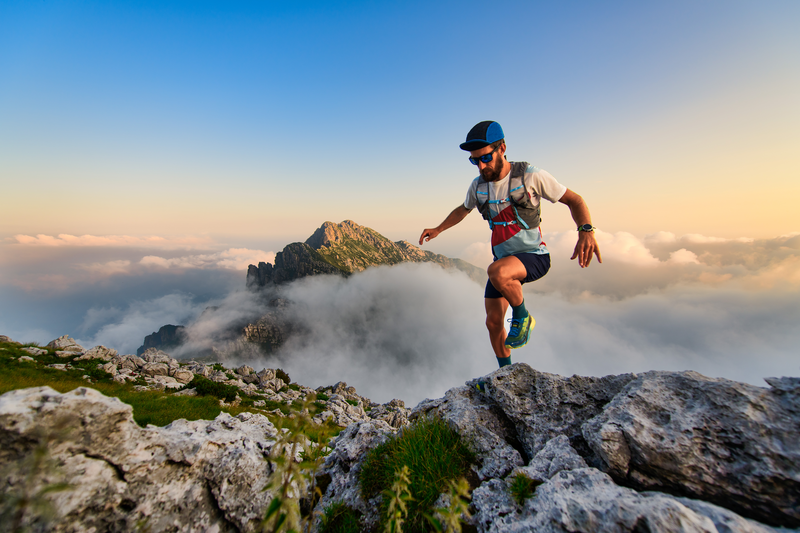
95% of researchers rate our articles as excellent or good
Learn more about the work of our research integrity team to safeguard the quality of each article we publish.
Find out more
ORIGINAL RESEARCH article
Front. Plant Sci. , 10 May 2023
Sec. Plant Bioinformatics
Volume 14 - 2023 | https://doi.org/10.3389/fpls.2023.1184416
This article is part of the Research Topic Mining and Utilization of Favorable Gene Resources in Rice View all 11 articles
Background: Salinity tolerance plays a vital role in rice cultivation because the strength of salinity tolerance at the seedling stage directly affects seedling survival and final crop yield in saline soils. Here, we combined a genome-wide association study (GWAS) and linkage mapping to analyze the candidate intervals for salinity tolerance in Japonica rice at the seedling stage.
Results: We used the Na+ concentration in shoots (SNC), K+ concentration in shoots (SKC), Na+/K+ ratio in shoots (SNK), and seedling survival rate (SSR) as indices to assess the salinity tolerance at the seedling stage in rice. The GWAS identified the lead SNP (Chr12_20864157), associated with an SNK, which the linkage mapping detected as being in qSK12. A 195-kb region on chromosome 12 was selected based on the overlapping regions in the GWAS and the linkage mapping. Based on haplotype analysis, qRT-PCR, and sequence analysis, we obtained LOC_Os12g34450 as a candidate gene.
Conclusion: Based on these results, LOC_Os12g34450 was identified as a candidate gene contributing to salinity tolerance in Japonica rice. This study provides valuable guidance for plant breeders to improve the response of Japonica rice to salt stress.
Soil salinity is an important limiting factor affecting high and stable resistance in rice and expansion areas for the economically important crop (Ouhibi et al., 2014). Salt stress inhibits plant protein synthesis, reduces photosynthetic efficiency, causes ion imbalances and high osmotic stress, and leads to plant wilting and apoptosis (Ma et al., 2017). This stress response significantly reduces crop yield and has become an important environmental factor affecting crop growth and development. But at present, due to unreasonable irrigation methods and excessive fertilization, the salinization of soil is becoming more and more serious (Qadir et al., 2014). Rice can effectively help people by ensuring food security and advancing sustainable agriculture development. Growing rice on saline land can improve the utilization of saline soils and the soil conditions of the land. Rice is a moderately salt-sensitive crop, and its seedling salinity tolerance is a key factor determining its final yield. Therefore, its fine positioning can not only reveal its molecular mechanism, but also provide a theoretical basis for improving salt-tolerant varieties of rice.
Multiple rice genes control the complex quantitative trait known as salinity tolerance (Liang et al., 2015), and scientists have made a series of important research advances in cloning salt stress genes (Lin et al., 2004; Bimpong et al., 2014; Yu et al., 2017; Li et al., 2022). For example, SKC1, rice’s first salinity tolerance QTL, encodes a transporter protein of the HKT family. Previous studies have shown that SKC1 protein is a sodium (Na+)-selective transporter protein that can effectively regulate the aboveground sodium and potassium (Na+/K+) balance and improve salinity tolerance (Ren et al., 2005). Meanwhile, Huang et al. (2009) reported a rice drought and salinity tolerance gene, DST, which negatively regulates salinity tolerance. The functional deletion of DST directly down-regulates the expression of genes related to hydrogen peroxide metabolism, reduces water evaporation under drought stress and Na+ entry into the plant, and ultimately improves the salinity tolerance of rice. Li et al. (2014) identified a lectin receptor-like kinase SIT1 in rice, which mediates the salt-sensitive response in rice, and its rapid increase in response to high salt stimulation activates the downstream effectors MAPK3 and MAPK6, which in turn increases ethylene content by activating ethylene synthase and causing an increase in reactive oxygen species, resulting in reduced survival under salt stress. Zhou et al. (2018) identified a receptor-like cytoplasmic kinase gene, STRK1, significantly improving salinity tolerance in rice. The STRK1 protein undergoes autophosphorylation upon salt stress and significantly increases its activity by phosphorylating and activating CatC, thereby degrading the large amount of hydrogen peroxide produced by salt stress.
Cloning salinity tolerance genes has produced important breakthroughs in the molecular mechanism elucidation of important complex traits in rice, which have become a reference for studying the genetic mechanisms behind complex traits in rice. This work has significant application value and lays the foundation for identifying and cloning salinity tolerance genes in crops. Combining GWAS and QTL mapping for gene mining can significantly improve the efficiency of QTL identification, and the results can be verified against each other, resulting in stable and reliable QTLs. For example, Zhang et al. (2019) identified a candidate gene, GmCDF1, which is closely linked to soybean salinity tolerance on chromosome 8 through linkage and association analysis. Wu et al. (2016) combined GWAS and linkage analysis to identify 125 QTLs regulating maize male inflorescence size. Tang et al. (2021) used linkage localization and GWAS analysis to locate one QTL region controlling rice grain length, further identifying OsGASR7 as a functional gene within this QTL interval. Therefore, combining the two methods is important for mining candidate genes for target traits.
This study explored the genetic mechanism of salinity tolerance as assessed by GWAS and linkage mapping using Na+ concentration in shoots (SNC), K+ concentration in shoots (SKC), Na+/K+ ratio in shoots (SNK), and seedling survival rate (SSR) at the rice seedling salt treatment. We identified Chr12_20864157 and qSK12 on chromosome 12 by GWAS and linkage mapping, identifying an overlapping region of the 195-kb as a candidate region. Using haplotype analysis, qRT-PCR, and sequence analysis, LOC_Os12g34450 was considered the most likely functional gene associated with salinity tolerance. The results procured by this study provide novel insights for improving salinity tolerance in Japonica rice.
It consists of 295 Japonica rice varieties widely grown at home and abroad, of which domestic materials are mainly from Heilongjiang, Jilin, Liaoning, and Ningxia provinces, and foreign varieties were mainly from Korea, Russia, and Japan. The 195 RILs that comprised the linkage mapping population were a cross between the salt-sensitive Kongyu131 and the salt-tolerant Xiaobaijingzi. The salinity tolerance phenotypes of KY131 and XBJZ were shown in Figure 1. All rice varieties have been studied previously (Li et al., 2019; Li et al., 2020; Duan et al., 2022).
Figure 1 Phenotypes of KY131 and XBJZ seedlings under contral and salt stress. (A) Represent under control conditions. Bar = 5 cm. (B) Represent under salt stress conditions. Bar = 5 cm.
The experiment was divided into two groups, named group T1 and group T2, and set up for three repetitions. In group T1, twenty-four uniformly germinated rice seeds of each variety were sown. One seed was placed in each hole and hydroponically grown with Yoshida nutrient solution. The germinated seeds were transferred to an artificial climatic chamber and incubated at 25 and 23°C with 14h light and 10h dark cycles, respectively. When seedlings grew to two leaves and one heart, salt stress treatment was performed with a pre-treatment sodium chloride (NaCl) concentration of 50 mmol/L.
After 3 days of pre-treatment, formal treatment was performed with a NaCl concentration of 120 mmol/L for 7 days. The shoot of each sample was dried at 120°C for 30 min and at 80°C to a constant weight. Weigh 0.1 grams (g) of the dry samples, add 5 ml of 1 mol/L HCl to the tube, and put it into a water bath for 6 h at 70°C in a constant temperature water bath shaker. The SNC and SKC of the samples were measured using a flame photometer (Sherwood 410, Cambridge, UK) and the SNK was calculated. In group T2, 100 uniformly germinated seeds of each variety were grown with Yoshida nutrient solution, with the same salt treatment as the T1 group. The culture medium was replaced every day, and replaced with the same medium as the control group after 7 days. After 10 days, the survival rate statistics counted the number of plants with new leaf production.
In total, 788,396 single nucleotide polymorphisms (SNPs) were used for genotyping 295 Japonica rice accessions for GWAS. The threshold for identifying significantly associated SNPs was set at –log10(P) > 5.26, according to a pre-laboratory study by Li et al. (2020). The Manhattan map was created using the R package ‘qqman’. Redundant SNPs with the smallest P values were filtered within a minimal distance interval and the LDBlockShow software was used to calculate the pairwise R2 value between any two SNPs in the interval of leading SNPs ± 2 Mb. In the interval of 1.5-2.0 Mb of leading SNPs, the average of the top 10% R2 values was recorded, plus 0.2 to define the LD attenuation interval of leading SNPs interval (Dong et al., 2021).
The genetic linkage map constructed using 195 RILs contained 527 bin markers (Figure S1). QTL localization was performed using the composite interval mapping method with QTL ICIMapping 4.2 software, and the threshold value was set to LOD>2.5, according to a pre-laboratory study by Li et al. (2020).
Non-synonymous mutant SNPs in the exonic regions of all genes in the candidate interval and SNPs in the promoter region (1.5 kb before ATG) were extracted from the RiceSNPSeekDatabase website (https://snp-seek.irri.org/_snp.zul) and haplotype analysis was performed using DnaSP software. Also, materials with different haplotypes needed to be greater than or equal to 10.
The expression levels of the four genes of KY131 and XBJZ were verified by qRT-PCR analysis under salinated and normal conditions. qRT-PCR analysis was performed using Roche LightCycler96. All primer sequences were shown in Table S3. The CDS and promoter regions of KY131 and XBJZ candidate genes were cloned using PCR. Sequences comparison were performed using DNAMAN.
In this study, we analyzed the phenotypes of 295 Japonica rice accessions and RIL lines at the seedling stage under salinity stress and evaluated four salinity tolerance indices: SNC, SKC, SNK, and SSR. Under the salinity stress treatment, the SNC, SKC, SNK, and SSR among the 295 rice accessions varied in range from 10.14–38.83 mmol/g, 5.21–32.82 mmol/g, 0.79–2.78, and 9.33–88.66%, respectively, and the coefficient of variation was 20.13%, 26.73%, 28.61%, and 28.12%, respectively (Table S1). The variation for SNC, SKC, SNK, and SSR in the RIL lines ranged from 8.12–29.56 mmol/g, 6.98–39.56 mmol/g, 0.46–2.19, and 8.66–89.33%, respectively, and the coefficient of variation was 20.48%, 40.58%, 37.01%, and 32.99%, respectively (Table S1). Phenotypic data indicate that this study’s natural and RIL populations have abundant phenotypic variation in rice seedling salinity tolerance. Meanwhile, the values of these four traits differed significantly between the two parents (Figures 2E–H; Table S1), the phenotype values of the four traits indicating that XBJZ was more salinity-tolerant than KY131. The SNC, SKC, SNK, and SSR phenotypic values in the 295 rice accessions and RIL lines were normally distributed, demonstrating that these indices are quantitative traits under the control of numerous factors (Figure 2).
Figure 2 Phenotypic variation in the SNC, SKC, SNK, and SSR in 295 Japonica rice accessions and RIL lines. (A–D) Represent the SNC, SKC, SNK, and SSR of 295 rice accessions. (E–H) Represent the SNC, SKC, SNK, and SSR of RIL lines.
The 788,396 SNPs obtained from previous studies were used for GWAS analysis (Li et al., 2019; Zheng et al., 2022). Manhattan and quantile–quantile plots were shown in Figure 3. Fourteen lead SNPs significantly associated with SNC, SNK, and SSR were provided in Table 1. Three QTLs associated with SNC were detected and located on chromosomes 1, 4, and 12, with R2 values ranging from 9.20–10.36%. No QTL associated with SKC was detected, and eight QTLs associated with SNK were detected and located on chromosomes 1, 4, 6, 9, 10, and 12, with R2 values ranging from 9.05–10.30%. Meanwhile, three QTLs associated with SSR were detected and located on chromosomes 4, 8, and 10, with R2 values ranging from 9.76–12.02% (Table 1).
Figure 3 Manhattan plots and quantile-quantile (Q-Q) plots of GWAS for the SNC, SKC, SNK, and SSR. (A–D) Manhattan plot for the SNC, SKC, SNK, and SSR. (E–H) Q-Q plot for the SNC, SKC, SNK, and SSR.
A total of five QTLs associated with SNC, SKC, SNK, and SSR were identified on chromosomes 1, 4, and 12 using linkage mapping (Table 2; Figure S1), with LOD values from 2.51–8.22 and proportions of phenotypic variation ranged from 5.49–18.27%. In addition, qSKC12 and qSNK12 were considered to be the same QTL because of the same interval, named qSK12 (chromosome 12) (Figure 4B), located in the physical region between markers C12_20029364 and C12_20873254 and explaining 11.54–18.27% of the phenotypic variation. The GWAS identified the lead SNPs, Chr12_20864157, associated with an SNK, which the linkage mapping detected as being in qSK12. The LD block region on chromosome 12 was predicted to be 20.718–20.905 Mb (186 kb) (Figure 4A). A 195-kb overlap region was filtered out based on the GWAS and the linkage mapping (Figure 4C).
Figure 4 Identification of candidate genes by GWAS and linkage mapping. (A) The local Manhattan plot (top) and LD heatmap (bottom) surround the lead SNP. (B) Salinity tolerance-related QTLs were identified in 195 RILs and mapped to the interval between markers C12_20029364 and C12_20873254 by linkage mapping. (C) The physical location of the lead SNP (C12_20864157) on chromosome 12 was detected by the GWAS (LD decay = 186 kb). (D) The 195-kb region contained 35 genes.
According to the Phytozome database, the 195-kb candidate interval did not include known salinity tolerance genes from previous studies. The 195-kb region on chromosome 12 contained 35 genes (Figure 4D; Table S2). We performed haplotype analysis of 35 genes and found that 4 genes (LOC_Os12g34320, LOC_Os12g34330, LOC_Os12g34450, and LOC_Os12g34460) within the overlapping interval were associated with significantly different haplotypes of SNKs. LOC_Os12g34320 and LOC_Os12g34450 were classified into two haplotypes by non-synonymous mutant SNPs in the exon region. One SNP located in the 5′ untranslated region of LOC_Os12g34330 formed two haplotypes, while no non-synonymous SNP was found in the exon region. The haplotype analysis of LOC_Os12g34460 indicated that 2 SNPs were identified in the promoter region and 4 non-synonymous SNPs were detected in the exon region (Figures 5A–D).
Figure 5 Haplotype analysis of LOC_Os12g34320, LOC_Os12g34330, LOC_Os12g34450, LOC_Os12g34460. (A–D) Represent the gene structure and haplotype analysis of LOC_Os12g34320, LOC_Os12g34330, LOC_Os12g34450, LOC_Os12g34460. (The * and ** suggest significance of ANOVA at P < 0.05 and P < 0.01, respectively).
The two parents, KY131 and XBJZ, were treated with 120 mM NaCl for 0, 1, 3, 6, 12, and 24 h, respectively. The expression patterns of these four genes were assessed by qRT-PCR analysis. The mean results in triplicate were shown in Figure 6. Among these four genes, salinity stress did not increase the expression levels of LOC_Os12g34320, LOC_Os12g34330, or LOC_Os12g34460 (Figures 6A, B, D). LOC_Os12g34450 was significantly induced by salinity stress, and the expression levels were significantly different between KY131 and XBJZ, which showed opposite expression patterns. The expression level of LOC_Os12g34450 was more than 18-fold higher in KY131 than in XBJZ under 6 h of salinity stress (Figure 6C). Meanwhile, we performed qRT-PCR analysis of other functionally annotated genes within the 195-kb region, and the results of the four genes expressed between the two parents are shown in Figure S2, which were not differentially expressed between the two parents. After observing these results, we completed further sequencing of the promoter regions and genes of LOC_O12g34450 in the two parents KY131 and XBJZ. Compared with the sequence of KY131, LOC_Os12g34450 of XBJZ had a 2-bp deletion (A and C bases) and 2 SNPs (T→G, G→A) in the first exon of the CDS region, 1 SNP (T→C) in the third exon, and multiple SNPs in the promoter region containing 1 cis-element (TGA-element) related to auxin response (AACGAC→GACGAT) (Figure S3). Therefore, LOC_Os12g34450 was considered a functional gene associated with salinity tolerance. LOC_Os12g34450 encodes an auxin-binding protein 4 precursor gene, which has not been reported to affect salinity tolerance in rice in previous studies. In subsequent studies, transgenic plants will be examined for the biological function of their associated genomic variant’s responses to salinity tolerance in Japonica rice.
Figure 6 Expression patterns of the four genes under normal growth conditions and salinity stress. (A–D) represent the gene expression of LOC_Os12g34320, LOC_Os12g34330, LOC_Os12g34450, LOC_Os12g3446 under normal growth conditions and salinity stress. (**P < 0.01, ***P < 0.001, Students’t-test).
Rice seedlings have poor salinity tolerance and are susceptible to salt stress, which seriously affects their planting and yield. Therefore, cultivating salinity tolerance in seedlings has great practical significance for agricultural production (Nam et al., 2015). This study selected SNC, SKC, SNK, and SSR as indicators to assess salinity tolerance in rice seedlings, which have also been used in previous studies (Rahman et al., 2016). Under salinity stress conditions, the Na+ content in rice seedling shoots and roots increases, and the K+ content decreases. This chemical change leads to a higher Na+/K+ ratio and disrupts the ionic balance by decreasing Mg, Zn, and Mn content (Tuncturk et al., 2008), also leading to osmotic stress and growth inhibition (Munns, 2011).
Salinity tolerance is a complex trait, and identifying such QTLs helps obtain relative salt tolerance genes for molecular-assisted breeding (Ganie et al., 2019). Eleven and three QTLs were identified by GWAS and linkage mapping, respectively, and these were close to or overlapped with the loci of some known genes and QTLs compared with the results of previous studies. For example, OsCLC-1, which encodes a voltage-gated chloride channel protein, could avoid ion toxicity by transporting chloride ions across the vesicle membrane to the vesicles (Nakamura et al., 2006); the lead SNP Chr4_21840798 identified by GWAS was approximately 45 kb closer to OsCLC-1. OsMYB9, encoding an R2R3-type MYB transcription factor that functions in the ABA-mediated signaling pathway, thereby improving the salinity tolerance of rice (Zhu et al., 2015), was within the qSNC12 identified by the linkage mapping. Additionally, Shen et al. (2017) found that OsNAC2, was able to regulate abiotic stress response, OsNAC2 overexpression plants showed reduced tolerance under salt stress, and OsNAC2 was within the qSSR4 identified by the linkage mapping.
Sabouri and Sabouri (2008) detected a salinity tolerance-associated QTL (qNAUP-1a) on chromosome 1, and the lead SNPs (Chr1_27088613 and Chr1_26805021), detected by GWAS, were located within qNAUP-1a. Islam et al. (2011) detected a salinity tolerance-related QTL (SalTol10-1) on chromosome 10, and the lead SNPs Chr10_7916859 and Chr10_16340314 detected by GWAS were both located within SalTol10-1. In addition, linkage mapping revealed that the QTL qSNC12 was within the salinity tolerance interval qSH12.1 (Wang et al., 2012), which supported our findings. In our study, GWAS and linkage mapping identified another salinity tolerance QTL, QSst12, containing Chr12_20864157 and qSK12.
The LOC_Os12g34450 was considered the most likely functional gene associated with salinity tolerance. The expression of LOC_Os12g34450 was significantly up-regulated in XBJZ and down-regulated in KY131 after salinity stress. Meanwhile, we found that LOC_Os12g34450 of XBJZ contained multiple SNPs in the promoter region, including a cis-element (TGA-element) related to auxin response (AACGAC→GACGAT), three non-synonymous mutation SNPs (T→G, G→A, T→C) in the CDS region, and a 2-bp deletion (A and C bases) in the first exon compared with the sequence of KY131. These SNPs and deletions result in a frameshift that leads to a premature stop codon, which leads to premature termination of the mRNA of the LOC_Os12g34450. LOC_Os12g34450 is predicted to encode an auxin-binding protein, and accumulating evidence in recent years supports an important role for auxin in abiotic stress responses in plants.
In Arabidopsis, the NTM2 plays an important role in regulating plant seed germination under high-salt stress (Jung and Park, 2011). Overexpression of OsmiR393 in rice resulted in the down-regulation of two auxin receptor gene homologs (OsTIR1 and OsAFB2), which reduced salinity tolerance in rice (Xia et al., 2012). Deng et al. (2022) found that RST1 encodes the growth factor response factor OsARF18 and that RST1 loss of function leads to up-regulation of OsAS1 expression, which improves nitrogen utilization, reduces Na+/K+ ratios, and decreases NH4+ overaccumulation by promoting asparagine synthesis, thereby improving salt tolerance and yield of plants.
The original contributions presented in the study are included in the article/Supplementary Material, further inquiries can be directed to the corresponding authors.
SX, DZ, and HZ designed the study and provided experimental materials. SX, JC, HC, SL, and TM performed the experiments. SX, JC, and HZ analyzed the results and wrote the manuscript. All authors contributed to the article and approved the submitted version.
This research was funded by the National Natural Science Foundation of China (grant No. U20A2025, 31872884), and the “Academic Backbone” Project of Northeast Agricultural University (grant No. 20XG24).
The authors declare that the research was conducted in the absence of any commercial or financial relationships that could be construed as a potential conflict of interest.
All claims expressed in this article are solely those of the authors and do not necessarily represent those of their affiliated organizations, or those of the publisher, the editors and the reviewers. Any product that may be evaluated in this article, or claim that may be made by its manufacturer, is not guaranteed or endorsed by the publisher.
The Supplementary Material for this article can be found online at: https://www.frontiersin.org/articles/10.3389/fpls.2023.1184416/full#supplementary-material
Supplementary Figure 1 | Genetic linkage map and QTL mapping results.
Supplementary Figure 2 | Expression patterns of the other four genes under normal growth conditions and salinity stress (** P < 0.01, *** P < 0.001, Students’t-test).
Supplementary Figure 3 | The gene structure and sequence difference analysis of LOC_Os12g34450. (A) The gene structure of LOC_Os12g34450. (B) The sequence difference analysis of LOC_Os12g34450 in Xiaobaijingzi, Kongyu131, and Nipponbare.
Bimpong, I. K., Manneh, B., Diop, B., Ghislain, K., Sow, A., Amoah, N. K. A., et al. (2014). New quantitative trait loci for enhancing adaptation to salinity in rice from hasawi, a Saudi landrace into three African cultivars at the reproductive stage. Euphytica 200 (1), 45–60. doi: 10.1007/s10681-014-1134-0
Cheng, L. R., Wang, Y., Meng, L. J., Hu, X., Cui, Y. R., Sun, Y., et al. (2012). Identification of salt-tolerant QTLs with strong genetic background effect using two sets of reciprocal introgression lines in rice. Genome 55 (1), 45–55. doi: 10.1139/g11-075
Deng, P., Jing, W., Cao, C., Sun, M., Chi, W., Zhao, S., et al. (2022). Transcriptional repressor RST1 controls salt tolerance and grain yield in rice by regulating gene expression of asparagine synthetase. Proc. Natl. Acad. Sci. U. S. A. 119 (50), e2210338119. doi: 10.1073/pnas.2210338119
Dong, S. S., He, W. M., Ji, J. J., Zhang, C., Yang, T. L. (2021). LDBlockShow: a fast and convenient tool for visualizing linkage disequilibrium and haplotype blocks based on variant call format files. Brief Bioinform. 22 (4), bbaa227. doi: 10.1093/bib/bbaa227
Duan, Y. X., Zheng, H. L., Wen, H. R., Qu, D., Cui, J. N., Li, C., et al. (2022). Identification of candidate genes for salt tolerance at the germination stage in japonica rice by genome-wide association analysis. Agriculture-Basel 12 (10), 15. doi: 10.3390/agriculture12101588
Ganie, S. A., Molla, K. A., Henry, R. J., Bhat, K. V., Mondal, T. K. (2019). Advances in understanding salt tolerance in rice. Theor. Appl. Genet. 132 (4), 851–870. doi: 10.1007/s00122-019-03301-8
Ghomi, K., Rabiei, B., Sabouri, H., Sabouri, A. (2013). Mapping QTLs for traits related to salinity tolerance at seedling stage of rice (Oryza sativa l.): an agrigenomics study of an Iranian rice population. OMICS 17 (5), 242–251. doi: 10.1089/omi.2012.0097
Huang, X. Y., Chao, D. Y., Gao, J. P., Zhu, M. Z., Shi, M., Lin, H. X. (2009). A previously unknown zinc finger protein, DST, regulates drought and salt tolerance in rice via stomatal aperture control. Genes Dev. 23 (15), 1805–1817. doi: 10.1101/gad.1812409
Islam, M. R., Salambr, M., Hassanbr, L., Collardbr, B., Gregorio, B. (2011). QTL mapping for salinity tolerance at seedling stage in rice. Journal of the Science of Food and Agriculture emir. J. Food Agric. 23 (2), 137–146(2). doi: 10.9755/ejfa.v23i2.6348
Jung, J.-H., Park, C.-M. (2011). Auxin modulation of salt stress signaling in arabidopsis seed germination. Plant Signal Behav. 6 (8), 1198–1200. doi: 10.4161/psb.6.8.15792
Li, C. H., Wang, G., Zhao, J. L., Zhang, L. Q., Ai, L. F., Han, Y. F., et al. (2014). The receptor-like kinase SIT1 mediates salt sensitivity by activating MAPK3/6 and regulating ethylene homeostasis in rice. Plant Cell 26 (6), 2538–2553. doi: 10.1105/tpc.114.125187
Li, N., Zheng, H. L., Cui, J. N., Wang, J. G., Liu, H. L., Sun, J., et al. (2019). Genome-wide association study and candidate gene analysis of alkalinity tolerance in japonica rice germplasm at the seedling stage. Rice 12, 12. doi: 10.1186/s12284-019-0285-y
Li, X. W., Zheng, H. L., Wu, W. S., Liu, H. L., Wang, J. G., Jia, Y., et al. (2020). QTL mapping and candidate gene analysis for alkali tolerance in japonica rice at the bud stage based on linkage mapping and genome-wide association study. Rice 13 (1), 11. doi: 10.1186/s12284-020-00412-5
Li, Y. X., Zhou, J. H., Li, Z., Qiao, J. Z., Quan, R. D., Wang, J., et al. (2022). SALT AND ABA RESPONSE ERF1 improves seed germination and salt tolerance by repressing ABA signaling in rice. Plant Physiol. 189 (2), 1110–1127. doi: 10.1093/plphys/kiac125
Liang, J. L., Qu, Y. P., Yang, C. G., Ma, X. D., Cao, G. L., Zhao, Z. W., et al. (2015). Identification of QTLs associated with salt or alkaline tolerance at the seedling stage in rice under salt or alkaline stress. Euphytica 201 (3), 441–452. doi: 10.1007/s10681-014-1236-8
Lin, H. X., Zhu, M. Z., Yano, M., Gao, J. P., Liang, Z. W., Su, W. A., et al. (2004). QTLs for na+ and k+ uptake of the shoots and roots controlling rice salt tolerance. Theor. Appl. Genet. 108 (2), 253–260. doi: 10.1007/s00122-003-1421-y
Ma, C. Q., Wang, Y. G., Gu, D., Nan, J. D., Chen, S. X., Li, H. Y. (2017). Overexpression of s-Adenosyl-L-Methionine synthetase 2 from sugar beet M14 increased arabidopsis tolerance to salt and oxidative stress. Int. J. Mol. Sci. 18 (4), 16. doi: 10.3390/ijms18040847
Mohammadi, R., Mendioro, M. S., Diaz, G. Q., Gregorio, G. B., Singh, R. K. (2013). Mapping quantitative trait loci associated with yield and yield components under reproductive stage salinity stress in rice (Oryza sativa l.). J. Genet. 92 (3), 433–443. doi: 10.1007/s12041-013-0285-4
Mukherjee, K., Choudhury, A. R., Gupta, B., Gupta, S., Sengupta, D. N. (2006). An ABRE-binding factor, OSBZ8, is highly expressed in salt tolerant cultivars than in salt sensitive cultivars of indica rice. BMC Plant Biol. 6, 14. doi: 10.1186/1471-2229-6-18
Munns, R. (2011). “Plant adaptations to salt and water stress: differences and commonalities,” in Plant responses to drought and salinity stress: developments in a post-genomic era. Ed. Turkan, I. (London: Academic Press Ltd-Elsevier Science Ltd), 1–32.
Nakamura, A., Fukuda, A., Sakai, S., Tanaka, Y. (2006). Molecular cloning, functional expression and subcellular localization of two putative vacuolar voltage-gated chloride channels in rice (Oryza sativa l.). Plant Cell Physiol. 47 (1), 32–42. doi: 10.1093/pcp/pci220
Nam, M. H., Bang, E., Kwon, T. Y., Kim, Y., Kim, E. H., Cho, K., et al. (2015). Metabolite profiling of diverse rice germplasm and identification of conserved metabolic markers of rice roots in response to long-term mild salinity stress. Int. J. Mol. Sci. 16 (9), 21959–21974. doi: 10.3390/ijms160921959
Ouhibi, C., Attia, H., Rebah, F., Msilini, N., Chebbi, M., Aarrouf, J., et al. (2014). Salt stress mitigation by seed priming with UV-c in lettuce plants: growth, antioxidant activity and phenolic compounds. Plant Physiol. Biochem. 83, 126–133. doi: 10.1016/j.plaphy.2014.07.019
Qadir, M., Quillerou, E., Nangia, V., Murtaza, G., Singh, M., Thomas, R. J., et al. (2014). Economics of salt-induced land degradation and restoration. Natural Resour. Forum 38 (4), 282–295. doi: 10.1111/1477-8947.12054
Rahman, A., Nahar, K., Hasanuzzaman, M., Fujita, M. (2016). Calcium supplementation improves Na+/K+ ratio, antioxidant defense and glyoxalase systems in salt-stressed rice seedlings. Front. Plant Sci. 7. doi: 10.3389/fpls.2016.00609
Ren, Z. H., Gao, J. P., Li, L. G., Cai, X. L., Huang, W., Chao, D. Y., et al. (2005). A rice quantitative trait locus for salt tolerance encodes a sodium transporter. Nat. Genet. 37 (10), 1141–1146. doi: 10.1038/ng1643
Sabouri, H., Sabouri, A. (2008). New evidence of QTLs attributed to salinity tolerance in rice. Afr. J. Biotechnol. 7 (24), 4376–4383. doi: 10.5897/AJB08.667
Shen, J. B., Lv, B., Luo, L. Q., He, J. M., Mao, C. J., Xi, D. D., et al. (2017). The NAC-type transcription factor OsNAC2 regulates ABA-dependent genes and abiotic stress tolerance in rice (vol 7, 40641, 2017). Sci. Rep. 7, 2. doi: 10.1038/srep46890
Tang, Z. B., Gao, X. Y., Zhan, X. Y., Fang, N. Y., Wang, R. Q., Zhan, C. F., et al. (2021). Natural variation in OsGASR7 regulates grain length in rice. Plant Biotechnol. J. 19 (1), 14–16. doi: 10.1111/pbi.13436
Tong, H. H., Mei, H. W., Yu, X. Q., Xu, X. Y., Li, M. S., Zhang, S. Q., et al. (2006). Identification of related QTLs at late developmental stage in rice (Oryza sativa l.) under two nitrogen levels. Yi Chuan Xue Bao. 33 (5), 458–467. doi: 10.1016/s0379-4172(06)60073-5
Tuncturk, M., Tuncturk, R., Yasar, F. (2008). Changes in micronutrients, dry weight and plant growth of soybean (Glycine max l. Merrill) cultivars under salt stress. Afr. J. Biotechnol. 7 (11), 1650–1654. doi: 10.5897/AJB08.248
Wang, Z. F., Cheng, J. P., Chen, Z. W., Huang, J., Bao, Y. M., Wang, J. F., et al. (2012). Identification of QTLs with main, epistatic and QTL x environment interaction effects for salt tolerance in rice seedlings under different salinity conditions. Theor. Appl. Genet. 125 (4), 807–815. doi: 10.1007/s00122-012-1873-z
Wu, X., Li, Y. X., Shi, Y. S., Song, Y. C., Zhang, D. F., Li, C. H., et al. (2016). Joint-linkage mapping and GWAS reveal extensive genetic loci that regulate male inflorescence size in maize. Plant Biotechnol. J. 14 (7), 1551–1562. doi: 10.1111/pbi.12519
Xia, K. F., Wang, R., Ou, X. J., Fang, Z. M., Tian, C. G., Duan, J., et al. (2012). OsTIR1 and OsAFB2 downregulation via OsmiR393 overexpression leads to more tillers, early flowering and less tolerance to salt and drought in rice. PLoS One 7 (1), 364–373. doi: 10.1371/journal.pone.0030039
Yu, J., Zao, W. G., He, Q., Kim, T. S., Park, Y. J. (2017). Genome-wide association study and gene set analysis for understanding candidate genes involved in salt tolerance at the rice seedling stage. Mol. Genet. Genomics 292 (6), 1391–1403. doi: 10.1007/s00438-017-1354-9
Zhang, W., Liao, X. L., Cui, Y. M., Ma, W. Y., Zhang, X. N., Du, H. Y., et al. (2019). A cation diffusion facilitator, GmCDF1, negatively regulates salt tolerance in soybean. PloS Genet. 15 (1), 27. doi: 10.1371/journal.pgen.1007798
Zheng, H. L., Sun, S. C., Bai, L. M., Jiang, S. K., Ding, G. H., Wang, T. T., et al. (2022). Identification of candidate genes for panicle length in oryza sativa l. ssp. japonica via genome-wide association study and linkage mapping. Euphytica 218 (2), 12. doi: 10.1007/s10681-022-02972-7
Zhou, Y. B., Liu, C., Tang, D. Y., Yan, L., Wang, D., Yang, Y. Z., et al. (2018). The receptor-like cytoplasmic kinase STRK1 phosphorylates and activates CatC, thereby regulating H2O2 homeostasis and improving salt tolerance in rice. Plant Cell 30 (5), 1100–1118. doi: 10.1105/tpc.17.01000
Keywords: Japonica rice, GWAS, linkage mapping, salinity tolerance, seedling stage
Citation: Xu S, Cui J, Cao H, Liang S, Ma T, Liu H, Wang J, Yang L, Xin W, Jia Y, Zou D and Zheng H (2023) Identification of candidate genes for salinity tolerance in Japonica rice at the seedling stage based on genome-wide association study and linkage mapping. Front. Plant Sci. 14:1184416. doi: 10.3389/fpls.2023.1184416
Received: 11 March 2023; Accepted: 11 April 2023;
Published: 10 May 2023.
Edited by:
Xueyong Li, Institute of Crop Sciences (CAAS), ChinaReviewed by:
Rao YuChun, Zhejiang Normal University, ChinaCopyright © 2023 Xu, Cui, Cao, Liang, Ma, Liu, Wang, Yang, Xin, Jia, Zou and Zheng. This is an open-access article distributed under the terms of the Creative Commons Attribution License (CC BY). The use, distribution or reproduction in other forums is permitted, provided the original author(s) and the copyright owner(s) are credited and that the original publication in this journal is cited, in accordance with accepted academic practice. No use, distribution or reproduction is permitted which does not comply with these terms.
*Correspondence: Detang Zou, zoudtneau@126.com; Hongliang Zheng, zhenghongliang008@126.com
†These authors have contributed equally to this work
Disclaimer: All claims expressed in this article are solely those of the authors and do not necessarily represent those of their affiliated organizations, or those of the publisher, the editors and the reviewers. Any product that may be evaluated in this article or claim that may be made by its manufacturer is not guaranteed or endorsed by the publisher.
Research integrity at Frontiers
Learn more about the work of our research integrity team to safeguard the quality of each article we publish.