- 1State Key Laboratory of Subtropical Silviculture, School of Forestry and Biotechnology, Zhejiang A&F University, Hangzhou, Zhejiang, China
- 2Hunan Academy of Forestry, Changsha, Hunan, China
Melatonin is a multifunctional molecule that has been widely discovered in most plants. An increasing number of studies have shown that melatonin plays essential roles in plant growth and stress tolerance. It has been extensively applied to alleviate the harmful effects of abiotic stresses. In view of its role in regulating aspects of plant growth and development, we ponder and summarize the scientific discoveries about seed germination, root development, flowering, fruit maturation, and senescence. Under abiotic and biotic stresses, melatonin brings together many pathways to increase access to treatments for the symptoms of plants and to counteract the negative effects. It has the capacity to tackle regulation of the redox, plant hormone networks, and endogenous melatonin. Furthermore, the expression levels of several genes and the contents of diverse secondary metabolites, such as polyphenols, terpenoids, and alkaloids, were significantly altered. In this review, we intend to examine the actions of melatonin in plants from a broader perspective, explore the range of its physiological functions, and analyze the relationship between melatonin and other metabolites and metabolic pathways.
Introduction
Melatonin (N-acetyl-5-methoxytryptamine), a bioactive molecule, is pervasive in organisms and has been well studied since it was first discovered in the pineal gland of cows (Lerner et al., 1958). Previous studies have demonstrated that melatonin is essential for maintaining human circadian rhythm, sleep, mood, body temperature, appetite, and immunological responses (Socaciu et al., 2020). Furthermore, antioxidant, anti-inflammatory, and other biological functions were also proven to be regulated by melatonin; thus, melatonin has been employed extensively for disease pathogenesis and drug development (Millet-Boureima et al., 2021).
A previous review on melatonin has provided insight into the biosynthesis, catabolism, and physiological and biochemical functions of this important molecule. The biosynthetic and metabolic pathways of melatonin, including tryptophan decarboxylase (TDC), tryptamine 5-hydroxylase (T5H), serotonin N-acetyltransferase (SNAT), N-acetylserotonin methyltransferase (ASMT), caffeic-O-methyltransferase (COMT), tryptophan hydroxylase (TPH), and hydroxyindole-O-methyltransferase (HIOMT), have been fully described by Zeng et al. (2022) and Kyoungwhan (2021). It is universally acknowledged that melatonin functions affect all aspects of the plant life cycle from seed germination to growth, maturation, and aging as well as aiding stressed plants in recovery (Zhang et al., 2022). Currently, scientists have elucidated the mechanisms by which melatonin alleviates stress by its function in antioxidants, photosynthesis, ion regulation, and stress signaling. Those basic roles of melatonin suggest that it could be an efficient method to ensure sustainable crop production and food safety. Here we review the myriad roles of melatonin and its possible molecular mechanisms integral to acclimatizing plants to climate action and self-consumption in times of stress. We examine the mechanisms of melatonin activities that underpin plant growth under various conditions and their effect on metabolic pathways and metabolites in response to stress.
The roles of melatonin in plant growth and development
An increasing number of studies have shown that melatonin has significant effects on growth and development, triggered by dramatic changes in metabolic status and diverse molecular and cellular processes (Wang et al., 2022a; Xie et al., 2022). The role of melatonin in plant life is shown in Figure 1.
Effect of melatonin on seed germination
Seed germination is the initial stage in the plant life cycle and involves the transportation of a series of signaling molecules and an array of gene expression changes through multiple complex physiological processes (Lee and Back, 2022). The crucial role of melatonin in stimulating seed germination was described by Zhang et al. (2022) and Wang et al. (2022), who reported that cell division, shoot initiation, and seed dormancy of Arabidopsis, cucumber (Cucumis sativus), bermudagrass (Cynodon dactylon), pepper (Capsicum annuum), and sweet corn were modulated by melatonin. Melatonin cooperates with hormones, such as indole-3-acetic acid (IAA), abscisic acid (ABA), gibberellin (GA), cytokinins (CKs), and salicylic acid (SA) (Yin et al., 2022)—for example, melatonin improves the germination rate of seeds by mediating changes in endogenous GA and ABA. Upregulating ABA catabolic genes (CYP707As and 8′hydroxylase genes) and GA biosynthesis genes (GA20ox and GA3ox) and downregulating ABA biosynthesis genes (NCEDs, LpZEP, and LpNCED1) contribute to improving hydrolytic enzyme activities (such as α-AMS and β-GAL) and provide the energy needed for seed germination and thus against germination constraints engendered by seed coat limits and embryo dormancy (Chen et al., 2021; Wang et al., 2022a). Genes belonging to the cytokinin-mediated signaling pathway (AtAHK2 and AtARRs) are also upregulated to promote cell division and shoot initiation in the presence of melatonin (Suzuki et al., 2002). The above-mentioned results imply that melatonin has multiple functions in the fine-tuning of hormone homeostasis and signaling in plants. Conversely, it should be noted that melatonin concentrations used to regulate seed germination are highly divergent among species, and its cooperation with ABA, GA, and auxin may impact sprout suppression (Dong D. et al., 2021; Yin et al., 2022). Furthermore, seeds coated with melatonin before sowing had improved germination rate and seed viability, which are specific to melatonin as an antioxidant to remove excess reactive oxygen species (ROS) accumulation and enhance the production capabilities of soluble sugars (Shi et al., 2015a; Wei et al., 2015; Teng et al., 2022). Under salt stress, promoting the synthesis of both new proteins and secondary metabolites is a key mechanism by which melatonin improves the seed germination rate in cotton (Gossypium hirsutum) (Chen et al., 2021), tomato (Solanum lycopersicum) (Xie et al., 2022), and cucumber (Zhang et al., 2017). In addition, melatonin upregulates protein levels, which are involved not only in stress tolerance but also in cell elongation, glycolysis, citric acid cycle, and glyoxylate cycle (Zhang et al., 2017). Therefore, melatonin works on seed germination partly by promoting energy production and invoking cellular processes and primary and secondary metabolism.
Regulation of melatonin on root development
Previous studies support the point that melatonin positively affects root developmental regulation (Park and Back, 2012; Mao et al., 2020). Larger root biomass and longer seminal root length are observed in many melatonin-treated plants, such as rice, tomato, and apple (Malus prunifolia) (Chen et al., 2019; Mao et al., 2020). Park and Back (2012) demonstrated the involvement of melatonin in an intervention for lateral root (LR) formation. Melatonin regulates the expression levels of the cell cycle-related genes SlCDKA1, SlCYCD3;1, and SlKRP2 by stimulating SlPAO1-H2O2 (polyamine oxidase) and SlRboh3/4-O2•− (respiratory burst oxidase homolog), leading to the initial development of lateral root primordia. Mao et al. (2020) showed that melatonin also promotes adventitious root formation. Additionally, the developmental process and correlations between melatonin and hormones (IAA, ABA, GA, and zeatin ribosid) are crucial for AR development—for instance, apple root formation is related to the melatonin-induced expression of WUSCHEL-RELATED HOMEOBOX GENE 11 (WOX11) along with IAA synthesis (Wang et al., 2022). However, the crosstalk is intricate—for example, melatonin impedes taproot growth regardless of its concentration in monocot canary grass (Phalaris canariensis) and oat (Avena sativa) (Wang et al., 2022a). Moreover, the advance of root development by melatonin is the discovery of novel signaling networks consisting of H2O2, NO, G protein, ROS, and Ca (Chen et al., 2019; Hu C. Z. et al., 2020). In melon (Cucumis melo), A. thaliana, and alfalfa (Medicago sativa), signal transduction provides deep insights for understanding the protective effects of exogenous and endogenous melatonin on LR formation in response to external stimuli (Hu Z. C. et al., 2020; Wang et al., 2022a).
Melatonin induces young branches, cuttings, calli, and in vitro cultures of sweet cherry and pomegranates to root (Wang et al., 2022a). Melatonin regulates the physiological and biochemical processes of callus regeneration and improves the differentiation of embryogenic cells, thus improving the regeneration rate under drought stress conditions (Zhou et al., 2022). Taken together, the fluctuation of endogenous melatonin contents may be a candidate determinant to reveal the seasonal effect on the tissue culture response and regeneration frequency of barley explants, which needs further study (Yang S. J. et al., 2022).
Various roles of melatonin in plant growth
Melatonin plays an indispensable role in plant growth. The growth, root yield, and sugar content of sugar beet seedlings were promoted when melatonin was applied (Zhang et al., 2021). Zhong et al. (2020) found that grape seedlings grew better when melatonin was applied by activating sucrose-decomposing enzymes and sucrose phosphate synthase to hydrolyze and synthesize sucrose as well as the transport of sucrose to nonphotosynthetic “sink” tissues (e.g., flowers, fruits, seeds, and roots). Furthermore, the regulation of melatonin in its own biosynthesis and degradation promotes specific mineral nutrition signal transduction, and within relatively stable ratios, it can balance the acquisition and appropriate of mineral nutrients, thus improving plant growth (Bawa et al., 2020; Sun et al., 2022). Regardless of whether nutrients (K, Fe, N, and S) are lacking or there is an excess of nutrients (N, Zn, Cu, and AI), there are detectable signs of optimization after melatonin application (Sun et al., 2022). Moreover, positive effects of external melatonin treatments under abiotic stress on seedling growth parameters and plant mineral contents (P, K, Ca, Mg, Fe, Zn, Cu, and Mn) were also observed by Bawa et al. (2020) and Sezer et al. (2021)—for example, when exposed to saline soil conditions, the exogenous application of melatonin to seedlings restores nutrient concentrations (Na+, Ca2+, and K+) and significantly increases Cu2+, Mn2+, and Zn2+ contents (Sezer et al., 2021).
Additionally, melatonin has been considered a circadian oscillator that affects the rhythm of the biological clock system and some physiological indicators in both mammals and plants (Chang et al., 2021). In the majority of plants (e.g., A. thaliana, rice, and barley), the melatonin concentrations are high at night (Chang et al., 2021; Ahn et al., 2021). Melatonin biosynthesis genes (TDC, T5H, SNAT, and particularly ASMT) are the core factors in adjusting the circadian clock of plant growth (Ahn et al., 2021). Consisting of light-dependent processes, melatonin production has regulatory relationships with some photoreceptors (such as phytochromes), whose deficiency will influence the gene expression duration of melatonin biosynthesis (Ahn et al., 2021). It is also worth noting that exogenous melatonin application restores the rhythmic expression of core circadian clock genes, while the absence of GIGANTEA genes leads to the non-rhythmic expression of ASMT, implying a potential melatonin-mediated signaling network (Chang et al., 2021; Ahn et al., 2021).
Additionally, melatonin influences plant growth by regulating the nitrogen (N) metabolism pathways. The nitrate transporter gene OsNPF6.5, glutamine synthetase gene OsGS2, and amino acid transporter gene OsAAP14 involved in N metabolism are induced in response to melatonin, and hence tiller number, nitrate uptake, and N use efficiency are affected (Wang et al., 2020). Melatonin regulates relevant key enzymes for N absorption and metabolism (such as S-nitrosoglutathione reductase and nitrate reductase) that are integral to triggering NO accumulation and its feedback (Zhang et al., 2022; Wang et al., 2022a). These valuable hints allow us to further appreciate the contribution of melatonin to plant growth and development, including but not limited to roots, stems, and leaves, which are apt to absorb nutrients and compete for resources.
The improvement of melatonin in plant reproductive growth
Melatonin has multiple functions in flowering. Melatonin is involved in distinct flowering pathways, including ambient temperature, vernalization, photoperiod, autonomous, GA, and age pathways (Wang et al., 2022a). In the autonomous pathway, it has been indicated that the effect of melatonin requires Flowering Locus C, which implies an innovative pathway in regulating floral transition in A. thaliana, with interconnectivity between melatonin and strigolactone (Yang et al., 2019). In GA pathways, the stabilization of transcriptional regulator DELLA proteins mediated by melatonin results in delayed translation (Yang et al., 2019; Wang et al., 2022a). Murch et al. (2009) and Lee and Back, (2019) found that the melatonin biosynthesis gene SNAT2 is significantly induced in Arabidopsis flowers and achieves the highest content in reproductive organs but gradually decreases as flowers mature. Fluctuations in melatonin levels also induce flowering delays, as shown in some algae and photoperiodic plants. In Antirrhinum majus, the number, size, and quality of flowers are improved by different concentrations of melatonin (Xiang et al., 2020). Furthermore, it is argued that melatonin influences volatile synthesis gene expression (such as TPS, DXS, BSMT, HAT, GGPS, and PAL in the terpenoid and benzenoid/phenylpropanoid pathways), thus regulating floral volatile compound content (Farhat et al., 2021).
Melatonin reverses the inhibitory effect of stress on pollen viability and germination (Hu W. et al., 2020). This might be attributed to exogenous melatonin at an appropriate dose, thus improving carbohydrate transport into the anthers (Hu et al., 2022). Melatonin promotes the transport of carbon assimilate from leaves to sink tissues by inducing the expression of sucrose transporters (SUT1 and SUT2) related to sucrose phloem loading under drought stress (Hu et al., 2022). Conversely, melatonin maintains carbohydrate metabolism in male and female tissues by accelerating sucrose decomposition and improving the availability of carbohydrates (Hu W. et al., 2020). In cotton anthers, the addition of 100 and 200 μM melatonin markedly stimulates the activities of the main rate-limiting enzymes of starch biosynthesis (AGPase and SSSase), and SuSy, cell wall, and vacuolar invertase activities are also elevated in wheat and tomato (Wang et al., 2022a). Further research revealed that melatonin contributes to higher male fertility of crops by increasing autophagy-related gene expression and autophagosome formation to restore the stability of tapetum cells under high temperature conditions (Qi et al., 2018). Similarly, Nguyen et al. (2010) also showed that melatonin regulates the tricarboxylic acid cycle to meet the energy demand under negative environmental conditions. As mentioned above, rather than being irretrievable, the losses of flower and crop yields recover due to melatonin regulation in multiple fields.
Melatonin regulates fruit maturation and post-management
Fruiting period and quality
Melatonin plays a role in the complicated process of fruit ripening, production, and quality (Wang et al., 2022). This stage is characterized by remarkable changes in the aroma, color, and flavor of the ripening fruit. Compared with control plants, the contents of organic acids, phenolics, flavonoids, peonidin derivatives, and apoptotic inhibitor proteins increased with melatonin treatment (Sun et al., 2016; Xie et al., 2022). Simultaneously, melatonin influences the expression of sucrose invertase genes and the net photosynthetic rate, thus increasing the solute content (e.g., soluble sugars) and pigment content (anthocyanins and carotenoids) in tomato, pear (Pyrus communis), and grape (Vitis labruscana) (Wang et al., 2022a; Xie et al., 2022). Melatonin triggers the metabolism of most hormones, e.g., melatonin increases ABA, H2O2, and ethylene content, participates in signaling molecules, and coordinates biochemical and developmental pathways that change the texture and nutritional quality (Xie et al., 2022). In apple, grape, tomato, and blackberry (Rubus fruticosus), the content of endogenous melatonin is changeable and accumulates the most in the flesh during the rapid growth phase, which coincides with the change trend of ethylene in the skin, suggesting its role in regulating phytohormone synthesis (Verde et al., 2022). Furthermore, melatonin-treated fruits have higher quality, number (e.g., higher 6.6% in blackberry), weight (e.g., higher 6.6% in grapes and 47.8% in pears), and size (Liu et al., 2019; Verde et al., 2022). Therefore, melatonin does have superior effects on all aspects of fruit development.
Post-management of fruit
After maturation, the occurrences of chilling injury, decay, moths, and microbes are the major limiting factors for post-management of the fruit, while melatonin has a unique superiority in maintaining fruits with good storability and quality (Xie et al., 2022). The present studies depict that, in banana, apple or pear, the exogenous application of melatonin distinctly reduces ethylene production during postharvest (Liu et al., 2019; Verde et al., 2022). Delay of senescence in cold-stored mangoes and tomato fruit by exogenous melatonin is attributed to the postponement of the climactic peak of ethylene due to a higher expression of biosynthesis-related genes (SlACS4), ethylene receptor genes (SlNR and SlETR4), and ethylene signaling-related genes (Sun et al., 2020; Dong J. X. et al., 2021). Additionally, during cold storage, melatonin reduces water runoff to regulate ethylene release (Anna and Petriccione, 2022). This view is justified based on two pieces of evidence: one is that melatonin-based coatings influence the expression of several genes, such as wax synthesis genes (CER1), cutin monomer genes (GPAT4/8), and aquaporin genes (PIP1;4, PIP2;7, and PIP22), which all determine the formation of a surface barrier composed of cuticle to reduce water outflow (Miranda et al., 2020; Anna and Petriccione, 2022). Another is that melatonin benefits metabolic processes (respiration and transpiration), which help to reduce the water vapor pressure gradient between the fruit and the surrounding atmosphere (Rastegar et al., 2020).
Fruits, such as mango and guava, treated with melatonin can maintain significantly higher unsaturated fatty acid levels and higher activities of enzymes (cytochrome c oxidase, H-ATPase, and Ca-ATPase) for the sake of a constant energy supply (Dong et al., 2021; Renu et al., 2022). Crucial enzymes of other processes activated by melatonin, such as the lipid metabolic pathway (LPS, LOX, and PLD), phenylpropanoid metabolic pathway (4CL and PAL), and shikimic acid pathway (P5CR, P5CS, and OAT), attenuate phosphoinositide and chlorophyll degradation or promote polyamine (PA) accumulation to aid in the fluidity and function of the cell membrane, particularly chloroplast membrane integrity (Aghdam et al., 2019; Silin et al., 2022; Renu et al., 2022). On the other hand, melatonin treatment improves the activities of antioxidant enzymes such as ascorbate peroxidase, glutathione S-transferase, and phenylalanine ammonia-lyase and upregulates genes coding for catalase, manganese superoxide dismutase, copper–zinc superoxide dismutase, monodehydroascorbate reductase, dehydroascorbate reductase, and glutathione reductase, which all decrease ROS, H2O2, and MDA production and polyphenol oxidase and lipoxygenase activities, resulting in the suppression of mildew and the visual symptoms (pitting, blackening, wrinkling, and browning) that are caused by microorganisms, insects, and brown pigments (Dong J. X. et al., 2021; Ze et al., 2021).
Melatonin functions against abiotic stress
In nature, the environment changes constantly, which is a huge challenge for plants suffering drought, fire, flood, and temperature stress. Melatonin has emerged as a defense potentiator to keep plants adapting by inducing the synthesis of endogenous melatonin or by improving the accumulation of metabolites (Zhang et al., 2022). By analyzing the transcriptional profile, the expression patterns of related genes (COMT, HIOMT, TDC, T5H, SNAT, and ASMT) under various abiotic conditions are shown (Ahn et al., 2021; Xing et al., 2021). Simultaneously, studies have reported that melatonin application modulates a variety of physiological processes to enhance plant tolerance, which is attributed to the increased endogenous melatonin content (Fan et al., 2018; Arnao et al., 2022). The subsequent chapters are organized according to the role of melatonin and related metabolites in determining plant stability under different stresses and how these changes occurred.
The effect of melatonin on photosynthesis
It is well known that plants gain energy through photosynthesis due to the sensitivity of chloroplasts to abiotic stresses; thus, the function of the photosystem and production yield are severely limited (Yang S. J. et al., 2021). Interestingly, the occurrence of photosynthesis and melatonin biosynthesis in the same organelle shows a complex connection, which is considered a natural self-protection strategy in plants to ensure stable photosynthesis and maximal food production under unstable environmental conditions (Marino et al., 2022). This result is supported by the phenotype of Arabidopsis overexpressing ASMT9, showing strong resistance to stress, in which melatonin concentrations, photosynthetic rate, fresh biomass, and dry biomass are significantly increased (Kang et al., 2010). Simultaneously, COMT1 knockout Arabidopsis exhibits reduced photosynthesis, carbon fixation, energy absorption and distribution, and heat stress reactions (Ahammed et al., 2018).
Melatonin has shown excellent protective effects on the integrity of the photosystem (Zhang et al., 2022)—for example, high salinity causes injury to the D1 subunit of PSII, but melatonin application is accompanied by a decline in the content of 34-kDa PSII reaction center protein (D1) and an increase in the content of PSII subunit S protein, which helps tomato and maize achieve high photosynthetic efficiency (Zho X. et al., 2016). Interestingly, melatonin reduces the photosystem protein levels under normal light but increases the PSII and PSI protein contents to cure photosystem damages under high light conditions (Yang S. J. et al., 2021). Melatonin is essential in maintaining a delicate balance between the breakdown and the synthesis of chlorophyll in plants (Khan et al., 2019). A comprehensive analysis of current results shows that melatonin protects chlorophyll from breakdown under stress in several ways, including the acceleration of de novo synthesis of chlorophyll or alleviation of chlorophyll loss (Yang S. J. et al., 2021). As mentioned above, those processes involve the expression of chlorophyll degradation-associated (NYC1, NOL, CLH, PPH, and PAO in melatonin-treated broccoli) and synthesis-associated genes (POR, CAO, and CHL G in tomato) as well as the accumulation of ABA and jasmonic acid (JA) to embody the renewal and stability of chlorophyll in the long term (Wu et al., 2021; Li et al., 2021). Melatonin substantially improves energy flux, which consists of absorption (ABS/RC), trapping energy (TRo/RC), and electron transport (ETo/RC), helping two Brassica napus cultivars reduce the toxic effects of polymetals (Ayyaz et al., 2021). Furthermore, in SNAT1-deficient Arabidopsis, the gene expression of chloroplast heat shock proteins (CpHSP70.1 and CpHSP70.2) and caseinolytic proteases (ClpR1, ClpR4, and ClpP1) is suppressed, while exogenous melatonin application reverses this response, implying that melatonin might participate in the quality control of chloroplast proteins (Lee and Back, 2018). Under the combined stress of low temperature and high humidity, melatonin pretreatment promotes the uptake and translocation of N, Mg, and Fe, which are responsible for chlorophyll biosynthesis and other physicochemical reactions (Amin et al., 2022). Evidence indicates that, when tomato is exposed to darkness for 4 days, melatonin increases the chlorophyll content, Fv/Fm value, and starch content and ameliorates carbon starvation-induced leaf chlorosis by activating the expression of miR171b or inhibiting glucan water dikinase gene expression (Wang et al., 2022b). Moreover, melatonin is involved in the process of the exchange between gas and water by changing the area and the density of stomata (Yang X. X. et al., 2022). Collectively, with the help of melatonin, photosynthetic mechanisms run smoothly, and cooperation efficiency and effectiveness are on the rise to adapt to ensuing changes under environmental stress.
Enhanced antioxidant system by melatonin
Studies have shown that melatonin is superior in equalizing the production and scavenging of ROS and reactive nitrogen species (RNS) (Lee and Back, 2022; Zhang et al., 2022). The mechanisms underlying the alleviation effects of melatonin on oxidative stress mainly involve crosstalk among various defensive response pathways (Zhang et al., 2022). One of the most effective ways to eliminate reactive oxygen species is to synthesize melatonin as a result of the enhanced antioxidant system (Zhang et al., 2022). In indirect ways, melatonin modulates antioxidant enzyme activities to improve their efficiency in ROS (H2O2 and O2−) detoxification. A series of antioxidant enzymes, POD, APX, SOD, and CAT, are induced by exogenous melatonin to dispose of excessive ROS accumulation (Zeng et al., 2022). Several studies have proposed that, under various abiotic stresses, the melatonin-mediated ascorbate–glutathione (AsA–GSH) cycle serves a crucial role in scavenging reactive oxygen species in cells (Xie et al., 2022; Zhang et al., 2022). Specifically, melatonin enhances the AsA/DHA and GSH/GSSG ratios and antioxidant capacity by stimulating the AsA–GSH cycle, thereby improving the scavenging capacity of O2·− and H2O2, reducing oxidative stress and providing an approach to reduce organic residues through plant detoxification mechanisms (Dong et al., 2018; Hodžić et al., 2021; Yan et al., 2022). In addition, melatonin could make a direct connection with various oxidizing agents, particularly with hydroxyl radicals, to reduce the damage to cell structures (one molecule of melatonin neutralizes a maximum of 10 molecules of ROS/RNS) (Simlat et al., 2018; Kyoungwhan, 2021). This evidence ties in neatly with the identity of melatonin as a direct antioxidant.
Transcription factors (TFs) are indispensable for regulating gene expression and cover stress-induced biological processes and related metabolic pathways that include cellular processes and primary and secondary metabolism (Xie et al., 2022). In cellular processes, melatonin-mediated gene expression requires TFs to further modulate metabolic processes during the aging of various seeds and maintain higher antioxidant enzyme activities than aging treatment alone (Biswojit et al., 2020). In particular, TFs such as WRKY, AP2/ERF-ERF, MYB, NAC, bZIP, and bHLH have a larger impact (Biswojit et al., 2020; Shi et al., 2015a; Zhao et al., 2021). Wei et al. (2018) confirmed that melatonin synthesis enzymes (MeTDC2 and MeASMT2/3) interact with MeWRKY20/75 to form a protein complex that extends the complex signaling of melatonin modulation. Not only ROS reduction in PSII but also circadian rhythm fluctuations have been mediated in melatonin-treated tomato, according to the weakened interaction between SlCV and SlPsbO/SlCAT3/SlM3H (Yu et al., 2022). In addition, a set of miRNAs (such as miR8029-3p, miR159-5p, miR858, and novel-m0048-3p) is able to negatively regulate target mRNAs in response to melatonin-mediated cold resistance (Li et al., 2016). Biswojit et al. (2020) observed the noticeable expression of secondary metabolite genes in the transcriptome profile. Similarly, phenolic compounds, flavonoids, and other representative nonenzymatic antioxidant compounds accumulate to control ROS levels via the NO-dependent pathway (Kim et al., 2010) and carotenoids (Sharma et al., 2020),
Carbohydrates/fatty acids and amino acids are well known to work as osmoprotectants and ROS scavengers (Marino et al., 2022). Iqbal et al. (2021) emphasized that melatonin initiates carbohydrate metabolism to resist oxidative stress by regulating related genes and repairing damaged membranes and proteins. A correlative study indicated that melatonin induces HSPs and autophagy and reduces oxidized proteins to promote cellular protein protection for the purpose of surmounting biological hurdles (Shi et al., 2015a; Xu et al., 2016). Furthermore, tobacco BY-2 cells, throughout the culture period, are able to synthesize starch by absorbing exogenous melatonin in large quantities to counteract stress-induced damage (Kobylińska et al., 2018). Several reports have shown that melatonin induces higher levels of 18 metabolites (such as cellobiose, galactose, and gentiobiose), 10 amino acids, five sugars (arabinose, mannose, maltose, glucopyranose, and turanose), five polyalcohols (dulcitol, galactinol, glycerol, myo-inositol, and sorbitol), one organic acid (propanoic acid), two sugar alcohols of the carbon metabolic pathway, and more expressed genes related to carbohydrate transcripts, including glycosyl-transferases, glycosyl-hydrolases, glycosyl-phosphatases, glycosyl-invertases, and glycosyl-mutases, hexokinases, mannosidases, α- and β-amylases, α- and β-glucan related-enzymes and several dehydrogenases (3-phosphoglycerate-, UDP-glucose-, alcohol- and aldehyde-), among others (Fan et al., 2015; Hernández-Ruiz et al., 2021). Specifically, in seeds, amid abiotic stress, plants generate important compatible solutes (such as starch and sucrose) to improve osmosis by advancing the expression of pectinesterase, malZ, sucrose-phosphate synthase, glgC, and PYG with the support of melatonin (Su et al., 2018). As previously described, it has been clearly demonstrated that melatonin mediates carbohydrate, polyalcohol, and other metabolite levels in response to osmoregulatory adaptation.
Melatonin-regulated signaling pathways in response to stress
Signal molecules significantly activate antioxidant responses when plants confront various environmental stress factors (Zhang et al., 2022). First, the regulation of defense-related gene expression, stomatal movement, root morphogenesis, and germination is evidently based on melatonin/NO-mediated signal cascades involving ROS, mitogen-activated protein kinase (MAPK), phytohormones, protein kinases (cyclic adenosine diphosphate ribose and cyclic guanosine monophosphate), and second messengers such as Ca2+ and cyclic guanosine monophosphate (Zhu et al., 2019). On the one hand, the multiple effects of NO and melatonin depend on their concentrations (Zhu et al., 2019)—for example, NO can regulate melatonin-related biosynthetic enzymes by forming N-nitrosomelatonin (a kind of NO donor in vitro as well) to promote melatonin production (Kaur and Bhatla, 2016). Melatonin improves nitrate reductase (NR) and NO synthase activities and related gene expression to regulate NO production, which further affects a variety of systems (Pardo-Hernández et al., 2020). In rapeseed under saline conditions, a signal cascade of NR and NO associated-1 is triggered by melatonin and NO in a concentration-dependent manner and influences the intensification of melatonin-induced S-nitrosylation that decreases because of the removal of NO (Zhao et al., 2018). Nitrosylation and NO2-Tyr are both NO-mediated posttranslational modifications that are related to the activities of some antioxidant proteins, thus helping to maintain the antioxidant capacity (Pardo-Hernández et al., 2020). Inextricably, melatonin stimulates endogenous NO content with a downregulation of s-nitroglutathione reductase expression in tomato seedlings, and an increased expression of glutathione S-transferase genes and AsA/GSH cycle genes (GR, APX, DHAR, and MDAR) in kiwifruit makes melatonin play a protective role in defending against damage by regulating antioxidant pathways (Liang et al., 2018; Xie et al., 2022). However, NO and melatonin interact in complex ways (Zhu et al., 2019). The reaction of compounds (such as serotonin, N-nitroso-melatonin, and sodium nitroprusside) releases NO, which interacts with melatonin to maintain oxidative homeostasis through the modulation of SOD isoforms (Cu/Zn SOD and Mn SOD) and the Na+/K+ ratio through the reprogramming of sodium hydrogen exchanger and salt overly sensitive 2 expression (Zhu et al., 2019). In Haematococcus pluvialis, the induction of melatonin enhanced light tolerance and nitrogen starvation resistance by activating the NO-dependent MAPK signal cascade (Zhu et al., 2019). Alleviation of salt-induced damage was also achieved effectively with the correlation factor of melatonin and nitric oxide signaling pathways and the accumulation of ONOO− (peroxynitrite anion) and O2·− (Arora and Bhatla, 2017).
Hormone signal transduction is essential for inducing biological processes and responses to environmental factors, which are known to activate gene transcription and regulate downstream metabolic processes (Su et al., 2018). Typically, melatonin helps to acclimate to thermal shock not only through the differential regulation of HSPs (HSFB3, HSFA1a, HSFA2b, HSP23, HSP70, HSP80, and HSP90) but also stimulates Ca2+ and hormone signal transduction to accelerate a general response to improve resistance (Xing et al., 2021). Eight subpathways (viz., IAA, ABA, CTK, GA, ETH, BR, JA, and SA) were also found to have genetic responses and were analyzed by Zhao et al. (2021)—for example, in the JA synthesis pathway, melatonin significantly regulates the transcripts of nine α-linolenic acid metabolism-related genes in maize seedling leaves (Zhao et al., 2021). In parallel, Jahan et al. (2021) presents a strong cross-connection among three signaling molecules (melatonin, GA, and ABA) and demonstrates that melatonin treatment repressed heat-induced leaf senescence either directly or indirectly by regulating the GA levels. The role of melatonin in the heat stress signaling response is evident in functioning upstream of H2S to reduce oxidative stress and increase antioxidative metabolism (Teng et al., 2022). This may suggest that melatonin traces the endogenous H2S-dependent pathway, wherein H+-ATPase-energized secondary active transport operates K+–Na+ homoeostasis (Teng et al., 2022). Siddiqui et al. (2021) refers to the stimuli of melatonin-mediated L-DES activity, endogenous H2S content, K+, and RWC retention that relies on the synergistic effect of melatonin and H2S on NaCl stress tolerance.
Furthermore, in melatonin-induced plants, sugars act as signaling molecules, while they positively or negatively regulate the expression of a variety of genes and enzyme activities of sugar-exporting (source) and sugar-importing (sink) tissues for the optimal synthesis and use of carbon and energy resources (Hernández-Ruiz et al., 2021). Teng et al. (2022) observed that treatment with 100 µM melatonin or high sucrose levels can trigger the downregulated expression of HXK and the upregulated expression of PFK7 so that source-to-sink phloem transport is promoted. Carbon metabolic flux of primary metabolism will be rebalanced as a consequence of elaborate management through these processes (TCA cycle and glycolysis) to meet the metabolism demand in organisms at risk (Teng et al., 2022). It is clear for melatonin-based mechanisms that various signal pathways are important for the integrity and fitness of living organisms.
Accumulation of secondary metabolites regulated by melatonin
To cope with plant stress signals, various melatonin-regulated metabolic pathways are accompanied by the accumulation of substances (such as flavonoids, polyamines, and phenolic compounds) and the improvement of organelle function (Marino et al., 2022). More attention to physiological and transcriptomic analyses of the effects of exogenous melatonin on stress tolerance has proven this perspective (Figure 2).
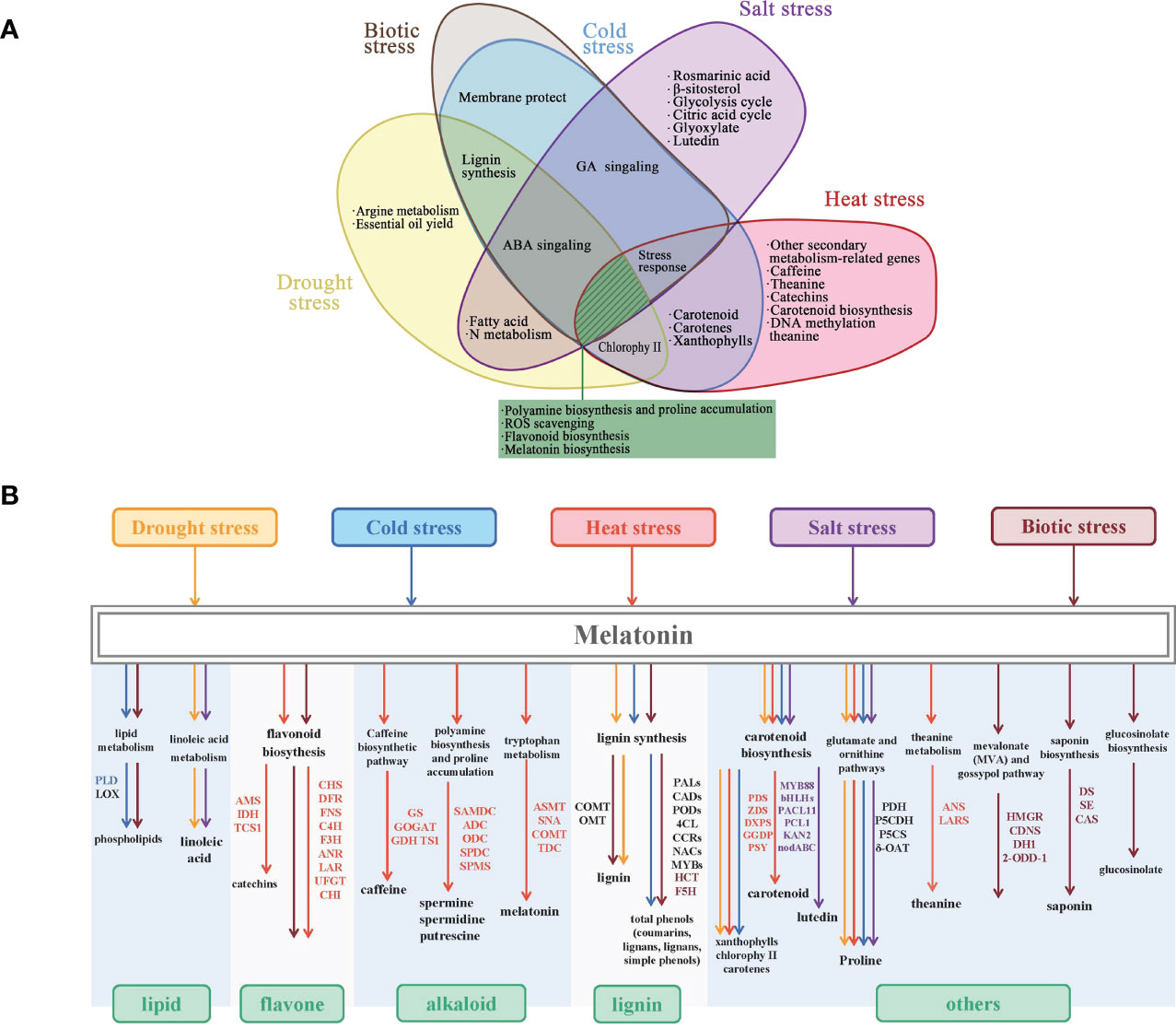
Figure 2 Synthesis and metabolism patterns of melatonin-induced secondary metabolites in response to stress. (A) Melatonin modulates various mechanisms against abiotic stresses (salt, cold, heat, and drought). (B) Melatonin induces common changes in gene expression in secondary metabolic pathways. PLD, phospholipase D; TCS1, tea caffeine synthase 1; CHS, chalcone synthase; DFR, dihydroflavonol 4-reductase; TIDH, inosine 5′-monophosphate dehydrogenase; LOX, lipoxygenase; AMS, S-adenosyl-l-methio-nine synthase; FNS, flavone synthase; C4H, cinnamic acid 4-hydroxylase; F3H, flavanone 3-hydroxylase; ANR, anthocyanidin reductase; LAR, leucoanthocyanidin 4-reductase; UFGT, UDP-glucose: flavonoid 3-O-glucosyltransferase; CHI, chalcone isomerase; GR, glutathione reductase; GSH, glutathione; GSNOR, S-nitrosoglutathione reductase; TS1, theanine synthase; ASMT, acetylserotonin O-methyltransferase; SNAT, serotonin N-acetyltransferase; COMT, caffeoyl-O-methyltransferase; TDC, tryptophan decarboxylase; OMT1, flavone 3′-O-methyltransferase 1; PAL, phenylalanine ammonia-lyase; CAD, cinnamyl alcohol dehydrogenase; POD, peroxidase; 4CL, 4-coumarate-CoA ligase; CCR, cinnamoyl CoA reductase; NACs, IAA-related factors; MYBs, regulator of CBF; PDS, phytoene desaturase; ZDS, ζ-carotene desaturase; bHLHs, basic helix–loop–helix; PCL1, phenylalanine ammonia lyase 1; PDH, pyruvate dehydrogenase; P5CDH, delta-1-pyrroline-5-carboxylate dehydrogenase; P5CS, pyrroline-5-carboxylate synthetase; OAT, ornithine aminotransferase; F5H, ferulic acid 5-hydroxylase; HCT, hydroxycinnamoyl transferase; HMGR, HMG-CoA reductase; CDNS, (+)-d-cadinene synthase; DH1, alcohol dehydrogenase; 2-ODD-1, 2-oxoglutarate/Fe(II)-dependent dioxygenase; DS, dammarene diol-II synthase; SE, squalene epoxidase; CAS, cyclohexene synthase.
Effects of melatonin on polyamine biosynthesis
PAs, namely, putrescine (Put), spermidine (Spd), and spermine (Spm), are considered a class of important regulators and have been shown to be involved in mitigating deleterious effects when plants suffer (Marino et al., 2022). Studies have demonstrated the association of melatonin and PA metabolism in response to environmental stress, as described in Bermuda grass (Shi et al., 2015b), carrot (Lei et al., 2004), peach (Cao et al., 2016), and Malus hupehensis (Gong et al., 2017)—for instance, melatonin, as a resistant initiator under diverse environmental stress conditions, including water shortage, low temperature, salt, and nutrient deficiency, can not only upregulate the synthesis genes (ODC, ADC, NCA, and SPDS) and downregulate the degradation genes (PAO and DAO) of PA but also stimulate the activities of related enzymes, including NR, ADC, ODC, nitrite reductase, glutamine synthetase (GS), glutamate synthase (GOGAT), and S-adenosylmethionine decarboxylase, inducing a significant increase in polyamines and endogenous melatonin content (Zhou C. et al., 2016; Talaat, 2021; Lei et al., 2022; Wang et al., 2022a). In addition, melatonin was found to accelerate the synthesis of amino acids (such as arginine and ornithine), which are precursors of bioactive compounds (such as Spd, Spm, Put, and GABA compounds) (Wang et al., 2022a). Therefore, by accelerating polyamine biosynthesis, melatonin attenuates apoptosis and disintegrates the membrane in cold-induced carrot and tomato (Ding et al., 2017; Aghdam et al., 2019). In melatonin-treated samples, the accumulated arginine and polyamine contents are also beneficial for nitrogen distribution and recycling, modulation of ion channel activities (specific polyamine-binding proteins) and Ca 2+ homeostasis, as well as the activation of NO signaling pathways, which are of prime importance to improve the resistance of Fe-deficient plants (Zhou C. et al., 2016; Wang et al., 2022a). Otherwise, the interplay between melatonin and SA, ABA, and ethylene accumulation plays crucial roles in the stress management of polyamines (Zhao et al., 2017). Both ABA and PA contents are increased in the crosstalk of melatonin and ABA, which upregulates an important stress-related gene, CsZat12, leading to the mitigation of chilling stress in cucumber (Zhao et al., 2017). However, in water-logged alfalfa, melatonin antagonizes ethylene production by suppressing ethylene biosynthesis-related gene expression and moves to PA biosynthesis (Zhang et al., 2019). Interestingly, melatonin metabolites (2-hydroxymelatonin) provide utility for regulating metabolism, particularly for enhancing Put, Spm, and Spd synthesis, and this exhibits a preferred orientation for studying the mechanism of melatonin to counteract environmental stress (Shah et al., 2020). A profound influence on plant resistance exerted by melatonin is evident herein through the elevated polyamine content, which scavenges free radicals, preserves nucleic acid and protein structures, and consequently improves membrane stability.
Effect of melatonin on flavonoid biosynthesis
Flavonoids have a main effect on protecting plants from abiotic stress by acting as a backup for peroxide production to eliminate various types of ROS (Dong et al., 2018). Studies designed with various stress conditions (such as cold, water deficiency, salt, etc.) show that melatonin promotes secondary metabolite accumulation, including flavones, flavanone, luteolin, and isoflavone (Ithal and Reddy, 2004; Song et al., 2022). It was also reported that the transcriptional levels of PCL, CHS, DFR, FNS, C4H, F3H, ANR, LAR, UFGT, CHI, and IFS genes involved in flavonoid biosynthesis were significantly increased by melatonin treatment (Dong et al., 2018; Song et al., 2022). In addition, PAL and PPO have been shown to be upregulated in Dracocephalum moldavica, Vigna radiata, and Sesamum indicum treated with melatonin, and damage inhibition was observed (Naghizadeh et al., 2019). In heat-treated chrysanthemum and kiwifruit, cold-stressed white clover and kiwifruit, and salt-induced pigeon pea, similar data have also been obtained—that is, melatonin favors the production of flavonoids, carotenoids, carotenes, xanthophylls, and chlorophyll II to protect the photosynthetic potential and mitigate adverse effects (Sun et al., 2016; Liang et al., 2018; Dong et al., 2018; Sun et al., 2020; Xing et al., 2021; Song et al., 2022). Isoflavone biosynthesis responds to melatonin profoundly through hormone biosynthesis involving upregulated genes (AOS, AAO, SAMA, and ACCO), which has conferred a broader understanding of the melatonin regulatory mechanism (Gyanendra et al., 2021). Otherwise, it has been verified by Song et al. (2022) that melatonin greatly decreases genomic DNA methylation and modified gene expression. Therefore, the outcome of increased disease resistance and flavonoid biosynthesis is at least partially achieved by utilizing melatonin to decrease the methylation of related gene promoters, such as EDS1 (Gao et al., 2020). Conversely, in some cases, among gardenia and apples, melatonin decreases the related gene expression levels of flavonoids but with a greater retention of carotenoids so that plants remain greener and have brighter leaves after 24 days in dark conditions. This internal indication illustrated that melatonin-treated plants adapted better to the adverse environment (Wang et al., 2018). Similarly, overexpressing MsASMT1 in alfalfa makes it grow faster and have higher endogenous melatonin levels, which, in turn, suppress flavonoid biosynthesis (28 downregulated genes), mainly quercetin, kaempferol, formononetin, and biochanin (Cen et al., 2020). Notably, flavonoids are potent in inhibiting in vivo melatonin biosynthesis by abolishing the activities of ASMT, SNA, and COMT (Kyungjin et al., 2018). In terms of the objective use of melatonin, some notable changes in flavonoids are used to establish the ideal dose to influence plant resistance to develop effective solutions and to provide a fresh perspective that explores the connection between melatonin and secondary metabolites.
Effect of melatonin on other secondary metabolites
Multiple secondary metabolisms are associated with plant stress tolerance, while in many persuasive studies, melatonin application shows an increase in total phenolic content, total flavonoid content, rosmarinic acid, luteolin flavone, apigenin flavone, photosynthetic pigment, ascorbic acid, phenol, and essential oil (EO) yield (Saad and Salem, 2019; Farinaz et al., 2020). Taking phenolic compounds as an example, the levels of resveratrol, caffeic, chlorogenic, and gallic acids can be redistributed by melatonin in stressful situations (Xu et al., 2018). Scientists have clearly demonstrated that melatonin function in moderately high-temperature-stressed tea is accompanied by increased contents of catechins, theanine, and caffeine, which are related to upregulated genes, including AMS, IDH, TCS1, ANS, LAR, GS, GOGAT, GDH, and TS1 (Xin et al., 2020).
As a crucial secondary metabolite generated through the phenylalanine metabolic pathway, lignin has practical effects on protective barriers (Wang et al., 2019). Melatonin addition can compensate for the blockaded lignin accumulation and the decreased oxidase activities (such as PAL, CAD, and POD) caused by low temperature. This evidence indicates that, under cold stress, melatonin regulates lignin synthesis genes (ZlPAL1/2/3/4, ZlCAD1/2/3, and ZlPOD1/2/3/4/5) and TF expression (NAC and MYB family) in bamboo and therefore is expected to benefit from horticultural management with melatonin application (Li C. T. et al., 2019). This result is consistent with that of Wang et al. (2021). Additionally, melatonin acts as a positive signal that enhances P. lactiflora stem strength and lignin accumulation by regulating the expression of genes (PAL, 4CL, CCR, and CAD) in lignin biosynthesis (Zhao et al., 2022). Given the resistance of V. dahliae, the gossypol and lignin contents in cotton were increased by melatonin through changes in the metabolic flux of different pathways [mevalonate (MVA), phenylpropanoid, and gossypol pathways] (Li Z. et al., 2019).
Other secondary metabolites, such as glucosinolates, are known to defend against herbivores and pathogenic attacks (Selmar, 2010). Some secondary metabolites are relevant to maintaining plant commercial life when florets of broccoli treated with melatonin can raise physical–chemical parameters, including color, texture, shine, and rates of weight loss (Arnao et al., 2022). Saponins and alkaloids, both mediated by melatonin, are beneficial for the self-protection of plants (Ptak et al., 2019; Yang Q. et al., 2021). For chromium-treated rosemary, melatonin applications will increase its yield and EO yield by up to 25% and 100%, respectively (Farouk and Al-Amri, 2019). Moreover, as an active phytosterol, β-sitosterol significantly lowers stress caused by high salt levels in tomato and sunflower (Helianthus annuus) and is contained in many plant species, and its density and production are significantly increased in dehydrated plants when exposed to melatonin treatment, thus hinting at the underlying relationship (Fawzia et al., 2016; Gamel et al., 2017; Ramadan et al., 2018).
Further exploration of the role of melatonin may greatly enhance specific secondary metabolite synthesis, thereby improving defense capability and leading to less cell death and disease in living organisms, instead of some stress-induced processes, such as lipid peroxidation.
Melatonin functions against biotic stress
Melatonin is unique in the mitigation of plant biological stress. First, the identification of melatonin as a scavenger has cleaned not only ROS and RNS but also viruses (Mohamed et al., 2019). Apples infected with apple stem grooving virus shed light on this discovery in such a way that melatonin eradicates the virus from previously infected shoot tips (Mohamed et al., 2019). The second is melatonin-activated bioactivity, such as phagocyte and plant resistance (R) proteins (Mohamed et al., 2019; Rahul et al., 2022). Relatedly, regulated genes are mapped to signal transduction networks (Rahul et al., 2022). Melatonin upregulates defense genes through SA, ethylene, and NO signaling, and another hormonal crosstalk (CKs, ABA, IAA, and GA) also involves melatonin in systemic acquired resistance (Mohamed et al., 2019; Rahul et al., 2022). Additionally, melatonin stimulation induces a response from the MAPK cascade as confirmed in (Pst)-DC3000-infected Arabidopsis and SlMPAK3-deficient tomato infected with Botrytis cineraria, which also leads to the initial PAMP-triggered immunity response and effector-triggered immunity response (Rahul et al., 2022). Thirdly, plants will gain strengthened physical obstacles in that more complex chemicals are generated, and genes of dammarene diol-II synthase (DS), squalene epoxidase (SE), and cyclohexene synthase in saponin biosynthesis, HMG-CoA reductase (HMGR), (+)-d-cadinene synthase, alcohol dehydrogenase in the MVA and gossypol pathways, and other genes in melatonin, flavonoid, lipid, and lignin biosynthesis are upregulated by melatonin stimuli (Mohamed et al., 2019; Gao et al., 2020; Yang Q. et al., 2021).
Conclusions
Melatonin has a unique role in every stage of the plant life cycle, particularly in reversing environmental pressure. It is produced in the cytoplasm, chloroplasts, and mitochondria, which may be conducive to easy movement and prompt response. Several interpretations to explain the stress protective function of melatonin in plants have been proposed in this paper and zeroes in on the regulation of primary and secondary metabolism with the objective of capturing its versatile role in the plant hormone network and general physiological processes. At the same time, melatonin regulates a wide range of molecular mechanisms, not only interacting with proteins and affecting nutrient metabolism in plants but also having functional effects on noncoding RNA. This potential gives melatonin priority over pesticides and fertilizers to become a sort of pollution-free solution maintaining the supply for plant-based foods and products. To varying degrees, melatonin and its precursors and metabolites help to enhance plant immunity and development. These data indicate another pioneering work on melatonin pathway application that provides multiple options for breeding work. Overall, the study of the real risks and benefits of many secondary metabolites in different plants is a way to decipher their genetic structures and inherent gifts. It would be a marked progress if we knew how they work with melatonin in such a way that arouses strong resistance toward stresses in unexpected ways.
Author contributions
YP and JZ: conceptualization. YP and XX: writing of the manuscript and preparation of the figures. LL, QS, QW, HH, ZT, and JZ: revising and editing. All authors contributed to the article and approved the submitted version.
Funding
This work was financially supported by Zhejiang Science and Technology Major Program on Agricultural New Variety Breeding (2021C02070-10), the National Natural Science Foundation of China (31971677), and Opening Project of State Key Laboratory of Tree Genetics and Breeding (Northeast Forestry University).
Conflict of interest
The authors declare that the research was conducted in the absence of any commercial or financial relationships that could be construed as a potential conflict of interest.
Publisher’s note
All claims expressed in this article are solely those of the authors and do not necessarily represent those of their affiliated organizations, or those of the publisher, the editors and the reviewers. Any product that may be evaluated in this article, or claim that may be made by its manufacturer, is not guaranteed or endorsed by the publisher.
References
Aghdam, M. S., Luo, L. H., Jannatizadeh, A., Sheikh-Assadi, M., Sharafi, Y., Farmani, B., et al. (2019). Employing exogenous melatonin applying confers chilling tolerance in tomato fruits by upregulating ZAT2/6/12 giving rise to promoting endogenous polyamines, proline, and nitric oxide accumulation by triggering arginine pathway activity. Food Chem. 275, 549–556. doi: 10.1016/j.foodchem
Ahammed, G. J., Xu, W., Liu, A., Chen, S. (2018). COMT1 silencing aggravates heat stress-induced reduction in photosynthesis by decreasing chlorophyll content, photosystem II activity, and electron transport efficiency in tomato. Front. Plant Sci. 9. doi: 10.3389/fpls.2018.00998
Ahn, H. R., Kim, Y., Lim, Y. J., Duan, S. C., Eom, S. H., Jung, K. H. (2021). Key genes in the melatonin biosynthesis pathway with circadian rhythm are associated with various abiotic stresses. Plants 48, 9–19. doi: 10.3390/plants10010129
Amin, B., Atif, M. J., Meng, H. W., Ali, M., Li, S. J., Alharby, H. F., et al. (2022). Melatonin rescues photosynthesis and triggers antioxidant defense response in cucumis sativus plants challenged by low temperature and high humidity. Front. Plant Science 13. doi: 10.3389/fpls.2022.855900
Anna, M., Petriccione, M. (2022). Melatonin treatment reduces qualitative decay and improves antioxidant system in highbush blueberry fruit during cold storage. J. Sci. Food Agriculture 102, 4229–4237. doi: 10.1002/jsfa.11774
Arnao, M. B., Cano, A., Hernández-Ruiz, J. (2022). Phytomelatonin: An unexpected molecule with amazing performances in plants. J. Exp. Bot 73, 5779–5800. doi: 10.1093/jxb/erac009
Arora, D., Bhatla, C. S. (2017). Melatonin and nitric oxide regulate sunflower seedling growth under salt stress accompanying differential expression of Cu/Zn SOD and Mn SOD. Free Radical Biol. Med. 106, 315–328. doi: 10.1016/j.freeradbiomed.2017.02.042
Ayyaz, A., Farooq, A. M., Dawood, M., Majid, A., Javed, M., Athar, H. R., et al. (2021). Exogenous melatonin regulates chromium stress-induced feedback inhibition of photosynthesis and antioxidative protection in brassica napus cultivars. Plant Cell. 40, 2063–2080. doi: 10.1007/s00299-021-02769-3
Bawa, G., Feng, L., Shi, J., Chen, G., Cheng, Y., Luo, J., et al. (2020). Evidence that melatonin promotes soybean seedlings growth from low-temperature stress by mediating plant mineral elements and genes involved in the antioxidant pathway. Funct. Plant Biol. 47, 815–824. doi: 10.1071/FP19358
Biswojit, D., Li, M., Liu, S., Pan, T. F., Ma, C. L., Qiu, D. L. (2020). Melatonin-mediate acid rain stress tolerance mechanism through alteration of transcriptional factors and secondary metabolites gene expression in tomato. Ecotoxicology Environ. Safety 200, 110720. doi: 10.1016/j.ecoenv.2020.110720
Cao, S., Song, C., Shao, J., Bian, K., Chen, W., Yang, Z. (2016). Exogenous melatonin treatment increases chilling tolerance and induces defense response in harvested peach fruit during cold storage. J. Agric. Food Chem. 64, 5215–5222. doi: 10.1021/acs.jafc.6b01118
Cen, H. F., Wang, T. T., Liu, H. Y., Wang, H., Tian, D. Y., Li, X., et al. (2020). Overexpression of MsASMT1 promotes plant growth and decreases flavonoids biosynthesis in transgenic alfalfa (Medicago sativa l.). Front. Plant Sci 11, 489. doi: 10.3389/fpls.2020.00489
Chang, T., Zhao, Y., He, H., Xi, Q., Fu, J., Zhao, Y. (2021). Exogenous melatonin improves growth in hulless barley seedlings under cold stress by influencing the expression rhythms of circadian clock genes. Peer J. 9, e10740. doi: 10.7717/peerj.10740
Chen, J., Li, H., Yang, K., Wang, Y. Z., Yang, L. F., Hu, L. B., et al. (2019). Melatonin facilitates lateral root development by coordinating PAO-derived hydrogen peroxide and rboh-derived superoxide radical. Free Radical Biol. Med. 143, 534–544. doi: 10.1016/j.freeradbiomed.2019.09.011
Chen, L., Lu, B., Liu, L. T., Duan, W. J., Jiang, D., Li, J., et al. (2021). Melatonin promotes seed germination under salt stress by regulating ABA and GA3 in cotton (Gossypium hirsutum l.). Plant Physiol. Biochem. 162, 506–516. doi: 10.1016/j.plaphy.2021.03.029
Ding, F., Liu, B., Zhang, S. X. (2017). Exogenous melatonin ameliorates cold-induced damage in tomato plants. Scientia Horticulturae 219, 264–271. doi: 10.1016/j.scienta.2017.03.029
Dong, L., Gao, F., Ni, Z. Y., Lin, L. J., Deng, Q. X., Tang, Y., et al. (2018). Melatonin improves heat tolerance in kiwifruit seedlings through promoting antioxidant enzymatic activity and glutathione s-transferase transcription. Molecules 23, 584. doi: 10.3390/molecules23030584
Dong, J. X., Kebbeh, M., Yan, R., Huan, C., Jiang, T. J., Zheng, X. L. (2021). Melatonin treatment delays ripening in mangoes associated with maintaining the membrane integrity of fruit exocarp during postharvest. Plant Physiol. Biochem. 169, 22–28. doi: 10.1016/j.plaphy.2021.10.038
Dong, D., Wang, M. D., Yinreuizhi, Li, ZC, L., SW, Li, YH, C., et al. (2021). Melatonin influences the early growth stage in zoysia japonica steud. by regulating plant oxidation and genes of hormones. Sci. Rep. 11, 12381. doi: 10.1038/s41598-021-91931-8
Fan, J. B., Hu, Z. G., Xie, Y., Chan, Z. L., Chen, K., Amombo, E., et al. (2015). Alleviation of cold damage to photosystem II and metabolisms by melatonin in bermudagrass. Front. Plant Sci 6, 925. doi: 10.3389/fpls.2015.00925
Fan, J., Xie, Y., Zhang, Z., Chen, L. (2018). Melatonin: A multifunctional factor in plants. Int. J. Mol. Sci. 19, 1528. doi: 10.3390/ijms19051528
Farhat, A., Zhou, Y. W., He, J. J., Ke, Y. G., Qin, W., Yu, R. C., et al. (2021). Metabolite and transcriptome profiling analysis revealed that melatonin positively regulates floral scent production in hedychium coronarium. Front. Plant Science 12. doi: 10.3389/fpls.2021.808899
Farinaz, V., Amooaghaiea, R., Ehsanzadehc, P., Ghanadiand, M., Talebie, M., Ghanati, F., et al. (2020). Melatonin and calcium modulate the production of rosmarinic acid, luteolin, and apigenin in dracocephalum kotschyi under salinity stress. Phytochemistry 177, 112422. doi: 10.1016/j.phytochem.2020.112422
Farouk, S., Al-Amri, S. M. (2019). Ameliorative roles of melatonin and/or zeolite on chromium-induced leaf senescence in marjoram plants by activating antioxidant defense, osmolyte accumulation, and ultrastructural modification. Ind. Crops Products 142, 111823. doi: 10.1016/j.indcrop.2019.111823
Fawzia, A. E., Ashraf, A. E., Samia, A. H., Hend, A. E. (2016). β-sitosterol ameliorates the chemical constituents of sunflower (Helianthus annuus l.) plants, grown under saline condition. J. Pharm. Biol. Sci 11, 36–45. doi: 10.9790/3008-1103033645
Gamel, R. E., Elsayed, A., Bashasha, J., Haroun, S. (2017). Priming tomato cultivars in β-sitosterol or gibberellic acid improves tolerance for temperature stress. Int. J. Bot. 13, 1–14. doi: 10.3923/ijb.2017.1.14
Gao, S. W., Ma, W. Y., Lyu, X. N., Cao, X. L., Yao, Y. X. (2020). Melatonin may increase disease resistance and flavonoid biosynthesis through effects on DNA methylation and gene expression in grape berries. BMC Plant Biol. 20, 231. doi: 10.1186/s12870-020-02445-w
Gong, X. Q., Shi, S. T., Dou, F. F., Song, Y., Ma, F. W. (2017). Exogenous melatonin alleviates alkaline stress in malus hupehensis rehd. by regulating the biosynthesis of polyamines. Molecules 22, 1542. doi: 10.3390/molecules22091542
Gyanendra, K., Saad, K. R., Puthusseri, B., Arya, M., Shetty, P. N., Giridhar, P. (2021). Exogenous serotonin and melatonin regulate dietary isoflavones profoundly through ethylene biosynthesis in soybean [Glycine max (L.) merr.]. J. Agric. 69, 1888–1899. doi: 10.1021/acs.jafc.0c07457
Hernández-Ruiz, J., Cano, A., Reiter, R. J. (2021). Melatonin and carbohydrate metabolism in plant cells. Plants 10, 1917. doi: 10.3390/plants10091917
Hodžić, E., Galijašević, S., Balaban, M., Rekanović, S., Makić, H., Kukavica, B., et al. (2021). The protective role of melatonin under heavy metal-induced stress in Melissa officinalis l. Turkish J. Of Chem. 45, 737–748. doi: 10.3906/kim-2012-7
Hu, W., Cao, Y. T., Loka, D. A., Harris-Shultz, R. K., Reiter, R. J., Ali, S., et al. (2020). Exogenous melatonin improves cotton (Gossypium hirsutum l.) pollen fertility under drought by regulating carbohydrate metabolism in Male tissues. Plant Physiol. Biochem. 151, 579–588. doi: 10.1016/j.plaphy.2020.04.001
Hu, Z. C., Fu, Q. S., Zheng, J., AA, Z., Wang, H. S. (2020). Transcriptomic and metabolomic analyses reveal that melatonin promotes melon root development under copper stress by inhibiting jasmonic acid biosynthesis. Horticulture Res. 7, 79. doi: 10.1038/s41438-020-0293-5
Hu, W., Cao, Y. T., Loka, D. A., Harris-Shultz, K. R., Reiter, R. J., Ali, S., et al. (2022). Exogenous melatonin improves cotton (Gossypium hirsutum L.) pollen fertility under 1 drought by regulating carbohydrate metabolism in male tissues. Plant Physiol. Biochem. 151, 579–588. doi: 10.3390/plants10091778
Iqbal, N., Fatma, M., Gautam, H., Umar, S., Sofo, A., Dippolito, I., et al. (2021). The crosstalk of melatonin and hydrogen sulfide determines photosynthetic performance by regulation of carbohydrate metabolism in wheat under heat stress. Plants 10, 1778. doi: 10.3390/plants10091778
Ithal, N., Reddy, A. R. (2004). Rice flavonoid pathway genes, OsDfr and OsAns, are induced by dehydration, high salt and ABA, and contain stress responsive promoter elements that interact with the transcription activator, OsC1-MYB. Plant Science 166, 1505–1513. doi: 10.1016/j.plantsci.2004.02.002
Jahan, M. S., Shu, S., Wang, Y., Hasan, M. M., El-Yazied, A. A., Alabdallah, NM, et al. (2021). Melatonin pretreatment confers heat tolerance and repression of heat-induced senescence in tomato through the modulation of ABA- and GA-mediated pathways. Front. Plant Science 12. doi: 10.3389/fpls.2021.650955
Kang, K., Lee, K., Park, S., Kim, Y., Back, K. (2010). Enhanced production of melatonin by ectopic overexpression of human serotonin n-acetyltransferase plays a role in cold resistance in transgenic rice seedlings. J. Pineal Res. 49, 176–182. doi: 10.1111/j.1600-079X
Kaur, H., Bhatla, S. C. (2016). Melatonin and nitric oxide modulate glutathione content and glutathione reductase activity in sunflower seedling cotyledons accompanying salt stress. Nitric. Oxide 59, 42–53. doi: 10.1016/j.niox.2016.07.001
Khan, M. N., Zhang, J., Luo, T., Liu, J. H., Rizwan, M., Fahad, S., et al. (2019). Seed priming with melatonin coping drought stress in rapeseed by regulating reactive oxygen species detoxification: antioxidant defense system, osmotic adjustment, stomatal traits and chloroplast ultrastructure perseveration. Ind. Crops Products 140, 111597. doi: 10.1016/j.indcrop.2019.111597
Kim, T. Y., Jo, M. H., Hong, J. H. (2010). Protective effect of nitric oxide against oxidative stress under UV-b radiation in maize leaves. J. Environ. Sci. 19, 1323–1334. doi: 10.1016/j.niox.2005.04.006
Kobylińska, A., Borek, S., Posmyk, M. M. (2018). Melatonin redirects carbohydrates metabolism during sugar starvation in plant cells. J. Pineal Res. 64, e12466. doi: 10.1111/jpi.12466
Kyoungwhan, B. (2021). Melatonin metabolism, signaling and possible roles in plants. Plant J. 105, 376–391. doi: 10.1111/tpj.14915
Kyungjin, L., Hwang, J. O., Reiter, R. J., Back, K. (2018). Flavonoids inhibit both rice and sheep serotonin n-acetyltransferases and reduce melatonin levels in plants. J Pineal Res 65, e12512. doi: 10.1111/jpi.12512. Dr Kyoungwhan Back.
Lee, Y. H., Back, K. (2018). Melatonin regulates chloroplast protein quality control via a mitogen-activated protein kinase signaling pathway. Antioxidants 10, 511. doi: 10.3390/antiox10040511
Lee, K., Back, K. (2019). Melatonin-deficient rice plants show a common semidwarf phenotype either dependent or independent of brassinosteroid biosynthesis. J. Pineal Res 66, e12537. doi: 10.1111/jpi.12537
Lee, H. Y., Back, K. (2022). 2-hydroxymelatonin promotes seed germination by increasing reactive oxygen species production and gibberellin synthesis in arabidopsis thaliana. Antioxidants 11, 737. doi: 10.3390/antiox11040737
Lei, W., Yang, M. Y., Dong, Y. Y., Reiter, R. J., Xu, Y. Q., Lin, X. Y., et al. (2022). Melatonin confers enhanced polyamine metabolism and cell tolerance in vitis vinifera against oxidative damage: Quantitative proteomic evidence. Postharvest Biol. Technol. 184, 111756. doi: 10.1016/j.postharvbio.2021.111756
Lei, X. Y., Zhu, R. Y., Zhang, G. Y., Dai, Y. R. (2004). Attenuation of cold-induced apoptosis by exogenous melatonin in carrot suspension cells: The possible involvement of polyamines. J. Pineal Res. 36, 126–131. doi: 10.1046/j.1600-079x.2003.00106.x
Lerner, A., Case, J., Takahashi, Y., Lee, T., Mori, W.. (1958). Isolation of melatonin, a pineal factor that lightens melanocytes. J. Am. Chem. Soc. 80, 2587. doi: 10.1021/ja01543a060
Liang, D., Shen, Y., Ni, Z., Wang, Q., Lei, Z., Xu, N., et al. (2018). Exogenous melatonin application delays senescence of kiwifruit leaves by regulating the antioxidant capacity and biosynthesis of flavonoids. Front. Plant Science 9. doi: 10.3389/fpls.2018.00426
Li, Z., Cheng, B., Yong, B., Liu, T., Peng, Y., Zhang, X., et al. (2019). Metabolomics and physiological analyses reveal βsitosterol as an important plant growth regulator inducing tolerance to water stress in white clover. Planta 250, 2033–2046. doi: 10.1007/s00425-019-03277-1
Li, H., Dong, Y. C., Chang, J. J., He, J., Chen, H., Liu, Q., et al. (2016). High-throughput MicroRNA and MRNA sequencing reveals that MicroRNAs may be involved in melatonin-mediated cold tolerance in citrullus lanatus l. Front. Plant Sci 8, 57. doi: 10.3389/fpls.2016.01231
Li, H., Guo, Y. L., Lan, Z. X., Xu, K., Chang, J. J., Ahammed, G. J., et al. (2021). Methyl jasmonate mediates melatonin-induced cold tolerance of grafted watermelon plants. Horticulture Res 7, 1231. doi: 10.1038/s41438-021-00496-0
Li, C. T., Suo, J. W., Xuan, L. L., Ding, M. Z., Zhang, H., Song, L. L., et al. (2019). Bamboo shoot-lignification delay by melatonin during low temperature storage. Postharvest Biol. Technology 156, 110933. doi: 10.1016/j.postharvbio.2019.110933
Liu, J., Yue, R., Si, M., Wu, M., Cong, L., Zhai, R., et al. (2019). Effects of exogenous application of melatonin on quality and sugar metabolism in ‘Zaosu’ pear fruit. J. Plant Growth Regul. 38, 1161–1169. doi: 10.1007/s00344-019-09921-0
Mao, J. P., Niu, C. D., Li, K., Tahir, M. M., Han, M. Y., Zhang, D. (2020). Melatonin activates adventitious root formation by promoting the function of MdWOX11 in apple. BMC Plant Biol. 20, 536. doi: 10.21203/rs.3.rs-29239/v1
Millet-Boureima, C., Ennis, C. C., Jamison, J., McSweeney, S., Park, A., Gamberi, C. (2021). Empowering melatonin therapeutics with drosophila models. Diseases 9, 67. doi: 10.3390/diseases9040067
Miranda, S., Vilches, P., Suazo, M., Pavez, L., García, K., Méndez, M. A., et al. (2020). Melatonin triggers metabolic and gene expression changes leading to improved quality traits of two sweet cherry cultivars during cold storage. Food Chem. 319, 126360. doi: 10.1016/j.foodchem.2020.126360
Mohamed, M. F., Almoneafy, A., Mahmoud, A., Elkelish, A., Arnao, M. B., Li, L., et al. (2019). Melatonin and its protective role against biotic stress impacts on plants. Biomolecules 10, 54. doi: 10.3390/biom10010054
Murch, S. J., Alan, A. R., Cao, J., Saxena, P. K. (2009). Melatonin and serotonin in flowers and fruits of datura metel l. J. Pineal Res. 47, 277–283. doi: 10.1111/j.1600-079X.2009.00711.x
Naghizadeh, M., Kabiri, R., Hatami, A., Oloumi, H., Nasibi, F., Tahmasei, Z, et al. (2019). Exogenous application of melatonin mitigates the adverse effects of drought stress on morpho-physiological traits and secondary metabolites in Moldavian balm (Dracocephalum moldavica). Physiol. Mol. Biol. Plants 25, 881–894. doi: 10.1007/s12298-019-00674-4
Nguyen, G., Hailstones, D., Wilkes, M., Sutton, B. (2010). Drought stress: Role of carbohydrate metabolism in drought-induced male sterility in rice anthers. J. Agron. Crop Science 196, 346–357. doi: 10.1111/j.1439-037X.2010.00423.x
Pardo-Hernández, M., López-Delacalle, M., Rivero, M. R. (2020). ROS and NO regulation by melatonin under abiotic stress in plants. Antioxidants 9, 1078. doi: 10.3390/antiox9111078
Park, S. K., Back, K. (2012). Melatonin promotes seminal root elongation and root growth in transgenic rice after germination: Melatonin promotes seminal root growth. J. Pineal Res. 53, 385–389. doi: 10.1111/j.1600-079X.2012.01008.x
Ptak, A., Simlat, M., Moranska, E., Skrzypek, E., Warchol, M., Tarakemeh, A., et al. (2019). Exogenous melatonin stimulated amaryllidaceae alkaloid biosynthesis in in vitro cultures of leucojum aestivum l. Ind. Crops Products 138, 111458. doi: 10.1016/j.indcrop.2019.06.021
Qi, Z. Y., Wang, K. X., Yan, M. Y., Kanwar, M., Li, D. Y., Wijaya, L., et al. (2018). Melatonin alleviates high temperature-induced pollen abortion in solanum lycopersicum. Molecules 23, 386. doi: 10.3390/molecules23020386
Rahul, K. T., Lal, K. M., Kumar, R., Mangal, V., Altaf, M. A., Sharma, S., et al. (2022). Insight into melatonin−mediated response and signaling in the regulation of plant defense under biotic stress. Plant Mol. Biol. 109, 385–399. doi: 10.1007/s11103-021-01202-3
Ramadan, A. M., Azeiz, A. A., Baabad, S., Hassanein, S., Gadalla, N. O., Hassan, S., et al. (2022). Control of β- sitosterol biosynthesis under light and watering in desert plant Calotropis procera. Steroids 141, 1–8. doi: 10.1016/j.steroids.2018.11.003
Rastegar, S., Khankahdani, H., Rahimzadeh, M. (2020). Effects of melatonin treatment on the biochemical changes and antioxidant enzyme activity of mango fruit during storage. Sci. Hortic. 259, 108835. doi: 10.1016/j.scienta.2019.108835
Renu, B., Aghdam, M. S., Arnao, M. B., Brecht, J. K., Fawole, O. A., Pareek, S. (2022). Melatonin alleviates chilling injury symptom development in mango fruit by maintaining intracellular energy and cell wall and membrane stability. Front. Nutr. 9. doi: 10.3389/fnut.2022.936932
Saad, F., Salem, M. (2019). Exogenous melatonin-mediated modulation of arsenic tolerance with improved accretion of secondary metabolite production, activating antioxidant capacity and improved chloroplast ultrastructure in rosemary herb. Ecotoxicology Environ. Safety 180, 333–347. doi: 10.1016/j.ecoenv.2019.05.021
Selmar, D. (2010). Biosynthesis of cyanogenic glycosides, glucosinolates and non-protein amino acids. Ann. Plant Rev., 92–181. doi: 10.1002/9781444320503.ch3
Sezer, I., Kiremit, M. S., Öztürk, E., Subrata, B. A. G., Osman, H. M., Akay, H., et al. (2021). Role of melatonin in improving leaf mineral content and growth of sweet corn seedlings under different soil salinity levels. Scientia Horticulturae 288, 110376. doi: 10.1016/j.scienta.2021.110376
Shah, A. A., Ahmed, S., Ali, A., Yasin, N. A. (2020). 2-hydroxymelatonin mitigates cadmium stress in cucumis sativus seedlings: Modulation of antioxidant enzymes and polyamines. Chemosphere 243, 125308. doi: 10.1016/j.chemosphere.2019.125308
Sharma, A., Wang, J., Xu, D., Tao, S., Chong, S., Yan, D., et al. (2020). Melatonin regulates the functional components of photosynthesis, antioxidant system, gene expression, and metabolic pathways to induce drought resistance in grafted carya cathayensis plants. Sci. Total Environ 713, 136675. doi: 10.1016/j.scitotenv.2020.136675
Shi, H., Tan, D. X., Reiter, R. J., Ye, T., Yang, F., Chan, Z. (2015a). Melatonin induces class A1 heat-shock factors (HSFA1s) and their possible involvement of thermotolerance in arabidopsis. J. Pineal Res. 58, 335–342. doi: 10.1111/jpi.12219
Shi, H., Wang, X., Tan, D. X., Reiter, R. J., Chan, Z.. (2015b). Comparative physiological and proteomic analyses reveal the actions of melatonin in the reduction of oxidative stress in Bermuda grass (Cynodon dactylon (L). pers.). J. Pineal Res. 59, 120–131. doi: 10.1111/jpi.12246
Siddiqui, M. H., Khan, M. N., Mukherjee, S., Basahi, R. A., Alamri, S., Alsubaie, Q. D., et al. (2021). Exogenous melatonin-mediated regulation of K + /Na + transport, h + -ATPase activity and enzymatic antioxidative defence operate through endogenous hydrogen sulphide signalling in NaCl-stressed tomato seedling roots. Plant Biol. 23, 797–805. doi: 10.1111/plb.13296
Silin, F., Xiong, T. T., Lei, Q. M., Tan, Q. Q., Cai, J. H., Song, Z. Y., et al. (2022). Melatonin treatment improves postharvest preservation and resistance of guava fruit (Psidium guajava l.). Foods 11, 262. doi: 10.3390/foods11030262
Simlat, M., Ptak, A., Skrzypek, E., Warchoł, M., Morań ska, E., Piórkowska, E., et al. (2018). Melatonin significantly influences seed germination and seedling growth of stevia rebaudiana bertoni. Peer J 6, e5009. doi: 10.7717/peerj.5009
Socaciu, A. I., Ionut, R., Socaciu, M. A., Ungur, A. P., Bârsan, M., Chiorean, A., et al. (2020). Melatonin, an ubiquitous metabolic regulator: functions, mechanisms and effects on circadian disruption and degenerative diseases. Rev. Endocrine Metab. Disord. 21, 465–478. doi: 10.1007/s11154-020-09570-9
Song, Z. H., Yang, Q., Dong, B. Y., Li, N., Wang, M. Y., Du, T. T., et al. (2022). Melatonin enhances stress tolerance in pigeon pea by promoting flavonoid enrichment, particularly luteolin in response to salt stress. J. Exp. Botany 73, 5992–6008. doi: 10.1093/jxb/erac276
Sun, Q., Liu, L., Zhang, L., Lv, H., He, Q., Guo, L., et al. (2020). Melatonin promotes carotenoid biosynthesis in an ethylene-dependent manner in tomato fruits. Plant Science 298, 110580. doi: 10.1016/j.plantsci.2020.110580
Sun, H. J., Luo, M. L., Zhou, X., Zhou, Q., Sun, Y. Y., Ge, W. Y., et al. (2020). PuMYB21/PuMYB54 coordinate to activate PuPLDβ1 transcription during peel browning of cold-stored ‘Nanguo’ pears. Hortic. Res. 7, 136. doi: 10.1038/s41438-020-00356-3
Sun, C. L., Sun, N., Ou, Y. Q., Gong, B., Jin, C. W., Shi, Q. H., et al. (2022). Phytomelatonin and plant mineral nutrition. J. Exp. Botany 73, 5903–5917. doi: 10.1093/jxb/erac289
Sun, Q., Zhang, N., Wang, J., Cao, Y., Li, X., Zhang, H., et al. (2016). A label-free differential proteomics analysis reveals the effect of melatonin on promoting fruit ripening and anthocyanin accumulation upon postharvest in tomato. J. Pineal Res. 61, 138–153. doi: 10.1111/jpi.12315
Su, X. Y., Xin, L. F., Li, Z., Zheng, H. F., Mao, J., Yang, Q. H. (2018). Physiology and transcriptome analyses reveal a protective effect of the radical scavenger melatonin in aging maize seeds. Free Radical Res. 52, 1094–1109. doi: 10.1080/10715762.2018.1472378
Suzuki, T., Ishikawa, K., Yamashino, T., Mizuno, T. (2002). An arabidopsis histidine-containing phosphotransfer (HPt) factor implicated in phosphorelay signal transduction: Overexpression of AHP2 in plants results in hypersensitiveness to cytokinin. Plant Cell Physiol. 43, 123–129. doi: 10.1093/pcp/pcf007
Talaat, N. B. (2021). Polyamine and nitrogen metabolism regulation by melatonin and salicylic acid combined treatment as a repressor for salt toxicity in wheat (Triticum aestivum l.) plants. Plant Growth Regulation 95, 315–329. doi: 10.1007/s10725-021-00740-6
Teng, Z. Y., Zheng, W. W., Jiang, S. F., Hong, S. B., Zhu, Z. J., Zang, Y. X. (2022). Role of melatonin in promoting plant growth by regulating carbon assimilation and ATP accumulation. Plant Science 319, 111276. doi: 10.1016/j.plantsci.2022.111276
Verde, A., Míguez, M. J., Gallardo, M. (2022). Role of melatonin in apple fruit during growth and ripening: Possible interaction with ethylene. Plants 11, 688. doi: 10.3390/plants11050688
Wang, K. X., Cai, S. Y., Xing, Q. F., Qi, Z. Y., Fotopoulos, V., Yu, J. Q., et al. (2022). Melatonin delays dark-induced leaf senescence by inducing MiR171b expression in tomato. J. Pineal Res 72, e12792. doi: 10.1111/jpi.12792
Wang, K. X., Xing, Q. F., Ahammed, J. G., Zhou, J.. (2022a). Functions and prospects of melatonin in plant growth, yield, and quality. J. Exp. Botany 73, 5928–5946. doi: 10.1093/jxb/erac233
Wang, K. X., Cai, S. Y., Xing, Q. F., Qi, Z. Y., Fotopoulos, V., Yu, J. Q., et al. (2022b). Melatonin delays dark-induced leaf senescence by inducing MiR171b expression intomato. J. Pineal Res. doi: 10.1111/jpi.12792
Wang, D., Chen, Q., Chen, W., Guo, Q., Xia, Y., Wu, D., et al. (2021). Melatonin treatment maintains quality and delays lignification in loquat fruit during cold storage. Scientia Horticulturae 284, 110126. doi: 10.1016/j.scienta.2021.110126
Wang, R., Cheng, M. L., Liu, H. N., Zhao, D. Q., Tao, J. (2018). Effects of melatonin on flavonoids content and related gene expression levels of gardenia under dark conditions. Bull. Botanical Researc 38, 559–567. doi: 10.7525/j.issn.1673-5102.2018.04.010
Wang, R., Qian, J., Fang, Z., Tang, J. (2020). Transcriptomic and physiological analyses of rice seedlings under different nitrogen supplies provide insight into the regulation involved in axillary bud outgrowth. BMC Plant Biol. 20, 197. doi: 10.1186/s12870-020-02409-0
Wang, Y. X., Teng, R. M., Wang, W. L., Wang, Y., Shen, W., Zhuang, J. (2019). Identification of genes revealed differential expression profiles and lignin accumulation during leaf and stem development in tea plant [Camellia sinensis (L.) o. kuntze]. Protoplasma 256, 359–370. doi: 10.1007/s00709-018-1299-9
Wei, W., Li, Q. T., Chu, Y. N., Reiter, R. J., XM, Yu, DH, Z., et al. (2015). Melatonin enhances plant growth and abiotic stress tolerance in soybean plants. J. Exp. Botany 66, 695–707. doi: 10.1093/jxb/eru392
Wei, Y. X., Liu, G. Y., Chang, Y. L., Lin, D. A., Reiter, R. J., He, C., et al. (2018). Melatonin biosynthesis enzymes recruit WRKY transcription factors to regulate melatonin accumulation and transcriptional activity on W-box in cassava. J. Pineal Res. 65, e12487. doi: 10.1111/jpi.12487
Wu, C. H., Cao, S. F., Xie, K. Q., Chi, Z., Wang, J., Wang, H., et al. (2021). Melatonin delays yellowing of broccoli during storage by regulating chlorophyll catabolism and maintaining chloroplast ultrastructure. Postharvest Biol. Technology 172, 111378. doi: 10.1016/j.postharvbio.2020.111378
Xiang, D. Y., Nguyen, C. D., Felter, L., Clark, D., Huo, H. Q. (2020). The effects of preharvest LED light, melatonin and AVG treatments on the quality of postharvest snapdragon and vase life. J. Floriculture Landscaping 6, 14–19. doi: 10.25081/jfcls.2020.v6.6236
Xie, Q. L., Zhang, Y., Cheng, Y. X., Tian, T. L., Luo, J. J., Hu, Z. L., et al. (2022). The role of melatonin in tomato stress response, growth and development. Plant Cell Rep. 41, 1631–1650. doi: 10.1007/s00299-022-02876-9
Xing, X. J., Ding, Y. R., Jin, J. Y., Song, A. P., Chen, S., Chen, F., et al. (2021). Physiological and transcripts analyses reveal the mechanism by which melatonin alleviates heat stress in chrysanthemum seedlings. Front. Plant Sci. 12. doi: 10.3389/fpls.2021.673236
Xin, L., Li, M. H., Deng, W. W., Ahammed, G. J., Wei, J. P., Yan, P., et al. (2020). Exogenous melatonin improves tea quality under moderate high temperatures by increasing epigallocatechin-3-gallate and theanine biosynthesis in camellia sinensis l. J. Plant Physiol. 253, 153273. doi: 10.1016/j.jplph.2020.153273
Xu, W., Cai, S. Y., Zhang, Y., Wang, Y., Ahammed, G. J., Xia, X. J., et al. (2016). Melatonin enhances thermotolerance by promoting cellular protein protection in tomato plants. J. Pineal Res. 61, 457–469. doi: 10.1111/jpi.12359
Xu, L., Yue, Q., Bian, F., Zhai, H., Yao, Y. (2018). Melatonin treatment enhances the polyphenol content and antioxidant capacity of red wine. Hortic. Plant J. 4, 144–150. doi: 10.1016/j.hpj.2018.05.004
Yang, X. X., Chen, J., Ma, Y., Huang, M. H., Qiu, T., Bian, H. W., et al. (2022). Function, mechanism, and application of plant melatonin: An update with a focus on the cereal crop, barley (Hordeum vulgare l.). Antioxidants 11, 634. doi: 10.3390/antiox11040634
Yang, S. J., Huang, B., Zhao, Y. Q., Hu, D., Chen, T., Ding, C. B., et al. (2021). Melatonin enhanced the tolerance of arabidopsis thaliana to high light through improving anti-oxidative system and photosynthesis. Front. Plant Science 12. doi: 10.3389/fpls.2021.752584
Yang, Q., Li, J., Ma, W., Zhang, S., Hou, S., Wang, Z., et al. (2021). Melatonin increases leaf disease resistance and saponin biosynthesis in panax notogiseng. J. Plant Physiol. 263, 153466. doi: 10.1016/j.jplph.2021.153466
Yang, M. Y., Wang, L., Belwal, T., Zhang, X. C., Lu, H. G., Chen, C. K., et al. (2019). Exogenous melatonin and abscisic acid expedite the flavonoids biosynthesis in grape berry of vitis vinifera cv. kyoho. Molecules 25, 12. doi: 10.3390/molecules25010012
Yang, S., Zhao, Y., Qin, X., Ding, C., Chen, Y., Tang, Z., et al. (2022). New insights into the role of melatonin in photosynthesis. J. Exp. Bot 73, 5918–5927. doi: 10.1093/jxb/erac230
Yan, R., Li, S., Cheng, Y., Kebbeh, M., Huan, C., Zheng, X. L. (2022). Melatonin treatment maintains the quality of cherry tomato by regulating endogenous melatonin and ascorbate-glutathione cycle during room temperature. J. Food Biochem 46, e14285. doi: 10.1111/jfbc.14285
Yin, X. M., Bai, Y. L., Gong, C. Y., Song, W. L., Yan Wu, Y., Ye, T. T., et al. (2022). The phytomelatonin receptor PMTR1 regulates seed development and germination by modulating abscisic acid homeostasis in arabidopsis thaliana. J. Pineal Res. 72, e12797. doi: 10.1111/jpi.12797
Yu, J. C., Lu, J. Z., Cui, X. Y., Guo, L., Wang, Z. J., Liu, Y. D., et al. (2022). Melatonin mediates reactive oxygen species homeostasis via SlCV to regulate leaf senescence in tomato plants. J. Pineal Res 73, 12810. doi: 10.1111/jpi.12810
Ze, Y., Gao, H., Li, T., Yang, B., Jiang, Y. (2021). Insights into the roles of melatonin in maintaining quality and extending shelf life of postharvest fruits. Trends Food Sci. Technol. 109, 569–578. doi: 10.1016/j.tifs.2021.01.051
Zeng, W., Mostafa, S., Lu, Z. G., Jin, B. (2022). Melatonin-mediated abiotic stress tolerance in plants. Front. Plant Science 13. doi: 10.3389/fpls.2022.847175
Zhang, P. F., Liu, L., Wang, X., Wang, Z. Y., Zhang, H., Chen, J. T., et al. (2021). Beneficial effects of exogenous melatonin on overcoming salt stress in sugar beets (Beta vulgaris l.). Plants 10, 886. doi: 10.3390/plants10050886
Zhang, Q., Liu, X. F., Zhang, Z. F., Liu, N. F., Li, D. Z., Hu, L. X. (2019). Melatonin improved waterlogging tolerance in alfalfa (Medicago sativa) by reprogramming polyamine and ethylene metabolism. Front. Plant Science 10. doi: 10.3389/fpls.2019.00044
Zhang, T. G., Wang, J., Sun, Y. P., Zhang, L., Zheng, S. (2022). Versatile roles of melatonin in growth and stress tolerance in plants. J. Plant Growth Regulation 41, 507–523. doi: 10.1007/s00344-021-10317-2
Zhang, N., Zhang, H., Sun, Q., Cao, Y., Li, X., Zhao, B., et al. (2017). Proteomic analysis reveals a role of melatonin in promoting cucumber seed germination under high salinity by regulating energy production. Sci. Rep. 7, 503. doi: 10.1038/s41598-017-00566-1
Zhao, D. Q., Luan, Y. T., Shi, W. B., Tang, Y. H., Huang, X. Q., Tao, J. (2022). Melatonin enhances stem strength by increasing lignin content and secondary cell wall thickness in herbaceous peony. J. Exp. Bot 73, 5974–5991. doi: 10.1093/jxb/erac165
Zhao, C. F., Yang, M., Wu, X., Wang, Y. F., Zhang, R. H. (2021). Physiological and transcriptomic analyses of the effects of exogenous melatonin on drought tolerance in maize (Zea mays l.). Plant Physiol. Biochem. 168, 128–142. doi: 10.1016/j.plaphy.2021.09.044
Zhao, H. L., Zhang, K., Zhou, X. T., Xi, L. J., Wang, Y. P., Xu, H. J., et al. (2017). Melatonin alleviates chilling stress in cucumber seedlings by up-regulation of CsZat12 and modulation of polyamine and abscisic acid metabolism. Sci. Rep. 7, 4998. doi: 10.1038/s41598-017-05267-3
Zhao, G., Zhao, Y., Yu, X., Kiprotich, F., Han, H., Guan, R., et al. (2018). Nitric oxide is required for melatonin-enhanced tolerance against salinity stress in rapeseed (Brassica napus l.) seedlings. Int. J. Mol. Sci. 19, 1912. doi: 10.3390/ijms19071912
Zhong, L. S., Lin, L. J., Yang, L., Liao, M. A., Wang, X., Wang, J., et al. (2020). Exogenous melatonin promotes growth and sucrose metabolism of grape seedlings. PloS One 15, e0232033. doi: 10.1371/journal.pone.0232033
Zhou, R., Cai, Y., Lin, T., Chai, M.. (2022). Effects of melatonin and epibrassinolide on the regeneration of long-term subcultured callus of zoysia matrella (L.) merr. under simulated drought stress. J. Zhejiang Univ. (Agriculture Life Sciences) 48, 36–44. doi: 10.3785/j.issn.1008-9209.2021.06.011
Zhou, C., Liu, Z., Zhu, L., Ma, Z. Y., Wang, J. F., Zhu, J. (2016). Exogenous melatonin improves plant iron deficiency tolerance via increased accumulation of polyamine-mediated nitric oxide. Int. J. Mol. Sci. 17, 1777. doi: 10.3390/ijms17111777
Zhou, X., Zhao, H., Cao, K., Hu, L., Du, T., Baluska, F., et al. (2016). Beneficial roles of melatonin on redox regulation of photosynthetic electron transport and synthesis of D1 protein in tomato seedlings under salt stress. Front. Plant Sci. 7. doi: 10.3389/fpls.2016.01823
Keywords: melatonin, abiotic stress, growth and development, stress tolerance, secondary metabolites
Citation: Pan Y, Xu X, Li L, Sun Q, Wang Q, Huang H, Tong Z and Zhang J (2023) Melatonin-mediated development and abiotic stress tolerance in plants. Front. Plant Sci. 14:1100827. doi: 10.3389/fpls.2023.1100827
Received: 17 November 2022; Accepted: 02 January 2023;
Published: 26 January 2023.
Edited by:
Qing Yang, Beijing Forestry University, ChinaReviewed by:
Daofeng Liu, Southwest University, ChinaHongxia Xu, Zhejiang Academy of Agricultural Sciences, China
Copyright © 2023 Pan, Xu, Li, Sun, Wang, Huang, Tong and Zhang. This is an open-access article distributed under the terms of the Creative Commons Attribution License (CC BY). The use, distribution or reproduction in other forums is permitted, provided the original author(s) and the copyright owner(s) are credited and that the original publication in this journal is cited, in accordance with accepted academic practice. No use, distribution or reproduction is permitted which does not comply with these terms.
*Correspondence: Zaikang Tong, emt0b25nQHphZnUuZWR1LmNu; Junhong Zhang, emhhbmdqdW5ob25nQHphZnUuZWR1LmNu
†These authors have contributed equally to this work