- 1Key Laboratory of Biology and Genetic Improvement of Horticultural Crops, Ministry of Agriculture, The Institute of Vegetables and Flowers, Chinese Academy of Agricultural Sciences, Beijing, China
- 2Open Key Laboratory of Horticultural Plant Physiology and Genetic Improvement, High School of Henan Province, College of Horticulture, Henan Agricultural University, Zhengzhou, China
- 3Institute of Horticulture, Henan Academy of Agricultural Sciences, Zhengzhou, China
Cuticular waxes play important roles in plant protection against various biotic and abiotic environmental stresses. The cuticular wax covering gives normal cabbage a glaucous appearance, but the appearance of waxless mutant is glossy green. Based on the present study, inheritance of the glossy green character of mutant HUAYOU2 follows a simple recessive pattern. Genetic analysis of an F2 population comprising 808 recessive individuals derived from HUAYOU2 (P1, maternal parent) and M36 (P2, paternal parent) revealed that a single recessive locus, BoWax1 (Brassica oleracea Wax 1), controls glossy green trait in B. oleracea. This locus was mapped to a region of 158.5 kb on chromosome C01. Based on nucleotide sequence analysis, Bol013612 was identified as the candidate gene for BoWax1. Sequencing results demonstrated that there is a deletion mutation of two nucleotides in the cDNA of Bol013612 of HUAYOU2, which may account for its glossy green trait. These results lay the foundation for functional analysis of BoWax1 and may accelerate research on wax metabolism in cabbage.
Introduction
The aerial epidermis of terrestrial plants is coated with a cuticle that consists of cutin and cuticular wax (Jetter et al., 2007). Cutin is composed of modified fatty acids and provides mechanical strength to the surface layer (Kerstiens, 1996; Beisson et al., 2012) The cuticular wax, which embeds in and covers the cutin polymer, is composed of very long chain fatty acids (VLCFAs) and their derivatives, including primary and secondary alcohols, alkanes, aldehydes, ketones, and wax esters (Rashotte et al., 1997; Lee and Suh, 2015). Together with cutin, cuticular wax plays an important role in the protection of plants from damage caused by environmental stresses, such as water deficiency (Kerstiens, 1996; Mawlong et al., 2015), insect attacks (Stork, 1980), pathogen infection (Wang et al., 2006), and UV irradiance (Shepherd and Wynne Griffiths, 2006).
Wax composition and content can vary according to species, ontogeny, and environment (Jenks and Ashworth, 1999; Laila et al., 2016). As noted previously, the major components of wax are VLCFAs and their derivatives, including alkanes, aldehydes, primary and secondary alcohols, ketones, and esters (Riederer and Müller, 2006; Bernard and Joubès, 2013). C16 and C18 acyl-CoAs are precursors in the biosynthesis of C20–C34 VLCFA-CoAs, which are formed by the addition of C2 units derived from malonyl-CoA by the enzymes of the fatty acid elongase (FAE) complex in the endoplasmic reticulum (ER) (Kunst and Samuels, 2009; Li-Beisson et al., 2013). The FAE complex consists of β-ketoacyl-CoA synthase (KCS), β-ketoacyl-CoA reductase (KCR), 3-hydroxyacyl-CoA dehydratase (HCD), and trans-2,3-enoyl-CoA reductase (ECR), The FAE complex catalyzes four consecutive enzymatic reactions, resulting in a two-carbon extension of the acyl chain during each elongation cycle (Kunst and Samuels, 2009; Li-Beisson et al., 2013). The produced VLCFA-CoAs produced by the FAE complex are modified by two distinct pathways: an alkane-forming pathway and an alcohol-forming pathway. Most of the wax components, including aldehydes, alkanes, secondary alcohols, and ketones, are biosynthesized in the alkane-forming pathway, while the products of the alcohol-forming pathway, including primary alcohols and wax esters, account for only 10–15% of the total wax in Arabidopsis stems (Rowland et al., 2006).
Many genes associated with cuticular wax metabolism and its regulation in Arabidopsis and other plants have been identified with the help of waxless mutants. These genes include the FAE-related enzymes KCS1, KCS2, KCS6, KCS9, KCS20, and KCR1 (Millar and Kunst, 1997; Millar et al., 1999; Todd et al., 1999; Fiebig et al., 2000; Pruitt et al., 2000; Hooker et al., 2002; Beaudoin and Kunst, 2009; Franke et al., 2009; Lee et al., 2009; Kim et al., 2013) and enzymes involved in the modification of VLCFA-CoAs, such as CER1, CER3, MAH1, CER4, and WSD1 (Rowland et al., 2006, 2007; Greer et al., 2007; Li et al., 2008; Bourdenx et al., 2011), which play various roles in wax biosynthesis. CER4 encodes an alcohol-forming fatty acyl-CoA reductase (FAR) that was reported to be responsible for the synthesis of primary alcohols in the epidermis of aerial tissues and in roots (Rowland et al., 2006).
Several genes implicated in wax biosynthesis of Brassica species have been reported in the recent studies. For example, the BrWax1 gene in Brassica rapa was mapped to linkage group A01, and Bra013809 was predicted to be the candidate gene for BrWax1 (Zhang et al., 2013). The BnaA.GL gene in Brassica napus was finely mapped to a linkage group close to the end of chromosome A9 (Pu et al., 2013). In cabbage, the BoGL1 gene was delimited to the end of chromosome C08 by a flanking marker, SSRC08–76, at a genetic distance of 0.2 cM (Liu D.et al., 2017). Although several genes in the alkane-forming pathway in Brassica species were isolated (Zhang et al., 2013; Liu Z. et al., 2017), no study concerning primary alcohol-forming pathway genes has been reported. In the present study, the BoWax1 gene was mapped to chromosome C01, and Bol013612 was identified as the candidate BoWax1 gene. Based on the lower expression level of Bol013612 and the differences in its sequence in the glossy green mutant HUAYOU2, the Bol013612 gene was predicted to participate in wax biosynthesis in the plant through the alcohol-forming pathway. These results provide insight into the elucidation of the alcohol-forming pathway and the molecular mechanism of wax biosynthesis in Brassica species.
Materials and Methods
Plant Materials
The plant materials in this study were provided by our team named the Cabbage and Broccoli Research Group, Institute of Vegetables and Flowers (IVF), Chinese Academy of Agricultural Sciences (CAAS) and were grown in the greenhouse in Changping (39°54′ N, 116°13′ E, Beijing, China).
Five glossy green cabbage mutants have been reported previously (Tang et al., 2015). HUAYOU2 is also a cabbage mutant with a glossy green appearance. The mutant plant HUAYOU2 was initially discovered from the highly inbred line HUA2, which was used as the wild type (WT) line in this research. HUAYOU2 (P1, maternal parent) was crossed with M36 (P2, paternal parent), an inbred line of Chinese kale (Brassica oleracea var. alboglabra) with a glaucous appearance, to generate the F1, F2, BC1P1, and BC1P2 populations for inheritance and mapping studies. The BC1P1 and BC1P2 populations were created by backcrossing the F1 plant (maternal parent) with HUAYOU2 (paternal parent) and M36 (paternal parent), respectively. The same F1 plant was used as the female parent in the backcrosses and was also used to obtain the F2 population.
SEM Analysis
Scanning electron microscopy (SEM) was employed to study the surfaces of the leaves of HUAYOU2 and WT plants. Fresh leaves from five-leaf-stage plants were fixed overnight in 2% glutaraldehyde, mounted on specimen stubs using double-sided tape and coated with gold particles in a SEMPrep2 sputter coater (Nanotech, England, United Kingdom). The phenotype was analyzed by SEM (S-4800, Hitachi, Japan) with a secondary electron detector at high voltage (10 kV).
DNA Isolation and PCR Amplification
Genomic DNA was extracted from fresh leaves using the cetyltrimethylammonium bromide (CTAB) method (Doyle, 1990). The concentration of each sample of genomic DNA was measured spectrophotometrically and adjusted to approximately 30 ng/μl.
Polymerase chain reaction (PCR) was carried out in a volume of 20 μl containing 2 μl of 10× PCR buffer (including Mg2+), 1.6 μl of dNTPs (2.5 mM each), 0.4 μl of Taq DNA polymerase (2.5 U/μl), 0.8 μl of forward primer (10 μM), 0.8 μl of reverse primer (10 μM), 2 μl of template DNA, and 10.4 μl of ddH2O. PCR was conducted on a Bio-Rad (United States) iCycler thermocycler as follows: initial denaturation step at 94°C for 5 min; 35 cycles at 94°C for 30 s, 55°C for 30 s, and 72°C for 45 s; and a final extension at 72°C for 5 min.
Inheritance Analysis and Mapping Strategy
The glossy and glaucous phenotypes in plants were identified visually and recorded at the five-leaf stage when the difference in appearance could be easily distinguished. Segregation ratios of the F2 and BC1P1 populations were analyzed with the Chi-square (χ2) test. Two DNA pools were constructed by mixing equal amounts of DNA from 12 glossy green and 12 glaucous F2 individuals. Then, 2000 pairs of simple sequence repeat (SSR) primers in the laboratory were used to successively screen the parental lines and the DNA pools. Primers that demonstrated polymorphism between the parents were subsequently used to screen the two pools. The linkage relationship between the target gene and the polymorphic primers was confirmed in 128 F2 individuals using JoinMap 4.0 (Van Ooijen, 2006). Linkage between markers and the BoWax1 gene was determined with the Kosambi mapping function, and a genetic map was constructed using MapDraw 2.1 software (Liu and Meng, 2003; Kosambi, 2016). To finely map the mutant gene, 50 pairs of new primers based on primary mapping results were developed. The new primers were used to analyze the parental lines and recombinants of 808 F2 recessive individuals. The sequences of all polymorphic markers used in this study are listed in Table 1
Sequence and Expression Analysis of the Bo13612 Gene
The InterPro annotations for B. oleracea and GO annotations for Arabidopsis thaliana for all the genes located within the mapped region were obtained from BRAD1 (Cheng et al., 2011). The expression level of the Bo13612 gene in WT and HUAYOU2 plants was determined by qPCR. Total RNA was extracted from fresh leaves at the five-leaf stage using the EasyPure® Plant RNA Kit (TRANS) as described by the manufacturer. First-strand cDNAs were synthesized in a 20-μl reaction volume containing approximately 7 μg of RNA and oligo(dT) primers using the TransScript One-Step gDNA Removal and cDNA Synthesis Kit (TRANS). Real-time PCR was performed with ABI SYBR Green on an ABI 7900HT Fast Real-Time PCR System (Applied Biosystems) according to the manufacturer’s instructions. The β-actin gene was used as an internal control (Okazaki et al., 2007). The 2-ΔΔCt method was used to analyze the data (Livak and Schmittgen, 2001). Three technical replicates were performed in this study. The sequence of the primers for qPCR, qPCR-013612 and qPCR-actin, are listed in Table 1. Each sample was tested in triplicate. The sequences of the Bo13612 gene were amplified using the gDNA and cDNA as templates with the KAPPA HiFi HotStart ReadyMix PCR Kit (Kappa Biosystems). The PCR products were sequenced by BGI TechSolutions, Co., Ltd., and the sequences were aligned using Multalin software2 (Corpet, 1988).
Primers used to amplify the gDNA sequence were designed using Primer Premier 5 software and date from the B. oleracea genome database (BRAD1). The primers were named Bol013612g1, Bol013612g2, Bol013612g3, Bol013612g4, and Bol013612g5 (Table 1) (Lalitha, 2000). The primer used to amplify the cDNA of the Bol013612 sequence was designed based on data from the B. oleracea transcriptome database3 and was named Bol013612c1 (Table 1) (Liu et al., 2014).
Results
The Morphological Appearance Is Altered in HUAYOU2
Regarding the coverage of cuticular wax, the appearance of the leaves in the WT cabbage was glaucous (Figure 1a). In contrast to WT, the leaves of HUAYOU2 tended to be glossy green, with little wax on the plant surface (Figure 1b). SEM was used to investigate the micro-characteristics and density of the wax crystals on the leaf surface. The results showed that the surface of the WT leaf is covered with compact wax crystals with a scale- and rod-like shape (Figure 1c). Unlike the WT, there are very few wax crystals covering the leaf surface of HUAYOU2 leaves, and these wax crystals tended to have a particle-type shaped (Figure 1d). This indicates that the glossy phenotype of HUAYOU2 is caused by a decrease in cuticular wax crystals, which is consistent with observations of many wax-deficient mutants in other plants (Pu et al., 2013).
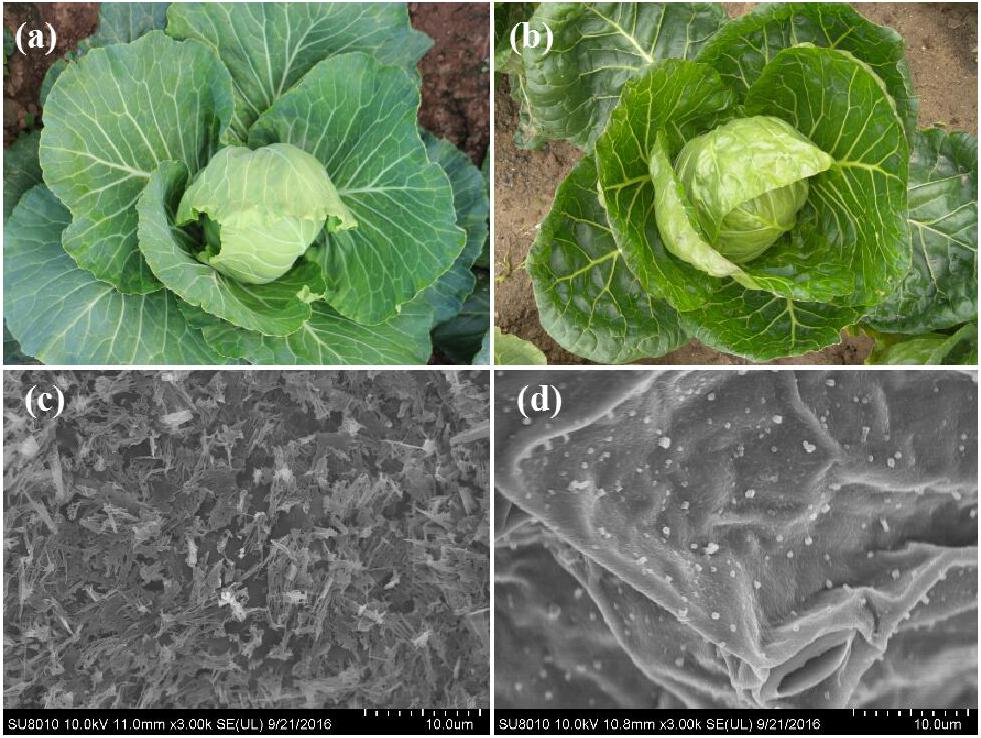
FIGURE 1. T Phenotypic characteristics of WT and HUAYOU2. (a) Appearance of leaf in WT; (b) appearance of leaf in HUAYOU2. (c) SEM showing wax crystals on the surface of leaves in WT; (d) wax crystals on the surface of leaves in HUAYOU2.
Inheritance Analysis of the Glossy Green Trait in HUAYOU2
The appearance of all 100 F1 plants from the crosses of HUAYOU2 and M36 was glaucous, indicating that the glaucous trait was dominant over the glossy green trait. Among 3328 F2 individuals, 2520 had glaucous leaves, and 808 had glossy green leaves, corresponding to a segregation ratio of 3:1 by the Chi-square test. The segregation ratio in the BC1P1 population was 1:1 (210 glaucous:222 glossy), and all the BC1P2 plants had a glaucous appearance (Table 2). These results demonstrated that inheritance of the glossy green trait in HUAYOU2 follows a monogenic recessive pattern.
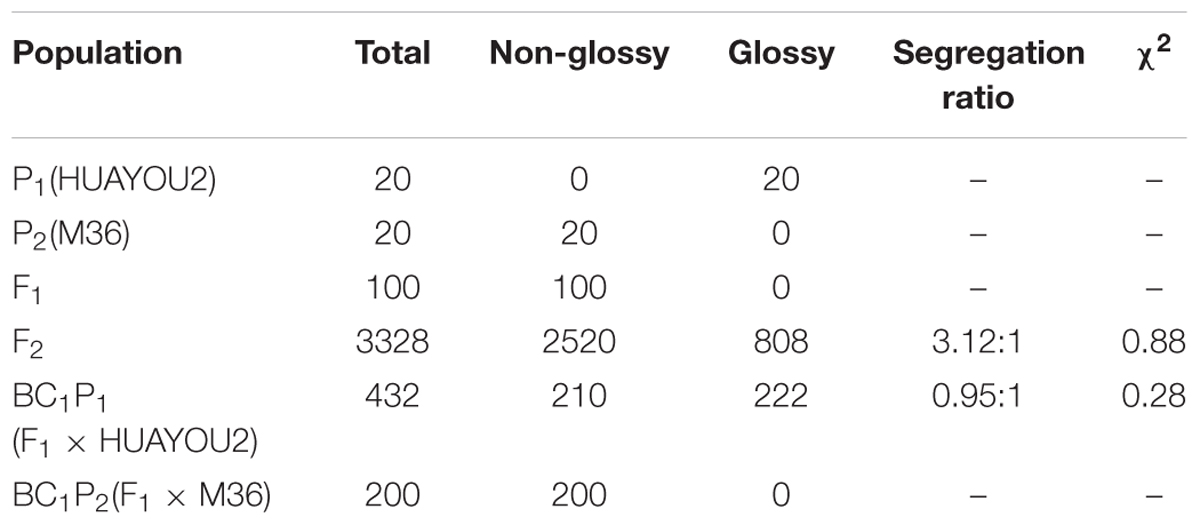
TABLE 2. Segregation of glaucous and glossy traits and the χ2 goodness-of-fit test of segregation in BC1P1 and F2 populations.
Fine Genetic and Physical Mapping of the BoWax1 Gene
A total of 2000 SSR markers available in the laboratory were used to identify polymorphisms in the parental lines (HUAYOU2 and M36) and in the glossy and glaucous DNA bulks. As a result, 114 pairs of primers were identified that showed polymorphism between the parental lines, but only BolD000046 was identified as being polymorphic between two DNA pools. Marker BolD000046 was mapped close to BoWax1 using 128 segregating F2 population plants (Figure 2A).
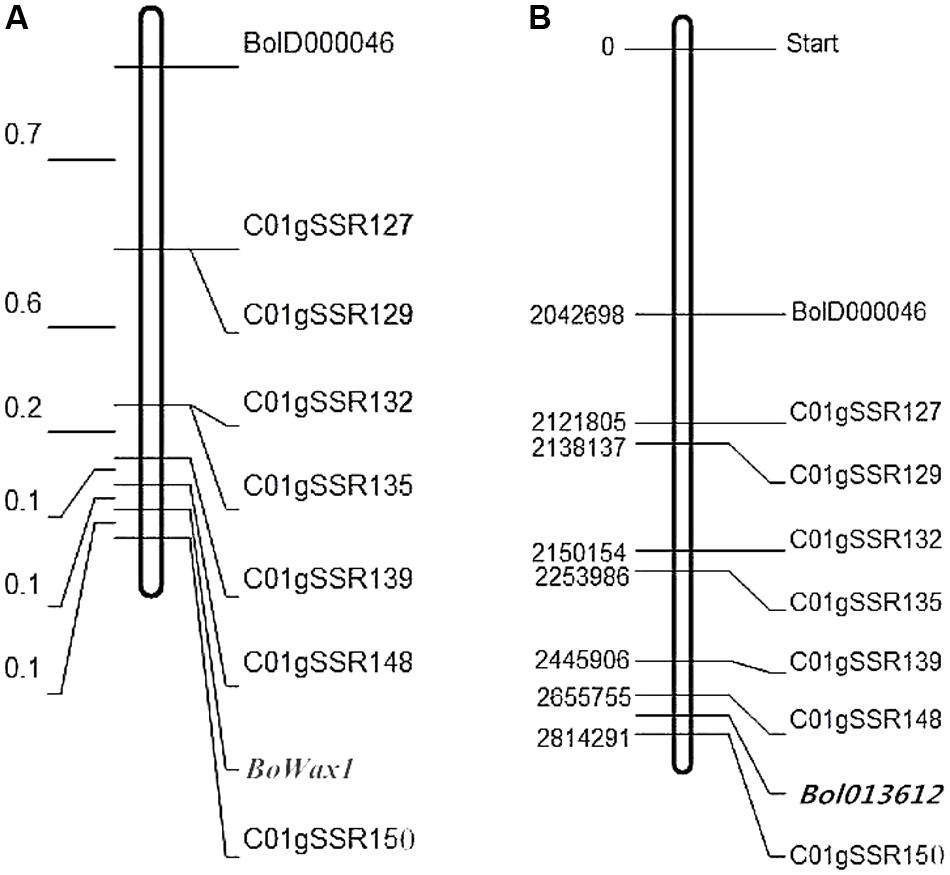
FIGURE 2. Genetic map (left, units: cM) of BoWax1 on chromsome C01 (A). The corresponding physical map positions (right, units: Mb) are also shown (B).
To saturate the genetic map containing BoWax1, 50 pairs of primers near primer BolD000046 were designed based on the physical position of marker BolD000046. Eight of the 50 newly developed primers showed polymorphism between parental lines HUAYOU2 and M36. The eight SSRs were subsequently screened to investigate recombination using 808 F2 recessive individuals. Based on information from the polymorphic markers and the recombinants, the genetic distance between the two closest flanking makers, C01gSSR148 and C01gSSR150 (Figure 2A), was found to be 0.2 cM. The physical distance between primers C01gSSR148 and C01gSSR150 is 158.5 kb (Figure 2B). All of the primers are on chromosome C01 and the order of the markers on the genetic map is consistent with that on the physical map.
Candidate Gene Analysis
Analysis of the genomic region between C01gSSR148 and C01gSSR150 was carried out. Gene annotations in BRAD and alignments with A. thaliana showed that gene Bol013612 contains the conserved FAR domain, which plays important roles in wax biosynthesis and is present in proteins that are responsible for primary alcohols synthesis (Rowland et al., 2006). At4g3370 is an example of an Arabidopsis gene that contains the conserved FAR domain and exhibits the same glossy stem wax phenotype in two mutant lines (cer4-1 and cer4-2). A deletion and/or rearrangement of the promoter region occurs in the cer4-1 mutant and a T-DNA insertion is present in the fourth intron of At4g33790 (at nucleotide 1,960 relative to the start codon) in the cer4-2 mutant (Rowland et al., 2006). According to the homologous analysis results, the Bol013612 gene was predicted to participate in the alcohol-forming pathway. Aside from Bol013612, no other genes in the mapped region were found to be related to plant wax metabolism (Gene analysis and annotations are supplied in Additional file 1: Supplementary 2). The Bol013612 gene was selected as the candidate for expression analysis using qPCR. The results showed that the expression level of Bol013612 is significantly decreased compared with that of the WT, implying that transcription of the Bol013612 gene was affected (Figure 3).
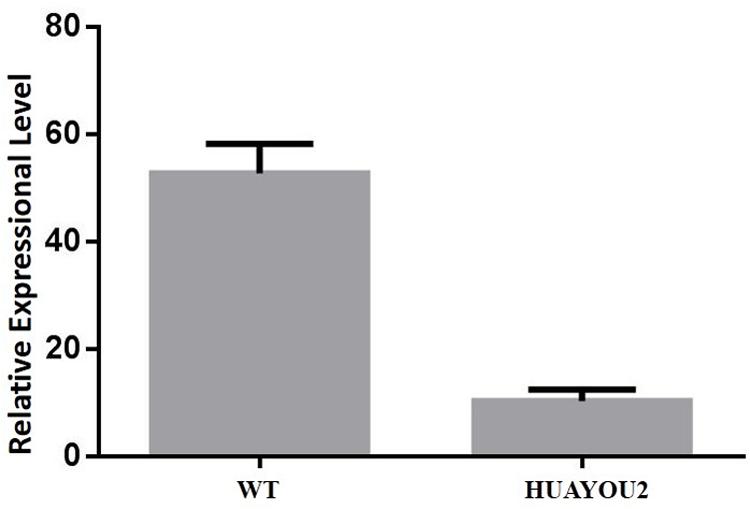
FIGURE 3. Expression level (Y axis) of gene Bol013612 in HUAYOU2 and WT. qPCR analysis showing expression level in HUAYOU2 is significantly decreased compared with that of the WT. Standard errors are also presented.
The Bol013612 gene was sequenced to further determine whether the glossy green trait of HUAYOU2 is caused by a mutation in Bol013612. The Bol013612 gene, numbered MG808275 in NCBI database, is 5,448 bp in length, including 10 exons and 9 introns (Figure 4A). The The coding sequence (CDS) of Bol013612 is 1,482 bp in length. The sequencing results showed that there was a deletion of two bases in the eighth exon of HUAYOU2 (sequences of WT and HUAYOU2 are supplied in Additional file 1: Supplementary 1). The deletion mutation was suspected of causing a frameshift mutation in the Bol013612 gene and a premature stop codon (Figures 4B,C). This might disrupt the function of this gene, which may influence the wax biosynthesis and subsequently cause the glossy appearance of HUAYOU2.
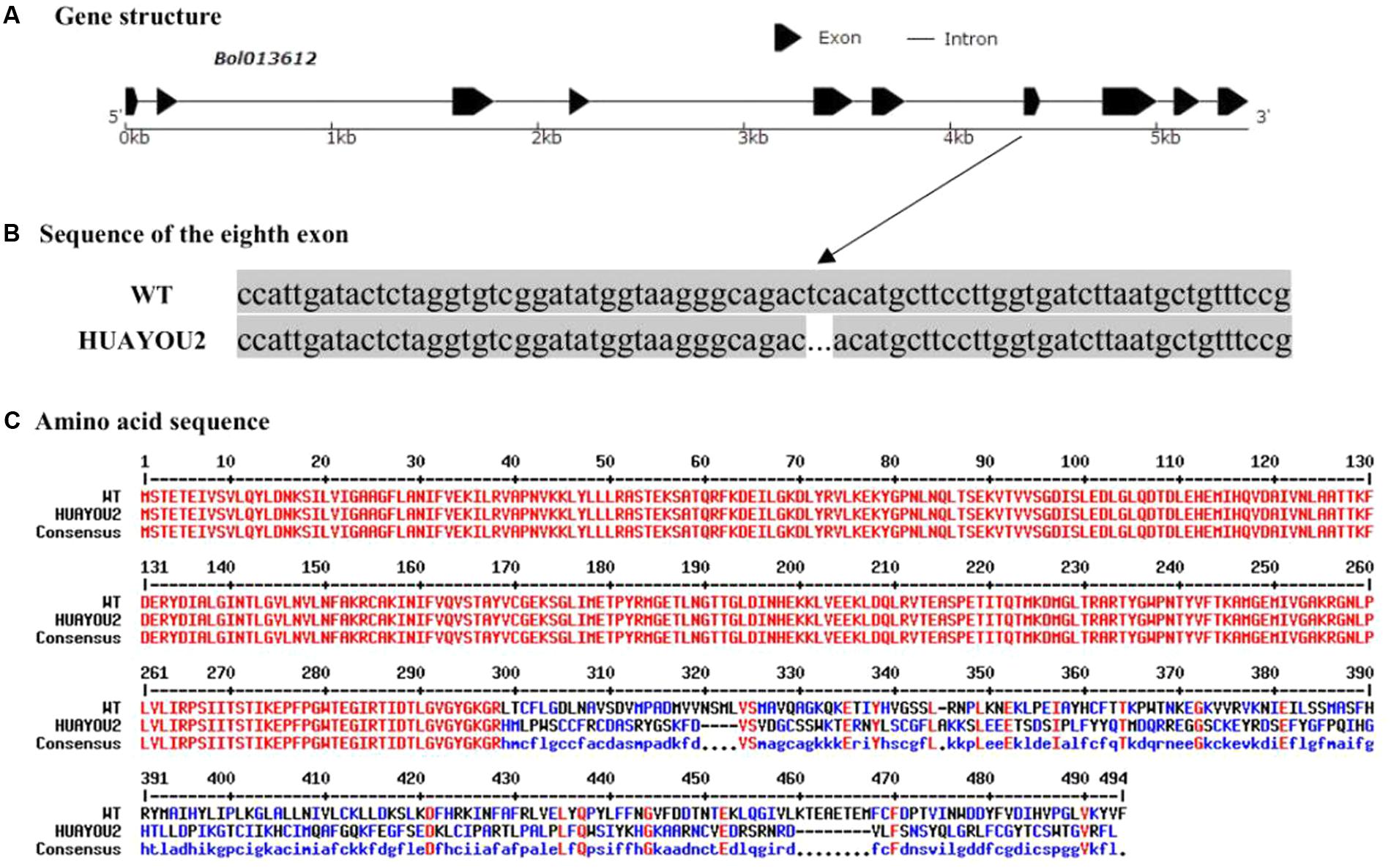
FIGURE 4. The gene structure and alleic variation in Bol013612 (A). Sequence alignment of exon 8 showing two bp deletion in HUAYOU2 as compared to WT (B). Amino sequence alignments showing premature stop codon (indicated with arrow) due to a frameshift mutation in Bol013612 gene in HUAYOU2 (C).
Discussion
Waxes are secreted onto the surface or into the interior of cuticles to form the first barrier against various environmental stresses (Millar et al., 1999). Since Dellaert discovered the first waxy cuticle eceriferum mutant (cer) in A. thaliana (Dellaert et al., 1979), many genes related to wax synthesis have been isolated, including wax synthesis-related enzymes (CER4, CER6, CER10, FATB, and GL8) (Fiebig et al., 2000; Bonaventure and Ohlrogge, 2003; Dietrich et al., 2005; Zheng et al., 2005; Rowland et al., 2006), wax transporters (CER5 and WBC11) (Pighin and Samuels, 2004; Bird et al., 2007; Panikashvili et al., 2007), and transcription factors (SHN1, MYB30, MYB96, and WXP1) (Broun et al., 2004; Zhang et al., 2005; Raffaele et al., 2008; Seo et al., 2011). Several genes implicated in Brassica plant wax biosynthesis pathway were reported in recent studies. Though these studies identify the same glossy waxless appearance, the inheritance patterns are different. For example, the waxless trait of the Chinese cabbage mutant 08A235-2 is controlled by a single recessive gene (Zhang et al., 2013), same as that of HUAYOY2 in this study. By contrast, a dominant mutant allele controls the waxless trait in Brassica napus mutant 6-1025 (Pu et al., 2013). The glossy appearance of HUAYOU2 in this study is also determined by a single recessive gene, but sequence and functional analysis of the candidate gene revealed that the novel locus for glossiness on chromosome C01 is involved in the primary alcohol-forming pathway, in contrast with the gene BnaA.GL in Brassica napus (Pu et al., 2013) and the gene BrWax1 in Brassica rapa (Zhang et al., 2013).
Cer4 mutant and cabbage mutant LD10GL are fertile in contrast with the cabbage glossy green mutant 10Q-961, in which only a few seeds were produced after self-pollination (Liu Z. et al., 2017). Enzymes containing the conserved FAR domain usually act on medium- and long-chain fatty acids and have been reported to be involved in the biosynthesis of plant cuticular wax (Rowland et al., 2006). In Arabidopsis, FAR has specificity for very-long-chain fatty acids and is present in enzymes responsible for the synthesis of primary alcohols in the epidermal cells of aerial tissues and in the roots (Rowland et al., 2006). CER4 is one of the genes encoding an FAR domain in Arabidopsis. The glossy wax-deficient trait is displayed in Arabidopsis mutant cer4 due to decreases in the production primary alcohols and wax esters.
By studying the cabbage mutant HUAYOU2, BoWax1 was finely mapped and the target gene was identified. In the F2 population, gene BoWax1 was localized to a region of 158.5 kb on cabbage chromosome C01. The sequence variation and abnormal expression of the Bol013612 gene imply that Bol013612 may be the target gene that accounts for the glossy green trait of HAUYOU2. These results will help us further elucidate the formation of the glossy trait in HUAYOU2 and accelerate wax biosynthesis studies in cabbage. More research needs to be carried out in subsequent work, such as functional studies of BoWax1, investigation of the metabolic mechanism of cabbage wax biosynthesis and identification of other genes involved.
Author Contributions
DL, LY, and ZF designed and supervised the study. XD, ZZL, JT, MZ, YZ, HL, YL, and ZSL participated in its design. DL participated in the mapping, RT-PCR, and sequence analysis. JT, ZZL, YL, and ZSL participated in the statistical analysis. DL and LY wrote the manuscript. All the authors discussed the results and contributed to the manuscript. All authors read and approved the final manuscript.
Funding
This work was financially supported by grants from the National Natural Science Foundation of China (31672155), the National High Technology Research and Development Program of China (863 Program) (2012AA100101), the Key Projects in the National Science and Technology Pillar Program during the Twelfth Five-Year Plan Period (2012BAD02B01), the Science and Technology Innovation Program of the Chinese Academy of Agricultural Sciences (CAAS-ASTIP-2013-IVFCAAS), the Modern Agro-Industry Technology Research System (CARS-25-B-01), and the Project of Science and Technology Commission of Beijing Municipality (Z141105002314020-1).
Conflict of Interest Statement
The authors declare that the research was conducted in the absence of any commercial or financial relationships that could be construed as a potential conflict of interest.
Supplementary Material
The Supplementary Material for this article can be found online at: https://www.frontiersin.org/articles/10.3389/fpls.2018.00309/full#supplementary-material
Footnotes
- ^ http://brassicadb.org/brad/index.php
- ^ http://multalin.toulouse.inra.fr/multalin/multalin.html
- ^ http://www.ncbi.nlm.nih.gov/geo/query/acc.cgi?acc=GSE42891
References
Beaudoin, F., and Kunst, L. (2009). Functional characterization of the Arabidopsis β-ketoacyl-coenzyme a reductase candidates of the fatty acid elongase. Plant Physiol. 150, 1174–1191. doi: 10.1104/pp.109.137497
Beisson, F., Li-Beisson, Y., and Pollard, M. (2012). Solving the puzzles of cutin and suberin polymer biosynthesis. Curr. Opin. Plant Biol. 15, 329–337. doi: 10.1016/j.pbi.2012.03.003
Bernard, A., and Joubès, J. (2013). Arabidopsis cuticular waxes: advances in synthesis, export and regulation. Prog. Lipid Res. 52, 110–129. doi: 10.1016/j.plipres.2012.10.002
Bird, D., Beisson, F., Brigham, A., Shin, J., Greer, S., Jetter, R., et al. (2007). Characterization of Arabidopsis ABCG11/WBC11, an ATP binding cassette (ABC) transporter that is required for cuticular lipid secretion. Plant J. 52, 485–498. doi: 10.1111/j.1365-313X.2007.03252.x
Bonaventure, G., and Ohlrogge, J. B. (2003). Disruption of the FATB gene in Arabidopsis demonstrates an essential role of saturated fatty acids in plant growth. Plant Cell 15, 1020–1033. doi: 10.1105/tpc.008946
Bourdenx, B., Bernard, A., Domergue, F., Pascal, S., Léger, A., Roby, D., et al. (2011). Overexpression of Arabidopsis ECERIFERUM1 promotes wax very-long-chain alkane biosynthesis and influences plant response to biotic and abiotic stresses. Plant Physiol. 156, 29–45. doi: 10.1104/pp.111.172320
Broun, P., Poindexter, P., Osborne, E., Jiang, C. Z., and Riechmann, J. L. (2004). WIN1, a transcriptional activator of epidermal wax accumulation in Arabidopsis. Proc. Natl. Acad. Sci. U.S.A. 101, 4706–4711. doi: 10.1073/pnas.0305574101
Cheng, F., Liu, S., Wu, J., Fang, L., Sun, S., Liu, B., et al. (2011). BRAD, the genetics and genomics database for Brassica plants. BMC Plant Biol. 11:136. doi: 10.1186/1471-2229-11-136
Corpet, F. (1988). Multiple sequence alignment with hierarchical clustering. Nucleic Acids Res. 16, 10881–10890. doi: 10.1093/nar/16.22.10881
Dellaert, L., Van Es, J., and Koornneef, M. (1979). Eceriferum mutants in Arabidopsis thaliana (L.) Heynh. II. Phenotypic and genetic analysis. Arabid. Inf. Serv. 16, 10–26.
Dietrich, C. R., Perera, M. A., Yandeau-Nelson, M. D., Meeley, R. B., Nikolau, B. J., and Schnable, P. S. (2005). Characterization of two GL8 paralogs reveals that the 3-ketoacyl reductase component of fatty acid elongase is essential for maize (Zea mays L.) development. Plant J. 42, 844–861. doi: 10.1111/j.1365-313X.2005.02418.x
Fiebig, A., Mayfield, J. A., Miley, N. L., Chau, S., Fischer, R. L., and Preuss, D. (2000). Alterations in CER6, a gene identical to CUT1, differentially affect long-chain lipid content on the surface of pollen and stems. Plant Cell 12, 2001–2008. doi: 10.1105/tpc.12.10.2001
Franke, R., Höfer, R., Briesen, I., Emsermann, M., Efremova, N., Yephremov, A., et al. (2009). The DAISY gene from Arabidopsis encodes a fatty acid elongase condensing enzyme involved in the biosynthesis of aliphatic suberin in roots and the chalaza-micropyle region of seeds. Plant J. 57, 80–95. doi: 10.1111/j.1365-313X.2008.03674.x
Greer, S., Wen, M., Bird, D., Wu, X., Samuels, L., Kunst, L., et al. (2007). The cytochrome P450 enzyme CYP96A15 is the midchain alkane hydroxylase responsible for formation of secondary alcohols and ketones in stem cuticular wax of Arabidopsis. Plant Physiol. 145, 653–667. doi: 10.1104/pp.107.107300
Hooker, T. S., Millar, A. A., and Kunst, L. (2002). Significance of the expression of the CER6 condensing enzyme for cuticular wax production in Arabidopsis. Plant Physiol. 129, 1568–1580. doi: 10.1104/pp.003707
Jenks, M. A., and Ashworth, E. N. (1999). Plant epicuticular waxes: function, production, and genetics. Hortic. Rev. 23, 1–68.
Jetter, R., Kunst, L., and Samuels, A. L. (2007). “Composition of plant cuticular waxes,” in Annual Plant Reviews: Biology of the Plant Cuticle, Vol. 23, eds M. Riederer and C. Müller (Oxford: Blackwell Publishing Ltd.), 145–181.
Kerstiens, G. (1996). Cuticular water permeability and its physiological significance. J. Exp. Bot. 47, 1813–1832. doi: 10.1093/jxb/47.12.1813
Kim, J., Jung, J. H., Lee, S. B., Go, Y. S., Kim, H. J., Cahoon, R., et al. (2013). Arabidopsis 3-ketoacyl-coenzyme a synthase9 is involved in the synthesis of tetracosanoic acids as precursors of cuticular waxes, suberins, sphingolipids, and phospholipids. Plant Physiol. 162, 567–580. doi: 10.1104/pp.112.210450
Kosambi, D. D. (2016). The Estimation of Map Distances from Recombination Values. Berlin: Springer, 125–130. doi: 10.1007/978-81-322-3676-4_16
Kunst, L., and Samuels, L. (2009). Plant cuticles shine: advances in wax biosynthesis and export. Curr. Opin. Plant Biol. 12, 721–727. doi: 10.1016/j.pbi.2009.09.009
Laila, R., Robin, A. H. K., Yang, K., Park, J.-I., Suh, M. C., Kim, J., et al. (2016). Wax content, wax composition and expression of wax biosynthetic genes in Brassica oleracea var. capitata vary with leaf development and genotype. Front. Plant Sci. 7:1972. doi: 10.3389/fpls.2016.01972
Lalitha, S. (2000). Primer premier 5. Biotech Softw. Internet Rep. 1, 270–272. doi: 10.1089/152791600459894
Lee, S. B., Go, Y. S., Bae, H.-J., Park, J. H., Cho, S. H., Cho, H. J., et al. (2009). Disruption of glycosylphosphatidylinositol-anchored lipid transfer protein gene altered cuticular lipid composition, increased plastoglobules, and enhanced susceptibility to infection by the fungal pathogen Alternaria brassicicola. Plant Physiol. 150, 42–54. doi: 10.1104/pp.109.137745
Lee, S. B., and Suh, M. C. (2015). Advances in the understanding of cuticular waxes in Arabidopsis thaliana and crop species. Plant Cell Rep. 34, 557–572. doi: 10.1007/s00299-015-1772-2
Li, F., Wu, X., Lam, P., Bird, D., Zheng, H., Samuels, L., et al. (2008). Identification of the wax ester synthase/acyl-coenzyme A: diacylglycerol acyltransferase WSD1 required for stem wax ester biosynthesis in Arabidopsis. Plant Physiol. 148, 97–107. doi: 10.1104/pp.108.123471
Li-Beisson, Y., Shorrosh, B., Beisson, F., Andersson, M. X., Arondel, V., Bates, P. D., et al. (2013). Acyl-lipid metabolism. Arabidopsis Book 11:e0161. doi: 10.1199/tab.0161
Liu, D., Tang, J., Liu, Z., Dong, X., Zhuang, M., Zhang, Y., et al. (2017). Fine mapping of BoGL1, a gene controlling the glossy green trait in cabbage (Brassica oleracea L. Var. capitata). Mol. Breed. 37:69. doi: 10.1007/s11032-017-0674-0
Liu, R. H., and Meng, J. L. (2003). MapDraw: a microsoft excel macro for drawing genetic linkage maps based on given genetic linkage data. Yi Chuan 25, 317–321.
Liu, S., Liu, Y., Yang, X., Tong, C., Edwards, D., Parkin, I. A., et al. (2014). The Brassica oleracea genome reveals the asymmetrical evolution of polyploid genomes. Nat. Commun. 5:3930. doi: 10.1038/ncomms4930
Liu, Z., Fang, Z., Zhuang, M., Zhang, Y., Lv, H., Liu, Y., et al. (2017). Fine-mapping and analysis of Cgl1, a gene conferring glossy trait in cabbage (Brassica oleracea L. var. capitata). Front. Plant Sci. 8:239. doi: 10.3389/fpls.2017.00239
Livak, K. J., and Schmittgen, T. D. (2001). Analysis of relative gene expression data using real-time quantitative PCR and the 2-Δ Δ CT method. Methods 25, 402–408. doi: 10.1006/meth.2001.1262
Mawlong, I., Ali, K., Srinivasan, R., Rai, R., and Tyagi, A. (2015). Functional validation of a drought-responsive AP2/ERF family transcription factor-encoding gene from rice in Arabidopsis. Mol. Breed. 35:163. doi: 10.1007/s11032-015-0290-9
Millar, A. A., Clemens, S., Zachgo, S., Giblin, E. M., Taylor, D. C., and Kunst, L. (1999). CUT1, an Arabidopsis gene required for cuticular wax biosynthesis and pollen fertility, encodes a very-long-chain fatty acid condensing enzyme. Plant Cell 11, 825–838. doi: 10.1105/tpc.11.5.825
Millar, A. A., and Kunst, L. (1997). Very-long-chain fatty acid biosynthesis is controlled through the expression and specificity of the condensing enzyme. Plant J. 12, 121–131. doi: 10.1046/j.1365-313X.1997.12010121.x
Okazaki, K., Sakamoto, K., Kikuchi, R., Saito, A., Togashi, E., Kuginuki, Y., et al. (2007). Mapping and characterization of FLC homologs and QTL analysis of flowering time in Brassica oleracea. Theor. Appl. Genet. 114, 595–608. doi: 10.1007/s00122-006-0460-6
Panikashvili, D., Savaldigoldstein, S., Mandel, T., Yifhar, T., Franke, R. B., Höfer, R., et al. (2007). The Arabidopsis DESPERADO/AtWBC11 transporter is required for cutin and wax secretion. Plant Physiol. 145, 1345–1360. doi: 10.1104/pp.107.105676
Pighin, J. A., and Samuels, A. L. (2004). Plant cuticular lipid export requires an ABC transporter. Science 306, 702–704. doi: 10.1126/science.1102331
Pruitt, R. E., Vielle-Calzada, J. P., Ploense, S. E., Grossniklaus, U., and Lolle, S. J. (2000). FIDDLEHEAD, a gene required to suppress epidermal cell interactions in Arabidopsis, encodes a putative lipid biosynthetic enzyme. Proc. Natl. Acad. Sci. U.S.A. 97, 1311–1316. doi: 10.1073/pnas.97.3.1311
Pu, Y., Gao, J., Guo, Y., Liu, T., Zhu, L., Xu, P., et al. (2013). A novel dominant glossy mutation causes suppression of wax biosynthesis pathway and deficiency of cuticular wax in Brassica napus. BMC Plant Biol. 13:215. doi: 10.1186/1471-2229-13-215
Raffaele, S., Vailleau, F., Léger, A., Joubès, J., Miersch, O., Huard, C., et al. (2008). A MYB transcription factor regulates very-long-chain fatty acid biosynthesis for activation of the hypersensitive cell death response in Arabidopsis. Plant Cell 20, 752–767. doi: 10.1105/tpc.107.054858
Rashotte, A. M., Jenks, M. A., Nguyen, T. D., and Feldmann, K. A. (1997). Epicuticular wax variation in ecotypes of Arabidopsis thaliana. Phytochemistry 45, 251–255. doi: 10.1016/S0031-9422(96)00792-3
Riederer, M., and Müller, C. (2006). Biology of the Plant Cuticle. Oxford: Blackwell. doi: 10.1002/9780470988718
Rowland, O., Lee, R., Franke, R., Schreiber, L., and Kunst, L. (2007). The CER3 wax biosynthetic gene from Arabidopsis thaliana is allelic to WAX2/YRE/FLP1. FEBS Lett. 581, 3538–3544. doi: 10.1016/j.febslet.2007.06.065
Rowland, O., Zheng, H., Hepworth, S. R., Lam, P., Jetter, R., and Kunst, L. (2006). CER4 encodes an alcohol-forming fatty acyl-coenzyme A reductase involved in cuticular wax production in Arabidopsis. Plant Physiol. 142, 866–877. doi: 10.1104/pp.106.086785
Seo, P. J., Lee, S. B., Suh, M. C., Park, M. J., Go, Y. S., and Park, C. M. (2011). The MYB96 transcription factor regulates cuticular wax biosynthesis under drought conditions in Arabidopsis. Plant Cell 23, 1138–1152. doi: 10.1105/tpc.111.083485
Shepherd, T., and Wynne Griffiths, D. (2006). The effects of stress on plant cuticular waxes. New Phytol. 171, 469–499. doi: 10.1111/j.1469-8137.2006.01826.x
Stork, N. E. (1980). Role of waxblooms in preventing attachment to Brassicas by the mustard beetle, Phaedon cochleariae. Entomol. Exp. Appl. 28, 100–107. doi: 10.1111/j.1570-7458.1980.tb02992.x
Tang, J., Liu, D., Liu, Z., Yang, L., Fang, Z., Liu, Y., et al. (2015). Preliminary study of the characteristics of several glossy cabbage (Brassica oleracea var. capitata L.) mutants. Hortic. Plant J. 1, 93–100.
Todd, J., Postbeittenmiller, D., and Jaworski, J. G. (1999). KCS1 encodes a fatty acid elongase 3-ketoacyl-CoA synthase affecting wax biosynthesis in Arabidopsis thaliana. Plant J. Cell Mol. Biol. 17, 119–130. doi: 10.1046/j.1365-313X.1999.00352.x
Van Ooijen, J. (2006). JoinMap 4: Software for the Calculation of Genetic Linkage Maps in Experimental Populations. Wageningen: Kyazma BV.
Wang, F., Zhang, P., Qiang, S., and Xu, L.-L. (2006). Interaction of plant epicuticular waxes and extracellular esterases of Curvularia eragrostidis during infection of Digitaria sanguinalis and Festuca arundinacea by the fungus. Int. J. Mol. Sci. 7, 346–357. doi: 10.3390/i7090346
Zhang, J. Y., Broeckling, C. D., Blancaflor, E. B., Sledge, M. K., Sumner, L. W., and Wang, Z. Y. (2005). Overexpression of WXP1, a putative Medicago truncatula AP2 domain-containing transcription factor gene, increases cuticular wax accumulation and enhances drought tolerance in transgenic alfalfa (Medicago sativa). Plant J. 42, 689–707. doi: 10.1111/j.1365-313X.2005.02405.x
Zhang, X., Liu, Z., Wang, P., Wang, Q., Yang, S., and Feng, H. (2013). Fine mapping of BrWax1, a gene controlling cuticular wax biosynthesis in Chinese cabbage (Brassica rapa L. ssp. pekinensis). Mol. Breed. 32, 867–874. doi: 10.1007/s11032-013-9914-0
Keywords: cabbage, glossy green mutant, inheritance, gene mapping, candidate gene
Citation: Liu D, Dong X, Liu Z, Tang J, Zhuang M, Zhang Y, Lv H, Liu Y, Li Z, Fang Z and Yang L (2018) Fine Mapping and Candidate Gene Identification for Wax Biosynthesis Locus, BoWax1 in Brassica oleracea L. var. capitata. Front. Plant Sci. 9:309. doi: 10.3389/fpls.2018.00309
Received: 20 June 2017; Accepted: 22 February 2018;
Published: 30 April 2018.
Edited by:
Maoteng Li, Huazhong University of Science and Technology, ChinaReviewed by:
Liezhao Liu, Southwest University, ChinaHong An, University of Missouri, United States
Zhongyun Piao, Shenyang Agricultural University, China
Harsh Raman, New South Wales Department of Primary Industries, Australia
Copyright © 2018 Liu, Dong, Liu, Tang, Zhuang, Zhang, Lv, Liu, Li, Fang and Yang. This is an open-access article distributed under the terms of the Creative Commons Attribution License (CC BY). The use, distribution or reproduction in other forums is permitted, provided the original author(s) and the copyright owner are credited and that the original publication in this journal is cited, in accordance with accepted academic practice. No use, distribution or reproduction is permitted which does not comply with these terms.
*Correspondence: Limei Yang, ylmcaas@163.com Zhiyuan Fang, fangzhiyuan@caas.cn
†These authors have contributed equally to this work and shared first authorship.