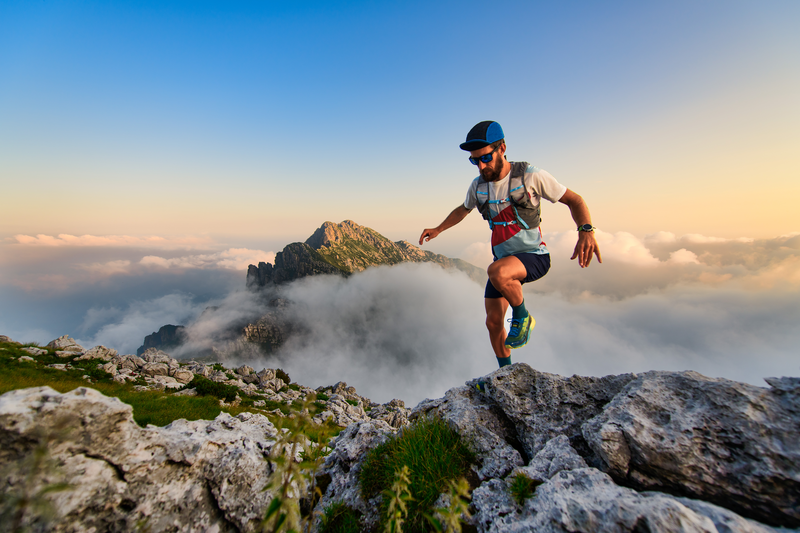
94% of researchers rate our articles as excellent or good
Learn more about the work of our research integrity team to safeguard the quality of each article we publish.
Find out more
OPINION article
Front. Plant Sci. , 28 June 2021
Sec. Plant Physiology
Volume 12 - 2021 | https://doi.org/10.3389/fpls.2021.680709
This article is part of the Research Topic Hormonal Crosstalk on the Regulation of Stress Responses View all 11 articles
Plants are continuously exposed to various biotic and abiotic stressors that limit their productivity. Any kind of stressor leads to the generation of Reactive Oxygen Species (ROS), majorly, through the activity of plasma membrane-localized respiratory burst oxidase homolog (RBOH) proteins that generate O2•- in the apoplast (Mittler, 2017). Owing to its reducing properties, O2•- is highly toxic and is involved in the peroxidation of membrane lipids and the conversion of Fe3+ to ferrous ions (Fe2+). In addition, O2•- can also be converted into H2O2 by the activity of superoxide dismutase (SOD), which may enter the cytosol through aquaporins to trigger signaling cascades (Rodrigues et al., 2017). Further, Fe2+ can directly interact with H2O2 through Fenton reaction and accelerates the production of hydroxyl radical (OH•), which is another lethal ROS (Mittler, 2017). Since ions of Fe play a major role in ROS production, plants keep a check on the concentration of these ions to maintain ROS homeostasis. In plants, two major groups of proteins participate in iron (Fe2+/Fe3+) sequestration including ferritin and plant defensin proteins. The latter group of proteins also participate in the plant defense and inhibit the growth of fungal pathogens. Recently, a pivotal role of iron in the hypersensitive response induced cell death, during plant defense signaling, was reported in rice upon infection with the fungal pathogen, Magnaporthe oryzae (Dangol et al., 2019). This type of iron-dependent cell death was first reported in mammalian tumor cells and was termed “ferroptosis” by Dixon and co-workers in 2012 (Dixon et al., 2012). Ferroptosis is, genetically, morphologically, and biochemically, distinct from other types of known cell death including apoptosis, necrosis, and autophagy (Dixon et al., 2012). The onset of ferroptosis requires high levels of ROS and Fe2+ and depletion of reduced glutathione (GSH) (Dixon and Stockwell, 2019). Subsequently, a GSH-dependent glutathione peroxidase-4 (GPX-4) was identified as a key player of mammalian ferroptosis (Angeli et al., 2014; Yang et al., 2014). GPX enzymes catalyze the reduction of H2O2 or organic hydroperoxides to water or corresponding alcohols using reduced GSH or thioredoxin (TRX) (Yang et al., 2016).
In plants, ferroptosis was first reported by Distéfano and co-workers in 2017 in Arabidopsis thaliana under heat stress conditions, and, similar to the animals, the plant ferroptosis was also found to be dependent on the GSH depletion, lipid peroxidation, and accumulation of ROS and ions of Fe (Distéfano et al., 2017). Although the literature evidence on plant ferroptosis is limited, it has been observed that it can be triggered by both abiotic (heat stress) and biotic stressors (M. oryzae), and both plant and animal ferroptosis exhibit similar morphological characteristics, such as cytoplasmic retraction, normal nuclei, and the formation of small lytic vacuoles (Distéfano et al., 2021). In addition, a noticeable shrinkage of mitochondria along with increased mitochondrial membrane density and decreased mitochondrial cristae has been observed in animal ferroptosis (Dixon et al., 2012); however, no such observation has been reported in the case of plants. By utilizing several agonists (Supplementary Table 1), Dangol et al. characterized the detailed process of plant ferroptosis, and their results collectively suggested the role of plasma membrane-localized NADPH-oxidases (RBOHs), NADP-malic enzyme, polymerization of actin microfilaments along with the depletion of GSH, peroxidation of membrane lipids, and accumulation of ions of Fe and ROS (Dangol et al., 2019). Similar to the animals, the plant ferroptosis also seems to be dependent on the activity of GPX-4 as treatment for erastin, an inducer of ferroptosis and inhibitor of GPX-4, resulted in the depletion of reduced and total GSH and accumulation of ROS and Fe3+ to result in ferroptosis in rice sheath cells (Dangol et al., 2019). Correspondingly, treatment of Arabidopsis cells with another GPX-4 inhibitor RSL3 led to cell death, which was prevented by the treatment of ferroptosis inhibitors including liproxstatin-1 and ferrostatin-1 (Hajdinák et al., 2019). A recent study demonstrated that silencing of GPX4 in Nicotiana benthamiana resulted in enhanced ferroptosis in response to Tobacco mosaic virus 24A+UPD infection (Macharia et al., 2020). However, some of the recent evidence suggests the possible involvement of others GPXs, such as membrane-localized GPXL5, in the plant ferroptosis (Meyer et al., 2020). Overall, these results suggest that ferroptosis is a highly regulated cell death process that is induced by both biotic and abiotic stressors. Since the analysis of ferroptosis during incompatible rice–M. oryzae interactions suggested a positive role of ferroptosis in preventing the infection by avirulent strains of the fungus, the process of ferroptosis can be manipulated in the future to develop the biotic and abiotic resilient crops (Dangol et al., 2019; Kazan and Kalaipandian, 2019).
Although the direct involvement of any phytohormones in the plant ferroptosis has not been reported yet, a growing body of evidence suggests the possible involvement of ethylene in the plant ferroptosis. It has been observed that all the components of ferroptosis, including GSH, ions of Fe, and ROS, are linked to the gaseous plant hormone ethylene, and thus, we hypothesize the involvement of ethylene in the regulation of plant ferroptosis based on the following literature evidence.
1. There is ample evidence that suggests ethylene induces the biosynthesis of GSH. For instance, exogenous treatment of ethylene has been shown to significantly elevate the levels of GSH and ascorbate (AsA) in Zea mays seedlings under cadmium (Cd) toxicity (Liu et al., 2019). In addition, the exogenous application of ethephon, an ethylene precursor, increased the amount of GSH in Cd-treated Brassica juncea (Khan et al., 2016), while the application of ethylene inhibitor Aminoethoxyvinylglycine (AVG) resulted in decreased GSH levels (Masood et al., 2012). Similarly, ethephon treated Glycine max plants showed an accumulation of GSH together with the increased activity of glutathione reductase (GR) under waterlogging conditions (Kim et al., 2018). In contrast, treatment using ethylene biosynthesis inhibitor resulted in reduced levels of GSH in Cd-exposed Lycium chinense plants compared with untreated plants, further confirming a direct role of ethylene in the regulation of cellular GSH levels (Guan et al., 2015).
2. The connection between Fe2+ and ethylene is well-established, and it has been known for years that excess ions of Fe trigger ethylene biosynthesis in plants (Peng and Yamauchi, 1993; Becker and Asch, 2005; Majerus et al., 2007). The ethylene biosynthetic pathway is relatively simple, taking place via only two committed enzymatic reactions catalyzed by 1-amino-cyclopropane-1-carboxylic acid (ACC) synthase (ACS) and ACC oxidase (ACO) (Riyazuddin et al., 2020). Both ACS and ACO are controlled at the transcriptional and post-translational levels, which enables a tailored regulation of ethylene production in plants (Pattyn et al., 2021). The final regulatory step of the ethylene biosynthetic pathway is the conversion of ACC to ethylene and is catalyzed by ACO, which requires Fe2+ as the active-site cofactor. Therefore, Fe2+ plays a critical role in ethylene biosynthesis by regulating the activity of ACO (Houben and Van de Poel, 2019). Subsequently, the deficiency or accumulation of Fe2+ may directly affect cellular ethylene production. In other words, Fe2+ may be the limiting factor in ethylene production, and the excess could result in higher production of ethylene (Peng and Yamauchi, 1993; Li et al., 2015). For instance, excess Fe2+ resulted in the upregulation of the transcript level of ethylene biosynthesis genes, such as AtACS2, AtACS7, AtACS8, AtACS11, and AtACO1 and AtACO2, and contributed to higher ethylene production in Arabidopsis (Li et al., 2015). In turn, ethylene, thus, produced triggered the expression of genes encoding Fe-sequestering ferritins, such as FER1, FER2, FER3, and FER4, and minimized Stelar and xylem Fe2+ concentrations to limit Fe accumulation and toxicity both in shoots and roots (Li et al., 2015). Further, FER1 and FER3 were found to be significantly elevated in the roots of Arabidopsis ethylene-overproduction mutant (eto1-1) as compared with wild type (WT) during Fe2+ toxicity (Li et al., 2015).
3. The interconnection between ethylene and ROS homeostasis is quite evident, and it is well-known that ethylene maintains ROS homeostasis by activating the enzymatic and non-enzymatic antioxidant defense to limit the accumulation of ROS and subsequent peroxidation of membrane lipids that result in ferroptosis (Riyazuddin et al., 2020). At first, it was shown that the exogenous application of ethylene precursor ACC resulted in the enhanced activities of ascorbate peroxidase (APX), catalase (CAT), SOD, and peroxidase (POX), and reduced the lipid peroxidation in creeping bentgrass (Larkindale and Huang, 2004). Similarly, exogenous application of ACC has been shown to improve the heat stress tolerance in rice seedlings by reducing lipid peroxidation and relative electrolyte leakage during heat stress (Wu and Yang, 2019). Correspondingly, the exogenous application of ethephon, another ethylene precursor, to Ni-treated Brassica juncea plants resulted in significantly increased activity of SOD, APX, GR, GPX, and the accumulation of proline (Khan et al., 2020). Similarly, exogenous application of ethephon substantially induced the activity of enzymatic and non-enzymatic antioxidants, such as SOD, APX, GR, GSH -S-transferase (GST), GPX, monodehydroascorbate reductase (MDHAR), dehydroascorbate reductase (DHAR), AsA, and GSH, under Zinc toxicity in B. juncea (Khan et al., 2019). In Lactuca sativa, the application of exogenous ethylene increased the activity of antioxidant enzymes, such as SOD, CAT, and APX, and reduced the H2O2 content (Ma et al., 2013), while in Nelumbo sp., ethylene treatment maintained endogenous ROS levels by regulating the AsA–GSH antioxidant system under Cd stress (Yuan et al., 2018). In Dendrobium nobile, ethephon treatment led to an increase in the contents of AsA and GSH, while exogenous treatment of ethylene inhibitor resulted in decreased contents of both of these antioxidants. In addition, APX and GR also showed a significant change under the ethylene regulator treatments (Zhang et al., 2021). Further, a growing body of evidence suggests that Ethylene response factors (ERFs) play a role in linking redox and hormonal regulation in plant responses to abiotic stresses. The overexpression of ERF96 gene enhanced selenium tolerance in Arabidopsis via elevation of CAT, GPX activities, and GSH content to cope with H2O2 compared with those in WT (Jiang et al., 2020). Similarly, overexpression of ERF38 resulted in reduced contents of MDA and H2O2 in the transgenic poplars as compared with WT by elevating the expression of POD and SOD and accumulation of proline and soluble proteins (Cheng et al., 2019). Furthermore, overexpression of ERF1 in tomatoes resulted in higher proline accumulation and lower lipid peroxidation as well as increased the activity of antioxidant enzymes (POD and SOD) under salt stress (Hu et al., 2014). ERF3 also regulated ROS metabolism in tobacco resulting in lower accumulation of ROS (Wu et al., 2008). Overall, all of these results confirm a tight correlation between ethylene levels and ROS homeostasis in plants.
4. In addition to regulating the enzymatic and non-enzymatic antioxidants, ethylene has also been shown to regulate the activities of NADPH-oxidases (RBOHs) to limit ROS production. At first, ethylene was shown to be involved in the regulation of H2O2 signaling by controlling the expression of Rboh genes under hypoxia stress in Arabidopsis (Yang and Hong, 2015). In addition, ACS1 mediated early ethylene production has been shown to temporarily inhibit the expression of NADPH-oxidase (RBOH-D and RBOH-F) genes to prevent the ROS burst in Brassica oleracea (Jakubowicz et al., 2010). On the contrary, reduced expressions of ethylene biosynthesis genes including ACS7 and ACS8 and ethylene signaling genes including ERF73 were observed in the rbohd-knockout mutants, further indicating an intricate relationship between NADPH-oxidase, ROS production, and ethylene (Yang and Hong, 2015).
Based on the presented literature evidence, it can be speculated that ethylene controls and inhibits the ferroptosis process in plants by at least three ways: (1) by limiting the excess accumulation of Fe2+ in the cells via increasing the iron sequestrating ferritin proteins and thus inhibiting the generation of excess ROS based on Fe2+ accumulation, (2) by activating the antioxidant defense mechanism to limit the excess ROS accumulation in the cells, and (3) by facilitating the synthesis of GSH (Figure 1). However, further experimentations are required to investigate the ethylene biosynthesis and/or signaling during iron- and ROS-dependent ferroptosis in plants.
Figure 1. A schematic representation highlighting the proposed role of ethylene in ferroptosis cell death in plants. Plant ferroptosis is characterized by the increase in ROS and subsequent peroxidation of membrane lipids, accumulation of iron, and depletion of reduced glutathione (GSH). Stress-induced accumulation of ROS is mainly dependent on the activity of plasma membrane-localized NADPH-oxidase (respiratory burst oxidase homolog, RBOH) proteins. NADP-malic enzyme supplies electrons to the RBOH proteins and thus contributes indirectly to ROS production and thus to plant ferroptosis. H2O2 produced in the apoplast, because of the conversion of superoxide radicals (O2•-) and/or the activities of the class III peroxidases (POX) and polyamine oxidases (PAO), enters the cytosol through plasma membrane intrinsic proteins (PIP; aquaporins) and mediates the conversion of Fe2+ to Fe3+ through the Fenton reaction. Fe2+ are otherwise involved in the biosynthesis of ethylene by functioning as a cofactor of enzyme ACC oxidase (ACO). Ethylene so produced triggers the synthesis of ferritin proteins that participate in the sequestration of ions of iron and thus prevent their accumulation, which is a prerequisite for ferroptosis cell death. In addition, ethylene also induces the synthesis of GSH and thus helps to maintain the GSH levels to prevent ferroptosis cell death.
RR and RG conceived the idea. Both the authors were involved in the writing of the manuscript, contributed equally to the drafts, and gave final approval for publication.
RR was supported by Postdoctoral Hungarian State Scholarship 2020/2021 (AK-00205-004/2020) and Bilateral State Scholarship 2019/2020 (AK-00210-002/2019), Tempus Public Foundation. RG was supported by the Ramalingaswami re-entry fellowship (BT/HRD/35/02/2006) from the Department of Biotechnology, Ministry of Science and Technology, Government of India.
The authors declare that the research was conducted in the absence of any commercial or financial relationships that could be construed as a potential conflict of interest.
The Supplementary Material for this article can be found online at: https://www.frontiersin.org/articles/10.3389/fpls.2021.680709/full#supplementary-material
Angeli, J. P. F., Schneider, M., Proneth, B., Tyurina, Y. Y., Tyurin, V. A., Hammond, V. J., et al. (2014). Inactivation of the ferroptosis regulator Gpx4 triggers acute renal failure in mice. Nat. Cell Biol. 16, 1180–1191. doi: 10.1038/ncb3064
Becker, M., and Asch, F. (2005). Iron toxicity in rice—conditions and management concepts. J. Soil Sci. Plant Nutr. 168, 558–573. doi: 10.1002/jpln.200520504
Cheng, Z., Zhang, X., Zhao, K., Yao, W., Li, R., Zhou, B., et al. (2019). Over-expression of ERF38 gene enhances salt and osmotic tolerance in transgenic poplar. Front. Plant Sci. 10:1375. doi: 10.3389/fpls.2019.01375
Dangol, S., Chen, Y., Hwang, B. K., and Jwa, N. S. (2019). Iron-and reactive oxygen species-dependent ferroptotic cell death in rice-Magnaporthe oryzae interactions. Plant Cell 31, 189–209. doi: 10.1105/tpc.18.00535
Distéfano, A. M., López, G. A., Setzes, N., Marchetti, F., Cainzos, M., Cascallares, M., et al. (2021). Ferroptosis in plants: triggers, proposed mechanisms, and the role of iron in modulating cell death. J. Exp. Bot. 72, 2125–2135. doi: 10.1093/jxb/eraa425
Distéfano, A. M., Martin, M. V., Córdoba, J. P., Bellido, A. M., D'Ippólito, S., Colman, S. L., et al. (2017). Heat stress induces ferroptosis-like cell death in plants. J. Cell Biol. 216, 463–476. doi: 10.1083/jcb.201605110
Dixon, S. J., Lemberg, K. M., Lamprecht, M. R., Skouta, R., Zaitsev, E. M., Gleason, C. E., et al. (2012). Ferroptosis: an iron-dependent form of nonapoptotic cell death. Cell 149, 1060–1072. doi: 10.1016/j.cell.2012.03.042
Dixon, S. J., and Stockwell, B. R. (2019). The hallmarks of ferroptosis. Ann. Rev. Cancer Biol. 3, 35–54. doi: 10.1146/annurev-cancerbio-030518-055844
Guan, C., Ji, J., Jia, C., Guan, W., Li, X., Jin, C., et al. (2015). A GSHS-like gene from Lycium chinense may be regulated by cadmium-induced endogenous salicylic acid and overexpression of this gene enhances tolerance to cadmium stress in Arabidopsis. Plant Cell Rep. 34, 871–884. doi: 10.1007/s00299-015-1750-8
Hajdinák, P., Czobor, Á., and Szarka, A. (2019). The potential role of acrolein in plant ferroptosis-like cell death. PLoS ONE 14:e0227278. doi: 10.1371/journal.pone.0227278
Houben, M., and Van de Poel, B. (2019). 1-Aminocyclopropane-1-carboxylic acid oxidase (ACO): the enzyme that makes the plant hormone ethylene. Front. Plant Sci. 10:695. doi: 10.3389/fpls.2019.00695
Hu, N., Tang, N., Yan, F., Bouzayen, M., and Li, Z. (2014). Effect of LeERF1 and LeERF2 overexpression in the response to salinity of young tomato (Solanum lycopersicum cv. Micro-Tom) seedlings. Acta Physiol. Plant. 36, 1703–1712. doi: 10.1007/s11738-014-1545-5
Jakubowicz, M., Gałgańska, H., Nowak, W., and Sadowski, J. (2010). Exogenously induced expression of ethylene biosynthesis, ethylene perception, phospholipase D, and Rboh-oxidase genes in broccoli seedlings. J. Exp. Bot. 61, 3475–3491. doi: 10.1093/jxb/erq177
Jiang, L., Yang, J., Liu, C., Chen, Z., Yao, Z., and Cao, S. (2020). Overexpression of ethylene response factor ERF96 gene enhances selenium tolerance in Arabidopsis. Plant Physiol. Biochem. 149, 294–300. doi: 10.1016/j.plaphy.2020.02.024
Kazan, K., and Kalaipandian, S. (2019). Ferroptosis: yet another way to die. Trends Plant Sci. 24, 479–481. doi: 10.1016/j.tplants.2019.03.005
Khan, M. I. R., Jahan, B., Alajmi, M. F., Rehman, M. T., and Khan, N. A. (2019). Exogenously-sourced ethylene modulates defense mechanisms and promotes tolerance to zinc stress in mustard (Brassica juncea L.). Plants 8:540. doi: 10.3390/plants8120540
Khan, M. I. R., Jahan, B., AlAjmi, M. F., Rehman, M. T., and Khan, N. A. (2020). Ethephon mitigates nickel stress by modulating antioxidant system, glyoxalase system and proline metabolism in Indian mustard. Physiol. Mol. Biol. Plants 26, 1201–1213. doi: 10.1007/s12298-020-00806-1
Khan, N. A., Asgher, M., Per, T. S., Masood, A., Fatma, M., and Khan, M. I. R. (2016). Ethylene potentiates sulfur-mediated reversal of cadmium inhibited photosynthetic responses in mustard. Front. Plant Sci. 7:1628. doi: 10.3389/fpls.2016.01628
Kim, Y., Seo, C. W., Khan, A. L., Mun, B. G., Shahzad, R., Ko, J. W., et al. (2018). Exo-ethylene application mitigates waterlogging stress in soybean (Glycine max L.). BMC Plant Biol. 18, 1–16. doi: 10.1186/s12870-018-1457-4
Larkindale, J., and Huang, B. (2004). Thermotolerance and antioxidant systems in Agrostis stolonifera: involvement of salicylic acid, abscisic acid, calcium, hydrogen peroxide, and ethylene. J. Plant Physiol. 161, 405–413. doi: 10.1078/0176-1617-01239
Li, G., Xu, W., Kronzucker, H. J., and Shi, W. (2015). Ethylene is critical to the maintenance of primary root growth and Fe homeostasis under Fe stress in Arabidopsis. J. Exp. Bot. 66, 2041–2054. doi: 10.1093/jxb/erv005
Liu, J., Zhao, H., Liu, Y., and Wang, J. (2019). Effect of exogenous ethylene on physiological metabolism of Zea mays seedlings under cadmium stress. Chinese J. Ecol. 27, 757–765.
Ma, J., Hu, W. Z., Bi, Y., and Jiang, A. L. (2013). Efect of exogenous ethylene and MeJA treatments on reactive oxygen metabolism in tissue of fresh-cut lettuce. Food Ind. Sci. Technol. 34, 338–341. doi: 10.13386/j.issn1002-0306.2013.17.047
Macharia, M., Das, P. P., Naqvi, N. I., and Wong, S. M. (2020). iTRAQ-based quantitative proteomics reveals a ferroptosis-like programmed cell death in plants infected by a highly virulent tobacco mosaic virus mutant 24A+ UPD. Phytopathol Res. 2, 1–13. doi: 10.1186/s42483-019-0043-5
Majerus, V., Bertin, P., and Lutts, S. (2007). Effects of iron toxicity on osmotic potential, osmolytes and polyamines concentrations in the African rice (Oryza glaberrima Steud.). Plant Sci. 173, 96–105. doi: 10.1016/j.plantsci.2007.04.003
Masood, A., Iqbal, N., and Khan, N. A. (2012). Role of ethylene in alleviation of cadmium-induced photosynthetic capacity inhibition by sulphur in mustard. Plant Cell Enviro. 35, 524–533. doi: 10.1111/j.1365-3040.2011.02432.x
Meyer, A. J., Dreyer, A., Ugalde, J. M., Feitosa-Araujo, E., Dietz, K. J., and Schwarzländer, M. (2020). Shifting paradigms and novel players in Cys-based redox regulation and ROS signaling in plants-and where to go next. Biol. Chem. 1:291. doi: 10.1515/hsz-2020-0291
Pattyn, J., Vaughan-Hirsch, J., and Van de Poel, B. (2021). The regulation of ethylene biosynthesis: a complex multilevel control circuitry. New Phytol. 229, 770–782. doi: 10.1111/nph.16873
Peng, X. X., and Yamauchi, M. (1993). Ethylene production in rice bronzing leaves induced by ferrous iron. Plant Soil 149, 227–234. doi: 10.1007/BF00016613
Riyazuddin, R., Verma, R., Singh, K., Nisha, N., Keisham, M., Bhati, K. K., et al. (2020). Ethylene: a master regulator of salinity stress tolerance in plants. Biomolecules 10:959. doi: 10.3390/biom10060959
Rodrigues, O., Reshetnyak, G., Grondin, A., Saijo, Y., Leonhardt, N., Maurel, C., et al. (2017). Aquaporins facilitate hydrogen peroxide entry into guard cells to mediate ABA-and pathogen-triggered stomatal closure. Proc. Natl. Acad. Sci. U. S. A. 114, 9200–9205. doi: 10.1073/pnas.1704754114
Wu, L., Zhang, Z., Zhang, H., Wang, X. C., and Huang, R. (2008). Transcriptional modulation of ethylene response factor protein JERF3 in the oxidative stress response enhances tolerance of tobacco seedlings to salt, drought, and freezing. Plant Physiol. 148, 1953–1963. doi: 10.1104/pp.108.126813
Wu, Y. S., and Yang, C. Y. (2019). Ethylene-mediated signaling confers thermotolerance and regulates transcript levels of heat shock factors in rice seedlings under heat stress. Botanical Stud. 60, 1–12. doi: 10.1186/s40529-019-0272-z
Yang, C.-Y., and Hong, C.-P. (2015). The NADPH oxidase Rboh D is involved in primary hypoxia signalling and modulates expression of hypoxia-inducible genes under hypoxic stress. Environ. Exp. Bot. 115, 63–72. doi: 10.1016/j.envexpbot.2015.02.008
Yang, S. L., Yu, P. L., and Chung, K. R. (2016). The glutathione peroxidase-mediated reactive oxygen species resistance, fungicide sensitivity and cell wall construction in the citrus fungal pathogen Alternaria alternate. Environ. Microbiol. 18, 923–935. doi: 10.1111/1462-2920.13125
Yang, W. S., SriRamaratnam, R., Welsch, M. E., Shimada, K., Skouta, R., Viswanathan, V. S., et al. (2014). Regulation of ferroptotic cancer cell death by GPX4. Cell 156, 317–331. doi: 10.1016/j.cell.2013.12.010
Yuan, M., Xu, Y. C., Niu, Y. Q., Zhou, H., An, Y. L., Jin, Q. J., et al. (2018). Efects of ethylene and NO on AsA-GSH in lotus under cadmium stress. Ying yong sheng tai xue bao J. Appl. Ecol. 29, 3433–3440. doi: 10.13287/j.1001-9332.201810.033
Keywords: ferroptosis, reactive oxygen species, glutathion (GSH), nicotinamide adenine dinucleotide phosphate oxidase, ethylene
Citation: Riyazuddin R and Gupta R (2021) Plausible Involvement of Ethylene in Plant Ferroptosis: Prospects and Leads. Front. Plant Sci. 12:680709. doi: 10.3389/fpls.2021.680709
Received: 15 March 2021; Accepted: 31 May 2021;
Published: 28 June 2021.
Edited by:
Md Tabibul Islam, Virginia Tech, United StatesReviewed by:
Jiayi Wang, Shanghai Jiao Tong University, ChinaCopyright © 2021 Riyazuddin and Gupta. This is an open-access article distributed under the terms of the Creative Commons Attribution License (CC BY). The use, distribution or reproduction in other forums is permitted, provided the original author(s) and the copyright owner(s) are credited and that the original publication in this journal is cited, in accordance with accepted academic practice. No use, distribution or reproduction is permitted which does not comply with these terms.
*Correspondence: Ravi Gupta, ZHIucmF2aWd1cHRhQGphbWlhaGFtZGFyZC5hYy5pbg==; cmF2aWd1cHRhMDdAeW1haWwuY29t
Disclaimer: All claims expressed in this article are solely those of the authors and do not necessarily represent those of their affiliated organizations, or those of the publisher, the editors and the reviewers. Any product that may be evaluated in this article or claim that may be made by its manufacturer is not guaranteed or endorsed by the publisher.
Research integrity at Frontiers
Learn more about the work of our research integrity team to safeguard the quality of each article we publish.