- 1School of Ecology and Nature Conservation, Beijing Forestry University, Beijing, China
- 2Donghu Experimental Station of Lake Ecosystems, State Key Laboratory of Freshwater Ecology and Biotechnology of China, Institute of Hydrobiology, Chinese Academy of Sciences, Wuhan, China
- 3College of Advanced Agricultural Sciences, University of Chinese Academy of Sciences, Beijing, China
The loss of submerged macrophytes from freshwater ecosystems is accelerating owing to the combined effects of eutrophication and climate change. Submerged macrophytes depend on spring clear water; however, increased water temperatures and excessive phosphorus (P) inputs often lead to the dominance of phytoplankton. It is still not clear how the stoichiometric characteristics of carbon (C), nitrogen (N), and P in different tissues of submerged macrophytes respond to P enrichment and temperature increases. In this study, we established 36 mesocosm ecosystems to explore the effects of warming and P addition on the leaf, turion, stem, and seed stoichiometry of Potamogeton crispus. The results revealed that different functional plant organs show distinct responses to P addition and warming, which demonstrates the importance of evaluating the responses of different submerged macrophyte organs to environmental changes. In addition, interactive effects between P addition and warming were observed in the leaf, turion, and seed C:N:P stoichiometry, which highlights the importance of multifactorial studies. Our data showed that warming caused a decrease in the C content in most organs, with the exception of the stem; P addition increased the P content in most organs, with the exception of seed; N content in the turion and seed were influenced by interactive effects. Collectively, P addition could help P. crispus to resist the adverse effects of high temperatures by aiding growth and asexual reproduction, and asexual propagules were found to be more sensitive to P enrichment than sexual propagules.
Introduction
Submerged macrophytes are the primary producers in lakes and play an important role in maintaining clean water (Su et al., 2017). However, globally, the loss of submerged aquatic vegetation is accelerating. A meta-analysis of 155 lakes found that, in 65.2% of the lakes, the aquatic vegetation cover decreased (Zhang et al., 2017). Eutrophication (Sondergaard et al., 2010) and climate change (Phillips et al., 2016) are the two main causes of the decline and disappearance of submerged macrophytes. Currently, eutrophication is accelerating, and eutrophication in lakes in mid- and low-latitude regions is more serious than that in lakes in high-latitude regions (Izmailova and Rumyantsev, 2016). The shallow lakes in the middle-lower reaches of the Yangtze River suffer from high-nutrient input from both inflows and sediments (Jin et al., 2005; Le et al., 2010; Qin et al., 2020). Shallow lakes with a large surface area to volume ratio and no thermal stratification are more sensitive to climate warming than other types of lakes (Gerten and Adrian, 2000; Mooij et al., 2005). The frequency and intensity of extreme climate events (such as heatwaves) are expected to increase over the next 100 years (Ferreira et al., 2010; Perkins et al., 2012). A regional model predicts that temperature will increase by 4.9 ± 0.95°C (RCP 8.5) over southern China by the century’s end, compared with the temperature from 1980 to 1999 (Chen and Frauenfeld, 2014a). Furthermore, the duration and intensity of heatwaves are predicted to increase significantly in the middle and lower reaches of the Yangtze River (Qi and Yang, 2019).
Carbon (C) is the most essential element that constitutes the dry matter in plants; nitrogen (N) determines plant growth by regulating the number and size of organs, and balancing nutrition and reproductive growth, whereas phosphorus (P) influences leaf formation and shape, as well as the plant’s flowering and seed formation (Plénet et al., 2000; Marschner, 2012). The N/C and P/C ratios determine the relative growth rate of plants (Ågren, 2004), and the N/P ratio reflects the restriction of plant growth (Wang et al., 2014). During plant growth, the C:N:P stoichiometry responds to environmental conditions (Zhang et al., 2013) and is related to important ecological processes, such as N2 fixation (Sañudo-Wilhelmy et al., 2001), litter decomposition (Güsewell and Gessner, 2009), species diversity (Ren et al., 2017; Chen and Chen, 2021), and the ability of organisms to adapt to environmental stress (Xie et al., 2018). Plant stoichiometry varies with the growth rate and the surrounding environment. Control experiments have shown that rising temperatures affect the growth, stoichiometry, and palatability of submerged macrophytes (Short et al., 2016; Zhang et al., 2019; Xu et al., 2020). Elevated temperatures lead to a decrease in N and P contents in aquatic plants, which results in an increase in C/N and C/P ratios. A study on terrestrial plants and phytoplankton showed that this effect was caused by an increase in nutrient use efficiency (An et al., 2005; De Senerpont Domis et al., 2014). The increase in temperature also changes the diffusion rate of nutrients in the water, owing to changes in the boundary layer surrounding organisms, which leads to changes in the stoichiometry of organisms (Raven and Geider, 1988). Studies have shown that warming may reduce the C/N ratio of terrestrial plants by increasing plant productivity, biological activity, and nutrient absorption (Welker et al., 2005; Aerts et al., 2009). However, in temperate terrestrial ecosystems, plant C/N and C/P ratios may increase (Sardans et al., 2012). The recognized importance of P as a limiting factor in terrestrial and aquatic ecosystems is increasing (Peñuelas et al., 2012). Nutrient addition can positively impact the nutritional quality of aquatic plants (Burkholder et al., 2007; Sardans et al., 2012; Bakker and Nolet, 2014). However, eutrophication can reduce the C/P ratio of algae in rivers (Frost and Elser, 2002; Dang et al., 2009), and low C/P ratios yield greater advantages to fast-growing species and disadvantages to slow-growing taxa (Frost and Elser, 2002), which may reduce ecosystem biodiversity (Evans-White et al., 2009). In addition, changes in the N/P ratio often favor algal species that can compete for restricted nutrients, which gives them a potential advantage (Granéli et al., 2008) and causes problems for the restoration of submerged macrophytes. In summary, these studies have found that temperature rise and nutrient enrichment have opposing effects on the stoichiometric characteristics of aquatic organisms. Temperature rise is expected to increase the ratio, whereas nutrient enrichment is expected to decrease the ratio. However, there are few reports about how the stoichiometry of submerged macrophytes responds to external environment change (Güsewell, 2004).
Potamogeton crispus is a perennial aquatic plant and the dominant species in freshwater areas of East Asia (Kunii, 1982; Xie et al., 2003). In particular, it is one of the few species that survives in nutrient-rich Chinese lakes (Lu et al., 2012). It has a strong resistance to stress brought on by pollution, and its ability to absorb nutrients is often used in wetland restoration (Jin et al., 1994; Xu et al., 2015). In the subtropical and temperate regions of the northern hemisphere, the life cycle of P. crispus differs from that of most submerged macrophytes. The optimum temperature range for P. crispus is 10–20°C, thus its biomass and productivity peak in the spring (Kunii, 1982). In summer, the leaves and stems rot and die, and propagule turions and seeds fall into the water body. P. crispus can reproduce either asexually or sexually (Shen et al., 2008). Turions formed at the top of the stem are important for the asexual reproduction of P. crispus. Seeds are produced at the same time as turions are formed (Sastroutomo et al., 1979; Rogers and Breen, 1980; Kunii, 1982), but the germination rate of P. crispus seeds is low under natural conditions. Therefore, P. crispus relies mainly on asexual reproduction, especially turion reproduction (Chen et al., 2006). P. crispus can grow well in water with a total nitrogen concentration of less than 2 mg/L and a total phosphorus concentration of less than 0.4 mg/L (Ma, 2007), but it cannot survive nutrient concentrations that are very high (Zhang, 2006). Currently, few studies have explored how nutrient increase and global warming, and their interactive effects, impact the C:N:P stoichiometric ratios of the different organs associated with plant growth and reproduction.
In this study, we used outdoor mesocosms to investigate the effects of warming and P enrichment on the C:N:P stoichiometric relationship within the organs of P. crispus. Our hypotheses are as follows: (1) the contents of C, N, and P in the organs of P. crispus will increase with additional P input, but the stoichiometric ratio will decrease; (2) P. crispus is not tolerant of high temperatures; thus, the contents of C, N, and P in the organs will decrease with increasing temperature, thereby increasing the stoichiometric ratio; (3) the stoichiometric characteristics of P. crispus’ organ responses vary under the interactive effects of warming and P input; and (4) seed stoichiometry is more stable than that of other organs because its function is to maintain the ability to reproduce in response to environmental changes.
Materials and Methods
Experiment Design
The outdoor mesocosm system was described in the study of Xu et al. (2020). The experiment was conducted from March 7 to May 30, 2018. Both water and plant samples were collected from Liangzi Lake. The treatments included two factors, temperature rise and P addition, with each treatment replicated six times. Tanks were randomly assigned to one of the six experiments, and the six treatments were divided into two categories: no phosphorus added and phosphorus added. The treatments were as follows: (1) no phosphorus added, which comprised natural water temperature with no added P (C); fixed heating with no added P (T), which comprised a constant increase in temperature that was 4°C above control conditions (in keeping with RCP 8.5 in the region by 2100) (Intergovernmental Panel on Climate Change (IPCC), 2013; Chen and Frauenfeld, 2014b); fluctuation of heating with no added P (V), where random temperature fluctuations between 0 and 8°C were applied based on the fixed heating group T. The total amount of warming over the duration of the experiment was equal for both temperature treatments (T and V). (2) Phosphorus added, which entailed P addition without warming (C + P) composed of the addition of 25 μg/L of KH2PO4 to the water every 2 weeks; fixed heating treatment and the P addition group (T + P), which comprised a constant increase in temperature that was 4°C above control conditions and the addition of 25 μg/L KH2PO4 every 2 weeks; fluctuation of heating and P addition (V + P), which had the same temperature setting as treatment V and had 25 μg/L KH2PO4 added every 2 weeks. The heating treatment began on the first day.
Sampling and Analysis
Water samples were collected from each tank using a Plexiglas tube (diameter 70 mm; length 1 m) and then taken to the laboratory to measure the total nitrogen (TN), total phosphorus (TP), and Chl-a in the water according to the standards of the Chinese water analysis methods (Wang et al., 2008). An ultraviolet spectrophotometer (Cleverchem380, DeChem-Tech., Germany) was used to calculate the TP and TN weekly. The chlorophyll-a concentration was determined by filtering 500 ml of the water using Whatman GF/C filters and then using a spectrophotometer (UV-2800, Unico, China) after ethanol extraction (Supplementary Table 1). P crispus stems and leaves were collected every month from each tank, whereas the turions and seeds were collected at the end of the experiment to analyze the C, N, and P contents. The plant samples were dried in an oven at 70°C for 48 h to a constant weight, after which the dried samples were ground into powder using a ball mill (Mini Beadbeater-16, Biospec product, United States). The C and N contents were analyzed using an elemental analyzer (FlashEA1112, CE instrument, Italy) (Bradshaw et al., 2012; Lü et al., 2012). The P content was analyzed via digested plant samples with H2SO4-H2O2 and molybdenum antimony spectrophotometry (Kuo, 1996).
Statistical Analysis
Two-way ANOVA was used to explore the effects of warming, P addition, and those of their interaction, on the TP, C/N, N/P, and C/P ratios of P. crispus turions, seeds, leaves, and stems using IBM SPSS Statistics 25 (SPSS, Chicago, Illinois, United States). The figures were drawn using Origin 2018 software (Origin Lab Corp., Massachusetts, United States).
Results
Effect of Warming and P Addition on the C, N, and P Contents and Stoichiometric Characteristics of Potamogeton crispus Leaves
The leaf C content was significantly influenced by warming (p = 0.001), P addition (p = 0.01), and their interactive effects (p = 0.008). Leaf C content decreased when temperature increased, and it also decreased when P addition, warming, and warming and P addition interacted (Table 1 and Figure 1A). The N content and C/N ratio in the leaf showed no response (p > 0.05) (Table 1 and Figures 1B,D). The P content of the leaves was significantly affected by warming and it significantly decreased when temperature increased (p = 0.007) (Table 1 and Figure 1C). Furthermore, the C/P ratio (p = 0.007) and N/P ratio (p = 0.002) in the leaf were significantly affected by warming, and both significantly increased as temperature increased (Table 1 and Figures 1E,F).
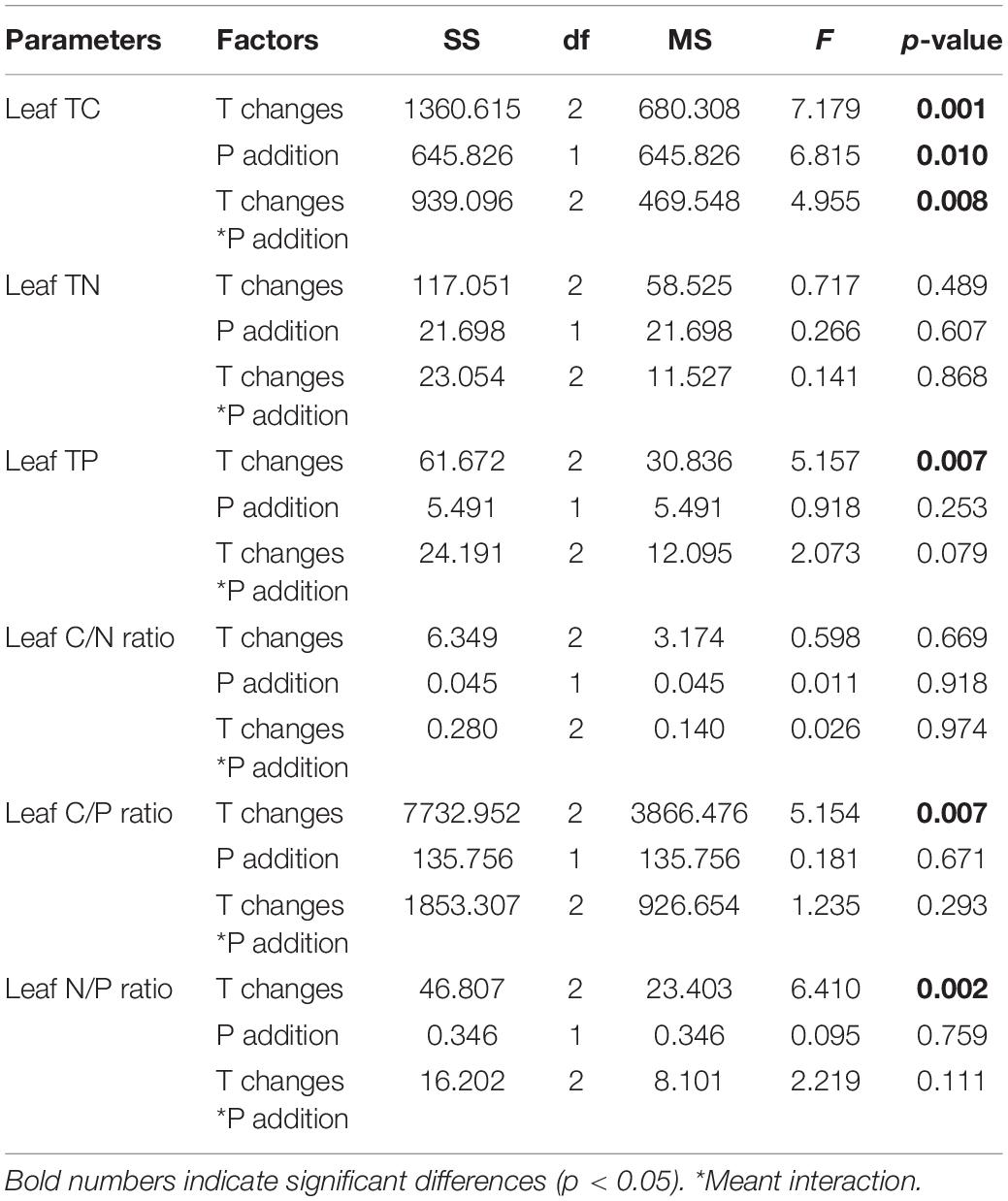
Table 1. Effects of different temperature scenarios and phosphorus addition on the TC, TN, and TP contents and the C/N, C/P, and N/P ratios in P. crispus leaves.
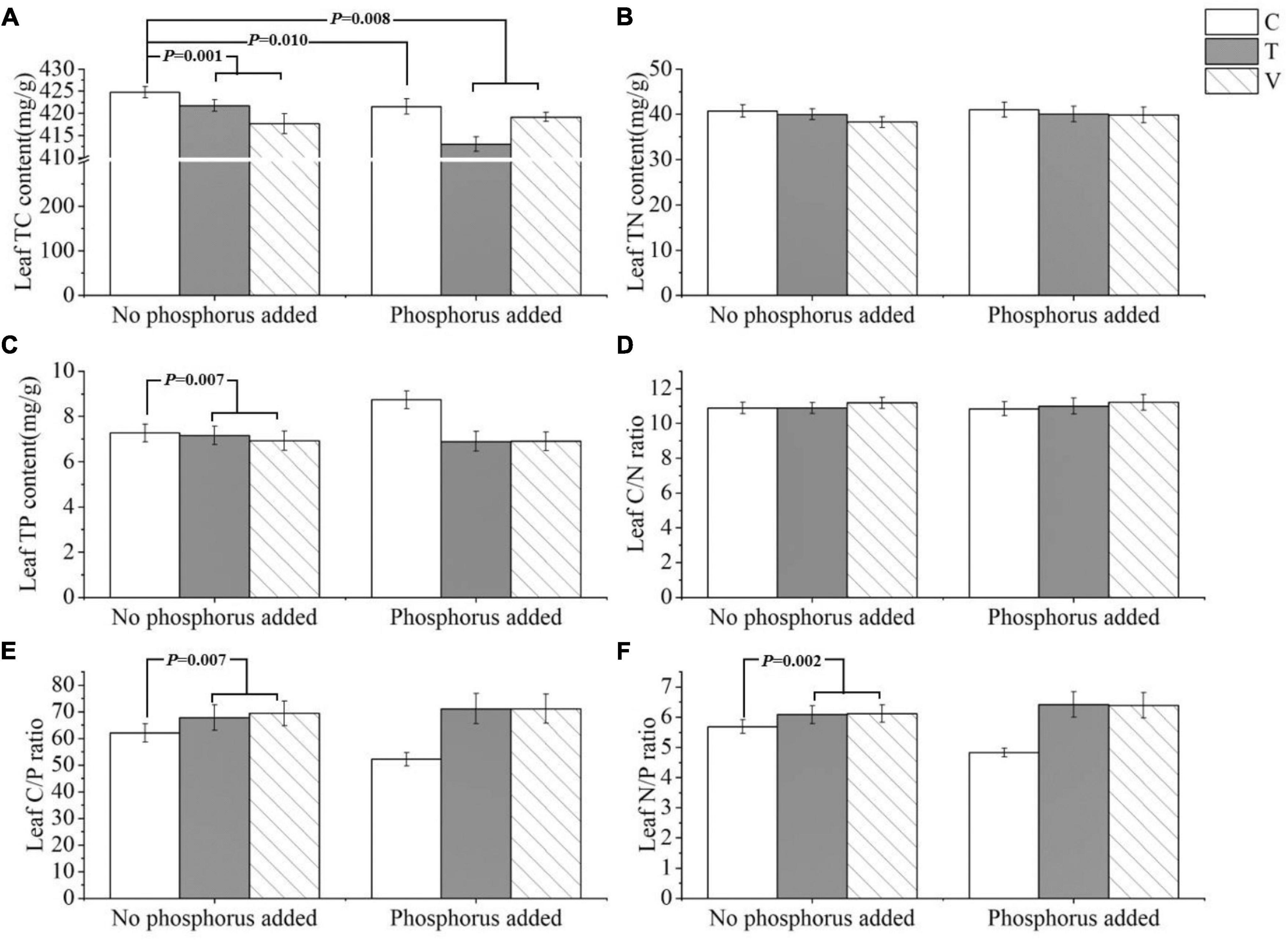
Figure 1. The stoichiometric characteristics of the (A) TC, (B) TN, and (C) TP contents and the (D) C/N, (E) C/P, and (F) N/P ratios of P. crispus leaves under different treatments. The C in “No phosphorus added” represent controls (C), T in “No phosphorus added” represent constant warming (T), and V in “No phosphorus added” represent variable warming (V). Meanwhile, the C in “Phosphorus Added” represent phosphorus addition (C + P), T + P in “Phosphorus Added” represent constant warming and phosphorus addition (T + P), and V + P in “Phosphorus Added” represent variable warming and phosphorus addition (V + P).
Effect of Warming and P Addition on the C, N, and P Contents and Stoichiometric Characteristics of Potamogeton crispus Stems
The C (p = 0.002) and P (p = 0.000) contents and C/P ratio (p = 0.000) in the stem of P. crispus varied significantly with P addition. Specifically, the C and P contents of the stems increased with P addition, and the C/P ratio decreased with P addition (Table 2 and Figures 2A,C,E), whereas the N content and C/N ratio showed no significant response (p > 0.05) (Table 2 and Figures 2B,D). The N/P ratio was significantly affected by warming and P addition, increasing significantly with warming (p = 0.041) and decreasing when P was added (p = 0.000) (Table 2 and Figure 2F).
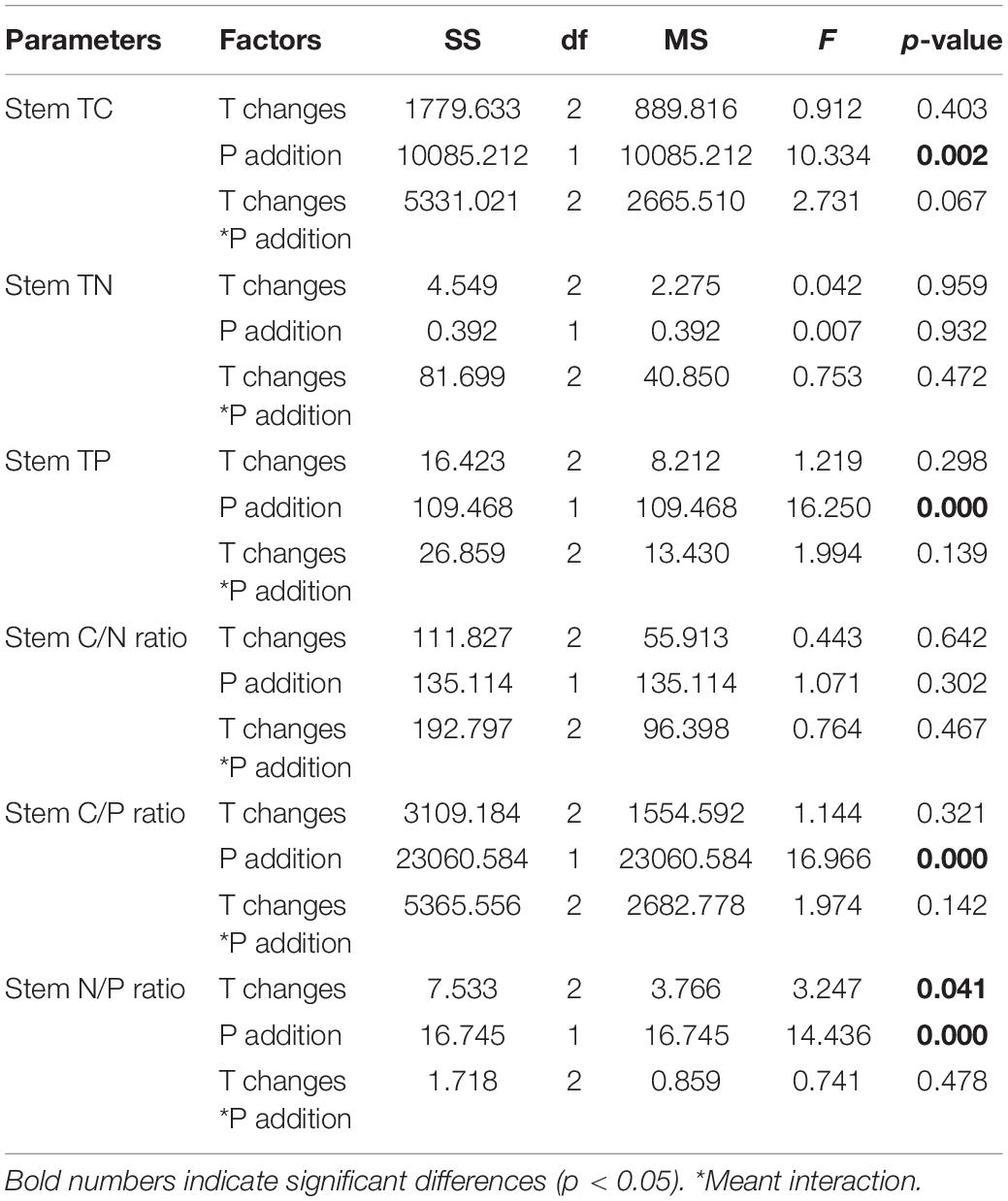
Table 2. Effects of different temperature scenarios and phosphorus addition on the TC, TN, and TP contents and the C/N, C/P, and N/P ratios in P. crispus stems.
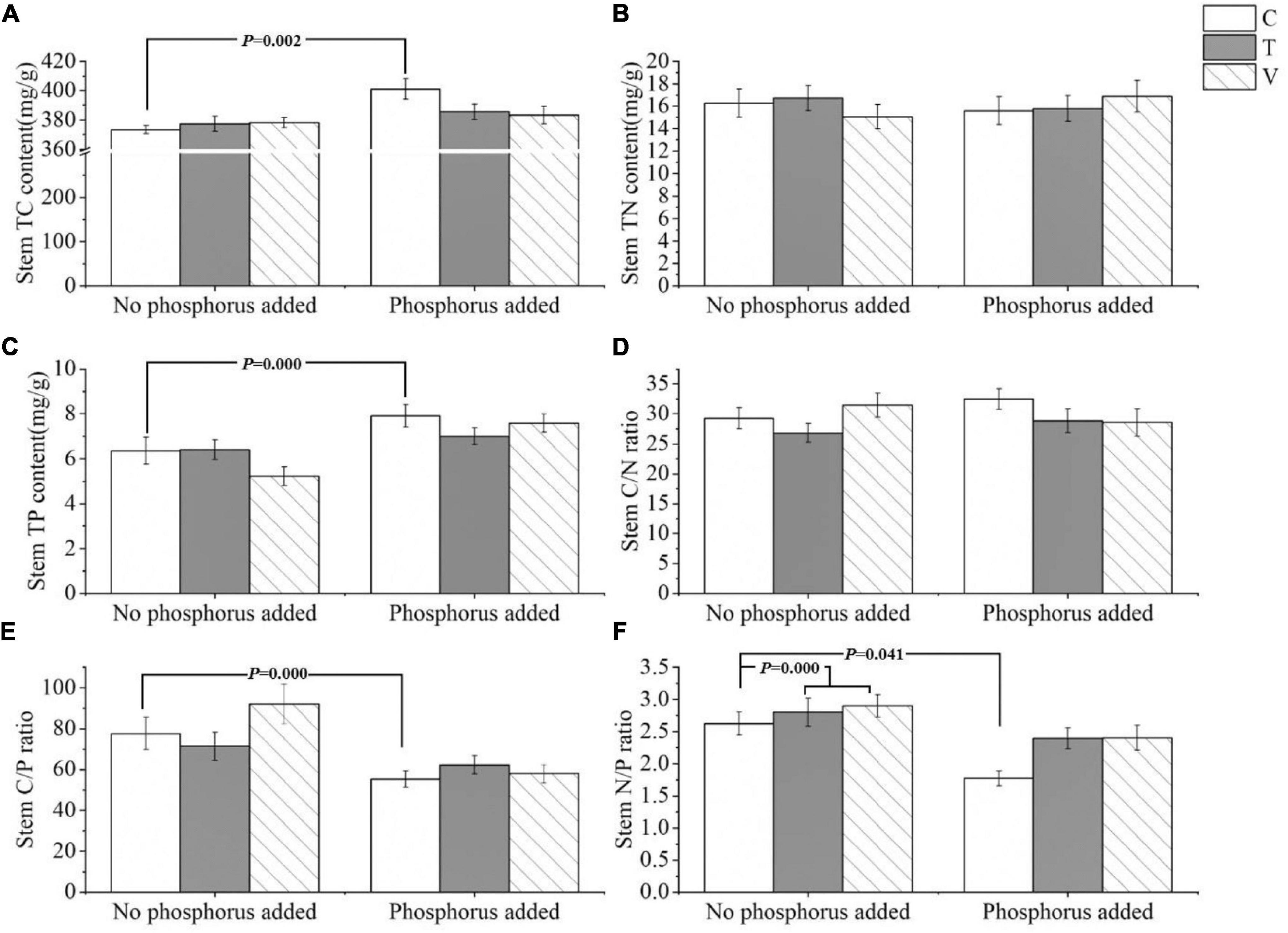
Figure 2. The stoichiometric characteristics of the (A) TC, (B) TN, and (C) TP contents and the (D) C/N, (E) C/P, and (F) N/P ratios of P. crispus stems under different treatments. The C in “No phosphorus added” represent controls (C), T in “No phosphorus added” represent constant warming (T), and V in “No phosphorus added” represent variable warming (V). Meanwhile, the C in “Phosphorus Added” represent phosphorus addition (C + P), T + P in “Phosphorus Added” represent constant warming and phosphorus addition (T + P), and V + P in “Phosphorus Added” represent variable warming and phosphorus addition (V + P).
Effect of Warming and P Addition on the C, N, P Contents and Stoichiometric Characteristics of Potamogeton crispus Turions
The C content of P. crispus turions was significantly affected by warming and when the temperature increased, the C content of P. crispus turions decreased significantly (p = 0.001) (Table 3 and Figure 3A). N content in the turions was significantly affected by warming (p = 0.036), P addition (p = 0.000), and their interactive effects (p = 0.006). Furthermore, when the temperature increased, the N content of the turions decreased significantly. The N content of the turions also decreased significantly with P addition, warming, and their interactive effects (Table 3 and Figure 3B). Turion P content was affected by P addition, warming (p = 0.000), and their interactions (p = 0.000). When only P was added, the turion P content decreased, and when P addition and warming interacted, the P content increased (Table 3 and Figure 3C). The turion C/N ratio significantly changed under warming (p = 0.017) and P addition (p = 0.000), both of which significantly increased (Table 3 and Figure 3D). Turion C/P and N/P ratios were significantly influenced by P addition (p = 0.000 and p = 0.000, respectively), and both significantly decreased with the interactive effects of warming and P addition (p = 0.000 and p = 0.007) (Table 3 and Figures 3E,F).
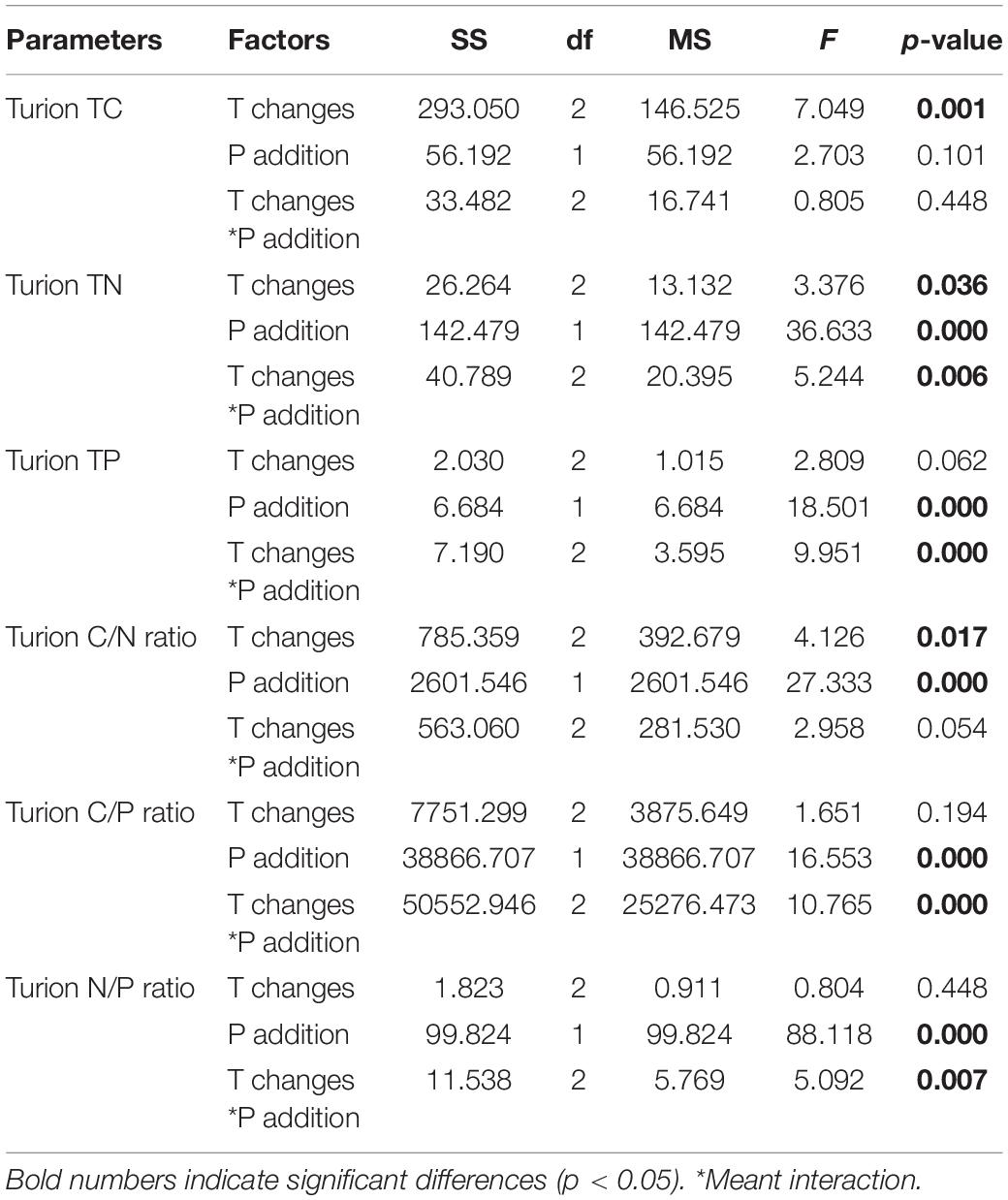
Table 3. Effects of different temperature scenarios and phosphorus addition on the TC, TN, and TP contents and the C/N, C/P, and N/P ratios in P.crispus turions.
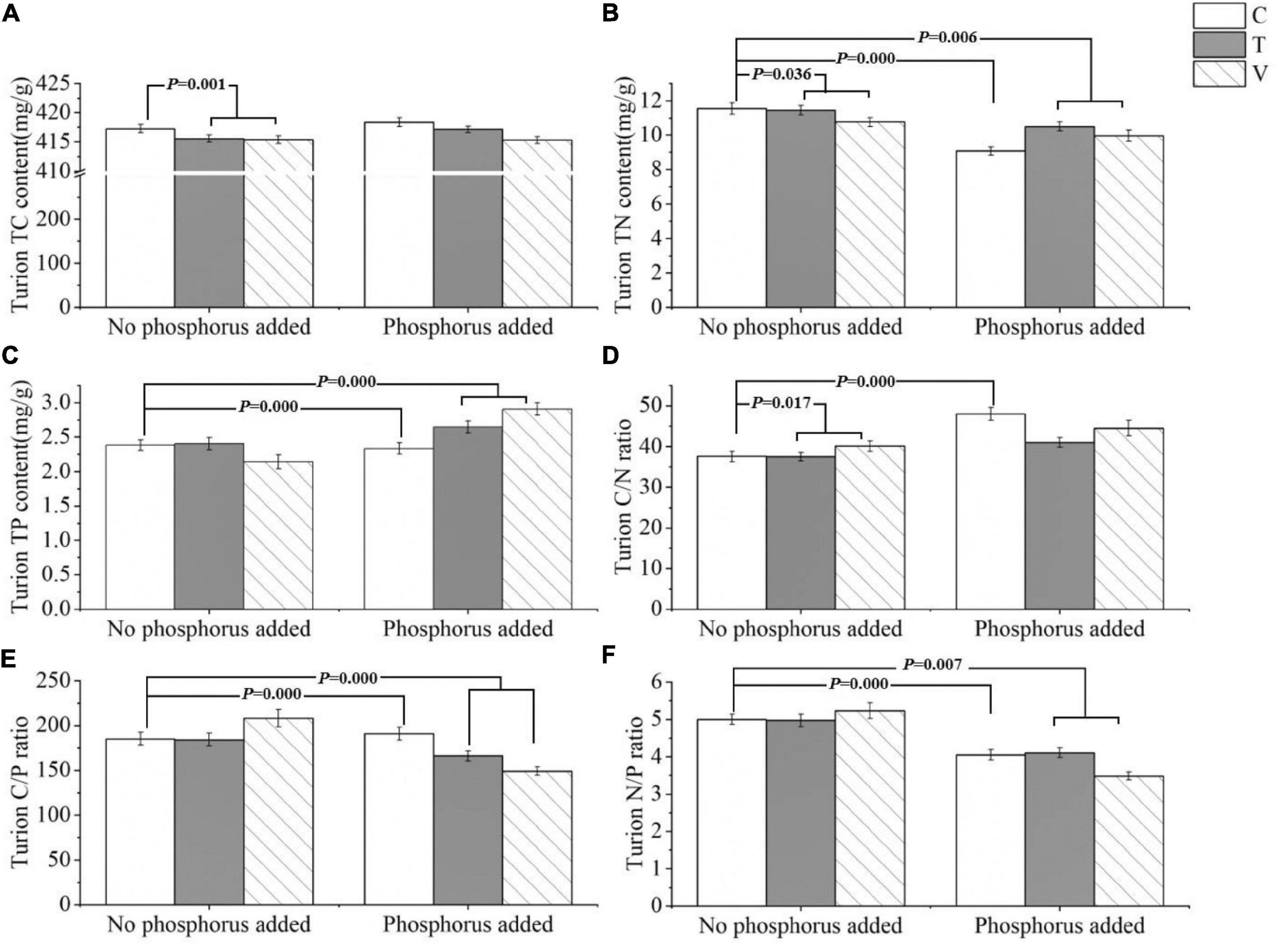
Figure 3. The stoichiometric characteristics of the (A) TC, (B) TN, and (C) TP contents and the (D) C/N, (E) C/P, and (F) N/P ratios of P. crispus turions under different treatments. The C in “No phosphorus added” represent controls (C), T in “No phosphorus added” represent constant warming (T), and V in “No phosphorus added” represent variable warming (V). Meanwhile, the C in “Phosphorus Added” represent phosphorus addition (C + P), T + P in “Phosphorus Added” represent constant warming and phosphorus addition (T + P), and V + P in “Phosphorus Added” represent variable warming and phosphorus addition (V + P).
Effect of Warming and P Addition on the C, N, P Contents and Stoichiometric Characteristics of Potamogeton crispus Seeds
The P. crispus seed C content was significantly affected by warming and P addition, and it decreased with increasing temperatures (p = 0.015) and P enrichment (p = 0.000) (Table 4 and Figure 4A). The N content (p = 0.035) and C/N ratio (p = 0.016) were significantly affected by the interaction between warming and P addition. Furthermore, the N content increased under the interaction between warming and P addition, whereas the C/N ratio decreased under this interaction (Table 4 and Figures 4B,D). The P content, C/P ratio, and N/P ratio showed no significant response (Table 4 and Figures 4C,E,F).
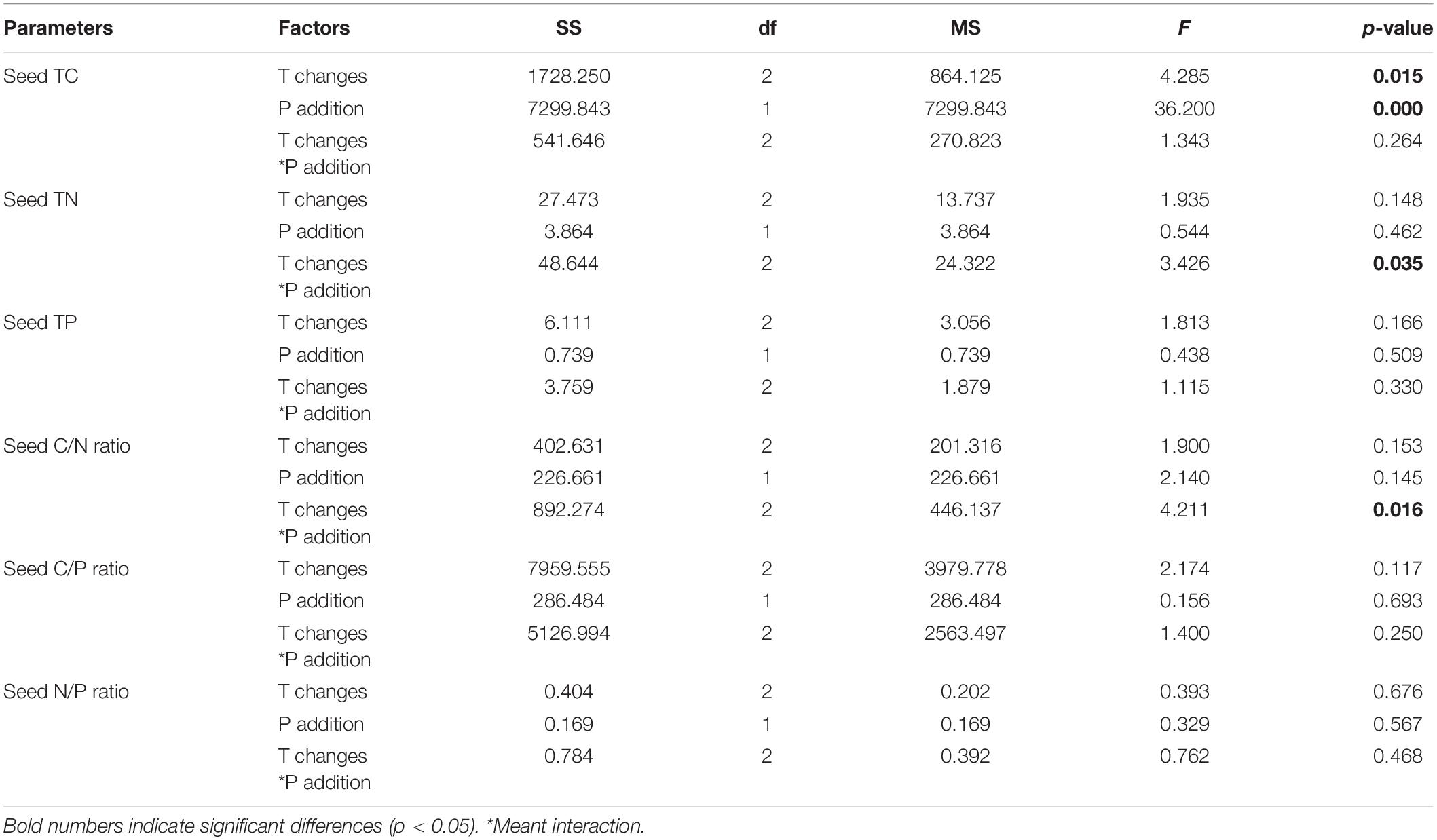
Table 4. Effects of different temperature scenarios and phosphorus addition on the TC, TN, and TP contents and the C/N, C/P, and N/P ratios in P. crispus seeds.
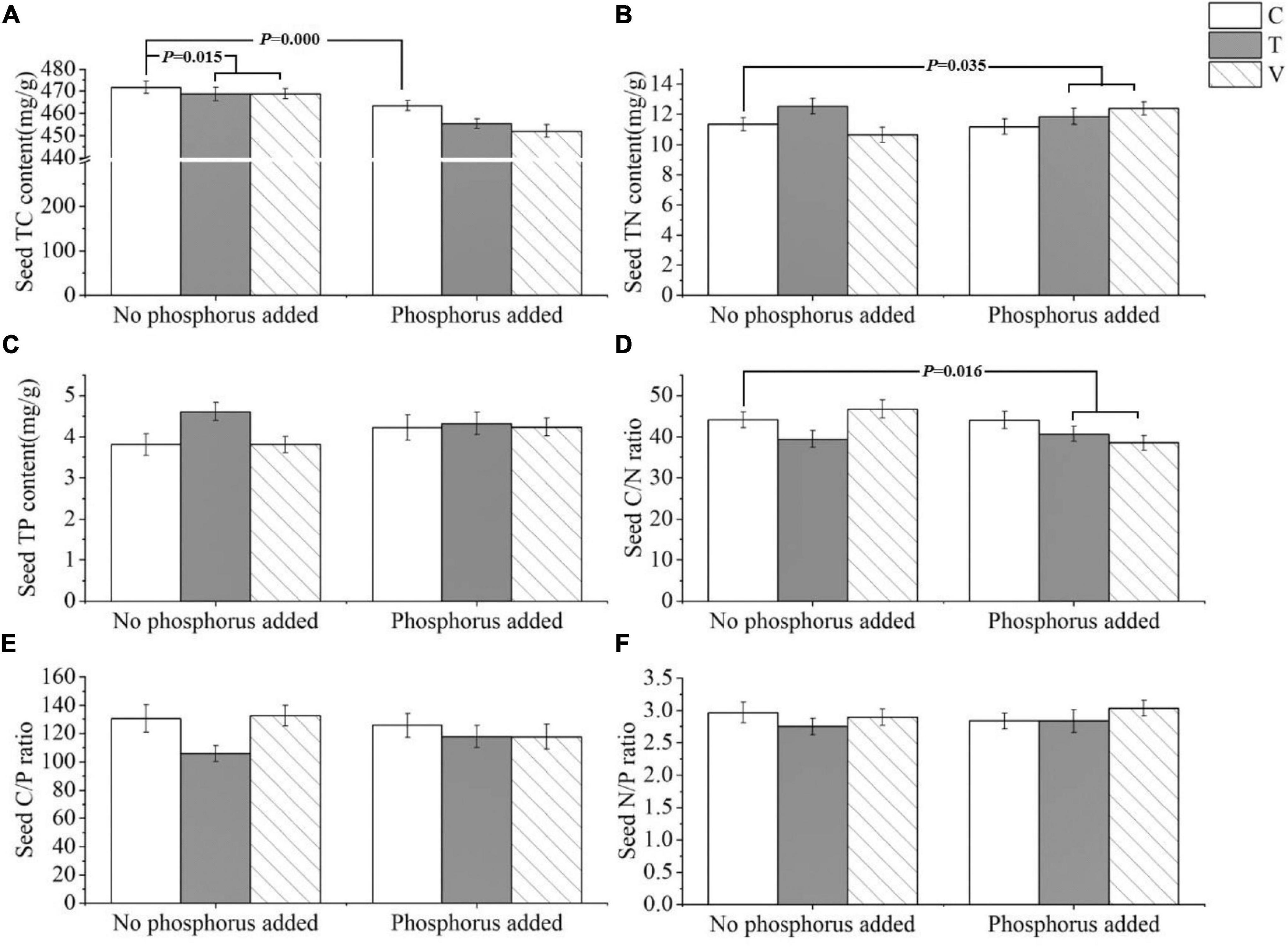
Figure 4. The stoichiometric characteristics of the (A) TC, (B) TN, and (C) TP contents and the (D) C/N, (E) C/P, and (F) N/P ratios of P. crispus seeds under different treatments. The C in “No phosphorus added” represent controls (C), T in “No phosphorus added” represent constant warming (T), and V in “No phosphorus added” represent variable warming (V). Meanwhile, the C in “Phosphorus Added” represent phosphorus addition (C + P), T + P in “Phosphorus Added” represent constant warming and phosphorus addition (T + P), and V + P in “Phosphorus Added” represent variable warming and phosphorus addition (V + P).
Discussion
The Response of Potamogeton crispus Turions and Seeds to Warming and P Addition
P. crispus practices both asexual and sexual reproduction and relies mainly on specialized turions for reproduction (Shen et al., 2008). Many researchers believe that low temperatures and sufficient light are beneficial for the germination of turions, whereas high temperatures inhibit their germination (Jian et al., 2003; Gao et al., 2005; Chen et al., 2006). The C and N contents in turions decreased slightly when the temperature increased, possibly because their growth was inhibited as the temperature increased, which results in a decrease in available inorganic C for turion sequestration. The N content of plants at high temperatures is usually related to respiration (Tjoelker et al., 1999; Crous et al., 2017). The previous studies have shown that an increase in C utilization leads to a decrease in the N content of submerged plants (Madsen et al., 1998; Dülger et al., 2017; Zhang et al., 2020). Our results are consistent with this observation because there is a negative covariance between plant C and N contents (Zhang et al., 2020), which indicates that these plants can self-regulate their internal nutritional composition. However, the N content decreased after P was added, as well as under the combined effects of P input and warming, which was inconsistent with our hypothesis. One possible explanation for this is that algal growth increases as the external nutrient load increases (Strecker et al., 2004; Daufresne et al., 2009) because algae may compete with submerged plants for inorganic C and light (Jones et al., 2002). Nitrogen is the basic component of all enzymes and chlorophyll in plants and plays a key role in controlling carbon assimilation and primary production (LeBauer and Treseder, 2008; Chen et al., 2013). As the C and N contents of the external nutrient load were low, the C and N contents of turions decreased after warming and the addition of P. Regarding the germination conditions of turions, their P content increased after the addition of P, which was consistent with our hypothesis. The addition of P led to a significant increase in the C/N ratio of the turions and a decrease in the C/P and N/P ratios. Under the interactive effects of warming and P enrichment, the C/P and N/P ratios decreased, which indicates that the utilization rate of P increased. The interactive effect of P addition and warming may increase the rate of P utilization by turions. Thus, warming may inhibit the growth of turions.
Carbon provides the foundation for growth, reproduction, and structure (Hessen et al., 2004; Elser et al., 2007). Under phosphorus addition, the decrease in seed C content may be caused by the distribution of more nutrients to the turions. Different C contents may be linked to the germination rate of the propagules. Whereas seeds, as sexual propagules, are important in long-distance transmission, the studies have shown that the germination rate of P. crispus seeds is extremely low, only 0.001% (Rogers and Breen, 1980); however, turions, the asexual propagules of P. crispus, are widely transplanted in artificial cultivation technology. The decrease in the C content after heating may have been caused by the high temperature. Other studies have demonstrated that temperature controls the germination rate and kinetics of charophytes, and that higher temperatures are not conducive to seed germination (Bonis and Grillas, 2002). Therefore, high temperatures can inhibit seed production. However, after the interaction of P and heating, the N content of the seeds increased and the C/N ratio decreased significantly. This suggests that when both factors act on the seeds simultaneously, the utilization efficiency of N may increase. These changes may further affect the germination rate and average germination time of propagules (Caliskan and Makineci, 2014), thereby affecting the distribution and abundance of the plant’s population. The stoichiometry of seeds was less sensitive to warming and P input and may be more stable than other organs because its function is to maintain the ability to reproduce in response to environmental changes.
The Response of Potamogeton crispus Leaves and Stems to Warming and P Input
P. crispus grows poorly under warmer conditions and most of them decay in the summer (Chen, 1985). The optimal temperature range for P. crispus is 10–12°C, and temperatures above and below this range affect its growth. Regarding the effects of temperature and nutrients on cold-water plants, the studies have shown that elevated temperatures deplete nutrient elements, thereby limiting the growth of P. crispus, and the effects on P. crispus stoichiometry are highly dependent on the nutrient conditions of the environment (Zhang et al., 2020). P. crispus grows faster in nutrient-rich sediments than in nutrient-poor sediments (Zhang et al., 2019). However, warming and nutrient addition may lead to increases in algae, turbidity, and total suspended matter, which may also inhibit the growth of P. crispus (Yan et al., 2021). Global warming and eutrophication can affect the structure and function of aquatic ecosystems by inhibiting the growth of submerged plants, which may lead to the freshwater ecosystem stability becoming more vulnerable in winter and spring. In this experiment, the content of C and P in the leaves decreased significantly with increasing temperature, which indicates that higher temperatures inhibit the growth of P. crispus leaves, which is in line with our hypothesis. Plants grown at higher temperatures have a lower respiration rate, which affects their metabolism and leaf growth (Dusenge et al., 2019). During the vigorous growing season, there is a high demand for P to produce sufficient rRNA and synthesize proteins. Therefore, during this period, the P content in the plant increases, which leads to a decrease in the N/P ratio (Gorokhova and Kyle, 2002). The C/P ratio of plant leaves is an important indicator of the physiological metabolism of plants, which can reflect the efficiency of plant P utilization; consequently, fast-growing organisms usually have a lower C/P ratio (Elser et al., 2000; Sterner and Elser, 2017). Our study showed that adding P increased the leaf utilization rate of P, which was reflected in the decrease in the C/P and N/P ratios of the leaves. The temperature-plant physiological hypothesis (Reich and Oleksyn, 2004) suggests that when temperatures rise, plants invest less nutrients into producing the proteins to maintain biochemical reactions (Oleksyn et al., 1998; Tjoelker et al., 1999; Xia et al., 2014), and thus, the C/P and N/P ratios increase significantly. Our results were consistent with this hypothesis. After heating, the C/N ratio of P. crispus leaves increased, reflecting the lower N-based biomass per unit C of the plant, which suggests that climate warming improved its nutrient use efficiency. Studies have shown that P. crispus is affected by an increase in temperature during the growing season and that the C/N ratio increases. Other angiosperms and phytoplankton communities have also shown a similar temperature-driven increase (Reich and Oleksyn, 2004; Domis et al., 2014; Zhang et al., 2016). However, in this experiment, the leaves were not sensitive to N reactions; therefore, the C/N ratio increased but not significantly. In nature, warming does not have an inhibitory effect on all organisms, and the overall trend indicates that warming is beneficial to smaller biota, such as phytoplankton. It does this by increasing the mineralization of organic carbon and offsetting the direct impact of the increase in the carbon storage capacity of the ecosystem caused by the increase in atmospheric carbon dioxide (Daufresne et al., 2009; Finkel et al., 2010). This may explain why some studies have not detected an effect of rising ambient temperature on the C/N ratio of certain plants.
Submerged plants tend to elongate their stems to reduce the stress associated with low-light conditions (Chen et al., 2016). In this experiment, when nutrient enriched, the C and P contents in the stem of P. crispus increased during the elongation stage. Stems play an indispensable role in connecting the aboveground and underground parts of plants. Therefore, P. crispus stems may need to meet its internal nutrient transport requirements to resist the stress associated with light reductions (Marschner, 2011; Grasset et al., 2015). This study proves that the addition of P increases the efficiency of the stem. Taken together, the stems also follow the temperature-plant physiology hypothesis (Reich and Oleksyn, 2004), and the N/P ratio increases significantly when the temperature increases. Studies have shown that under moderate nutrient concentrations (TN: 3 mg/L, TP: 0.2 mg/L), warming reduces the stem N/P ratio of P. crispus (Yan et al., 2021). However, in this study, the interaction did not have a significant effect on the N/P ratio, which may be caused by different nutrient concentrations. In this experiment, warming led to a reduction in the C and P contents in leaves, but had no significant effect on the C and P contents in stems. Furthermore, warming had less effect on the N content of the leaves and stems. This result indicated that warming was not conducive to the effective utilization of C and P in P. crispus leaves, which results in a decrease in the leaves’ ability to accumulate C and P. The leaves of submerged plants may become more heated than the stems because of their larger surface area. In addition, we found that nutrient addition had a greater effect than warming on the stoichiometric characteristics of stems.
Conclusion
We conclude that different plant organs exhibit different responses to P addition and warming, which demonstrates the importance of assessing the responses of different submerged plant organs to environmental changes. Furthermore, interactive effects between P addition and warming were observed in the leaf, turion, and seed C:N:P stoichiometry, which highlights the importance of multifactorial studies on this topic. Our data indicated that warming resulted in a decrease in the C content in most organs except the stems; P addition increased the P content in most organs except the seeds and turions; and the N content in the seeds were affected by an interactive effect of both conditions. We also found that P addition had a greater effect than warming on the stoichiometric characteristics of the stem. Overall, the addition of P can help P. crispus to resist the adverse effects of high temperatures by aiding growth and asexual reproduction, and asexual propagules are more sensitive to P enrichment than sexual propagules.
Data Availability Statement
The raw data supporting the conclusions of this article will be made available by the authors, without undue reservation.
Author Contributions
YW, MD, TW, and JX designed the study. TW and MD conducted the field and laboratory measurements. MD and YW analyzed the data and wrote the manuscript. TW, JX, and YW revised the manuscript. All authors have approved the final manuscript.
Funding
This research was supported by the National Key R&D Program of China (grant no. 2018YFD0900904), the International Cooperation Project of the Chinese Academy of Sciences (grant no. 152342KYSB20190025), the National Natural Science Foundations of China (grant no. 31872687), and the Water Pollution Control and Management Project of China (grant no. 2018ZX07208005).
Conflict of Interest
The authors declare that the research was conducted in the absence of any commercial or financial relationships that could be construed as a potential conflict of interest.
Publisher’s Note
All claims expressed in this article are solely those of the authors and do not necessarily represent those of their affiliated organizations, or those of the publisher, the editors and the reviewers. Any product that may be evaluated in this article, or claim that may be made by its manufacturer, is not guaranteed or endorsed by the publisher.
Acknowledgments
We would like to thank Jinyu Huang for constructing and maintaining the temperature regulatory system. Furthermore, we are grateful to Terry Townshend and Editage (www.editage.cn) for improving the English language of this manuscript.
Supplementary Material
The Supplementary Material for this article can be found online at: https://www.frontiersin.org/articles/10.3389/fpls.2022.814255/full#supplementary-material
References
Aerts, R., Callaghan, T. V., Dorrepaal, E., van Logtestijn, R. S. P., and Cornelissen, J. H. C. (2009). Seasonal climate manipulations result in species-specific changes in leaf nutrient levels and isotopic composition in a sub-arctic bog. Funct. Ecol. 23, 680–688. doi: 10.1111/j.1365-2435.2009.01566.x
Ågren, G. I. (2004). The C:N:P stoichiometry of autotrophs-theory and observations. Ecol. Lett. 7, 185–191. doi: 10.1111/j.1461-0248.2004.00567.x
An, Y., Wan, S., Zhou, X., Subedar, A. A., Wallace, L. L., and Luo, Y. (2005). Plant nitrogen concentration, use efficiency, and contents in a tallgrass prairie ecosystem under experimental warming. Glob. Chang. Biol. 11, 1733–1744. doi: 10.1111/j.1365-2486.2005.01030.x
Bakker, E. S., and Nolet, B. A. (2014). Experimental evidence for enhanced top-down control of freshwater macrophytes with nutrient enrichment. Oecologia 176, 825–836. doi: 10.1007/s00442-014-3047-y
Bonis, A., and Grillas, P. (2002). Deposition, germination and spatio-temporal patterns of charophyte propagule banks: a review. Aquat. Bot. 72, 235–248. doi: 10.1016/S0304-3770(01)00203-0
Bradshaw, C., Kautsky, U., and Kumblad, L. (2012). Ecological stoichiometry and multi-element transfer in a coastal ecosystem. Ecosystems 15, 591–603. doi: 10.1007/s10021-012-9531-5
Burkholder, J. M., Tomasko, D. A., and Touchette, B. W. (2007). Seagrasses and eutrophication. J. Exp. Mar. Biol. Ecol. 350, 46–72. doi: 10.1016/j.jembe.2007.06.024
Caliskan, S., and Makineci, E. (2014). Variations in carbon and nitrogen ratios and their effects on seed germination in Cupressus sempervirens populations. Scand. J. For. Res. 29, 162–169. doi: 10.1080/02827581.2014.881544
Chen, H. D. (1985). The life history, biomass and asexual reproduction of Potamogeton crispus. Acta Hydrobiol. Sin. 9, 32–39.
Chen, J., Cao, T., Zhang, X., Xi, Y., Ni, L., and Jeppesen, E. (2016). Differential photosynthetic and morphological adaptations to low light affect depth distribution of two submersed macrophytes in lakes. Sci. Rep. 6:34028. doi: 10.1038/srep34028
Chen, L., and Frauenfeld, O. W. (2014a). A comprehensive evaluation of precipitation simulations over China based on CMIP5 multimodel ensemble projections. J. Geophys. Res. Atmos. 119, 5767–5786.
Chen, L., and Frauenfeld, O. W. (2014b). Surface air temperature changes over the twentieth and twenty-first centuries in China simulated by 20 CMIP5 models. J. Clim. 27, 3920–3937. doi: 10.1175/jcli-d-13-00465.1
Chen, X. F., Chen, K. Y., Xiao, Y. E., Zhang, S. D., and Wang, Q. Y. (2006). Effects of light and matrix on turion germination, seedling growth and leaf photosynthesis efficiency of Potamogeton crispus. J. Appl. Ecol. 17, 1413–1418.
Chen, X. L., and Chen, H. Y. H. (2021). Plant mixture balances terrestrial ecosystem C:N:P stoichiometry. Nat. Commun. 12, 4562–4562. doi: 10.1038/S41467-021-24889-W
Chen, Y., Han, W., Tang, L., Tang, Z., and Fang, J. (2013). Leaf nitrogen and phosphorus concentrations of woody plants differ in responses to climate, soil and plant growth form. Ecography 36, 178–184.
Crous, K. Y., Wallin, G., Atkin, O. K., Uddling, J., and Ekenstam, A. (2017). Acclimation of light and dark respiration to experimental and seasonal warming are mediated by changes in leaf nitrogen in Eucalyptus globulus. Tree Physiol. 37, 1069–1083. doi: 10.1093/treephys/tpx052
Dang, C. K., Harrison, S., Sturt, M. M., Giller, P. S., and Jansen, M. A. K. (2009). Is the elemental composition of stream invertebrates a determinant of tolerance to organic pollution? J. North Am. Benthol. Soc. 28, 778–784. doi: 10.1899/08-163.1
Daufresne, M., Lengfellnera, K., and Sommer, U. (2009). Global warming benefits the small in aquatic ecosystems. Proc. Natl. Acad. Sci. USA 106, 12788–12793. doi: 10.1073/pnas.0902080106
De Senerpont Domis, L. N., Van de Waal, D. B., Helmsing, N. R., Donk, E., and Mooij, W. M. (2014). Community stoichiometry in a changing world: combined effects of warming and eutrophication on phytoplankton dynamics. Ecology 95, 1485–1495. doi: 10.1890/13-1251.1
Domis, L. N. D., Van de Waal, D. B., Helmsing, N. R., Van Donk, E., and Mooij, W. M. (2014). Community stoichiometry in a changing world: combined effects of warming and eutrophication on phytoplankton dynamics. Ecology 95, 1485–1495.
Dülger, E., Heidbüchel, P., Schumann, T., Mettler-Altmann, T., and Hussner, A. (2017). Interactive effects of nitrate concentrations and carbon dioxide on the stoichiometry, biomass allocation and growth rate of submerged aquatic plants. Freshwat. Biol. 62, 1094–1104. doi: 10.1111/fwb.12928
Dusenge, M. E., Duarte, A. G., and Way, D. A. (2019). Plant carbon metabolism and climate change: elevated CO2 and temperature impacts on photosynthesis, photorespiration and respiration. New Phytol. 221, 32–49.
Elser, J. J., Bracken, M. E. S., Cleland, E. E., Gruner, D. S., Harpole, W. S., Hillebrand, H., et al. (2007). Global analysis of nitrogen and phosphorus limitation of primary producers in freshwater, marine and terrestrial ecosystems. Ecol. Lett. 10, 1135–1142. doi: 10.1111/j.1461-0248.2007.01113.x
Elser, J. J., Sterner, R. W., Gorokhova, E., Fagan, W. F., Markow, T. A., Cotner, J. B., et al. (2000). Biological stoichiometry from genes to ecosystems. Ecol. Lett. 3, 540–550. doi: 10.1111/j.1461-0248.2000.00185.x
Evans-White, M. A., Dodds, W. K., Huggins, D. G., and Baker, D. S. (2009). Thresholds in macroinvertebrate biodiversity and stoichiometry across water-quality gradients in Central Plains (USA) streams. J. North Am. Benthol. Soc. 28, 855–868. doi: 10.1899/08-113.1
Ferreira, V., Goncalves, A. L., Godbold, D. L., and Canhoto, C. (2010). Effect of increased atmospheric CO2 on the performance of an aquatic detritivore through changes in water temperature and litter quality. Glob. Change Biol. 16, 3284–3296. doi: 10.1111/j.1365-2486.2009.02153.x
Finkel, Z. V., Beardall, J., Flynn, K. J., Quigg, A., Rees, T. A. V., and Raven, J. A. (2010). Phytoplankton in a changing world: cell size and elemental stoichiometry. J. Plankton Res. 32, 119–137. doi: 10.1093/plankt/fbp098
Frost, P. C., and Elser, J. J. (2002). Effects of light and nutrients on the net accumulation and elemental composition of epilithon in boreal lakes. Freshwater Biol. 47, 173–183. doi: 10.1046/j.1365-2427.2002.00796.x
Gao, J., Luo, Q., and Li, G. (2005). The effects of dissolved oxygen, temperature, nitrogen and phosphorus on the turion germination and growth of Potamogeton crispus L. J. Wuhan University 51, 511–516.
Gerten, D., and Adrian, R. (2000). Climate-Driven changes in spring plankton dynamics and the sensitivity of shallow polymictic lakes to the north atlantic oscillation. Limnol. Oceanogr. 45, 1058–1066. doi: 10.4319/lo.2000.45.5.1058
Gorokhova, E., and Kyle, M. (2002). Analysis of nucleic acids in Daphnia: development of methods and ontogenetic variations in RNA-DNA content. J. Plankton Res. 24, 511–522. doi: 10.1093/plankt/24.5.511
Granéli, E., Weberg, M., and Salomon, P. S. (2008). Harmful algal blooms of allelophatic microalgal species: the role of eutrophication. Harmful Algae 8, 94–102. doi: 10.1016/j.hal.2008.08.011
Grasset, C., Delolme, C., Arthaud, F., and Bornette, G. (2015). Carbon allocation in aquatic plants with contrasting strategies: the role of habitat nutrient content. J. Veg. Sci. 26, 946–955. doi: 10.1111/jvs.12298
Güsewell, S. (2004). N:P ratios in terrestrial plants: variation and functional significance. New Phytol. 164, 243–266. doi: 10.1111/j.1469-8137.2004.01192.x
Güsewell, S., and Gessner, M. O. (2009). N:P ratios influence litter decomposition and colonization by fungi and bacteria in microcosms. Funct. Ecol. 23, 211–219. doi: 10.1111/j.1365-2435.2008.01478.x
Hessen, D. O., Ågren, G. I., Anderson, T. R., Elser, J. J., and de Ruiter, P. C. (2004). Carbon sequestration in ecosystems: the role of stoichiometry. Ecology 85, 1179–1192. doi: 10.1890/02-0251
Intergovernmental Panel on Climate Change (IPCC) (2013). “Climate change 2013: the physical science basis,” in Working Group I Contribution of to the Fifth Assessment. Report of the Intergovernmental Panel on Climate Change, eds T. F. Stocker, D. H. Qin, G.-K. Plattner, M. M. B. Tignor, S. K. Allen, J. Boschung, et al. (Cambridge, UK: Cambridge University Press), 89, 1–28.
Izmailova, A. V., and Rumyantsev, V. A. (2016). Trophic status of the largest freshwater lakes in the world. Lakes Res. 21, 20–30. doi: 10.1111/lre.12123
Jian, Y. X., Li, B., Wang, J. B., and Chen, J. K. (2003). Control of turion germination in Potamogeton crispus. Aquat Bot. 75, 59–69.
Jin, S. D., Li, Y. H., Ni, C. H., and Wang, B. (1994). Potamogeton crispus uptake of nitrogen and phosphorus in water and some influencing factors. Acta Ecol. Sin. 14, 168–173.
Jin, X. C., Xu, Q. J., and Huang, C. Z. (2005). Current status and future tendency of lake eutrophication in China. Sci. China Ser. C 48, 948–954.
Jones, J. I., Young, J. O., Eaton, J. W., and Moss, B. (2002). The influence ofnutrient loading, dissolved inorganic carbon and higher trophic levels on the interaction between submerged plants and periphyton. J. Ecol. 90, 12–24. doi: 10.1046/j.0022-0477.2001.00620.x
Kunii, H. (1982). Life cycle and growth of Potamogeton crispus L. in a shallow pond. Ojaga-Ike. J. Plant Res. 95, 109–124. doi: 10.1007/BF02488578
Kuo, S. (1996). “Phosphorus,” in Methods of Soil Analysis: Part 3—Chemical Methods, ed. D. L. Sparks (Madison, WI: Soil Science Society of America), 869–919.
Le, C., Zha, Y., Li, Y., Sun, D., Lu, H., and Yin, B. (2010). Eutrophication of lake waters in China: cost, causes, and control. Environ Manage 45, 662–668. doi: 10.1007/s00267-010-9440-3
LeBauer, D. S., and Treseder, K. K. (2008). Nitrogen limitation of net primary productivity in terrestrial ecosystems is globally distributed. Ecology 89, 371–379. doi: 10.1890/06-2057.1
Lu, J., Wang, H., Pan, M., Xia, J., Xing, W., and Liu, G. (2012). Using sediment seed banks and historical vegetation change data to develop restoration criteria for a eutrophic lake in China. Ecol. Eng. 39, 95–103. doi: 10.1016/j.ecoleng.2011.11.006
Lü, X. T., Kong, D. L., Pan, Q. M., Simmons, M. E., and Han, X. G. (2012). Nitrogen and water availability interact to affect leaf stoichiometry in a semi-arid grassland. Oecologia 168, 301–310. doi: 10.1007/s00442-011-2097-7
Ma, T. (2007). Effect of water nutrient on the growth of Potamageton crispus. [Ph.D thesis]. China: Nanjing Normal University.
Madsen, T. V., Hahn, P., and Johansen, J. (1998). Effects of inorganic carbon supply on the nitrogen requirement of two submerged macrophytes, Elodea canadensis and Callitriche cophocarpa. Aquat. Bot. 62, 95–106. doi: 10.1016/S0304-3770(98)00087-4
Mooij, W. M., Hülsmann, S., Domis, L. N. D. S., Nolet, B. A., Bodelier, P. L. E., Boers, P. C. M., et al. (2005). The impact of climate change on lakes in the Netherlands: a review. Aquat. Ecol. 39, 381–400. doi: 10.1007/s10452-005-9008-0
Oleksyn, J., Modrzınski, J., Tjoelker, M., Reich, P., and Karolewski, P. (1998). Growth and physiology of Picea abies populations from elevational transects: common garden evidence for altitudinal ecotypes and cold adaptation. Funct. Ecol. 12, 573–590. doi: 10.1046/j.1365-2435.199800236.x
Peñuelas, J., Sardans, J., Rivas-Ubach, A., and Janssens, I. (2012). The human induced inbalance between C, N and P in Earth’s life-system. Glob. Chang. Biol. 18, 3–6. doi: 10.1111/j.1365-2486.2011.02568.x
Perkins, S. E., Alexander, L. V., and Nairn, J. R. (2012). Increasing frequency, intensity and duration of observed global heatwaves and warm spells. Geophys. Res. Lett. 39:L20714. doi: 10.1029/2012GL053361
Phillips, G., Willby, N., and Moss, B. (2016). Submerged macrophyte decline in shallow lakes: what have we learnt in the last forty years? Aquat. Bot. 135, 37–45. doi: 10.1016/j.aquabot.2016.04.004
Plénet, D., Mollier, A., and Pellerin, S. (2000). Growth analysis of maize field crops under phosphorus deficiency: II. Plant Soil 224, 259–272. doi: 10.1023/A:1004835621371
Qi, X., and Yang, J. (2019). Extended-range prediction of a heat wave event over the Yangtze River Valley: role of intraseasonal signals. Atmos. Ocean. Sci. Lett. 12, 451–457. doi: 10.1080/16742834.2019.1669408
Qin, B., Zhou, J., Elser, J. J., Gardner, W. S., Deng, J., and Brookes, J. D. (2020). Water depth underpins the relative role and fates of nitrogen and phosphorus in lakes. Environ. Sci. Technol. 54, 3191–3198. doi: 10.1021/acs.est.9b05858
Raven, J. A., and Geider, R. J. (1988). Temperature and algal growth. New Phytol. 110, 441–461. doi: 10.1111/j.1469-8137.1988.tb00282.x
Reich, P. B., and Oleksyn, J. (2004). Global patterns of plant leaf N and P in relation to temperature and latitude. Proc. Natl. Acad. Sci. U.S.A. 101, 11001–11006. doi: 10.1073/pnas.0403588101
Ren, C. J., Chen, J., Deng, J., Zhao, F. Z., Han, X. H., Yang, G. H., et al. (2017). Response of microbial diversity to C:N:P stoichiometry in fine root and microbial biomass following afforestation. Biol. Fertil. Soils 53, 457–468. doi: 10.1007/s00374-017-1197-x
Rogers, K. H., and Breen, C. M. (1980). Growth and reproduction of Potamogeton crispus in a South African lake. J. Ecol. 68, 561–571. doi: 10.2307/2259422
Sañudo-Wilhelmy, S. A., Kustka, A. B., Gobler, C. J., Hutchins, D. A., Yang, M., Lwiza, K., et al. (2001). Phosphorus limitation of nitrogen fixation by Trichodesmium in the central Atlantic Ocean. Nature 411, 66–69. doi: 10.1038/35075041
Sardans, J., Rivas-Ubach, A., and Peñuelas, J. (2012). The C:N:P stoichiometry of organisms and ecosystems in a changing world: a review and perspectives. Perspect Plant Ecol. 14, 33–47. doi: 10.1016/j.ppees.2011.08.002
Sastroutomo, S. S., Ikusima, I., Numata, M., and Iizumi, S. (1979). The importance of turions in the propagation of pondweed (Potamogeton crispus L.). Ecol. Rev. 19, 75–88.
Shen, J., Xu, W., and Shi, F. C. (2008). Effects of Weight and Store Temperature on Turion Germination and Seedling growth of Potamogeton crispus L. Bull. Entomol. Res. 28, 477–481.
Short, F. T., Kosten, S., Morgan, P. A., Malone, S., and Moore, G. E. (2016). Impacts of climate change on submerged and emergent wetland plants. Aquat. Bot. 135, 3–17. doi: 10.1016/j.aquabot.2016.06.006
Sondergaard, M., Johansson, L. S., Lauridsen, T. L., Jorgensen, T. B., Liboriussen, L., and Jeppesen, E. (2010). Submerged macrophytes as indicators of the ecological quality of lakes. Freshwater Biol. 55, 893–908. doi: 10.1111/j.1365-2427.2009.02331.x
Sterner, R. W., and Elser, J. J. (2017). Ecological stoichiometry: the biology of elements from molecules to the biosphere. Princeton: Princeton University Press.
Strecker, A. L., Cobb, T. P., and Vinebrooke, R. D. (2004). Effects of experimental greenhouse warming on phytoplankton and zooplankton communities in fishless alpine ponds. Limnol. Oceanogr. 49, 1182–1190. doi: 10.4319/lo.2004.49.4.1182
Su, H. J., Wu, Y., Xia, W. L., Xie, P., and Cao, T. (2017). Community level stoichiometric characteristics of submerged macrophytes and their influencing factors in the mid-lower Yangtze lakes. J. Lake Sci. 29, 430–438. doi: 10.18307/2017.0219
Tjoelker, M., Reich, P., and Oleksyn, J. (1999). Changes in leaf nitrogen and carbohydrates underlie temperature and CO2 acclimation of dark respiration in five boreal tree species. Plant Cell Environ. 22, 767–778. doi: 10.1046/j.1365-3040.1999.00435.x
Wang, H. J., Liang, X. M., Jiang, P. H., Wang, J., Wu, S. K., and Wang, H. Z. (2008). TN: TP ratio and planktivorous fish do not affect nutrient-chlorophyll relationships in shallow lakes. Freshwater Biol. 53, 935–944. doi: 10.1111/j.1365-2427.2007.01950.x
Wang, N., Gao, J., Zhang, S. Q., and Wang, G. X. (2014). Variations in leaf and root stoichiometry of Nitraria tangutorum along aridity gradients in the Hexi Corridor, northwest China. Contemp. Probl. Ecol. 7, 308–314. doi: 10.1134/S1995425514030123
Welker, J. M., Fahnestock, J. T., Sullivan, P. F., and Chimner, R. A. (2005). Leaf mineral nutrition of Arctic plants in response to warming and deeper snow in northern Alaska. Oikos 109, 167–177. doi: 10.1111/j.0030-1299.2005.13264.x
Xia, C., Yu, D., Wang, Z., and Xie, D. (2014). Stoichiometry patterns of leaf carbon, nitrogen and phosphorous in aquatic macrophytes in eastern China. Ecol. Eng. 70, 406–413. doi: 10.1016/j.ecoleng.2014.06.018
Xie, H. T., Yu, M. K., and Cheng, X. R. (2018). Leaf non-structural carbohydrate allocation and C:N:P stoichiometry in response to light acclimation in seedlings of two subtropical shade-tolerant tree species. Plant Physiol. Bioch. 124, 146–154. doi: 10.1016/j.plaphy.2018.01.013
Xie, Y. H., Yu, D., and Geng, X. H. (2003). Effects of elevated CO2 concentration on phenotypic, physiological and biochemical characteristics of submersed plant Potamogeton crispus leaf. Chin. J. Plan. Ecol. 27, 218–222. doi: 10.17521/cjpe.2003.0033
Xu, J., Wang, T., García, M. J., Li, C., Hu, B., Pan, M., et al. (2020). Effects of warming, climate extremes and phosphorus enrichment on the growth, sexual reproduction and propagule carbon and nitrogen stoichiometry of Potamogeton crispus L. Environ. Int. 137:105502. doi: 10.1016/j.envint.2020.105502
Xu, W. W., Hu, W. P., Deng, J. C., Zhu, J. G., Li, Q. Q., and Zhang, H. M. (2015). Influence of harvesting Potamogeton Crispus in a submerged plant community on the growth of submerged aquatic plants and their effects on water quality. Ecol. Environ. Sci. 24, 1222–1227. doi: 10.16258/j.cnki.1674-5906.2015.07.022
Yan, Z., Wang, Q., Li, Y., Wu, L., Wang, J., Xing, B., et al. (2021). Combined effects of warming and nutrient enrichment on water properties, growth, reproductive strategies and nutrient stoichiometry of Potamogeton crispus. Environ. Exp. Bot. 190:104572. doi: 10.1016/j.envexpbot.2021.104572
Zhang, H. Y., Wu, H. H., Yu, Q., Wang, Z. W., Wei, C. Z., Long, M., et al. (2013). Sampling date, leaf age and root size: implications for the study of plant C:N:P stoichiometry. PLoS One 8:e60360. doi: 10.1371/journal.pone.0060360
Zhang, L. F. (2006). Effect of Water Quality on the Growth of Submerged Macrophytes (Elodea nattalii and potamageton crispus). Ph.D thesis. China: HoHai university.
Zhang, P. Y., Bakker, E. S., Zhang, M., and Xu, J. (2016). Effects of warming on Potamogeton crispus growth and tissue stoichiometry in the growing season. Aquat. Bot. 128, 13–17. doi: 10.1016/j.aquabot.2015.08.004
Zhang, P. Y., Grutters, B. M. C., Van Leeuwen, C. H. A., Xu, J., Petruzzella, A., Van Den Berg, R. F., et al. (2019). Effects of rising temperature on the growth, stoichiometry, and palatability of aquatic plants. Front. Plant Sci. 9:1947. doi: 10.3389/fpls.2018.01947
Zhang, P. Y., Kuramae, A., Van Leeuwen, C. H., Velthuis, M., Van Donk, E., Xu, J., et al. (2020). Interactive effects of rising temperature and nutrient enrichment on aquatic plant growth, stoichiometry, and palatability. Front. Plant Sci. 11:58. doi: 10.3389/fpls.2020.00058
Keywords: stoichiometric characteristics, eutrophication, climate change, growth organs, reproductive organs
Citation: Dai M, Wang T, Wang Y and Xu J (2022) Effects of Warming and Phosphorus Enrichment on the C:N:P Stoichiometry of Potamogeton crispus Organs. Front. Plant Sci. 13:814255. doi: 10.3389/fpls.2022.814255
Received: 13 November 2021; Accepted: 25 February 2022;
Published: 29 March 2022.
Edited by:
Wen-Hua You, Jiangsu University, ChinaReviewed by:
Xinsheng Chen, Institute of Subtropical Agriculture (CAS), ChinaFeng Li, Institute of Subtropical Agriculture (CAS), China
Meng Zhang, Jiangxi Academy of Environmental Sciences, China
Copyright © 2022 Dai, Wang, Wang and Xu. This is an open-access article distributed under the terms of the Creative Commons Attribution License (CC BY). The use, distribution or reproduction in other forums is permitted, provided the original author(s) and the copyright owner(s) are credited and that the original publication in this journal is cited, in accordance with accepted academic practice. No use, distribution or reproduction is permitted which does not comply with these terms.
*Correspondence: Yuyu Wang, d2FuZ3l5QGJqZnUuZWR1LmNu; Jun Xu, eHVqdW5AaWhiLmFjLmNu
†These authors have contributed equally to this work