- 1College of Life Sciences, Sun Yat-sen University, Guangzhou, China
- 2Institute of Marine Research, Bergen, Norway
- 3College of Life Science and Technology, Guangxi University, Nanning, China
Haematococcus pluvialis can be used as a green additive in aquafeeds due to it contains rich astaxanthin and polyunsaturated fatty acid. In the present study, a newly strain of H. pluvialis GXU-A23 with high concentration of astaxanthin was firstly isolated by a newly culture strategy in our laboratory. In addition, H. pluvialis GXU-A23 was applied in the Litopenaeus vannamei feed for determining whether it has positive effects on the growth performance, antioxidant and anti-inflammatory status, metabolic capacity and mid-intestine morphology of juvenile L. vannamei. Shrimp with 0.63 g approximately initial body weight were fed diets supplemented with/without 50 g/kg H. pluvialis GXU-A23. After 8 weeks feeding intervention, significantly higher growth performance of L. vannamei was obtained in the H. pluvialis GXU-A23 treatment group compared to the control group (p < 0.05). At the same time, L. vannamei fed with H. pluvialis GXU-A23 acquired significantly better antioxidant and anti-inflammatory status than the control group (p < 0.05). In addition, higher RNA expression level of hepatopancreas digestive enzyme, hepatopancreas lipid and glucose metabolic enzymes as well as better mid-intestine morphology were found in the H. pluvialis GXU-A23 treatment group than the control group (p < 0.05). These results indicated that 50 g/kg H. pluvialis GXU-A23 was suitable for the L. vannamei feed, which could improve the growth performance, antioxidant and anti-inflammatory status, metabolic capacity and mid-intestine morphology of juvenile L. vannamei.
Introduction
The speedy development of aquaculture provided considerable high-quality protein for human (Costello et al., 2020; Cottrell et al., 2021). In fact, high production is attributed to high density farming (Bostock et al., 2010). On the other hand, many aquaculture environments were polluted due to the improvement of human activities (Zhang et al., 2019). However, these two factors might cause the growth of pathogen microorganisms, such as white spot syndrome virus (WSSV) (Verbruggen et al., 2016) and Vibrio parahaemolyticus (Soto-Rodriguez et al., 2015), in water and thus inducing the low survival rate of Litopenaeus vannamei, which severely limited the development of the shrimp industry. In order to reduce adverse effects of shrimp as caused by pathogen microorganisms, antibiotics were widely used in recent 20 years (Romero et al., 2012). However, limitations of antibiotic used in aquaculture are antibiotic resistance and drug residues (Sørum, 2005; Santos and Ramos, 2016). Therefore, to promote the development of aquaculture industry, proper green additives must be exploited for substituting the antibiotic used during the farming.
The flesh pigment is one of the essential factors which might influence the shrimp price since customers generally regard the optimal pigment as high quality (Diler and Gokoglu, 2004). However, crustaceans are unable to biosynthesize carotenoids de novo, while they can obtain and convert pigment from the feed into carotenoids and then deposit in the flesh (Niu et al., 2009). Therefore, optimization of the flesh pigment could be taken into consideration when it comes to exploiting a shrimp additive.
Astaxanthin, one of the keto carotenoids, is mainly existing in algae (like Haematococcus pluvialis, Chlorella zofingiensis), bacteria (like Phaffia rhodozyma) and crustaceans (Johnson and Lewis, 1979; Ip and Chen, 2005). The antioxidant property of astaxanthin was demonstrated more 100–500 folds than vitamin E to inhibit the lipid peroxidation in vitro (Ni et al., 2015). Dietary supplementation of astaxanthin bring many benefits to aquatic animals. For example, improving the growth performance (Wang et al., 2018), reducing the interval of molt cycle (Petit et al., 1997), enhancing the antioxidant and anti-inflammatory capacity (Xie J. et al., 2020), optimization of shrimp pigment (Ju et al., 2011).
Newly strain of H. pluvialis GXU-A23 with high concentration astaxanthin (33 g/kg) was isolated and cultured by a newly two-step batch culture strategy in our laboratory (Wang et al., 2019). In this method, modified Bold’s Basal medium (mBBM) (Wang et al., 2019) with 9.0 mM urea was provided to culture the H. pluvialis GXU-A23. Compared to the modified BG-11 medium (mBG-11) (Gao et al., 2016), the H. pluvialis could obtain remarkably higher astaxanthin content in the mBBM (Domınguez-Bocanegra et al., 2004; Nahidian et al., 2018). Apart from that, the H. pluvialis showed the better astaxanthin accumulation property in the urea as nitrogen source than NaNO3 and NH4HCO3 (Wang et al., 2019). In our previous study, the two-step batch culture strategy was used to successfully culture H. pluvialis JNU35, which contained 31.70 g/kg astaxanthin (Zhao et al., 2021). Since astaxanthin was mostly produced by nature H. pluvialis, the newly strain of H. pluvialis GXU-A23 could remarkably improve the production industry of astaxanthin as well as reduce the product budget. In addition, this microalga also could be used as a green additive in aquafeeds and beneficial for sustainable development of aquaculture.
The present study aims to evaluate whether there are beneficial effects of the newly isolated strain of H. pluvialis GXU-A23 on L. vannamei. Therefore, an 8 weeks feeding experiment was conducted to investigate effects of H. pluvialis GXU-A23 on the growth performance, antioxidant and anti-inflammatory status, metabolic capacity and mid-intestine morphology of juvenile L. vannamei. These results might provide a reference for feed formulation of L. vannamei.
Materials and Methods
Haematococcus pluvialis GXU-A23 Culture
H. pluvialis GXU-A23 was obtained from Zixi Mountain of Chuxiong (YunNan, China), and these microalgae were bacteria-free cultured in our laboratory. The culture method of H. pluvialis GXU-A23 was following the manuscript by Wang et al. (2019). Briefly, H. pluvialis GXU-A23 was scale-up cultured in a flat glass photobioreactor (length: 240 cm; height: 120 cm) with 6 cm light paths. mBBM (9.0 mM urea), bubbled gas of 1% CO2 (v/v) as well as 100 μmol/m2 s−1 continuous unilateral lighting were provided to culture the H. pluvialis GXU-A23 for 15 days. Afterward, H. pluvialis GXU-A23 was transferred into the same sized photobioreactor with nitrogen-free medium, 3 cm light paths and continuous bilateral illumination of 400 μmol m−2 s−1 for 15 days to accumulate astaxanthin. Afterward, red cells of H. pluvialis GXU-A23 were harvested by auto-precipitation and freeze-dried using freezing dryer. The freeze-drying biomass of H. pluvialis GXU-A23 contained 33 g/kg astaxanthin.
Diet Preparation
As shown in Table 1, two isonitrogen and isolipids experimental diets were formulated with/without H. pluvialis GXU-A23 (D1: 0; D2: 50 g/kg) respectively. Dietary ingredients were purchased from Guangzhou Chengyi Company Ltd. (Guangzhou, China). The level of H. pluvialis GXU-A23 used in the present study was referred to our previous study (Zhao, et al., 2020), which was normally the highest concentration of additive used in the aquafeed.
Measured nutrition values of diets were 7% crude lipid and 40% protein approximately (Table 1). The method of diet preparation was following the reported by Yu et al. (2016). Briefly, all dried ingredients and oils were weighted following table 1 and then completely homogeneous in the Hobart-type mixer (A-200T Mixer, Canada). Then, deionized water (250 ml/kg dried ingredients mixture) was added into the above ingredient to thoroughly mix for 15 min. Then, diets (1.2 mm diameters) were extruded using the pelletizer (South China University of Technology, China). Then, diets were heated in the 50°C ventilated oven for 120 min. Then, diets were stored at −20°C and kept away from the light until the feeding trial.
Feeding Experiment
Juvenile L. vannamei were obtained and cultured at the experimental station of the Chinese Academy of Fishery Science (Lingshui, China). Before the trial, shrimp were acclimated to the experimental environment by feeding with D1 diet for 30 days 320 lively shrimp with an initial body weight of 0.63 g approximately were distributed randomly into the recirculating water system with eight cylindrical fiber tanks (300 L). Each of diets was randomly allocated to quadruplicate tanks. The feeding frequency was three times daily at 06:00, 12:00, and 18:00 with 8% of total shrimp weight and lasted for 8 weeks. During the period of feeding, environmental conditions were maintained as follows: water temperature: 26.8–28.1°C; pH: 7.5–7.7; salinity: 29—32‰; dissolved oxygen: > 7.0 mg/L; total ammonia nitrogen: < 0.1 mg/L; sulfide: < 0.05 mg/L. Natural light-dark (12–12 h) cycle was used during the feeding trial.
Sample Collection
After 8 weeks feeding, L. vannamei were starved for 24 h. Then, all shrimp from each tank were weighed, counted and then recorded. Then, eight individuals from each tank were randomly collected and anesthetized (MS-222, 98%, Sigma, United States) for obtaining the blood sample. Then, hepatopancreas samples were removed for analysis of antioxidant parameters and mRNA expression; same sections of mid-intestine were removed and fixed in 4% paraformaldehyde (Beyotime, China) for intestinal histological examination. Blood samples were stored at the fridge (4°C, 12 h) and then centrifuged (7,100 g, 10 min, at 4°C) to obtain hemolymph for antioxidant parameters analysis. All hepatopancreas and hemolymph samples were separated rapidly and then maintained at −80°C until examination.
Astaxanthin Analysis of Haematococcus pluvialis GXU-A23 and Feeds
Astaxanthin contents of H. pluvialis GXU-A23 and feeds were determined by spectrophotometrically as the description by Li, et al. (2012).
Chemical Analysis of Feeds
Chemical compositions (moisture, crude lipid, crude protein and ash) of feeds were determined according to standard methods of AOAC (Horwitz, 2010). Briefly, moisture was analyzed by drying in the ventilated oven at 105°C until constant weight; crude lipid examination was performed following the Soxhlet extractor method (Soxtec System HT6, Tecator, Sweden); crude protein (N × 6.25) was measured following the Kjeldahl method (1030—Autoanalyzer; Tecator, Höganäs, Sweden); ash was analyzed using muffle furnace at 550°C until constant weight.
Quantification of Hepatopancreas and Hemolymph Parameters Related to Antioxidant Status
Hepatopancreas were homogenized and centrifuged according to the description of Fang et al. (2021). Briefly, hepatopancreas were homogenized (1:9) in phosphate buffer. Afterward, above homogenates were centrifuged (10 min, 4°C, 1200 g) and then supernatants were collected.
Enzyme activities of total superoxide dismutase (T-SOD) (A001-1), total antioxidant capacity (T-AOC) (A015–2), glutathione peroxidase (GSH-PX) (A005-1) as well as the content of malondialdehyde (MDA) (A003-1) were measured according to instructions of reagent (Nanjing Jiancheng Bioengineering Institute, Nanjing, China) (Instructions of reagent were shown in additional files).
Examination of Mid-intestine Histology
Mid-intestine sections were obtained and stained following the manuscript of Zhao et al. (2020). Briefly, tissue sections were stained using the hematoxylin and eosin (Beyotime, China), and mid-intestine histology were observed using the microscope (Olympus CKX41 microscope, Tokyo, Japan). The villus height and the mucosal layer thickness are equating to the average value of randomly selected eight villi and eight mucosal per slide respectively (Chen et al., 2020).
mRNA Isolation and Expression Quantification
Hepatopancreas total RNA isolation and mRNA expression examination were performed following our previous manuscript (Fang et al., 2019). Briefly, the total RNA was isolated using Trizol® reagent (Invitrogen, United States) following the manufacturer’s instruction. 1% agarose gel electrophoresis and spectrophotometer (NanoDrop 2000; Thermo Fisher, United States) were used to ascertain RNA quality and quantity, respectively. Afterward, cDNA was synthesized using the PrimeScript TM RT Reagent kit (Takara, Japan), following the manufacturer’s instruction. Real-time PCR for the target genes were performed using SYBR® Premix Ex TaqTM II (Takara, Japan) and quantified on the LightCycler 480 (Roche Applied Science, Basel, Switzerland).
Primers related to the present study were listed in table 2. The elongation factor a (ef1a) was used as a housekeeping gene for RNA expression analysis (Guzmán-Villanueva et al., 2020). The relative mRNA expression of target genes was determined using the 2−ΔΔCT method (Livak and Schmittgen, 2001).
Statistical Analysis
Experimental data in the present study are shown as means ± standard error (SE). Data were checked for normality and homogeneity of variance in the software of SPSS 22.0 (Chicago, United States) and then analyzed by independent-sample t-test. p < 0.05 was regarded as the significant difference between groups.
Result
Growth Performance and Feed Utilization
As shown in Table 3, dietary H. pluvialis GXU-A23 supplementation significantly altered the growth performance of L. vannamei. Significantly higher final body weight (FBW), weight gain rate (WGR) and specific growth rate (SGR) of L. vannamei were found in the D2 group than that of the D1 group (p < 0.05). However, dietary H. pluvialis GXU-A23 supplementation was unable to change the feed conversion ratio (FCR) of L. vannamei (p > 0.05). After 8 weeks feeding, survival rate (SR) of L. vannamei fed with/without H. pluvialis GXU-A23 were 96% approximately (p > 0.05).
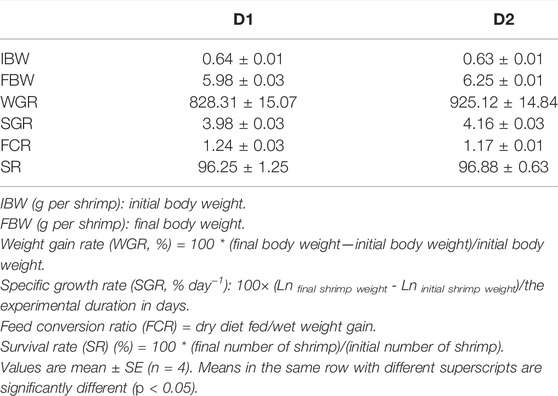
TABLE 3. Growth performance and feed utilization of L. vannamei fed diet supplemented with/without H. pluvialis GXU-A23 for 56 days.
Oxidative Status Parameters
Antioxidant parameters of L. vannamei under different dietary intervention were shown in Table 4. Results showed that enzyme activities of hepatopancreas T-SOD, hepatopancreas GSH-PX as well as hemolymph T-SOD were significantly decreased in the D2 group than that in the D1 group (p < 0.05). Meanwhile, relatively lower hepatopancreas MDA content (p > 0.05) and remarkably lower hemolymph MDA content (p < 0.05) were found in the dietary H. pluvialis GXU-A23 supplementation group than the control group. No statistical differences of hepatopancreas T-AOC, hemolymph T-AOC and hemolymph GSH-PX were obtained between two experimental groups (p > 0.05).
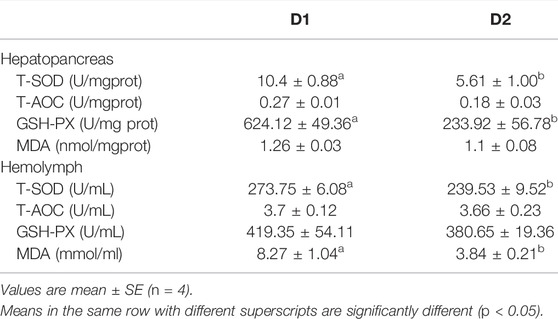
TABLE 4. Hepatopancreas and hemolymph antioxidant status parameters of L. vannamei fed diet supplemented with/without H. pluvialis GXU-A23 for 56 days.
Hepatopancreas mRNA Expression Related to Immunity
mRNA expression levels of genes related to antioxidation of L. vannamei fed diet supplemented with/without H. pluvialis GXU-A23 were shown in Figure 1. Compared to the control group, the dietary H. pluvialis GXU-A23 supplementation group obtained significantly lower mRNA expression levels of sod, gsh-px and cat (p < 0.05).
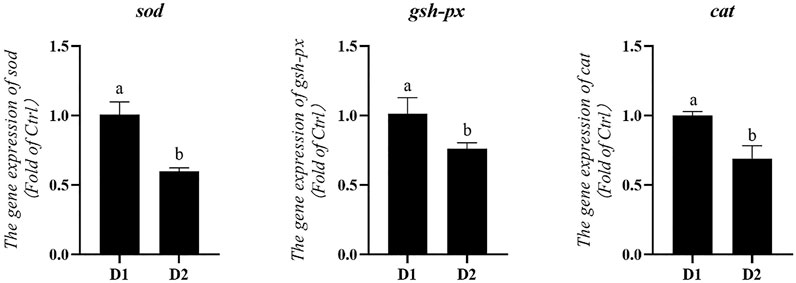
FIGURE 1. Hepatopancreas mRNA levels of antioxidative genes of L. vannamei fed diet supplemented with/without H. pluvialis GXU-A23 for 56 days. Values are mean ± SE (n = 4). The small letters indicated significant differences at p < 0.05.
mRNA expression levels of anti-inflammatory genes of L. vannamei fed diet supplemented with/without H. pluvialis GXU-A23 were shown in Figure 2. Remarkably lower mRNA expression level of relish was obtained in the D2 group compared to the control group (p < 0.05). No statistical difference of the rho mRNA expression level was observed between two groups (p > 0.05).
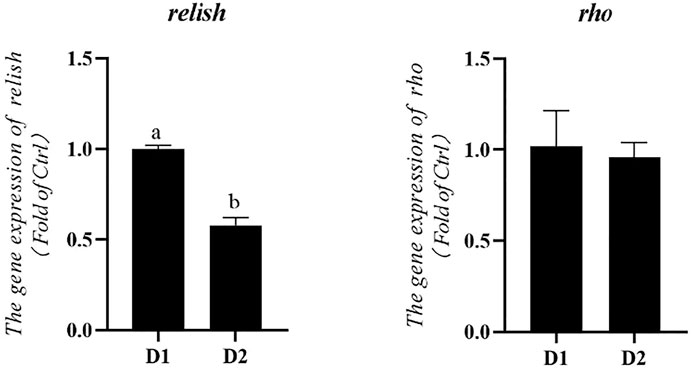
FIGURE 2. Hepatopancreas mRNA levels of anti-inflammatory genes of L. vannamei fed diet supplemented with/without H. pluvialis GXU-A23 for 56 days. Values are mean ± SE (n = 4). The small letters indicated significant differences at p < 0.05.
Hepatopancreas mRNA Expression Related to Digestive and Metabolic Enzymes
mRNA expression levels of digestive enzymes of L. vannamei fed diet supplemented with/without H. pluvialis GXU-A23 were shown in Figure 3. The mRNA expression level of chymotrypsin of L. vannamei was significantly increased after dietary H. pluvialis GXU-A23 intervention (p < 0.05). However, no statistical difference of trypsin mRNA expression level was observed between two groups (p > 0.05).
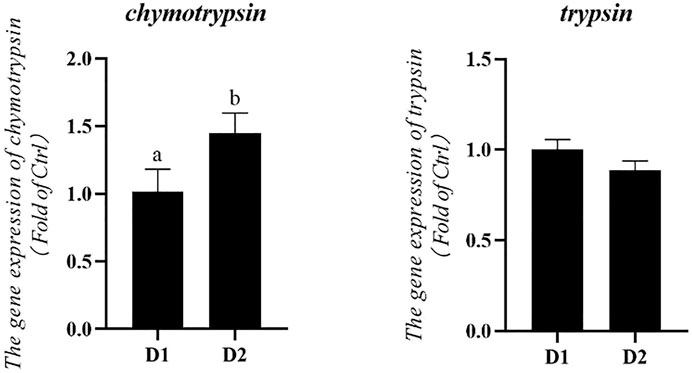
FIGURE 3. Hepatopancreas mRNA levels of digestive enzyme genes of L. vannamei fed diet supplemented with/without H. pluvialis GXU-A23 for 56 days. Values are mean ± SE (n = 4). The small letters indicated significant differences at p < 0.05.
Dietary H. pluvialis GXU-A23 supplementation significantly altered the mRNA expression level of metabolic enzymes of L. vannamei (Figure 4). mRNA expression levels of hexokinase (hk) and fatty acid synthase (fas) were significantly higher in the H. pluvialis GXU-A23 treatment group compared to the control group (p < 0.05).
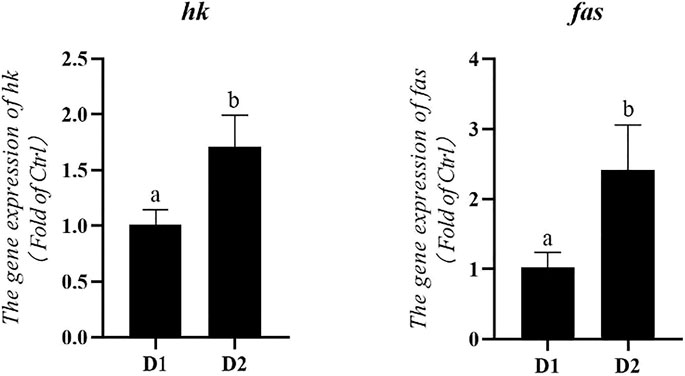
FIGURE 4. Hepatopancreas mRNA levels of metabolic enzymes genes of L. vannamei fed diet supplemented with/without H. pluvialis GXU-A23 for 56 days. Values are mean ± SE (n = 4). The small letters indicated significant differences at p < 0.05.
Light Microscopy Observation of Mid-intestine Morphology
Light microscopy of mid-intestine morphology of L. vannamei exposed to different dietary treatment for 56 days was shown in Figure 5. Results showed that the intestinal mucosal layer thickness and villa height of L. vannamei fed with H. pluvialis GXU-A23 was significantly higher than that of the control group (p < 0.05).
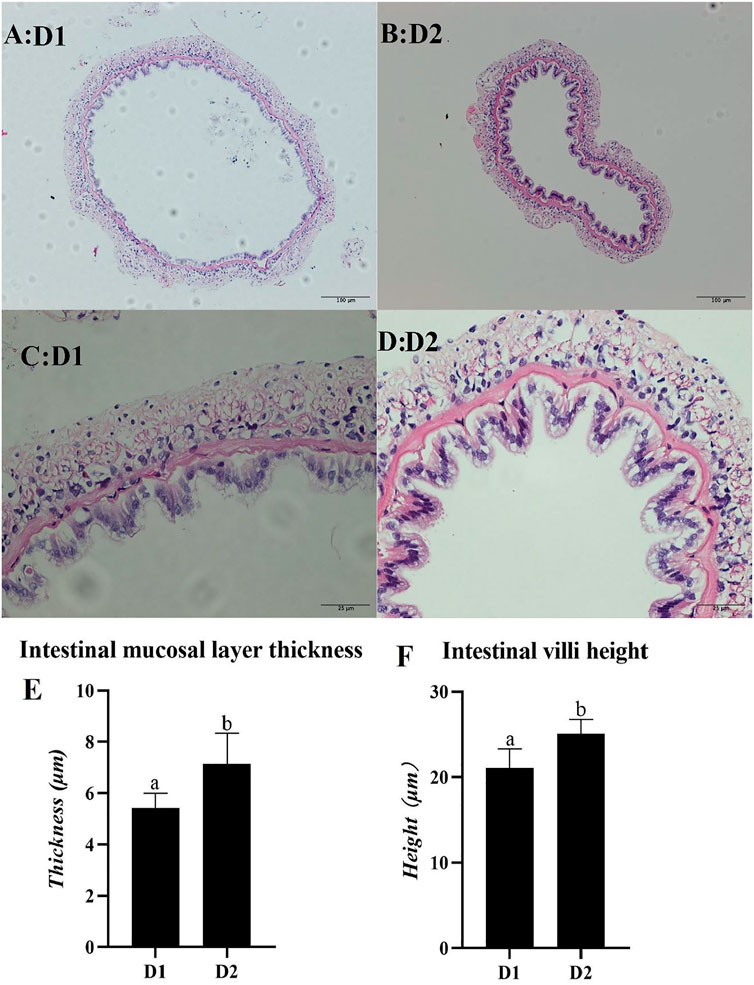
FIGURE 5. Light microscopy of mid-intestine morphology of L. vannamei fed diet supplemented with/without H. pluvialis GXU-A23 for 56 days. The scale bars of picture (A,B) were 100 μm, while the scale bars of picture (C,D) were 25 μm respectively. Picture (E,F) represents the intestinal mucosal layer thickness and intestinal villi height of L. vannamei respectively. Values are mean ± SE (n = 4). The small letters indicated significant differences at p < 0.05.
Discussion
In recent years, microalgae had been gained widely attention in aquafeeds due to it is the green additive with high nutrition (Roy and Pal, 2015). Different microalgae might contain different nutrients, such as high lipid and protein (Aaronson et al., 1980; Webb, 1983), proper amino acid pattern (Becker, 2004), polysaccharide (Chu et al., 1982; Lama et al., 1996), pigments (Metting, 1996) and vitamins (Brown and Farmer, 1994). Supplementation of microalgae in aquafeed can partly substitute for minerals (Fabregas and Herrero, 1986), fishmeal and fish oil (Shah et al., 2018). Microalgae as an aquafeed additive for improving the growth performance and immunity of animals was also widely reported (Cerezuela et al., 2012; Reyes-Becerril et al., 2013, 2014).
In the present study, L. vannamei fed with the H. pluvialis GXU-A23 diet obtained the better growth performance (WG and SGR) compared to that of the control group. Similar results also reported in Pseudosciaena crocea (Li et al., 2014), Trachinotus ovatus (Zhao et al., 2021), L. vannamei (initial weight: ∼ 1.0 g) (Ju et al., 2012). H. pluvialis GXU-A23 contains astaxanthin with 3S-3′S type which is the same structure in Salmo salar and other aquatic animals (Higuera-Ciapara et al., 2006). The main reason for astaxanthin could improve the growth performance of aquatic animals is that this pigment could mediate intermediate metabolism, resulting in enhancing nutrients utilization and thus optimization of the growth performance of L. vannamei (Han et al., 2018). However, H. pluvialis was unable to alter the growth performance in post-larval L. vannamei (5 days after metamorphosing of mysis stage) (Xie et al., 2018), L. vannamei (initial weight: 0.94–0.99 g) (Ju et al., 2011) and Cichlasoma citrinellum (Pan and Chien, 2009). These different results might be attributed to the source and dose used of dietary H. pluvialis, the growth stage of animals as well as the experimental environment. Besides, the hepatopancreas mRNA expression level of chymotrypsin was upregulated in the H. pluvialis GXU-A23 feeding group than that of the control group. High expression of protease could improve the digestion and absorption of protein, and thus enhancing growth (Zokaeifar et al., 2012). Apart from digestive enzyme, the intestine morphology was strongly contributed to the growth of shrimp. Higher intestinal villi height represented the larger contact surface area between the intestine and nutrients (Emami et al., 2012), and the increasing of intestinal mucosal layer thickness meaning the improvement digestion and absorption ability of shrimp (Chen et al., 2020). In the present study, remarkably higher intestinal villi height and intestinal mucosal layer thickness were found in the dietary H. pluvialis GXU-A23 treatment group compared to the control group, indicating that H. pluvialis GXU-A23 has protective effect on mid-intestine morphology of L. vannamei and thus improves the growth performance of shrimp, which is consistent with the present result.
Generally, aquatic animals have the poor glucose utilization capacity because of the low level of insulin released (Chen et al., 2020). However, glycolysis is the only pathway of glucose metabolism in animals (Li et al., 2018). Among them, hepatic HK was a fundamental limitation enzyme in the glycolysis process (Lu et al., 2018). In the present study, the H. pluvialis GXU-A23 feeding L. vannamei group obtained higher hepatopancreas mRNA expression level of hk than the control group, indicating that dietary H. pluvialis GXU-A23 supplementation could improve the utilization capacity of blood glucose for satisfying higher energy requirement. Apart from glucose metabolism, lipid metabolism also plays a major role in health of aquatic animals. In particular, FAS plays an essential role in lipogenesis by catalyzing the de novo biosynthesis of fatty acids (Lu et al., 2018). In the present study, higher mRNA expression level of fas in the L. vannamei fed with H. pluvialis GXU-A23 group than that in the control group, indicating dietary H. pluvialis GXU-A23 supplementation was beneficial for the synthesis of hepatopancreas fatty acids.
When shrimp was subjected to environmental pressures, the breathing burst would be occurred to produce reactive oxygen species (ROS) for attacking invading microorganisms (Zhao et al., 2020). However, overproduction ROS might attack normal cells and then cause oxidative damages to shrimp. To avoid the riskiness of ROS, cells have developed an antioxidant system which involve various antioxidant enzymes, like SOD, GSH-PX, CAT (Zhao et al., 2017). In the present study, significantly lower antioxidant enzyme activities (hepatopancreas T-SOD, hepatopancreas GSH-PX and hemolymph T-SOD) as well as hepatopancreas mRNA expression levels (sod, gsh-px and cat) were obtained in dietary H. pluvialis GXU-A23 treatment group compared to the control group. Lower antioxidant parameters in the D2 group was attributed to the astaxanthin in H. pluvialis GXU-A23, which contains the ionone ring with hydroxyl and keto and thus it could scavenge ROS in crustaceans (Ambati et al., 2014). As a result, L. vannamei was unnecessary to produce more antioxidant enzymes. MDA is a lipid peroxidation product which is generally regarded as an essential parameter to evaluate the oxidative damage of animals (Larbi Ayisi et al., 2018). In the present study, L. vannamei fed with H. pluvialis GXU-A23 diet obtained the remarkably lower hemolymph MDA compared to the control group, indicating H. pluvialis GXU-A23 could prohibit the lipid peroxidation of cells and enhance the antioxidant capacity of L. vannamei.
Except for the antioxidant system, aquatic animals also responses to environmental stresses by regulating inflammatory responses (Fazelan et al., 2020). If subjected to stress, inflammatory mediators (like cytokines or prostaglandins) would be produced in cells for mediating the inflammatory system to remove detrimental irritations (Boltana et al., 2018). However, excessive inflammation response might lead to various pathological diseases, such as fever (Evans et al., 2015), loss of tissue function (Takeuchi and Akira, 2010). NF-κB signal pathway is closely correlated with the pathogenesis of inflammatory diseases (Yu et al., 2020). Among them, relish was a key NF-κB family protein in L. vannamei (Qiu et al., 2014). In the present study, the mRNA expression level of relish in the dietary H. pluvialis GXU-A23 supplementation group was significantly higher than that of the control group, indicating H. pluvialis GXU-A23 have a positive effect on inhibiting the NF-κB singal pathway. The prohibition of NF-κB pathway might narrow the production of pro-inflammatory cytokines, resulting in mitigating inflammatory responses (Xie et al., 2011). Therefore, H. pluvialis GXU-A23 plays an important role in alleviating inflammatory responses of L. vannamei.
Conclusion
Overall, our present study demonstrated that dietary H. pluvialis GXU-A23 supplementation enhanced the growth performance of L. vannamei by improving antioxidant and anti-inflammatory status, metabolic metabolism and mid-intestine morphology. Therefore, 50 g/kg H. pluvialis GXU-A23 was recommended for the L. vannamei feed.
Data Availability Statement
The original contributions presented in the study are included in the article/Supplementary Material, further inquiries can be directed to the corresponding authors.
Ethics Statement
The animal study was reviewed and approved by The Experimental Animal Ethics Committee of Sun-Yat San University.
Author Contributions
HF, JN, and WZ designed the study, LH culture the Haematococcus pluvialis GXU-A23, HF and ZZ analyzed data, HF carried out the experiment and wrote this paper, ZW modified the language.
Funding
This work was supported by the Fund of China Agriculture Research System of MOF and MARA 48 (CARS 48), and Project of Science and Technology of Guangdong Province (2019B110209005), and Project of Science and Technology of Guangdong Province (2021B0202050002), and Youth Science and Technology Innovation Talent of Guangdong TeZhi Plan Talent (2019TQ05N129).
Conflict of Interest
The authors declare that the research was conducted in the absence of any commercial or financial relationships that could be construed as a potential conflict of interest.
Publisher’s Note
All claims expressed in this article are solely those of the authors and do not necessarily represent those of their affiliated organizations, or those of the publisher, the editors and the reviewers. Any product that may be evaluated in this article, or claim that may be made by its manufacturer, is not guaranteed or endorsed by the publisher.
Acknowledgments
Thanks to my supervisors and others who assisted my experiment.
References
Aaronson S., Berner T., Dubinsky Z. (1980). “Microalgae as a Source of Chemicals and Natural Products,” in Algae Biomass Prod. Amsterdam, Netherlands: Elsevier Press. Editors G. Shelef, and C. J. Soeder.
Ambati R., Phang S.-M., Ravi S., Aswathanarayana R. (2014). Astaxanthin: Sources, Extraction, Stability, Biological Activities and its Commercial Applications-A Review. Mar. Drugs 12, 128–152. doi:10.3390/md12010128
Becker W., (2004). “18 Microalgae in Human and Animal Nutrition,” in Handbook of Microalgal Culture: Biotechnology and Applied Phycology (United States: Wiley Online Library).
Boltana S., Sanhueza N., Donoso A., Aguilar A., Crespo D., Vergara D., et al. (2018). The Expression of TRPV Channels, Prostaglandin E2 and Pro-inflammatory Cytokines during Behavioural Fever in Fish. Brain Behav. Immun. 71, 169–181. doi:10.1016/j.bbi.2018.03.023
Bostock J., McAndrew B., Richards R., Jauncey K., Telfer T., Lorenzen K., et al. (2010). Aquaculture: Global Status and Trends. Phil. Trans. R. Soc. B 365, 2897–2912. doi:10.1098/rstb.2010.0170
Brown M. R., Farmer C. L. (1994). Riboflavin Content of Six Species of Microalgae Used in Mariculture. J. Appl. Phycol. 6, 61–65. doi:10.1007/bf02185905
Cerezuela R., Guardiola F. A., Meseguer J., Esteban M. Á. (2012). Enrichment of Gilthead Seabream (Sparus aurata L.) Diet with Microalgae: Effects on the Immune System. Fish. Physiol. Biochem. 38, 1729–1739. doi:10.1007/s10695-012-9670-9
Chen M., Chen X.-Q., Tian L.-X., Liu Y.-J., Niu J. (2020). Beneficial Impacts on Growth, Intestinal Health, Immune Responses and Ammonia Resistance of pacific white Shrimp (Litopenaeus Vannamei) Fed Dietary Synbiotic (Mannan Oligosaccharide and Bacillus Licheniformis). Aquacult. Rep. 17, 100408. doi:10.1016/j.aqrep.2020.100408
Chu F.-L. E., Dupuy J. L., Webb K. L. (1982). Polysaccharide Composition of Five Algal Species Used as Food for Larvae of the American Oyster, Crassostrea virginica. Aquacul. 29, 241–252. doi:10.1016/0044-8486(82)90138-7
Costello C., Cao L., Gelcich S., Cisneros-Mata M. Á., Free C. M., Froehlich H. E., et al. (2020). The Future of Food from the Sea. Nature 588, 95–100. doi:10.1038/s41586-020-2616-y
Cottrell R. S., Metian M., Froehlich H. E., Blanchard J. L., Sand Jacobsen N., McIntyre P. B., et al. (2021). Time to Rethink Trophic Levels in Aquaculture Policy. Rev. Aquac. 13, 1583–1593. doi:10.1111/raq.12535
Diler I., Gokoglu N. (2004). Investigation of the Sensory Properties of the Flesh of Rainbow trout (Oncorhynchus mykiss) Fed Diets with Astaxanthin, Shrimp Waste Meal and Red Pepper Meal. Eur. Food Res. Technol. 219, 217–222. doi:10.1007/s00217-004-0923-4
Domınguez-Bocanegra A. R., Legarreta I. G., Jeronimo F. M., Campocosio A. T. (2004). Influence of Environmental and Nutritional Factors in the Production of Astaxanthin from Haematococcus pluvialis. Bioresour. Technol. 92, 209–214. doi:10.1016/j.biortech.2003.04.001
Duan Y., Wang Y., Zhang J., Liu Q., Ding X. (2018). Morphologic, Digestive Enzymes and Immunological Responses of Intestine from Litopenaeus Vannamei after Lipopolysaccharide Injection. J. Invertebr. Pathol. 153, 186–194. doi:10.1016/j.jip.2018.03.003
Evans S. S., Repasky E. A., Fisher D. T. (2015). Fever and the thermal Regulation of Immunity: the Immune System Feels the Heat. Nat. Rev. Immunol. 15, 335–349. doi:10.1038/nri3843
Fabregas J., Herrero C. (1986). Marine Microalgae as a Potential Source of Minerals in Fish Diets. Aquaculture 51, 237–243. doi:10.1016/0044-8486(86)90315-7
Fang H., Xie J., Liao S., Guo T., Xie S., Liu Y., et al. (2019). Effects of Dietary Inclusion of Shrimp Paste on Growth Performance, Digestive Enzymes Activities, Antioxidant and Immunological Status and Intestinal Morphology of Hybrid Snakehead (Channa Maculata ♀ × Channa argus ♂). Front. Physiol. 10, 1027. doi:10.3389/fphys.2019.01027
Fang H., Zhao W., Xie J., Yin P., Zhuang Z., Liu Y., et al. (2021). Effects of Dietary Lipid Levels on Growth Performance, Hepatic Health, Lipid Metabolism and Intestinal Microbiota on Trachinotus Ovatus. Aquac. Nutr. 27, 1554–1568. doi:10.1111/anu.13296
Fazelan Z., Hoseini S. M., Yousefi M., Khalili M., Hoseinifar S. H., Van Doan H. (2020). Effects of Dietary Eucalyptol Administration on Antioxidant and Inflammatory Genes in Common Carp (Cyprinus carpio) Exposed to Ambient Copper. Aquaculture 520, 734988. doi:10.1016/j.aquaculture.2020.734988
Gao B., Yang J., Lei X., Xia S., Li A., Zhang C. (2016). Characterization of Cell Structural Change, Growth, Lipid Accumulation, and Pigment Profile of a Novel Oleaginous Microalga, Vischeria Stellata (Eustigmatophyceae), Cultured with Different Initial Nitrate Supplies. J. Appl. Phycol. 28, 821–830. doi:10.1007/s10811-015-0626-1
Guzmán-Villanueva L. T., Escobedo-Fregoso C., Barajas-Sandoval D. R., Gomez-Gil B., Peña-Rodríguez A., Martínez-Diaz S. F., et al. (2020). Assessment of Microbial Dynamics and Antioxidant Enzyme Gene Expression Following Probiotic Administration in Farmed Pacific white Shrimp (Litopenaeus Vannamei). Aquaculture 519, 734907. doi:10.1016/j.aquaculture.2019.734907
Han T., Li X., Wang J., Wang C., Yang M., Zheng P. (2018). Effects of Dietary Astaxanthin (AX) Supplementation on Pigmentation, Antioxidant Capacity and Nutritional Value of Swimming Crab, Portunus Trituberculatus. Aquac. 490, 169–177. doi:10.1016/j.aquaculture.2018.02.030
Higuera-Ciapara I., Félix-Valenzuela L., Goycoolea F. M. (2006). Astaxanthin: a Review of its Chemistry and Applications. Crit. Rev. Food Sci. Nutr. 46, 185–196. doi:10.1080/10408690590957188
Horwitz W. (20101997). “Official Methods of Analysis of AOAC International,” in Volume I, Agricultural Chemicals, Contaminants, Drugs. Editor W. Horwitz (Gaithersburg (Maryland): AOAC International).
Ip P.-F., Chen F. (2005). Production of Astaxanthin by the green Microalga Chlorella Zofingiensis in the Dark. Process Biochem. 40, 733–738. doi:10.1016/j.procbio.2004.01.039
Johnson E. A., Lewis M. J. (1979). Astaxanthin Formation by the Yeast Phaffia Rhodozyma. J. Gen. Microbiol. 115, 173–183. doi:10.1099/00221287-115-1-173
Ju Z. Y., Deng D.-F., Dominy W. (2012). A Defatted Microalgae (Haematococcus pluvialis) Meal as a Protein Ingredient to Partially Replace Fishmeal in Diets of Pacific white Shrimp (Litopenaeus Vannamei, Boone, 1931). Aquaculture 354-355 (355), 50–55. doi:10.1016/j.aquaculture.2012.04.028
Ju Z. Y., Deng D.-F., Dominy W. G., Forster I. P. (2011). Pigmentation of Pacific white Shrimp, Litopenaeus Vannamei, by Dietary Astaxanthin Extracted from Haematococcus pluvialis. J. World Aquac. Soc. 42, 633–644. doi:10.1111/j.1749-7345.2011.00511.x
Khodambashi Emami N., Samie A., Rahmani H. R., Ruiz-Feria C. A. (2012). The Effect of Peppermint Essential Oil and Fructooligosaccharides, as Alternatives to Virginiamycin, on Growth Performance, Digestibility, Gut Morphology and Immune Response of Male Broilers. Anim. Feed Sci. Tech. 175, 57–64. doi:10.1016/j.anifeedsci.2012.04.001
Lama L., Nicolaus B., Calandrelli V., Manca M. C., Romano I., Gambacorta A. (1996). Effect of Growth Conditions on Endo- and Exopolymer Biosynthesis in Anabaena Cylindrica 10 C. Phytochemistry 42, 655–659. doi:10.1016/0031-9422(95)00985-x
Larbi Ayisi C., Zhao J., Wu J.-W. (2018). Replacement of Fish Oil with palm Oil: Effects on Growth Performance, Innate Immune Response, Antioxidant Capacity and Disease Resistance in Nile tilapia (Oreochromis niloticus). PLoS One 13, e0196100. doi:10.1371/journal.pone.0196100
Li M., Wu W., Zhou P., Xie F., Zhou Q., Mai K. (2014). Comparison Effect of Dietary Astaxanthin and Haematococcus pluvialis on Growth Performance, Antioxidant Status and Immune Response of Large Yellow Croaker Pseudosciaena Crocea. Aquaculture 434, 227–232. doi:10.1016/j.aquaculture.2014.08.022
Li R., Liu H., Dong X., Chi S., Yang Q., Zhang S., et al. (2018). Molecular Characterization and Expression Analysis of Glucose Transporter 1 and Hepatic Glycolytic Enzymes Activities from Herbivorous Fish Ctenopharyngodon idellus in Respond to a Glucose Load after the Adaptation to Dietary Carbohydrate Levels. Aquaculture 492, 290–299. doi:10.1016/j.aquaculture.2018.04.028
Li Y., Miao F., Geng Y., Lu D., Zhang C., Zeng M. (2012). Accurate Quantification of Astaxanthin from Haematococcus Crude Extract Spectrophotometrically. Chin. J. Ocean. Limnol. 30, 627–637. doi:10.1007/s00343-012-1217-5
Livak K. J., Schmittgen T. D. (2001). Analysis of Relative Gene Expression Data Using Real-Time Quantitative PCR and the 2−ΔΔCT Method. methods 25, 402–408. doi:10.1006/meth.2001.1262
Lu S., Wu X., Gao Y., Gatlin D. M., Wu M., Yao W., et al. (2018). Effects of Dietary Carbohydrate Sources on Growth, Digestive Enzyme Activity, Gene Expression of Hepatic GLUTs and Key Enzymes Involved in Glycolysis-Gluconeogenesis of Giant Grouper Epinephelus Lanceolatus Larvae. Aquaculture 484, 343–350. doi:10.1016/j.aquaculture.2017.07.033
Metting F. B. (1996). Biodiversity and Application of Microalgae. J. Ind. Microbiol. Biotechnol. 17, 477–489. doi:10.1007/bf01574779
Nahidian B., Ghanati F., Shahbazi M., Soltani N. (2018). Effect of Nutrients on the Growth and Physiological Features of Newly Isolated Haematococcus pluvialis TMU1. Bioresour. Tech. 255, 229–237. doi:10.1016/j.biortech.2018.01.130
Ni Y., Nagashimada M., Zhuge F., Zhan L., Nagata N., Tsutsui A., et al. (2015). Astaxanthin Prevents and Reverses Diet-Induced Insulin Resistance and Steatohepatitis in Mice: A Comparison with Vitamin E. Sci. Rep. 5, 1–15. doi:10.1038/srep17192
Niu J., Tian L.-X., Liu Y.-J., Yang H.-J., Ye C.-X., Gao W., et al. (2009). Effect of Dietary Astaxanthin on Growth, Survival, and Stress Tolerance of Postlarval Shrimp, Litopenaeus Vannamei. J. World Aquac. Soc. 40, 795–802. doi:10.1111/j.1749-7345.2009.00300.x
Pan C.-H., Chien Y.-H. (2009). Effects of Dietary Supplementation of algaHaematococcus pluvialis(Flotow), Synthetic Astaxanthin and β-carotene on Survival, Growth, and Pigment Distribution of Red Devil,Cichlasoma citrinellum(Günther). Aquac. Res. 40, 871–879. doi:10.1111/j.1365-2109.2008.02153.x
Petit H., Nègre-Sadargues G., Castillo R., Trilles J.-P. (1997). The Effects of Dietary Astaxanthin on Growth and Moulting Cycle of Postlarval Stages of the Prawn, Penaeus Japonicus (Crustacea, Decapoda). Comp. Biochem. Physiol. A: Physiol. 117, 539–544. doi:10.1016/s0300-9629(96)00431-8
Qiu W., Zhang S., Chen Y.-G., Wang P.-H., Xu X.-P., Li C.-z., et al. (2014). Litopenaeus Vannamei NF-Κb Is Required for WSSV Replication. Dev. Comp. Immunol. 45, 156–162. doi:10.1016/j.dci.2014.02.016
Reyes-Becerril M., Angulo C., Estrada N., Murillo Y., Ascencio-Valle F. (2014). Dietary Administration of Microalgae Alone or Supplemented with Lactobacillus Sakei Affects Immune Response and Intestinal Morphology of Pacific Red Snapper (Lutjanus peru). Fish Shellfish Immunol. 40, 208–216. doi:10.1016/j.fsi.2014.06.032
Reyes-Becerril M., Guardiola F., Rojas M., Ascencio-Valle F., Esteban M. Á. (2013). Dietary Administration of Microalgae Navicula Sp. Affects Immune Status and Gene Expression of Gilthead Seabream (Sparus aurata). Fish Shellfish Immunol. 35, 883–889. doi:10.1016/j.fsi.2013.06.026
Romero J., Feijoó C. G., Navarrete P. (2012). Antibiotics in Aquaculture–Use, Abuse and Alternatives. Heal. Environ. Aquac. 159, 159–198.
Roy S. S., Pal R. (2015). Microalgae in Aquaculture: a Review with Special References to Nutritional Value and Fish Dietetics, Proc. Zool Soc. 68. 1–8. doi:10.1007/s12595-013-0089-9
Santos L., Ramos F. (2016). Analytical Strategies for the Detection and Quantification of Antibiotic Residues in Aquaculture Fishes: A Review. Trends Food Sci. Tech. 52, 16–30. doi:10.1016/j.tifs.2016.03.015
Shah M. R., Lutzu G. A., Alam A., Sarker P., Kabir Chowdhury M. A., Parsaeimehr A., et al. (2018). Microalgae in Aquafeeds for a Sustainable Aquaculture Industry. J. Appl. Phycol. 30, 197–213. doi:10.1007/s10811-017-1234-z
Sørum H. (2005). “Antimicrobial Drug Resistance in Fish Pathogens”. in Antimicrob. Resist. Bact. Anim. Orig., 213–238. Hoboken, NJ, United States: Wiley Press
Soto-Rodriguez S. A., Gomez-Gil B., Lozano-Olvera R., Betancourt-Lozano M., Morales-Covarrubias M. S. (2015). Field and Experimental Evidence of Vibrio Parahaemolyticus as the Causative Agent of Acute Hepatopancreatic Necrosis Disease of Cultured Shrimp (Litopenaeus Vannamei) in Northwestern Mexico. Appl. Environ. Microbiol. 81, 1689–1699. doi:10.1128/aem.03610-14
Takeuchi O., Akira S. (2010). Pattern Recognition Receptors and Inflammation. Cell 140, 805–820. doi:10.1016/j.cell.2010.01.022
Verbruggen B., Bickley L., Van Aerle R., Bateman K., Stentiford G., Santos E., et al. (2016). Molecular Mechanisms of white Spot Syndrome Virus Infection and Perspectives on Treatments. Viruses 8, 23. doi:10.3390/v8010023
Wang F., Gao B., Wu M., Huang L., Zhang C. (2019). A Novel Strategy for the Hyper-Production of Astaxanthin from the Newly Isolated Microalga Haematococcus pluvialis JNU35. Algal Res. 39, 101466. doi:10.1016/j.algal.2019.101466
Wang W., Ishikawa M., Koshio S., Yokoyama S., Dawood M. A., Zhang Y. (2018). Effects of Dietary Astaxanthin Supplementation on Survival, Growth and Stress Resistance in Larval and post-larval Kuruma Shrimp, Marsupenaeus japonicus. Aquac. Res. 49, 2225–2232. doi:10.1111/are.13679
Webb K. L. (1983). “Phytoplankton as a Food Source for Bivalve Larvae,” in Proceedings of the Second International Conference on Aquaculture Nutrition: Biochemical and Physiological Approaches to Shellfis, Baton Rouge: Lousiana State University Press, 272–291.
Xie C., Kang J., Ferguson M. E., Nagarajan S., Badger T. M., Wu X. (2011). Blueberries Reduce Pro-inflammatory Cytokine TNF-α and IL-6 Production in Mouse Macrophages by Inhibiting NF-Κb Activation and the MAPK Pathway. Mol. Nutr. Food Res. 55, 1587–1591. doi:10.1002/mnfr.201100344
Xie J., Fang H., He X., Liao S., Liu Y., Tian L., et al. (2020). Study on Mechanism of Synthetic Astaxanthin and Haematococcus pluvialis Improving the Growth Performance and Antioxidant Capacity under Acute Hypoxia Stress of golden Pompano (Trachinotus Ovatus) and Enhancing Anti-inflammatory by Activating Nrf2-ARE Pathway to Antagonize the NF-Κb Pathway. Aquaculture 518, 734657. doi:10.1016/j.aquaculture.2019.734657
Xie S., Fang W., Wei D., Liu Y., Yin P., Niu J., et al. (2018). Dietary Supplementation of Haematococcus pluvialis Improved the Immune Capacity and Low Salinity Tolerance Ability of post-larval white Shrimp, Litopenaeus Vannamei. Fish Shellfish Immunol. 80, 452–457. doi:10.1016/j.fsi.2018.06.039
Xie S., Wei D., Fang W., Yin P., Liu Y., Niu J., et al. (2020). Survival and Protein Synthesis of post-larval White Shrimp, Litopenaeus Vannamei Were Affected by Dietary Protein Level. Anim. Feed Sci. Tech. 263, 114462. doi:10.1016/j.anifeedsci.2020.114462
Xie S., Wei D., Yin P., Zheng L., Guo T., Liu Y., et al. (2019). Dietary Replacement of Fish-Meal Impaired Protein Synthesis and Immune Response of Juvenile Pacific white Shrimp, Litopenaeus Vannamei at Low Salinity. Comp. Biochem. Physiol. B: Biochem. Mol. Biol. 228, 26–33. doi:10.1016/j.cbpb.2018.11.002
Yang P., He C., Qin Y., Wang W., Mai K., Qin Q., et al. (2021). Evaluation of Composite Mixture of Protein Sources in Replacing Fishmeal for Pacific white Shrimp (Litopenaeus Vannamei): Based on the Changing Pattern of Growth Performance, Nutrient Metabolism and Health Status. Aquacult. Rep. 21, 100914. doi:10.1016/j.aqrep.2021.100914
Yu H., Lin L., Zhang Z., Zhang H., Hu H. (2020). Targeting NF-Κb Pathway for the Therapy of Diseases: Mechanism and Clinical Study. Signal. Transduct. Target. Ther. 5, 209–223. doi:10.1038/s41392-020-00312-6
Yu Y.-Y., Chen W.-D., Liu Y.-J., Niu J., Chen M., Tian L.-X. (2016). Effect of Different Dietary Levels of Gracilaria Lemaneiformis Dry Power on Growth Performance, Hematological Parameters and Intestinal Structure of Juvenile Pacific white Shrimp ( Litopenaeus Vannamei ). Aquaculture 450, 356–362. doi:10.1016/j.aquaculture.2015.07.037
Zhang Y., Yu J., Su Y., Du Y., Liu Z. (2019). Long-term Changes of Water Quality in Aquaculture-Dominated Lakes as Revealed by Sediment Geochemical Records in Lake Taibai (Eastern China). Chemosphere 235, 297–307. doi:10.1016/j.chemosphere.2019.06.179
Zhao W., Fang H.-H., Gao B.-Y., Dai C.-M., Liu Z.-Z., Zhang C.-W., et al. (2020). Dietary Tribonema Sp. Supplementation Increased Growth Performance, Antioxidant Capacity, Immunity and Improved Hepatic Health in golden Pompano (Trachinotus Ovatus). Aquaculture 529, 735667. doi:10.1016/j.aquaculture.2020.735667
Zhao W., Fang H. H., Liu Z. Z., Huang M. Q., Su M., Zhang C. W., et al. (2021). A Newly Isolated Strain of Haematococcus pluvialis JNU35 Improves the Growth, Antioxidation, Immunity and Liver Function of golden Pompano ( Trachinotus Ovatus ). Aquacult. Nutr. 27, 342–354. doi:10.1111/anu.13188
Zhao W., Wang L., Liu M., Jiang K., Wang M., Yang G., et al. (2017). Transcriptome, Antioxidant Enzyme Activity and Histopathology Analysis of Hepatopancreas from the white Shrimp Litopenaeus Vannamei Fed with Aflatoxin B1(AFB1). Dev. Comp. Immunol. 74, 69–81. doi:10.1016/j.dci.2017.03.031
Zokaeifar H., Balcázar J. L., Saad C. R., Kamarudin M. S., Sijam K., Arshad A., et al. (2012). Effects of Bacillus Subtilis on the Growth Performance, Digestive Enzymes, Immune Gene Expression and Disease Resistance of white Shrimp, Litopenaeus Vannamei. Fish Shellfish Immunol. 33, 683–689. doi:10.1016/j.fsi.2012.05.027
Keywords: Haematococcus pluvialis GXU-A23, Litopenaeus vannamei, growth performance, hepatopancreas health, metabolism, intestine morphology
Citation: Fang H, Zhuang Z, Huang L, Niu J and Zhao W (2022) A Newly Isolated Strain of Haematococcus pluvialis GXU-A23 Improves the Growth Performance, Antioxidant and Anti-Inflammatory Status, Metabolic Capacity and Mid-intestine Morphology of Juvenile Litopenaeus vannamei. Front. Physiol. 13:882091. doi: 10.3389/fphys.2022.882091
Received: 23 February 2022; Accepted: 06 April 2022;
Published: 25 April 2022.
Edited by:
Benjamin Costas, University of Porto, PortugalReviewed by:
Muhammad Shahid Riaz Rajoka, Shenzhen University, ChinaFrancisco Javier Alarcón, University of Almeria, Spain
Copyright © 2022 Fang, Zhuang, Huang, Niu and Zhao. This is an open-access article distributed under the terms of the Creative Commons Attribution License (CC BY). The use, distribution or reproduction in other forums is permitted, provided the original author(s) and the copyright owner(s) are credited and that the original publication in this journal is cited, in accordance with accepted academic practice. No use, distribution or reproduction is permitted which does not comply with these terms.
*Correspondence: Jin Niu, niuj3@mail.sysu.edu.cn; Wei Zhao, zwtjnxysc@163.com