- 1School of Liquor and Food Engineering, Guizhou University, Guiyang, Guizhou, China
- 2Qiandongnan Engineering and Technology Research Center for Comprehensive Utilization of National Medicine, Kaili University, Kaili, China
Cucumber mosaic virus (CMV), one of the main viruses, is responsible for Passiflora spp. (passion fruit) virus diseases, which negatively affect its planting, cultivation, and commercial quality. In this study, a laboratory anti-CMV activity screening model for Passiflora spp. CMV disease was first established. Then, the effects of different antiviral agents of chitosan oligosaccharide (COS), dufulin (DFL), and ningnanmycin (Ning) on CMV virulence rate in Passiflora spp. were determined. The virulence rate and anti-CMV activity in Passiflora spp. treated with COS were 50% and 45.48%, respectively, which were even better than those of DFL (66.67% and 27.30%, respectively) and Ning (83.30% and 9.17%, respectively). Field trials test results showed COS revealed better average control efficiency (47.35%) against Passiflora spp. CMV disease than those of DFL (40.93%) and Ning (33.82%), indicating that COS is effective in the control of the Passiflora spp. CMV disease. Meanwhile, the nutritional quality test results showed that COS could increase the contents of soluble solids, titratable acids, vitamin C, and soluble proteins in Passiflora spp. fruits as well as enhance the polyphenol oxidase (PPO), superoxide dismutase (SOD), and peroxidase (POD) activity in the leaves of Passiflora spp. seedlings. In addition, the combined transcriptome and proteome analysis results showed that COS mainly acted on the Brassinosteroids (BRs) cell signaling pathway, one of plant hormone signal transduction pathway, in Passiflora spp., thus activating the up-regulated expression of TCH4 and CYCD3 genes to improve the resistance to CMV disease. Therefore, our study results demonstrated that COS could be used as a potential plant immune inducer to control the Passiflora spp. CMV disease in the future.
1 Introduction
Virus disease, the most common disease in Passiflora spp. (passion fruit), could affect the yield and quality of Passiflora spp. fruits, thus creating a great threat to the development of Passiflora spp. industry (Balendres and Bengoa, 2019; Chen et al., 2021). More than 20 kinds of viruses have been currently identified that could cause Passiflora spp. virus disease (Ye et al., 2019). Of which, CMV infection can adversely affect or regulate the phytochemical content of Passiflora spp., which will further damage the growth of Passiflora spp. (Morales et al., 2002; Yeturu et al., 2017; Mesa et al., 2018; da Cruz et al., 2019; Munguti et al., 2019; Chen et al., 2021; Fu et al., 2021; Huang et al., 2022). However, until now, there are a wide variety of antiviral commercial agents such as chitosan oligosaccharide (COS), dufulin (DFL), and ningnanmycin (Ning) on the market, but the agents mainly targeting virus disease of Passiflora spp. caused by CMV have hardly been reported. On another side, the interaction relationship between antiviral commercial agents and Passiflora spp. is still vague, resulting in the lack of high-efficiency prevention and control methods.
COS, a chitosan derivative, has been widely applied in diverse fields due to the advantages of low molecular weight, harmless, rapid absorption, and non-toxic stability (Guan and Feng, 2022). Previous studies have shown that COS could promote plant growth, increase the antioxidant enzyme activity, and activate plant resistance (Yuan et al., 2019; Ahmed et al., 2020; Liu et al., 2021; Li et al., 2022a; Yu, 2015). In recent years, COS has been widely applied to control a variety of plant diseases and insect pests, such as Peronospora parasitica on Chinese cabbage (Luo and Yan, 2022), wheat powdery mildew (Wu et al., 2022), potato virus Y disease (Yang et al., 2022), kiwifruit soft rot (He et al., 2021), and so on. However, there have been no report on COS used to control the Passiflora spp. virus disease caused by CMV.
Proteomics and transcriptome sequencing technologies play a significant role in understanding the protein and gene expression in organism and are one of the best ways to characterize candidate action targets for biological functions (Morozova et al., 2009; Kumar et al., 2016). The difference in protein and gene expression related to biological control process could be identified by the proteomics and transcriptome methods, which are conducive to understanding the mechanism of disease resistance and laying the theoretical foundation for the further prevention and control of viral diseases (Wang et al., 2017; Ji et al., 2019; Wei et al., 2022). Furthermore, a combination of proteomics and transcriptome analysis is greatly helpful to clarify the pathogenicity information and understand the mechanism of action of differentially expressed genes (DEGs) and differentially expressed proteins (DEPs) in related pathways (Kirsch et al., 2012).
In order to find an effective antiviral commercial agent against Passiflora spp. CMV disease, in this study, the in laboratory anti-CMV activity screening test and field trials test of COS, DFL, and Ning against Passiflora spp. CMV disease were determined. Our results showed that COS is effective in the control of the Passiflora spp. CMV disease. Meanwhile, in order to understand the mechanism of action of COS against Passiflora spp. CMV disease, the combined transcriptome and proteome analysis was also performed.
2 Materials and methods
2.1 Model construction of anti-CMV activity test
In June 2021, the branches of Passiflora spp., treated with root powder beforehand, were placed in flowerpots with nutrient soil. After 1 mouth, the Passiflora spp. seedlings with uniform growth were selected to construct the laboratory screening model for anti-CMV activity test by mechanical friction inoculation method (Yael et al., 2006; Zhou et al., 2012). The solutions of 5% chitosan oligosaccharide AS (COS, 750-fold dilution), 30% dufulin WP (DFL, 1000-fold dilution), and 8% ningnanmycin AS (Ning, 600-fold dilution) diluted with distilled water were sprayed on the whole Passiflora spp. seedlings, respectively. Five Passiflora spp. seedlings were treated as one group, each group was repeated for three times. The distilled water was served as the blank control (CK group). After 2 days of spraying, the leaves of Passiflora spp. seedlings were inoculated with CMV using a brush dipped and subsequently washed with water. Then, the Passiflora spp. seedlings were grown in light incubators with the constant humidity of 90% at 28 °C for 14 h during the day and 26 °C for 10 h at night. After 7 days of inoculation, the leaves of Passiflora spp. seedlings were selected and stored at –80 °C for the subsequent laboratory anti-CMV activity experiments.
2.2 Laboratory anti-CMV activity test
Total RNA of the leaves of Passiflora spp. seedlings was extracted according to the manufacturer’s instructions of the plant RNA rapid extraction kit (ComWin Biotech Co., Ltd., Beijing, China). cDNA was reverse-transcribed according to the manufacturer’s instructions of a Goldenstar™ RT6 cDNA synthesis kit (Tsingke Biotechnology, Beijing, China). After that, the cDNAs were amplified by the polymerase chain reaction (PCR) analysis with the forward primers of 5’-ATGGACAAATCTGAATCAACCAGTGC-3’ and reverse primer of 5’-TCAGACTGGGAGCACCCCAGAC-3’. The amplification conditions were set at 94 °C for 2 min, followed by 35 cycles of 30 s at 94 °C, 30 s at 58 °C, and 70 s at 72 °C, and extended at 72°C and 12°C for 10 min, respectively. After that, the PCR products were electrophoresed on 0.75% agarose gel for 30 min to calculate the virulence rate and anti-CMV activity according to the reported method (Kang et al., 2021).
2.3 Field trials test
In 2021, the field trials of COS (750-fold dilution), DFL (1000-fold dilution), and Ning (600-fold dilution) against on Passiflora spp. CMV disease were performed in Zhenning city. Sterile distilled water was served as the blank control (CK group). On the 7th day after the first, second, and third spraying, the control efficiencies of COS, DFL, and Ning were calculated by the reported method (Montasser et al., 1998).
2.4 Nutritional quality test of Passiflora spp. fruits
To investigate the effect of COS on the nutritional quality of Passiflora spp. fruits, herein, the soluble solids, titratable acids, vitamin C, and soluble proteins contents of Passiflora spp. fruits collected on the 7th day after the third spraying were determined according to the reported methods (da Rocha et al., 2022; Xu et al., 2022; Yang et al., 2022; Zhang et al., 2022).
2.5 Determination of antioxidant enzyme activity
The antioxidant enzyme activities of peroxidase (POD), superoxide dismutase (SOD), and polyphenol oxidase (PPO) in the leaves of Passiflora spp. seedlings of COS treatment and CK groups on the 7th day after spraying were determined using the commercially available enzyme assay reagent kits (Suzhou Comin Bioengineering Institute, Suzhou, China).
2.6 Transcriptome sequencing
The Passiflora spp. seedlings with uniform growth were selected and the solution of 5% COS AS (750-fold dilution) were sprayed on the whole Passiflora spp. seedlings. After 7 days of spraying, the leaves of Passiflora spp. seedlings were collected. Transcriptome sequencing of the leaves of Passiflora spp. seedlings were completed by Hangzhou Lianchuan Biological Co., LTD. and sequenced using the Illumina HiSeq™ 2000 (Illumina Inc., San Diego, CA, USA). The raw data were deposit at National Center for Biotechnology Information (NCBI, https://www.ncbi.nlm.nih.gov/) database with the IDs of GSM6813002 and GSM6813001, respectively. To obtain high-quality reads, Cutadapt v1.9.3 software was used to remove the low-quality and undefined reads (Qu et al., 2021). HISAT was used to compare the reference genome of the preprocessed valid data (Kim et al., 2015). The gene expression was defined based on the FPKM value (Moon and Zhao, 2021). Salmon was used to calculate the expression level of Unigenes (Li et al., 2022a). R language package was used to identify DEGs (p < 0.05 and -log2FC > 1) (Zhang et al., 2019). Then, Gene Ontology (GO) annotation (named biological processes (BP), cellular components (CC), and molecular function (MF)) and Kyoto Encyclopedia of Genes and Genomes (KEGG) pathway enrichment for the DEGs were carried out at http://www.geneontology.org/ and http://www.genome.jp/Pathway, respectively (Yu et al., 2018).
2.7 Proteomics sequencing
The Passiflora spp. seedlings with uniform growth were selected and the solution of 5% COS AS (750-fold dilution) were sprayed on the whole Passiflora spp. seedlings. After 7 days of spraying, the leaves of Passiflora spp. seedlings were collected for proteomics analysis according to our reported method (Gao et al., 2017). The raw data were deposited to the ProteomeXchange Consortium (http://proteomecentral.proteomexchange.org) with the ID of PXD038881. The raw data were quantified by the MaxQuant software (version 1.5.8.3) (Cox et al., 2011; Yu et al., 2018). DEPs (expression level > 2.0-fold, p < 0.01) were identified from the Uniprot database (http://www.uniprot.org/). Then, GO annotation related at three ontologies (namely BP, CC, and MF) and KEGG pathway enrichment for the DEPs were carried out at http://www.geneontology.org/and http://www.genome.jp/Pathway, respectively (Yu et al., 2018).
2.8 Statistical analysis
The results of the nutritional quality in the fruits of Passiflora spp. and antioxidant enzyme activity in the leaves of Passiflora spp. seedlings were statistically analyzed using one-way ANOVA followed by LSD test using Origin 2021.
3 Results and discussion
3.1 Results of laboratory anti-CMV activity and field trials tests
Virus disease is the most common type of disease in Passiflora spp. and is also known as a phloem disease (Fischer and Rezende, 2008). Once infected by the virus disease, it can cause the whole plant of Passiflora spp. not to bloom and fruit, resulting in a continuous reduction in the yield (Carvalho et al., 2021). Unfortunately, it is well-known that the antiviral agents that are currently available on the market cannot effectively control virus disease, and the mechanism of action between antiviral agents and virus disease has not been clarified, resulting in the research on the control of Passiflora spp. virus disease becoming difficult. In this study, a laboratory anti-CMV activity screening model for CMV disease of Passiflora spp. seedlings was first established and the effects of COS, DFL, and Ning on CMV virulence rates in Passiflora spp. seedlings were determined and the results were listed in Table 1. Table 1 showed that the virulence rate and anti-CMV activity in Passiflora spp. treated with COS were 50.00% and 45.48%, respectively, which were superior to those of DFL (66.67% and 27.30%, respectively) and Ning (83.30% and 9.17%, respectively), demonstrating that COS could effectively control the CMV disease in Passiflora spp. seedlings. Meanwhile, to study the control efficiency of COS, DFL, and Ning on the control of CMV disease of Passiflora spp., a preliminary study on the control of Passiflora spp. CMV disease was conducted through field efficacy trials. As shown in Table 2, on the 7th day after the first, second and third spraying, COS was effective in reducing Passiflora spp. CMV disease in the field (the average control efficiency is 47.35%) relative to those of DFL (40.93%) and Ning (33.82%). In the past few years, many studies had demonstrated that COS revealed good antiviral activity. Yu et al. (2002) and Li et al. (2019) reported that COS had obvious control efficiency on CMV disease in cucumber and tobacco. There are fewer studies related to the control of Passiflora spp. virus disease with antiviral agents. Kitajima et al. (2003) and Crestani et al. (1986) reported the first outbreak of Passiflora spp. virus disease in Brazil, and it was demonstrated experimentally that the incidence of the disease could be significantly reduced by the following antiviral agents hexythiazox, quinomethionate, dicofol, propargite, or fenbutatin-oxide.
3.2 Results of nutritional quality of Passiflora spp. fruits
In recent years, as the people attach increasing importance to their health, the nutritional quality of fruits has been widely requested by consumers (Wang et al., 2020). As known to all, nutritional quality indexes, such as soluble solids, titratable acids, vitamin C, and soluble proteins contents, are the most important factors on fruit quality, the taste and flavor of fruits, and the consumer purchasing decision (Huang et al., 2021). Soluble solids content, an important index of fruit ripening process and economic benefits, is associated with fruit taste and harvest time (Li et al., 2016; Han et al., 2017). As shown in Figure 1A, COS could increase the content of soluble solids in Passiflora spp. fruits. Titratable acids are the main index affecting fruit taste and the substrate of respiration metabolism (Guo et al., 2022). After COS treatment, as shown in Figure 1B, the increase of titratable acids content could a certain extent improve the Passiflora spp. fruits respiration. Vitamin C, one of the nutritional components, is an important antioxidant in fruits to reduce the damage to cell membranes (Nair et al., 2018). Figure 1C showed that COS treatment could significantly increase the vitamin C content in Passiflora spp. fruits which not only improved the nutritional quality of fruit, but also increased the disease resistance. Soluble proteins might be attributed to hydrolysis of cell membranes induced by ripening. Reactive oxygen species (ROS) damage and fungal infection could cause the decrease trend of soluble protein in the fruit (Scandalios, 1993; Alia-Tejacal et al., 2007). Figure 1D showed that COS could increase the content of soluble proteins. To sum up, COS could improve the taste and flavor of Passiflora spp. fruits. Similar results were also reported by He et al. (2018), they found that COS could significantly increase the contents of soluble solids, titratable acids, vitamin C, and soluble proteins in strawberry.
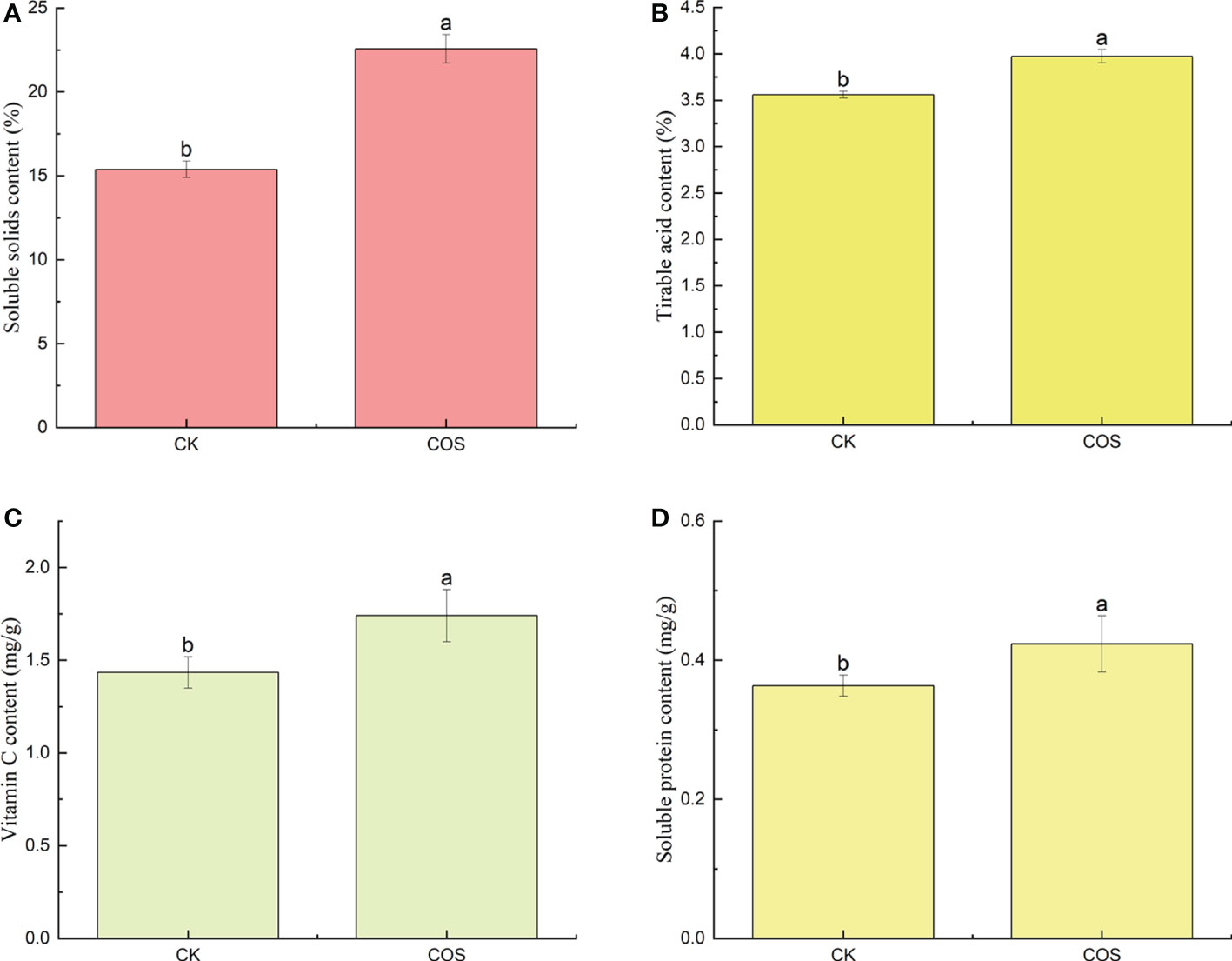
Figure 1 Effect of COS on the soluble solids (A), titratable acids (B), vitamin C (C), and soluble proteins (D) contents of Passiflora spp. fruits. Different lowercase letters indicated the effect of COS on the soluble solids, titratable acids, vitamin C, and soluble proteins contents of Passiflora spp. fruits with significant difference compared with CK group (p < 0.05).
3.3 Effect on the antioxidant enzyme activity
Plant disease resistance is associated with the activation of a series of defense responses, such as induce defense responses in the form of enzymes, that slow down or stop infection during certain stages of host-pathogen interaction. POD can induce the biosynthetic pathway of salicylic acid (SA) to activate the systemic acquired resistance (SAR), thus enhancing the tolerance to pathogenic microorganism infection (Wu et al., 2013; Yang et al., 2019). SOD is a key enzyme of oxygen metabolism in plants, which plays a critical role in eliminating superoxide free radicals, alleviating lipid peroxidation and membrane damage under stress (Díaz et al., 2001). PPO activity plays an important role in plant defense mechanisms which can help the plant resist adversity and develop adaptive strategies to enhance plant resilience (Taranto et al., 2021). In this study, to clarify the effect of COS on the antioxidant enzyme activity in the leaves of Passiflora spp. seedlings, the POD, SOD, and PPO activity were studied. As shown in Figure 2, after 7 days of spraying, COS could increase the POD, SOD, and PPO activity in the leaves of Passiflora spp. seedlings to activate the antioxidant defense system in Passiflora spp. seedlings against CMV disease. Liu et al. (2021) reported that COS could significantly increase the antioxidant enzyme (POD, SOD, and PPO) activity to promote the growth of wheat. Li et al. (2022b) found that the application of physcion and COS combination is more effective in facilitating the SOD, POD activities of the maize plants, resulting in plants with high stress resistance. Ou et al. (2022) reported that COS could enhance the antioxidant activity of SOD and POD in tea plants.
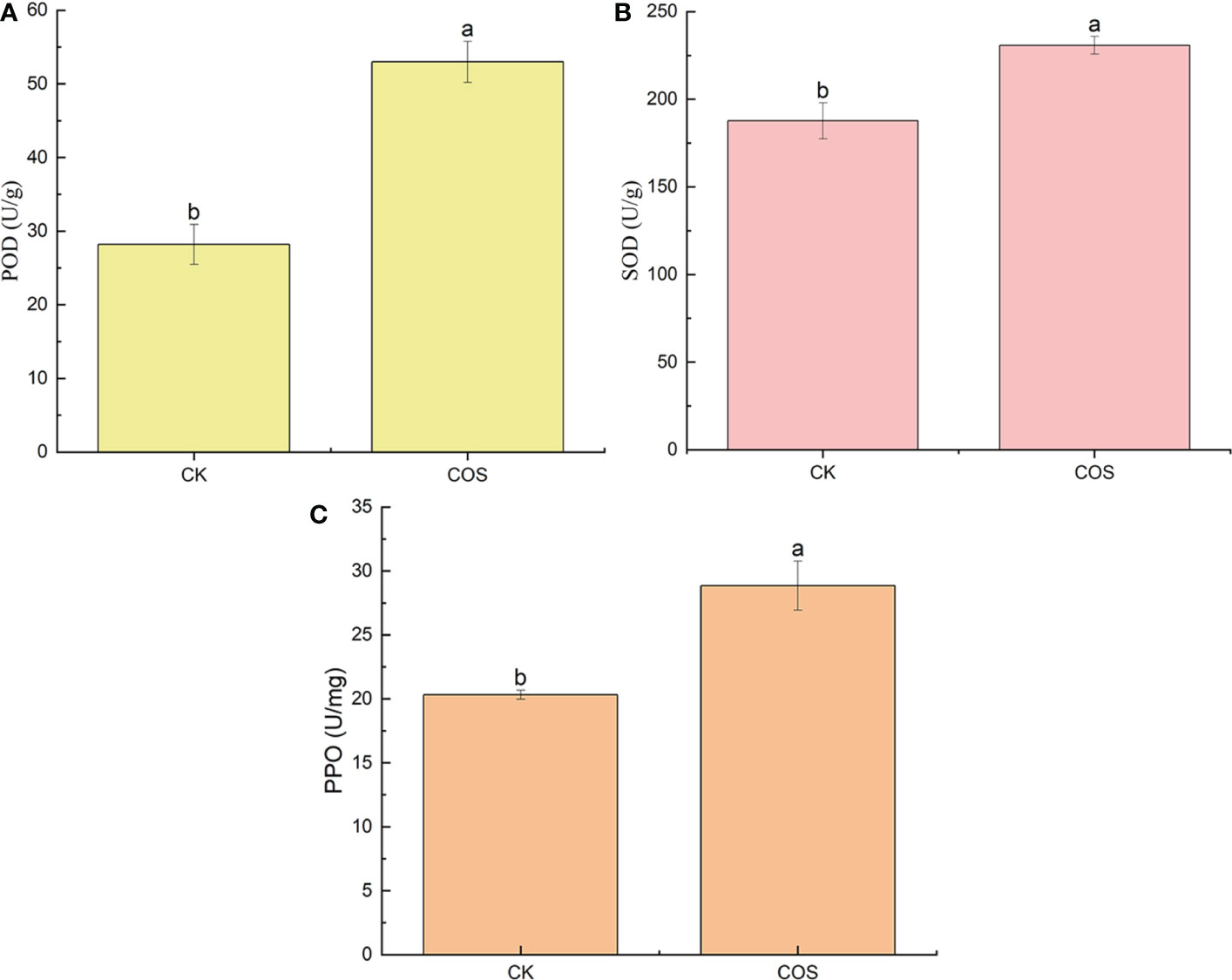
Figure 2 Effect of COS on the POD (A), SOD (B), and PPO (C) activity in the leaves of Passiflora spp. seedlings. Different lowercase letters indicated the effect of COS on the POD, SOD, and PPO activity in the leaves of Passiflora spp. seedlings with significant difference compared with CK group (p < 0.05).
3.4 Quality check of transcriptome sequencing data
The cDNA libraries of the leaves of Passiflora spp. seedlings of COS treatment and CK groups were characterized using Illumina HiSeq platform to detect the transcriptome level of gene expression information according to the reported methods (Chen et al., 2014; Wang et al., 2022). The data obtained by up-sequencing were removed from sequencing junctions and low-quality, and the reads with unidentifiable base information and the proportion of more than 5% were filtered out. The original sequencing volume (raw data), effective sequencing volume (valid data), as well as the contents of Q20, Q30 and GC were counted and the summary results were displayed in Table 3. Table 3 showed that the transcriptome sequencing data were of high quality for the subsequent bioinformatics analysis with the valid ratios for both samples > 92%, the proportions of Q20 and Q30 bases for both samples > 97%, and the GC contents for both samples > 44%.
3.5 Results of DEGs and bioinformatic analysis
Compared COS treatment group with CK group, a total of 911 DEGs were obtained in the transcriptome sequencing (Table S1), of which, the up- and down-regulated genes were 557 and 354, respectively. Meanwhile, Figure 3 showed that DEGs between the COS treatment and CK groups were analyzed for GO categories in BP (regulation of transcription, DNA-templated (GO:0006355), transcription, DNA-templated (GO:0006351), oxidation-reduction process (GO:0055114), protein phosphorylation (GO:0006468), multicellular organism development (GO:0007275), phosphorylation (GO:0016310), signal transduction (GO:0007165), response to salt stress (GO:0009651), cell wall organization (GO:0071555), and lipid catabolic process (GO:0016042)), CC (nucleus (GO:0005634), plasma membrane (GO:0005886), integral component of membrane (GO:0016021), cytoplasm (GO:0005737), extracellular region (GO:0005576), chloroplast (GO:0009507), cytosol (GO:0005829), membrane (GO:0016020), mitochondrion (GO:0005739), and cell wall (GO:0005618)), and MF (protein binding (GO:0005515), DNA-binding transcription factor activity (GO:0003700), ATP binding (GO:0005524), DNA binding (GO:0003677), metal ion binding (GO:0046872), kinase activity (GO:0016301), protein serine/threonine kinase activity (GO:0004674), sequence-specific DNA binding (GO:0043565), carboxylic ester hydrolase activity (GO:0052689), and hydrolase activity (GO:0016787)).
In addition, Figure 4 showed that the top 20 enriched KEGG pathways of the DEGs were plant hormone signal transduction (ko04075), pentose and glucuronate interconversions (ko 00040), glycerolipid metabolism (ko00561), phenylpropanoid biosynthesis (ko00940), starch and sucrose metabolism (ko00500), plant-pathogen interaction (ko04626), MAPK signaling pathway–plant (ko04016), cysteine and methionine metabolism (ko00270), ABC transporters (ko02010), cutin, suberine and wax biosynthesis (ko00073), glycolysis/gluconeogenesis (ko00010), photosynthesis (ko00195), glycerophospholipid metabolism (ko00564), oxidative phosphorylation (ko00190), protein processing in endoplasmic reticulum (ko04141), ribosome (ko03010), sulfur metabolism (ko00920), other glycan degradation (ko00511), sphingolipid metabolism (ko00600), and fatty acid degradation (ko00071).
3.6 Results of DEPs and bioinformatic analysis
A total of 667 proteins were identified, of which, the up- and down-regulated proteins were 42 and 30, respectively, in COS treatment group compared to the CK group (Table S2). Meanwhile, as shown in Figure 5, the GO term enrichment analysis revealed that the DEPs were mainly involved in the following enrichment categories: translation (GO:0006412), isoleucine biosynthetic process (GO:0009097), ubiquitin-dependent protein catabolic process (GO:0006511), glucose metabolic process (GO:0006006), and response to oxidative stress (GO:0006979) in terms of BP category; ten categories, namely, nucleus (GO:0005634), cytoplasm (GO:0005737), cytosol (GO:0005829), mitochondrion (GO:0005739), chloroplast (GO:0009507), chloroplast thylakoid membrane (GO:0009535), chloroplast stroma (GO:0009570), and cell wall (GO:0005618) in terms of CC category; ten categories, namely, protein binding (GO:0005515), copper ion binding (GO:0005507), ATP binding (GO:0005524), DNA binding (GO:0003677), RNA binding (GO:0003723), mRNA binding (GO:0003729), translation elongation factor activity (GO:0003746), structural constituent of ribosome (GO:0003735), and GTPase activity (GO:0003924) in terms of MF category.
In addition, the KEGG pathways of the DEPs were also identified. Figure 6 showed that the top twenty KEGG pathways were ribosome (ko03010), photosynthesis (ko00195), glycolysis/gluconeogenesis (ko00010), glycine, serine and threonine metabolism (ko00260), glutathione metabolism (ko00480), oxidative phosphorylation (ko00190), RNA transport (ko03013), galactose metabolism (ko00052), valine, leucine and isoleucine biosynthesis (ko00290), cyanoamino acid metabolism (ko00460), pantothenate and CoA biosynthesis (ko00770), pyrimidine metabolism (ko00240), phenylpropanoid biosynthesis (ko00940), ascorbate and aldarate metabolism (ko00053), carbon fixation in photosynthetic organisms (ko00710), and protein processing in endoplasmic reticulum (ko04141).
3.7 Combined analysis of transcriptomic and proteomic technology
The combined transcriptome and proteome analysis results showed that COS mainly affected the Brassinosteroids (BRs) cell signaling pathway in Passiflora spp. to improve the resistance to CMV disease. BRs cell signaling pathway is a class of plant hormone signal transduction, which plays diverse roles in plant growth and development (Hecht et al., 2001; Li et al., 2002; Zipfel, 2008; Yang et al., 2011). As shown in Figure 7, after COS treatment, the gene expression of cell membrane surface receptor kinase Brassinosteroid insensitive 1 (BRI1) was stimulated, and the gene and protein expression of its negative regulatory protein BRI1 kinase inhibitor 1 (BKI1) were up-regulated, thus inhibiting the BRs cell signaling pathway and then making it unable to complete phosphorylation signal transduction to form Brassinosteroid insensitive 2 (BIN2) to inhibit the transcription factor BZR1 and BZR2 (BZR1/2). After that, BZR1/2 could bind to specific regions on promotors of the downstream target genes to activate the up-regulated expression of TCH4 and CYCD3 genes to improve the disease resistance. TCH4 gene plays an important role in the biological processes such as cell elongation, resistance to disease, and adaptation to environmental stress in plants (Zhang et al., 2008; Friedrichsen and Chory, 2001; Xu et al., 2001). Studies have demonstrated that CYCD3 gene, involved in the BRs cell signaling pathway, is involved in cell division and may play an important role in blocking pathogen infection by regulating cell wall remodeling in the early stage of resistant varieties (Hu et al., 2000; Yan et al., 2021).
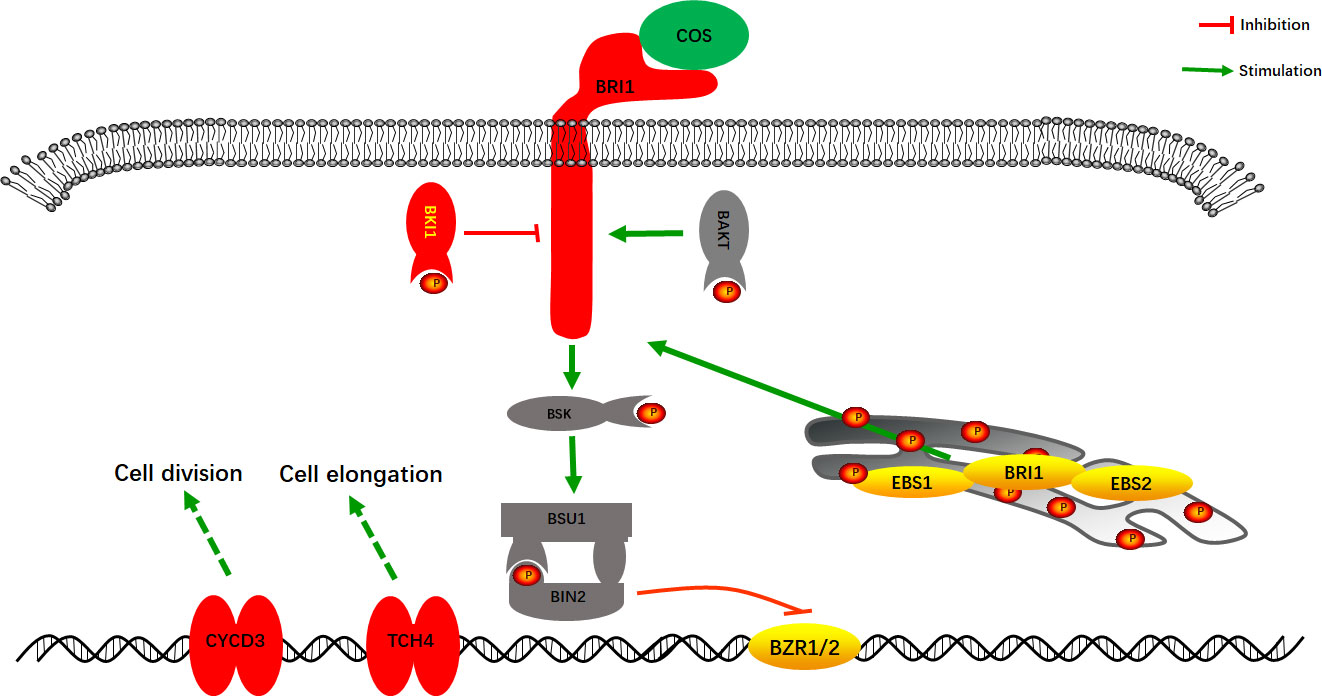
Figure 7 A model of the BRs cell signaling pathway in Passiflora spp. response to COS. Red, up-regulated expression; Yellow, down-regulated expression; P, phosphorylation.
4 Conclusions
In conclusion, our study results demonstrated that COS revealed an effective control efficiency against CMV disease of Passiflora spp. and could be used as a potential plant immune inducer by mainly acting on the BRs cell signaling pathway in Passiflora spp. to improve the resistance to CMV disease. Therefore, this study lays the foundation for a more in-depth study of the molecular mechanism of disease resistance in Passiflora spp. and provides a theoretical basis for application effect of COS as a plant immune inducer on the Passiflora spp. CMV disease.
Data availability statement
The datasets presented in this study can be found in online repositories. The names of the repository/repositories and accession number(s) can be found in the article.
Author contributions
Methodology, LZ and ZZ. Data analysis, LZ and ZZ. Writing—review and editing, LY, PL, and ST. Funding acquisition, LY and PL. All authors contributed to the article and approved the submitted version.
Funding
This research was funded by the National Natural Science Foundation of China, grant number 32102267, the Guizhou Provincial Science and Technology Projects, grant number [2020]1Y130, ZK[2021]137, and ZK[2022]138.
Conflict of interest
The authors declare that the research was conducted in the absence of any commercial or financial relationships that could be construed as a potential conflict of interest.
Publisher’s note
All claims expressed in this article are solely those of the authors and do not necessarily represent those of their affiliated organizations, or those of the publisher, the editors and the reviewers. Any product that may be evaluated in this article, or claim that may be made by its manufacturer, is not guaranteed or endorsed by the publisher.
Supplementary material
The Supplementary Material for this article can be found online at: https://www.frontiersin.org/articles/10.3389/fpls.2023.1131766/full#supplementary-material
References
Ahmed, K. B. M., Khan, M. A., Siddiqui, H., Jahan, A. (2020). Chitosan and its oligosaccharides, a promising option for sustainable crop production-a review. Carbohyd. Polym. 227, 115331. doi: 10.1016/j.carbpol.2019.115331
Alia-Tejacal, I., Villanueva-Arce, R., Pelayo-Zaldívar, C., Colinas-León, M. T., López-Martínez, V., Bautista-Baños, S. (2007). Postharvest physiology and technology of sapote mamey fruit (Pouteria sapota (Jacq.) HE Moore & stearn). Postharvest Biol. Technol. 45, 285–297. doi: 10.1016/j.postharvbio.2006.12.024
Balendres, M. A., Bengoa, J. C. (2019). Diseases of dragon fruit (Hylocereus species): Etiology and current management options. Crop Prot. 126, 104920. doi: 10.1016/j.cropro.2019.104920
Carvalho, B. M., Viana, A. P., da Silva, F. A., dos Santos, P. H. D., Eiras, M., Santos, E. A. (2021) How segregating populations of passion fruit react to CABMV infection? Eur. J. Plant Pathol. 160, 855–866. doi: 10.1007/s10658-021-02289-6
Chen, C. M., Khaleel, S. S., Huang, H. Z., Wu, C. H. (2014). Software for pre-processing illumina next-generation sequencing short read sequences. Source Code Biol. Med. 9, 8. doi: 10.1186/1751-0473-9-8
Chen, L. J., Sun, D. L., Zhang, X. X., Shao, D. Q., Lu, Y. L., An, Y. X. (2021). Transcriptome analysis of yellow passion fruit in response to cucumber mosaic virus infection. PloS One 16 (2), e0247127. doi: 10.1371/journal.pone.0247127
Cox, J., Neuhauser, N., Michalski, A., Scheltema, R. A., Olsen, J. V., Mann, M. (2011). Andromeda: a peptide search engine integrated into the MaxQuant environment. J. Proteome Res. 10 (4), 1794–1805. doi: 10.1021/pr101065j
Crestani, O. A., Kitajima, E. W., Lin, M. T., Marinho, V. L. A. (1986). Passion fruit yellow mosaic virus, a new tymovirus found in Brazil. Phytopathology 76, 951–955. doi: 10.1094/Phyto-76-951
da Cruz, M. P., Mazaro, S. M., Bruzamarello, J., de Souza Vismara, E., Ghedin, Á.L., Possenti, J. C., et al. (2019). Bioactive compounds of ganoderma lucidum activate the defense mechanisms of soybean plants and reduce the severity of powdery mildew. J. Agric. Sci. 11 (13), 99–114. doi: 10.5539/jas.v11n13p99
da Rocha, T. S., de Lima, A., Silva, J., d., N., Sampaio, G. R., Soares Freitas, R. A. M., et al. (2022). Vitamin c and phenolic antioxidants of jua (Ziziphus joazeiro m.) pulp: A rich underexplored Brazilian source of ellagic acid recovered by aqueous ultrasound-assisted extraction. Molecules 27, 627. doi: 10.3390/molecules27030627
Díaz, J., Bernal, M., Pomar, F., Merino, F. (2001). Induction of shikimate dehydrogenase and peroxidase in pepper (Capsicum annuum l.) seedlings in response to copper stress and its relation to lignification. Plant Sci. 161, 179–188. doi: 10.1016/S0168-9452(01)00410-1
Fischer, I. H., Rezende, J. A. M. (2008). Diseases of passion flower (Passiflora spp.). Pest Technol. 2 (1), 1–19.
Friedrichsen, D., Chory, J. (2001). Steroid signaling in plants: from the cell surface to the nucleus. Bioessays 23, 1028–1036. doi: 10.1002/bies.1148
Fu, X. D., Jiang, J. M., Luo, L. T., Du, Q. L., Li, X. Y., Afandi, A., et al. (2021). Development of reverse transcription loop-mediated isothermal amplification assay for rapid and visual detection of telosma mosaic virus (TeMV) in passion fruit. Crop Prot. 150, 105795. doi: 10.1016/j.cropro.2021.105795
Gao, M. N., Yu, L., Song, X. P., Chen, Z., He, M., Song, B. A. (2017). Label-free quantitative proteomic analysis of inhibition of Xanthomonas axonopodis pv. citri by the novel bactericide fubianezuofeng. Pestic. Biochem. Phys. 138, 37–42. doi: 10.1016/j.pestbp.2017.02.004
Guan, Z. W., Feng, Q. (2022). Chitosan and chitooligosaccharide: The promising non-plant-derived prebiotics with multiple biological activities. Int. J. Mol. Sci. 23 (12), 6761. doi: 10.3390/ijms23126761
Guo, X. M., Luo, T., Han, D. M., Zhu, D. F., Li, Z. Y., Wu, Z. Y., et al. (2022). Multi-omics analysis revealed room temperature storage affected the quality of litchi by altering carbohydrate metabolism. Sci. Hortic. 293, 110663. doi: 10.1016/j.scienta.2021.110663
Han, Q., Gao, H. Y., Chen, H. J., Fang, X. J., Wu, W. J. (2017). Precooling and ozone treatments affects postharvest quality of black mulberry (Morus nigra) fruits. Food Chem. 221, 1947–1953. doi: 10.1016/j.foodchem.2016.11.152
He, Y. Q., Bose, S. K., Wang, W. X., Jia, X. C., Lu, H., Yin, H. (2018). Pre-harvest treatment of chitosan oligosaccharides improved strawberry fruit quality. Int. J. Mol. Sci. 19, 2194. doi: 10.3390/ijms19082194
Hecht, V., Vielle-Calzada, J. P., Hartog, M. V., Schmidt, E. D., Boutilier, K., Grossniklaus, U., et al. (2001). The arabidopsis somatic embryogenesis receptor kinase 1 gene is expressed in developing ovules and embryos and enhances embryogenic competence in culture. Plant Physiol. 127, 803–816. doi: 10.1104/pp.010324
He, L. N., Shi, J. Q., Zhang, R. Q., Luo, G. H., Yuan, T., Fan, R., et al. (2021). The control effects of the binary mixture of tebuconazole and oligosaccharides on kiwifruit soft rot Botryosphaeria dothidea. world pest. 43 10), 25–29. doi: 10.16201/j.cnki.cn10-1660/tq.2021.10.05
Huang, A. J., Gu, P. P., Wang, Y., Li, J. L., Yang, Z. X., Yi, L. (2022). Simultaneous detection and differentiation of four viruses in passion fruit plants by a multiplex RT-PCR. trop. Plant Pathol. doi: 10.1007/s40858-022-00538-5
Huang, X., Wang, H. K., Luo, W. J., Xue, S., Hayat, F., Gao, Z. H. (2021). Prediction of loquat soluble solids and titratable acid content using fruit mineral elements by artificial neural network and multiple linear regression. Sci. Hortic. 278, 109873. doi: 10.1016/j.scienta.2020.109873
Hu, Y. X., Bao, F., Li, J. Y. (2000). Promotive effect of brassinosteroids on cell division involves a distinct CycD3-induction pathway in arabidopsis. Plant J. 24, 693–701. doi: 10.1046/j.1365-313x.2000.00915.x
Ji, Z., Zeng, Y. X., Liang, Y., Qian, Q., Yang, C. D. (2019). Proteomic dissection of the rice-Fusarium fujikuroi interaction and the correlation between the proteome and transcriptome under disease stress. BMC Genomics 20, 91. doi: 10.1186/s12864-019-5435-5
Kang, B. R., Park, J., Jung, W. J. (2021). Antiviral activity by lecithin-induced fengycin lipopeptides as a potent key substrate against cucumber mosaic virus. Microb. Pathogenesis 155, 104910. doi: 10.1016/j.micpath.2021.104910
Kim, D., Langmead, B., Salzberg, S. L. (2015). HISAT: a fast spliced aligner with low memory requirements. Nat. Methods 12, 357–360. doi: 10.1038/nmeth.3317
Kirsch, R., Wielsch, N., Vogel, H., Svatoš, A., David G Heckel, D. G., Pauchet, Y. (2012). Combining proteomics and transcriptome sequencing to identify active plant-cell-wall-degrading enzymes in a leaf beetle. BMC Genomics 13, 587. doi: 10.1186/1471-2164-13-587
Kitajima, E. W., Rezende, J. A. M., Rodrigues, J. C. V. (2003). Passion fruit green spot virus vectored by Brevipalpus phoenicis (Acari: Tenuipalpidae) on passion fruit in Brazil. Exp. Appl. Acarol. 30 (1), 225–231. doi: 10.1023/B:APPA.0000006551.74604.84
Kumar, D., Bansal, G., Narang, A., Basak, T., Abbas, T., Dash, D. (2016). Integrating transcriptome and proteome profiling: Strategies and applications. Proteomics 16 (19), 2533–2544. doi: 10.1002/pmic.201600140
Li, J. C., Han, A. H., Zhang, L., Meng, Y., Xu, L., Ma, F. X., et al. (2022b). Chitosan oligosaccharide alleviates the growth inhibition caused by physcion and synergistically enhances resilience in maize seedlings. Sci. Rep. 12, 162. doi: 10.1038/s41598-021-04153-3
Li, P. L., Liu, W., Lu, W. Q., Wang, J. L. (2022a). Biochemical indices, gene expression, and SNPs associated with salinity adaptation in juvenile chum salmon (Oncorhynchus keta) as determined by comparative transcriptome analysis. PeerJ 10, e13585. doi: 10.7717/peerj.13585
Li, J., Pan, A. H., Xie, M. S., Zhang, P. P., Gu, X. P. (2019). Characterization of a thermostable κ-carrageenase from a hot spring bacterium and plant protection activity of the oligosaccharide enzymolysis product. J. Sci. Food Agric. 99 (4), 1812–1819. doi: 10.1002/jsfa.9374
Li, J. L., Sun, D. W., Cheng, J. H. (2016). Recent advances in nondestructive analytical techniques for determining the total soluble solids in fruits: A review. compr. Rev. Food Sci. Food Saf. 15 (5), 897–911. doi: 10.1111/1541-4337.12217
Liu, R. Q., Li, J. C., Wang, Y. S., Zhang, F. L., Li, D. D., Ma, F. X., et al. (2021). Amino-oligosaccharide promote the growth of wheat, increased antioxidant enzymes activity. Biol. Bull. 48, 459–467. doi: 10.1134/S1062359021040099
Li, J., Wen, J., Lease, K. A., Doke, J. T., Tax, F. E., Walker, J. C. (2002). BAK1, an arabidopsis LRR receptor-like protein kinase, interacts with BRI1 and modulates brassinosteroid signaling. Cell 110, 213–222. doi: 10.1016/S0092-8674(02)00812-7
Luo, X. M., Yan, K. (2022). Control effects of oligosaccharins compounded with four chemical fungicides against Peronospora parasitica on Chinese cabbage in the field. Mod. Agrochem. 21 (4), 66–69. doi: 10.3969/j.issn.1671-5284.2022.04.013
Mesa, H. J., Montoya, M. M., Gutiérrez, P. A. (2018). Molecular characterization of Soybean mosaic virus (SMV) infecting purple passion fruit (Passiflora edulis f. edulis) in antioquia, Colombia. Arch. Phytopathol. Plant Prot. 51 (11–12), 617–636. doi: 10.1080/03235408.2018.1505411
Montasser, M. S., Tousignant, M. E., Kaper, J. M. (1998). Viral satellite RNAs for the prevention of cucumber mosaic virus (CMV) disease in field-grown pepper and melon plants. Plant Dis. 82 (12), 1298–1303. doi: 10.1094/PDIS.1998.82.12.1298
Moon, S., Zhao, Y. T. (2021). Spatial, temporal and cell-type-specific expression profiles of genes encoding heparan sulfate biosynthesis enzymes and proteoglycan core proteins. Glycobiology 31 (10), 1308–1318. doi: 10.1093/glycob/cwab054
Morales, F. J., Lozano Potes, I., Castaño, M., Arroyave, J. A., Velasco, A. C., Varon, F. (2002). Partial characterization of a tymovirus infecting passion fruit in Colombia, south America. J. Phytopathol. 150 (4–5), 292–296. doi: 10.1046/j.1439-0434.2002.00740.x
Morozova, O., Hirst, M., Marra, M. A. (2009). Applications of new sequencing technologies for transcriptome analysis. Annu. Rev. Genomics Hum. Genet. 10, 135–151. doi: 10.1146/annurev-genom-082908-145957
Munguti, F., Maina, S., Nyaboga, E. N., Kilalo, D., Kimani, E., Macharia, M., et al. (2019). Transcriptome sequencing reveals a complete genome sequence of Cowpea aphid-borne mosaic virus from passion fruit in Kenya. Microbiol. Resour. Announc. 8 (2), e01607–e01618. doi: 10.1128/MRA.01607-18
Nair, M. S., Saxena, A., Kaur, C. (2018). Effect of chitosan and alginate based coatings enriched with pomegranate peel extract to extend the postharvest quality of guava (Psidium guajava l.). Food Chem. 240, 245–252. doi: 10.1016/j.foodchem.2017.07.122
Ou, L. N., Zhang, Q. Q., Ji, D. Z., Li, Y. Y., Zhou, X., Jin, L. H. (2022). Physiological, transcriptomic investigation on the tea plant growth and yield motivation by chitosan oligosaccharides. Horticulturae 8, 68. doi: 10.3390/horticulturae8010068
Qu, X. L., Zhu, K. X., Li, Z. X., Zhang, D. F., Hou, L. J. (2021). The alteration of M6A-tagged transcript profiles in the retina of rats after traumatic optic neuropathy. Front. Genet. 12. doi: 10.3389/fgene.2021.628841
Scandalios, J. G. (1993). Oxygen stress and superoxide dismutases. Plant Physiol. 101 (1), 7–12. doi: 10.1104/pp.101.1.7
Taranto, F., Mangini, G., Miazzi, M. M., Stevanato, P., Vita, P. D. (2021). Polyphenol oxidase genes as integral part of the evolutionary history of domesticated tetraploid wheat. Genomics 113 (5), 2989–3001. doi: 10.1016/j.ygeno.2021.06.030
Wang, J., Islam, F., Li, L., Long, M. J., Yang, C., Jin, X. L., et al. (2017). Complementary RNA-sequencing based transcriptomics and iTRAQ proteomics reveal the mechanism of the alleviation of quinclorac stress by salicylic acid in oryza sativa ssp. japonica. Int. J. Mol. Sci. 18, 1975. doi: 10.3390/ijms18091975
Wang, Z. J., Li, Y. H., Zhang, H. Y., Yan, X. X., Cui, H. (2022). Methyl jasmonate treatment, aphid resistance assay, and transcriptomic analysis revealed different herbivore defensive roles between tobacco glandular and non-glandular trichomes. Plant Cell Rep. 41, 195–208. doi: 10.1007/s00299-021-02801-6
Wang, Z. Y., Yue, L., Dhankher, O. P., Xing, B. S. (2020). Nano-enabled improvements of growth and nutritional quality in food plants driven by rhizosphere processes. Environ. Int. 142, 105831. doi: 10.1016/j.envint.2020.105831
Wei, J. P., Zheng, G. Q., Dong, X. Y., Li, H., Liu, S. S., Wang, Y., et al. (2022). Integration of transcriptome and proteome analysis reveals the mechanism of freezing tolerance in winter rapeseed. Plant Growth Regul. 96, 103–118. doi: 10.1007/s10725-021-00763-z
Wu, Y. X., Wang, Y. J., Han, S., Li, Q. S., Kong, ,. L. X. (2022). Effect of reduced application of pesticides during the control of wheat powdery mildew by mixed use of amino-oligosaccharins and pyraclostrobin. Agrochemicals 61 (6), 449–452. doi: 10.16820/j.nyzz.2022.0037
Wu, Y. L., Yi, G. J., Peng, X. X., Huang, B. Z., Liu, E. E., Zhang, J. J. (2013). Systemic acquired resistance in Cavendish banana induced by infection with an incompatible strain of fusarium oxysporum f. sp. cubense. J. Plant Physiol. 170 (11), 1039–1046. doi: 10.1016/j.jplph.2013.02.011
Xu, W., Purugganan, M. M., Polisensky, D. H., Antosiewicz, D. M., Fry, S. C., Braam, J. (2001). Arabidopsis TCH4, regulated by hormones and the environment, encodes a xyloglucan endotransglycosylase. Plant Cell 7, 1555–1567. doi: 10.1105/tpc.7.10.1555
Xu, X., Wang, J. H., Wu, H. H., Yuan, Q. H., Wang, J. H., Cui, J., et al. (2022). Effects of selenium fertilizer application and tomato varieties on tomato fruit quality: A meta-analysis. Sci. Hortic. 304, 111242. doi: 10.1016/j.scienta.2022.111242
Yael, L. D., Marianna, O., Elena, S., Yigal, E., Dalia, R. D., Alexander, V. J. (2006). Expression and functional analyses of the plastid lipid-associated protein CHRC suggest its role in chromoplastogenesis and stress. Plant Physiol. 142, 233–244. doi: 10.1104/pp.106.082404
Yang, Z. B., Li, P., He, Y. J. (2019). Design, synthesis, and bioactivity evaluation of novel isoxazole-amide derivatives containing an acylhydrazone moiety as new active antiviral agents. Molecules 24, 3766. doi: 10.3390/molecules24203766
Yang, S., Li, X. Y., Yang, Y. P., Huang, Y., Gu, Y. T., Tang, X. (2022). Evaluation and analysis of control effect of four medicament in potato virus y disease. Agrochemicals 61 (2), 136–139. doi: 10.16820/j.cnki.1006-0413.2022.02.014
Yang, C. J., Zhang, C., Lu, Y. N., Jin, J. Q., Wang, X. L. (2011). The mechanisms of brassinosteroids' action: From signal transduction to plant development. Mol. Plant 4 (4), 588–600. doi: 10.1093/mp/ssr020
Yang, X. H., Zhu, L. C., Huang, X., Zhang, Q., Li, S., Chen, Q. L., et al. (2022). Determination of the soluble solids content in korla fragrant pears based on visible and near-infrared spectroscopy combined with model analysis and variable selection. Front. Plant Sci. 13. doi: 10.3389/fpls.2022.938162
Yan, W. Q., Ni, Y. X., Liu, X. T., Zhao, H., Chen, Y. H., Jia, M., et al. (2021). The mechanism of sesame resistance against macrophomina phaseolina was revealed via a comparison of transcriptomes of resistant and susceptible sesame genotypes. BMC Plant Biol. 21, 159. doi: 10.1186/s12870-021-02927-5
Ye, T., Guo, Y., Zheng, H. G., Kuang, J. J., Zhang, W. Z., Ming, Y. L. (2019). Advance researches and control methods of Passiflora mosaic disease. Subtropical Plant Sci. 48 (04), 397–403. doi: 10.3969/j.issn.1009-7791.2019.04.017
Yeturu, S., Paz, L., Intriago, D., Castro, J., Álvarez, H., Viera, W. (2017). First report of mosaic disease caused by Cucumber mosaic virus infecting passion fruit in Ecuador. Plant Dis. 102 (2), 458. doi: 10.1094/PDIS-06-17-0814-PDN
Yu, L. (2015). Popularization and application of haidaosu (5% amino-oligosaccharin) as a plant immunity inducer in main crops cultured by corps. Agric. Sci. Technol. 16 (12), 2733–2741. doi: 10.16175/j.cnki.1009-4229.2015.12.032
Yuan, X. B., Zheng, J. P., Jiao, S. M., Cheng, G., Feng, C., Du, Y. G., et al. (2019). A review on the preparation of chitosan oligosaccharides and application to human health, animal husbandry and agricultural production. Carbohyd. Polym. 220, 60–70. doi: 10.1016/j.carbpol.2019.05.050
Yu, Q., Liu, Y., Mo, X. H., Yang, C. J., Jiang, L. H. (2002). Applying amino-oligosaccharin on tobacco for controlling tobacco virus disease. Chin. J. Biol. Control 18 (3), 128–131.
Yu, L., Wang, W. L., Zeng, S., Chen, Z., Yang, A. M., Shi, J. (2018). Label-free quantitative proteomics analysis of cytosinpeptidemycin responses in southern rice black-streaked dwarf virus-infected rice. Pestic. Biochem. Phys. 147, 20–26. doi: 10.1016/j.pestbp.2017.06.005
Zhang, Y., Liang, Y., Han, J., Hu, X. H., Li, X. J., Zhao, H. L., et al. (2022). Interactive effects of iron and photoperiods on tomato plant growth and fruit quality. J. Plant Growth Regul 42, 376–389. doi: 10.1007/s00344-021-10554-5
Zhang, C. Q., Wang, J., Gao, X. (2008). Computational identification of transcriptional regulatory elements in Arabidopsis TCH4 promoter. Hereditas 30 (5), 620–626. doi: 10.3724/SP.J.1005.2008.00620
Zhang, H. P., Zou, J., Yin, Y., Zhang, B., Hu, Y. L., Wang, J. J., et al. (2019). Bioinformatic analysis identifies potentially key differentially expressed genes in oncogenesis and progression of clear cell renal cell carcinoma. PeerJ 7, e8096. doi: 10.7717/peerj.8096
Zhou, T., Zhang, H., Lai, T. F., Cheng, Q., Shi, N. N., Wang, H. Z., et al. (2012). Virus-induced gene complementation reveals a transcription factor network in modulation of tomato fruit ripening. Sci. Rep. 2, 836. doi: 10.1038/srep00836
Keywords: chitosan oligosaccharide, anti-CMV activity, Passiflora spp., plant immune inducer, brassinosteroids cell signaling pathway
Citation: Zhang L, Yu L, Zhao Z, Li P and Tan S (2023) Chitosan oligosaccharide as a plant immune inducer on the Passiflora spp. (passion fruit) CMV disease. Front. Plant Sci. 14:1131766. doi: 10.3389/fpls.2023.1131766
Received: 26 December 2022; Accepted: 25 January 2023;
Published: 06 February 2023.
Edited by:
Yang Jian, Ningbo University, ChinaReviewed by:
Songbai Zhang, Hunan Academy of Agricultural Sciences (CAAS), ChinaServet Aras, Bozok University, Türkiye
Guo Ping Zhang, Huaibei Normal University, China
Copyright © 2023 Zhang, Yu, Zhao, Li and Tan. This is an open-access article distributed under the terms of the Creative Commons Attribution License (CC BY). The use, distribution or reproduction in other forums is permitted, provided the original author(s) and the copyright owner(s) are credited and that the original publication in this journal is cited, in accordance with accepted academic practice. No use, distribution or reproduction is permitted which does not comply with these terms.
*Correspondence: Lu Yu, yuji570@163.com; Pei Li, pl19890627@126.com; Shuming Tan, smtan@gzu.edu.cn
†These authors have contributed equally to this work