- 1Zhejiang Provincial Key Laboratory of Biometrology and Inspection and Quarantine, College of Life Science, China Jiliang University, Hangzhou, China
- 2Zhejiang Tonglu Huifeng Biosciences Co., Ltd., Hangzhou, China
Management of the rice brown planthopper Nilaparvata lugens Stål is challenging because it can rapidly adapt to new pesticides within several generations. Combined use of chemical insecticides and antimicrobials was proposed as an alternative strategy to control N. lugens. Our previous experiments identified two effective agents (chemical insecticide: pymetrozine and antimicrobial: zhongshengmycin) that act on different targets in N. lugens. However, conditions and effectiveness of combinations of antimicrobials and insecticides against N. lugens are still unknown. Here, we evaluated separate and combined effects of pymetrozine and zhongshengmycin on third instar nymphs of N. lugens under laboratory and greenhouse conditions. Results showed that zhongshengmycin exerts significant inhibitory effects on the three endosymbionts Pichia guilliermondii, Cryptococcus peneaus, and Pichia anomala cultured in vitro of N. lugens. Combinations of pymetrozine and zhongshengmycin under laboratory conditions produced additive or synergistic effects on N. lugens and caused higher mortality in third instar nymphs than either of them used alone. Experiments under greenhouse conditions further demonstrated that effective component quality ratio of pymetrozine to zhongshengmycin of 1:10 and 1:40 with co-toxicity coefficients of 221.63 and 672.87, respectively, also produced significant synergistic effects against N. lugens. Our results indicated that chemical insecticides combined with antimicrobials may provide a potential novel strategy for controlling N. lugens by inhibiting its endosymbionts.
1 Introduction
The brown planthopper Nilaparvata lugens Stål (Hemiptera: Delphacidae) is a migratory and monophagous destructive pest of rice herbivore (Cheng et al., 2013). N. lugens obtains necessary nutrients from phloem sap of rice plant leaf sheath, causes indirect damage to rice plants through transmission of plant viruses in South and East Asia rice cultivation regions, and results in major yield reduction and economic losses (Garrood et al., 2016; Yang et al., 2017). Although chemical pesticides are still the main control strategy for N. lugens, this approach inevitably leads to the development of insecticide resistance, insect resurgence, and toxicity to natural enemies (Hu et al., 2014). Moreover, the time for N. lugens to develop resistance to common agents has remarkably reduced due to extensive and irregular use of chemical insecticides.
Therefore, developing increasingly effective control methods for the integrated management of N. lugens, which is closely related to people’s livelihood and environmental protection, is important. As it is known to us, endosymbionts include fungi and bacteria are ubiquitous in insects (Li et al., 2016). Endosymbionts have evolved from an organism into a kind of organelle-like structure in the long process of coevolution. A large number of studies have shown that N. lugens and endosymbionts form a stable mutualistic symbiosis, mutual dependence, and influence relationship due to their irreplaceable functional requirements (Sapp, 2002). Previous studies on endosymbionts of N. lugens mainly focused on diversity and dynamics of microbial communities at different developmental stages or feeding on different resistant rice varieties and their role in mediating host resistance (Cai et al., 2020; Jin et al., 2021; Zhang et al., 2021). Synergy effect of antimicrobials and insecticides on N. lugens population should be further explored given the close relationship between N. lugens and endosymbionts. The entire genome sequencing of N. lugens and its endosymbionts revealed that complementarity of three genomes with regard to nutritional pathways, including essential amino acid and steroid biosynthesis by the fungal symbiont and vitamin B supplementation by the bacterial symbiont, enables N. lugens to thrive on a low-nutrient diet provided solely by rice (Xue et al., 2014; Shi et al., 2021). The 3D reconstruction of N. lugens indicated that three structurally different endosymbionts, namely, yeast-like symbionts (YLSs), thread-, and rod-like bacterial symbionts, residing in fat body mycetocytes or midgut account for more than 22% of the abdominal volume of N. lugens (Song et al., 2021; Wang et al., 2021). Hence, endosymbionts are vital to the growth of N. lugens and may be potential targets of pest control.
Pymetrozine, a representative of pyridine azomethine compounds, is a chemical insecticide that exerts a significant control effect on specific species of stinging and sucking pest (Maienfisch et al., 2012; Li et al., 2021). Pymetrozine can be used to control aphids, leafhoppers, Bemisia tabacis, and rice planthoppers due to its high selectivity while showing safety to biological natural enemies of rice planthoppers, such as spiders and Anagrus nilaparvataes, during the control process (Preetha et al., 2010; Lin et al., 2021). The resistance of N. lugens to pymetrozine, sulfoxaflflor, nitenpyram, ect chemical insecticides has increased evidently in recent years (Liao et al., 2019; Zhang et al., 2021). Some field populations of N. lugens in China still remained susceptible to pymetrozine in 2010, but the resistance increased to medium or high levels in 2011 and 2012 (Zhang et al., 2014). The trend of increasing pymetrozine resistance was associated with irrational use of insecticide doses against rice planthoppers by managers and over-reliance on the use of chemical insecticides, such as pymetrozine, in Southeast Asia given that rice planthoppers in China typically migrate from Southeast Asia (Wang et al., 2013). Exploring improved methods for controlling and managing rice planthoppers is urgently necessary to avoid resistance development of N. lugens to additional types of chemical insecticides.
Zhongshengmycin is a broad-spectrum agro-antibiotic that has been investigated and developed in 1996 and demonstrated satisfactory effects on preventing and controlling crop pathogenic microorganisms, such as Xanthomonas campestris, Erwinia carotovora, Piricularia oryzae, Pseudomonas solanacearum, and Physalospora piricola (Xie et al., 1990; Zhao et al., 1993; Jiang et al., 1997). Zhongshengmycin works by interrupting the synthesis of protein peptide bonds of pathogenic microorganisms to inhibit the growth of bacteria, fungal mycelium, and spore germination (Yao et al., 2019). The successful exploitation of zhongshengmycin has replaced the use of some conventional chemical pesticides to a certain extent and presented high potential for common usage and application (Lin et al., 1991; Zhang et al., 1998). Therefore, the synergistic use of agro-antibiotics and chemical insecticides is an important strategy for pest control by inhibiting different targets, microbial endosymbionts and insects, respectively (Singh et al., 2016). Previous studies showed that the combination of pymetrozine and buprofezin 25% suspending agent is effective against Sogatella furcifera and N. lugens at the nymphal stage (Xing et al., 2011). Moreover, toxicity tests indicated that mixtures of imidacloprid and ethofenprox as well as thiamethoxam and ethofenprox exert excellent synergistic effects on N. lugens (Yu et al., 2015). The interaction between amitraz and malathion on Aphis gossypii presents a synergistic effect at all concentrations (Shojaei et al., 2018). Furthermore, a significant synergistic effect was observed when the mixture of destruxins and botanical insecticide rotenone was used at a concentration ratio of 9:1 in A. gossypii control (Yi et al., 2012). Accordingly, antimicrobials mixed with chemical agents may be a valuable strategy for pest control and provide an effective way of reducing the amount of chemical pesticides applied to crops while retarding resistance development of pests. The utilization of appropriately selected insecticides in association with antimicrobials can inhibit endosymbiont growth and result in synergistic and additive effects on N. lugens control.
Thus, we hypothesized in the present study that the combination of pymetrozine and zhongshengmycin at different concentration ratios may be effective against N. lugens. Our objective was to test whether combined effects of pymetrozine and zhongshengmycin were synergistic, additive, or antagonistic on N. lugens and obtain the optimal combination ratio of the mixture for the control of N. lugens. Furthermore, the optimal combination was used to test the mortality of N. lugens third instar nymphs under greenhouse conditions.
2 Materials and Methods
2.1 Insect and Antimicrobials
The rice variety used in this study was the susceptible strain TN1. Rice seeds were sown in the artificial climate room, and N. lugens was raised from rice seedlings in the tillering period. The N. lugens population used in this study was initially collected from rice fields in Hangzhou, China (continuously cultivated for more than ten generations under greenhouse conditions). Insects were reared on TN1 rice seedlings in an artificial climate chamber under conditions of 26 ± 1°C, relative humidity of 70%–80%, continuous 16 h light/8 h dark photoperiod, and nonexposure to any insecticide. Pymetrozine [96.6% active ingredient (a.i.) w/w] was purchased from Jiangsu Weunite Fine Co., Ltd. Zhongshengmycin (12% a.i. w/w) was provided by Fujian Kaili Biotechnology Co., Ltd. Tebuconazole (97% a.i. w/w) was obtained from Udragon Chemical Co., Ltd. N, N-Dimethylformamide (99.9%) was supplied by Shanghai Aladdin Bio-Chem Technology Co., Ltd.
2.2 Inhibitory Effect of Antimicrobials on Yeast-Like Symbionts
Pichia guilliermondii, Cryptococcus peneaus, and P. anomala strains were originally isolated from the fat body of N. lugens and preserved in Zhejiang Provincial Key Laboratory of Biometrology and Inspection and Quarantine. These isolates were activated in a plate of potato dextrose agar (PDA; 200 g of potato, 20 g of dextrose, and 20 g of agar in 1 L of distilled water) at 28°C ± 1°C. YLS suspensions were prepared by scraping YLS from the surface of the culture medium into a sterile PD solution (PD, 200 g of potato and 20 g of dextrose in 1 L of distilled water). Inhibitory effects of antimicrobials were determined by spreading YLS suspensions onto PDA plates containing various antimicrobial concentrations, followed by incubation at 28°C for an indicated duration.
2.3 Toxicity of Antimicrobials and Insecticides to N. lugens
Indoor toxicity testing of third instar nymphs of N. lugens was performed using rice seedling dip method (Ban et al., 2012; Liao et al., 2017). LC50 values of pymetrozine and zhongshengmycin against third instar nymphs were determined with a technical regulation method described previously [NY/T 1708-2009 technological rules for monitoring insecticide resistance in the rice brown planthopper Nilaparvata lugens (Stål)]. First, rice plants at the tillering stage were cut to a height of 10 cm. Second, three stems of rice plants were dipped into a plastic cup (10 cm in diameter and 18 cm in height) with a series of diluted solutions of pymetrozine and zhongshengmycin for 30 s and then placed rice stems in another test cup after air dried in the room temperature. Each insecticide or antimicrobial was diluted in six concentrations. Controls were treated with 0.1% Tween 80 water solution instead of the insecticide solution. Third, 20 third instar nymphs were collected into the test cup with a homemade aspirator device. All treatments were maintained at 26 ± 1°C and relative humidity of 70%–80% with a photoperiod of 16:8 (L:D) h. Each treatment was conducted in three independent biological replicates. Mortality of insects was monitored daily for 5 days after exposure. Nymphs were considered dead when they failed to move after prodding gently with a fine brush.
2.4 Screening of the Compound Proportion of Pymetrozine and Zhongshengmycin
On the basis of the toxicity bioassay and results of Wu and Si (2006), the toxic effect of different proportions of pymetrozine and zhongshengmycin on N. lugens was measured in this experiment. The synergistic prescription was selected according to laboratory experiments via interactive measurement. According to the results of medial lethal concentrations and toxicity measurement of pymetrozine and zhongshengmycin, toxic effect ratios of the two agents were set using 11 concentration gradients to determine the optimal compound proportion. 30 third instar nymphs were tested at each treatment concentration. Each treatment was conducted in three independent biological replicates. Expected mortality and toxic effect ratio are expressed as follows:
Expected mortality = Ma × the volume proportion of agent A in the mixture + Mb × the volume proportion of agent B in the mixture.
Note: Ma: the observed mortality caused by agent A alone.
Mb: the observed mortality caused by agent B alone.
The concertration of agent A and agent B were both LC50 (median lethal concentration) on test insects
2.5 Determining the Co-Toxicity Coefficient of Pymetrozine and Zhongshengmycin Compound
On the basis of the toxic effect ratio results, combinations with a toxic effect ratio greater than 1.25 were selected to show a synergistic effect for the determination of the co-toxicity coefficient of the optimal combination. Antagonism exists when the toxic effect ratio was less than 0.75. An additive effect was observed when the toxic effect ratio was approximately equal to 1.00. Experiments were conducted according to the procedure in Section 2.3. Each concentration treatment was repeated three times. Insect mortalities were recorded after 120 h. The co-toxicity coefficient, toxicity regression curve and its standard error, LC50 value, and confidence interval of 95% were determined on the basis of standard probit analysis via DPS 7.05. Toxicity index, actual toxicity index, theoretical toxicity index, and cotoxicity coefficient can be expressed as follows:
Theoretical toxicity index (TTI) of mixture (A+B) = TIA×mass percentage of agent A in the mixture + TIB×mass percentage of agent B in the mixture.
Note: standard insecticide: the single agent with a larger LC50 was used as the standard insecticide, and its toxicity index was regarded as 100.
2.6 Data Analysis
Bioassay data were analyzed with DPS 7.05. Mortality data were corrected using the control mortality of Abbott’s formula (Richard and Arthur, 1985). LC50 values, 95% confidence intervals (CI), slopes of regression lines, standard errors, and other relevant data were estimated via probit analysis (Finney, 1971). Treatment effects on mortality levels were assessed using one-way ANOVA. Differences between treatments were deemed significant when p < 0.05. A synergistic effect exists between the two pesticides when the toxic effect ratio of measured insecticides was greater than 1.25. Antagonism exists when the toxic effect ratio was less than 0.75. An additive effect exists when the toxic effect ratio was approximately equal to 1.00. The proportion of compounding agents with a toxic effect ratio greater than 1.25 was selected for indoor toxicity determination, and the toxicity regression curve and LC50 value of the compound were further obtained. The toxicity index of the single agent and theoretical toxicity index, actual toxicity index, and co-toxicity coefficient of the compound were calculated using the method of Sun Yun-pei (Sun and Johnson, 1960). Compounding agents were mutually synergistic when the cotoxicity coefficient was greater than 120. The interaction was additive when the cotoxicity coefficient was between 80 and 120. An antagonistic effect was observed when the cotoxicity coefficient was less than 80.
3 Results
3.1 Inhibitory Effect of Different Fungicides on the Yeast-Like Symbiont
Table 1 and Figure 1 showed the growth of P. guilliermondii, C. peneaus, and P. anomala under different concentrations of tebuconazole and zhongshengmycin on PDA medium. First, P. guilliermondii, C. peneaus, and P. anomala all showed significant growth on the PDA control plate but negative on two kinds of PDA plates with concentrations of 1 μg/L tebuconazole or zhongshengmycin. Second, P. guilliermondii and C. peneaus presented a small amount of growth on the PDA plate with 0.01 and 0.1 μg/L of tebuconazole. Third, P. guilliermondii, C. peneaus, and P. anomala demonstrated major, negative and minimal growth on the PDA plate with 0.01 μg/L of zhongshengmycin, respectively. No or nearly no growth was observed on PDA plates with 0.1 μg/L of zhongshengmycin.
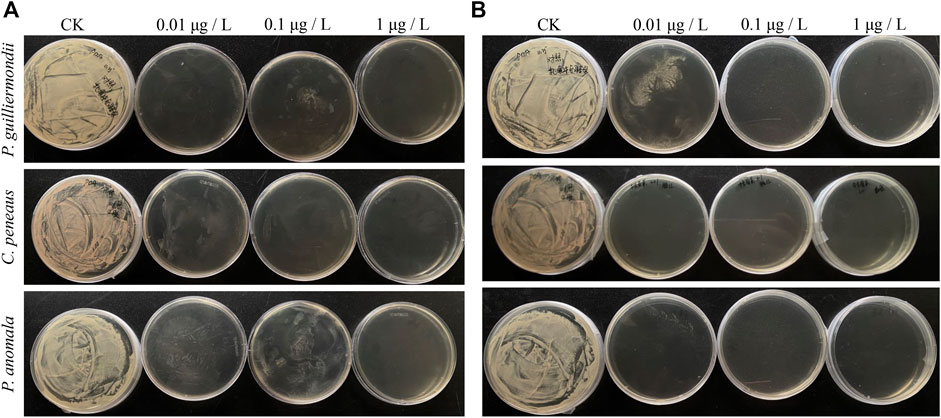
FIGURE 1. The growth of YLSs isolated from Nilaparvata lugens at different concentrations of antimicrobials. (A) Tebuconazole; (B) Zhongshengmycin.
Different concentrations of Zhongshengmycin generally demonstrated better inhibition effect on P. guilliermondii, C. peneaus, and P. anomala than those of tebuconazole. Therefore, Zhongshengmycin may present optimal efficiency in controlling N. lugens by inhibiting its endosymbionts.
3.2 Median Lethal Concentration of Pymetrozine and Zhongshengmycin
As shown in Table 2, pymetrozine and zhongshengmycin present high biological activity to third instar nymphs and the relationship between nymph mortality and insecticide concentration is clearly described through the probit model. Estimated LC50 values of pymetrozine and zhongshengmycin for third instar nymphs of N. lugens were 3.80 (95% confidence interval: 1.84–7.85 mg/L) and 152.61 (95% confidence interval: 45.34–513.70 mg/L) mg/L while LC95 values were estimated at 113.04 (95% confidence interval: 38.73–329.94 mg/L) and 895.00 (95% confidence interval: 262.10–3056.18 mg/L) mg/L, respectively, at 5 days after application.

TABLE 2. Median lethal concentrations of pymetrozine and zhongshengmycin on third instar nymphs of Nilaparvata lugens.
3.3 Effect of Interaction Between Pymetrozine and Zhongshengmycin
The proportion screening of pymetrozine and zhongshengmycin in the mixture was carried out through toxic effect ratio (Table 3). The combination of pymetrozine and zhongshengmycin demonstrated a synergistic effect on third instar nymphs, with toxic effect ratios of 1.28 and 1.29, respectively, in groups 3 and 6. Groups 2, 4, 5, 7, 8, 9, and 10 showed additive effects with the toxic effect ratio between 1 and 1.25. Hence, combinations of pymetrozine and zhongshengmycin targeting third-instar nymphs of N. lugens showed synergistic and additive effects without antagonism. The mortality of all combination treatments was higher than that of individual treatments with zhongshengmycin alone; when compared with pymetrozine alone, the mortality of the combination treatments was partially higher than that of the individual treatments. The treatment results showed that at some ratio of combination with zhongshengmycin and pymetrozine had better control effect than that of zhongshengmycin or pymetrozine alone. Overall, these findings indicated that combinations of pymetrozine and zhongshengmycin increase the mortality of N. lugens. Furthermore, groups 3 and 6 with the synergistic effect are selected for indoor toxicity determination through the comparison of toxic effect ratios.
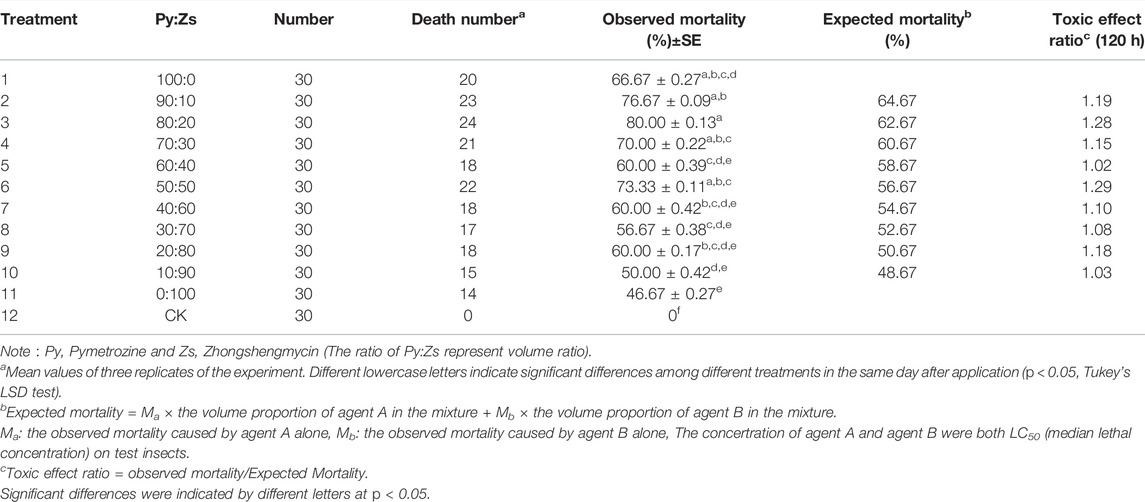
TABLE 3. Interactions between pymetrozine and zhongshengmycin against third instar nymphs of Nilaparvata lugens 5 days after treatment.
3.4 Combined Effects of Pymetrozine and Zhongshengmycin Against N. lugens Under Greenhouse Conditions
Tables 3, 4 indicate that the effective component quality ratio of group 3 (pymetrozine:zhongshengmycin = 80:20) is the combination of pymetrozine: zhongshengmycin = 1:10 for laboratory toxicity determination. LC50 of the combination to third instar nymphs was 15.1012 mg/L (95% confidence interval: 8.6673–26.3113 mg/L) and co-toxicity coefficient was 221.63 at the effective component quality ratio of pymetrozine and zhongshengmycin was 1:10. LC50 of the combination to third instar nymphs was 11.6010 mg/L (95% confidence interval: 6.6270–20.3082 mg/L) and co-toxicity coefficient was 672.87 at the effective component quality ratio of pymetrozine and zhongshengmycin was 1:40. Co-toxicity coefficients of the two combinations with different effective component quality ratios were all greater than 120, thereby indicating the significant synergistic effect. On the basis of these experimental results, treatments with combinations of pymetrozine and zhongshengmycin resulted in higher mortality and the formation of a synergistic effect compared with treatments with insecticide or fungicide alone.

TABLE 4. Determination of the cotoxicity coefficient of pymetrozine and zhongshengmycin on third instar nymphs of N. lugens and statistical results of the adjustment to the log-probit model under greenhouse condition.
4 Discussion
Pymetrozine is one of the recommended alternative insecticides for controlling N. lugens. However, N. lugens has become highly resistant to pymetrozine (Liu et al., 2013; Zhu et al., 2020). Combined effects of pymetrozine and an antimicrobial (zhongshengmycin) on nymphs of N. lugens were investigated in the present study to delay pymetrozine resistance development and prolong the effective application of this insecticide. Our findings indicated that all combined groups exert synergistic or additive effects on the control of N. lugens. The mortality of third-instar nymphs increased in the combinations treatment of pymetrozine and zhongshengmycin, while compared with that of the treatments used pymetrozine and zhongshengmycin alone. Additionally, the effective component quality ratio of pymetrozine and zhongshengmycin of 1:10 and 1:40 proved to have significant synergistic effects against third instar nymphs under greenhouse conditions by calculating the co-toxicity coefficient. Compared with chemical insecticides, microbial metabolites present characteristics of lower toxicity and lower environmental pollution coefficient (Chen et al., 2009; Cao et al., 2015). Therefore, the synergistic use of antimicrobials and chemical insecticides is a safe, efficient, and potential approach for pest management for presenting advantages of obvious biocontrol effect and reduced use of chemical insecticides.
The use of alternative substances to chemical insecticides has attracted considerable research interest due to its lowered risk to the environment and human health and increased food safety. Combinations of antimicrobials and insecticides can exhibit synergistic, antagonistic, or additive effects on pests. For example, combined application of Serratia marcescens S-JS1 with spirotetramat or thiamethoxam resulted in increased effcacy against N. lugens under both laboratory and greenhouse conditions (Niu et al., 2018). In addition, positive synergistic interactions in combined treatments of pymetrozine and thiamethoxam against N. lugens revealed that the controlling effect of combined treatments on rice planthoppers is better than that of the commercial insecticide 2.2% abamectin–imidacloprid emulsifiable concentrate at comparable doses (Sadigh and Erhart, 2012). Willmott et al. (2013) reported that spinosad and pymetrozine mixtures are clearly compatible and combination index calculations showed that mixtures are synergistic against western flower thrips. Cao et al. (2015) revealed that 70% propineb and 50% tebuconazole+25% trifloxystrobin exert evident inhibitory effects on P. anomala isolated from Laodelphax striatellus in vitro and L. striatellus fed with treated wheat seedlings show significantly higher mortality than the control. Controlling N. lugens with fungicides has been extensively investigated. For instance, injection of propiconazole into N. lugens reduced not only fecundity of insects but also significantly reduced the total number of YLS and Hypomyces chrysospermus in hemolymph of insects. This finding led to significantly higher mortality of N. lugens than that of the control group. Survival and fecundity of N. lugens also decreased significantly after feeding on susceptible species TN1 sprayed with propiconazole (Shentu et al., 2019). Moreover, high mortlity of N. lugens due to the administration of 27% toyocamycin + tetramycin P + tetrin B + tetramycin A, 0.01% trichodermin, and 75% trifloxystrobin + tebuconazole WG inhibited the YLS (Shentu et al., 2016). The application of another fungicide jinggangmycin for controlling rice sheath blight can successfully inhibit the reproduction of L. striatellus and S. furcifera but also stimulate the reproduction of N. lugens (Zhang et al., 2015; Ding et al., 2017). However, we provided a new combined formulation of pymetrozine and zhongshengmycin for N. lugens control. Our results have showed that two combinations of pymetrozine and zhongshengmycin at the effective component quality ratio of 1:10 and 1:40 have significant synergistic effects on the control of N. lugens. The mixing ratio and resulting economic benefits should be considered in practical applications given that the two combined groups demonstrated significant synergistic effects against N. lugens. Furthermore, reasons behind the ability of combined pymetrozine with zhongshengmycin to produce synergistic or additive effects on N. lugens are presented in the following section.
Applying combinations of antimicrobials with insecticides may improve not only the efficacy of agents but also provide a potential strategy for reducing insecticide use in pest control (Lacey et al., 2015). Zhongshengmycin exerted a significant inhibitory effect on P. guilliermondii, C. peneaus, and P. anomala, which were isolated from N. lugens within 1–3 days of in vitro culture in the present study. We hypothesized that zhongshengmycin can help control N. lugens by inhibiting endosymbionts, which play a vital role in the growth, development, and reproduction of their host insects. However, difference may exist in the inhibitory effect on YLSs between in vivo and in vitro cultures. Moreover, in vitro culturing of many kinds of symbionts in N. lugens, including endosymbiotic bacteria and YLSs, is impossible. Determining whether zhongshengmycin exerts inhibitory effects on uncultured symbionts is challenging from an evolutionary perspective because important symbionts are difficult to culture in vitro. Therefore, the specific kind of YLS in N. lugens inhibited by zhongshengmycin requires further investigation.
In conclusion, our study emphasized the importance of combining chemical insecticides with antimicrobials for the control of N. lugens by inhibiting endosymbionts under both laboratory and greenhouse conditions. We first screened out zhongshengmycin, an antimicrobial with strong inhibitory effect on YLS, in N. lugens. Then, we applied the combination of zhongshengmycin and pymetrozine to prevent N. lugens and verify that the increased mortality of N. lugens is caused by the inhibitory effect of the combination on YLSs in N. lugens. The results of this study addressed the efficacy gap of single agent treatment in N. lugens control and improved the efficacy in controlling N. lugens given that all test combinations produced additive or synergistic effects. We also revealed that synergistic and additive effects produced by the combinations of zhongshengmycin and pymetrozine may vary depending on their concentrations and types. This finding may provide an effective option for reducing concentrations and doses of chemical insecticides in the future. However, the exact function of the compound in symbionts of N. lugens remains unclear. We speculated that the function may be caused by the change in endosymbionts. Hence, further investigations on the number and function of these endosymbionts under different chemical insecticides or antimicrobials are necessary to address such problems. Understanding the effect of these chemical insecticides or antimicrobials on endosymbionts of N. lugens and using the combination of chemical insecticides and antimicrobials can be an important treatment for integrated pest management of N. lugens in the future.
Data Availability Statement
The original contributions presented in the study are included in the article/Supplementary Material, further inquiries can be directed to the corresponding authors.
Author Contributions
All authors listed have made a substantial, direct, and intellectual contribution to the work and approved it for publication.
Funding
This study was funded by the National Natural Science Foundation of China (U21A20223) and Zhejiang Provincial Programs for Science and Technology Development (2019C02015 and 2022C02047).
Conflict of Interest
Author ZL is employed by Zhejiang Tonglu Huifeng Biosciences Co., Ltd.
The remaining authors declare that the research was conducted in the absence of any commercial or financial relationships that could be construed as a potential conflict of interest.
Publisher’s Note
All claims expressed in this article are solely those of the authors and do not necessarily represent those of their affiliated organizations, or those of the publisher, the editors and the reviewers. Any product that may be evaluated in this article, or claim that may be made by its manufacturer, is not guaranteed or endorsed by the publisher.
References
Ban L., Zhang S., Huang Z., He Y., Peng Y., Gao C. (2012). Resistance Monitoring and Assessment of Resistance Risk to Pymetrozine in Laodelphax Striatellus (Hemiptera: Delphacidae). Jnl. Econ. Entom. 105 (6), 2129–2135. doi:10.1603/EC12213
Cai T., Zhang Y., Liu Y., Deng X., He S., Li J., et al. (2020). Wolbachia Enhances Expression of NlCYP4CE1 in Nilaparvata Lugens in Response to Imidacloprid Stress. Insect Sci. 28, 355–362. doi:10.1111/1744-7917.12834
Cao W., Ma Z., Yu X. P. (2015). Isolation and Sensitivity to Fungicides of the Yeast-like Symbiont Pichia Anomala (Hemiascomycetes: Saccharomycetaceae) from Laodelphax Striatellus (Hemiptera: Delphacidae). Acta. Entomol. Sin. doi:10.16380/j.kcxb.2015.03.006-en
Chen J. M., He Y. P., Zhang J. F., Li N., Chen L. Z., Yu X. P. (2009). Effects of Insecticides and Fungicides on Growth of Endosymbiotes Isolated from the Brown Planthopper, Nilaparvata Lugens. Plant Prot. Sci. 35 (6), 47–51. doi:10.3724/sp.j.1006.2009.00795
Chen Z., Erhart P. (2012). Study on Twenty-Five Percent Pymetrozine-Thiamethoxam Suspension Concentrate as an Insecticide against Rice Planthopper. Afr. J. Agric. Res. 7 (13), 4172–4181. doi:10.5897/AJAR12.007
Cheng X., Zhu L., He G. (2013). Towards Understanding of Molecular Interactions between Rice and the Brown Planthopper. Mol. Plant 6, 621–634. doi:10.1093/mp/sst030
Ding J., Wu Y., You L.-L., Xu B., Ge L.-Q., Yang G.-Q., et al. (2017). Jinggangmycin-suppressed Reproduction in the Small Brown Planthopper (SBPH), Laodelphax Striatellus (Fallen), Is Mediated by Glucose Dehydrogenase (GDH). Pesticide Biochem. Physiology 139, 73–78. doi:10.1016/j.pestbp.2017.05.003
Finney D. J. (1971). Probit Analysis: A Statistical Treatment of the Sigmoid Response Curve. third ed. London: Cambridge University Press.
Garrood W. T., Zimmer C. T., Gorman K. J., Nauen R., Bass C., Davies T. G. (2016). Field‐evolved Resistance to Imidacloprid and Ethiprole in Populations of Brown Planthopper Nilaparvata Lugens Collected from across South and East Asia. Pest. Manag. Sci. 72 (1), 140–149. doi:10.1002/ps.3980
Hu G., Lu F., Zhai B.-P., Lu M.-H., Liu W.-C., Zhu F., et al. (2014). Outbreaks of the Brown Planthopper Nilaparvata Lugens (Stål) in the Yangtze River Delta: Immigration or Local Reproduction? Plos One 9 (2), e88973. doi:10.1371/journal.pone.0088973
Jiang X. L., Xie D. L., Ni C. F., Zhu C. X., Song P. G. (1997). The Antibiotic Action of Zhongshengmycin. Acta. Phytopathol. Sin. 027 (002), 133–138.
Jin R., Mao K., Xu P., Wang Y., Liao X., Wan H., et al. (2021). Inheritance Mode and Fitness Costs of Clothianidin Resistance in Brown Planthopper, Nilaparvata Lugens (Stål). Crop Prot. 140, 105414. doi:10.1016/j.cropro.2020.105414
Lacey L. A., Grzywacz D., Shapiro-Ilan D. I., Frutos R., Brownbridge M., Goettel M. S. (2015). Insect Pathogens as Biological Control Agents: Back to the Future. J. Invertebr. Pathology 132, 1–41. doi:10.1016/j.jip.2015.07.009
Li Q., Fan J., Sun J. R., Wang M. Q., Frederic F., Chen J. L. (2016). Research Progress in the Interactions Among the Plants, Insects and Endosymbionts. J. Plant Protec. 43 (6), 881–891.
Li Y., Liu X., Wang N., Zhang Y., Hoffmann A. A., Guo H. (2020). Background‐dependent Wolbachia ‐mediated Insecticide Resistance in Laodelphax Striatellus. Environ. Microbiol. 22, 2653–2663. doi:10.1111/1462-2920.14974
Li Y., Wei X. L., Cui Y., Chou J. Y. (2021). A Product for Controlling Sucking Pests-Pymetrozine. Agrochemicals 60 (7), 522–528.
Liao X., Mao K., Ali E., Jin R., Li Z., Li W., et al. (2019). Inheritance and Fitness Costs of Sulfoxaflor Resistance in Nilaparvata Lugens (Stål). Pest. Manag. Sci. 75, 2981–2988. doi:10.1002/ps.5412
Liao X., Mao K., Ali E., Zhang X., Wan H., Li J. (2017). Temporal Variability and Resistance Correlation of Sulfoxaflor Susceptibility Among Chinese Populations of the Brown Planthopper Nilaparvata Lugens (Stål). Crop Prot. 102, 141–146. doi:10.1016/j.cropro.2017.08.024
Lin Y. G., Ji H. J., Cao X. C., Cen Y. J., Chen Y. M., Ji S. S., et al. (2021). Knockdown of Amp-Activated Protein Kinase Increases the Insecticidal Efficiency of Pymetrozine to Nilaparvata Lugens - Sciencedirect. Pestic. Biochem. Phys. 175, 104856. doi:10.1016/j.pestbp.2021.104856
Lin Z. L., Lin G., Su Y. (1991). Residue Analysis for Zhongshengmycin(751) in Vegetables Soil with "Before-Column Derivatization" Method. Chin. J. Biol. Control. 7 (2), 67–70.
Liu J., Zhang J., Qin X., Chen Y., Yuan F., Zhang R. (2013). Toxic Effects of Pymetrozine on the Brown Planthopper, Nilaparvata Lugens (Stål) (Homoptera: Delphacidae). J. Entomological Sci. 48 (1), 17–22. doi:10.18474/0749-8004-48.1.17
Maienfisch P., Dekeyser M. A., Saito S., Sakamoto N., Loiseleur O., Slaats B. (2012). New Unknown Modes of Action. Modern Crop Protection Compounds. Second Edition, 1-3. Wiley-VCH Verlag GmbH & Co. KGaA. doi:10.1002/9783527644179.ch33
Niu H., Wang N., Liu B., Xiao L., Wang L., Guo H. (2018). Synergistic and Additive Interactions of Serratia marcescens S-JS1 to the Chemical Insecticides for Controlling Nilaparvata Lugens (Hemiptera: Delphacidae). J. Econ. Entomol. 111, 823–828. doi:10.1093/jee/tox348
Preetha G., Stanley J., Suresh S., Samiyappan R. (2010). Risk Assessment of Insecticides Used in Rice on Miridbug, Cyrtorhinus Lividipennis Reuter, the Important Predator of Brown Planthopper, Nilaparvata Lugens (Stal.). Chemosphere 80 (5), 498–503. doi:10.1016/j.chemosphere.2010.04.070
Richard F., Arthur R. (1985). Evaluating Single Treatment Data Using Abbott's Formula with Reference to Insecticides. J. Econ. Entomol. 6, 1179–1181. doi:10.1093/jee/78.6.1179
Sapp J. (2002). Paul Buchner (1886-1978) and Hereditary Symbiosis in Insects. Int. Microbiol. 5 (3), 145–150. doi:10.1007/s10123-002-0079-7
Shentu X.-P., Li D.-T., Xu J.-F., She L., Yu X.-P. (2016). Effects of Fungicides on the Yeast-like Symbiotes and Their Host, Nilaparvata Lugens Stål (Hemiptera: Delphacidae). Pesticide Biochem. Physiology 128, 16–21. doi:10.1016/j.pestbp.2015.10.010
Shentu X., Wang X., Xiao Y., Yu X. (2019). Effects of Fungicide Propiconazole on the Yeast-like Symbiotes in Brown Planthopper (BPH, Nilaparvata Lugens Stål) and its Role in Controlling BPH Infestation. Front. Physiol. 10. doi:10.3389/fphys.2019.00089
Shi J., Song Y., Shentu X., Yu X. (2021). Antimicrobials Affect the Fat Body Microbiome and Increase the Brown Planthopper Mortality. Front. Physiol. 12. doi:10.3389/fphys.2021.644897
Shojaei A., Jahromi K. T., Hosseininaveh V., Sabahi G. (2018). Synergistic Effects of Amitraz on Imidacloprid and Malathion against Cotton Aphid, Aphis Gossypii (Hemiptera: Aphididae). J. Agr. Sci. Tech-Iran. 20 (2), 299–308.
Singh A. K., Singh A., Joshi P. (2016). Combined Application of Chitinolytic Bacterium Paenibacillus Sp. D1 with Low Doses of Chemical Pesticides for Better Control of Helicoverpa Armigera. Int. J. Pest Manag. 62 (3), 222–227. doi:10.1080/09670874.2016.1167267
Song Y., Shi J., Xiong Z., Shentu X., Yu X. (2021). Three Antimicrobials Alter Gut Microbial Communities and Causing Different Mortality of Brown Planthopper, Nilaparvata Lugens Stål. Pesticide Biochem. Physiology 174 (1), 104806. doi:10.1016/j.pestbp.2021.104806
Sun Y.-P., Johnson E. R. (1960). Analysis of Joint Action of Insecticides against House Flies. J. Econ. Entomol. 53 (5), 887–892. doi:10.1093/jee/53.5.887
Wang P., Xing Z. P., Zhang S., Jiang T. T., Tan L. R., Dong S., et al. (2013). Resistance Monitoring to Conventional Insecticides in Brown Planthopper, Nilaparvata Lugens (Hemiptera: Delphacidae) in Main Rice Growing Regions in China.Chin J Rice Sci. Chin. J. Rice Sci. 27, 191–197. doi:10.3969/j.issn.10017216.2013.02.012
Wang X.-Q., Guo J.-s., Li D.-T., Yu Y., Hagoort J., Moussian B., et al. (2021). Three-dimensional Reconstruction of a Whole Insect Reveals its Phloem Sap-Sucking Mechanism at Nano-Resolution. eLife Sci. eLife Sci. 10. doi:10.7554/eLife.62875
Willmott A. L., Cloyd R. A., Zhu K. Y. (2013). Efficacy of Pesticide Mixtures against the Western Flower Thrips (Thysanoptera: Thripidae) under Laboratory and Greenhouse Conditions. Jnl. Econ. Entom. 106 (1), 247–256. doi:10.1603/EC12264
Wu R. F., Si S. Y. (2006). The Screening of Optimum Ratio of Abamectin and Alphacypermethrin against Liriomyza Sativae Blanchard. Morden Agrochem. 5 (3), 49–51.
Xie D. L., Ni C. F., Zhu C. X. (1990). Control of Bactreial Soft Rot of Crucifers with a Newly Developed Antibiotic. Chin. J. Bio Control 6 (2), 74–77.
Xing H., Jiang W. Y., Qian X. U., Dou X. H. (2011). Efficacy Trials of Pymetrozine+buprofezin 25% Sc Controlling Planthoppers. Modern Agrochemicals.
Xue J., Zhou X., Zhang C.-X., Yu L.-L., Fan H.-W., Wang Z., et al. (2014). Genomes of the Rice Pest Brown Planthopper and its Endosymbionts Reveal Complex Complementary Contributions for Host Adaptation. Genome Biol. 15 (12), 521. doi:10.1186/s13059-014-0521-0
Yang L., Han Y., Li P., Wen L., Hou M. (2017). Silicon Amendment to Rice Plants Impairs Sucking Behaviors and Population Growth in the Phloem Feeder Nilaparvata Lugens (Hemiptera: Delphacidae). Sci. Rep. 7 (1), 1101. doi:10.1038/s41598-017-01060-4
Yao Y. L., Pan Z. C., Zheng P. F., Gao M. N., Yang H. B., Li P. M. (2019). Advance in Application of Zhongshengmycin Prevention and Treatment of Plant Disease. J. Hengyang Norm. Univ. 40 (6), 93–98.
Yi F., Zou C., Hu Q., Hu M. (2012). The Joint Action of Destruxins and Botanical Insecticides (Rotenone, Azadirachtin and Paeonolum) against the Cotton Aphid, Aphis Gossypii Glover. Molecules 17, 7533–7542. doi:10.3390/molecules17067533
Yu Y., Li M., Li R. Y., Fan L. F., Lu C. (2015). Toxicity of Imidacloprid, Thiamethoxam, Ethofenprox and Their Mixtures on Nilaparvata Lugens in Guizhou Province. Guangdong Agri Sci. Guangdong Agric. Sci. 042 (001), 73–77.
Zhang G. F., Cheng H. M., Lu C. T., Wang K. R. (1998). Study on the Control Technology of Rice Bacterial Leaf Blight (Xanthomonas Oryzae). J. Plant Prot. J. Plant Prot. 25 (4), 295–299.
Zhang X., Liu X., Zhu F., Li J., You H., Lu P. (2014). Field Evolution of Insecticide Resistance in the Brown Planthopper (Nilaparvata Lugens Stål) in China. Crop Prot. 58, 61–66. doi:10.1016/j.cropro.2013.12.026
Zhang Y.-X., Ge L.-Q., Jiang Y.-P., Lu X.-L., Li X., Stanley D., et al. (2015). RNAi Knockdown of Acetyl-CoA Carboxylase Gene Eliminates Jinggangmycin-Enhanced Reproduction and Population Growth in the Brown Planthopper, Nilaparvata Lugens. Sci. Rep. 5, 15360. doi:10.1038/srep15360
Zhang Y., Cai T., Ren Z., Liu Y., Yuan M., Cai Y., et al. (2021). Decline in Symbiont-dependent Host Detoxification Metabolism Contributes to Increased Insecticide Susceptibility of Insects under High Temperature. ISME J. 15, 3693–3703. doi:10.1038/s41396-021-01046-1
Zhao B. G., Kong J., Shen X. C., Wang W. X., Cheng H. M., Zhang G. F. (1993). Preliminary Studies on the Biological Control of Ring Rot of Apple Fruit. Acta Agric. boreali—sinica. Acta Agri Bore Sin. 8 (4), 67–70.
Zhu J., Sun W.-q., Li Y., Ge L.-q., Yang G.-q., Xu J.-x., et al. (2020). Effects of a Novel Mesoionic Insecticide, Triflumezopyrim, on the Feeding Behavior of Rice Planthoppers, Nilaparvata Lugens and Sogatella Furcifera (Hemiptera: Delphacidae). J. Integr. Agric. 19 (10), 2488–2499. doi:10.1016/S2095-3119(20)63197-5
Keywords: Nilaparvata lugens, chemical insecticide, antimicrobials, combination, synergistic effect
Citation: Zhao R, Li D, Wang X, Li Z, Yu X and Shentu X (2022) Synergistic and Additive Interactions of Zhongshengmycin to the Chemical Insecticide Pymetrozine for Controlling Nilaparvata lugens (Hemiptera: Delphacidae). Front. Physiol. 13:875610. doi: 10.3389/fphys.2022.875610
Received: 14 February 2022; Accepted: 29 April 2022;
Published: 30 May 2022.
Edited by:
Senthil-Nathan Sengottayan, Manonmaniam Sundaranar University, IndiaReviewed by:
Hu Wan, Huazhong Agricultural University, ChinaJianhong Li, Huazhong Agricultural University, China
Copyright © 2022 Zhao, Li, Wang, Li, Yu and Shentu. This is an open-access article distributed under the terms of the Creative Commons Attribution License (CC BY). The use, distribution or reproduction in other forums is permitted, provided the original author(s) and the copyright owner(s) are credited and that the original publication in this journal is cited, in accordance with accepted academic practice. No use, distribution or reproduction is permitted which does not comply with these terms.
*Correspondence: Xiaoping Yu, eXhwQGNqbHUuZWR1LmNu; Xuping Shentu, c3R4cEBjamx1LmVkdS5jbg==
†These authors have contributed equally to this work