashaki, K., Asgari Lajayer, B., Astatkie, T. (2022). “Chapter 2—Application of biostimulants to improve agronomic and physiological responses of plants: A review,” in New and Future Developments in Microbial Biotechnology and Bioengineering. Sustainable Agriculture: Revisiting Green Chemicals. Eds. Singh, H. B., Vaishnav, A. (Elsevier, Amsterdam, The Netherlands), 31–44. doi: 10.1016/B978-0-323-85581-5.00012-4
Moreno-Hernández, J. M., Benítez-García, I., Mazorra-Manzano, M. A., Ramírez-Suárez, J. C., Sánchez, E. (2020). Strategies for production, characterization and application of protein-based biostimulants in agriculture: A review. Chilean J. Agric. Res. 80, 274–289. doi: 10.4067/S0718-58392020000200274
Muhie, S. H. (2023). Plant biostimulants in organic horticulture: A review. J. Plant Growth Regul. 42, 2698–2710. doi: 10.1007/s00344-022-10738-7
Navari-Izzo, F., Quartacci, M. F., Izzo, R. (1990). Water-stress induced changes in protein and free amino acids in field grown maize and sunflower. Plant Physiol. Biochem. 28, 531–537.
Nunes, A. R., Gonçalves, A. C., Falcão, A., Alves, G., Silva, L. R. (2021). Prunus avium L. (Sweet cherry) by-products: A source of phenolic compounds with antioxidant and anti-hyperglycemic properties—A review. Appl. Sci. 11, 8516. doi: 10.3390/app11188516
Osaki, M., Shinano, T., Tadano, T. (1991). Redistribution of carbon and nitrogen compounds from the shoot to the harvesting organs during maturation in field crops. Soil Sci. Plant Nutr. 37, 117–128. doi: 10.1080/00380768.1991.10415017
Parađiković, N., Teklić, T., Zeljković, S., Lisjak, M., Špoljarević, M. (2019). Biostimulants research in some horticultural plant species—A review. Food Energy Secur. 8, e00162. doi: 10.1002/fes3.162
Pylak, M., Oszust, K., Frąc, M. (2019). Review report on the role of bioproducts, biopreparations, biostimulants and microbial inoculants in organic production of fruit. Rev. Environ. Sci. Bio/Technol. 18, 597–616. doi: 10.1007/s11157-019-09500-5
Quentin, A. G., Close, D. C., Hennen, L. M. H. P., Pinkard, E. A. (2013). Down-regulation of photosynthesis following girdling, but contrasting effects on fruit set and retention, in two sweet cherry cultivars. Plant Physiol. Biochem. 73, 359–367. doi: 10.1016/j.plaphy.2013.10.014
Rasheed, R., Iqbal, M., Ashraf, M. A., Hussain, I., Shafiq, F., Yousa, A., et al. (2018). Glycine betaine counteracts the inhibitory effects of waterlogging on growth,photosynthetic pigments, oxidative defence system, nutrient composition, and fruitquality in tomato. J. Hortic. Sci. Biotechnol. 93, 385–391. doi: 10.1080/14620316.2017.1373037
Reich, P. B., Ellsworth, D. S., Walters, M. B. (2000). Specific leaf area regulates photosynthesis-nitrogen relations: global evidence from within and across species and functional groups. Funct. Ecol. 14, 155–164. doi: 10.1080/13614530009516806
Rojas, G., Fernandez, E., Whitney, C., Luedeling, E., Cuneo, I. F. (2021). Adapting sweet cherry orchards to extreme weather events—Decision Analysis in support of farmers’ investments in Central Chile. Agric. Syst. 187, 103031. doi: 10.1016/j.agsy.2020.103031
Rosa, M., Prado, C., Podazza, G., Interdonato, R., González, J. A., Hilal, M., et al. (2009). Soluble sugars. Plant Signaling Behav. 4, 388–393. doi: 10.4161/psb.4.5.8294
Rouphael, Y., Colla, G. (2020). Toward sustainable agriculture through plant biostimulants: From experimental data to practical applications. Agronomy 10, 1461. doi: 10.3390/agronomy10101461
Rouphael, Y., De Micco, V., Arena, C., Raimondi, G., Colla, G., De Pascale, S. (2017). Effect of Ecklonia maxima seaweed extract on yield, mineral composition, gas exchange, and leaf anatomy of zucchini squash grown under saline conditions. J. Appl. Phycol 29, 459–470. doi: 10.1007/s10811-016-0937-x
Sachdev, S., Ansari, S. A., Ansari, M. I., Fujita, M., Hasanuzzaman, M. (2021). Abiotic stress and reactive oxygen species: Generation, signaling, and defense mechanisms. Antioxidants 10, 277. doi: 10.3390/antiox10020277
Salvadores, Y., Bastías, R. M. (2023). Environmental factors and physiological responses of sweet cherry production under protective cover systems: A review. Chilean J. Agric. Res. 83, 484–498. doi: 10.4067/S0718-58392023000400484
Savé, R., Terradas, J., Castell, C. (1999). “Gas exchange and water relations. An ecophysiological approach to plant response to environment,” in Ecology of mediterranean evergreen oak forests, ecological studies, vol. 137 . Eds. Savé, R., Terradas, J., Castell, C., Rodà, F., Retana, J., Gracia, C. A., et al (Springer-Verlag, Berlin), 135–147.
Serapicos, M., Afonso, S., Gonçalves, B., Silva, A. P. (2022). Exogenous application of glycine betaine on sweet cherry tree (Prunus avium L.): effects on tree physiology and leaf properties. Plants 11 (24), 3470. doi: 10.3390/plants11243470
Sestak, Z., Catský, J., Jarvis, P. G. (1971). Plant photosynthetic production. Manual of methods. Plant photosynthetic production: Manual of methods (The Hague, Netherlands: Dr W. Junk Publishers).
Shan, T., Jin, P., Zhang, Y., Huang, Y., Wang, X., Zheng, Y. (2016). Exogenous glycine betaine treatment enhances chilling tolerance of peach fruit during cold storage. Postharvest Biol. Technol. 114, 104–110. doi: 10.1016/j.postharvbio.2015.12.005
Sharma, A., Pathania, A., Sharma, P., Bhardwaj, R., Sharma, I. (2023). “Role of glycine betaine in regulating physiological and molecular aspects of plants under abiotic stress,” in The Role of Growth Regulators and Phytohormones in Overcoming Environmental Stress, 327–353. doi: 10.1016/C2020-0-04073-9
Singleton, V. L., Rossi, J. A. (1965). Colorimetry of total phenolics with phosphomolybdic-phosphotungstic acid reagents. Am. J. Enol. Vitic. 16 (3), 144–158. doi: 10.5344/ajev.1965.16.3.144
Sofo, A., Dichio, B., Xiloyannis, C., Masia, A. (2004). Effects of different irradiance levels on some antioxidant enzymes and on malondialdehyde content during rewatering in olive tree. Plant Sci. 166, 293–302. doi: 10.1016/j.plantsci.2003.09.018
Sorrentino, M., Panzarova, K., Spyroglou, I., Spíchal, L., Buffagni, V., Ganugi, P., et al. (2022). Integration of phenomics and metabolomics datasets reveals different mode of action of biostimulants based on protein hydrolysates in Lactuca sativa L. and Solanum lycopersicum L. under salinity. Front. Plant Sci. 12, 808711. doi: 10.3389/fpls.2021.808711
Sytykiewicz, H., Sprawka, I., Czerniewicz, P., Sempruch, C., Leszczyński, B., Sikora, M. (2013). Biochemical characterisation of chlorophyllase from leaves of selected Prunus species-A comparative study. Acta Biochim. Polonica 60, 457–465. doi: 10.18388/abp.2013_2007
Thalmann, M., Santelia, D. (2017). Starch as a determinant of plant fitness under abiotic stress. New Phytol. 214, 943–951. doi: 10.1111/nph.2017.214.issue-3
Thimann, K. W. (1980). “The senescence of leaves,” in Senescence in plants. Ed. Thimann, K. W. (CRC Press, Boca Raton, Florida, USA), 85–117.
Tudela, V., Sarricolea, P., Serrano-Notivoli, R., Meseguer-Ruiz, O. (2023). A pilot study for climate risk assessment in agriculture: a climate-based index for cherry trees. Natural Hazards 115, 163–185. doi: 10.1007/s11069-022-05549-8
von Caemmerer, S., Farquhar, G. D. (1981). Some relationships between the biochemistry of photosynthesis and the gas exchange of leaves. Planta 153, 376–387. doi: 10.1007/BF00384257
Yakhin, O. I., Lubyanov, A. A., Yakhin, I. A., Brown, P. H. (2017). Biostimulants in plant science: a global perspective. Front. Plant Sci. 7, 2049. doi: 10.3389/fpls.2016.02049
Zargar Shooshtari, F., Souri, M. K., Hasandokht, M. R., Jari, S. K. (2020). Glycine mitigates fertilizer requirements of agricultural crops: case study with cucumber as a high fertilizer demanding crop. Chem. Biol. Technol. Agric. 7, 19. doi: 10.1186/s40538-020-00185-5
Zulfiqar, F., Ashraf, M., Siddique, K. H. (2022). Role of glycine betaine in the thermotolerance of plants. Agronomy 12, 276. doi: 10.3390/agronomy12020276
Keywords: Prunus avium L., spray treatments, glycine betaine, Ecklonia maxima, leaf gas exchange, leaf metabolites, water status
Citation: Afonso S, Oliveira I, Guedes F, Meyer AS and Gonçalves B (2024) GLYCINE betaine and seaweed-based biostimulants improved leaf water status and enhanced photosynthetic activity in sweet cherry trees. Front. Plant Sci. 15:1467376. doi: 10.3389/fpls.2024.1467376
Received: 19 July 2024; Accepted: 07 November 2024;
Published: 20 December 2024.
Edited by:
Karel Dolezal, Academy of Sciences of the Czech Republic, CzechiaCopyright © 2024 Afonso, Oliveira, Guedes, Meyer and Gonçalves. This is an open-access article distributed under the terms of the Creative Commons Attribution License (CC BY). The use, distribution or reproduction in other forums is permitted, provided the original author(s) and the copyright owner(s) are credited and that the original publication in this journal is cited, in accordance with accepted academic practice. No use, distribution or reproduction is permitted which does not comply with these terms.
*Correspondence: Sílvia Afonso, c2Fmb25zb0B1dGFkLnB0