- Key Laboratory of Biology, Genetics and Breeding of Special Economic Animals and Plants, Ministry of Agriculture and Rural Affairs, Tea Research Institute of the Chinese Academy of Agricultural Sciences, Hangzhou, China
Aroma is an important factor affecting the character and quality of tea. The improvement of aroma trait is a crucial research direction of tea plant breeding. Volatile terpenes, as the major contributors to the floral odors of tea products, also play critical roles in the defense responses of plants to multiple stresses. However, previous studies have largely focused on the aroma formation during the manufacture of tea or the comparison of raw tea samples. The mechanisms causing different aroma profiles between tea cultivars have remained underexplored. In the current study, a high-density genetic linkage map of tea plant was constructed based on an F1 population of ‘Huangdan’ × ‘Jinxuan’ using genotyping by sequencing. This linkage map covered 1754.57 cM and contained 15 linkage groups with a low inter-marker distance of 0.47 cM. A total of 42 QTLs associated with eight monoterpene contents and 12 QTLs associated with four sesquiterpenes contents were identified with the average PVE of 12.6% and 11.7% respectively. Furthermore, six candidate genes related to volatile terpene contents were found in QTL cluster on chromosome 5 by RNA-seq analysis. This work will enrich our understanding of the molecular mechanism of volatile terpene biosynthesis and provide a theoretical basis for tea plant breeding programs for aroma quality improvement.
1 Introduction
Tea is one of the most popular nonalcoholic beverages in the world, made by tender shoots of tea plant. Aroma is a critical factor influencing the quality of tea products, affecting consumer preference (Yang et al., 2013). In addition to the manufacturing process and environmental factors, tea cultivars are one of the key factors affecting the aroma of tea products (Zheng et al., 2016). As early as 1981, it was reported that the concept of terpene index (TI) was proposed by studying the aroma characteristics and systematic variation among tea cultivars, pointing out that the content of monoterpenes, such as linalool and geraniol, were species-specific (Takeo, 1981). Another report found that the varietal differences of aromatic profile remain significant for tea cultivars with very close genetic background (Lin et al., 2013). A recent study on ten tea cultivars with different leaf areas demonstrated a positive correlation between linalool content and leaf area (Zeng et al., 2021). Therefore, selecting high-aroma cultivars is one of the most interesting targets for tea plant breeding. In comparison to conventional breeding methods, marker assisted selection (MAS) through the discovery of favorable alleles and application of suitable molecular markers can greatly shorten the breeding process to accelerate the traditional tea plant breeding. However, previous studies have largely focused on the aroma formation during the manufacture of tea or the comparison of raw tea samples (Feng et al., 2019; Shi et al., 2019; Yu et al., 2021). The mechanisms causing different aroma profiles between tea cultivars have remained underexplored. The quantitative trait loci (QTL) mapping and candidate gene identification of aroma traits in tea plant have not yet been reported, which has become a technical bottleneck for efficient genetic breeding of high-aroma tea cultivars.
Volatile monoterpenes and sesquiterpenes are important components of tea aroma, often with strong floral, sweet, and woody scents. For example, geraniol has a sweet, oral aroma similar to that of roses, while linalool has a lily-like aroma, which are particularly important for the aroma quality of green, black, and oolong teas (Wang et al., 2017; Guo et al., 2022; Shi et al., 2022). Apart from being a major contributor to the floral odors of plant products, terpenoids have been shown to have a variety of biological activities (Singh and Sharma, 2014). Terpene volatiles in tea plant such as α-farnesene and ocimene, play important roles in plant-to-plant communication (Zeng et al., 2017). Sesquiterpene (E)-nerolidol was found to be a volatile signal involved in response to abiotic and biotic stresses (Chen et al., 2020a; Zhao et al., 2020). Although more and more studies in recent years have focused on the metabolic mechanism of volatile terpenes, the research on the synthesis and regulation of volatile terpenes in tea plant still has to be improved compared with model plants (Mei et al., 2017; Liu et al., 2018; Xu et al., 2018b; Liu et al., 2020).
The tea plant is basically self-incompatible with high heterozygosity and long growth cycle (Zhang et al., 2016). Hence it is difficult to generate a population fitting for QTL mapping, such as recombinant inbred lines (RIL) and chromosome segment substitution lines. Although several genetic maps were reported in tea plant, most of them chose hybrid parents cultivars based on the content of nonvolatile metabolites, such as flavonoid, caffeine, and amino acid (Ma et al., 2018; Xu et al., 2018a; Huang et al., 2022b). Therefore, a more suitable population is needed for the QTL mapping of aroma compounds. In this study, we initially constructed an F1 population from a controlled cross between the high-aroma tea cultivar ‘Huangdan’ ('HD') and ‘Jinxuan’ ('JX'). As a reference standard for breeding oolong tea cultivars in China, 'HD' is well known for its high levels of aroma. It originated from Fujian Province and has been used to breed a series of excellent hybrid offspring (Wang et al., 2022b). The other parent 'JX' is an oolong tea that originated from Taiwan Province with a unique fragrance. To elucidate the molecular mechanism of aroma differences among tea cultivars and the key genes controlling the accumulation of volatile terpenes, we constructed a high-density SNP linkage map using 148 individuals of F1 population. Headspace solid-phase microextraction (HS-SPME) combined with gas chromatography-time-of-flight mass spectrometry (GC-TOF MS) was used to analyze the aroma components. The major differential volatile terpene compounds and their genetic variation were investigated in the parents and their F1 progeny. Further QLT mapping and comparative transcriptomics analyses were performed to screen the candidate genes associated with terpenoid contents. This work will enrich the theoretical basis of the molecular mechanism of volatile terpene biosynthesis and breeding of high-aroma tea cultivars.
2 Materials and methods
2.1 Plant materials
The mapping population composed of 148 F1 individuals was derived from a controlled cross between ‘Huangdan’ ('HD') and ‘Jinxuan’ ('JX'). The parents and all F1 individuals were planted in the Shengzhou Experimental Station of Tea Research Institute of the Chinese Academy of Agricultural Sciences. The healthy ‘two and a bud’ (apical bud with two adjacent young leaves) tea samples were collected from each tea plant for DNA extraction, RNA extraction, and aroma analysis in the spring of 2020 and 2021.
2.2 SNP identification by genotyping by sequencing and linkage map construction
Approximately 0.1 g of each tea sample was ground in liquid nitrogen for genomic DNA isolation using an improved CTAB method (Dellaporta et al., 1983). The DNAs of 'HD', 'JX', and 148 F1 individuals were digested by NlaIII and MspI restriction enzymes, and the genotyping by sequencing (GBS) libraries were constructed. After purification, the GBS libraries was sequencing using Illumina Novaseq 6000 platform (Novogene, Beijing, China). Clean data were obtained by removing low quality reads and then aligned to the genome of the parent 'HD' via BWA and SAMTOOLS software. The genome sequences and gene annotation files were downloaded in the BIG Data Center (https://bigd.big.ac.cn/) under project number PRJCA003382 (Wang et al., 2021). SNP calling was performed for all samples using the GATK software. The SNPs were screened by filtering out false-positive SNPs and those showed distorted segregation (P < 0.001, χ2 test). Using the high-quality SNP markers, the genetic map was constructed using JoinMap (Ver. 4.1, Kyazma, Wageningen, Netherlands) with the maximum-likelihood method.
2.3 GC-TOF MS analysis
Extraction of volatiles from tea samples was achieved by HS-SPME as previously described with some modifications (Chen et al., 2021). Briefly, 0.6 g of samples were ground to fine powders with the precooled miller after lyophilization, placed into a 20 mL sealed headspace vial and incubated at 80°C for 2 min before extraction. A 1 cm, 65 μm 50/30 μm divinylbenzene/carboxen/polydimethylsiloxane (DVB/CAR/PDMS) SPME fiber (Supelco, Bellefonte, PA, USA) was used to collect volatiles for 60 min at 80°C. All volatile compounds absorbed onto the SPME fiber were desorbed at 250°C for 5 min in splitless mode and analyzed using an Agilent 7890B gas chromatograph (Agilent Co., Santa Clara, CA, USA) coupled with a Pegasus 4D time-of-flight mass spectrometer (LECO Co., Saint Joseph, MI, USA) (GC-TOF MS). A Restek Rxi®-5Sil MS capillary column (30 m×0.25 mm×0.25 µm film thickness) was employed with high purity helium as the carrier gas at a flow rate of 3 mL/min. The injection and transfer line temperature were controlled at 250°C. During the analysis, the oven temperature program started at 50°C and maintained for 2 min, increasing to 265°C at a rate of 4°C/min and maintaining for 5 min. The mass spectra ion source temperature and electron energy were set to 220 °C and -70 eV, respectively. The detector voltage was 1,550 V. The scan range was 33-500 atomic mass unit (AMU) with 10 spectra/s acquisition rate. Three biological repetitions were performed for volatile extraction. Quality control (QC) samples were prepared by mixing 1.0 g of every sample to become a combined sample. QC samples were injected throughout the analytical runs (every five samples) to check the instrument performance.
Raw data acquired by GC-TOF MS were processed by ChromaTOF software (Ver. 4.51.6, LECO, St. Joseph, MI, USA) with default settings. Aroma compounds were identified or tentatively identified by comparing their retention time, retention indices (RI) and mass spectra with those of authentic standards or against the NIST library (http://webbook.nist.gov/). Quantitative measurements of volatiles were based on the mean area of selected characteristic ions after normalization. The orthogonal partial least squares-discriminant analysis (OPLS-DA) was performed to identify differential volatile metabolites using Simca-P (Ver. 14.1, Umetrics AB, Umeå, Sweden) after Pareto scaling. Violin plots of the distribution of the main aroma compounds in F1 population were made using Python program seaborn.
2.4 QTL mapping
QTL mapping was performed using MapQTL (Ver. 6.0, Kyazma, Wageningen, Netherlands) with the interval mapping (IM) method. A LOD threshold of 3.0 was set to identify significant QTL. Ranges above the LOD of 3.0 were identified as QTL intervals (Wang et al., 2019b). The QTL detected across years for each trait was assigned to be the same stable QTL if confidence intervals overlapped. QTL positions were drawn using MapChart (Ver. 2.32, Wageningen, Netherlands).
2.5 Gene expression analysis using RNA-seq and qRT-PCR
Approximately 0.1 g of tea samples (three biological replicates per genotype) were used for RNA extraction. Total RNAs were extracted with the RNAprep Pure Plant Kit (Tiangen, Beijing, China) following the manufacturer’s procedures. Sequencing libraries were generated and paired-end 150 bp sequencing was performed on an Illumina Novaseq 6000 platform using a customer sequencing service (Novogene, Beijing, China). Clean reads were obtained by removing reads containing adapter, reads containing ploy-N and low quality reads from raw data. The index of the reference genome was built and paired-end clean reads were aligned to the reference genome (Wang et al., 2021) with the Hisat2 (Ver. 2.0.5). Read numbers mapped to each gene of the reference genome were counted using featureCounts (Ver. 1.5.0). Fragments per kilobase of exon model per million mapped reads (FPKM) were used to quantify transcript abundance. The DESeq2 R package (Ver. 1.20.0) was applied to identify differentially expressed genes (DEGs) with Padj < 0.05 and an absolute value of fold change > 1.5. The heatmap was generated with TBtools (Chen et al., 2020b) to visualize expression patterns of DEGs between samples.
Candidate gene transcript expressions were validated by quantitative real time PCR (qRT-PCR). The cDNA was reversely transcribed using PrimeScriptTM RT reagent Kit (Takara, Dalian, China). Gene specific primers of candidate genes were designed and given in Table S3. Relative gene expression was calculated by the 2-ΔΔCT method using the CsGAPDH sequence (accession no. KA295375) as the reference gene.
3 Results
3.1 Genotyping by sequencing of the F1 population
A total of 139.34G clean bases were derived from sequencing assembly of 148 F1 individuals using the GBS approach with an average complete digestion rate was 90.24%. The Average Q20, Q30, and GC content were 95.35%, 87.96%, and 36.38% respectively, proving the high quality of the sequencing results. The 985.52 Mb clean reads of F1 offspring were obtained by aligning with the 'HD' reference genome with an average matching rate of 98.81%. The average read depth was 9.04×. Based on the genotyping result of two parental lines, a total of 11,462,958 single nucleotide polymorphisms (SNPs) were found. Among them, only segregation types lm × ll (2,001,025), nn × np (2,918,476), and hk × hk (6,380,260) were selected for genotyping in F1 individuals. The final number of available SNPs was 11,299,761 (Figure S1). After removing abnormal bases and deleting segregation distortion (P < 0.001), we obtained 13,289 effective SNPs used for linkage analysis.
3.2 Construction of a high-density linkage map
As a result, 3770 SNP markers were finally mapped into 15 linkage groups (LGs), corresponding to the haploid chromosome number of tea plant (Figure 1). The linkage map covered a total genetic distance of 1754.57 cM, with an average marker distance of 0.47 cM. The average length of the 15 linkage groups was 116.97 cM. The longest linkage group was LG06 (165.01 cM), and the shortest was LG07 (79.99 cM). A total of 99.68% marker interval size was below 5 cM, and only 12 of them were between 5 and 10 cM (Table 1). The information on 3770 markers in the linkage map was presented in Table S1.
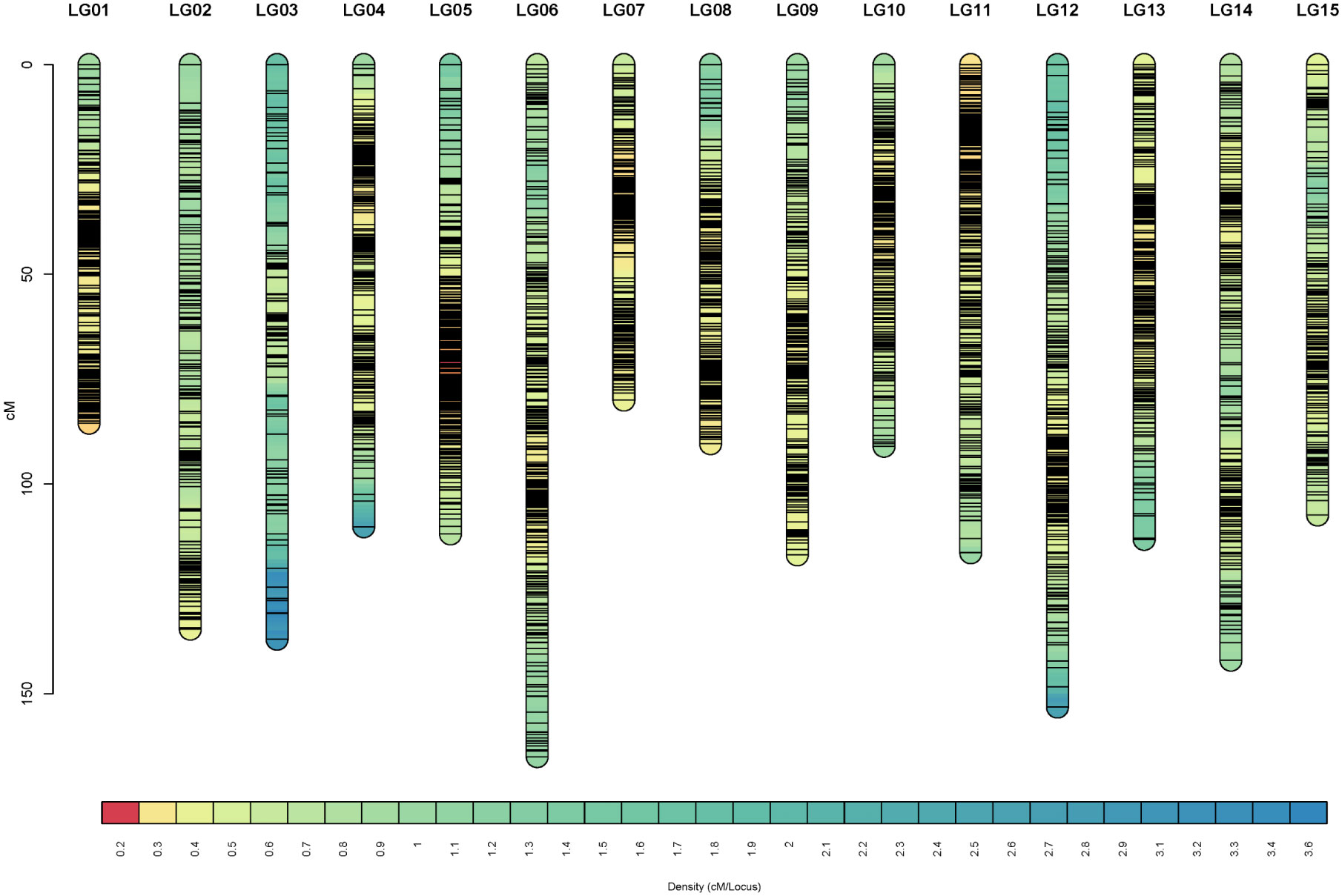
Figure 1 Marker distribution across the linkage map. The x-axis, y-axis, and lines in the column represent the linkage group, genetic distance, and unique marker, respectively.
3.3 Phenotypic variations of volatile terpene contents
To clarify the differences in terpene volatile contents between 'HD' and 'JX'. The aroma compound phenotypes of two parental lines were measured by HS-SPME GC-TOF MS in 2020 and 2021. The OPLS-DA model was built using two years of data respectively to select differential volatile metabolites that make a significant contribution to classification. As a result, 12 volatile terpenes showed significant differences (P < 0.05 and at least one year VIP > 1), including 8 monoterpenes ((E)-β-ocimene, cis-linalool oxide (furanoid), trans-linalool oxide (furanoid), linalool, trans-linalool oxide (pyranoid), α-terpineol, (Z)-nerol, and geraniol) and 4 sesquiterpenes (α-cubebene, δ-cadinene, calamenene, and (E)-nerolidol) (Table 2). The contents of these 12 volatile terpenes were higher in 'HD' than in 'JX' in both years. Among them, geraniol was the most significantly different volatile terpene. The VIP values were greater than 10 for both years (10.84 in 2020, 15.19 in 2021). In addition, linalool and trans-linalool oxide (furanoid) also showed significant differences with both VIP values and fold changes higher than 2 in these two years.
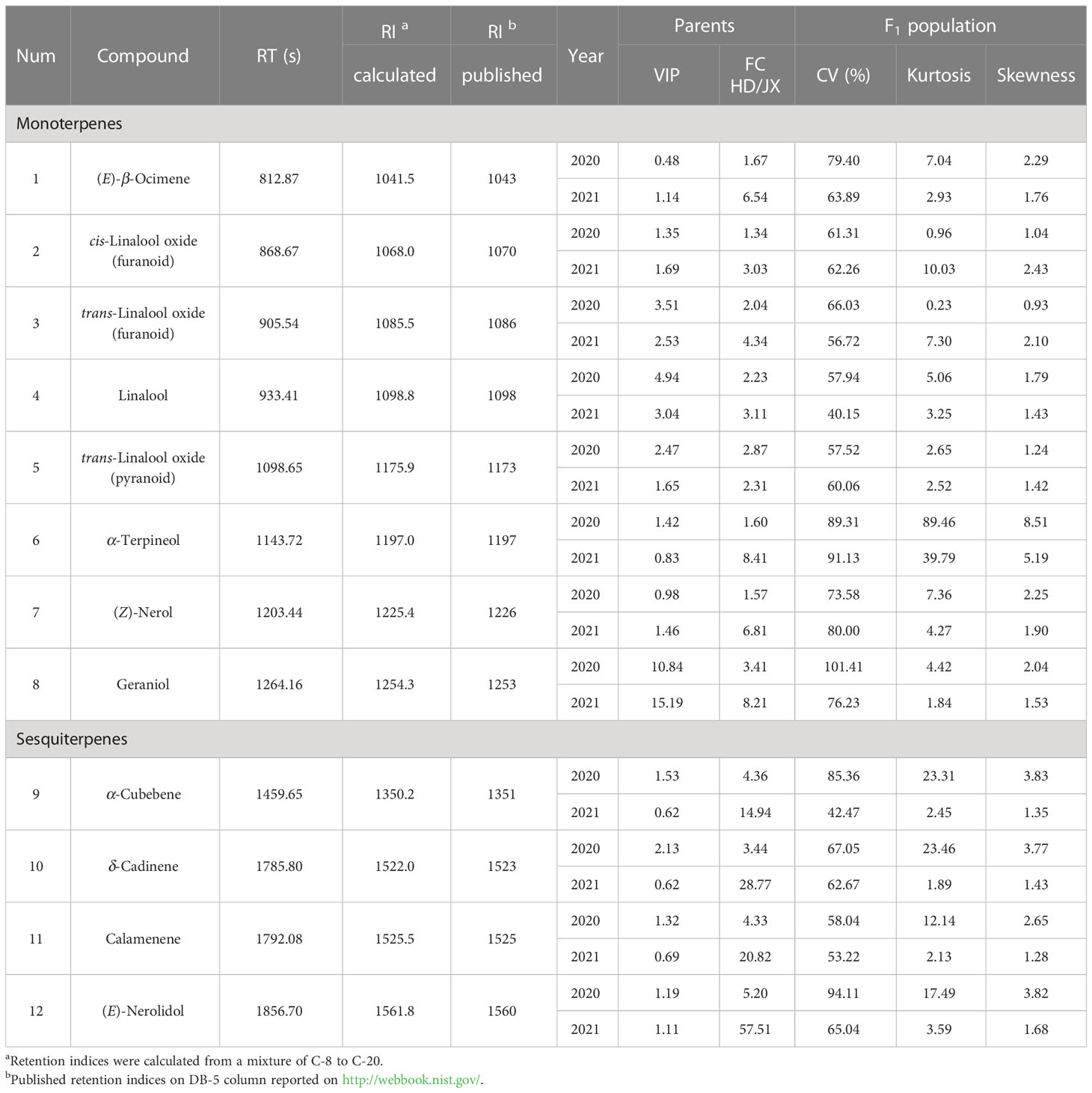
Table 2 Phenotypic variation of the volatile terpene contents in the ‘HD’ and ‘JX’ parents and F1 progeny.
As a result, these 12 aroma compounds mentioned above were chosen to be analyzed for the phenotypic variations in the F1 population of 2020 and 2021. The coefficient of variation (CV), kurtosis, and skewness were calculated for each volatile terpene (Table 2). Significant variations of aroma compounds were found in the F1 population (Minimum CV > 40%). The frequency distribution of aroma compounds in the F1 population was illustrated in Figure 2A. Most aroma compounds exhibited a near-normal distribution in the F1 population suggested that these aroma compounds were quantitative traits. The correlations between different aroma compounds were carried out and depicted in Figure 2B. It was noteworthy that a significant positive correlation observed in monoterpenes and sesquiterpenes respectively, indicating that to be related to their synthetic pathways. A strong positive correlation were found between cis-linalool oxide (furanoid) and trans-linalool oxide (furanoid), as well as between (E)-β-ocimene, (Z)-nerol and geraniol (r > 0.77). In addition, α-cubebene, δ-cadinene, and calamenene also showed a strong positive correlation (r > 0.80).
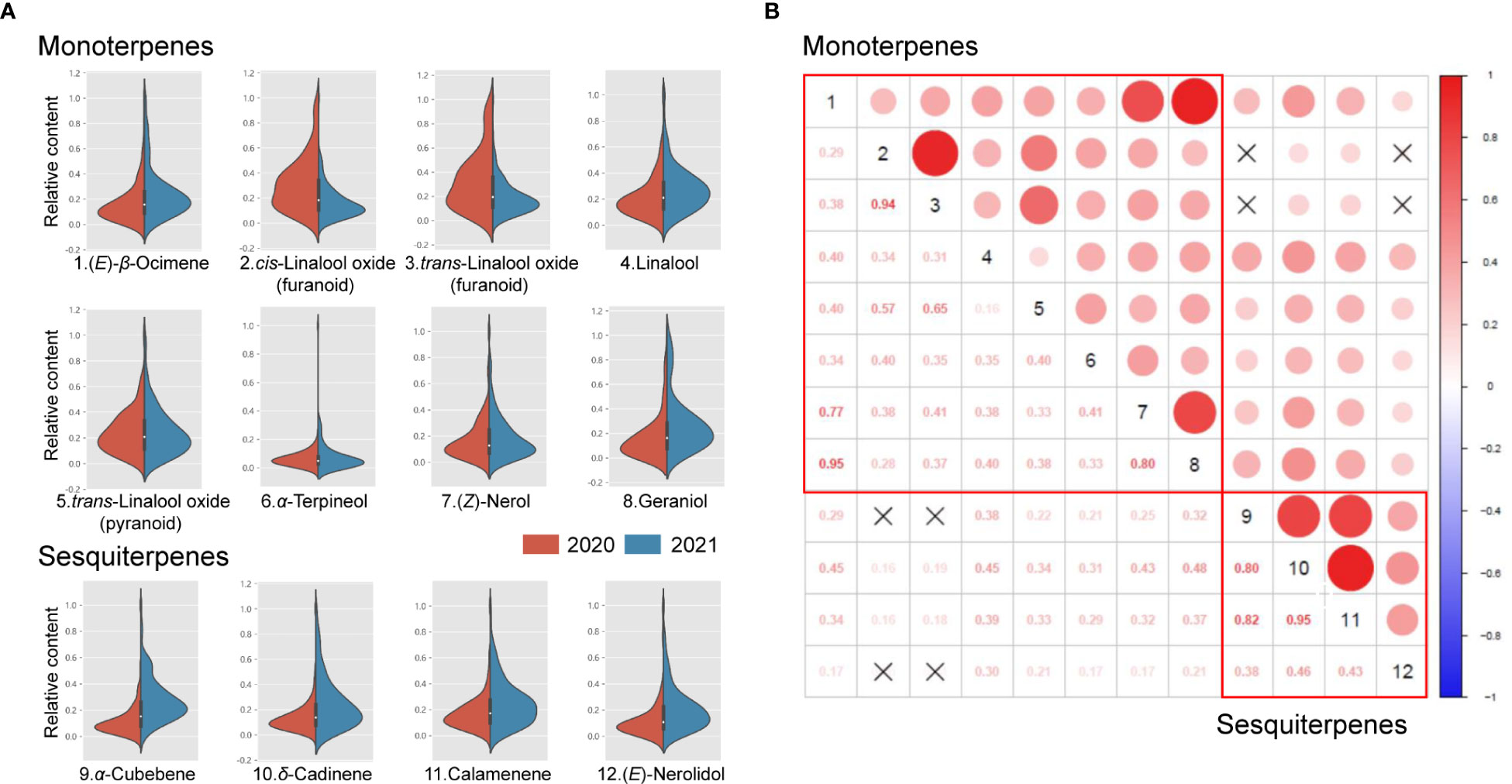
Figure 2 Violin-plot distribution and correlation analysis of volatile terpenes in the ‘HD’ × ‘JX’ F1 population. Violin-plot distribution of eight monoterpenes and four sesquiterpenes (A). Pearson’s correlations among 12 volatile terpenes (B). Crossed symbols indicate P > 0.05.
3.4 QTL mapping of volatile terpene contents in the F1 population
We performed QTL analysis of volatile terpene contents with a phenotypic dataset from the F1 populations based on the linkage maps constructed in this study. A total of 54 QTLs were screened for 12 volatile terpenes. These QTLs were distributed on 11 linkage groups, including LG03-09 and LG11-13 (Figure 3), with a LOD score ranging from 3.08 to 6.57 (Table 3). The number of QTL per trait ranged from one to eight. There are eight QTLs associated with (E)-β-ocimene, cis-linalool oxide (furanoid) and geraniol respectively, while only one QTL controlling α-cubebene was identified. The proportion of phenotype variance explained (PVE) by each QTL ranged from 9.10% to 18.50%, and 47 loci had a PVE >10%. Six stable QTLs were validated in 2020 and 2021, including two for (E)-β-ocimene, two for linalool, one for (Z)-nerol, and one for geraniol.
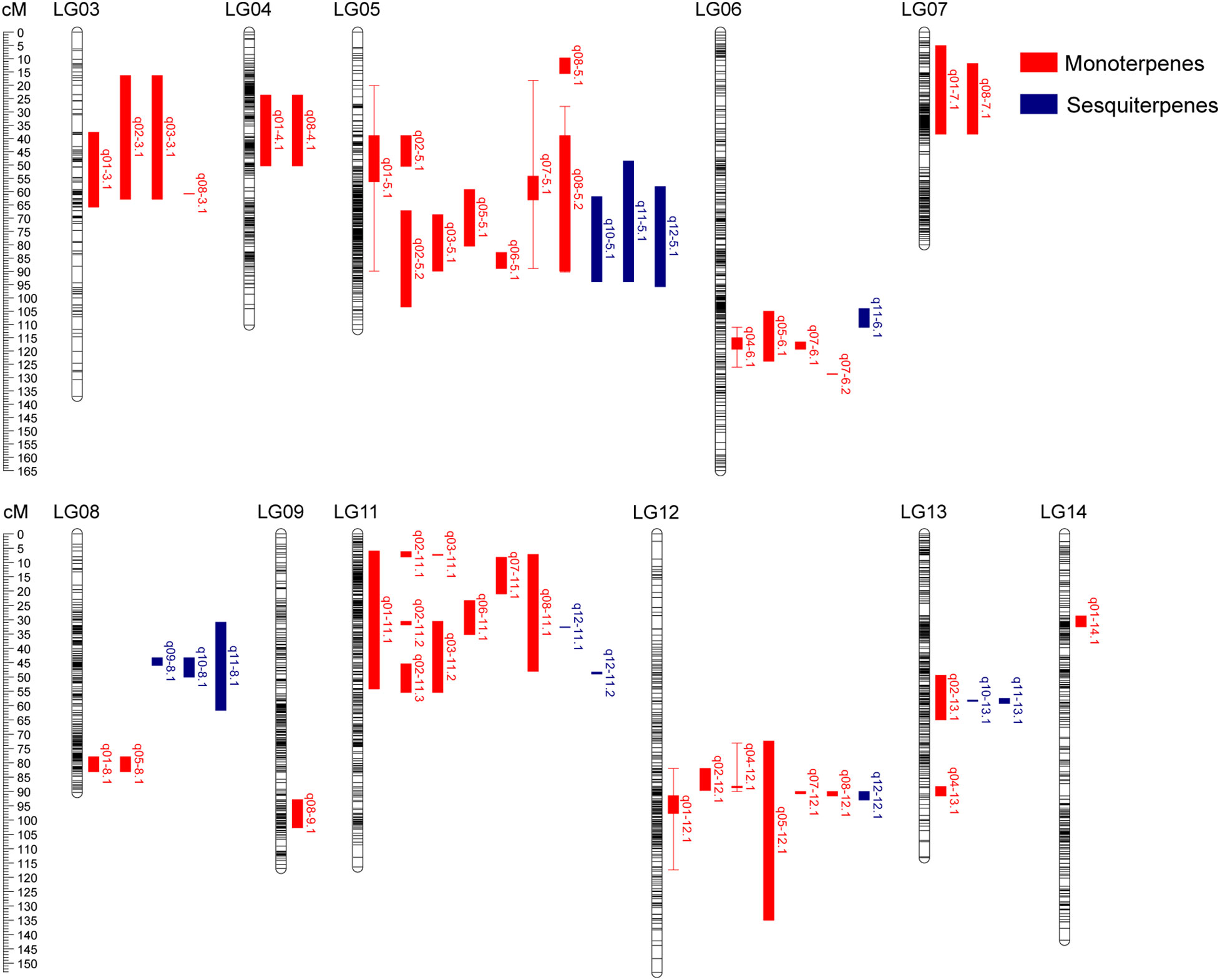
Figure 3 Distribution of QTL associated with volatile terpene contents identified in the ‘HD’ × ‘JX’ F1 population. QTL intervals are shown on the right of linkage groups, represented by bars of different color (monoterpenes, red; sesquiterpenes, blue).
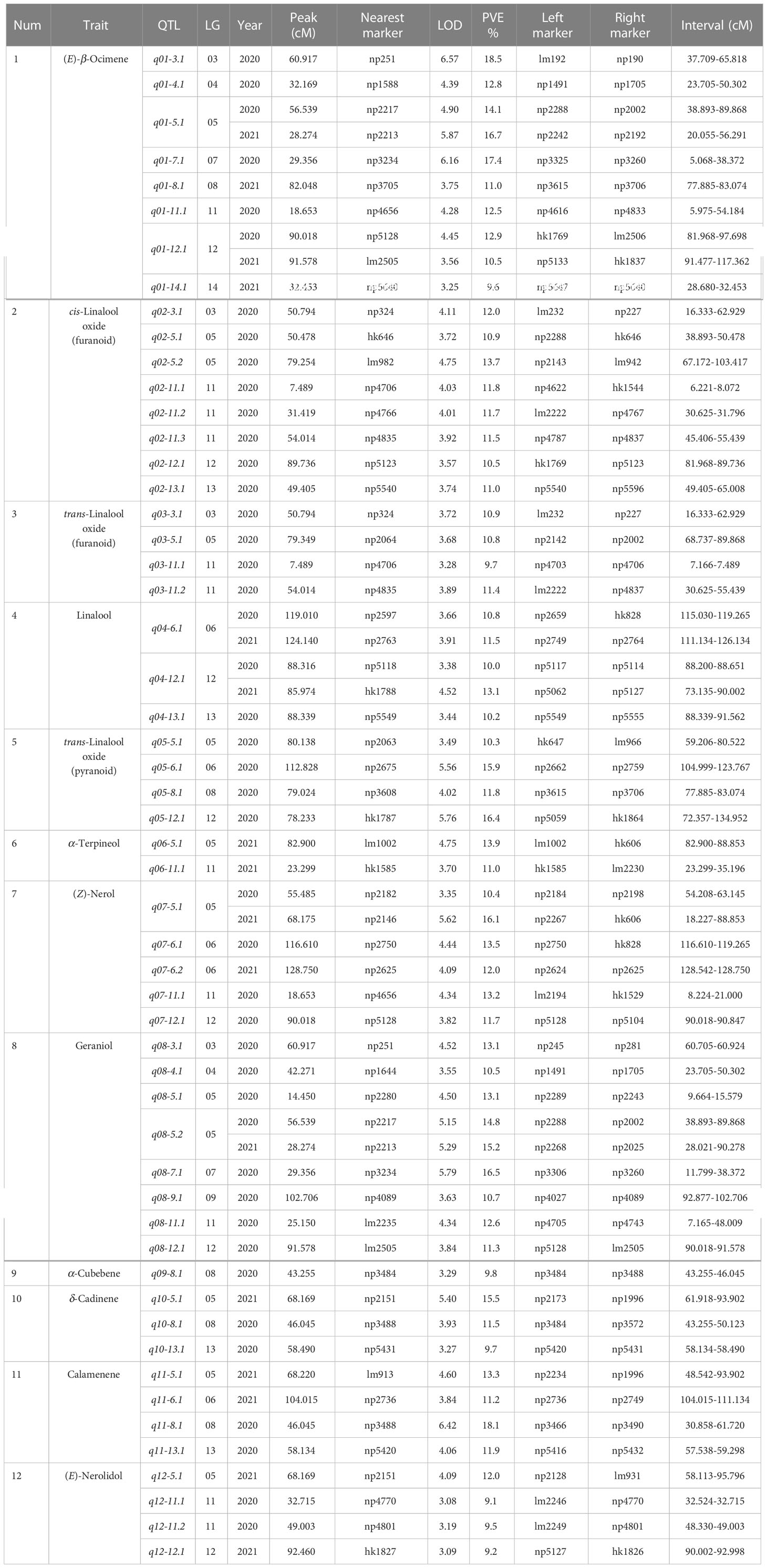
Table 3 Overview of QTLs associated with volatile terpene contents detected in the ‘HD’ × ‘JX’ F1 population.
Forty-two QTLs controlling monoterpene contents were identified with an average PVE of 12.6%. The LOD score of QTL q01-3.1 associated with (E)-β-ocimene was the highest, which explained 18.5% of the phenotypic variation in 2020, and it was located between lm192 and np190 in LG03. Two stable QTLs associated (E)-β-ocimene were found to be located on LG05 and LG12. QTL q01-5.1 was located between np2242 and np2002. It explained 15.40% of the phenotypic variation on average. Another QTL, q01-12.1, explained 12.9% and 10.5% of the phenotypic variation for 2020 and 2021 respectively, and the LOD peak was located at the position of 90.018 and 91.578. Two stable QTLs controlling linalool were identified on LG06 and LG12. QTL q04-6.1 was located between markers np2749 and np2764, with an average PVE of 11.2% over two years. Another QTL, q04-12.1, was located on LG12, nearby the marker np5118 in 2020 and hk1788 in 2021, with the PVE of 10.0% and 13.1% respectively. The stable QTL, q07-5.1, associated with (Z)-nerol was detected on LG05. The LOD peak was present at the position of 55.485 cM in 2020 and 68.175 cM in 2021. The stable QTL associated with geraniol, q08-5.2, was identified on LG05. The confidence interval ranged from 28.021 to 90.278 cM over two years, and the overlapped region was from 38.893 to 89.868 cM, with a length of 50.975 cM. The QTL explained 14.8% and 15.2% of the phenotypic variance for 2020 and 2021 respectively.
For sesquiterpenes, 12 QTLs were identified on six linkage groups with an average PVE of 11.7%. The LOD score of QTL q11-8.1 associated with calamenene was the highest, which explained 18.1% of the phenotypic variation in 2020. It was located between markers n3466 and np3490, in which q09-8.1, q10-8.1, and q11-8.1 showed co-localization. Three QTLs (q10-5.1, q11-5.1, and q12-5.1) associated with δ-cadinene, calamenene, and (E)-nerolidol were located on LG05 between markers np2234 and lm931 in 2021, ranging from 48.542 to 95.796 cM. However, there were no stable QTL associated sesquiterpenes in the two years.
To summarize, two QTL clusters that carried more than 10 QTLs were found on LG05 and LG11, associated with both monoterpenes and sesquiterpenes (Table 4). The Chr11 cluster included 9 QTLs for monoterpenes and 2 QTLs for sesquiterpenes, ranging from 5.975 to 55.439 cM. The Chr05 cluster carried 3 QTLs for sesquiterpenes and 8 QTLs for monoterpenes, including 3 stable QTLs for (E)-β-ocimene, (Z)-nerol, and geraniol. It ranged from 18.227 to 103.417 cM, and the overlapped region for three stable QTLs was from 28.021 to 88.853 cM, with a length of 60.832 cM. It is worth noting that this cluster was associated with almost all aroma traits except linalool and α-cubebene, indicating that it might be key synthetic genes or potential regulatory network for volatile terpenes.
3.5 Gene expression profiling in Chr05 QTL cluster based on RNA-seq analysis
Transcriptomic analysis is a powerful tool for screening candidate genes in F1 hybrid progeny (Huang et al., 2022a). To further investigate the candidate genes in Chr05 cluster, we conducted comparative transcriptomics analyses on ten F1 individuals with distinct contents of volatile terpenes in 2021, including five high-content individuals and five low-content individuals. According to the VIP values and the PVE of QTLs mentioned above, geraniol was selected as representative volatile terpene. The average fold changes of geraniol content in the two groups were more than ten times in the two years (Figure 4A). Overall, ~1.38 billion paired-end reads were obtained by Illumina sequencing, with an average of 44.8 million reads per library. The average Q20 and Q30 were 96.91% and 91.73% respectively. Approximately 89.22% of the reads were mapped against the 'HD' genome, with on average 85.39% of uniquely mapped reads. The correlations between gene expression levels and geraniol contents in 2021 were carried out. After filtering for low correlation genes (Pearson’s correlation |r| < 0.6), a comparison of differentially expressed genes (DEGs) (Average FPKM of higher group > 5, Padj < 0.05 and |fold change| > 1.5) among different groups was illustrated in the heatmap (Figure 4B). In comparison to the low-content group, 60 (28 up-regulated and 32 down-regulated) genes were found to be differentially expressed in the Chr05 cluster. Among them, an aldehyde dehydrogenase gene (HD.08G0014050) showed the most significant up-regulated, while the most down-regulation was observed at more than 14 folds for transcript HD.08G0005440 encoding ankyrin repeat-containing protein. The information on DEGs was shown in Table S2.
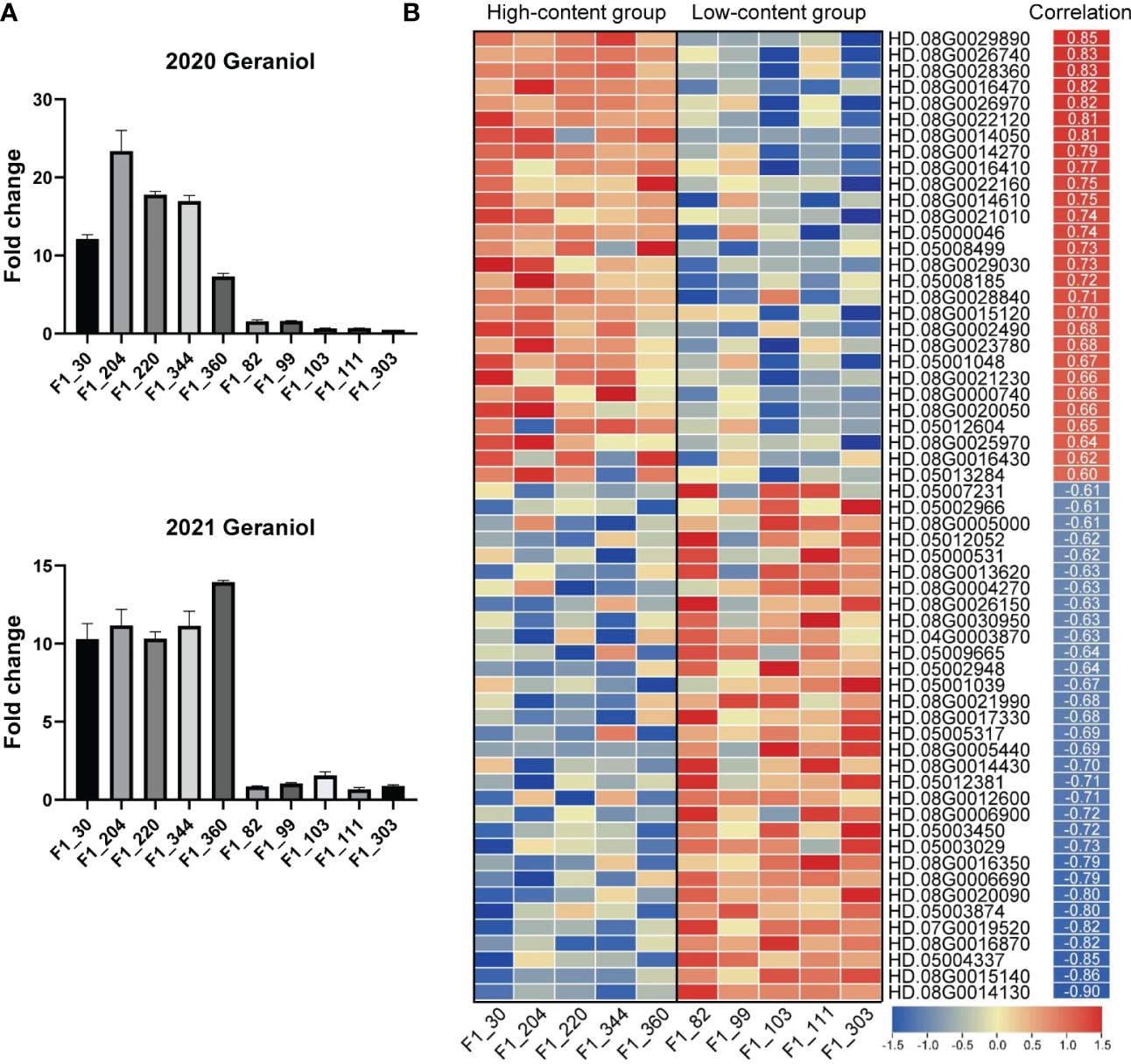
Figure 4 RNA-Seq data analysis of candidate genes for volatile terpene contents in tea plant. The contents of geraniol in ten selected F1 individuals in 2020 and 2021 (A). Results are expressed as mean ± SEM (n = 3). Expression patterns of candidate genes in Chr05 cluster (B). Pearson’s correlations between geraniol contents and the expression levels of candidate genes in 2021 are shown on the right of heatmap.
Among the up-regulated genes in the Chr06 cluster, four genes directly related to terpene synthesis were found, including three terpene synthase (TPS) genes (HD.08G0016410, HD.08G0016430, and HD.08G0016470) and a 1-deoxy-D-xylulose-5-phosphate synthase (DXS) gene (HD.08G0002490). HD.08G0016410 has the highest average expression level among the three TPS genes, the average FPKM in the high-content group was 49. The max fold change was observed in HD.08G0016470 (fold change = 5.27). The correlation coefficients with geraniol contents were 0.77, 0.62, 0.82 and 0.68 for HD.08G0016410, HD.08G0016430, HD.08G0016470, and HD.08G0002490, respectively. These four genes could be the candidate synthase genes for volatile terpene accumulation.
Transcription factors (TFs) are master regulators that orchestrate the regulation of many different aspects of plant development and responses (Shen et al., 2022; Zhao et al., 2022). In order to find potential regulatory genes of volatile terpene contents, we also searched for transcription factors in DEGs. As a result, a GRAS family gene (HD.08G0021230) showed significant up-regulation in the high-content group (fold change = 2.03). In addition, a strong positive correlation (r = 0.88) was found between geraniol content and the expression levels of a bHLH gene (HD.08G0006200).
To verify the accuracy of RNA-Seq results, four candidate genes related to terpene synthesis pathway and two TFs were selected for for qRT-PCR analysis. As shown in Figure 5, all candidate genes showed highly significant differences (n = 15, P < 0.01) between high-content group and low-content group, consist with RNA-Seq results.
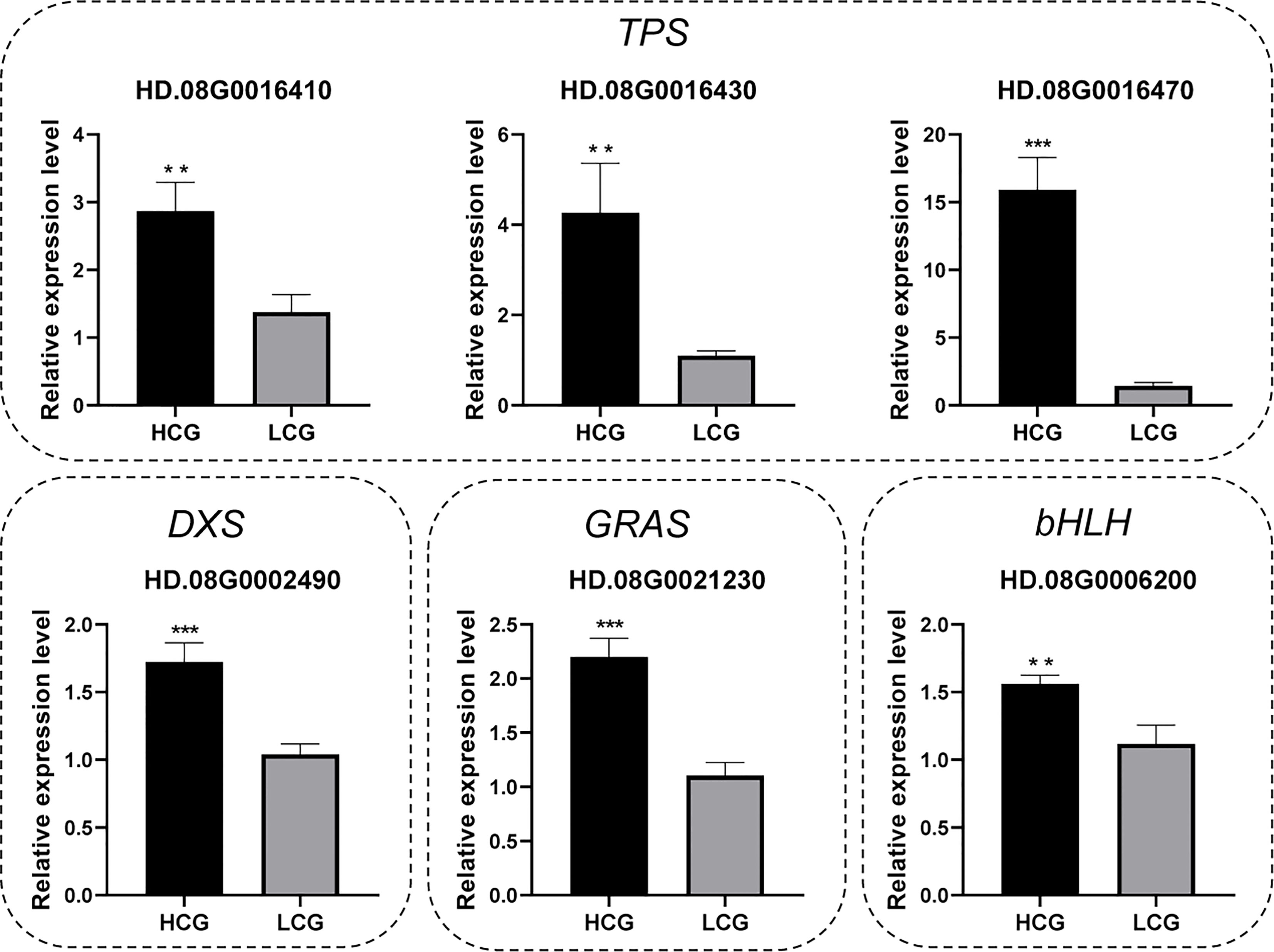
Figure 5 Verification of the candidate gene expression levels by qRT–PCR. Results are expressed as mean ± SEM (n = 15, ∗∗∗P < 0.001, ∗∗P < 0.01). HCG: high-content group, LCG: low-content group.
4 Discussion
4.1 QTL identification for volatile terpene contents
Mapping QTLs of aroma traits is challenging. Volatiles are influenced by a variety of environmental factors, and the determination of aroma is more complicated than soluble substances such as amino acids and caffeine. QTL mapping of fruit aroma compounds has been studied in several plants. For instance, aroma volatiles of Citrus reticulata were investigated in the 116 ‘Fortune’ × ‘Murcott’ F1 progeny using GC–MS, and a total of 206 QTLs were identified for 94 volatile compounds (Yu et al., 2017). In cucumber, a total of 28 QTLs associated with C6 and C9 aldehydes and alcohols were screened in a RIL population developed from Q16 × Q24 (Sun et al., 2022). Similar studies have been done on kiwifruit and apple (Yauk et al., 2015; Zeng et al., 2020). Nevertheless, QTL mapping of aroma phenotypes in tea plant has not yet been reported.
A fitting population and high-density genetic map suggested an effective way to identify QTLs related to aroma phenotypes. In this study, we constructed a high-density SNP linkage map using 148 F1 individuals developed from the controlled cross between two high-aroma oolong tea cultivars 'HD' and 'JX' using GBS. Compared with previously reported linkage maps, we achieved a higher map density as well as shorter marker distances (0.47 cM), which is comparable to the genetic map derived from the cross of ‘Longjing 43’ and ‘Baihaozao’ with an interval distance of 0.40 cM (Xu et al., 2018a), higher than that from the crossed between ‘Longjing 43’ and ‘Baijiguan’ (0.69 cM) (Huang et al., 2022b). Briefly, we identified 54 QTLs associated with 12 volatile terpenes for the first time. Previous studies have demonstrated the contribution of these targeted aromas to tea products, especially for those compounds with high contents and low thresholds such as geraniol, linalool and linalool oxides (Schuh and Schieberle, 2006; Xiao et al., 2022). Among them, six stable QTL were validated in these two years, the PVE of two stable QTLs (q01-5.1 and q08-5.2) for (E)-β-ocimene and geraniol were higher than 15%, which could be considered as major QTLs. Further, we found more than ten QTLs clustered in the same region on LG05 and LG11. The same result was also found in grape, major QTLs located at the top LG5 found for 14 monoterpenes, explained 14.7–48.1% of the total phenotypic variance (Koyama et al., 2022). These results indicated the presence of the same synthetic or regulatory pathway for those volatile terpenes in this study.
4.2 Candidate genes related to volatile terpene contents in Chr05 QTL cluster
Terpenoids as the most abundant class of natural products have been well-studied in plants (Singh and Sharma, 2014). In higher plants, terpenoids are synthesized either via the methylerythritol phosphate (MEP) pathway or the mevalonate (MVA) pathway (Dudareva et al., 2006). The common precursors for terpenoid synthesis, dimethylallyl pyrophosphate (DMAPP) and isopentenyl pyrophosphate (IPP), are produced independently by the MVA and MEP pathways, and it has been shown that there might be crosstalk and competition for substrates between the two pathways (Wu et al., 2006). The precursors of mono-, sesqui- and diterpenes, including geranylgeranyl pyrophosphate (GPP), farnesyl pyrophosphate (FPP) and geranylgeranyl pyrophosphate (GGPP), are formed by the condensation of 1:1, 2:1, and 3:1 IPP and DMAPP respectively, followed by TPS to form various terpenoids (Chen et al., 2011). Among the synthetic genes of terpenoid backbone, DXS is the first rate-limiting enzyme in the MEP pathway (Tian et al., 2022). The enzymatic activity of DXS was significantly correlated with the content of monoterpenes in grape (Dalla Costa et al., 2018). In this study, a differentially expressed DXS gene (HD.08G0002490) was found in Chr05 cluster. The expression levels of it showed positively correlated with the contents of geraniol. As we mentioned above, the Chr05 cluster has associated with almost all aroma traits and most of the volatile terpene contents showed a positive correlation. Hence, the substantial differences in expression level of this gene may suggest its essential role for total volatile terpene accumulation.
Most monoterpenes and sesquiterpenes synthesized by TPSs using GPP and FPP as direct substrates, respectively (Bohlmann et al., 1998). In tea plant, some TPS genes involved in the synthesis of (E)-nerolidol, α-farnesene, and linalool have been reported (Zhou et al., 2017; Liu et al., 2018; Wang et al., 2019a). But the mechanism of synthesis of some other volatile terpenes such as geraniol and nerol have remained unclear. Here, we found nine TPS genes in the Chr05 cluster according to gene annotation and three genes showed up-regulation in the high-content group which were consistent with the phenotypes. To predict the function of these three genes, we constructed a neighbor-joining phylogenetic tree that contained representative TPS sequences from several plant species (Figure S2). The results showed that all three genes are clustered into the TPS-b subclade, which belongs to the monoterpene synthase subfamily (Bohlmann et al., 1998). Previous studies have shown that a single terpene synthase may produce multiple products of terpenoid. For instance, a TPS-b α-farnesene synthase from apple (MdAFS1), can also synthesize the (E)-β-ocimene (Green et al., 2011). Likewise, distinct transcript splicing regulation of terpene synthase gene CsLIS/NES leads to the transformation of enzymatic function (nerolidol synthase or linalool synthase) in tea plant (Liu et al., 2018). Therefore, their specific functions merits further investigations.
Several TFs have been found to activate or repress terpenoid metabolism by binding to the promoter regions of TPSs or genes coding. LcERF19, an AP2/ERF transcription factor, could binding to LcTPS42 promoter and promoted its activity, which is related to the synthesis of geranial and neral in Litsea cubeba (Wang et al., 2022a). Belonging to the bHLH family, MYC2 has been shown to be involved in JA-regulated volatile aroma synthesis under stress in tea plant (Li et al., 2019). We found several TFs in Chr05 QTL cluster, such as bHLH, MYB, GRAS, and WRKY. Among them, the expression levels of a GRAS gene and a bHLH gene showed significant differences. In addition to bHlH family, it has been shown that the GRAS family is also involved in the regulation of volatile terpenes. For example, a scarecrow‐like (SCL) subfamily transcription factor SlSCL3 led to a significant decrease of expression of the corresponding TPS genes and volatile terpene contents when its transcription level was down-regulated in tomato (Yang et al., 2021). The results of QTL identification together with differences in expression suggested the potential roles of these two candidate TFs for volatile terpene accumulation. Further study on determining whether they can directly bind to the candidate TPS genes is warranted.
5 Conclusion
In summary, a high-density genetic linkage map of tea plant was constructed using GBS. The linkage map contained 15 linkage groups with a low inter-marker distance of 0.47 cM. A total of 42 QTLs associated with monoterpene contents and 12 QTLs associated with sesquiterpenes contents were identified. The high-density linkage map will serve as a foundation for tea genetic improvement. In the future, we will continue to adopt a strategy to fine map and identify QTL mapping intervals to further identify favorable target genes and verify their function.
Data availability statement
The datasets presented in this study can be found in online repositories. The names of the repository/repositories and accession number(s) can be found at https://www.ncbi.nlm.nih.gov/bioproject/PRJNA916459/.
Author contributions
LC and JM conceived and designed the experiments. SC, XL, and YL performed the experiments. SC, XL, and JC analyzed the data. SC, JM, and LC wrote the manuscript. All authors contributed to the article and approved the submitted version.
Funding
This work was supported by the National Natural Science Foundation of China (32202553, 32072631, U19A2030), the China Postdoctoral Science Foundation (2021M703546), and the Major Project of Agricultural Science and Technology in Breeding of Tea Plant Variety in Zhejiang Province (2021C02067).
Acknowledgments
We appreciate the Instrumental Analysis Center of Tea Research Institute Chinese Academy of Agricultural Sciences for analytical technique support.
Conflict of interest
The authors declare that the research was conducted in the absence of any commercial or financial relationships that could be construed as a potential conflict of interest.
Publisher’s note
All claims expressed in this article are solely those of the authors and do not necessarily represent those of their affiliated organizations, or those of the publisher, the editors and the reviewers. Any product that may be evaluated in this article, or claim that may be made by its manufacturer, is not guaranteed or endorsed by the publisher.
Supplementary material
The Supplementary Material for this article can be found online at: https://www.frontiersin.org/articles/10.3389/fpls.2023.1130582/full#supplementary-material
References
Bohlmann, J., Meyer-Gauen, G., Croteau, R. (1998). Plant terpenoid synthases: Molecular biology and phylogenetic analysis. Proc. Natl. Acad. Sci. U. S. A. 95 (8), 4126–4133. doi: 10.1073/pnas.95.8.4126
Chen, C., Chen, H., Zhang, Y., Thomas, H. R., Frank, M. H., He, Y., et al. (2020a). TBtools: an integrative toolkit developed for interactive analyses of big biological data. Mol. Plant 13 (8), 1194–1202. doi: 10.1016/j.molp.2020.06.009
Chen, F., Tholl, D., Bohlmann, J., Pichersky, E. (2011). The family of terpene synthases in plants: A mid-size family of genes for specialized metabolism that is highly diversified throughout the kingdom. Plant J. 66 (1), 212–229. doi: 10.1111/j.1365-313X.2011.04520.x
Chen, S., Xie, P., Li, Y., Wang, X., Liu, H., Wang, S., et al. (2021). New insights into stress-induced β-ocimene biosynthesis in tea (Camellia sinensis) leaves during oolong tea processing. J. Agric. Food Chem. 69 (39), 11656–11664. doi: 10.1021/acs.jafc.1c04378
Chen, S., Zhang, L., Cai, X., Li, X., Lei, B., Luo, Z., et al. (2020b). (E)-nerolidol is a volatile signal that induces defenses against insects and pathogens in tea plants. Hortic. Res. 7, 52. doi: 10.1038/s41438-020-0275-7
Dalla Costa, L., Emanuelli, F., Trenti, M., Moreno-Sanz, P., Lorenzi, S., Coller, E., et al. (2018). Induction of terpene biosynthesis in berries of microvine transformed with VvDXS1 alleles. Front. Plant Sci. 8, 2244. doi: 10.3389/fpls.2017.02244
Dellaporta, S. L., Wood, J., Hicks, J. B. (1983). A plant DNA minipreparation: Version II. Plant Mol. Biol. Rep. 1 (4), 19–21. doi: 10.1007/BF02712670
Dudareva, N., Negre, F., Nagegowda, D. A., Orlova, I. (2006). Plant volatiles: Recent advances and future perspectives. Crit. Rev. Plant Sci. 25 (5), 417–440. doi: 10.1080/07352680600899973
Feng, Z., Li, Y., Li, M., Wang, Y., Zhang, L., Wan, X., et al. (2019). Tea aroma formation from six model manufacturing processes. Food Chem. 285, 347–354. doi: 10.1016/j.foodchem.2019.01.174
Green, S., Baker, E. N., Laing, W. (2011). A non-synonymous nucleotide substitution can account for one evolutionary route to sesquiterpene synthase activity in the TPS-b subgroup. FEBS Lett. 585 (12), 1841–1846. doi: 10.1016/j.febslet.2011.04.038
Guo, X., Schwab, W., Ho, C.-T., Song, C., Wan, X. (2022). Characterization of the aroma profiles of oolong tea made from three tea cultivars by both GC–MS and GC-IMS. Food Chem. 376, 131933. doi: 10.1016/j.foodchem.2021.131933
Huang, F., Duan, J., Lei, Y., Kang, Y., Luo, Y., Chen, Y., et al. (2022a). Metabolomic and transcriptomic analyses reveal a MYB gene, CsAN1, involved in anthocyanins accumulation separation in F1 between ‘Zijuan’ (Camellia sinensis var. assamica) and ‘Fudingdabaicha’ (C. sinensis var. sinensis) tea plants. Front. Plant Sci. 13, 1008588. doi: 10.3389/fpls.2022.1008588
Huang, R., Wang, J.-Y., Yao, M.-Z., Ma, C.-L., Chen, L. (2022b). Quantitative trait loci mapping for free amino acid content using an albino population and SNP markers provides insight into the genetic improvement of tea plants. Hortic. Res. 9, uhab029. doi: 10.1093/hr/uhab029
Koyama, K., Kono, A., Ban, Y., Bahena-Garrido, S. M., Ohama, T., Iwashita, K., et al. (2022). Genetic architecture of berry aroma compounds in a QTL (quantitative trait loci) mapping population of interspecific hybrid grapes (Vitis labruscana × Vitis vinifera). BMC Plant Biol. 22 (1), 458. doi: 10.1186/s12870-022-03842-z
Li, J., Zeng, L., Liao, Y., Gu, D., Tang, J., Yang, Z. (2019). Influence of chloroplast defects on formation of jasmonic acid and characteristic aroma compounds in tea (Camellia sinensis) leaves exposed to postharvest stresses. Int. J. Mol. Sci. 20 (5), 1044. doi: 10.3390/ijms20051044
Lin, J., Zhang, P., Pan, Z., Xu, H., Luo, Y., Wang, X. (2013). Discrimination of oolong tea (Camellia sinensis) varieties based on feature extraction and selection from aromatic profiles analysed by HS-SPME/GC-MS. Food Chem. 141 (1), 259–265. doi: 10.1016/j.foodchem.2013.02.128
Liu, G.-F., Liu, J.-J., He, Z.-R., Wang, F.-M., Yang, H., Yan, Y.-F., et al. (2018). Implementation of CsLIS/NES in linalool biosynthesis involves transcript splicing regulation in Camellia sinensis. Plant Cell Environ. 41 (1), 176–186. doi: 10.1111/pce.13080
Liu, G., Yang, M., Fu, J. (2020). Identification and characterization of two sesquiterpene synthase genes involved in volatile-mediated defense in tea plant (Camellia sinensis). Plant Physiol. Biochem. 155, 650–657. doi: 10.1016/j.plaphy.2020.08.004
Ma, J.-Q., Jin, J.-Q., Yao, M.-Z., Ma, C.-L., Xu, Y.-X., Hao, W.-J., et al. (2018). Quantitative trait loci mapping for theobromine and caffeine contents in tea plant (Camellia sinensis). J. Agric. Food Chem. 66 (50), 13321–13327. doi: 10.1021/acs.jafc.8b05355
Mei, X., Liu, X., Zhou, Y., Wang, X., Zeng, L., Fu, X., et al. (2017). Formation and emission of linalool in tea (Camellia sinensis) leaves infested by tea green leafhopper (Empoasca (Matsumurasca) onukii Matsuda). Food Chem. 237, 356–363. doi: 10.1016/j.foodchem.2017.05.124
Schuh, C., Schieberle, P. (2006). Characterization of the key aroma compounds in the beverage prepared from Darjeeling black tea: Quantitative differences between tea leaves and infusion. J. Agric. Food Chem. 54 (3), 916–924. doi: 10.1021/jf052495n
Shen, Y., Li, X., Ma, G., Zhao, Y., Jiang, X., Gao, L., et al. (2022). Roles of YABBY tanscription factors in the regulation of leaf development and abiotic stress responses in Camellia sinensis. Beverage Plant Res. 2, 4. doi: 10.48130/BPR-2022-0004
Shi, J., Xie, D., Qi, D., Peng, Q., Chen, Z., Schreiner, M., et al. (2019). Methyl jasmonate-induced changes of flavor profiles during the processing of green, oolong, and black tea. Front. Plant Sci. 10, 781. doi: 10.3389/fpls.2019.00781
Shi, Y., Zhu, Y., Ma, W., Lin, Z., Lv, H. (2022). Characterisation of the volatile compounds profile of Chinese pan-fried green tea in comparison with baked green tea, steamed green tea, and sun-dried green tea using approaches of molecular sensory science. Curr. Res. Food Sci. 5, 1098–1107. doi: 10.1016/j.crfs.2022.06.012
Singh, B., Sharma, R. A. (2014). Plant terpenes: defense responses, phylogenetic analysis, regulation and clinical applications. 3 Biotech. 5, 129–151. doi: 10.1007/s13205-014-0220-2
Sun, Y., Li, X., Ma, Z., Chen, S. (2022). Quantitative trait locus mapping of fruit aroma compounds in cucumber (Cucumber sativus L.) based on a recombinant inbred line population. Hortic. Res. 9, uhac151. doi: 10.1093/hr/uhac151
Takeo, T. (1981). Variation in amounts of linalol and geraniol produced in tea shoots by mechanical injury. Phytochemistry 20 (9), 2149–2151. doi: 10.1016/0031-9422(81)80104-5
Tian, S., Wang, D., Yang, L., Zhang, Z., Liu, Y. (2022). A systematic review of 1-Deoxy-D-xylulose-5-phosphate synthase in terpenoid biosynthesis in plants. Plant Growth Regul. 96, 221–235. doi: 10.1007/s10725-021-00784-8
Wang, M., Gao, M., Zhao, Y., Chen, Y., Wu, L., Yin, H., et al. (2022a). LcERF19, an AP2/ERF transcription factor from Litsea cubeba, positively regulates geranial and neral biosynthesis. Hortic. Res. 9, uhac093. doi: 10.1093/hr/uhac093
Wang, P., Gu, M., Yu, X., Shao, S., Du, J., Wang, Y., et al. (2022b). Allele-specific expression and chromatin accessibility contribute to heterosis in tea plants (Camellia sinensis). Plant J. 112 (5), 1194–1211. doi: 10.1111/tpj.16004
Wang, P., Yu, J., Jin, S., Chen, S., Yue, C., Wang, W., et al. (2021). Genetic basis of high aroma and stress tolerance in the oolong tea cultivar genome. Hortic. Res. 8, 107. doi: 10.1038/s41438-021-00542-x
Wang, X., Zeng, L., Liao, Y., Li, J., Tang, J., Yang, Z. (2019a). Formation of α-farnesene in tea (Camellia sinensis) leaves induced by herbivore-derived wounding and its effect on neighboring tea plants. Int. J. Mol. Sci. 20 (17), 4151. doi: 10.3390/ijms20174151
Wang, C., Zhang, C., Kong, Y., Peng, X., Li, C., Liu, S., et al. (2017). A comparative study of volatile components in dianhong teas from fresh leaves of four tea cultivars by using chromatography-mass spectrometry, multivariate data analysis, and descriptive sensory analysis. Food Res. Int. 100, 267–275. doi: 10.1016/j.foodres.2017.07.013
Wang, Z., Zhang, Z., Tang, H., Zhang, Q., Zhou, G., Li, X. (2019b). High-density genetic map construction and QTL mapping of leaf and needling traits in Ziziphus jujuba mill. Front. Plant Sci. 10, 1424. doi: 10.3389/fpls.2019.01424
Wu, S., Schalk, M., Clark, A., Miles, R. B., Coates, R., Chappell, J. (2006). Redirection of cytosolic or plastidic isoprenoid precursors elevates terpene production in plants. Nat. Biotechnol. 24 (11), 1441–1447. doi: 10.1038/nbt1251
Xiao, Y., Tan, H., Huang, H., Yu, J., Zeng, L., Liao, Y., et al. (2022). Light synergistically promotes the tea green leafhopper infestation-induced accumulation of linalool oxides and their glucosides in tea (Camellia sinensis). Food Chem. 394, 133460. doi: 10.1016/j.foodchem.2022.133460
Xu, Q., He, Y., Yan, X., Zhao, S., Zhu, J., Wei, C. (2018b). Unraveling a crosstalk regulatory network of temporal aroma accumulation in tea plant ( Camellia sinensis ) leaves by integration of metabolomics and transcriptomics. Environ. Exp. Bot. 149, 81–94. doi: 10.1016/j.envexpbot.2018.02.005
Xu, L.-Y., Wang, L.-Y., Wei, K., Tan, L.-Q., Su, J.-J., Cheng, H. (2018a). High-density SNP linkage map construction and QTL mapping for flavonoid-related traits in a tea plant (Camellia sinensis) using 2b-RAD sequencing. BMC Genomics 19, 955. doi: 10.1186/s12864-018-5291-8
Yang, Z., Baldermann, S., Watanabe, N. (2013). Recent studies of the volatile compounds in tea. Food Res. Int. 53 (2), 585–599. doi: 10.1016/j.foodres.2013.02.011
Yang, C., Marillonnet, S., Tissier, A. (2021). The scarecrow-like transcription factor SlSCL3 regulates volatile terpene biosynthesis and glandular trichome size in tomato (Solanum lycopersicum). Plant J. 107 (4), 1102–1118. doi: 10.1111/tpj.15371
Yauk, Y.-K., Chagné, D., Tomes, S., Matich, A. J., Wang, M. Y., Chen, X., et al. (2015). The O-methyltransferase gene MdoOMT1 is required for biosynthesis of methylated phenylpropenes in ripe apple fruit. Plant J. 82 (6), 937–950. doi: 10.1111/tpj.12861
Yu, Y., Bai, J., Chen, C., Plotto, A., Yu, Q., Baldwin, E. A., et al. (2017). Identification of QTLs controlling aroma volatiles using a 'Fortune' x 'Murcott' (Citrus reticulata) population. BMC Genomics 18, 646. doi: 10.1186/s12864-017-4043-5
Yu, X., He, C., Li, Y., Zhou, J., Chen, Y., Yu, Z., et al. (2021). Effects of different spreading treatments on the formation of aroma quality in green tea. Beverage Plant Res. 1, 14. doi: 10.48130/BPR-2021-0014
Zeng, L., Liao, Y., Li, J., Zhou, Y., Tang, J., Dong, F., et al. (2017). α-farnesene and ocimene induce metabolite changes by volatile signaling in neighboring tea (Camellia sinensis) plants. Plant Sci. 264, 29–36. doi: 10.1016/j.plantsci.2017.08.005
Zeng, Y., Wang, M. Y., Hunter, D. C., Matich, A. J., McAtee, P. A., Knäbel, M., et al. (2020). Sensory-directed genetic and biochemical characterization of volatile terpene production in kiwifruit. Plant Physiol. 183 (1), 51–66. doi: 10.1104/pp.20.00186
Zeng, L., Xiao, Y., Zhou, X., Yu, J., Jian, G., Li, J., et al. (2021). Uncovering reasons for differential accumulation of linalool in tea cultivars with different leaf area. Food Chem. 345, 128752. doi: 10.1016/j.foodchem.2020.128752
Zhang, C.-C., Wang, L.-Y., Wei, K., Wu, L.-Y., Li, H.-L., Zhang, F., et al. (2016). Transcriptome analysis reveals self-incompatibility in the tea plant (Camellia sinensis) might be under gametophytic control. BMC Genomics 17 (1), 359. doi: 10.1186/s12864-016-2703-5
Zhao, H., Mallano, A. I., Li, F., Li, P., Wu, Q., Wang, Y., et al. (2022). Characterization of CsWRKY29 and CsWRKY37 transcription factors and their functional roles in cold tolerance of tea plant. Beverage Plant Res. 2, 15. doi: 10.48130/BPR-2022-0015
Zhao, M., Zhang, N., Gao, T., Jin, J., Jing, T., Wang, J., et al. (2020). Sesquiterpene glucosylation mediated by glucosyltransferase UGT91Q2 is involved in the modulation of cold stress tolerance in tea plants. New Phytol. 226 (2), 362–372. doi: 10.1111/nph.16364
Zheng, X.-Q., Li, Q.-S., Xiang, L.-P., Liang, Y.-R. (2016). Recent advances in volatiles of teas. Molecules 21 (3), 338. doi: 10.3390/molecules21030338
Keywords: tea plant, linkage analysis, quantitative trait loci, volatile terpene, RNA-seq
Citation: Chen S, Li X, Liu Y, Chen J, Ma J and Chen L (2023) Identification of QTL controlling volatile terpene contents in tea plant (Camellia sinensis) using a high-aroma ‘Huangdan’ x ‘Jinxuan’ F1 population. Front. Plant Sci. 14:1130582. doi: 10.3389/fpls.2023.1130582
Received: 23 December 2022; Accepted: 06 March 2023;
Published: 29 March 2023.
Edited by:
Mohan Lal, North East Institute of Science and Technology (CSIR), IndiaReviewed by:
Kai Zhang, Southwest University, ChinaChanglong Wen, Beijing Vegetable Research Center, China
Xiumin Fu, South China Botanical Garden (CAS), China
Copyright © 2023 Chen, Li, Liu, Chen, Ma and Chen. This is an open-access article distributed under the terms of the Creative Commons Attribution License (CC BY). The use, distribution or reproduction in other forums is permitted, provided the original author(s) and the copyright owner(s) are credited and that the original publication in this journal is cited, in accordance with accepted academic practice. No use, distribution or reproduction is permitted which does not comply with these terms.
*Correspondence: Jianqiang Ma, bWFqaWFucWlhbmdAdHJpY2Fhcy5jb20=; Liang Chen, bGlhbmdjaGVuQHRyaWNhYXMuY29t