- Louvain Institute of Biomolecular Science and Technology (LIBST), UCLouvain, Louvain-la-Neuve, Belgium
Several recombinant proteins have been successfully produced in plants. This usually requires Agrobacterium-mediated cell transformation to deliver the T-DNA into the nucleus of plant cells. However, some genetic instability may threaten the integrity of the expression cassette during its delivery via A. tumefaciens, especially when the protein of interest is toxic to the bacteria. In particular, we found that a Tn3 transposon can be transferred from the pAL4404 Ti plasmid of A. tumefaciens LBA4404 into the expression cassette when using the widely adopted 35S promoter, thereby damaging T-DNA and preventing correct expression of the gene of interest in Nicotiana tabacum BY-2 suspension cells.
1 Introduction
The production of recombinant proteins using transient and stable expression in plants has become an alternative to more conventional animal cell line platforms (Schillberg and Spiegel, 2022). Also, expression in plants offers the possibility for metabolic engineering of plant bioactive pathways (Reed and Osbourn, 2018). Agrobacterium tumefaciens-mediated transformation is the method of choice to deliver the genetic material into plants. The gene(s) of interest under the control of suitable promoter and terminator sequences is inserted between the left and right borders delineating the T-DNA in a binary vector. Generally, the 35S promoter from Cauliflower mosaic virus (CaMV), and its derivatives like the enhanced CaMV 35S promoter consisting of a 250 bp tandem duplication of sequence upstream of the core promoter, is preferred to direct constitutive expression of transgenes in both stable and transient expression systems (Amack and Antunes, 2020). The 35S promoter is active in a large number of plant species, both dicots and monocots. However, it is important to bear in mind that this promoter is also active in Escherichia coli (Assaad and Signer, 1990) and A. tumefaciens (Ohta et al., 1990; Vancanneyt et al., 1990), which can be of concern when the gene to be expressed is toxic to the bacteria. Indeed, in this case, the binary plasmid is generally mutated or rearranged to prevent the expression of the toxic protein. The toxic effect on the host bacteria can be reduced by lowering the culture temperature. In addition, plant introns have been previously proposed to stabilize plasmids in bacteria (Johansen, 1996).
Here, we examined the fate of two expression cassettes corresponding to a viral envelope glycoprotein (gP) when transferred into A. tumefaciens and delivered into BY-2 cells. We found that a replicative DNA transposon was integrated within Agrobacterium LBA4404 into the T-DNA when the gP coding sequence was under the control of p35S, but not if a plant-specific promoter was preferred.
2 Method
2.1 Construction of the genes and vectors
To design the binary vectors, the gP expression cassettes were constructed using the Golden Gate Modular Cloning (MoClo) assembly method (Weber et al., 2011) and DNA parts from the MoClo Plant toolbox (Engler et al., 2014). Oligonucleotides encoding the Medicago sativa protein disulfide isomerase (PDI) signal peptide flanked by BpiI recognition and restriction sites (PDIforward/PDIreverse; Table S1) were hybridized and cloned into the pICH41258 level 0 acceptor plasmid. Oligonucleotides encoding a 6xHis tag flanked by BpiI recognition and restriction sites (Histagforward/Histagreverse; Table S1) were hybridized and cloned into the pAGM1301 level 0 acceptor plasmid. The promoter of plasma membrane ATPase 4 gene from Nicotiana plumbaginifolia, reinforced with two CaMV35S enhancers (De Muynck et al., 2009), contained four BpiI recognition sites, which had to be removed to avoid hampering the efficacy of subsequent Golden Gate reactions. Appropriate mutations were therefore introduced by PCR using three primer sets (PMA1/PMA2, PMA3/PMA4, PMA5/6; Table S1). The three fragments were flanked by BpiI recognition and restriction sites, enabling them to be reassembled in the pICH41295 level 0 acceptor plasmid. The sequence of the gP ectodomain (Ala21-Gly419) optimized for plant expression was first amplified by PCR with the primer set gPforwardnoSP/gPreverse (Table S1) and cloned into pGEMT-Easy vector. The sequence of the glycoprotein cannot currently be disclosed for intellectual property reasons. The sequence of the Saccharomyces cerevisiae PMP1 terminator was amplified by PCR as a 0.6 kb fragment using the primer set tPMP1forward/tPMP1reverse (Table S1) and a pEMBL12- vector containing the PMP1 gene (Navarre et al., 1994), and cloned into the pGEMT-Easy vector. The pGEMT-Easy vectors were sequenced and further used as level 0 Golden Gate modules.
By a Golden Gate reaction, the plasmids containing the enhanced 35S promoter (a tandem duplication of 327 bp sequence upstream of the core promoter (-90 region) + 5’ untranslated Ω leader (pICH51288), the sequences encoding the PDI signal peptide, gP and the 6xHis-tag, and the PMP1 terminator were digested with BsaI and then assembled into the level 1 acceptor position 2 Golden Gate pICH47742. Alternatively, the plasmids containing the PMA4 promoter, the sequences encoding the PDI signal peptide, gP and the 6xHis-tag, and the PMP1 terminator were digested with BsaI and then assembled into the level 1 acceptor position 2 Golden Gate pICH47742.
N. tabacum RB7 SAR genetic insulator was PCR amplified from the pPZP-nptII-mcherry-gB-gB-SAR binary vector (Herman et al., 2023) using primers flanked by BpiI recognition and restriction sites (SARforward/SARreverse; Table S1). The PCR fragment was then cloned into pICH41331. By Golden Gate reaction, the SAR sequence was digested with BsaI and ligated into the level 1 acceptor plasmid level 3 pICH47751. The hptI hygromycin resistance gene under the control of the NOS promoter and terminator was PCR amplified with primers flanked by BpiI recognition and restriction sites (hptIforward/hptIreverse; Table S1) from the pPZP-hptI-HIgG1-LoBM2 binary vector (Magy et al., 2014) and then cloned into the level 1 position 1 vector pICH47732. A nptII kanamycin resistance cassette under the control of the NOS promoter and the OCS terminator (pICSL70004) was transferred into the level 1 acceptor position 1 pICH47732 by Golden Gate with BsaI.
The two gP expression cassettes (p35S-PDI-gP and PMA4-PDI-gP) were moved by Golden Gate reaction to a level M multigene vector containing a spectinomycin resistance gene by digestion with BpiI followed by ligation in the acceptor vector pAGM831 (PMA4-PDI-gP) or pAGM8043 (p35S-PDI-gP) (Figure 1). For the selection of stable BY-2 transformants, the cassette containing the hygromycin phosphotransferase (hpt) gene or the neomycin phosphotransferase (nptII) gene was added in position 1. For the PMA4-PDI-gP construct, the SAR cassette was also added in position 3. Finally, to close the level M acceptors, the level M end-link 2 (pICH50881) was used for p35S-PDI-gP construct whereas the level M end-link 3 (pICH50892) was used for PMA4-PDI-gP construct.
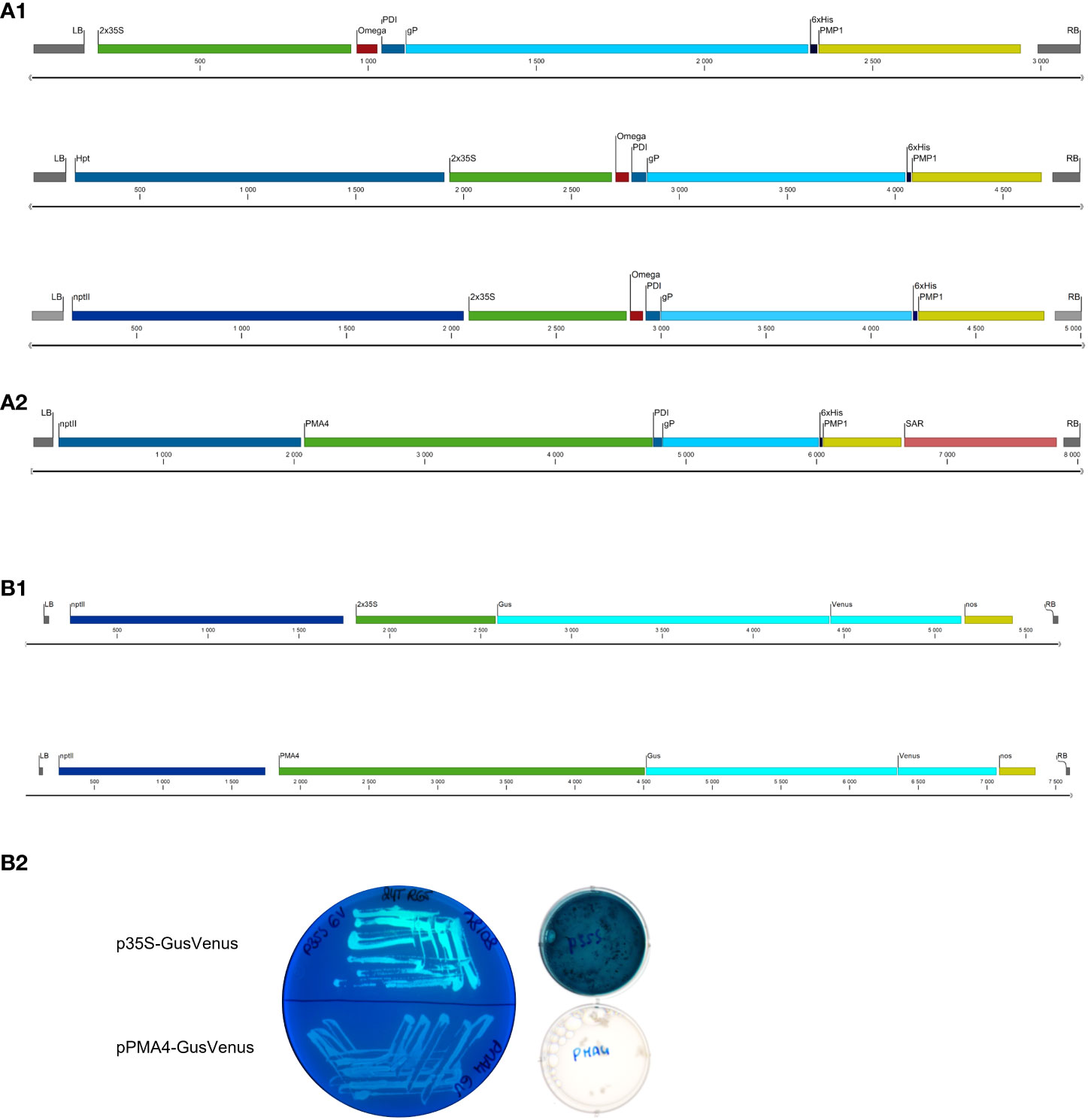
Figure 1 Schematic representation of the expression cassettes within the T-DNA in the binary plasmids used. (A) gP expression cassettes (A1) p35S-SPPDI-gP-6His-tPMP1. (A2) pPMA4-PDI-gP-6His-tPMP1 (B) GusVenus expression cassettes (B1) p35S-GusVenus-tnos; pPMA4-GusVenus-tnos (B2) Venus fluorescence and Gus staining in Agrobacterium cells.
The binary plasmids pPZP-nptII-pPMA4-GusVenus and pPZP-nptII-p35S-GusVenus in which the GusPlusVenus bi-functional reporter construct was either under the control of the enhanced PMA4 promoter of N. plumbaginifolia or the enhanced 35S promoter (a tandem duplication of 327 bp sequence upstream of the core promoter (-90 region) + the 35S core promoter (-90,+1)) were already available in our lab (Figure 1B1) (Navarre et al., 2011).
2.2 Transformation of Agrobacterium tumefaciens
The binary vectors corresponding to gP expression cassettes were transferred into the electrocompetent A. tumefaciens LBA4404 virG strain (van der Fits et al., 2000) or GV3101::pMP90, and selected on 2YT medium (16 g/L Bacto-tryptone, 10 g/L Bacto Yeast Extract, 5 g/L NaCl, 2 g/L Glucose, 0.4 g/L MgSO4) containing rifampicin, gentamicin and spectinomycin (50 µg/mL). Extraction and purification of plasmids from A. tumefaciens were carried out using the SMARTPURE SK-PLPU-100 kit (Kaneka Eurogentec, Seraing, Belgium). The binary plasmids purified from A. tumefaciens transformants were re-transformed into E. coli TOP10 cells, selected for spectinomycin resistance and sequenced.
Both vectors containing to GusVenus cassette were transferred into the electrocompetent A. tumefaciens LBA4404 virG strain. Detection of Venus expression was visualized with an Amersham Imager 600 using a light source and the Cy2 (480 nm) and CY3 (520 nm) filters. For Gus in vivo staining, late logarithmic Agrobacterium cultures were stained for 1 h with 2 mM of the indigogenic substrate X-Gluc (5-bromo-4-chloro-3-indolyl beta-D-glucuronic acid cyclohexylammonium) as reported by (Vancanneyt et al., 1990).
2.3 Transformation of BY-2 cells
The XylT/FucT KO BY-2 cell line, knocked out for genes encoding β(1,2)-xylosyltransferases and α(1,3)fucosyltransferases (Mercx et al., 2017), was used to generate transgenic cell lines expressing gP via A. tumefaciens LBA4404VirG-mediated stable transformation of BY-2 cells as described (Navarre and Chaumont, 2022). Biolistic particle delivery in BY-2 cells was carried out with a Biolistic PDS1000/He device (Bio-Rad, Hercules, CA, USA) as previously described (Herman et al., 2021).
2.4 Western blotting analysis of proteins
Samples of total soluble proteins (TSP) were analyzed by SDS-PAGE (pre-casted 4–20% polyacrylamide, Kaneka Eurogentec, Seraing, Belgium) after denaturation for 5 min at 100°C in Laemmli buffer containing 0.1 M DTT, and transferred onto a PVDF membrane (Bio-Rad, Trans-Blot Turbo, 1704156, Hercules, CA, USA). The PVDF membrane was incubated with a rabbit polyclonal antibody against gP (proprietary antibodies, 1/1,000) followed by anti-rabbit, alkaline phosphatase (AP)-coupled polyclonal antibodies (Sigma-Aldrich, A3687, St Louis, MO, USA, 1/10,000). Western blots were revealed with BM Purple AP substrate, precipitating (Roche, 11442074001, Basel, Switzerland).
3 Results
The gP expression cassette was constructed by assembling the sequences encoding the signal peptide of the M. sativa protein disulfide isomerase (PDI), the gP ectodomain, and the C-terminal 6-His tag, under the control of the 2x35S promoter fused to the 5’ untranslated Ω-leader of the CaMV, and the terminator of the yeast PMP1 gene (Figure 1A1). While the binary plasmid containing this p35S-PDI-gP construct was correctly obtained in E. coli, A. tumefaciens LBA4404 transformation led to unexpected digestion profiles that varied according to the transformant. This likely indicated that the binary plasmid containing the gP cassette was altered in A. tumefaciens LBA4404. The binary plasmids purified from eight different A. tumefaciens LBA4404 transformants (coming from two independent electrocompetent Agrobacterium batches) were retransformed in E. coli, analyzed by restriction analysis (Figure 2A), and sequenced. Sequencing with LB and internal primers showed that the insertion of an additional DNA fragment occurred within the gP cassette (Figure 2B).
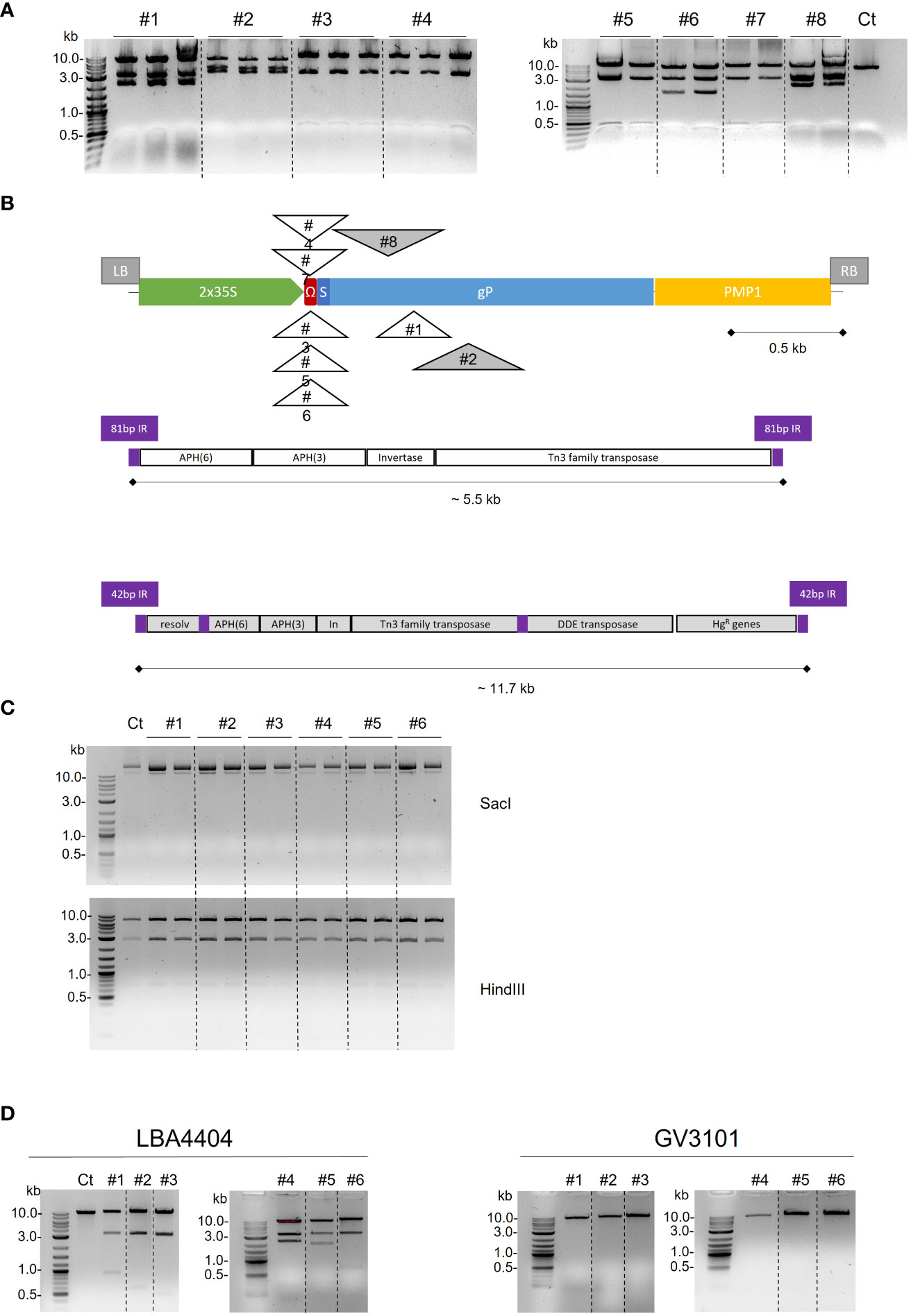
Figure 2 Alteration of binary plasmid p35S-gP T-DNA after A. tumefaciens passage. (A) Electrophoretic analysis of the p35S-SPPDI-gP-6His-tPMP1 plasmid purified from eight A. tumefaciens LBA4404 transformants, retransformed in E. coli and digested with SacI. A control plasmid without any passage in Agrobacterium is used as a control (Ct). (B) Schematic diagram of transposon elements insertions in T-DNA of plasmid p35S-SPPDI-gP-6His-tPMP1 after passage in A. tumefaciens LBA4404. Both orientations of Tn3 are represented above or below the T-DNA. Genes within the 5.5 kb and 11.7 kb transposon elements are detailed. (C) Electrophoretic analysis of the nptII-pPMA4-SPPDI-gP-6His-tPMP1 plasmid purified from six A. tumefaciens LBA4404 transformants, retransformed in E. coli and digested with SacI or HindIII. A control plasmid without any passage in Agrobacterium is used as a control (Ct). (D) Electrophoretic analysis of the nptII-p35S-SPPDI-gP-6His-tPMP1 plasmid purified from six A. tumefaciens LBA4404 or six A. tumefaciens GV3101 transformants, retransformed in E. coli and digested with SacI. A control plasmid without any passage in Agrobacterium is used as a control (Ct).
For six out of the eight sequences, the left and right ends of the inserted DNA matched with two regions comprised between positions 1,066 and 6,534 of the 140-kb-long pAL4404 tumor inducing plasmid (pTi) of the A. tumefaciens LBA4404 strain (accession number KY000037.1) (Figure 2B). Analysis of the published pAL4404 annotations showed that this 5.5-kb long sequence is bordered by 81 bp inverted repeats and encodes two aminoglycoside 3’-O-phosphotransferases (APH(6)-Id and APH(3’)-Ib), a transposon DNA invertase and a transposase of the Tn3 transposon family (Figure 2B). This sequence corresponds to the Tn904 transposon. In five cases out of six, the Tn904 transposon was inserted within the 5’ Ω-leader and, in the sixth case, the insertion occurred within the gP sequence (Thr171).
The last two sequences showed different borders that also matched with two regions comprised between positions 1 and 11,727 of pAL4404 (Figure 2B). This 11.7 kb sequence is flanked by 42 bp inverted repeats and contains the same 5.5 kb sequence that was detected in the other six sequences and identified as the Tn904 transposon, as well as mercury resistance genes and additional transposases. Both insertions occurred within the gP sequence (Val159 or Ile211).
Altogether, our findings indicated that the binary plasmid containing the cassette encoding the gP ectodomain under the control of the 35S promoter was altered in A. tumefaciens LBA4404 possibly to prevent its expression. To confirm the activity of 35S promoter in A. tumefaciens LBA4404, overnight cultures of the strain containing the gene encoding the GusVenus bi-functional reporter under the control of the 2x35S promoter were incubated with X-gluc, a substrate for Gus, or checked for Venus fluorescence (Figure 1B2). As a control, A. tumefaciens containing the GusVenus bi-functional reporter gene under the control of the strong constitutive PM4 promoter from N. plumbaginifolia showed no activity in the same conditions.
We therefore used biolistics to obtain stable BY-2 transformed cells without going through an Agrobacterium co-cultivation step. The binary plasmid containing the PDI-gP expression cassette as well as a hygromycin resistance marker (Figure 1A) was coated on golden particles and delivered into BY-2 cells by high-speed particle bombardment. A transgenic cell line selected on hygromycin-supplemented growth medium was analyzed by Western blotting using polyclonal anti-gP antibodies. Compared to the control BY-2 cell line producing the gB ectodomain from human cytomegalovirus (Herman et al., 2023), an additional band at 50 kDa was detected, corresponding to the predicted size of gP (Figure 3A).
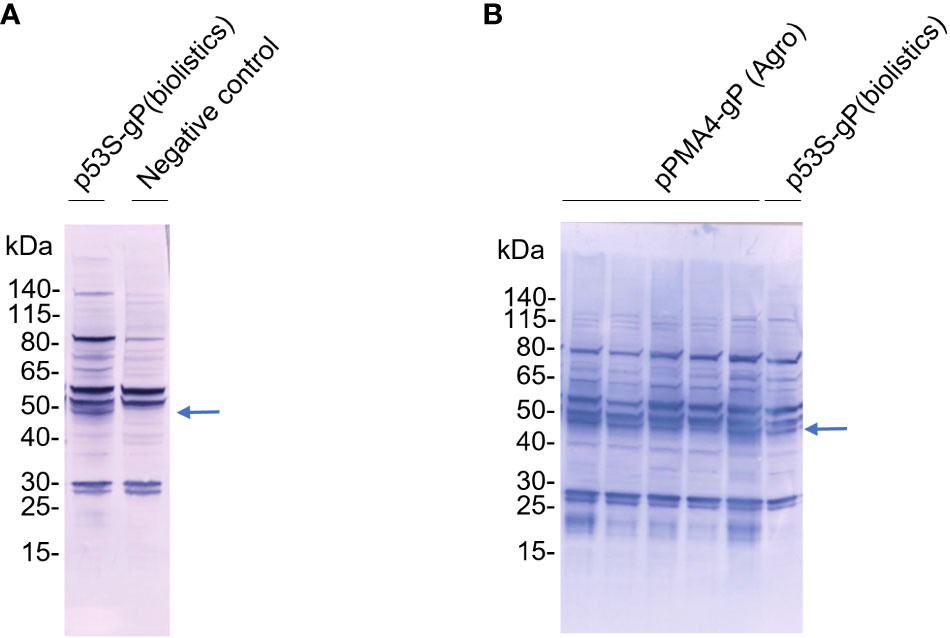
Figure 3 Expression of gP in XylT/FucT KO BY-2 cell line. TSP from gP transgenic cell lines obtained after biolistics or Agrobacterium transformation were analysed by Western Blotting using antibodies raised against gP and rabbit AP-coupled secondary antibodies. (A) Sixty µg of TSP from the p35S-gP transgenic cell line (Biolistics) and a transgenic control cell line expressing gB from HCMV (negative control); (B) Thirty µg of TSP from five independent PMA4-gP transgenic cell lines (Agrobacterium) and the p35S-gP transgenic cell line (Biolistics).
An alternative to overcome the transposon issue in A. tumefaciens LBA4404 consisted of replacing the 35S promoter with a plant promoter that has no activity in A. tumefaciens, like the plant plasma membrane proton pump ATPase PMA4 promoter (Figure 1A2). The corresponding binary plasmid containing the PDI-gP open reading frame under the control of the PMA4 promoter, and the kanamycin resistance marker was transferred in A. tumefaciens. The binary plasmids purified from six different A. tumefaciens LBA4404 transformants were retransformed in E. coli. The integrity of the binary plasmid was checked by restriction analysis (Figure 2C). Transgenic BY-2 cell lines were then generated via A. tumefaciens co-cultivation and selected on kanamycin-supplemented growth medium. A specific band at 50 kDa was detected by Western blotting analysis using polyclonal anti-gP antibodies (Figure 3B).
4 Discussion
For BY-2 cells transformation, we routinely use the A. tumefaciens strain LBA4404 virG (van der Fits et al., 2000), which has proved to be much more effective than others in obtaining transgenic BY-2 calli (Geelen and Inze, 2001; De Saeger et al., 2021). The LBA4404 strain directly derives from the LBA4213 strain, itself a derivative of the wild type Ach5 strain. To label the A. tumefaciens pTiAch5 plasmid regions, the Tn904 transposon, coding for streptomycin resistance and originating from Pseudomonas aeruginosa, was inserted into the Ti plasmid pAL102 present in A. tumefaciens Ach5 (Klapwijk et al., 1980; Ooms et al., 1980). Among the mutants that were not affected in virulence, the strain LBA4213 harboring the pAL231 pTi mutant was used to generate the strain LB4404, which contains a large deletion in the T-DNA in its non-oncogenic pAL4404 pTi (Ooms et al., 1982). Both pAL231 and pAL4404 pTi plasmids contain the same Tn904 insertion. Our results clearly showed that the gP-encoding cassette in the binary plasmid with a pRK2 origin was altered in A. tumefaciens LBA4404 by the insertion of transposons from the Tn3 family, which originated from pAL4404. This phenomenon was previously reported for a pGA472 derived binary vector, in which the Tn5393 transposon was inserted in the terminator sequence of the hygromycin-resistant selectable marker (Kim and An, 2012). Interestingly, this corruption of the expression cassette was not detected when gP was under the control of the plant PMA4 promoter, which has no activity in Agrobacterium. Moreover, in all tested transformants, the Tn904 transposon was inserted into the 5’ Ω-leader or in the gP open reading frame. We hypothesized that gP expression under the control of the 35S promoter was toxic to A. tumefaciens LBA4404 and that a counter-selection pressure occurred in Agrobacterium transformants, leading to the survival of only those colonies that had undergone a transposition event leading to the inhibition of gP expression.
Actually, certain Agrobacterium strains like LBA4404 appear to be more prone to plasmid instability than others (De Saeger et al., 2021). Another strain frequently used in plant biotechnology is the strain C58-derived GV3101. We transformed the binary plasmid that contains the gP open reading frame under the control of the 35S promoter and the kanamycin resistance cassette (Figure 1A) in the strains GV3101 or LBA4404, and showed no alteration of the binary plasmid restriction profile after GV3101 passage, while the plasmid was corrupted after passage in LBA4404 (Figure 2D). Whole sequencing of the gP expression cassette in the six binary plasmids derived from GV3101 confirmed its full integrity. These data indicate that the plasmid instability is dependent on the Agrobacterium strain and the presence of the transposon Tn904.
Given that previous data also reported that the transposon Tn5393 can be inserted into the plant genome along with the T-DNA, possibly via horizontal gene transfer (Zhao et al., 2009; Kim and An, 2012; Philips et al., 2017), caution should be taken when using A. tumefaciens LBA4404 T-DNA delivery.
In conclusion, this study highlights the necessity for a careful examination of each step while expressing recombinant proteins in plant cells, especially when using Agrobacterium-mediated transformation. More precisely, we recommend a close selection of the A. tumefaciens transformants when using LBA4404.The use of a promoter that shows no activity in bacterial cells should be preferred. The integration of an intron into the transgene coding sequence is another alternative. Finally, a modified Agrobacterium LBA4404 strain lacking the Tn904 transposon would be very useful, like in the patent recently published (Annaluru and Bass, 2022).
Data availability statement
The data analyzed in this study is subject to the following licenses/restrictions: This article utilises proprietary data. Requests to access these datasets should be directed to Catherine Navarre, Y2F0aGVyaW5lLm5hdmFycmVAdWNsb3V2YWluLmJl.
Author contributions
CN: Conceptualization, Data curation, Funding acquisition, Investigation, Methodology, Supervision, Writing – original draft, Writing – review & editing. RO: Investigation, Writing – original draft. NB: Investigation, Writing – review & editing. MP: Investigation, Writing – review & editing. FC: Conceptualization, Funding acquisition, Supervision, Writing – review & editing.
Funding
The author(s) declare financial support was received for the research, authorship, and/or publication of this article. This work was supported by the SPW Wallonie-Belgium in WALInnov project “Glycocell” (1810010). Nicolas Bailly is a recipient of a fellowship from the Fonds pour la Formation à la Recherche dans l’Industrie et l’Agriculture (FRIA, Belgium). Marie Peeters is a recipient of a fellowship from the Fonds National de la Recherche Scientifique (FNRS, Belgium).
Acknowledgments
We thank Adeline Courtoy (UCLouvain) for her excellent technical assistance. We are grateful to Professor Marc Boutry for his constant interest in our research and his careful reading of this manuscript.
Conflict of interest
The authors declare that the research was conducted in the absence of any commercial or financial relationships that could be construed as a potential conflict of interest.
Publisher’s note
All claims expressed in this article are solely those of the authors and do not necessarily represent those of their affiliated organizations, or those of the publisher, the editors and the reviewers. Any product that may be evaluated in this article, or claim that may be made by its manufacturer, is not guaranteed or endorsed by the publisher.
Supplementary material
The Supplementary Material for this article can be found online at: https://www.frontiersin.org/articles/10.3389/fpls.2023.1266775/full#supplementary-material
References
Amack, S. C., Antunes, M. S. (2020). CaMV35S promoter – A plant biology and biotechnology workhorse in the era of synthetic biology. Curr. Plant Biol. 24, 100179. doi: 10.1016/j.cpb.2020.100179
Annaluru, S. V., Bass, S. H. (2022). Modified Agrobacterium strains and use thereof for plant transformation, U.S. Appl. N° 17,440,303. Washington, DC: U.S. Patent and Trademark Office.
Assaad, F. F., Signer, E. R. (1990). Cauliflower mosaic virus P35S promoter activity in Escherichia coli. Mol. Gen. Genet. MGG 223 (3), 517–520. doi: 10.1007/BF00264462
De Muynck, B., Navarre, C., Nizet, Y., Stadlmann, J., Boutry, M. (2009). Different subcellular localization and glycosylation for a functional antibody expressed in Nicotiana tabacum plants and suspension cells. Transgenic Res. 18 (3), 467–482. doi: 10.1007/s11248-008-9240-1
De Saeger, J., Park, J., Chung, H. S., Hernalsteens, J. P., Van Lijsebettens, M., Inzé, D., et al. (2021). Agrobacterium strains and strain improvement: Present and outlook. Biotechnol. Adv. 53, 107677. doi: 10.1016/j.bioteChadv.2020.107677
Engler, C., Youles, M., Gruetzner, R., Ehnert, T. M., Werner, S., Jones, J. D., et al. (2014). A golden gate modular cloning toolbox for plants. ACS Synth Biol. 3 (11), 839–843. doi: 10.1021/sb4001504
Geelen, D. N. V., Inze, D. G. (2001). A bright future for the bright yellow-2 cell culture. Plant Physiol. 127 (4), 1375–1379. doi: 10.1104/pp.010708
Herman, X., Far, J., Courtoy, A., Bouhon, L., Quinton, L., De Pauw, E., et al. (2021). Inactivation of N-acetylglucosaminyltransferase I and α1,3-fucosyltransferase genes in nicotiana tabacum BY-2 cells results in glycoproteins with highly homogeneous, high-mannose N-glycans. Front. Plant Sci. 12. doi: 10.3389/fpls.2021.634023
Herman, X., Far, J., Peeters, M., Quinton, L., Chaumont, F., Navarre, C. (2023). In vivo deglycosylation of recombinant glycoproteins in tobacco BY-2 cells. Plant Biotechnol. J 21, 1773-1784. doi: 10.1111/pbi.14074
Johansen, I. E. (1996). Intron insertion facilitates amplification of cloned virus cDNA in Escherichia coli while biological activity is reestablished after transcription in vivo. Proc. Natl. Acad. Sci. U.S.A. 93 (22), 12400–12405. doi: 10.1073/pnas.93.22.12400
Kim, S. R., An, G. (2012). Bacterial transposons are co-transferred with T-DNA to rice chromosomes during Agrobacterium-mediated transformation. Mol. Cells 33 (6), 583–589. doi: 10.1007/s10059-012-0010-9
Klapwijk, P. M., van Breukelen, J., Korevaar, K., Ooms, G., Schilperoort, R. A. (1980). Transposition of Tn904 encoding streptomycin resistance into the octopine Ti plasmid of Agrobacterium tumefaciens. J. Bacteriol. 141 (1), 129–136. doi: 10.1128/jb.141.1.129-136.1980
Magy, B., Tollet, J., Laterre, R., Boutry, M., Navarre, C. (2014). Accumulation of secreted antibodies in plant cell cultures varies according to the isotype, host species and culture conditions. Plant Biotechnol. J. 12 (4), 457–467. doi: 10.1111/pbi.12152
Mercx, S., Smargiasso, N., Chaumont, F., De Pauw, E., Boutry, M., Navarre, C. (2017). Inactivation of the β(1,2)-xylosyltransferase and the α(1,3)-fucosyltransferase genes in Nicotiana tabacum BY-2 Cells by a Multiplex CRISPR/Cas9 Strategy Results in Glycoproteins without Plant-Specific Glycans. Front. Plant Sci. 8. doi: 10.3389/fpls.2017.00403
Navarre, C., Catty, P., Leterme, S., Dietrich, F., Goffeau, A. (1994). Two distinct genes encode small isoproteolipids affecting plasma membrane H(+)-ATPase activity of Saccharomyces cerevisiae. J. Biol. Chem. 269 (33), 21262–21268. doi: 10.1016/S0021-9258(17)31958-0
Navarre, C., Chaumont, F. (2022). Production of recombinant glycoproteins in nicotiana tabacum BY-2 suspension cells. Methods Mol. Biol. 2480, 81–88. doi: 10.1007/978-1-0716-2241-4_5
Navarre, C., Sallets, A., Gauthy, E., Maîtrejean, M., Magy, B., Nader, J., et al. (2011). Isolation of heat shock-induced Nicotiana tabacum transcription promoters and their potential as a tool for plant research and biotechnology. Transgenic Res. 20 (4), 799–810. doi: 10.1007/s11248-010-9459-5
Ohta, S., Mita, S., Hattori, T., Nakamura, K. (1990). Construction and expression in tobacco of a β-glucuronidase (GUS) reporter gene containing an intron within the coding sequence. Plant Cell Physiol. 31 (6), 805–813. doi: 10.1093/oxfordjournals.pcp.a077982
Ooms, G., Hooykaas, P. J. J., Van Veen, R. J. M., Van Beelen, P., Regensburg-Tuïnk, T. J. G., Schilperoort, R. A. (1982). Octopine Ti-plasmid deletion mutants of Agrobacterium tumefaciens with emphasis on the right side of the T-region. Plasmid 7 (1), 15–29. doi: 10.1016/0147-619X(82)90023-3
Ooms, G., Klapwijk, P. M., Poulis, J. A., Schilperoort, R. A. (1980). Characterization of Tn904 insertions in octopine Ti plasmid mutants of Agrobacterium tumefaciens. J. Bacteriol. 144 (1), 82–91. doi: 10.1128/jb.144.1.82-91.1980
Philips, J. G., Naim, F., Lorenc, M. T., Dudley, K. J., Hellens, R. P., Waterhouse, P. M. (2017). The widely used Nicotiana benthamiana 16c line has an unusual T-DNA integration pattern including a transposon sequence. PloS One 12 (2), e0171311. doi: 10.1371/journal.pone.0171311
Reed, J., Osbourn, A. (2018). Engineering terpenoid production through transient expression in Nicotiana benthamiana. Plant Cell Rep. 37 (10), 1431–1441. doi: 10.1007/s00299-018-2296-3
Schillberg, S., Spiegel, H. (2022). Recombinant protein production in plants: A brief overview of strengths and challenges. Methods Mol. Biol. 2480, 1–13. doi: 10.1007/978-1-0716-2241-4_1
Vancanneyt, G., Schmidt, R., O'Connor-Sanchez, A., Willmitzer, L., Rocha-Sosa, M. (1990). Construction of an intron-containing marker gene: Splicing of the intron in transgenic plants and its use in monitoring early events in Agrobacterium-mediated plant transformation. Mol. Gen. Genet. MGG 220 (2), 245–250. doi: 10.1007/BF00260489
van der Fits, L., Deakin, E. A., Hoge, J. H. C., Memelink, J. (2000). The ternary transformation system: constitutive virG on a compatible plasmid dramatically increases Agrobacterium-mediated plant transformation. Plant Mol. Biol. 43 (4), 495–502. doi: 10.1023/A:1006440221718
Weber, E., Engler, C., Gruetzner, R., Werner, S., Marillonnet, S. (2011). A modular cloning system for standardized assembly of multigene constructs. PloS One 6 (2), e16765. doi: 10.1371/journal.pone.0016765
Keywords: Agrobacterium, bacterial transposon, LBA4404, Ti plasmid, BY-2 cells
Citation: Navarre C, Orval R, Peeters M, Bailly N and Chaumont F (2023) Issue when expressing a recombinant protein under the control of p35S in Nicotiana tabacum BY-2 cells. Front. Plant Sci. 14:1266775. doi: 10.3389/fpls.2023.1266775
Received: 25 July 2023; Accepted: 24 October 2023;
Published: 13 November 2023.
Edited by:
Sylvain Legay, Luxembourg Institute of Science and Technology (LIST), LuxembourgReviewed by:
Andreas Schiermeyer, Fraunhofer Society (FHG), GermanyBeatriz Xoconostle-Cázares, National Polytechnic Institute of Mexico (CINVESTAV), Mexico
Copyright © 2023 Navarre, Orval, Peeters, Bailly and Chaumont. This is an open-access article distributed under the terms of the Creative Commons Attribution License (CC BY). The use, distribution or reproduction in other forums is permitted, provided the original author(s) and the copyright owner(s) are credited and that the original publication in this journal is cited, in accordance with accepted academic practice. No use, distribution or reproduction is permitted which does not comply with these terms.
*Correspondence: Catherine Navarre, Q2F0aGVyaW5lLm5hdmFycmVAdWNsb3V2YWluLmJl; François Chaumont, ZnJhbmNvaXMuY2hhdW1vbnRAdWNsb3V2YWluLmJl