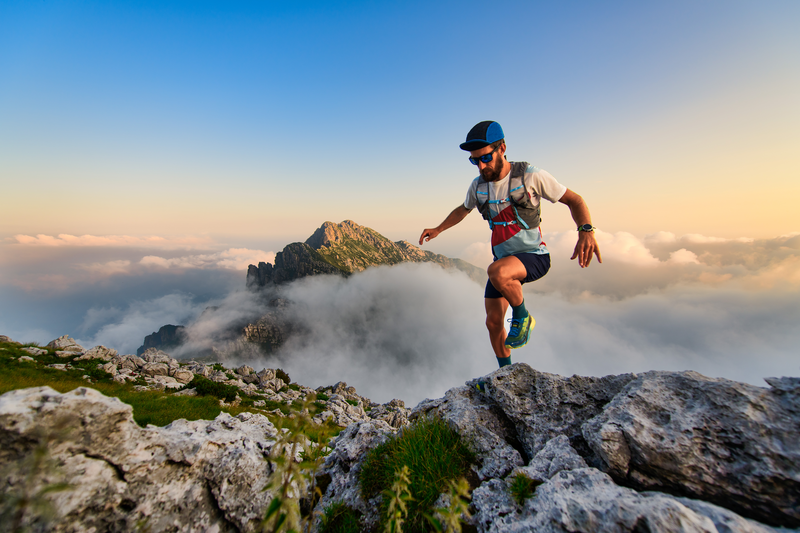
94% of researchers rate our articles as excellent or good
Learn more about the work of our research integrity team to safeguard the quality of each article we publish.
Find out more
ORIGINAL RESEARCH article
Front. Plant Sci. , 10 June 2022
Sec. Crop and Product Physiology
Volume 13 - 2022 | https://doi.org/10.3389/fpls.2022.906060
A quantitative understanding of the factors driving changes in grain filling is essential for effective prioritization of increasing maize yield. Grain filling is a significant stage in maize yield formation. Solar radiation is the energy source for grain filling, which is the ultimate driving factor for final grain weight and grain filling capacity that determine maize yield. Here, we first confirmed the quantitative relationships between grain filling parameters and photosynthetically active radiation (PAR) by conducting field experiments using different shading and plant density conditions and cultivars in 2019 and 2020 in Xinjiang, China. The results showed that with every 100 MJ m−2 increase in PAR, the average grain filling rate (Gave), maximum grain-filling rate (Gmax), and the kernel weight at the time of maximum grain-filling rate (Wmax) increased by 0.073 mg kernel−1 day−1, 0.23 mg kernel−1 day−1, and 0.24 mg kernel−1, and the time of maximum grain-filling rate (Tmax) delayed by 0.91 day. Relative changes in PAR were significantly and positively correlated with relative changes in yield and Gave. With every 1% change in PAR, yield and Gave changed by 1.16 and 0.17%, respectively. From the perspective of grain filling capacity, DH618 was a more shade-resistant cultivar than XY335 and ZD958. It is urgent to breed maize cultivars with low light tolerance and high grain yield in the face of climate change, particularly the decrease in solar radiation.
Maize (Zea mays L.) is one of the most economically important crops, playing a vital role in maintaining food security in China and throughout the world (Hou et al., 2020; Liu et al., 2021c, 2022). Maize requires adequate solar radiation throughout the duration of growth to produce sufficient photoassimilates (Ren et al., 2016; Shi et al., 2018). However, recent studies have revealed that the intensity of photosynthetically active radiation (PAR) reaching the crop canopy is often reduced due to environmental pollution, overcast or rainy, and scant lighting the rainy weather frequently occurs during the crop growing season (Liu et al., 2012; Gao et al., 2017a; Zhang et al., 2019; Poonam et al., 2022). There have been varying degrees of reduction in radiation both nationally and worldwide (Ren et al., 2016; Zhou et al., 2020; Liu et al., 2021d), which could lead to reduced biomass production and maize yield (Hou et al., 2021). The reduction of light availability, especially during grain filling, threatens photosynthesis and carbohydrate synthesis, restricting maize yield (Jia et al., 2007; Zhang et al., 2007b; Ren et al., 2016; Gao et al., 2017a, 2020; Shao et al., 2020).
The grain filling stage is a crucial period during the entire growth season, with grain filling capacity determining final grain weight and yield (Shi et al., 2013; Cao et al., 2022). In the Southern and Huanghuaihai regions in China, maize yield has been limited by light deprivation during the grain filling stage (Wang et al., 2020a). The grain filling rate is one of the main limiting factors for yield increase (Ottaviano and Camussi, 1981; Xu et al., 2018). Zhou (2004) introduced that grain filling rate was one of the manifestations of grain’s ability to accumulate the photosynthate. Other studies have investigated both the rate and duration of grain filling, which jointly determine the formation of maize grain weight (Johnson and Tanner, 1972; Liu and Zhang, 2010). Grain filling in maize is influenced by various factors, for instance, genetics (Borrás et al., 2009; Li et al., 2020), irrigation methods and drought stress (Ahmad et al., 2022; Cao et al., 2022), fertilizer application (Zhang et al., 2021; Wu et al., 2022), and planting patterns and sowing densities (Jia et al., 2018). However, the amount of solar radiation received during the growing season is the most critical factor that determines the grain filling rate of maize (Shi et al., 2013, 2018; Chen et al., 2014; Rizzo et al., 2022).
Field shading is a common method used to vary solar radiation and study its effects on crop growth and development (Barnett and Pearce, 1983; Zhang et al., 2007b; Lu et al., 2013; Wang et al., 2020b). There have been many conclusions about the effects of shading on crop growth and the grain filling process in maize (Shi et al., 2013; Chen et al., 2014). It has been demonstrated that canopy shading reduces grain filling rate (Arisnabarreta and Miralles, 2008) and duration (Sandaña et al., 2009). Shi et al. (2013) reported that shading decreased the yield of summer maize by reducing the dry matter accumulation and maximum grain filling rate. Data published by Wang et al. (2020b) showed that shading stress at the grain filling stage depressed endosperm cell differentiation, which reduced storage capacity and decreased wheat yield. Furthermore, variations in solar radiation cause differences in grain filling characteristics at several growth stages (Shi et al., 2013; Chen et al., 2014). Previous studies have analyzed the qualitative effects of shading on grain filling in maize. However, the quantitative relationships between solar radiation and grain filling parameters have remained under-studied. In the present study, four shading levels were tested to investigate the effects of solar radiation on grain filling parameters, to deepen understanding of the process underlying grain weight formation, and develop effective interventions targeted to the critical stage of grain filling. We specifically aimed to (1) explore the response of final grain weight and grain-filling parameters to shading, and (2) quantify the relationship between grain filling parameters and PAR.
Field experiments were conducted at the Qitai Farm in Xinjiang, Northwestern China (43°49′N, 89°48′E; 1,020 m above sea level) in the 2019 and 2020 growing seasons. This region is characterized by the most abundant sunshine hours in China (Xue et al., 2016). Meteorological data for the 2019 and 2020 maize growing seasons were obtained from a “Watch Dog” Data Logger (Spectrum Technologies, Inc., United States) located in the experimental field. The 2-year meteorological data are summarized in Table 1. The study was conducted using a split-split plot design with three replications. Three maize cultivars were used: Denghai618 (DH618), Xianyu 335 (XY335), and Zhengdan 958 (ZD958). These three cultivars have high yield potential and are widely planted throughout China (Hou et al., 2012; Gao et al., 2017b; Liu et al., 2021b). The sowing dates were April 19, 2019 and April 18, 2020. Two planting densities were tested: 7.5 × 104 (D1) and 12 × 104 plants ha−1 (D2). Four shading treatments were applied from the three-leaf stage until maturity: 15% (85% of natural light, 15% shaded; S1), 30% (70% of natural light, 30% shaded; S2), and 50% (50% of natural light, 50% shaded; S3) shading compared to natural light, and no shading (CK). The shade nets were built with temporary scaffolding and nylon nets, and a distance of 1.5 m was maintained between the top of the maize canopy and the shade nets to keep microclimate conditions (other than solar radiation) consistent with the unshaded portions of the field. Each experimental plot area was 11 × 10 m in an alternating narrow–wide-row planting pattern (0.4 + 0.7 m) and adjacent plots were spaced 1 m apart.
Table 1. Mean daily maximum temperature (T-max), minimum temperature (T-min), diurnal temperature variation (Td), solar radiation, relative humidity (RH), and precipitation (Pre) during the maize growing season at Qitai Farm, Xinjiang, China in 2019 and 2020.
Maize was irrigated and fertilized using a drip irrigation system, with irrigation water pumped from groundwater (Zhang et al., 2017). In the 2-year field experiment, base fertilizers including urea (150 kg ha−1 N), super phosphate (225 kg ha−1 P2O5), and potassium sulfate (75 kg ha−1 K2O) were applied before sowing; additional urea (300 kg ha−1 N) was applied by fertigation during the entire irrigation period of the growing stage to ensure an adequate supply of nutrients. The experiments were conducted with no visible water stress, and pests and weeds were adequately controlled throughout the growing seasons.
At the silking stage, plants at the same growth stage were labeled. From silking until maturity, five tagged ears from each plot were sampled at 10-day intervals; 100 grains were sampled from the middle part of the ear and oven-dried at 85°C to a constant weight. The grain-filling process of maize cultivars differing in maturity was analyzed using a logistic model:
where y = grain weight (mg); x = number of days after silking; A is the potential kernel weight (mg), B and C are coefficients determined by regression. This model was used to evaluate the dynamics of accumulated grain weight in maize plants growth under different treatments (Gao et al., 2017b). The weight of a kernel at the time of the maximum grain-filling rate (Wmax) was equal to A/2 (Chen et al., 2014). The grain filling rate was derived from the first derivative of the sigmoidal equation. To determine yield (at 14% water content), an area of 16.5 m−2 comprising the central three rows of each plot (which were 5 m in length) were hand-harvested from each plot at maturity and grain moisture content was determined using a PM8188 portable moisture meter (Kett Electric Laboratory, Tokyo, Japan). PAR was measured in the wide and narrow rows with a diagonal orientation on clear days using a SunScan device (Delta-T Devices, Cambridge, United Kingdom; Liu et al., 2019; Yang et al., 2021a). Net photosynthetic rate (Pn) was measured under ambient conditions using a LI-6400 portable photosynthesis system (LI-COR Biosciences, Lincoln, Nebraska, United States) from the ear leaves of three representative plants during the grain-filling stage (30 days after silking). Cuvette conditions were 400 μmol CO2 mol−1, and PAR was 2,000 μmol m−2 s−1. The ambient temperature was 24°C–26°C. The cuvette area was 6 cm2.
Statistical analyses were performed in Excel 2016 (Microsoft, Redmond, WA, United States) and SPSS v18.0 (IBM SPSS, Chicago, IL, United States). The differences of yield, grain weight (yP), the average grain filling rate (Gave), the time of maximum grain-filling rate (Tmax), maximum grain-filling rate (Gmax), kernel weight at Tmax (Wmax), and Pn between different treatments were tested by using one-way ANOVA with the least significant difference test (LSD, α = 0.05). Pearson correlations were calculated to identify relationships between yield, PAR, Pn, yP, and grain filling parameters. We conducted univariate analyses to examine interactions with yP, and grain filling parameters as dependent variables and the year (Y), cultivar (C), planting densities (D), and shading level (S) as independent variables. Figures were produced with Origin 2018 (OriginLab, Northampton, MA, United States).
Plant growth was recorded from sowing to maturity (Table 2). The three cultivars had a similar growth duration; DH618, XY335, and ZD958 had average growth durations (sowing to maturity) of 161.4, 165.9, and 162.7 days, respectively, in the 2 experimental years. For shading treatments, the silking and maturity stages delayed, and the duration of growth was longer than the control by 2.8, 3.1, and 7.6 days for S1, S2, and S3, respectively. The yield decreased in the order of S3 > S2 > S1 > CK after shading in 2019 and 2020, significantly. The average yield for DH618, XY335, and ZD958 under all shaded conditions decreased by 6.3, 7.2, and 5.2 t ha−1, respectively, at D1 and by 6.2, 7.5, and 7.3 t ha−1 at D2 compared to the CK yields. The grain yields of the three cultivars decreased in the order of XY335 > ZD958 > DH618. At lower planting density (D1), DH618 and XY335 were more sensitive to shading than ZD958 was, but yield decreases were smaller in DH618 than in XY335 and ZD958 at the higher planting density (D2). This indicated that DH618 were better able to tolerate low light under high-density planting.
Table 2. Yield, phenological information for the three maize cultivars at different shading levels (CK, natural light; S1, 15% of natural light; S2, 30% of natural light; and S3, 50% of natural light) and densities (D1 = 7.5 × 104 plants ha−1 and D2 = 12 × 104 plants ha−1) and the total intercepted PAR in the whole stage at the Qitai research station in 2019 and 2020.
As shown in Table 3, logistic fitting results showed that the potential grain weight yP (when x = 80), differed between shading treatments; cultivar DH618 had the maximum yP value and ZD958 had the minimum value. After shading, the potential grain weight yP significantly decreased by 9.5% for ZD958, 6.7% for XY335, and 5.7% for DH618. The reduction in yP at low planting density was higher than it was at high density (9.0 and 5.6%, respectively). Compared with CK, yP decreased by 3.0, 6.7, and 12.1% in treatments S1, S2, and S3, respectively. Gave, Gmax, and Wmax significantly decreased and Tmax was delayed with increased shading levels (Table 3). Gave (among all cultivars and densities) was 2.9, 6.5, and 11.7% lower in treatment groups S1, S2, and S3, respectively, than in the CK. Likewise, Gmax was 4.5, 9.6, and 18.0% lower in S1, S2, and S3, respectively, compared to CK; Wmax was 2.7, 5.9, and 9.1% lower than CK, and Tmax was delayed by 1.0, 2.9, and 6.5 days, respectively. There were significant differences in grain filling parameters between cultivars and shading levels. On average across shading levels and densities, Gave was 4.57, 4.23, and 4.18 mg kernel−1 day−1 for cultivars DH618, XY335, and ZD958; these values were 5.6, 6.4, and 9.1% lower than the CK, respectively. Gmax was 9.24, 8.26, and 8.29 mg kernel−1 day−1, representing a decrease of 9.6, 10.4, and 12.1%, respectively, compared to the CK. Tmax was 37.9, 41.4, and 41.7 days for cultivars DH618, XY335, and ZD958, respectively, which were later than the CK by 2.7, 4.0, and 3.8 days. Wmax was 192.7, 180, and 177.5 mg kernel−1 day−1 for the three cultivars, a decrease of 4.9, 5.1, and 7.7% compared to the CK. Shading treatment had a great effect on Gave and Wmax at low planting density (D1), whereas high planting density (D2) had a great effect on Gmax and Tmax compared with CK on average across all shading levels and cultivars.
As shown in Figure 1, ear leaf photosynthetic rate (Pn) decreased significantly in all three cultivars with shading in 2019 (Figure 1A) and 2020 (Figure 1B). On average across years, cultivars, and densities, the mean Pn decreased by 7.6, 17.6, and 32.6% in treatment groups S1, S2, and S3, respectively, compared with CK. On average across years, shading levels, and densities, mean Pn decreased in cultivars XY335, ZD958, and DH618 by 19.9, 18.6, and 17.5%, respectively, compared with CK. On average across years, shading levels, and cultivars, mean Pn decreased by 16.4 and 20.9% under the D1 and D2 planting densities, respectively, compared to CK.
Figure 1. Ear leaf photosynthetic rate (Pn) at the grain-filling stage for maize grown in 2019 (A) and 2020 (B). DH, Denghai618; XY, Xianyu 335; ZD, Zhengdan 958; CK, natural light; S1, 85% of natural light (15% shaded); S2, 70% of natural light; S3, 50% of natural light; D1, 7.5 × 104 plants ha−1; and D2, 12 × 104 plants ha−1. Error bars represent the SD of three replicates. Lowercase letters indicate statistically significant differences (p < 0.05).
Gave, Gmax, and Wmax were significantly and positively correlated with PAR, whereas Tmax was negatively correlated with PAR (Figure 2). With every 100 MJ m−2 increase in PAR, Gave (Figure 2A), Gmax (Figure 2B), and Wmax (Figure 2D) increased by 0.073, 0.23 mg kernel−1 day−1, and 0.24 mg kernel−1, respectively, and Tmax (Figure 2C) was delayed by 0.91 days. Relative changes in yield and Gave between different shading treatments were significantly positively correlated with relative changes in PAR (Figure 3). With every 1% increase in PAR, yield (Figure 3A) and Gave (Figure 3B) increased by 1.16 and 0.17%, respectively.
Figure 2. Relationships between maize grain filling parameters and [Gave, average grain filling rate (A); Gmax, maximum grain-filling rate (B); Tmax, time to maximum grain-filling rate (C); Wmax, kernel weight at Tmax (D)] photosynthetically active radiation (PAR) under different treatments. Gave, average grain filling rate; Gmax, maximum grain-filling rate; Tmax, time to maximum grain-filling rate; Wmax, kernel weight at Tmax; DH, Denghai618; XY, Xianyu 335; ZD, Zhengdan 958; D1, 7.5 × 104 plants ha−1; and D2, 12 × 104 plants ha−1. *p < 0.05; **p < 0.01.
Figure 3. Relationships between relative change of PAR and relative changes of yield (A) and average grain filling rate (Gave; B). The equations for relative changes of PAR, yield, and Gave across 2 years and planting densities are inset in the top left corner of each graph. **p < 0.01.
We found that Gave, Gmax, Wmax, and Pn were significantly positively correlated with grain weight and yield. Furthermore, Tmax was delayed with increased shading levels and was significantly negatively correlated with grain weight and yield (Table 4). Significant interaction influences of year (Y), cultivar (C), planting densities (D), and shading level (S) on yP, Gave, Gmax, Tmax, and Wmax existed (Table 5). As shown in Table 5, yP and Wmax were significantly affected by the interaction of Y × D. yP, Tmax, and Wmax were significantly affected by the interactions of Y × C and Y × S. yP, Wmax, and Gmax were significantly affected by the interactions of D × C and D × S. Tmax was significantly affected by the interaction of D × C. Gave was significantly affected by the interaction of Y × D × S. yP, Tmax, Wmax, and Gmax were significantly affected by the interactions of Y × D × C, Y × D × S, Y × C × S, D × C × S, and Y × D × C × S.
Table 5. Interaction influences of year (Y), cultivar (C), planting densities (D), and shading level (S) on grain weight [yP (x = 80)], average grain filling rate (Gave), maximum grain-filling rate (Gmax), time to maximum grain-filling rate (Tmax), and the kernel weight at Tmax (Wmax).
Maize yield formation is determined by grain filling capacity and final grain weight (Zhang et al., 2007b; Chen et al., 2014). Solar radiation is the energy source for photoassimilates (Shi et al., 2013), which are the ultimate driving factor of maize development and growth (Hou et al., 2021). Previous studies concluded that plant growth was more severely inhibited by low light as the level of shading increased (Bidhari et al., 2021). The present study supported those findings; we here found that shading negatively affected yield (Table 2), final grain weight, grain filling parameters (Table 3), and Pn (Figure 1), with higher levels of shade having greater effects. Other researchers have shown that the correlative coefficient of Gave and Gmax to 100-grain weight is highest in the late grain filling stage (Zhang et al., 2007a; Liu et al., 2013). Additionally, Zhang et al. (2007b) reported that this change could be a result of photosynthesis during grain filling. The results of the present study are consistent with that report, showing that Pn decreased significantly in all three cultivars in response to shading (Figure 1), which may be the underlying cause of the decrease in final grain weight. It has also previously been reported that decreasing both photosynthesis and the activity of key starch synthesis enzymes limits photosynthesis (Lu et al., 2013; Rivera-Amado et al., 2020; Feng et al., 2021) and caused decreases in transportation and distribution of photosynthates from leaves to grains (Zhang et al., 2008; Kromdijk et al., 2016; Gao et al., 2020; Feng et al., 2021). In addition, temperature condition during grain filling is one of the most important climatic drivers that determine the rate of crop development and biomass accumulation (Borrás et al., 2009; Kromdijk and Long, 2016; Hou et al., 2021). Previous studies have shown maize photosynthesis and grain filling rate are limited by temperature (Jia et al., 2018). However, there are many studies reported that there were no significant changes in the relative humidity and temperature at different positions inside and outside the shade shelter, after shading treatments (Zhang et al., 2007b; Feng et al., 2021). Similarly, in this study, a distance of 1.5 m was maintained between the top of the maize canopy and the shade nets to keep microclimate conditions consistent with the unshaded treatment. But for S3 treatment, the temperature might be decreased after shading which together with decreased light affected the growth of maize. This needs further research in the future.
A quantitative understanding of the factors driving changes in grain filling is essential to developing agricultural information technology, crop growth models, and crop options for adaptation to climate change (Hou et al., 2021; Liu et al., 2021a; Tao et al., 2022). There is a wide geographical range in which Chinese maize is grown; one of large differences between regions is the level of solar radiation (Liu et al., 2021a). Xinjiang is in Northwest China and has the most abundant light radiation (Xue et al., 2016; Yang et al., 2020). Shading treatments in this region can simulate the light environment in other regions and allow quantitative analysis of the relationship between maize growth and solar radiation. We here confirmed the quantitative relationships between maize grain filling parameters and PAR using different shading treatments and planting densities (Figure 2), which had not previously been widely reported (Chen et al., 2014). The study design allowed us to establish the relationship between relative changes in PAR and maize yield/Gave (Figure 3). These data can be used to estimate the yield and Gave of maize in different years or regions with varying levels of solar radiation, and contribute to the improvement of crop models.
Grain filling rate is affected by both genotype and environmental conditions, and light stress responses vary by genotype (Li et al., 2005). However, Rizzo et al. (2022) concluded that genetic improvement accounted for only 13% of yield increase, and that if genetic progress in yield potential was also slowing in other environments and crops, future crop-yield gains would increasingly rely on improved agronomic practices. Additionally, Li and Wang (2009) reported that breeding cultivars tolerant to high planting density and other adverse conditions would be the most effective cultivation measure for improving maize grain yield. Some studies also stated that as planting density increases the number of grains per ear decreases, and the maximum and average maize grain-filling rate significantly decrease (Jia et al., 2018). Previous studies also showed that the photosynthate produced under high density and weak light could not satisfy yield formation (Yang et al., 2021a). Besides, shading delayed vegetative and reproductive growth, and reduced the kernel number per ear and kernel weight because of the limited assimilates supply to the developing ear shoot (Cui et al., 2015; Yang et al., 2021b). In this study, shading had greater effects on Gave and Wmax at low planting density (D1), but smaller effects at high planting density (D2) which might be due to that Gave and Wmax under CK were much higher at low density than that at high density. In other words, under the higher planting density of D2, self-shading was more prominent by denser canopies and increased the proportion of partially shaded leaves (Kromdijk et al., 2016), therefore the shading treatments were less consequential. Moreover, the average yield and grain weight of DH618 plants were generally higher compared to the other two cultivars (Tables 2, 3). This may be because Gave and other grain filling parameters were higher in cultivar DH618 than the other two cultivars after shading (Table 3). Therefore, we think that cultivars DH618 was a kind of suitable for dense planting under insufficient light conditions (Table 2). In addition, the decreases in grain filling parameters were smaller in DH618 than in XY335 and ZD958 after shading. This could be because cultivar DH618 is an erect-type hybrid, which can intercept as much solar radiation as possible (Liu et al., 2021a).
Understanding the quantitative relationship between solar radiation and grain filling parameters of maize is essential for improving maize production and developing options for adaptation to climate change. In this study, it was found that there were significant differences in maize final grain weight and other grain filling parameters in response to shading treatments. Quantitative relationships were discovered between PAR and grain yield, Gave, Gmax, Tmax, and Wmax. DH618 had better grain filling ability and tolerance to high planting density than the other two cultivars under weak light conditions. This type of cultivar should be selected and bred for low light adaptation to achieve high grain yield in the face of climate change, particularly the decrease in solar radiation.
The original contributions presented in the study are included in the article/supplementary material, further inquiries can be directed to the corresponding authors.
YY carried out the measurements and data analysis and wrote the manuscript. YY, PH, and SL designed the experiment. YY, GL, XG, and WL performed the study. YY, GL, XG, WL, JX, BM, RX, KW, PH, and SL made substantial contributions to conception and critically revised the manuscript. All authors contributed to the article and approved the submitted version.
This study was supported by the National Natural Science Foundation of China (31871558 and 32172118), the National Key Research and Development Program of China (2016YFD0300110 and 2016YFD0300101), and the China Agriculture Research System of MOF and MARA.
The authors declare that the research was conducted in the absence of any commercial or financial relationships that could be construed as a potential conflict of interest.
All claims expressed in this article are solely those of the authors and do not necessarily represent those of their affiliated organizations, or those of the publisher, the editors and the reviewers. Any product that may be evaluated in this article, or claim that may be made by its manufacturer, is not guaranteed or endorsed by the publisher.
We are grateful to the reviewers for helping us to improve the original manuscript.
Ahmad, S., Wang, G. Y., Muhammad, I., Farooq, S., Kamran, M., Ahmad, I., et al. (2022). Application of melatonin-mediated modulation of drought tolerance by regulating photosynthetic efficiency, chloroplast ultrastructure, and endogenous hormones in maize. Chem. Biol. Technol. Agric. 9:5. doi: 10.1186/s40538-021-00272-1
Arisnabarreta, S., and Miralles, D. J. (2008). Radiation effects on potential number of grains per spike and biomass partitioning in two- and six-rowed near isogenic barley lines. Field Crop Res. 107, 203–210. doi: 10.1016/j.fcr.2008.01.005
Barnett, K. H., and Pearce, R. B. (1983). Source-sink ratio alteration and its effect on physiological parameters in maize. Crop Sci. 23, 294–299. doi: 10.2135/cropsci1983.0011183X002300020028x
Bidhari, L. A., Effendi, R., Andayani, N. N., and Bambang, S. (2021). Screening of shade tolerant hybrid maize based on stress tolerance index. IOP Conf. Ser. Earth Environ. Sci. 911:12018. doi: 10.1088/1755-1315/911/1/012018
Borrás, L., Zinselmeier, C., Senior, M. L., Westgate, M. E., and Muszynski, M. G. (2009). Characterization of grain-filling patterns in diverse maize germplasm. Crop Sci. 49, 999–1009. doi: 10.2135/cropsci2008.08.0475
Cao, Y. X., Cai, H. J., Sun, S. K., Gu, X. B., Mu, Q., Duan, W. N., et al. (2022). Effects of drip irrigation methods on yield and water productivity of maize in Northwest China. Agric. Water Manag. 259:107227. doi: 10.1016/j.agwat.2021.107227
Chen, C. Y., Wang, R. H., Zhao, J. R., Xu, T. J., Wang, Y. D., Liu, X. Z., et al. (2014). Effects of shading on grain-filling properties and yield of maize at different growth stages. Acta Agron. Sin. 40, 1650–1657. doi: 10.3724/sp.J.1006.2014.01650
Cui, H. Y., Camberato, J. J., Jin, L. B., and Zhang, J. W. (2015). Effects of shading on spike differentiation and grain yield formation of summer maize in the field. Int. J. Biometeorol. 59, 1189–1200. doi: 10.1007/s00484-014-0930-5
Feng, Y., Cui, X., Shan, H., Shi, Z. S., Li, F. H., Wang, H. W., et al. (2021). Effects of solar radiation on photosynthetic physiology of barren stalk differentiation in maize. Plant Sci. 312:111046. doi: 10.1016/j.plantsci.2021.111046
Gao, Z., Liang, X. G., Zhang, L., Lin, S., Zhao, X., Zhou, L. L., et al. (2017b). Spraying exogenous 6-benzyladenine and brassinolide at tasseling increases maize yield by enhancing source and sink capacity. Field Crop Res. 211, 1–9. doi: 10.1016/j.fcr.2017.05.027
Gao, J., Liu, Z., Zhao, B., Dong, S. T., Liu, P., and Zhang, J. W. (2020). Shade stress decreased maize grain yield, dry matter, and nitrogen accumulation. Agron. J. 112, 2768–2776. doi: 10.1002/agj2.20140
Gao, J., Zhao, B., Dong, S. T., Liu, P., Ren, B. C., and Zhang, J. W. (2017a). Response of summer maize photosynthate accumulation and distribution to shading stress assessed by using 13CO2 stable isotope tracer in the field. Front. Plant Sci. 8:1821. doi: 10.3389/fpls.2017.01821
Hou, P., Gao, Q., Xie, R. Z., Li, S. K., Meng, Q. F., Kirkby, E. A., et al. (2012). Grain yields in relation to N requirement: optimizing nitrogen management for spring maize grown in China. Field Crop Res. 129, 1–6. doi: 10.1016/j.fcr.2012.01.006
Hou, P., Liu, Y. E., Liu, W. M., Liu, G. Z., Xie, R. Z., Wang, K. R., et al. (2020). How to increase maize production without extra nitrogen input. Resour. Conserv. Recycl. 160:104913. doi: 10.1016/j.resconrec.2020.104913
Hou, P., Liu, Y. E., Liu, W. M., Yang, H., Xie, R. Z., Wang, K. R., et al. (2021). Quantifying maize grain yield losses caused by climate change based on extensive field data across China. Resour. Conserv. Recycl. 174:105811. doi: 10.1016/j.resconrec.2021.105811
Jia, S. F., Dong, S. T., Wang, K. J., Wang, Z. J., and Liu, P. (2007). Effects of light stress on grain yield and potosynthetic traits of maize. Chin. J. Appl. Ecol. 18, 2456–2461. doi: 10.13287/j.1001-9332.2007.0409
Jia, Q. M., Sun, L. F., Mou, H. Y., Ali, S., Liu, D. H., Zhang, Y., et al. (2018). Effects of planting patterns and sowing densities on grain-filling, radiation use efficiency and yield of maize (Zea mays L.) in semi-arid regions. Agric. Water Manag. 201, 287–298. doi: 10.1016/j.agwat.2017.11.025
Johnson, D. R., and Tanner, J. W. (1972). Calculation of the rate and duration of grain filling in corn (Zea mays L.). Crop Sci. 12, 485–486. doi: 10.2135/cropsci1972.0011183X001200040028x
Kromdijk, J., Glowacka, K., Leonelli, L., Gabilly, S. T., Iwai, M., Niyogi, K. K., et al. (2016). Improving photosynthesis and crop productivity by accelerating recovery from photoprotection. Science 354, 857–861. doi: 10.1126/science.aai8878
Kromdijk, J., and Long, S. P. (2016). One crop breeding cycle from starvation? How engineering crop photosynthesis for rising CO2 and temperature could be one important route to alleviation. Proc. R. Soc. B 283:20152578. doi: 10.1098/rspb.2015.257
Li, Q., Du, L. J., Feng, D. J., Ren, Y., Li, Z. X., Kong, F. L., et al. (2020). Grain-filling characteristics and yield differences of maize cultivars with contrasting nitrogen efficiencies. Crop J. 8, 990–1001. doi: 10.1016/j.cj.2020.04.001
Li, C. H., Luan, L. M., Yin, F., Wang, Q., and Zhao, Y. L. (2005). Effects of light stress at different stages on the growth and yield of different maize genotypes (Zea mays L.). Acta Ecol. Sin. 25, 824–830. doi: 10.3321/j.issn:1000-0933.2005.04.025
Li, S. K., and Wang, C. T. (2009). Evolution and development of maize production techniques in China. Sci. Agric. Sin. 42, 1941–1951. doi: 10.3864/j.issn.0578-1752.2009.06.009
Liu, G. Z., Hou, P., Xie, R. Z., Ming, B., Wang, K. R., Liu, W. M., et al. (2019). Nitrogen uptake and response to radiation distribution in the canopy of high-yield maize. Crop Sci. 59, 1236–1247. doi: 10.2135/cropsci2018.09.0567
Liu, G. Z., Liu, W. M., Hou, P., Ming, B., Yang, Y. S., Guo, X. X., et al. (2021a). Reducing maize yield gap by matching plant density and solar radiation. J. Integr. Agric. 20, 363–370. doi: 10.1016/s2095-3119(20)63363-9
Liu, W. M., Liu, Y. E., Liu, G. Z., Xie, R. Z., Ming, B., Yang, Y. S., et al. (2022). Estimation of maize straw production and appropriate straw return rate in China. Agric. Ecosyst. Environ. 328:107865. doi: 10.1016/j.agee.2022.107865
Liu, W. M., Liu, G. Z., Yang, Y. S., Guo, X. X., Ming, B., Xie, R. Z., et al. (2021c). Spatial variation of maize height morphological traits for the same cultivars at a large agroecological scale. Eur. J. Agron. 130:126349. doi: 10.1016/j.eja.2021.126349
Liu, M., Qi, H., Zhang, W. J., Zhang, Z. P., Li, X. F., Song, Z. W., et al. (2013). Effects of deep loosening and nitrogen application methods on grain filling, yield and quality of spring maize. J. Maize Sci. 21, 115–119. doi: 10.3969/j.issn.1005-0906.2013.03.022
Liu, Z. H., Yang, J., Chang, Y., Weisberg, P. J., and He, H. S. (2012). Spatial patterns and drivers of fire occurrence and its future trend under climate change in a boreal forest of Northeast China. Glob. Chang. Biol. 18, 2041–2056. doi: 10.1111/j.1365-2486.2012.02649.x
Liu, Z. J., Yang, X. G., Lin, X. M., Zhang, Z. T., Sun, S., and Ye, Q. (2021d). From dimming to brightening during 1961 to 2014 in the maize growing season of China. Food Energy Secur. 10, 329–340. doi: 10.1002/fes3.275
Liu, G. Z., Yang, H. S., Xie, R. Z., Yang, Y. S., Liu, W. M., Guo, X. X., et al. (2021b). Genetic gains in maize yield and related traits for high-yielding cultivars released during 1980s to 2010s in China. Field Crop Res. 270:108223. doi: 10.1016/j.fcr.2021.108223
Liu, Z. H., and Zhang, Z. H. (2010). Research progress on grain filling rate in maize. J. Northeast. Agric. Univ. 41, 148–153. doi: 10.19720/j.cnki.issn.1005-9369.2010.11.028
Lu, D. L., Sun, X. L., Wang, X., Yan, F. B., and Lu, W. P. (2013). Effect of shading during grain filling on the physicochemical properties of fresh waxy maize. J. Integr. Agric. 12, 1560–1567. doi: 10.1016/s2095-3119(13)60560-2
Ottaviano, E., and Camussi, A. (1981). Phenotypic and genetic relationships between yield components in maize. Euphytica 30, 601–609. doi: 10.1007/BF00038787
Poonam, Y., Kalidindi, U., and Bhupinder, S. (2022). “Air pollution mitigation and global dimming: a challenge to agriculture under changing climate,” in Climate Change and Crop Stress. eds. A. K. Shanker, C. Shanker, A. Anand, and M. Maheswari (Cambridge: Academic Press).
Ren, B. Z., Cui, H. Y., Camberato, J. J., Dong, S. T., Liu, P., Zhao, B., et al. (2016). Effects of shading on the photosynthetic characteristics and mesophyll cell ultrastructure of summer maize. Sci. Nat. 103:67. doi: 10.1007/s00114-016-1392-x
Rivera-Amado, C., Molero, G., Trujillo-Negrellos, E., Reynolds, M., and Foulkes, J. (2020). Estimating organ contribution to grain filling and potential for source upregulation in wheat cultivars with a contrasting source–sink balance. Agronomy 10:1527. doi: 10.3390/agronomy10101527
Rizzo, G., Monzon, J. P., Tenorio, F. A., Howard, R., Cassman, K. G., and Grassini, P. (2022). Climate and agronomy, not genetics, underpin recent maize yield gains in favorable environments. Proc. Natl. Acad. Sci. U. S. A. 119:e2113629119. doi: 10.1073/pnas.2113629119
Sandaña, P. A., Harcha, C. I., and Calderini, D. F. (2009). Sensitivity of yield and grain nitrogen concentration of wheat, lupin and pea to source reduction during grain filling. A comparative survey under high yielding conditions. Field Crop Res. 114, 233–243. doi: 10.1016/j.fcr.2009.08.003
Shao, L., Liu, Z., Li, H., Zhang, Y., Dong, M., Guo, X., et al. (2020). The impact of global dimming on crop yields is determined by the source-sink imbalance of carbon during grain filling. Glob. Chang. Biol. 27, 689–708. doi: 10.1111/gcb.15453
Shi, J. G., Cui, H. Y., Zhao, B., Dong, S. T., Liu, P., and Zhang, J. W. (2013). Effect of light on yield and characteristics of grain-filling of summer maize from flowering to maturity. Sci. Agric. Sin. 46, 4427–4434. doi: 10.3864/j.issn.0578-1752.2013.21.004
Shi, K., Gu, X. T., Lu, W. P., and Lu, D. L. (2018). Effects of weak-light stress during grain filling on the physicochemical properties of normal maize starch. Carbohydr. Polym. 202, 47–55. doi: 10.1016/j.carbpol.2018.08.114
Tao, F. L., Zhang, L. L., Zhang, Z., and Chen, Y. (2022). Climate warming outweighed agricultural managements in affecting wheat phenology across China during 1981–2018. Agric. For. Meteorol. 316:108865. doi: 10.1016/j.agrformet.2022.108865
Wang, J., Shi, K., Lu, W., and Lu, D. J. P. (2020a). Post-silking shading stress affects leaf nitrogen metabolism of spring maize in southern China. Plan. Theory 9:210. doi: 10.3390/plants9020210
Wang, Y., Zhang, Z. K., Liang, Y. Y., Han, Y. L., Han, Y. L., and Tan, J. F. (2020b). High potassium application rate increased grain yield of shading-stressed winter wheat by improving photosynthesis and photosynthate translocation. Front. Plant Sci. 11:134. doi: 10.3389/fpls.2020.00134
Wu, P., Liu, F., Chen, G., Wang, J., Huang, F., Cai, T., et al. (2022). Can deep fertilizer application enhance maize productivity by delaying leaf senescence and decreasing nitrate residue levels? Field Crop Res. 277:108417. doi: 10.1016/j.fcr.2021.108417
Xu, T. J., Lyu, T. F., Zhao, J. R., Wang, R. H., Chen, C. Y., Liu, Y. E., et al. (2018). Photosynthetic characteristics, dry matter accumulation and translocation, grain filling parameter of three main maize varieties in production. Acta Agron. Sin. 44, 414–422. doi: 10.3724/sp.J.1006.2018.00414
Xue, J., Gou, L., Zhao, Y. S., Yao, M. N., Yao, H. H., Tian, J. S., et al. (2016). Effects of light intensity within the canopy on maize lodging. Field Crop Res. 188, 133–141. doi: 10.1016/j.fcr.2016.01.003
Yang, Y. S., Guo, X. X., Hou, P., Xue, J., Liu, G. Z., Liu, W. M., et al. (2020). Quantitative effects of solar radiation on maize lodging resistance mechanical properties. Field Crop Res. 255:107906. doi: 10.1016/j.fcr.2020.107906
Yang, Y. S., Guo, X. X., Liu, H. F., Liu, G. Z., Liu, W. M., Ming, B., et al. (2021b). The effect of solar radiation change on the maize yield gap from the perspectives of dry matter accumulation and distribution. J. Integr. Agric. 20, 482–493. doi: 10.1016/s2095-3119(20)63581-x
Yang, Y. S., Guo, X. X., Liu, G. Z., Liu, W. M., Xue, J., Ming, B., et al. (2021a). Solar radiation effects on dry matter accumulations and transfer in maize. Front. Plant Sci. 12:727134. doi: 10.3389/fpls.2021.727134
Zhang, H. Y., Dong, S. T., and Gao, R. Q. (2007a). Analysis on kernel filling characteristics for different maize types. J. Maize Sci. 15, 67–70. doi: 10.13597/j.cnki.maize.science.2007.03.016
Zhang, J. W., Dong, S. T., Wang, K. J., Hu, C. H., and Liu, P. (2007b). Effects of shading in field on photosynthetic characteristics in summer corn. Acta Agron. Sin. 29, 883–890. doi: 10.13287/j.1001-9332.201803.021
Zhang, J. W., Dong, S. T., Wang, K. J., Hu, C. H., and Liu, P. (2008). Effects of shading in field on key enzymes involved in starch synthesis of summer maize. Acta Agron. Sin. 34, 1470–1474. doi: 10.3724/sp.j.1006.2008.01470
Zhang, G. Q., Liu, C. W., Xiao, C. H., Xie, R. Z., Ming, B., Hou, P., et al. (2017). Optimizing water use efficiency and economic return of super high yield spring maize under drip irrigation and plastic mulching in arid areas of China. Field Crop Res. 211, 137–146. doi: 10.1016/j.fcr.2017.05.026
Zhang, J. W., Zhang, Y. Y., Song, N. Y., Chen, Q. L., Sun, H. Z., Peng, T., et al. (2021). Response of grain-filling rate and grain quality of mid-season indica rice to nitrogen application. J. Integr. Agric. 20, 1465–1473. doi: 10.1016/S2095-3119(20)63311-1
Zhang, Q., Zheng, Y. X., Tong, D., Shao, M., Wang, S. X., Zhang, Y. H., et al. (2019). Drivers of improved PM2.5 air quality in China from 2013 to 2017. Proc. Natl. Acad. Sci. U. S. A. 116, 24463–24469. doi: 10.1073/pnas.1907956116
Zhou, X. D. (2004). Grain capablilty of photosynthates and its relationship with hull in rice (Oryza sativa L.). [dissertation’s thesis]. [Yangzhou (JiangSu)]: YangZhou University. Available at: http://cdmd.cnki.com.cn/Article/CDMD-11117-2004108867.htm
Keywords: maize, solar radiation, cultivar, grain filling rate, quantitative relationship
Citation: Yang Y, Liu G, Guo X, Liu W, Xue J, Ming B, Xie R, Wang K, Hou P and Li S (2022) Quantitative Relationship Between Solar Radiation and Grain Filling Parameters of Maize. Front. Plant Sci. 13:906060. doi: 10.3389/fpls.2022.906060
Received: 28 March 2022; Accepted: 24 May 2022;
Published: 10 June 2022.
Edited by:
Johannes Kromdijk, University of Cambridge, United KingdomReviewed by:
Patrick Morgan, Bayer Crop Science, United StatesCopyright © 2022 Yang, Liu, Guo, Liu, Xue, Ming, Xie, Wang, Hou and Li. This is an open-access article distributed under the terms of the Creative Commons Attribution License (CC BY). The use, distribution or reproduction in other forums is permitted, provided the original author(s) and the copyright owner(s) are credited and that the original publication in this journal is cited, in accordance with accepted academic practice. No use, distribution or reproduction is permitted which does not comply with these terms.
*Correspondence: Peng Hou, aG91cGVuZ0BjYWFzLmNu; Shaokun Li, bGlzaGFva3VuQGNhYXMuY24=
Disclaimer: All claims expressed in this article are solely those of the authors and do not necessarily represent those of their affiliated organizations, or those of the publisher, the editors and the reviewers. Any product that may be evaluated in this article or claim that may be made by its manufacturer is not guaranteed or endorsed by the publisher.
Research integrity at Frontiers
Learn more about the work of our research integrity team to safeguard the quality of each article we publish.