- 1Citrus Research Institute, Southwest University/National Citrus Engineering Research Center, Chongqing, China
- 2Guangxi Academy of Specialty Crops, Guangxi Citrus Breeding and Cultivation Research Center of Engineering Technology, Guangxi, China
- 3Fruit Research Institute, Guizhou Provincial Academy of Agricultural Sciences, Guizhou, China
Introduction: Asian citrus psyllid (ACP, Diaphorina citri) is an important transmission vector of “Candidatus Liberibacter asiaticus” (CLas), the causal agent of Huanglongbing (HLB), the most destructive citrus disease in the world. As there are currently no HLB-resistant rootstocks or varieties, the control of ACP is an important way to prevent HLB. Some viruses of insect vectors can be used as genetically engineered materials to control insect vectors.
Methods: To gain knowledge on viruses in ACP in China, the prevalence of five RNA and DNA viruses was successfully determined by optimizing reverse transcription polymerase chain reaction (RT-PCR) in individual adult ACPs. The five ACP-associated viruses were identified as follows: diaphorina citri bunyavirus 2, which was newly identified by high-throughput sequencing in our lab, diaphorina citri reovirus (DcRV), diaphorina citri picorna-like virus (DcPLV), diaphorina citri bunyavirus (DcBV), and diaphorina citri densovirus-like virus (DcDV).
Results: DcPLV was the most prevalent and widespread ACP-associated virus, followed by DcBV, and it was detected in more than 50% of all samples tested. DcPLV was also demonstrated to propagate vertically and found more in salivary glands among different tissues. Approximately 60% of all adult insect samples were co-infected with more than one insect pathogen, including the five ACP-associated viruses and CLas.
Discussion: This is the first time these viruses, including the newly identified ACP-associated virus, have been detected in individual adult ACPs from natural populations in China’s five major citrus-producing provinces. These results provide valuable information about the prevalence of ACP-associated viruses in China, some of which have the potential to be used as biocontrol agents. In addition, analysis of the change in prevalence of pathogens in a single insect vector is the basis for understanding the interactions between CLas, ACP, and insect viruses.
1 Introduction
Citrus is the most widely cultivated fruit tree in southern China and is an important economic fruit (Zhou, 2018). Moreover, China leads the world’s production with its yield amounting to 44.6 million metric tons and accounting for 28% of the global output in summer 2021 and winter 2021/22 citrus seasons according to the World Citrus Organization (WCO, https://worldcitrusorganisation.org/). However, Huanglongbing (HLB) is a threat to citrus production and causes premature fruit drop, resulting in a 30-100% yield reduction and producing the most severe economic losses to the citrus industry (Zhou, 2020; Chinyukwi et al., 2023). Candidatus Liberibacter asiaticus (CLas) is the bacterial pathogen causing HLB. Citrus trees affected by CLas have reduced water absorption and accumulation of nutrients, which may cause plant death (Atta et al., 2020). While Asian citrus psyllid (ACP) is an insect vector that transmits CLas, it is also an important factor in the prevalence of HLB (Vyas et al., 2015; Snyder et al., 2022). At present, effective methods for the prevention and control of HLB have not been found (Ghosh et al., 2022). The prevention and control of its vector, ACP, is one of the most important measures to prevent HLB epidemics. ACP population control relies heavily on insecticides, but chemical strategies lead to the development of chemical resistance among ACP populations, pollute the environment, and may have negative effects on beneficial organisms (Leong et al., 2022). To avoid the development of insecticide-resistant insect populations, biological control strategies are being considered.
Viruses, as the most abundant microorganisms on Earth, are present in all groups of organisms (Weitz and Wilhelm, 2012). With the development of metagenomics, viruses from families, including Baculoviridae, Parvoviridae, Flaviviridae, and Bunyaviridae, are being increasingly discovered (Simmonds et al., 2017). Some viruses have complex relationships with their insect hosts, affecting the growth and development of insects or helping to spread pathogens (Bolling et al., 2015; Marklewitz et al., 2015). Marutani-Hert et al. (2009) discovered two diaphorina citri reovirus (DcRV) sequences in ACP through high-throughput sequencing. Nouri et al. (2016) discovered diaphorina citri picorna-like virus (DcPLV), diaphorina citri bunyavirus (DcBV), diaphorina citri associated C virus (DcACV), and a DNA virus called diaphorina citri densovirus (DcDV). According to phylogenetic analysis, DcPLV is close to the Iflaviridae based on the RNA-dependent RNA polymerase (RdRp); DcRV is closely related to Fijivirus; DcDV placed closer to the viruses from the genus Iteradensovirus based on NS2 amino acid; DcBV was most closely to Phasmaviridae (Nouri et al., 2016; Chen et al., 2020). Britt et al. (2020) found diaphoerina citri flavi-like virus (DcFLV) and another reovirus, diaphorina citri cimodo-like virus (DcCLV), and detected the prevalence of these viruses in Florida in 5 ACPs as a group sample. They found that DcACV had the highest detection rate in ACPs in Florida. However, it is unclear which virus is the most prevalent in ACPs in citrus groves in China.
Interactions between viral and bacterial pathogens in insects have been reported. Insect symbiotic bacterium Sulcia harbors a viral pathogen (rice dwarf virus) and mediates its transovarial transmission to offspring in leafhoppers (Jia et al., 2017). Teixeira et al. (2008) reported that the bacterial symbiont Wolbachia renders Drosophila melanogaster more resistant to the Drosophila C virus, reducing the viral load of infected flies. The effects of CLas infection on the metagenome of the Diaphorina citri gut endosymbiont were recently reported (Pan et al., 2023). Moreover, the titer of Wolbachia has been demonstrated to have a positive correlation with the CLas titer (Fagen et al., 2012). However, little is known about the relationship between CLas and ACP-associated viruses. Whether these insect viruses also have similar effects with CLas in ACP needs to be further explored.
In the present study, the main objectives were to determine the prevalence of the newly identified diaphorina citri bunyavirus 2 (DcBV2) which also belongs to Bunyavirales as well as DcBV (unpublished data) and four previously identified ACP-associated viruses, namely DcRV, DcPLV, DcBV, and DcDV, in individual adult ACPs from citrus groves in China, using PCR-based methods and to evaluate the relationship between CLas and ACP-associated viruses. Adult ACP populations were collected from five of China’s major citrus-producing and ACP-inhabiting regions: Guizhou, Jiangxi, Guangdong, Guangxi, and Sichuan. This study will provide foundational knowledge of the above viruses with regard to their infection rate in ACP in five major citrus-producing and ACP-inhabiting provinces.
2 Materials and methods
2.1 Materials
A total of 551 ACPs were gathered in Guizhou, Jiangxi, Guangdong, Guangxi, and Sichuan from 2019 to 2023 during the outbreak (Supplementary Table S1). ACP samples were collected randomly from semi-unmanaged citrus orchards by manual aspiration with flukes. The fluke is made of leather tube, mesh yarn and suction head. Immediately after collection, they were stored in 50 ml centrifuge tubes and transported to the Citrus Seedling Detoxification Center Laboratory of the Citrus Research Institute of Southwest University on dry ice and stored at −80°C for later use.
2.2 Total RNA extraction
Each ACP was removed and placed in a 1.5 mL centrifuge tube. A volume of 200 μL of Trizol reagent (Invitrogen, Beijing, China), and three 1.5 mm steel balls were added to the tube. These were then ground at 50 Hz for 2 min in a high-throughput tissue grinder (SCIENTZ-48L, Ningbo, China) at −20°C. Total RNA of individual adult ACPs was extracted according to the Trizol reagent instruction manual (Invitrogen, Beijing, China). RNA samples were stored at −20°C for later use.
2.3 RT-PCR detection of ACP virus
Using the total RNA of individual ACPs as a template, the primers shown in Table 1 were used for RT-PCR to detect DcRV, DcPLV, DcBV, DcDV, CLas, and DcBV2. β-Actin of ACP was used as the internal reference gene to verify successful RNA extraction. RT-PCR was carried out in a 10 μL reaction mixture containing 5 μL of 2×1 Step Buffer, 0.4 μL of the forward primer (10 μM), 0.4 μL of the reverse primer (10 μM), 0.4 μL of PrimeScript 1 Step Enzyme Mix (Takara, Sichuan, China), 2.8 μL RNase Free dH2O, and 1 μL RNA. RT-PCR cycling parameters were as follows: 50°C for 30 min, 94°C for 2 min, and 35 cycles of denaturation at 94°C for 30 s, extension at 55°C for 30 s, and 72°C for 1 min. The RT-PCR products were separated by 1.2% agarose gel electrophoresis and purified using the Easy Pure® Quick Gel Extraction Kit (Transgene, EG101-02). The purified products were connected to the pGEM-T Easy vector (Promega, Beijing, China) and transferred to Escherichia coli DH5α (WeiDi, Shanghai, China). The positive clones were sent to Qingke or BGI Biotechnology Co., Ltd. for sequencing. The sequencing results of the positive clones of each fragment were compared with sequences recorded in the NCBI database.
2.4 Collection of ACPs’ offspring
The nascent male and female ACPs were reared on the new shoots of clean orange jessamine and covered with gauze ribbons to prevent ACPs from escaping. After the male and female ACPs mated and laid eggs, we removed the ACP parents and left the offspring to grow. Then when ACP generation had developed to 5th instar nymph, they were individually taken out to determine whether they were infected with DcPLV using RT-PCR as methods shown in 2.2.
2.5 RT-qPCR for DcPLV detection
We collected 80 adult ACPs, dissected them with forceps in 0.1 M phosphate-buffered saline (PBS, pH 7.2) under a stereo microscope (Leica, Wetzlar, Germany) and collected guts, salivary glands, testes, ovaries, Malpighian tubules, and remnant tissues. RNA was extracted from each tissue with 4 biological replicates per sample. The concentration of RNA isolated from various organs of ACP was shown in Supplementary Table S2.
First-strand cDNA was synthesized using an All-In-One 5×RT MasterMix kit (abm, Chongqing, China) according to the manufacturer’s instructions. RNA isolated from various organs of the insect was added to 20 µl reactions with 1 µg as a template for reverse transcription. Quantification PCR was performed in 20 µL reactions with 100 ng cDNA using a BlasTaq™ 2×qPCR MasterMix kit (abm, Chongqing, China) and primers (forward primer: AAACAGTGGCGAGGAACGAT, reverse primer: CCACCAAATCCGGTCTGTCA) at concentrations of 0.25 μM according to the manufacturer’s instructions in the CFX96 touch system (Bio-Rad, Berkeley, California, USA). β-Actin of ACP was selected to normalize the DcPLV-expression level.
2.6 Statistical analysis
All detection data were calculated using Excel software. Quantitative analyses for the relative levels of gene accumulation were analyzed according to the 2^−ΔΔCt method. One-way analysis of variance (ANOVA) followed by the Tukey’s Honestly Significant Difference test were used for multiple comparisons (different numbers of “*” denoted by p < 0.05). All statistical analyses were performed using GraphPad Prism 8.0 software.
3 Results
3.1 ACP-associated virus and CLas screening with RT-PCR in individual insects
Using the RNA in adults of ACPs as a template and the primers listed in Table 1, the target gene fragments of six pathogens, DcRV, DcPLV, DcBV, DcDV, CLas, and DcBV2, and the internal reference gene β-Actin were successfully detected (Supplementary Figure S1). The blasting results in the NCBI database showed more than 98% similarity, indicating that the target fragments of each pathogen and internal reference gene were successfully amplified.
ACPs were collected from Guizhou, Guangdong, Guangxi, Sichuan, and Jiangxi in southern China to detect infection by CLas and 5 viruses in individual insects. As shown in Table 2, Figure 1, the detection rate of DcPLV was 55.35% in all ACP samples. In this study, 551 ACPs were collected, 305 of which had DcPLV. The detection rate of DcPLV was highest in ACPs in Guizhou and Guangdong, with detection rates of 69.44% and 66.39%, respectively. The detection rate of DcRV was also high in Guangdong and Sichuan ACPs (57.14% and 46.30%, respectively), while it was 28.49% in all samples. However, DcRV and DcDV were not detected in ACPs from Guizhou. DcBV had a slightly higher detection rate than DcBV2, both of which belong to Bunyavirales. In addition, the infection rate of CLas in all ACPs was 19.78%.
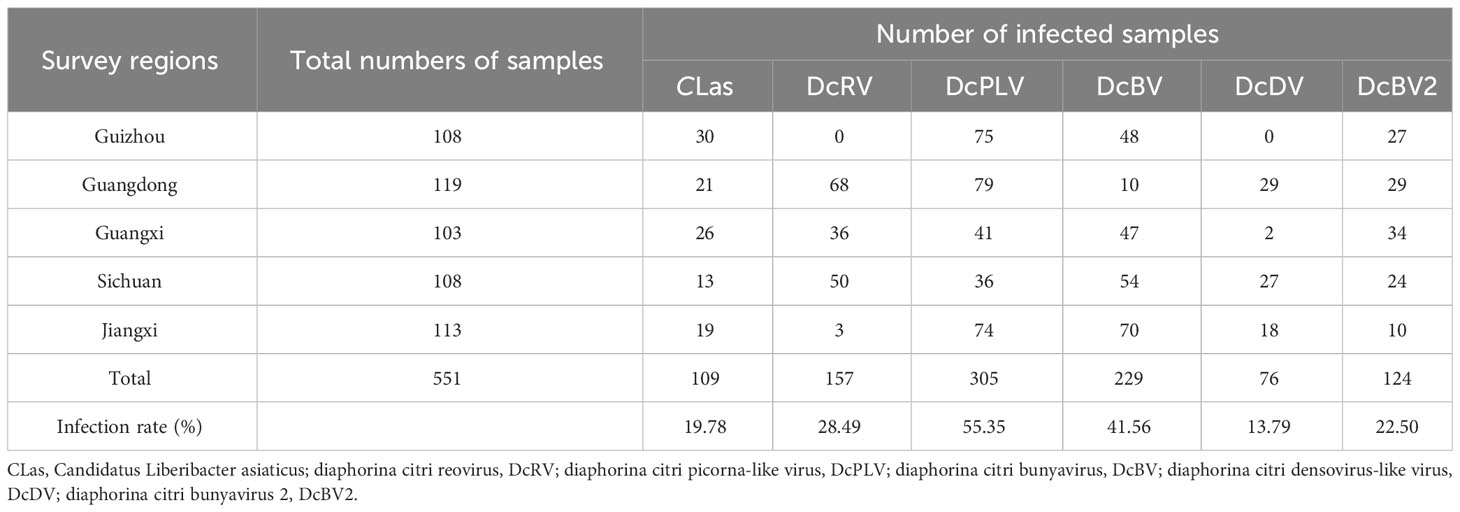
Table 2 Total number and detection percentages of CLas and Asian citrus psyllid (ACP)-associated viruses in various surveyed regions.
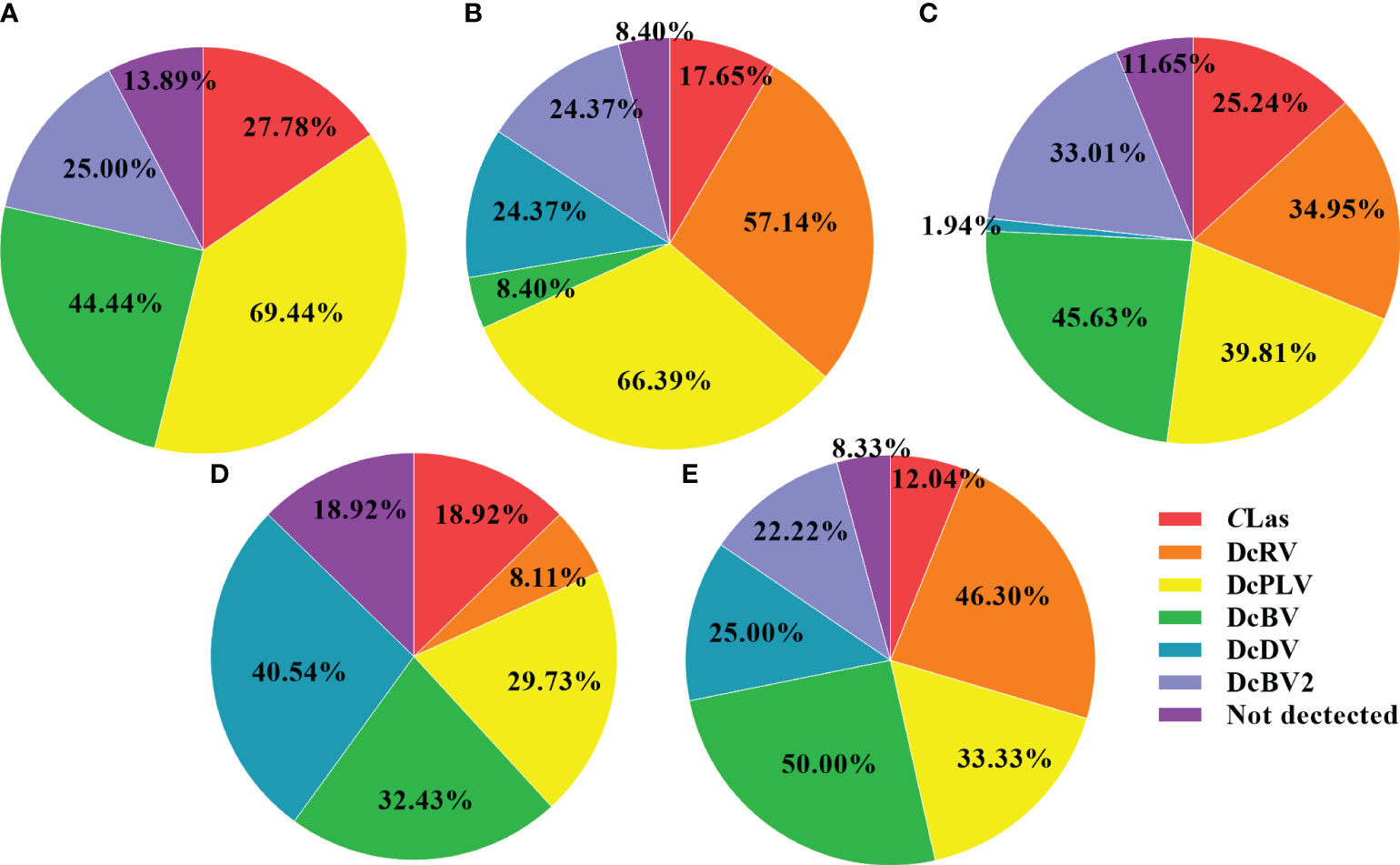
Figure 1 Pie chart showing the percentage of CLas and five viruses detected in different regions. (A), Guizhou; (B), Guangdong; (C), Guangxi; (D), Jiangxi; (E), Sichuan. Note that in the majority of the five regions of China, there were multiple samples that had multiple pathogens, therefore, most of the percentages in the pie charts do not equal 100%. CLas, Candidatus Liberibacter asiaticus; diaphorina citri reovirus, DcRV; diaphorina citri picorna-like virus, DcPLV; diaphorina citri bunyavirus, DcBV; diaphorina citri densovirus-like virus, DcDV; diaphorina citri bunyavirus 2, DcBV2.
3.2 Co-infection of CLas and 5 ACP-associated viruses in individual ACPs
Among all evaluated ACPs, 61.16% were co-infected with multiple symbionts, as shown in Table 3. Of the combinations of two symbionts co-infecting ACPs, DcPLV+DcBV had the highest co-infection rate, reaching 10.16% of all samples, followed by CLas+DcPLV with 4.17%. Among the co-infections involving three pathogens, DcRV+DcPLV+DcBV had the highest infection rate (3.45%), followed by CLas+DcPLV+DcBV (3.27%). In addition, the infection rate of ACPs infected with more than 4 pathogens was 4.36%.
Among 109 CLas-positive ACPs, 83.49% were co-infected with one or more ACP-associated virus (data not shown). The infection rates of DcRV, DcDV, DcBV and DcBV2 in CLas-positive samples were lower than those in CLas-free ACPs, especially for DcRV. The detection rate of DcRV was close to 50% lower in CLas-positive ACPs than in CLas-free ACPs (Figure 2). A similar result was seen for the detection of CLas in DcRV-infected ACP (Figure 3). No decrease in the detection of DcPLV was observed between CLas-positive and CLas-free ACPs (Figure 2). A similar result was observed for CLas detection in DcPLV- infected ACPs (Figure 3).
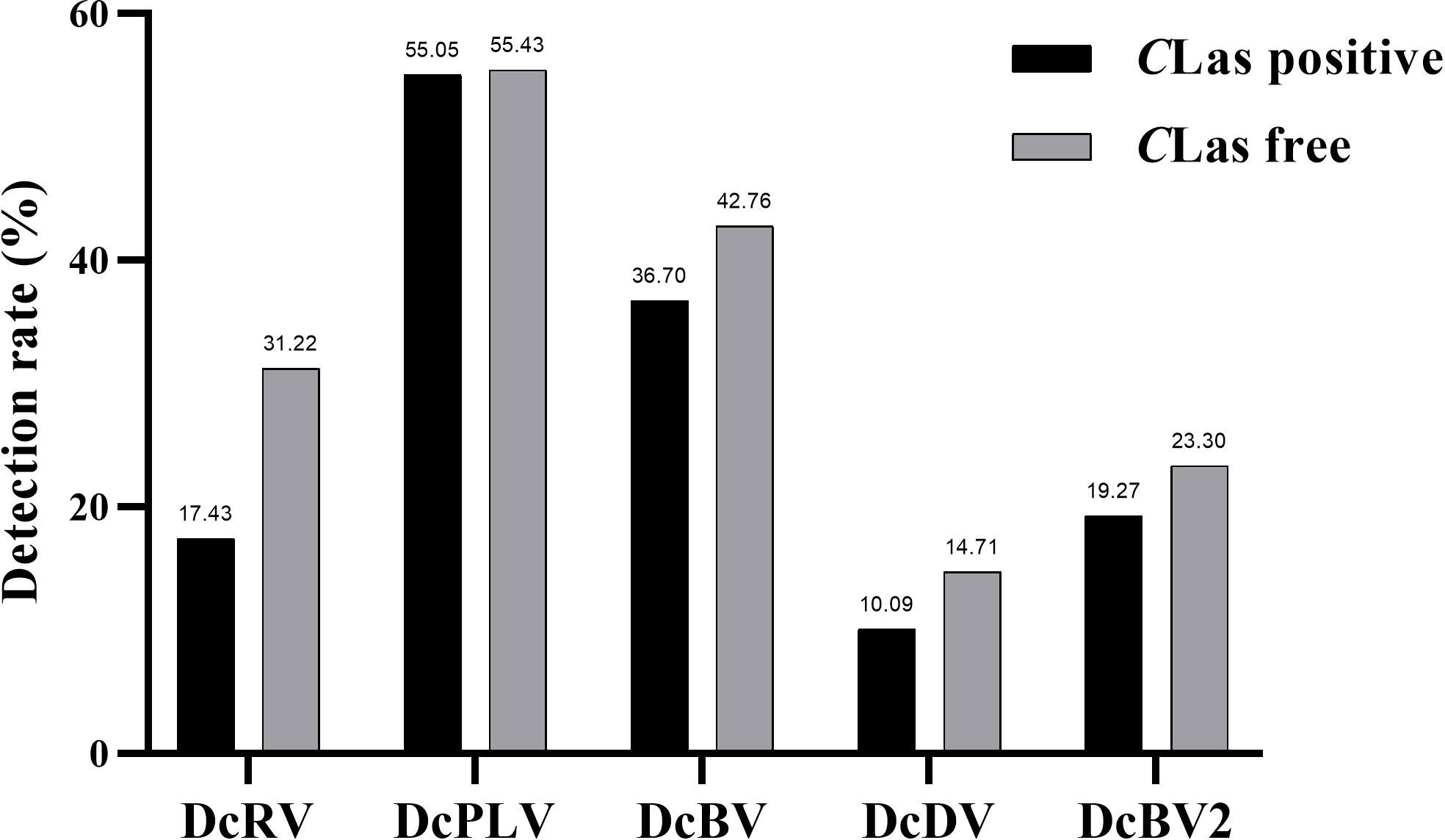
Figure 2 Detection rate of Asian citrus psyllid (ACP)-associated viruses in Candidatus Liberibacter asiaticus (CLas)-positive and CLas-free ACP samples. diaphorina citri reovirus, DcRV; diaphorina citri picorna-like virus, DcPLV; diaphorina citri bunyavirus, DcBV; diaphorina citri densovirus-like virus, DcDV; diaphorina citri bunyavirus 2, DcBV2.
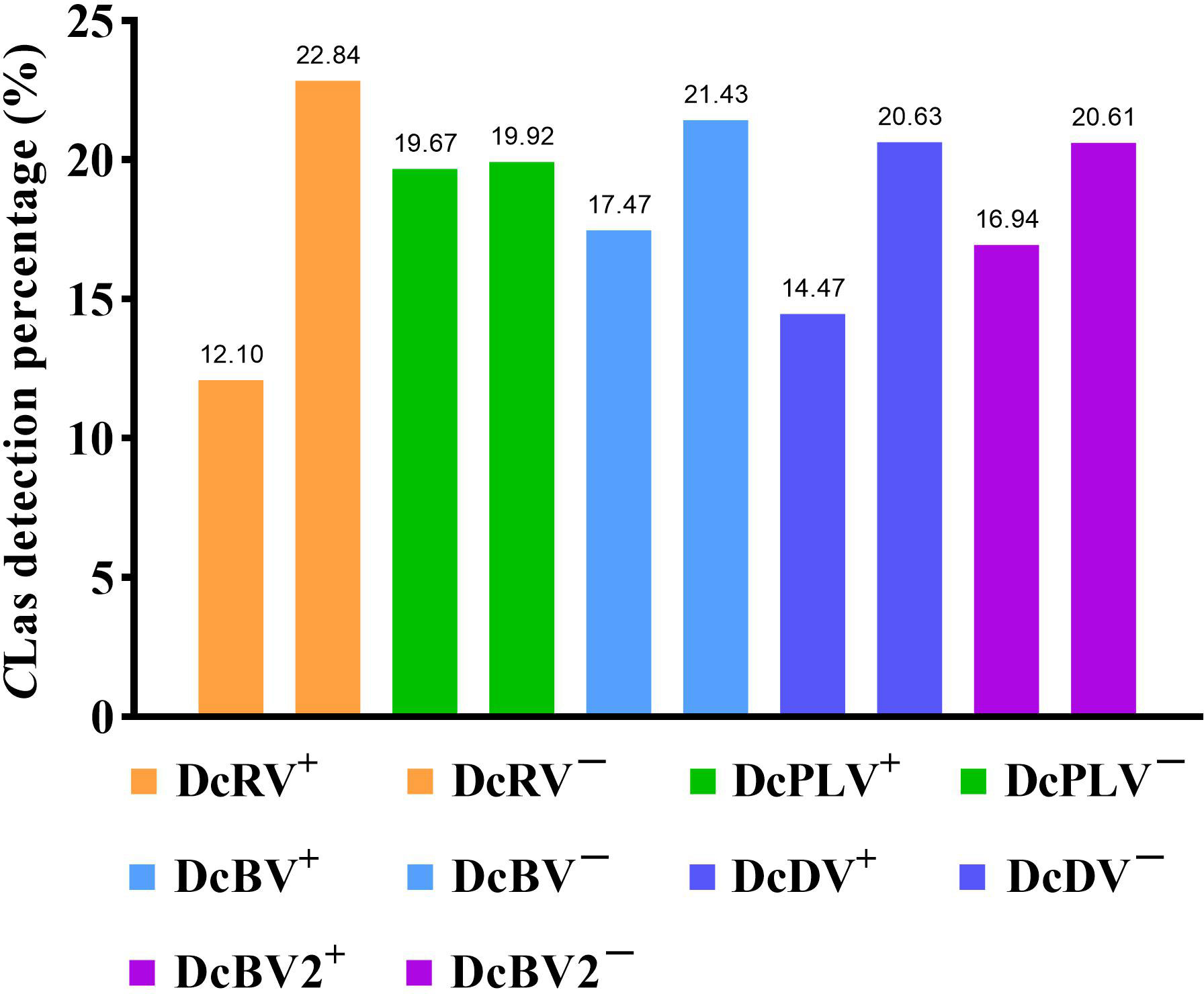
Figure 3 Detection percentage of Candidatus Liberibacter asiaticus (CLas) in Asian citrus psyllid (ACP)-associated virus-positive and virus-free ACP samples. +: ACP-associated virus-infected, −: ACP-associated virus-uninfected. diaphorina citri reovirus, DcRV; diaphorina citri picorna-like virus, DcPLV; diaphorina citri bunyavirus, DcBV; diaphorina citri densovirus-like virus, DcDV; diaphorina citri bunyavirus 2, DcBV2.
3.3 Distribution and transmission of DcPLV in ACPs
To understand the distribution of DcPLV in different organs of ACPs, we assessed the levels of DcPLV in the guts, testicles, ovaries, salivary glands, Malpighian tubules, and remnant tissues. RT-qPCR results showed that DcPLV was highest in salivary glands among the 5 tissues. In Malpighian tubules, we hardly detected DcPLV (Figure 4).
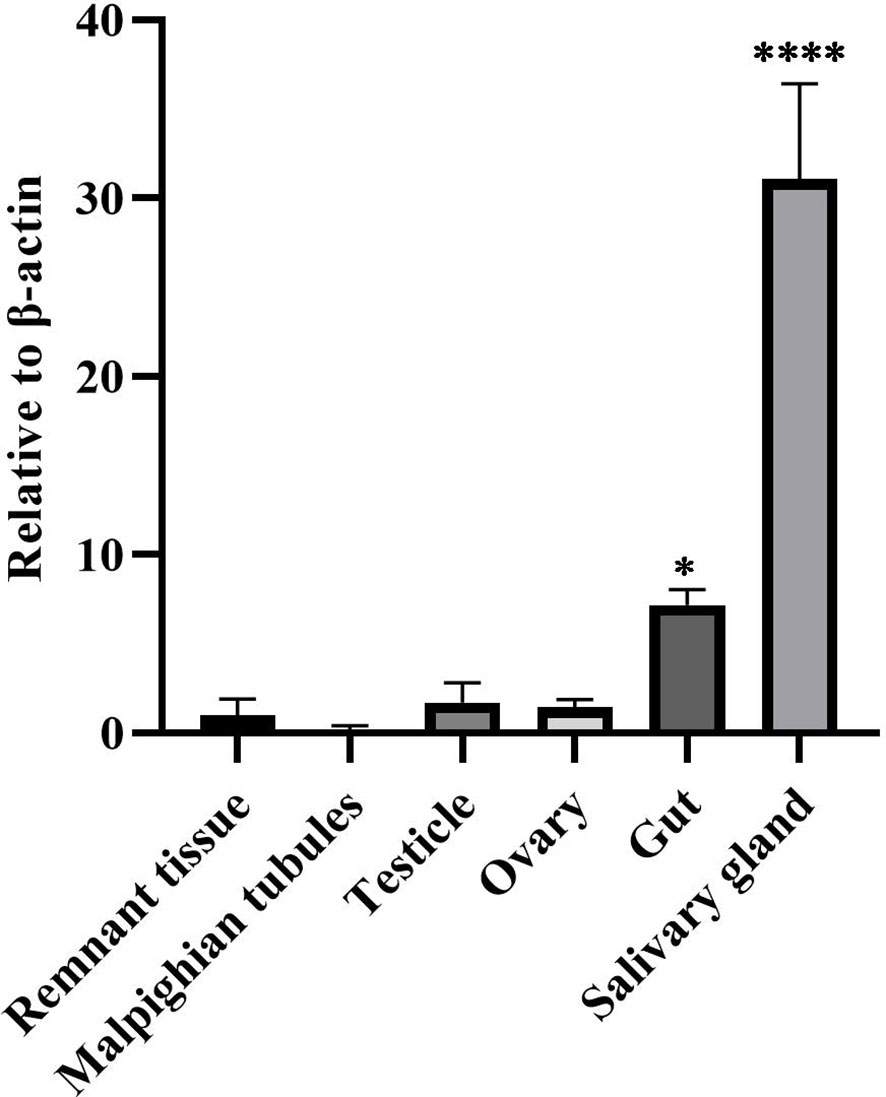
Figure 4 Relative contents of diaphorina citri picorna-like virus (DcPLV) in different tissues of Asian citrus psyllid (ACP). Data are means (± SE) of four biological replications per sample; β-Actin was used to normalize the expression of DcPLV as the reference gene. One-way ANOVA followed by the Tukey’s Honestly Significant Difference test were used for multiple comparisons (different numbers of “*” denoted by p < 0.05). The greater the number of *, the greater the variability.
We collected 56 offspring of ACPs, 22 of which carried DcPLV. The proportion of DcPLV-positive offspring was 39.29%. We also performed RNA testing on the leaves of orange jessamine that ACPs fed on and determined the presence of DcPLV. However, we did not identify DcPLV in the leaves that ACPs did not feed on at the same time as orange jessamine.
4 Discussion
HLB is a major threat to the citrus industry. Its vector, ACP, is also a key control target. ACPs not only carry CLas but are also hosts for many insect viruses. Prior to this study, Britt et al. (2020) investigated insect viruses in ACPs in Florida with five ACPs as a group sample and found that the most prevalent virus was DcACV. To understand the prevalence of some insect viruses and CLas in ACPs in China, this study collected ACP samples from the five main citrus-producing regions in China, Guizhou, Jiangxi, Guangdong, Guangxi, and Sichuan, and detected DcRV, DcPLV, DcBV, DcDV, CLas, and the newly discovered virus DcBV2 in individual ACPs using RT-PCR. DcPLV was the most prevalent virus in the collected ACP samples in China in this study. Regional differences may result in these population differences. In addition, we attempted to detect two previously identified viruses, DcACV and DcFLV. However, positive ACP samples were not found for these viruses. More samples may be needed to determine whether the two viruses are present. Chen et al. (2020) investigated the infection of ACPs with DcRV in Fuzhou, Fujian, China, and their incidence rate was 58-100%, similar to the detection rates of DcRV in Guangdong and Sichuan. However, the detection rates of DcRV in ACPs in other regions were relatively low. This suggests that the virus prevalence varies among countries and regions. To our knowledge, this is the first time that a comprehensive survey has been conducted in China to detect the prevalence of these viruses in individual ACPs. In addition, the detection rate of CLas was lowest in ACPs in Sichuan, at 12.04%, which is similar to the detection rate of CLas in citrus samples from Sichuan province (Cui et al., 2022).
Studies have shown that some insect viruses may affect host adaptation and reproduction (Vasilakis and Tesh, 2015). For instance, ABV-1 reduced the total aphid nymphal duration and induced reproduction (An et al., 2022). The aphid-lethal paralysis virus affects the movement and lifespan of Rhopalosiphum padi (Williamson et al., 1988). Zhang et al. (2022) found that crude extract containing seven endosymbiotic viruses, including DcRV and DcPLV, could significantly reduce the total egg production of female populations after injection into ACPs. However, whether the 5 viruses (DcRV, DcPLV, DcBV, DcDV, and DcBV2) play a role is not clear. In this study, DcPLV had the highest detection rate among the ACPs. DcPLV has been suggested as a new and unclassified picorna-like virus, although a phylogenetic tree generated based on the RdRp placed DcPLV close to Iflaviridae (Nouri et al., 2016). The picorna-like viruses, with a close taxonomic relationship to DcPLV, are also present in the wild lime psyllid and the potato psyllid (Stuehler et al., 2023). Because of this, we need to have some understanding of DcPLV. According to our survey, DcPLV is not only capable of propagating vertically but also has the potential to propagate horizontally. However, this does not mean that DcPLV can infect plants; it was not found in the leaves of the same plant not fed on by ACP. DcPLV was most prevalent in the salivary glands, indicating that it may spread horizontally. The picorna-like deformed wing virus has been associated with wing deformities in adult honeybees. It can infect the various developmental stages of bees (Mcmenamin and Flenniken, 2018). However, another picorna-like virus, helicoverpa armigera Nora virus (HaNV), has been shown to be efficiently horizontally transmitted between hosts via contaminated food and transmitted vertically from parent to offspring. Moreover, HaNV is not overtly pathogenic to its host (Yang et al., 2019). The high prevalence of DcPLV in China’s ACP populations indicates that it might be well adapted to ACPs. However, whether DcPLV can affect the growth habits of ACPs is unclear. It is unknown whether DcPLV has the potential to be used as a genetically engineered viral candidate to control insect vectors using RNA interference technology. Therefore, more studies on DcPLV as a virus vector are needed.
Previous studies have shown that the interaction between different pathogens in insect vectors may be antagonistic or cooperative (Domingo-Calap et al., 2020). The acquisition or transmission of one symbiont in insects can be affected by another symbiont. The Mal de Río Cuarto virus titer was reduced after its planthopper vector was co-infected with a wheat rhabdovirus (Dumon et al., 2018). Glaser and Meola (2010) suggested that the infection of Wolbachia in vector insects can induce resistance to the West Nile virus. The commensal bacterium Sulcia can interact with the Rice dwarf virus to co-localize to oocytes, helping the virus spread vertically through the egg to the offspring of leafhoppers (Wu et al., 2019). Moreover, CLas has been found to affect the endosymbiont abundance of ACPs using high-throughput metagenome sequencing technology (Pan et al., 2023). In the present study, CLas and four insect viruses (DcRV, DcDV, DcBV and DcBV2), especially DcRV, are prone to mutually reduced detection in the ACP population in the field. The number of ACPs co-infected with CLas and DcRV decreased by about 50% compared to those infected with only one pathogen, indicating that co-infection had a negative effect on the insect host. DcRV, a new species of the genus Fijivirus, is a persistent infection in its psyllid host and is distributed throughout the bodies of ACPs, including the gut and salivary glands (Chen et al., 2019). In addition, as CLas has a circulative–propagative transmission cycle in psyllids, CLas is associated with the gut, hemolymph, salivary glands, and fat bodies (Glaser and Meola, 2010; Ammar et al., 2011). CLas induces changes in different pathways including the insect’s metabolism and immune system (Kruse et al., 2017). Because of the similar distribution, CLas and DcRV could compete for resources within ACP, such as the metabolism of amino acids. CLas and DcRV could also alter similar host-immune responses. Our results were just analyzed based on infection from ACP in the field. More studies need to be performed on the specific change in CLas content with DcRV and other viruses in ACP under strict experimental control.
5 Conclusion
The infection rates of CLas and five insect viruses in ACP samples from five main citrus-producing regions in China were analyzed. DcPLV was the most prevalent and widespread ACP-associated virus. DcPLV was also demonstrated to propagate vertically and found more in salivary glands among different tissues. Approximately 60% of adult insect samples were co-infected with more than one insect pathogen. These results provide valuable information about the prevalence of ACP-associated viruses in China. In addition, analysis of the change in endosymbiont infection in a single insect vector is the basis for understanding the interaction between CLas, ACP, and insect viruses.
Data availability statement
The raw data supporting the conclusions of this article will be made available by the authors, without undue reservation.
Ethics statement
The manuscript presents research on animals that do not require ethical approval for their study.
Author contributions
LL: Data curation, Formal analysis, Investigation, Writing – original draft, Writing – review & editing. JC: Data curation, Investigation, Writing – review & editing. JJ: Investigation, Software, Writing – review & editing. JML: Data curation, Validation, Writing – review & editing. YS: Data curation, Validation, Writing – review & editing. QC: Data curation, Methodology, Writing – review & editing. FY: Data curation, Methodology, Writing – review & editing. ZB: Writing – review & editing. ZS: Conceptualization, Writing – review & editing. JXL: Project administration, Validation, Data curation, Conceptualization, Writing – original draft, Writing – review & editing.
Funding
The author(s) declare financial support was received for the research, authorship, and/or publication of this article. This research was supported by the National Key R&D Program of China (Grant No. 2021YFD1400800).
Acknowledgments
We thank Professor Y. J. Cen (South China Agricultural University), Q. D. Chen (Sichuan Academy of Agricultural Sciences), Professor B. H. Lou (Guangxi Academy of Specialty Crops), Tao Peng (Citrus Research Institute, SWU/CAAS), and Professor W. Hu (China School of Navel Orange, Gannan Normal University) for providing citrus psyllid samples. We also thank Professor M. J. Cao (Citrus Research Institute, SWU/CAAS) for helping sequence analysis of virus DcBV2.
Conflict of interest
The authors declare that the research was conducted in the absence of any commercial or financial relationships that could be construed as a potential conflict of interest.
Publisher’s note
All claims expressed in this article are solely those of the authors and do not necessarily represent those of their affiliated organizations, or those of the publisher, the editors and the reviewers. Any product that may be evaluated in this article, or claim that may be made by its manufacturer, is not guaranteed or endorsed by the publisher.
Supplementary material
The Supplementary Material for this article can be found online at: https://www.frontiersin.org/articles/10.3389/fpls.2024.1357163/full#supplementary-material
Supplementary Figure 1 | RT-PCR amplification and verification of 5 Asian citrus psyllid associated viruses, CLas and β-Actin by 1.2% agarose gel electrophoresis. CLas, Candidatus Liberibacter asiaticus; diaphorina citri reovirus, DcRV; diaphorina citri picorna-like virus, DcPLV; diaphorina citri bunyavirus, DcBV; diaphorina citri densovirus-like virus, DcDV; diaphorina citri bunyavirus 2, DcBV2.
References
Ammar, E., Shatters, J. R. G., Hall, D. G. (2011). Localization of candidatus liberibacter asiaticus, associated with citrus huanglongbing disease, in its psyllid vector using fluorescence in situ hybridization. J. Phytopathol. 159, 726–734. doi: 10.1111/j.1439-0434.2011.01836.x
An, X., Zhang, W., Ye, C., Smagghe, G., Wang, J. J., Niu, J. (2022). Discovery of a widespread presence bunyavirus that may have symbiont-like relationships with different species of aphids. Insect Sci. 29, 1120–1134. doi: 10.1111/1744-7917.12989
Atta, A. A., Morgan, K. T., Hamido, S. A., Kadyampakeni, D. M., Mahmoud, K. A. (2020). Water and soil nutrient dynamics of huanglongbing-affected citrus trees as impacted by ground-applied nutrients. Agronomy 10, 1485. doi: 10.3390/AGRONOMY10101485
Bolling, B. G., Weaver, S. C., Tesh, R. B., Vasilakis, N. (2015). Insect-specific virus discovery: significance for the arbovirus community. Viruses 7, 4911–4928. doi: 10.3390/v7092851
Britt, K., Gebben, S., Levy, A., Al Rwahnih, M., Batuman, O. (2020). The detection and surveillance of Asian citrus psyllid (Diaphorina citri)—associated viruses in Florida citrus groves. Front. Plant Sci. 10. doi: 10.3389/fpls.2019.01687
Chen, Q., Godfrey, K., Liu, J., Mao, Q., Kuo, Y., Falk, B. W. (2019). A nonstructural protein responsible for viral spread of a novel insect reovirus provides a safe channel for biparental virus transmission to progeny. J. Virol. 15 (93), e00702-19. doi: 10.1128/JVI.00702-19
Chen, Q., Nouri, S., Zhang, Y., Erickson, A., Falk, B. W. (2020). Diaphorina citri reovirus is most closely related to Fijiviruses. Virology 547, 20–26. doi: 10.1016/j.virol.2020.04.012
Chinyukwi, T., Kadyampakeni, D. M., Rossi, L. (2023). Optimization of macronutrient and micronutrient concentrations in roots and leaves for Florida HLB-affected sweet orange trees. J. Plant Nutr. 47, 226–239. doi: 10.1080/01904167.2023.2275068
Cui, X., Liu, K., Huang, J., Fu, S., Chen, Q., Liu, X., et al. (2022). Population diversity of 'candidatus liberibacter asiaticus' and diaphorina citri in Sichuan: a case study for huanglongbing monitoring and interception. Plant Dis. 106, 1632–1638. doi: 10.1094/PDIS-07-21-1539-RE
Domingo-Calap, M. L., Moreno, A. B., Diaz, P. J., Moreno, A., Fereres, A., Lopez-Moya, J. J. (2020). Assessing the impact on virus transmission and insect vector behavior of a viral mixed infection in melon. Phytopathology 110, 174–186. doi: 10.1094/PHYTO-04-19-0126-FI
Dumon, A. D., Arguello, C. E., Mattio, M. F., Alemandri, V., Del, V. M., Truol, G. (2018). Co-infection with a wheat rhabdovirus causes a reduction in mal de Río Cuarto virus titer in its planthopper vector. B. Entomol. Res. 108, 232–240. doi: 10.1017/S0007485317000803
Fagen, J. R., Giongo, A., Brown, C. T., Davis-Richardson, A. G., Gano, K. A., Triplett, E. W. (2012). Characterization of the relative abundance of the citrus pathogen candidatus Liberibacter asiaticus in the microbiome of its insect vector, diaphorina citri, using high throughput 16s rRNA sequencing. Open Microbiol. J. 6, 29–33. doi: 10.2174/1874285801206010029
Ghosh, D., Kokane, S., Savita, B. K., Kumar, P., Sharma, A. K., Ozcan, A., et al. (2022). Huanglongbing pandemic: current challenges and emerging management strategies. Plants (Basel Switzerland) 12, 160. doi: 10.3390/plants12010160
Glaser, R. L., Meola, M. A. (2010). The native Wolbachia endosymbionts of drosophila melanogaster and culex quinquefasciatus increase host resistance to West Nile virus infection. PloS One 5, e11977. doi: 10.1371/journal.pone.0011977
Jagoueix, S., Bove, J. M., Garnier, M. (1994). The phloem-limited bacterium of greening disease of citrus is a member of the alpha subdivision of the Proteobacteria. Int. J. @ Syst. Bacteriol 44, 379–386. doi: 10.1099/00207713-44-3-379
Jia, D., Mao, Q., Chen, Y., Liu, Y., Chen, Q., Wu, W., et al. (2017). Insect symbiotic bacteria harbour viral pathogens for transovarial transmission. Nat. Microbiol. 2, 17025. doi: 10.1038/nmicrobiol.2017.25
Kruse, A., Fattah-Hosseini, S., Saha, S., Johnson, R., Warwick, E., Sturgeon, K., et al. (2017). Combining 'omics and microscopy to visualize interactions between the Asian citrus psyllid vector and the huanglongbing pathogen candidatus liberibacter asiaticus in the insect gut. PloS One 12, e179531. doi: 10.1371/journal.pone.0179531
Leong, S. S., Leong, S. C. T., Beattie, G. A. C. (2022). Integrated pest management strategies for Asian citrus psyllid Diaphorina citri Kuwayama (Hemiptera: Psyllidae) and huanglongbing in citrus for Sarawak, East Malaysia, Borneo. Insects 13, 960. doi: 10.3390/insects13100960
Liu, K., He, J., Guan, Z., Zhong, M., Pang, R., et al. (2020). Transcriptomic and metabolomic analyses of diaphorina citri kuwayama infected and non-infected with candidatus liberibacter asiaticus. Front. Physiol. 11, 630037. doi: 10.3389/fphys.2020.630037
Marklewitz, M., Zirkel, F., Kurth, A., Drosten, C., Junglen, S. (2015). Evolutionary and phenotypic analysis of live virus isolates suggests arthropod origin of a pathogenic RNA virus family. Proc. Natl. Acad. Sci. 112, 7536–7541. doi: 10.1073/pnas.1502036112
Marutani-Hert, M., Hunter, W. B., Katsar, C. S., Sinisterra, X. H., Hall, D. G., Powell, C. A. (2009). Reovirus-like sequences isolated from adult Asian citrus psyllid, (Hemiptera: Psyllidae: Diaphorina citri). Florida entomologist 92, 314–320. doi: 10.1653/024.092.0216
Mcmenamin, A. J., Flenniken, M. L. (2018). Recently identified bee viruses and their impact on bee pollinators. Curr. Opin. Insect Sci. 26, 120–129. doi: 10.1016/j.cois.2018.02.009
Nouri, S., Salem, N., Nigg, J. C., Falk, B. W. (2016). Diverse array of new viral sequences identified in worldwide populations of the Asian citrus psyllid (diaphorina citri) using viral metagenomics. J. Virol. 90, 2434–2445. doi: 10.1128/JVI.02793-15
Pan, Q., Yu, S. J., Lei, S., Li, S. C., Ding, L. L., Liu, L., et al. (2023). Effects of candidatus liberibacter asiaticus infection on metagenome of diaphorina citri gut endosymbiont. Sci. Data 10, 478. doi: 10.1038/s41597-023-02345-2
Simmonds, P., Adams, M. J., Benkő, M., Breitbart, M., Brister, J. R., Carstens, E. B., et al. (2017). Consensus statement: virus taxonomy in the age of metagenomics. Nat. Rev. Microbiol. 15, 161–168. doi: 10.1038/nrmicro.2016.177
Snyder, J., Dickens, K. L., Halbert, S. E., Dowling, S., Russell, D., Henderson, R., et al. (2022). The development and evaluation of insect traps for the Asian citrus psyllid, diaphorina citri (Hemiptera: Psyllidae), vector of citrus huanglongbing. Insects 13, 295. doi: 10.3390/insects13030295
Stuehler, D. S. J. R., Hunter, W. B., Carrillo-Tarazona, Y., Espitia, H., Cicero, J. M., Bell, T., et al. (2023). Wild lime psyllid Leuronota fagarae Burckhardt (Hemiptera: Psylloidea) picorna-like virus full genome annotation and classification. J. Invertebr. Pathol. 201, 107995. doi: 10.1016/j.jip.2023.107995
Teixeira, L., Ferreira, A., Ashburner, M. (2008). The bacterial symbiont Wolbachia induces resistance to RNA viral infections in drosophila melanogaster. PloS Biol. 6, e2. doi: 10.1371/journal.pbio.1000002
Vasilakis, N., Tesh, R. B. (2015). Insect-specific viruses and their potential impact on arbovirus transmission. Curr. Opin. Virol. 15, 69–74. doi: 10.1016/j.coviro.2015.08.007
Vyas, M., Fisher, T. W., He, R., Nelson, W., Yin, G., Cicero, J. M., et al. (2015). Asian citrus psyllid expression profiles suggest candidatus liberibacter asiaticus-mediated alteration of adult nutrition and metabolism, and of nymphal development and immunity. PloS One 10, e130328. doi: 10.1371/journal.pone.0130328
Weitz, J. S., Wilhelm, S. W. (2012). Ocean viruses and their effects on microbial communities and biogeochemical cycles. F1000 Biol. Rep. 4, 17. doi: 10.3410/B4-17
Williamson, C., Rybicki, E. P., Kasdorf, G., Vonwechmar, M. B. (1988). Characterization of a new picorna-like virus isolated from aphids. J. Gen. Virol. 69, 787–795. doi: 10.1099/0022-1317-69-4-787
Wu, W., Huang, L., Mao, Q., Wei, J., Li, J., Zhao, Y., et al. (2019). Interaction of viral pathogen with porin channels on the outer membrane of insect bacterial symbionts mediates their joint transovarial transmission. Philos. Trans. R. Soc. London. Ser. B Biol. Sci. 374, 20180320. doi: 10.1098/rstb.2018.0320
Yang, X., Xu, P., Yuan, H., Graham, R. I., Wilson, K., Wu, K. (2019). Discovery and characterization of a novel picorna-like RNA virus in the cotton bollworm helicoverpa armigera. J. Invertebr. Pathol. 160, 1–7. doi: 10.1016/j.jip.2018.11.003
Zhang, Y., Li, Z., Chen, Q. (2022). Effect of seven viral endosymbionts on the fecundity of diaphorina citri. J. biosafety 31, 163–170. doi: 10.3969/j.issn.2095-1787.2022.02.011
Zhou, C. (2018). Reconsideration on the control strategy of citrus huanglongbing. Plant Prot. 44, 30–33. doi: 10.16688/j.zwbh.2018293
Keywords: Asian citrus psyllid, Candidatus Liberibacter asiaticus, insect viruses, Huanglongbing, co-infection
Citation: Liu L, Chen J, Jiang J, Liang J, Song Y, Chen Q, Yan F, Bai Z, Song Z and Liu J (2024) Detection of Candidatus Liberibacter asiaticus and five viruses in individual Asian citrus psyllid in China. Front. Plant Sci. 15:1357163. doi: 10.3389/fpls.2024.1357163
Received: 17 December 2023; Accepted: 18 January 2024;
Published: 06 February 2024.
Edited by:
Antonio Figueira, University of São Paulo, BrazilReviewed by:
Ved Prakash, Kansas State University, United StatesTong Zhang, South China Agricultural University, China
Copyright © 2024 Liu, Chen, Jiang, Liang, Song, Chen, Yan, Bai, Song and Liu. This is an open-access article distributed under the terms of the Creative Commons Attribution License (CC BY). The use, distribution or reproduction in other forums is permitted, provided the original author(s) and the copyright owner(s) are credited and that the original publication in this journal is cited, in accordance with accepted academic practice. No use, distribution or reproduction is permitted which does not comply with these terms.
*Correspondence: Jinxiang Liu, Liujinxiang@cric.cn