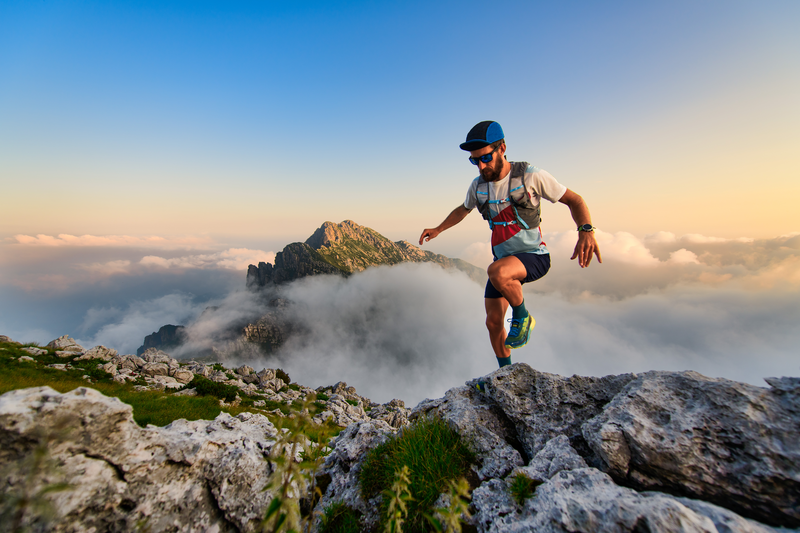
94% of researchers rate our articles as excellent or good
Learn more about the work of our research integrity team to safeguard the quality of each article we publish.
Find out more
OPINION article
Front. Plant Sci. , 03 January 2025
Sec. Plant Abiotic Stress
Volume 15 - 2024 | https://doi.org/10.3389/fpls.2024.1365133
This article is part of the Research Topic Enhancing Salinity Tolerance in Crop Plants through Agronomic, Genetic, Molecular, and Physiological Approaches View all 18 articles
The rapid alkalinization factor (RALF) polypeptide is a small (composed of 49 amino acids) molecule that was originally found in tobacco (Nicotiana tabacum) leaves (Pearce et al., 2001) and has been studied for over 20 years as a plant development regulator (Blackburn et al., 2020). RALF peptides are widely distributed in the plant kingdom and regulate several key developmental processes including root development (Wu et al., 2007; Murphy et al., 2016), pollen growth (Ge et al., 2017), and salinity stress (SS) tolerance (Zhao et al., 2018). However, the evolutionary analysis of homologous genes encoding RALF precursor proteins showed that RALF initially had the function of accelerating apical growth, and ultimately differentiated into new functions in vascular plants through multiple tandem replication events (Cao and Shi, 2012; Sharma et al., 2016; Campbell and Turner, 2017; Ginanjar et al., 2022). The functional differences in RALFs drive complex morphogenesis in land plants and facilitate other novel processes (Liu et al., 2024).
Structural analysis of the RALF proteins shows that RALFs consist of a single peptide and a mature RALF peptide (Figure 1A). The mature peptide has relatively conserved domains and is mainly divided into four major clades and two distinct functional subgroups. The mature RALF sequence’s N- and C-terminal regions can interact with different receptor proteins, thus forming different complexes by producing different conformational changes. For instance, the N-terminus of AtRALF23 formed the α-helix in the complex, which binds to the large surface groove on LLG2, while the C-terminus can bind to the extracellular domain of FERONIA (FER) (Xiao et al., 2019). In the RALF-LLG- BUDDHA’S PAPER SEAL 1/2/ANXUR 1/2 complex, the N-terminal region of RALF4 is necessary for its biological function (Ge et al., 2019), indicating that RALFs can bind to different proteins to form different complexes.
Figure 1. (A) Structural composition of rapid alkalinization factor (RALF) peptide, (B) role of RALF in regulating salinity tolerance in plants, and (C) phylogenetic tree of different RALF genes in plant species. The lower side of the cell shows the activity of RALFs under salt stress; NaCl-induced Ca2+ is sensed by the extracellular domain of FERONIA (FER) and co-receptor LLGs, which also senses the cell wall perturbation and initiates cell wall repair (Feng et al., 2018). Higher Na+ entry into cytosol induces cell wall perturbation, dissociates RALF22/23 from LRXs, and promotes RALF22/23-induced internalization of FER, and finally, RALF inhibits the signal transduction ability of FER (Zhao et al., 2018). Moreover, RALF complex with LRX and FER induces cell death under salt stress through loss of ABA homeostasis, higher Na+ accumulation, and ROS production mediated by the Respiratory burst oxidase homolog (RBOH) gene (Zhao et al., 2021). Phylogenetic tree of different RALF genes in Arabidopsis, rice, and soybean. We considered one model plant species (Arabidopsis thaliana L.), one cereal (rice—Oryza sativa L.), and one legume (soybean—Glycine max L.). We first found the number of RALF genes; for example, 27 soybeans, 41 rice, and 34 Arabidopsis RALF protein sequences were downloaded from the phytozome (https://phytozome-next.jgi.doe.gov/), rice Genomics Network (https://rice.uga.edu/cgi-bin/gbrowse/rice/), and TAIR (www.arabidopsis.org/) databases, respectively. The phylogenetic tree was produced using MEGA 11 software via the Neighbor-Joining (NJ) method with 1,000 bootstrap replicates.
Many RALF peptides can cause extracellular alkalization by increasing the extracellular pH. For instance, in H+-ATPase 2 (AHA2) protein, the phosphorylation of Thr881 and Thr947 activated H+ transport (Li et al., 2022b), while the RALF–FER interaction phosphorylates plasma membrane (PM) H+-ATPase at Ser899 and then mediates the inhibition of proton transport (Haruta et al., 2014), indicating that the inhibition of H+ pump activity is important for the increasing extracellular pH caused by RALF.
SS is a worldwide dilemma and improving salinity tolerance (ST) in plants is highly complex due to the involvement of several key players in regulating ST in plants (Khan et al., 2020; Tanveer and Ahmed, 2020). The SS reduces plant growth by inducing membrane depolarization, which later increases ROS production in the cytosol and activated voltage-gated and ROS-activated K+-outward rectifying channel and transporter at the PM (Figure 1B) (Wegner et al., 2011; Shabala et al., 2015). Thus, in this context, the activation of PM-H+-ATPase and Ca2+ signaling is critical in regulating cytosolic K+ homeostasis and ST in plants.
Compared with alkalization, higher activation of H+-ATPase is required to reduce the SS-induced activation of voltage-gated K+ outward rectifying channels (e.g., GORK) at the PM (Shabala S. et al., 2016). SS results in a significant membrane depolarization leading to a considerable disturbance in cell ionic balance and metabolism. Plants’ ability to maintain highly negative membrane potential (MP) values has been firmly associated with their tolerance to SS (Chen et al., 2007; Bose et al., 2015; Chakraborty et al., 2016). In this context, RALF proteins are important for alkalization and may play an important role in regulating PM-H+-ATPase to regulate ST in plants. RALF regulates the activity of H+-ATPase (Gjetting et al., 2020), which later imposes a massive implication to the regulation of plant ionic homeostasis via controlling cell MP at the PM. Though H+-ATPase activity is critical in determining cell MP (Palmgren and Nissen, 2011), more negative MPs are required for the operation of voltage-gated ion channels and ion transporters. The H+ gradients between extracellular and intracellular space create an H+ motive force for the secondary active transport of other ions (e.g., K+, NH4+, NO3-; PO42-; SO42-) via H+-coupled co-transport systems (Shabala S. et al., 2016). Thus, RALF-induced modulation of H+-ATPase activity may be an essential factor in controlling cellular MP and, ultimately, cell metabolism under stress conditions. However, it has been argued that higher H+-ATPase activity may lead to ATP reduction in the cells, which later affects the ability of plants to survive under SS (Rubio et al., 2020). Thus, tight regulation between the activation of RALF-mediated H+-ATPase and ATP reduction is required. However, there is also less evidence to prove that RALF as an upstream inhibitor of H+-ATPase blocks some channels or transporters (e.g., SOS1 and HAK family) that are driven by H+ gradient. Moreover, owing to the diverse ability of RALFs to interact with other receptor proteins, it can be suggested that different RALFs may affect different signal pathways to activate H+ transporters/channels in the cell and regulate ST in plants. The LRR domains of LRX3 and LRX4 can interact with both AtRALF22/23 and FER to regulate salt stress response (Zhao et al., 2018). Recently, Gjetting et al. (2020) showed that the H+ pump activity was increased three times after the application of 1 µM RALF33/36, while the aha mutant was hypersensitive to AtRALF1, which was some unknown H+ transporters or channels that led to the net influx of H+ in the cytoplasm and an increase in extracellular pH in highly possible (Li et al., 2022a). However, how cells accurately perceive the extracellular environmental pH and release RALF to regulate it is a problem that needs to be solved.
SS also triggers apoplastic alkalization and thereby inhibits plant growth (Kesten et al., 2019); however, it can also induce the formation of mature RALFs (Zhao et al., 2018). In two halophytes, higher activation of H+-ATPases under SS contributes to Na+ efflux from cytosol and low apoplastic pH associated with higher Na+/H+ exchanger at PM (Bose et al., 2015). Such SS-induced apoplastic alkalization could later be mediated by RALF–FER–AHA2 and apoplastic acidification is important for ST. This aspect needs to be examined in future studies.
Ca2+ being an important component of the cell wall (CW) and membrane structure (Bascom et al., 2018) and the oscillations of the cytoplasmic Ca2+ concentration as a second messenger are involved in various physiological reactions and signal transmission processes (Sanders et al., 1999; Thor, 2019). So far, only a few extracellular mediators have been found to affect cytoplasmic Ca2+ “signatures”. In Arabidopsis, AtRALF1 increased the cytoplasmic Ca2+ level by promoting the influx of extracellular Ca2+ and reducing the efflux of intracellular Ca2+ (Haruta et al., 2008). Later, the relationship between RALF-induced Ca2+ signal and pH was confirmed as RALF-induced extracellular pH change depends on Ca2+ signal, which occurs before alkalinization (Gjetting et al., 2020). AtRALF1 also interacts in a Ca2+- and pH-dependent manner with calmodulin-like 38 for regulating root growth (Campos et al., 2018). Furthermore, RALF33 treatment did not affect the characteristics of Ca2+ and H+ while RALFL36 treatment showed some effects in the fer Arabidopsis mutant (Gjetting et al., 2020), again suggesting that different RALF peptides may bind with different receptors to trigger intracellular Ca2+, which later may activate H+ pumping at PM. Therefore, exploring other unknown RALF receptor-induced Ca2+ oscillations and testing whether their signal pathways overlap is a direction noteworthy in the future (Tanveer et al., 2018; Choudhary et al., 2021).
RALF proteins regulate the overall plant growth and redox homeostasis by regulating ROS production (Zhang X. et al., 2020). For instance, FER positively regulates root hair polar growth by regulating auxin-mediated ROS production (Yu et al., 2012). Likewise, FER and related proteins regulate ROS production by regulating the transcription of respiratory burst oxidase homologs (RBOH) (Franck et al., 2018). For instance, the FER–LLG1–Rop–Guanine Nucleotide Exchange factor complex regulates RBOH dependence ROS production (Duan et al., 2010; Li et al., 2015). ANX1 and ANX2 also maintain ROS production and regulate CW integrity during pollen tube growth (Boisson-Dernier et al., 2013); thus, RALFs protein complex requires ROS as important signaling molecules for regulating cell growth (Zhang X. et al., 2020). Nonetheless, the overproduction of ROS under SS is inevitable, and ST is linked to maintaining an equilibrium between overall ROS production and ROS scavenging; thus, tight regulation is required (Tanveer and Shabala, 2018; Khan et al., 2020). Moreover, the effects of SS on the overall redox state are highly tissue-specific and NaCl dose-specific (Shah et al.). This should be explored in future studies.
CW biosynthesis is a very complex mechanism in plants, and to examine the CW status, plants exhibit the CW integrity maintenance (CWIM) system. The CWIM system assists plants in adapting to stress conditions without compromising the integrity and organization of CW (Liu et al., 2021). SS can adversely affect CWI; thus, plants’ ability to maintain the CWIM system is essential for ST. Having said that, Ca2+ being a universal secondary messenger is actively involved in the operation of CWIM system in plants. However, the maintenance of balance between Ca2+ concentrations in CW, apoplast, and cytoplasm raises the question relating to the validity of this concept. In this context, Feng et al. (2018) showed that FER is required for the activation of Ca2+ influx and maintenance of CWI under salt stress (Figure 1B). FER is an important CWIM sensor and required for Ca2+ influx into cytoplasm under SS (Feng et al., 2018). FER contains two malectin domains that directly bind with de-methyl-esterified HG in vitro and in vivo (Feng et al., 2018; Lin et al., 2018; Liu et al., 2021). This suggests that FER probably senses the CW changes directly via its extracellular domain and then transduces the CW signals to the cytoplasm via its cytoplasmic kinase domain. Later, it was shown that SS may dissociate the LRX3/4/5-RALFs complex via the SS-induced ROS and pH changes in the apoplast, and the released RALFs bind to the LLG1–FER complex and thereby allow the transduction of CW signals (Zhao et al., 2018). The mechanism behind the dissociation of LRX3/4/5 and RALFs under SS needs to be further investigated. Moreover, FEI1 and FEI2 are two other important LRR–RLK complexes that regulate cellulose synthesis in the CW (Xu et al., 2008), and loss-of-function mutants of FEI1 and FEI2 showed roots with reduced cellulose contents (Basu et al., 2015), thus indicating the role of FEIs in CWI sensing.
Hormonal regulation under SS is also important, as different phytohormones regulate different physiological processes (Tanveer et al., 2018; Choudhary et al., 2021). Abscisic acid (ABA) is an essential hormone of plant stress resistance and tolerance. The signal crosstalk between RALF and ABA is involved in the response of plants to abiotic stresses including SS and water deficit (Chen et al., 2016). Studies showed that the RALF1-FER signaling pathway activates ABI2 (ABA Insensitive 2) phosphatase by the GEF1/4/10-ROP11 pathway and further inhibits ABA response (Yu et al., 2012; Chen et al., 2016). The LRX is an important receptor of CWI signal, and the LRX3/4/5 triple mutants as well as FER mutants displayed salt hypersensitivity, which was mimicked by overexpression of RALF22/23 (Zhao et al., 2018). In Arabidopsis, LRX3/4/5-RALF22/23-FER regulated ST by regulating equilibrium between ROS production and accumulation of phytohormones (ABA, JA, and SA) (Zhao et al., 2021). Moreover, RALF acts as upstream of the ROS regulatory pathway and has been shown to interact with ABA to regulate the growth of plant roots (Chen et al., 2016).
The AGB1-a G protein β-subunit involved in ABA mediating stomatal opening and FER-ABG1 are reported to be involved in salt stress responses (Yu et al., 2018a). Mutants lacking FER and ABG1 showed a hypersensitive phenotype to salt stress (Yu et al., 2018a), and even the application of AtRALF was not effective in reducing hypersensitivity to salt stress (Zhao et al., 2018). In contrast, AtRALF1 mutants did not show any response to higher salt stress levels (Feng et al., 2018). Taking an example of non-vascular plants, PpRALF3 knockout lines showed higher resistance under SS and ROS stress in moss (Physcomitrium patens), implying the functional role of RALFs in regulating ST (Solís-Miranda et al., 2023). However, the relationship of RALFs with other phytohormones, e.g., melatonin, should be considered in future studies. Melatonin-induced enhancement of PM H+-ATPase activity may negate salinity-induced MP depolarization, preventing the activation of outward K+ channels and thereby leading to higher ST (Yu et al., 2018b). Moreover, the abiotic stress regulatory role of MEL has also been reported elsewhere (Tanveer and Shabala, 2020; Huang et al., 2022, 2024).
Given that, RALFs’ gene expression is highly specific to plant species and tissues (Kim et al., 2021). For instance, five homologs of RALFs were observed in poplar (Haruta and Constabel, 2003) while 37 homologs were in Arabidopsis (Abarca et al., 2021). A total of 765 RALF proteins were identified from 51 plant species (Campbell and Turner, 2017). Recently, a genome-wide association study revealed 163 RALF genes in seven species from the Rosaceae family, including 45 mature RALF genes (Zhang H. et al., 2020). A phylogenetic tree analysis showed the diversification of different RALF genes in different plant species (Figure 1C), thus indicating that the genetic differences among different plant species could also govern the regulation of RALF genes in plants.
RALFs are widely distributed in plants and RALF-induced cell expansion is the result of the interaction of the changes of intra- and extracellular ions, deposition of new CW materials, and the rearrangement of existing CWs. During the transition from vegetative growth to reproductive growth, RALFs have different functions in maintaining the normal life activities of plants. This is achievable because RALF forms a complex signal network to complete these complex functions. For instance, the RALF–FER signal transduction pathway is highly conserved in plants and is very important for mediating RALF signaling. Thus, the physiological significance of the regulatory activities of RALF and some membrane receptors is still unknown and several outstanding questions should be focused on while examining the role of RALFs in regulating ST in plants. For instance,
● How RALF-induced FER-specific internalization conducts Ca2+ transduction in CW is also a focus of research.
● Equally interesting is the question of intracellular signal transduction of RALF-induced FER-specific internalization. While it is widely accepted that RALFs regulate plant growth and development together with CW components and PM receptors, spatiotemporal aspects of such regulation remain largely unknown, in the light of the apparent dual function of RALF.
● It is also worth noting that some plant hormones such as ABA or melatonin are also involved in ST; however, whether RALF is directly or indirectly involved in crosstalk with these phytohormones to mediate ST is not fully comprehended.
● Moreover, whether RALF is directly or indirectly involved in ion rebalance and transport under SS via regulating Ca2+ signaling and H+-ATPase is not yet clear. Therefore, exploring the mechanism of RALF under SS is also an important direction and a great challenge for future research in developing ST crops.
● Given that the RALF peptide family is very diverse and binds to large arrays of receptors, mechanisms regulating the specificity of the RALF–receptor interaction under different growth conditions should be examined.
LH: Writing – original draft. XL: Visualization, Writing – review & editing. QW: Writing – review & editing. WC: Writing – review & editing. WF: Writing – review & editing. YG: Writing – original draft, Writing – review & editing.
The author(s) declare financial support was received for the research, authorship, and/or publication of this article. This work was supported by the National Natural Science Foundation of China (grant no. 31901202), the Higher Education Department of Guangdong province (2020KCXTD025), Key Laboratory Project of Guangdong province (grant No. 2022B1212010015).
Author YG was employed by the company Foshan ZhiBao Ecological Technology Co. Ltd.
The remaining authors declare that the research was conducted in the absence of any commercial or financial relationships that could be construed as a potential conflict of interest.
All claims expressed in this article are solely those of the authors and do not necessarily represent those of their affiliated organizations, or those of the publisher, the editors and the reviewers. Any product that may be evaluated in this article, or claim that may be made by its manufacturer, is not guaranteed or endorsed by the publisher.
Abarca, A., Franck, C. M., Zipfel, C. (2021). Family-wide evaluation of rapid alkalinization factor peptides. Plant Physiol. 187, 996–1010. doi: 10.1093/plphys/kiab308
Bascom, C. S., Hepler, P. K., Bezanilla, M. (2018). Interplay between ions, the cytoskeleton, and cell wall properties during tip growth. Plant Physiol. 176, 28–40. doi: 10.1104/pp.17.01466
Basu, D., Wang, W., Ma, S., DeBrosse, T., Poirier, E., Emch, K., et al. (2015). Two hydroxyproline galactosyltransferases, GALT5 and GALT2, function in arabinogalactan-protein glycosylation, growth and development in Arabidopsis. PloS One 10(5), e0125624. doi: 10.1371/journal.pone.0125624
Blackburn, M. R., Haruta, M., Moura, D. S. (2020). Twenty years of progress in physiological and biochemical investigation of RALF peptides. Plant Physiol. 182, 1657–1666. doi: 10.1104/pp.19.01310
Boisson-Dernier, A., Lituiev, D. S., Nestorova, A., Franck, C. M., Thirugnanarajah, S., Grossniklaus, U. (2013). ANXUR receptor-like kinases coordinate cell wall integrity with growth at the pollen tube tip via NADPH oxidases. PloS Biol. 11, e1001719. doi: 10.1371/journal.pbio.1001719
Bose, J., Rodrigo-Moreno, A., Lai, D., Xie, Y., Shen, W., Shabala, S. (2015). Rapid regulation of the plasma membrane H+-ATPase activity is essential to salinity tolerance in two halophyte species, Atriplex lentiformis and Chenopodium quinoa. Ann. Bot. 115, 481–494. doi: 10.1093/aob/mcu219
Campbell, L., Turner, S. R. (2017). A comprehensive analysis of RALF proteins in green plants suggests there are two distinct functional groups. Front. Plant Sci. 8. doi: 10.3389/fpls.2017.00037
Campos, W. F., Dressano, K., Ceciliato, P. H. O., Guerrero-Abad, J. C., Silva, A. L., Fiori, C. S., et al. (2018). Arabidopsis thaliana rapid alkalinization factor 1–mediated root growth inhibition is dependent on calmodulin-like protein 38. J. Biol. Chem. 293, 2159–2171. doi: 10.1074/jbc.m117.808881
Cao, J., Shi, F. (2012). Evolution of the RALF gene family in plants: gene duplication and selection patterns. Evol. Bioinf. 8, EBO.S9652. doi: 10.4137/ebo.s9652
Chakraborty, K., Bose, J., Shabala, L., Shabala, S. (2016). Difference in root K+ retention ability and reduced sensitivity of K+ -permeable channels to reactive oxygen species confer differential salt tolerance in three Brassica species. J. Exp. Bot. 67, 4611–4625. doi: 10.1093/jxb/erw236
Chen, J., Yu, F., Liu, Y., Du, C. Q., Li, X. S., Zhu, S. R., et al. (2016). FERONIA interacts with ABI2-type phosphatases to facilitate signaling cross-talk between abscisic acid and RALF peptide in Arabidopsis. Proc. Natl. Acad. Sci. 113, E5519–E5527. doi: 10.1073/pnas.1608449113
Chen, F., Yuan, Y., Li, Q., He, Z. (2007). Proteomic analysis of rice plasma membrane reveals proteins involved in early defense response to bacterial blight. PROTEOMICS 7, 1529–1539. doi: 10.1002/pmic.200500765
Choudhary, P., Pramitha, L., Rana, S., Verma, S., Aggarwal, P. R., Muthamilarasan, M. (2021). Hormonal crosstalk in regulating salinity stress tolerance in graminaceous crops. Physiol. Plant. 173, 1587–1596. doi: 10.1111/ppl.13558
Duan, Q., Kita, D., Li, C., Cheung, A. Y., Wu, H. M. (2010). FERONIA receptor-like kinase regulates RHO GTPase signaling of root hair development. Proc. Natl. Acad. Sci. U.S.A. 107, 17821–17826. doi: 10.1073/pnas.1005366107
Feng, W., Kita, D., Peaucelle, A., Cartwright, H. N., Doan, V., Duan, Q., et al. (2018). The FERONIA receptor kinase maintains cell-wall integrity during salt stress through Ca2+ signaling. Curr. Biol. 28, 666–675. doi: 10.1016/j.cub.2018.01.023
Franck, C. M., Westermann, J., Boisson-Dernier, A. (2018). Plant malectin-like receptor kinases: from cell wall integrity to immunity and beyond. Annu. Rev. Plant Biol. 69 (2018), 301–328. doi: 10.1146/annurev-arplant-042817-040557
Ge, Z., Bergonci, T., Zhao, Y., Zou, Y. J., Du, S., Liu, M. C., et al. (2017). Arabidopsis pollen tube integrity and sperm release are regulated by RALF-mediated signaling. Science 358, 1596–1600. doi: 10.1126/science.aao3642
Ge, Z., Cheung, A. Y., Qu, L. (2019). Pollen tube integrity regulation in flowering plants: insights from molecular assemblies on the pollen tube surface. New Phytol. 222, 687–693. doi: 10.1111/nph.15645
Ginanjar, E. F., Teh, O., Fujita, T. (2022). Characterisation of rapid alkalinisation factors in Physcomitrium patens reveals functional conservation in tip growth. New Phytol. 233, 2442–2457. doi: 10.1111/nph.17942
Gjetting, S. K., Mahmood, K., Shabala, L., Kristensen, A., Shabala, S., Fuglsang, A. T. (2020). Evidence for multiple receptors mediating RALF-triggered Ca2+ signaling and proton pump inhibition. Plant J. 104, 433–446. doi: 10.1111/tpj.14935
Haruta, M., Constabel, C. P. (2003). Rapid alkalinization factors in poplar cell cultures. Peptide Isolation, cDNA Cloning, and Differential Expression in Leaves and Methyl Jasmonate-Treated Cells. Plant Physiol. 131, 814–823. doi: 10.1104/pp.014597
Haruta, M., Monshausen, G., Gilroy, S., Sussman, M. R. (2008). A cytoplasmic Ca2+ functional assay for identifying and purifying endogenous cell signaling peptides in Arabidopsis Seedlings: identification of AtRALF1 peptide. Biochemistry 47, 6311–6321. doi: 10.1021/bi8001488
Haruta, M., Sabat, G., Stecker, K., Minkoff, B. B., Sussman, M. R. (2014). A peptide hormone and its receptor protein kinase regulate plant cell expansion. Science 343, 408–411. doi: 10.1126/science.1244454
Huang, L., Fu, W., Zhang, Y., Liu, X., Wang, Q., Wang, L., et al. (2024). The role of melatonin in regulating horticultural crop production under various abiotic stresses. Scientia Horticult. 323, 112508. doi: 10.1016/j.scienta.2023.112508
Huang, X., Tanveer, M., Min, Y., Shabala, S. (2022). Melatonin as a regulator of plant ionic homeostasis: implications for abiotic stress tolerance. J. Exp. Bot. 73, 5886–5902. doi: 10.1093/jxb/erac224
Kesten, C., Wallmann, A., Schneider, R., McFarlane, H. E., Diehl, A., Khan, G. A., et al. (2019). The companion of cellulose synthase 1 confers salt tolerance through a Tau-like mechanism in plants. Nat. Commun. 10, 857. doi: 10.1038/s41467-019-08780-3
Khan, W. U. D., Tanveer, M., Shaukat, R., Ali, M., Pirdad, F. (2020). An overview of salinity tolerance mechanism in plants. Salt drought Stress tolerance plants: Signaling Networks adaptive mechanisms, 1–16. doi: 10.1007/978-3-030-40277-8_1
Kim, D., Yang, J., Gu, F., Park, S., Combs, J., Adams, A., et al. (2021). A temperature-sensitive FERONIA mutant allele that alters root hair growth. Plant Physiol. 185, 405–423. doi: 10.1093/plphys/kiaa051
Li, L., Chen, H., Alotaibi, S. S., Pěnčík, A., Adamowski, M., Novák, O., et al. (2022a). RALF1 peptide triggers biphasic root growth inhibition upstream of auxin biosynthesis. Proc. Natl. Acad. Sci. 119, e2121058119. doi: 10.1073/pnas.2121058119
Li, C., Yeh, F. L., Cheung, A. Y., Duan, Q., Kita, D., Liu, M. C., et al. (2015). Glycosylphosphatidylinositol-anchored proteins as chaperones and co-receptors for FERONIA receptor kinase signaling in Arabidopsis. eLife 4, e06587. doi: 10.7554/elife.06587
Li, Y., Zeng, H., Xu, F., Yan, F., Xu, W. (2022b). H+-ATPases in plant growth and stress responses. Annu. Rev. Plant Biol. 73, 495–521. doi: 10.1146/annurev-arplant-102820-114551
Lin, W., Tang, W., Anderson, C. T., Yang, Z. (2018). FERONIA’s sensing of cell wall pectin activates ROP GTPase signaling in Arabidopsis. BioRxiv, 269647. doi: 10.1101/269647
Liu, L., Liu, X., Bai, Z., Tanveer, M., Zhang, Y., Chen, W., et al. (2024). Small but powerful: RALF peptides in plant adaptive and developmental responses. Plant Sci. 343, 112085. doi: 10.1016/j.plantsci.2024.112085
Liu, J., Zhang, W., Long, S., Zhao, C. (2021). Maintenance of cell wall integrity under high salinity. Int. J. Mol. Sci. 22, 3260. doi: 10.3390/ijms22063260
Murphy, E., Vu, L. D., Broeck, L., Zhefeng Lin, Z. F., Ramakrishna, P., Cotte, B., et al. (2016). RALFL34 regulates formative cell divisions in Arabidopsis pericycle during lateral root initiation. J. Exp. Bot. 67, 4863–4875. doi: 10.1093/jxb/erw281
Palmgren, M. G., Nissen, P. (2011). P-type ATPases. Annu. Rev. Biophys. 40, 243–266. doi: 10.1146/annurev.biophys.093008.131331
Pearce, G., Moura, D. S., Stratmann, J., Ryan, C. A. (2001). RALF, a 5-kDa ubiquitous polypeptide in plants, arrests root growth and development. Proc. Natl. Acad. Sci. 98, 12843–12847. doi: 10.1073/pnas.201416998
Rubio, F., Nieves-Cordones, M., Horie, T., Shabala, S. (2020). Doing ‘business as usual’comes with a cost: evaluating energy cost of maintaining plant intracellular K+ homeostasis under saline conditions. New Phytol. 225 ((3)), 1097–1104. doi: 10.1111/nph.15852
Sanders, D., Brownlee, C., Harper, J. F. (1999). Communicating with calcium. Plant Cell. 11, 691. doi: 10.1105/tpc.11.4.691
Shabala, S., Bose, J., Fuglsang, A. T., Pottosin, I. (2016). On a quest for stress tolerance genes: membrane transporters in sensing and adapting to hostile soils. J. Exp. Bot. 67, 1015–1031. doi: 10.1093/jxb/erv465
Shabala, S., Wu, H., Bose, J. (2015). Salt stress sensing and early signalling events in plant roots: Current knowledge and hypothesis. Plant Sci. 241, 109–119. doi: 10.1016/j.plantsci.2015.10.003
Shabala, L., Zhang, J., Pottosin, I., Bose, J., Zhu, M., Fuglsang, A. T., et al. (2016). Cell-type-specific H+-ATPase activity in root tissues enables K+ retention and mediates acclimation of barley (Hordeum vulgare) to salinity stress. Plant Physiol. 172 (4), 2445–2458. doi: 10.1104/pp.16.01347
Sharma, A., Hussain, A., Mun, B.-G., Imran, Q. M., Falak, N., Lee, S. U., et al. (2016). Comprehensive analysis of plant rapid alkalization factor (RALF) genes. Plant Physiol. Biochem. 106, 82–90. doi: 10.1016/j.plaphy.2016.03.037
Solís-Miranda, J., Juárez-Verdayes, M. A., Nava, N., Rosas, P., Leija-Salas, A., Cárdenas, L., et al. (2023). The phaseolus vulgaris receptor-like kinase pvFER1 and the small peptides pvRALF1 and pvRALF6 regulate nodule number as a function of nitrate availability. Int. J. Mol. Sci. 24, 5230. doi: 10.3390/ijms24065230
Tanveer, M., Ahmed, H. A. I. (2020). ROS signalling in modulating salinity stress tolerance in plants. Salt Drought Stress Tolerance Plants: Signaling Networks Adapt. Mechan., 299–314. doi: 10.1007/978-3-030-40277-8_11
Tanveer, M., Shabala, S. (2018). Targeting Redox Regulatory Mechanisms for Salinity Stress Tolerance in Crops. Salinity Responses and Tolerance in Plants 1, 213–234. doi: 10.1007/978-3-319-75671-4_8
Tanveer, M., Shabala, S. (2020). Neurotransmitters in signalling and adaptation to salinity stress in plants. Neurotransmit. Plant Signaling commun., 49–73. doi: 10.1007/978-3-030-54478-2_3
Tanveer, M., Shahzad, B., Sharma, A., Biju, S., Bhardwaj, R. (2018). 24-Epibrassinolide; an active brassinolide and its role in salt stress tolerance in plants: A review. Plant Physiol. Biochem. 130, 69–79. doi: 10.1016/j.plaphy.2018.06.035
Wegner, L. H., Stefano, G., Shabala, L., Rossi, M., Mancuso, S., Shabala, S. (2011). Sequential depolarization of root cortical and stelar cells induced by an acute salt shock–implications for Na+ and K+ transport into xylem vessels. Plant Cell Environ. 34 (5), 859–869. doi: 10.1111/j.1365-3040.2011.02291.x
Wu, J., Kurten, E. L., Monshausen, G., Hummel, G. M., Gilroy, S., Baldwin, I. T. (2007). NaRALF, a peptide signal essential for the regulation of root hair tip apoplastic pH in Nicotiana attenuata, is required for root hair development and plant growth in native soils. Plant J. 52, 877–890. doi: 10.1111/j.1365-313x.2007.03289.x
Xiao, Y., Stegmann, M., Han, Z., DeFalco, T. A., Parys, K., Xu, L., et al. (2019). Mechanisms of RALF peptide perception by a heterotypic receptor complex. Nature 572, 270–274. doi: 10.1038/s41586-019-1409-7
Xu, S. L., Rahman, A., Baskin, T. I., Kieber, J. J. (2008). Two leucine-rich repeat receptor kinases mediate signaling, linking cell wall biosynthesis and ACC synthase in Arabidopsis. Plant Cell 20 (11), 3065–3079. doi: 10.1105/tpc.108.063354
Yu, Y., Chakravorty, D., Assmann, S. M. (2018a). The G protein β-subunit, AGB1, interacts with FERONIA in RALF1-regulated stomatal movement. Plant Physiol. 176, 2426–2440. doi: 10.1104/pp.17.01277
Yu, F., Qian, L., Nibau, C., Duan, Q. B., Kita, D., Levasseur, K., et al. (2012). FERONIA receptor kinase pathway suppresses abscisic acid signaling in Arabidopsis by activating ABI2 phosphatase. Proc. Natl. Acad. Sci. 109, 14693–14698. doi: 10.1073/pnas.1212547109
Yu, Y., Wang, A., Li, X., Kou, M., Wang, W., Chen, X., et al. (2018b). Melatonin-stimulated triacylglycerol breakdown and energy turnover under salinity stress contributes to the maintenance of plasma membrane H+–ATPase activity and K+/Na+ homeostasis in sweet potato. Front. Plant sci. 9. doi: 10.3389/fpls.2018.00256
Zhang, H., Jing, X., Chen, Y., Liu, Z., Xin, Y., Qiao, Y. (2020). The genome-wide analysis of RALF-like genes in strawberry (wild and cultivated) and five other plant species (Rosaceae). Genes 11, 174. doi: 10.3390/genes11020174
Zhang, X., Yang, Z., Wu, D., Yu, F. (2020). RALF–FERONIA signaling: linking plant immune response with cell growth. Plant Commun. 1 (4), 100084. doi: 10.1016/j.xplc.2020.100084
Zhao, C., Jiang, W., Zayed, O., Xin Liu, X., Tang, K., Nie, W. F., et al. (2021). The LRXs-RALFs-FER module controls plant growth and salt stress responses by modulating multiple plant hormones. Natl. Sci. Review. 8, nwaa149. doi: 10.1093/nsr/nwaa149
Keywords: salinity tolerance, Ca 2+ signaling, PM-depolarization, root activity, H + -ATPase, hormonal regulation, ROS production
Citation: Huang L, Liu X, Wang Q, Chen W, Fu W and Guo Y (2025) RALF proteins—a monitoring hub for regulating salinity tolerance in plants. Front. Plant Sci. 15:1365133. doi: 10.3389/fpls.2024.1365133
Received: 03 January 2024; Accepted: 31 October 2024;
Published: 03 January 2025.
Edited by:
Lorenzo Barbanti, University of Bologna, ItalyReviewed by:
Muhammad Ahsan Altaf, Hainan University, ChinaCopyright © 2025 Huang, Liu, Wang, Chen, Fu and Guo. This is an open-access article distributed under the terms of the Creative Commons Attribution License (CC BY). The use, distribution or reproduction in other forums is permitted, provided the original author(s) and the copyright owner(s) are credited and that the original publication in this journal is cited, in accordance with accepted academic practice. No use, distribution or reproduction is permitted which does not comply with these terms.
*Correspondence: Liping Huang, TGlwaHVhbmdAZm9zdS5lZHUuY24=; Yongjun Guo, MTY5NTUzMzYyM0BxcS5jb20=
Disclaimer: All claims expressed in this article are solely those of the authors and do not necessarily represent those of their affiliated organizations, or those of the publisher, the editors and the reviewers. Any product that may be evaluated in this article or claim that may be made by its manufacturer is not guaranteed or endorsed by the publisher.
Research integrity at Frontiers
Learn more about the work of our research integrity team to safeguard the quality of each article we publish.