- 1College of Life Sciences and Technologies, Tarim University, Alar, China
- 2Laboratory of Systematic & Evolutionary Botany and Biodiversity, College of Life Sciences, Zhejiang University, Hangzhou, China
- 3National Academy of Medical Sciences of Ukraine, Kyiv, Ukraine
- 4Department of Environment & Biodiversity, University of Salzburg, Salzburg, Austria
- 5College of Life Science, Tonghua Normal University, Tonghua, China
- 6Shanghai Chenshan Botanical Garden, Shanghai, China
- 7Department of Environment and Forest Resources, Chungnam National University, Daejeon, South Korea
- 8Key Laboratory of Biosystem Homeostasis and Protection, Ministry of Education, Zhejiang University, Hangzhou, China
Subfamily Nandinoideae Heintze (Berberidaceae), comprising four genera and ca. 19 species, is disjunctively distributed in eastern North America vs. Eurasia (eastern Asia, Central Asia, Middle East, and southeastern Europe), and represents an ideal taxon to explore plastid phylogenomics and plastome evolution in Berberidaceae. Many species of this subfamily have been listed as national or international rare and endangered plants. In this study, we sequenced and assembled 20 complete plastomes, representing three genera and 13 species of Nandinoideae. Together with six plastomes from GenBank, a total of 26 plastomes, representing all four genera and 16 species of Nandinoideae, were used for comparative genomic and phylogenomic analyses. These plastomes showed significant differences in overall size (156,626–161,406 bp), which is mainly due to the expansion in inverted repeat (IR) regions and/or insertion/deletion (indel) events in intergenic spacer (IGS) regions. A 75-bp deletion in the ndhF gene occurred in Leontice and Gymnospermium when compared with Nandina and Caulophyllum. We found a severe truncation at the 5’ end of ycf1 in three G. altaicum plastomes, and a premature termination of ropC1 in G. microrrhynchum. Our phylogenomic results support the topology of {Nandina, [Caulophyllum, (Leontice, Gymnospermium)]}. Within the core genus Gymnospermium, we identified G. microrrhynchum from northeastern Asia (Clade A) as the earliest diverging species, followed by G. kiangnanense from eastern China (Clade B), while the rest species clustered into the two sister clades (C and D). Clade C included three species from West Tianshan (G. albertii, G. darwasicum, G. vitellinum). Clade D consisted of G. altaicum from northern Central Asia, plus one species from the Caucasus Mountains (G. smirnovii) and three from southeastern Europe (G. odessanum, G. peloponnesiacum, G. scipetarum). Overall, we identified 21 highly variable plastome regions, including two coding genes (rpl22, ycf1) and 19 intergenic spacer (IGS) regions, all with nucleotide diversity (Pi) values > 0.02. These molecular markers should serve as powerful tools (including DNA barcodes) for future phylogenetic, phylogeographic and conservation genetic studies.
Introduction
Berberidaceae Juss. (Ranunculales), with typical temperate shrubs/perennial herbs and disjunct distributions in the Northern Hemisphere, has attracted the interest of botanists for more than a century (Hutchinson, 1920; Stearn, 1938; Fukuda, 1967; Ying, 1979; Ying et al., 1984; Loconte and Blackwell, 1985; Xiang et al., 2000; Wang et al., 2007; Zhang et al., 2007; Yu and Chung, 2017; Sun et al., 2018; Chen et al., 2020). Within Berberidaceae, three subfamilies, namely Berberidoideae Eaton, Podophylloideae Eaton, and Nandinoideae Heintze, have recently been supported by phylogenomic analyses of whole-plastome sequence data (Sun et al., 2018; Hsieh et al., 2022), hence corroborating earlier phylogenetic inferences based on ‘traditional’ nuclear ribosomal (ITS) and/or plastid DNA markers (rbcL: Kim and Jansen, 1996; ndhF: Kim et al., 2004; ITS2 + matK, rbcL: Wang et al., 2007; ITS + accD, ndhF, rbcL: Yu and Chung, 2017).
Here, we focus on subfamily Nandinoideae, which consists of four genera, including Nandina Thunb. (1 spp.), Caulophyllum Michx. (3 spp.), Leontice L. (4 spp.) and Gymnospermium Spach (ca. 11 spp.). Together, these ca. 19 species form a disjunct distribution across the northern temperate zones in eastern North America vs. Eurasia, including eastern Asia, Central Asia, Middle East, and southeastern Europe (Loconte and Estes, 1989; Kim et al., 2004; Wang et al., 2007; Ying et al., 2011; Barina et al., 2017; Rosati et al., 2018; Figure 1). More specifically, the only species of Nandina, i.e., N. domestica Thunb., is a shrub that occurs in montane forests of China and Japan (Ying et al., 2011; Govaerts et al., 2021). All species of the other three genera are perennial herbs. All three species of Caulophyllum grow in mesophytic forests, one (C. robustum Maxim.) in eastern China and two [C. thalictroides (L.) Michx., C. giganteum (Farw.) Loconte and W. H. Blackw.] in eastern North America (Loconte and Estes, 1989; Xiang et al., 2000; Ying et al., 2011). Leontice consists of four species, L. leontopetalum Hook. fil. and Thomson, L. armeniaca Bél., L. ewersmannii Bunge and L. incerta Pall., all of which are distributed in semiarid to arid regions of Central Asia, Middle East, and southeastern Europe (Loconte and Estes, 1989). Gymnospermium, is the most species-rich genus, including about 11 species found in deciduous forests or forest margins, two in eastern Asia [G. microrrhynchum (S. Moore) Takht. and G. kiangnanense (P. L. Chiu) Loconte], one in the Altai Mountains of Central Asia [G. altaicum (Pallas) Spach], four in the west Tianshan Mountains of Central Asia [G. albertii (Regel) Takht., G. darwasicum (Regel) Takht., G. vitellinum M. Král, G. silvaticum (Freitag) Takht.] (Barina et al., 2015), one in the Caucasus Mountains [G. smirnovii (Trautv.) Takht.], and the remaining three in southeastern Europe [G. odessanum (DC.) Takht., G. peloponnesiacum (Phitos) Strid, G. scipetarum Papar. and Qosja ex E. Mayer and Pulević] (Loconte and Estes, 1989; Ying et al., 2011; Barina et al., 2015, 2017; Rosati et al., 2018; Abidkulova et al., 2021).
Notably, most species of Nandinoideae are rich in alkaloids with important medicinal values (Peng, 2006). For example, in Chinese and Japanese traditional medicine, the roots, stems, leaves, and fruits of Nandina domestica are primarily used to treat coughing and asthma (Peng et al., 2014), whereby the fruits are additionally used for the treatment of pharynx tumor, colon cancer and tooth abscess (Hess et al., 1977; Ikuta and Itokawa, 1988; Son et al., 2019). The tubers of Leontice leontopetalum and L. ewersmannii are used in Turkish traditional medicine for the treatment of epilepsy (Baytop, 1984; Gresser et al., 1993; Kolak et al., 2011; Al-Snafi, 2019). The rhizomes of Caulophyllum thalictroides and C. giganteum have been used traditionally by Native Americans for inducing childbirth and as anti-inflammatory and anti-pyretic agents (Erichsen-Brown, 1989; Duke, 2002; Rader and Pawar, 2013). Most species of Gymnospermium are likewise rich in alkaloids with medicinal properties, such as G. albertii (Iskandarov et al., 1967), G. darwasicum (Zunnunzhanov et al., 1971), G. kiangnanense (Liao et al., 2001), G. smirnovii (Tabatadze et al., 2010), and so on. Additionally, many species of Gymnospermium have been listed as national or international rare and endangered plants (Chang et al., 2004; Doroftei and Mierla, 2007; Karl and Strid, 2009; Mikatadze-Pantsulaia et al., 2010; Abidkulova et al., 2021).
Plastid phylogenomic analyses have resolved some recalcitrant relationships at various taxonomic levels, for instance among families of early-diverging eudicots (Sun et al., 2016), among genera of Berberidaceae (Sun et al., 2018; Hsieh et al., 2022), and even among species of Epimedium Tourn. ex L. (Podophylloideae; Zhang et al., 2016). Comparative plastome analyses have also provided detailed insights into the factors of plastome gene variation and evolution, including gene indel events, expansions and contractions of the inverted repeat (IR) regions, or structural rearrangements (Sun et al., 2016, 2018; Zhang et al., 2016; Ye et al., 2018; Hsieh et al., 2022). Some special structures of plastomes have previously been identified in Berberidoideae and Podophylloideae. For example, 249-bp and 315-bp insertions were seen in the first exon of the clpP gene in Vancouveria hexandra C. Morren and Decne. and Epimedium, respectively (Sun et al., 2016, 2018). Moreover, the plastome of Bongardia chrysogonum (L.) Spach was found to contain an inversion of 14 genes (ca. 19 kb) in the large single-copy (LSC) region (Sun et al., 2018), while the plastomes of Berberis L. and Mahonia Nutt. revealed a large expansion in the IR regions (Ma et al., 2013; Sun et al., 2018). Finally, there have been recent reports of a severely truncated rps7 gene in the plastomes of several Podophylloideae species, including Diphylleia sinensis H. L. Li, Dysosma versipellis (Hance) M. Cheng ex T. S. Ying and Podophyllum peltatum L. (Sun et al., 2018; Ye et al., 2018).
Within Nandinoideae, only six out of the ca. 19 species have fully sequenced plastomes, i.e., Nandina domestica, Caulophyllum robustum, Leontice armeniaca, L. incerta, Gymnospermium microrrhynchum, and G. kiangnanense (Moore et al., 2006; Sun et al., 2018; Yang et al., 2018; He et al., 2019). In this study, we newly sequenced 20 complete plastomes of Nandinoideae, representing three genera and 13 species of this subfamily (including additional accessions of C. robustum, G. microrrhynchum, and G. kiangnanense). Together with the six plastomes previously reported, we included a total of 26 complete plastomes, representing all four genera and 16 species of Nandinoideae (ca. 84% of the group’s total species diversity). Based on this extensive plastome dataset, our specific aims were to (1) characterize and compare the structure as well as gene content and order among these plastomes to gain further insights into their evolution; (2) infer phylogenetic relationships within Nandinoideae, especially for the core genus Gymnospermium; and (3) identify highly variable plastome regions in Nandinoideae for future phylogenetic, phylogeographic and/or conservation genetic studies.
Materials and Methods
Plant Materials and DNA Extraction
For whole plastome sequencing, we collected fresh leaves of 13 Nandinoideae species, including 10 of Gymnospermium, one of Leontice, and two of Caulophyllum, resulting in 20 individuals overall (1–2 individuals per species, see Supplementary Table 1). Voucher specimens were deposited at the herbarium of Tarim University (TARU), Alar, Xinjiang, China (Table 1). Total genomic DNA was extracted from the silica-gel dried leaf tissues using DNA Plantzol Reagent (Invitrogen, Carlsbad, CA, United States), following the manufacturer’s protocol. The quality and quantity of genomic DNA was determined using both 1% agarose gel electrophoresis and an ultraviolet spectrophotometer (K5800, KAIAO, Beijing, China).
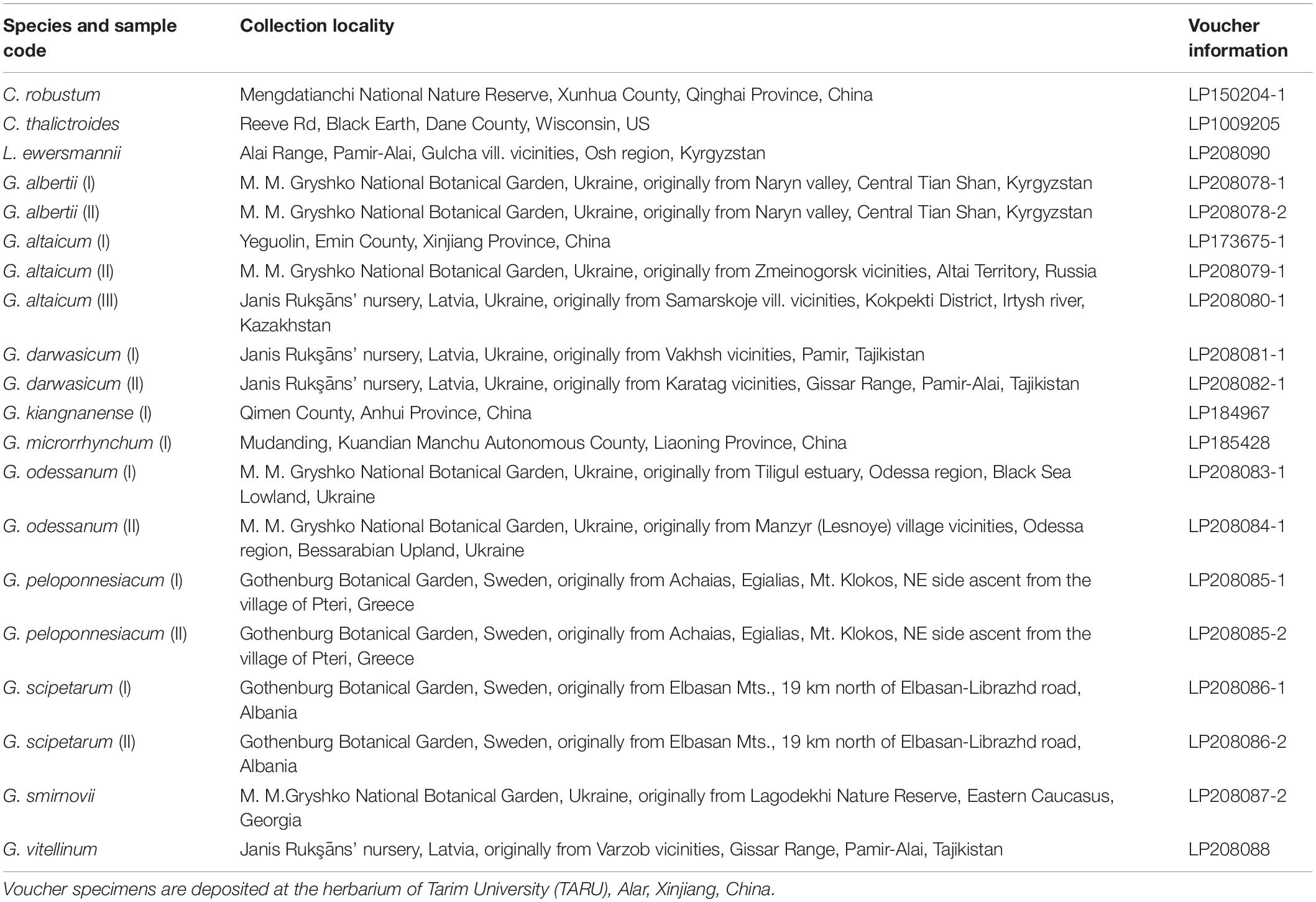
Table 1. Collection locality and voucher information of the Nandinoideae species and accessions used in the present study.
Genome Sequencing, Assembly, and Annotation
Short-insert (500-bp) paired-end libraries were constructed using the Genomic DNA Sample Prep Kit (Illumina, San Diego, CA, United States), following the manufacturer’s instructions. We used tags to index DNA from each species and pooled samples together for sequencing on a HiSeq™ 2500 platform at the Beijing Genomics Institute (BGI, Shenzhen, China). We assembled raw reads into contigs using NOVOPlasty 2.6.3 (Dierckxsens et al., 2017) with the matK gene of G. kiangnanense (MH298010) used as a seed (Yang et al., 2018). The contigs of each Nandinoideae individuals were re-mapped with the reference sequence (MH298010) for whole-plastome assemblage in Geneious Prime® 2021.2.21. For plastome annotation, we used the ‘2544-plastome’ dataset of CPGAVAS2 (Shi et al., 2019) with default parameter settings. All 20 newly sequenced plastomes were illustrated with the online tool OrganellarGenome DRAW v1.3.1 (Greiner et al., 2019) and deposited in GenBank (Table 1). To complement our taxon-sampling, we downloaded six additional plastome sequences of Nandinoideae from NCBI, i.e., N. domestica (DQ923117); C. robustum (MH423066), L. armeniaca (MH423070), L. incerta (MH940295), G. microrrhynchum (KM057373), and G. kiangnanense (MH298010) and reannotated them with above method. Altogether, a total of 16 species and 26 accessions (account for around 84% of Nandinoideae species) were used next comparative plastome analyses.
Comparative Plastome Analyses
Gene differences among all above 26 Nandinoideae plastomes, including those downloaded from GenBank (see “Genome Sequencing, Assembly, and Annotation”), were performed in Shuffle-LAGAN mode on mVISTA (Frazer et al., 2004). Rearrangements of plastomes were checked by Mauve 2.3.0 (Darling et al., 2004). The structure and junctions between the SSC/LSC regions and the IR regions (see Results) were investigated using IRscope (Amiryousefi et al., 2018). Protein-coding (CDS), intergenic spacer (IGS), and intron regions with alignment length > 200 bp and containing at least one mutation were extracted sequentially. Nucleotide diversity (Pi) values for each of those regions were calculated in DNASP v6 (Rozas et al., 2017).
Phylogenetic Analyses
All 80 protein-coding (CDS) regions of the 26 Nandinoideae plastomes and two outgroups [Berberis amurensis Rupr. (KM057374) and B. weiningensis T. S. Ying (MW018363)] were extracted and aligned using PhyloSuite v1.2.2 (Zhang et al., 2018) and MAFFT v7 (Katoh and Standley, 2013), respectively. These 80 CDS-genes were then concatenated by PhyloSuite with a set of default parameters. Based on the 80 CDSs, phylogenetic trees of the 28 plastomes (including outgroups) were constructed using maximum likelihood (ML) and Bayesian inference (BI) methods in RAxML-HPC2 on XSEDE v8.2.12 (Stamatakis, 2014) and MrBayes on XSEDE v3.2.7 (Ronquist et al., 2012), respectively. Both analyses are implemented on the CIPRES Science Gateway website2. The best-fitting nucleotide substitution model (GTR + I + G) is based on the Akaike Information Criterion (AIC) in jModelTest v2.1.6 (Darriba et al., 2012). For the ML analysis, we set 1,000 bootstrap replicates and defaulted the other parameters. For the BI analysis, we run two independent Markov chain MonteCarlo (MCMC) chains, each for 1,000,000 generations, and sampling every 1,000 generations; the first 25% of the trees were discarded.
Results
Plastome Features
All 26 plastomes of Nandinoideae, representing 16 species and four genera, exhibited the typical angiosperm quadripartite structure, including a pair of IR regions (IRa, IRb), separated by SSC and LSC regions (Figure 2). All the 26 plastomes contained 114 unique genes (20 in the IR regions), including 80 protein-coding (CDS) genes, 30 tRNA genes, and four rRNA genes (Supplementary Table 1). Of those 114 genes, 17 contained one intron, while three CDS genes (clpP, rps12, ycf3) possessed two introns. Notably, we found two separate rps12 gene sequences, one located in the LSC and the other in IRa. For 20 newly sequenced plastomes, the lengths varied significantly, ranging from 156,626 bp in Leontice ewersmannii to 161,406 bp in Gymnospermium smirnovii (I) (Table 2). The average coverages of the 20 plastomes ranged from 179 × (Caulophyllum thalictroides) to 17,448 × [(G. scipetarum (I)] (Table 2). The LSC ranged from 85,149 bp in C. thalictroides to 87,702 bp in G. microrrhynchum (KM057373), the SSC from 14,981 bp in G. smirnovii (I) to 20,042 bp in G. microrrhynchum (I), and the IRs from 26,292 bp in Caulophyllum thalictroides to 30,169 bp in G. smirnovii (I) (Table 2).
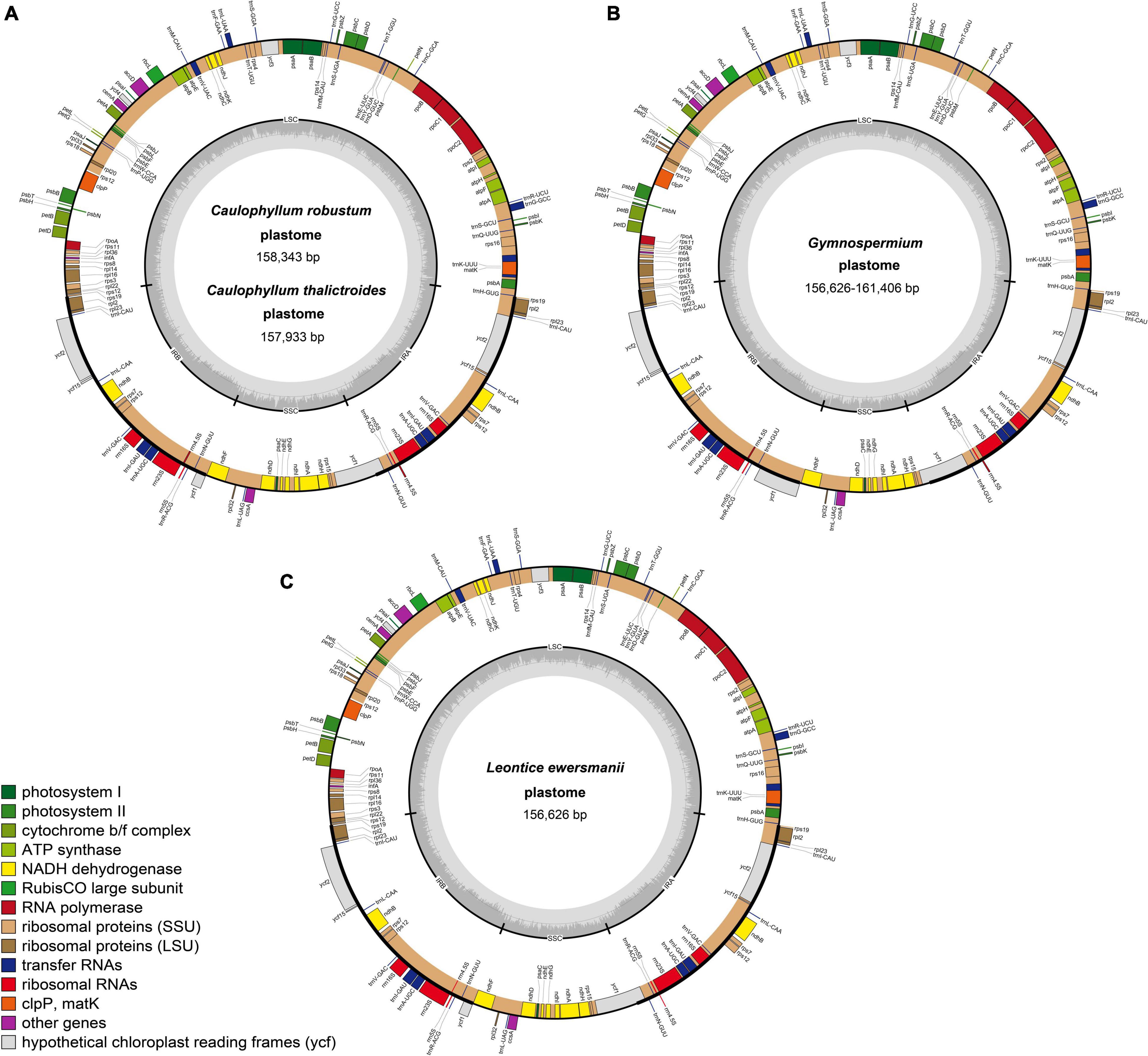
Figure 2. Representative plastome maps of the newly sequenced plastomes of Nandinoideae (20 in total): (A) Caulophyllum robustum and C. thalictroides, (B) Gymnospermium, (C) Leontice ewersmannii.
All 26 plastomes of Nandinoideae had their LSC/IRb junctions located within the rps19 gene, and thus contained a ψrps19 (62–173 bp) in IRa (Figure 3). In almost all plastomes, the SSC/IRa boundary was located within the ycf1 gene, except for G. altaicum (II), in which the SSC/IRa boundary was situated in the spacer region ycf1-trnN-GUU (Figure 3). Therefore, there was no ψycf1 in G. altaicum (II), while for the remaining 25 plastomes, there was a ψycf1 with different length. The shortest ψycf1 (98 bp) was found in two (of the three) G. altaicum accessions (I and III), and the longest (4,786 bp) in G. smirnovii; in the other 23 plastomes, the length of this pseudogene varied from 868 to 1,184 bp (Figure 3). Hence, within Nandinoideae, the plastome of G. smirnovii (161,406 bp) was significantly longer than other plastomes, and this is mainly due to the increased length of ψycf1, which resulted in a significant expansion of the respective IR regions.
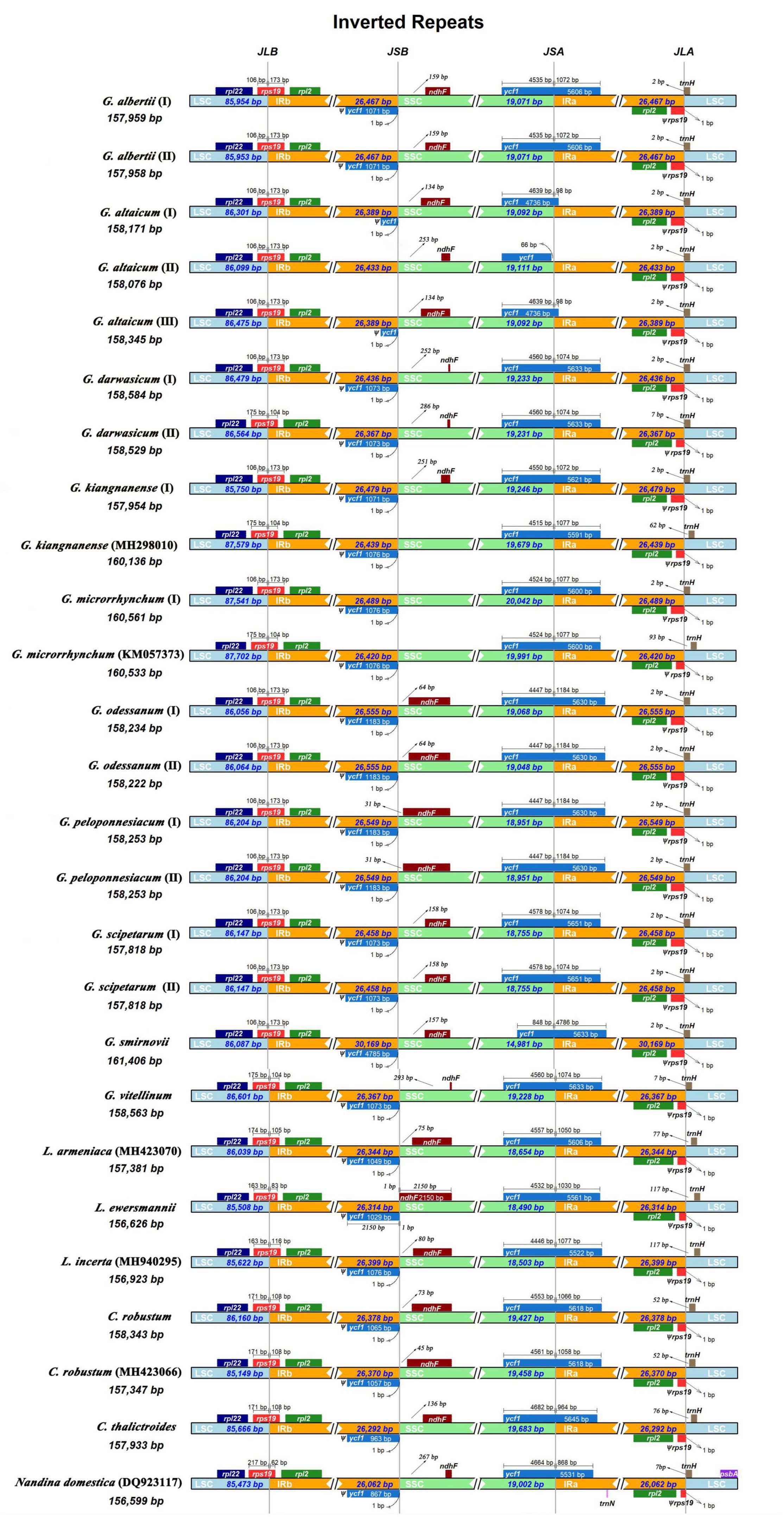
Figure 3. Comparison of the LSC/IRb/SSC/IRa junctions among the 26 complete plastomes of Nandinoideae.
Of all the 114 unique genes identified across the 26 Nandinoideae plastomes, 26 differed in length, mostly less than 10 bp, albeit with some exceptions (see Supplementary Tables 1, 2). Specifically, in all three accessions of G. altaicum (I–III), the 5′ end of the ycf1 gene was ca. 1,000 bp shorter (I and III: 919 bp; II: 1,127 bp) than in the other plastomes, i.e., the ycf1 genes were seriously deleted at the 5′ end of the three plastomes of G. altaicum. In G. microrrhynchum (I), the ropC1 gene was ca. 750 bp shorter than the other 25 Nandinoideae plastomes due to an insertion of “A” in the locus of 1214 bp/1314, which resulted in its premature termination. For all the plastomes of Leontice and Gymnospermium, the ndhF gene had a 75-bp deletion (in the middle part) when compared with that of Nandina and Caulophyllum, the two early diverging genera of Nandinoideae (see “Comparative Plastome Analysis and Identification of diversity hotspot regions”). The GC content of the 26 plastomes ranged from 37.7% to 38.2%, whereby the highest values occurred in the IR regions (41.5–43.2%), followed by the LSC (36.1–36.6%) and SSC (30.7–32.6%) regions (Table 2).
Comparative Plastome Analysis and Identification of Diversity Hotspot Regions
The global visualization alignment with mVISTA and MAUVE revealed that all the 26 Nandinoideae plastomes had a consistent gene order, whereby the IR regions showed a higher level of sequence identity than the two single-copy (SSC, LSC) regions (Supplementary Figures 1, 2). Across these 26 plastomes, we calculated levels of nucleotide diversity (Pi values) for a total of 137 regions, including 58 intergenic spacer (IGS) regions, 61 protein-coding (CDS) regions, 17 intron regions (of CDS/tRNA genes), and one rRNA gene (Figure 4). In general, the IGS regions exhibited higher levels of diversity than the CDS and intron regions. More specifically, for the 61 CDS regions, Pi ranged from 0.00061 (psbE) to 0.02346 (ycf1), yet only two genes, ycf1 and rpl22 showed remarkably high diversity (Pi > 0.02). By contrast, for the 58 IGS regions, Pi ranged from 0.00129 (rrn16S-trnI) to 0.03757 (trnH-psbA), and 19 of those showed remarkably high diversity (Pi > 0.02; i.e., rps8-rpl14, ndhE-ndhG, psbE-petL, trnT-psbD, psbZ-trnG, petN-psbM, trnP-psaJ, rpl32-trnL, trnK-rps16, trnD-trnY, petA-psbJ, psaC-ndhE, rps15-ycf1, ndhC-trnV, rps16-trnQ, ndhG-ndhI, trnT-trnL, ndhF-rpl32, and trnH-psbA; see Figure 4). The sizes and Pi values of these 21 hotspot regions were shown in Supplementary Table 3, and phylogenetic trees based on each region were shown in Supplementary Figure 3.
Phylogenetic Analyses
The 80 plastome CDS regions of the 16 species (n = 26 accessions) of Nandinoideae and the two Berberis outgroup species were aligned with a total length of 70,192 bp. The topologies of the resulting ML and BI trees (Figure 5) were fully congruent and well resolved, with highly supported nodes. In fact, all three multi-species genera (Caulophyllum, Leontice and Gymnospermium) and all species with multiple accessions formed distinct clades, with both maximum ML bootstrap support (BS = 100%) and highest posterior probability (PP = 1). Only a few nodes (2 for ML tree vs. 4 for BI tree) in the tree received no full support. Within Nandinoideae, Nandina (N. domestica) was identified as the first diverging lineage, followed by Caulophyllum (represented by 2 spp./n = 3) and the sister genera Leontice (3 spp./n = 3) and Gymnospermium (10 spp./n = 19). Within Leontice, L. armeniaca was recovered as sister to L. ewersmannii + L. incerta. Within Gymnospermium, G. microrrhynchum (northeastern Asia) was the first diverging species (Clade A), followed by the East China endemic G. kiangnanense (Clade B). All the remaining species formed two highly supported sister clades (C, D), comprising three species from West Tianshan (Clade C: G. albertii as sister to G. vitellinum + G. darwasicum) vs. five species (Clade D) distributed in the Altai Mountains (G. altaicum), Caucasus Mountains (G. smirnovii), and southeastern Europe (G. odessanum, G. peloponnesiacum, G. scipetarum). Noticeably, within the subclade G. darwasicum (I and II) + G. vitellinum, the latter was embedded within the former, with low support (BS = 62%, PP = 0.8) at the node of G. darwasicum (II) and G. vitellinum. Within Clade D, G. scipetarum + G. smirnovii appeared to be a sister group to a subclade (BS = 91%, PP = 1) comprised of G. altaicum + G. odessanum + G. peloponnesiacum.
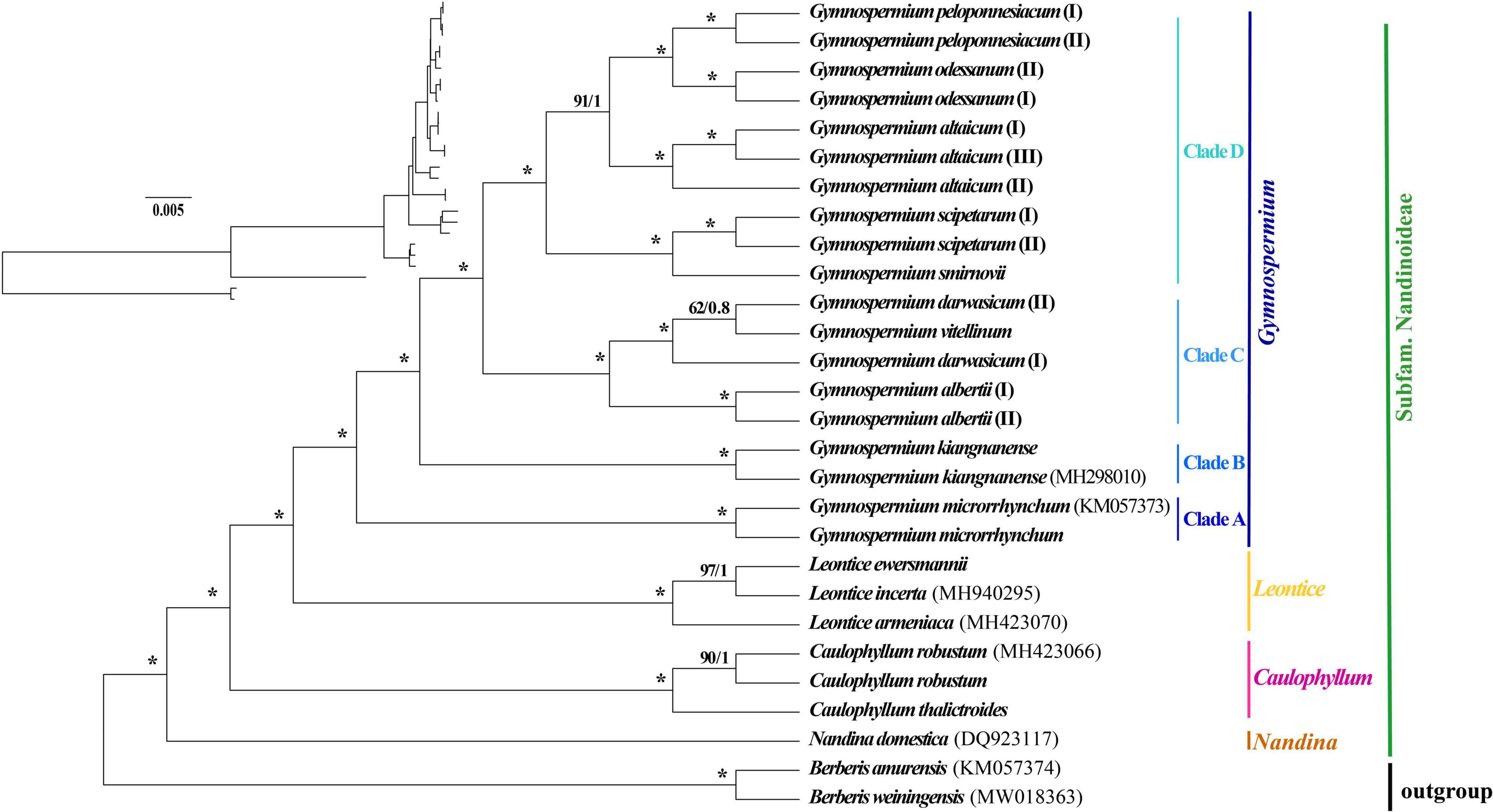
Figure 5. Phylogenetic tree reconstructions of 16 species of Nandinoideae (n = 26 accessions) plus two outgroup species of Berberis based on 80 plastome-derived CDS genes inferred from maximum likelihood (ML) and Bayesian inference (BI), respectively. Consecutive numbers at each node represent ML bootstrap support (BS) and BI posterior probability (PP) values, respectively. Asterisks indicate maximum support by both measures (i.e., BS = 100%/PP = 1).
Discussion
Comparative Plastome Genomics
All the 20 plastomes of Nandinoideae newly sequenced in this study contained 114 unique genes and in the same order (Supplementary Table 1 and Supplementary Figures 1, 2). This is consistent with six previously reported plastomes of this subfamily (Moore et al., 2006; Sun et al., 2018; Yang et al., 2018; He et al., 2019), and also agrees with the gene content and order of plastomes of Podophylloideae (Ye et al., 2018). However, these Nandinoideae plastomes have one more gene (rpoA) than the genera of Berberidoideae, such as Alloberberis C. C. Yu and K. F. Chung, Berberis, Mahonia, and Moranothamnus C. C. Yu and K. F. Chung (Hsieh et al., 2022), and two more genes (infA, ycf15) than Epimedium (Zhang et al., 2016), a member of Podophylloideae. In the present study, we also observed that the ψycf1 pseudogene of Gymnospermium smirnovii was ∼3,700 bp longer than in the 25 other Nandinoideae plastomes, which resulted in a significant IR expansion, as likewise found in several species of Berberidoideae and Podophylloideae (Epimedium ecalcaratum G. Y. Zhong, MN939634; E. brevicornu Maxim., MN371716) (Ma et al., 2013; Sun et al., 2018; Zheng et al., 2019; Hsieh et al., 2022). Insertions/deletions (indels) in the intergenic spacer (IGS) regions also contributed significantly to plastome length variations among species of Nandinoideae. As a case in point, the plastomes of two accessions of Gymnospermium microrrhynchum (I and KM057373) and one of G. kiangnanense (MH298010) were > 160,100 bp, while most other plastomes were < 158,600 bp, except for G. smirnovii (161,406 bp). Moreover, within G. kiangnanense, the plastome of accession ‘I’ was 2,180 bp shorter than that of ‘MH298010,’ mainly due to indels in the IGS regions. However, such large differences were not found in other species of Nandinoideae, and further study is needed to clarify the apparently dynamic evolution of the G. kiangnanense plastome. In general, indel and premature gene termination events are well known factors of plastome evolution in angiosperms (Fu et al., 2017; Wang et al., 2019; Zhang et al., 2020), including species of Berberidaceae (Sun et al., 2016, 2018; Hsieh et al., 2022). However, the severe truncation at the the 5′ end of the ycf1 gene in the three analyzed plastomes of G. altaicum plastomes (I and III: 919 bp; II: 1,127 bp), and the premature termination in the ropC1 gene have not been reported in the previous complete plastome analyses of Berberidaceae. Notably, a 75-bp deletion in the ndhF gene occurred in Leontice and Gymnospermium, which thus can be interpreted to present a molecular synapomorphy of these sister genera (Figure 5). As to another interesting observation, the length of the rps7 gene was consistently 468 bp across the 26 Nandinoideae plastomes analyzed, similar length (460 bp) with most genera of Berberidaceae (Berberis, Mahonia, Achlys DC., Bongardia C. A. Mey., Vancouveria C. Morren and Decne., Epimedium and Jeffersonia W. Bartram), while this gene is considerably short (63–78 bp) in some species of Podophylloideae (Zhang et al., 2016; Sun et al., 2018; Ye et al., 2018; Hsieh et al., 2022). On the other hand, significant variation in the length of the accD gene has recently been reported for Berberidoideae (Hsieh et al., 2022), but this is not observed in Nandinoideae (Supplementary Table 2).
Identification of Highly Variable Plastome Regions in Nandinoideae
Plastid markers combined with nuclear ribosomal DNA (ITS) sequences have proven useful in resolving the backbone phylogeny of Berberidaceae. Kim et al. (1996, 2004) identified the four clades of Beberidaceae consistent with the chromosomal groups (n = 7 for Berberidoideae, n = 6 for Podophylloideae, and n = 8/10 for Nandinoideae), as well as the systematic position of Ranzania T. Itô, Bongardia, Diphylleia Michx. and Nandina based on ITS, rbcL and ndhF. More recently, Yu and Chung (2017) proposed two new genera, Alloberberis and Moranothamnus (see “Comparative Plastome Genomics”), by concatenating ITS with plastid sequences of three coding regions (accD, ndhF, rbcL) and one IGS region (psbA-trnH). Nevertheless, phylogenetic and phylogeographic studies within Nandinoideae are still rare. Barina et al. (2017) and Rosati et al. (2018) used ITS and different IGS plastid regions (ndhF-trnL and trnL-trnF, respectively) to explore the phylogenetic relationships of European Gymnospermium taxa, albeit with low levels of resolution. Our hotspot region analysis of whole plastomes disclosed that two coding genes (rpl22, ycf1) as well as 19 IGS regions (detailed in §3.2) had nucleotide diversity (Pi) values > 0.02. For seven of these 21 regions (ycf 1, trnT-trnL, trnH-psbA, trnK-rps16, ndhF-rpl32, psbE-petL, rps15-ycf1), the monophyly of all genera was successfully recovered (Supplementary Figure 3). Hence, these genes and regions, in particular, will be powerful molecular markers for future phylogenetic, phylogeographic and/or conservation genetic studies in Nandinoideae. Moreover, these markers should serve as ideal DNA barcodes to discriminate among the often rare, endangered, and medicinally important species of this subfamily (see also Chang et al., 2004; Doroftei and Mierla, 2007; Karl and Strid, 2009; Mikatadze-Pantsulaia et al., 2010; Abidkulova et al., 2021).
Phylogenetic, Taxonomic and Biogeographic Inferences
Our phylogenomic ML and BI analyses of Nandinoideae, based on 80 plastome-derived CDS genes from 16 (out of ca. 20) species and 26 accessions, plus two species of Berberis as outgroup (Figure 5), is the most comprehensive phylogenetic study of this subfamily by now, and provided a robust resolution for both generic and species relationships. Our results revealed a sister relationship between Gymnospermium and Leontice, which is consistent with previous studies (Kim and Jansen, 1996; Wang et al., 2007; Sun et al., 2018; Hsieh et al., 2022). However, this result differs from previous morphological-cladistic studies (Loconte and Estes, 1989; Nickol, 1995), which inferred Leontice as sister to either Caulophyllum or Bongardia. Within Leontice, we identified L. armeniaca from Western Asia as sister to a clade comprising two other species from Central Asia (L. ewersmannii, L. incerta). In recent studies, Gymnospermium maloi Kit Tan & Shuka has been reduced to a heterotypic synonym of G. scipetarum, based on morphological, karyological and molecular evidence (Tan et al., 2011; Barina et al., 2015, 2017). Hence, our sampling of Gymnospermium contained all species except one, G. silvaticum from western Tianshan. Rosati et al. (2018) sampled six Gymnospermium species for molecular analysis based on ITS and trnL-F sequences, and identified Italian (southern Apennine) populations of Gymnospermium representing the genus’ western range limit, as a subspecies of G. scipetarum (subsp. eddae Rosati, Farris, Fascetti and Selvi). In an earlier phylogenetic study, Barina et al. (2017) had sampled seven species of Gymnospermium to infer the genus’ ‘European’ evolutionary history, but lacked particular sequence information for G. microrrhynchum (ITS) and G. kiangnanense (ndhF-trnL), and neither included any West Tianshan species. Our phylogeny (Figure 5) is the first to identify G. microrrhynchum (Clade A) from northeastern Asia as the genus’ earliest diverging species, followed by G. kiangnanense (Clade B) from eastern China (representing the genus’ southern range limit). Three species from West Tianshan (G. albertii, G. darwasicum, G. vitellinum) formed a highly supported derived clade (‘C’), with G. vitellinum showing closer relationships to the two samples of G. darwasicum (Figure 5). Indeed, based on morphological characters, G. vitellinum has previously been inferred to be most closely related to G. darwasicum (Kral, 1981), yet due to lack of resolution, our phylogenomic data cannot exclude the possibility that these two species might be conspecific and/or hybridizing (etc.). As sister to the West Tianshan Clade C, we identified a highly supported group (Clade ‘D’) of mostly (but not exclusively) westerly distributed species (see also Tian and Mullaj, 2001a,b; Barina et al., 2007; 2015; 2017; Rosati et al., 2014), including G. smirnovii (Caucasus Mountains) + G. scipetarum (central Albania, southern Montenegro, Italy/southern Apennines) as likely sister group of a subclade (BS = 91, PP = 1) comprising G. altaicum (Central Asia; Abidkulova et al., 2021) as putative sister to G. odessanum (northern Black Sea area) + G. peloponnesiacum (southern Greece/Peloponnese). Clearly, these relationships have to be treated with some caution given that one species of Gymnospermium is missing in our taxon sampling, and further studies are required to clarify their biogeographic history, but which already now seems to point at an east-to-west expansion. Considering the apparently close relationship between G. peloponnesiacum and G. altaicum, it is noteworthy they retained several distinct morphological features even when cultivated together in the Göteborg Botanical Garden (Karl and Strid, 2009). Moreover, with regard to the putative sister pair G. smirnovii/G. scipetarum (I, II), it is worth to recall that the plastome of only the former species accession shows a significant expansion of the IR regions (caused by ycf1 length variation); yet again a wider sampling within both species would be needed to clarify whether the presence/absence of this IR expansion could serve as a species-diagnostic molecular marker. Altogether, we suggest that the five species of Clade D should be considered as independent species, rather than subspecies or varieties of G. altaicum (Stearn et al., 1993; Tian and Mullaj, 2001a; Phitos et al., 2003; Doroftei and Mierla, 2007).
Considering broader, yet preliminary biogeographic inferences from our phylogeny (Figure 5), it seems possible that Gymnospermium originated in northeast Asia and subsequently spread southward and westward, resulting in the genus’ high species diversity seen today. Notably, in our field surveys in China (Pan Li and Zhaoping Yang, personal observation), species of the Eurasian/North American genus Erythronium L. (Liliaceae; ca. 27–32 spp.) were often found to be associated with Gymnospermium, for instance, G. microrrhynchum with E. japonicum Decne. in northeast China, and G. altaicum with E. sibiricum (Fisch. and C. A. Mey.) Krylov in the Altai Mountains of northwest China. Based on an earlier molecular (plastid/nuclear) phylogeny of Erythronium (Allen et al., 2003), there is relatively little divergence among the four Eurasian species (distributed from Portugal to Japan). In addition, we found many other spring ephemerals accompanying Gymnospermium and Erythronium in northeast and northwest China, such as Gagea Salisb. and Tussilago L., all of which share similar distribution patterns across Eurasia (Peterson et al., 2008; Chen and Nordenstam, 2011). Hence, it would be extremely interesting to clarify in future comparative phylogeographic studies whether all these spring ephemerals share a similar biogeographical history of east-to-west expansion routes across Eurasia, including joint areas of (glacial) refugial survival.
Finally, it worth to briefly review previous time estimates of divergence within Nandinoideae. For example, in their plastid (rbcL) study on plant (sister-) species pairs with disjunct distributions in East Asia (EA) and eastern North America (ENA), Xiang et al. (2000) estimated the divergence time between Caulophyllum robustum (EA) and C. thalictroides (ENA) to the Early Pleistocene, ca. 2.38 ± 1.69 million years ago (Mya). By contrast, Barina et al. (2017), using nuclear (ITS) and plastid sequences (ndhF-trnL), dated the split between Leontice and Gymnospermium (likely in East Asia) to the mid-Miocene, ca. 16.4 (32.7–7.4) Mya, while the onset of diversification the latter genus, including the westward spread of the G. altaicum lineage to the Black Sea area, likely occurred in the early Late Miocene, ca. 11.4 (23.4–4.8) Mya. Based on a plastid phylogenomic approach, Sun et al. (2018) dated the divergence of Nandina and the other three genera of Nandinoideae at 33–13 Mya, and ca. 5 Mya for Leontice and Gymnospermium. Altogether, these authors included only one species for each genus of Nandinoideae, and failed to reconstruct the ancestral areas of Caulophyllum, Leontice, and Gymnospermium, with uncertain ancestral area (Sun et al., 2018). As a result, the evolutionary history of Nandinoideae was still unclear. In total, these inferences of divergence times and dispersal routes were mainly based on a limited sampling of taxa and mostly plastid (Xiang et al., 2000; Sun et al., 2018), or more rarely, plastid and nuclear (ITS) data (Barina et al., 2017). Hence, further phylogenomic analyses of both plastome and nuclear genomic (or transcriptomic) data are required to fully uncover the evolutionary and biogeographic history of Nandinoideae.
Data Availability Statement
The datasets presented in this study can be found in online repositories. The names of the repository/repositories and accession number(s) can be found in the article/Supplementary Material.
Author Contributions
PL and ZY designed the research and provided the research resources. PL, DZ, ZY, SS, XL, XZ, and JL collected the plant materials. SS, HL, and ZY assembled and analyzed the data and prepared the figures and tables. ZY, SS, PL, and HC wrote the manuscript. All authors revised the manuscript.
Funding
This research was supported by the National Natural Science Foundation of China (Grant Nos. 32060053 and 31970225) and the National Science and Technology Basic Program of China (2019FY100204) and Chenshan Special Foundations from Shanghai Municipal Administration of Forestation and City Appearances (G222404).
Conflict of Interest
The authors declare that the research was conducted in the absence of any commercial or financial relationships that could be construed as a potential conflict of interest.
Publisher’s Note
All claims expressed in this article are solely those of the authors and do not necessarily represent those of their affiliated organizations, or those of the publisher, the editors and the reviewers. Any product that may be evaluated in this article, or claim that may be made by its manufacturer, is not guaranteed or endorsed by the publisher.
Acknowledgments
We are grateful to Xinjie Jin and Xiaokai Fan for helpful suggestions in the data analyses.
Supplementary Material
The Supplementary Material for this article can be found online at: https://www.frontiersin.org/articles/10.3389/fpls.2022.913011/full#supplementary-material
Supplementary Figure 1 | Comparison of the 26 plastomes of Nandinoideae analyzed in this study using mVISTA, with Ranzania japonica (MG234280) as a reference.
Supplementary Figure 2 | MAUVE alignment of the 26 Nandinoideae plastomes analyzed in this study, with Gymnospermium albertii (I) as a reference.
Supplementary Figure 3 | Maximum likelihood (ML) trees based on each of the 21 hotspot regions (Pi > 0.02). (A) ycf1; (B) trnT-trnL; (C) trnT-psbD; (D) rpl32-trnL; (E) trnH-psbA; (F) petN-psbM; (G) trnK-rps16; (H) ndhG-ndhI; (I) ndhF-rpl32; (J) psbE-petL; (K) ndhC-trnV; (L) rpl22; (M) petA-psbJ; (N) trnP-psaJ; (O) rps15-ycf1; (P) rps16-trnQ; (Q) psbZ-trnG; (R) trnD-trnY; (S) rps8-rpl14; (T) psaC-ndhE; U, ndhE-ndhG. Numbers listed at each node represents bootstrap support (BS) values. The hyphen indicates BS < 50%.
Footnotes
References
Abidkulova, D. M., Ivashchenko, A. A., Sramko, G., Kurbatova, N. V., and Abidkulova, K. T. (2021). Gymnospermium altaicum (Pall.) Spach (Berberidaceae), an early spring element of wild fruit forests of the Trans-Ili Alatau. Exp. Biol. 86, 14–26. doi: 10.26577/eb.2021.v86.i1.02
Allen, G. A., Soltis, D. E., and Soltis, P. S. (2003). Phylogeny and Biogeography of Erythronium (Liliaceae) inferred from chloroplast matK and nuclear rDNA ITS sequences. Syst. Bot. 28, 512–523.
Al-Snafi, A. E. (2019). Constituents and pharmacological effects of Leontice leontopetalum – a review. Chem. J. 3, 103–108.
Amiryousefi, A., Hyvonen, J., and Poczai, P. (2018). IRscope: an online program to visualize the junction sites of chloroplast genomes. Bioinformatics 34, 3030–3031. doi: 10.1093/bioinformatics/bty220
Barina, Z., Caković, D., Pifkó, D., Schönswetter, P., Somogyi, G., and Frajman, B. (2017). Phylogenetic relationships, biogeography and taxonomic revision of European taxa of Gymnospermium (Berberidaceae). Bot. J. Linn. Soc. 184, 298–311. doi: 10.1093/botlinnean/box028
Barina, Z., Pifco, D., and Kiraly, G. (2007) “Data on the flora of Griba Mountains (South-Albania),” in Abstracts, 4th Balkan Botanical Congress, Sofia, 20–26 June 2006 (Sofia: Bulgarian Academy of Sciences), 265.
Barina, Z., Pintér, B., and Pifkó, D. (2015). Morphometrical studies on Gymnospermium scipetarum and G. maloi (Berberidaceae). Wulfenia 22, 209–220.
Baytop, T. (1984). Therapy with Medicinal Plants in Turkey (Past and Present). Istanbul: Istanbul University Press.
Chang, C. S., Kim, H., Park, T. Y., and Maunder, M. (2004). Low levels of genetic variation among southern peripheral populations of the threatened herb. Leontice microrhyncha (Berberidaceae) in Korea. Biol. Conserv. 119, 387–396. doi: 10.1016/j.biocon.2003.12.003
Chen, X. H., Xiang, K. L., Lian, L., Peng, H. W., Erst, A. S., Xiang, X. G., et al. (2020). Biogeographic diversification of Mahonia (Berberidaceae): implications for the origin and evolution of East Asian subtropical evergreen broadleaved forests. Mol. Phylogenetics Evol. 151:106910. doi: 10.1016/j.ympev.2020.106910
Chen, Y. L., and Nordenstam, B. (2011). “Asteraceae-Tussilago L,” in Flora of China, eds Z. Y. Wu, P. H. Raven, and D. Y. Hong (St. Louis: Missouri Botanical Garden Press), 714–800.
Darling, A. C., Mau, B., Blattner, F. R., and Perna, N. T. (2004). Mauve: multiple alignment of conserved genomic sequence with rearrangements. Genome Res. 14, 1394–1403. doi: 10.1101/gr.2289704
Darriba, D., Taboada, G. L., Doallo, R., and Posada, D. (2012). jModelTest 2: more models, new heuristics and parallel computing. Nat. Methods 9:772. doi: 10.1038/nmeth.2109
Dierckxsens, N., Mardulyn, P., and Smits, G. (2017). NOVOPlasty: de novo assembly of organelle genomes from whole genome data. Nucleic Acids Res. 45:e18. doi: 10.1093/nar/gkw955
Doroftei, M., and Mierla, M. (2007). Gymnospermium altaicum in northern Dobrogea. Brukenthal Acta Musei 2, 49–54.
Erichsen-Brown, C. (1989). Medicinal and Other uses of North American Plants: a Historical Survey with Special Reference to the Eastern Indian Tribes. New York, NY: Dover publications.
Frazer, K. A., Pachter, L., Poliakov, A., Rubin, E. M., and Dubchak, I. (2004). VISTA: computational tools for comparative genomics. Nucleic Acids Res. 32, W273–W279. doi: 10.1093/nar/gkh458
Fu, C. N., Li, T. H., Milne, R., Zhang, T., Ma, P. F., Yang, J., et al. (2017). Comparative analyses of plastid genomes from fourteen cornales species: inferences for phylogenetic relationships and genome evolution. BMC Genom. 18:956. doi: 10.1186/s12864-017-4319-9
Govaerts, R., Nic Lughadha, E., Black, N., Turner, R., and Paton, A. (2021). The world checklist of vascular plants, a continuously updated resource for exploring global plant diversity. Sci. Data 8:215 doi: 10.1038/s41597-021-00997-6
Greiner, S., Lehwark, P., and Bock, R. (2019). OrganellarGenomeDRAW (OGDRAW) version 1.3.1: expanded toolkit for the graphical visualization of organellar genomes. Nucleic Acids Res. 47, W59–W64. doi: 10.1093/nar/gkz238
Gresser, G., Bachmann, P., Witte, L., and Czygan, F. C. (1993). Distribution and taxonomic significance of quinolizidine alkaloids in Leontice leontopetalum and L. ewersmannii (Berberidaceae). Biochem. Syst. Ecol. 21, 679–685. doi: 10.1016/0305-1978(93)90072-Y
He, P. Z., Ma, Q., Dong, M. F., Yang, Z. P., and Liu, L. X. (2019). The complete chloroplast genome of Leontice incerta and phylogeny of Berberidaceae. Mitochondrial DNA Part B 4, 101–102.
Hess, H. E., Landolt, E., and Hirzel, R. (1977). Berberidaceae” in Flora der Schweiz und Angrenzender Gebiete. Zurich: Birkhäuser Basel Press, 103–105.
Hsieh, C. L., Yu, C. C., Huang, Y. L., and Chung, K. F. (2022). Mahonia vs. Berberis unloaded: generic delimitation and infrafamilial classification of Berberidaceae based on plastid phylogenomics. Front. Plant Sci. 12:720171. doi: 10.3389/fpls.2021.720171
Hutchinson, J. (1920). Jeffersonia and Plagiorhegma. Bull. Misc. Inform. 1920, 242–245. doi: 10.2307/4107483
Ikuta, A., and Itokawa, H. (1988). Alkaloids of tissue cultures of Nandina domestica. Phytochemistry 27, 2143–2145.
Iskandarov, S., Nuriddinov, R. N., and Yunusov, S. Y. (1967). Alkaloids of Leontice albertii. Chem. Nat. Compd. 3, 297–298. doi: 10.1007/BF00574645
Karl, R., and Strid, A. (2009). Bongardia chrysogonum (Berberidaceae) rediscovered on the East Aegean island of Chios. Phytol. Balc. 15, 337–342.
Katoh, K., and Standley, D. M. (2013). MAFFT multiple sequence alignment software version 7: improvements in performance and usability. Mol. Biol. Evol. 30, 772–780. doi: 10.1093/molbev/mst010
Kim, Y. D., and Jansen, R. K. (1996). Phylogenetic implications of rbcL and ITS sequence variation in the Berberidaceae. Syst. Bot. 21, 381–396. doi: 10.2307/2419666
Kim, Y. D., Kim, S. H., Kim, C. H., and Jansen, R. K. (2004). Phylogeny of Berberidaceae based on sequences of the chloroplast gene ndhF. Biochem. Syst. Ecol. 32, 291–301. doi: 10.1016/j.bse.2003.08.002
Kolak, U., Hacıbekiroðlu, I., Boða, M., Özgökçe, F., Ünal, M., Choudhary, M. I., et al. (2011). Phytochemical investigation of Leontice leontopetalum L. subsp. ewersmannii with antioxidant and anticholinesterase activities. Rec. Nat. Prod. 5, 309–313.
Kral, M. (1981). Gymnospermium vitellinum, a new species of the genus Gymnospermium. Preslia 53, 67–68.
Liao, M. C., Wang, Y. W., and Xiao, P. G. (2001). Study on dioctive compounds of Leontice kiangnanensis. Chin. J. Plant Sci. 19, 513–516.
Loconte, H., and Blackwell, W. H. (1985). Intrageneric taxonomy of Caulophyllum (Berberidaceae). Rhodora 87, 463–469.
Loconte, H., and Estes, J. R. (1989). Generic relationships within Leonticeae (Berberidaceae). Can. J. Bot. 67, 2310–2316. doi: 10.1139/b89-295
Ma, J., Yang, B., Zhu, W., Sun, L., Tian, J., and Wang, X. (2013). The complete chloroplast genome sequence of Mahonia bealei (Berberidaceae) reveals a significant expansion of the inverted repeat and phylogenetic relationship with other angiosperms. Gene 528, 120–131. doi: 10.1016/j.gene.2013.07.037
Mikatadze-Pantsulaia, T., Barblishvili, T., Trivedi, C., Kikodze, D., and Khutsishvili, M. (2010). Ex situ conservation of some endemic and protected plant species in Georgia. Kew Bull. 65, 643–648.
Moore, M. J., Dhingra, A., Soltis, P. S., Shaw, R., Farmerie, W. G., Folta, K. M., et al. (2006). Rapid and accurate pyrosequencing of angiosperm plastid genomes. BMC Plant Biol. 6:17. doi: 10.1186/1471-2229-6-17
Nickol, M. G. (1995). Phylogeny and inflorescences of Berberidaceae –a morphological survey. Plant Syst. Evol. 9, 327–340. doi: 10.1007/978-3-7091-6612-3_35
Peng, C. Y., Liu, J. Q., Zhang, R., and Shu, J. C. (2014). A new alkaloid from the fruit of Nandina domestica Thunb. Nat. Prod. Res. 28, 1159–1164. doi: 10.1080/14786419.2014.921166
Peng, Y. (2006). A pharmacophylogenetic study of the Berberidaceae (s.l.). Acta Phytotax. Sin. 44, 241–257. doi: 10.1360/aps040149
Peterson, A., Levichev, I. G., and Peterson, J. (2008). Systematics of Gagea and Lloydia (Liliaceae) and infrageneric classification of gagea based on molecular and morphological data. Mol. Phylogenet. Evol. 46, 446–465. doi: 10.1016/j.ympev.2007.11.016
Phitos, D., Strid, A., and Tan, K. (2003). “Gymnospermium Spach,” in Flora Hellenica, eds A. Strid and K. Tan (Hessen: Koeltz Botanical Books Press), 81–82.
Rader, J. I., and Pawar, R. S. (2013). Primary constituents of blue cohosh: quantification in dietary supplements and potential for toxicity. Anal. Bioanal. Chem. 405, 4409–4417. doi: 10.1007/s00216-013-6783-7
Ronquist, F., Teslenko, M., Van Der Mark, P., Ayres, D. L., Darling, A., Hohna, S., et al. (2012). MrBayes 3.2: efficient Bayesian phylogenetic inference and model choice across a large model space. Syst. Biol. 61, 539–542. doi: 10.1093/sysbio/sys029
Rosati, L., Coppi, A., Farris, E., Fascetti, S., Becca, G., Peregrym, M., et al. (2018). The genus Gymnospermium (Berberidaceae) in Italy: identity and relationships of the populations at the western limit of the genus range. Plant Biosyst. 153, 796–808. doi: 10.1080/11263504.2018.1549613
Rosati, L., Farris, E., Tilia, A., Potenza, G., and Fascetti, S. (2014). “Gymnospermium scipetarum (Berberidaceae) specie nuova per la flora italiana,” in Floristica, Sistematica ed Evoluzione, Comunicazioni, eds L. Peruzzi and G. Domina (Rome: Societa Botanica Italiana Press), 7–8.
Rozas, J., Ferrer-Mata, A., Sanchez-Delbarrio, J. C., Guirao-Rico, S., Librado, P., Ramos-Onsins, S. E., et al. (2017). DnaSP 6: DNA sequence polymorphism analysis of large data sets. Mol. Biol. Evol. 34, 3299–3302. doi: 10.1093/molbev/msx248
Shi, L., Chen, H., Jiang, M., Wang, L., Wu, X., Huang, L., et al. (2019). CPGAVAS2, an integrated plastome sequence annotator and analyzer. Nucleic Acids Res. 47, W65–W73. doi: 10.1093/nar/gkz345
Son, Y., An, Y., Jung, J., Shin, S., Park, I., Gwak, J., et al. (2019). Protopine isolated from Nandina domestica induces apoptosis and autophagy in colon cancer cells by stabilizing p53. Phytother Res. 33, 1689–1696. doi: 10.1002/ptr.6357
Stamatakis, A. (2014). RAxML version 8: a tool for phylogenetic analysis and post-analysis of large phylogenies. Bioinformatics 30, 1312–1313. doi: 10.1093/bioinformatics/btu033
Stearn, W. T. (1938). Epimedium and Vancouveria (Berberidaceae), a monograph. Bot. J. Linn. Soc. 51, 409–535. doi: 10.1111/j.1095-8339.1937.tb01914.x
Stearn, W. T., Webb, D. A., Tutin, T. G., Heywood, V. H., Burges, N. A., Valentine, D. H., et al. (1993). “Gymnospermium Spach,” in Flora Europaea, ed. T. G. Tutin (Cambridge: Cambridge University Press), 29–295.
Sun, Y., Moore, M. J., Landis, J. B., Lin, N., Chen, L., Deng, T., et al. (2018). Plastome phylogenomics of the early-diverging eudicot family Berberidaceae. Mol. Phylogenet. Evol. 128, 203–211. doi: 10.1016/j.ympev.2018.07.021
Sun, Y., Moore, M. J., Zhang, S., Soltis, P. S., Soltis, D. E., Zhao, T., et al. (2016). Phylogenomic and structural analyses of 18 complete plastomes across nearly all families of early-diverging eudicots, including an angiosperm-wide analysis of IR gene content evolution. Mol. Phylogenet. Evol. 96, 93–101. doi: 10.1016/j.ympev.2015.12.006
Tabatadze, N., Bun, S. S., Tabidze, B., Mshvildadze, V., Dekanosidze, G., Ollivier, E., et al. (2010). New triterpenoid saponins from Leontice smirnovii. Fitoterapia 81, 897–901. doi: 10.1016/j.fitote.2010.05.021
Tian, K., and Mullaj, A. (2001a). “Gymnospermium altaicum subsp. scipetarum,” in Med-Checklist Notulae, eds W. Greuter and T. H. Raus (Berlin: Willdenowia Press), 319–320.
Tian, K., and Mullaj, A. (2001b). “Gymnospermium altaicum,” in Endemic plants of Greece – The Peloponnese, eds K. Tan and G. Iatrou (Copenhagen: Gad Publishers), 138. doi: 10.1186/2241-5793-21-9
Tan, K., Shuka, L., Siljak-Yakovlev, S., Malo, S., and Pustahija, F. (2011). The genus Gymnospermium (Berberidaceae) in the Balkans. Phytotaxa 25, 1–17. doi: 10.11646/PHYTOTAXA.25.1.1
Wang, H. X., Liu, H., Moore, M. J., Landrein, S., and Wang, H. F. (2019). Plastid phylogenomic insights into the evolution of the Caprifoliaceae s.l. (Dipsacales). Mol. Phylogenet. Evol. 142:106641. doi: 10.1016/j.ympev.2019.106641
Wang, W., Chen, Z. D., Liu, Y., Li, R. Q., and Li, J. H. (2007). Phylogenetic and biogeographic diversification of Berberidaceae in the Northern Hemisphere. Syst. Bot. 32, 731–742.
Xiang, Q.-Y., Soltis, D. E., Soltis, P. S., Manchester, S. R., and Crawford, D. J. (2000). Timing the eastern Asian–eastern North American floristic disjunction: molecular clock corroborates paleontological estimates. Mol. Phylogenet. Evol. 15, 462–472. doi: 10.1006/mpev.2000.0766
Yang, Z., Peng, Z., Zhang, H., Lee, J., Liu, X., and Fu, C. (2018). The complete chloroplast genome of Gymnospermium kiangnanense (Berberidaceae): an endangered species endemic to Eastern China. Mitochondrial DNA B: Resour. 3, 713–714. doi: 10.1080/23802359.2018.1483760
Ye, W. Q., Yap, Z. Y., Li, P., Comes, H. P., and Qiu, Y. X. (2018). Plastome organization, genome-based phylogeny and evolution of plastid genes in Podophylloideae (Berberidaceae). Mol. Phylogenet. Evol. 127, 978–987. doi: 10.1016/j.ympev.2018.07.001
Ying, J., Boufford, D. E., and Brach, A. R. (2011). “Berberidaceae,” in Flora of China, eds Z. Y. Wu, P. H. Raven, and D. Y. Hong (St. Louis: Missouri Botanical Garden Press), 714–800.
Ying, T. S. (1979). On Dysosma Woodson and Sinopodophyllum Ying, gen. nov. of the Berberidaceae. Acta Phytotax. Sin. 17, 15–23.
Ying, T. S., Terabayashi, S., and Boufford, D. E. (1984). A monograph of Diphylleia (Berberidaceae). J. Arnold Arbor. 65, 57–94.
Yu, C. C., and Chung, K. F. (2017). Why Mahonia? Molecular recircumscription of Berberis s.l., with the description of two new genera, Alloberberis and Moranothamnus. Taxon 66, 1371–1392. doi: 10.12705/666.6
Zhang, D., Gao, F., Li, W. X., Jakovlić, I., Zou, H., Zhang, J., et al. (2018). PhyloSuite: an integrated and scalable desktop platform for streamlined molecular sequence data management and evolutionary phylogenetics studies. Mol. Ecol. Resour. 20, 348–355. doi: 10.1111/1755-0998.13096
Zhang, M. L., Uhink, C. H., and Kadereit, J. W. (2007). Phylogeny and biogeography of Epimedium/ Vancouveria (berberidaceae): Western North american - East Esian disjunctions, the origin of european mountain plant taxa, and east asian species diversity. Syst. Bot. 32, 81–92. doi: 10.1600/036364407780360265
Zhang, R., Wang, Y. H., Jin, J. J., Stull, G. W., Anne, B., Domingos, C., et al. (2020). Exploration of plastid phylogenomic conflict yields new insights into the deep relationships of leguminosae. Syst. Biol. 69, 613–622. doi: 10.1093/sysbio/syaa013
Zhang, Y., Du, L., Liu, A., Chen, J., Wu, L., Hu, W., et al. (2016). The complete chloroplast genome sequences of five Epimedium species: lights into phylogenetic and taxonomic analyses. Front. Plant Sci. 7:306. doi: 10.3389/fpls.2016.00306
Zheng, G., Zhang, C., Yang, J., and Xu, X. (2019). Characterization of the complete chloroplast genome of (Berberidaceae). Mitochondrial DNA B: Resour. 4, 3681–3682. doi: 10.1080/23802359.2019.1678429
Keywords: Caulophyllum, Gymnospermium, Leontice, North America-Eurasia disjunction, phylogenomics, plastome evolution
Citation: Song S, Zubov D, Comes HP, Li H, Liu X, Zhong X, Lee J, Yang Z and Li P (2022) Plastid Phylogenomics and Plastome Evolution of Nandinoideae (Berberidaceae). Front. Plant Sci. 13:913011. doi: 10.3389/fpls.2022.913011
Received: 05 April 2022; Accepted: 30 May 2022;
Published: 30 June 2022.
Edited by:
Sarah Covshoff, Independent Researcher, Las Vegas, NV, United StatesReviewed by:
Eduardo Cires, University of Oviedo, SpainJelena Mlinarec, Oikon Ltd. – Institute of Applied Ecology, Croatia
Copyright © 2022 Song, Zubov, Comes, Li, Liu, Zhong, Lee, Yang and Li. This is an open-access article distributed under the terms of the Creative Commons Attribution License (CC BY). The use, distribution or reproduction in other forums is permitted, provided the original author(s) and the copyright owner(s) are credited and that the original publication in this journal is cited, in accordance with accepted academic practice. No use, distribution or reproduction is permitted which does not comply with these terms.
*Correspondence: Zhaoping Yang, yzpzky@163.com; Pan Li, panli_zju@126.com