- 1Guangdong Provincial Key Laboratory of Insect Developmental Biology and Applied Technology, Institute of Insect Science and Technology, School of Life Sciences, South China Normal University, Guangzhou, China
- 2Guangdong Provincial Key Laboratory of Insect Developmental Biology and Applied Technology, Guangmeiyuan R&D Center, South China Normal University, Meizhou, China
In the field of insect endocrinology, juvenile hormone (JH) is one of the most wondrous entomological terms. As a unique sesquiterpenoid hormone produced and released by the endocrine gland, corpus allatum (CA), JH is a critical regulator in multiple developmental and physiological processes, such as metamorphosis, reproduction, and behavior. Benefited from the precise genetic interventions and simplicity, the fruit fly, Drosophila melanogaster, is an indispensable model in JH studies. This review is aimed to present the regulatory factors on JH biosynthesis and an overview of the regulatory roles of JH in Drosophila. The future directions of JH studies are also discussed, and a few hot spots are highlighted.
Introduction
Juvenile hormone (JH) primarily produced and secreted from the corpus allatum (CA), fulfills essential roles in many aspects of insect physiology. JH was originally discovered by Wigglesworth (1934) in the triatomine bug, Rhodnius prolixus, to be associated with regulating metamorphosis. Subsequently, JH has been extensively studied in insects, where multiple physiological processes are demonstrated to be controlled by JH, such as reproduction, caste determination and differentiation, diapause, immunity, aging, and behavior (Goodman and Cusson, 2012; Rivera-Perez et al., 2020).
Unlike some holometabolous insects, the application of JH or JH analogs to the fruit fly, Drosophila melanogaster (D. melanogaster), exhibits prolongation of the final larval instar or lethality during pupal-adult transition instead of causing extra larval molting (Bryant and Sang, 1968; Ashburner, 1970; Madhavan, 1973; Postlethwait, 1974; Riddiford and Ashburner, 1991). Despite the disadvantage of studying JH on metamorphosis, Drosophila has been developed as a powerful model system to investigate molecular mechanisms of JH action on a diverse range of biological processes, by capitalizing on a vast array of powerful genetic and molecular approaches (Noriega, 2014; Li K. et al., 2019; Riddiford, 2020). This review attempts to provide an overview of JH research in Drosophila and outline the potential of this organism to understand hormonal regulation of insect development.
Juvenile Hormone Metabolism
Corpus Allatum
The Drosophila CA, which originates from the migration of ectodermal cells in the maxilla and labium during embryogenesis controlled by Hox proteins (Sánchez-Higueras et al., 2014), is an anteromedial subtissue of the ring gland adjacent to the prothoracic gland (PG) and corpora cardiac (CC) during the larval stage (King et al., 1966). CA cells are maintained to be the progenitor of the adult CA, whereas PG is broken down during metamorphosis (Dai and Gilbert, 1991). The mitochondria and smooth endoplasmic reticulum (SER) in the CA cells are considered to be major organelles involved in JH biosynthesis (King et al., 1966; Dai and Gilbert, 1991). CA cell size is proportional to the number of cellular components, which are likely correlated with the production capacity of JH, but the only increase of CA size might not be the principal factor for JH biosynthesis (Zhang J. et al., 2021).
Studies have reported that JH production by the CA maximizes at the larval stage, then declines dramatically after pupariation, sustains a low level in the inactive CA cells of pupa, and increases again after adult emergence (Richard et al., 1989a,b; Altaratz et al., 1991; Dai and Gilbert, 1991). Over 2 days after eclosion, the JH titer appears to be decaying once again (Zhang S. X. et al., 2021). Even so, it is also necessary to perform more accurately qualitative analysis to study the JH biosynthesis in the CA at the different timeline that relies on the development of new technologies (Rivera-Perez et al., 2012). The Aug21-Gal4 is a CA-specific driver (Siegmund and Korge, 2001), which was used for either complete (Liu et al., 2009; Riddiford et al., 2010) or incomplete genetic ablation (Gruntenko et al., 2010, 2012; Bilen et al., 2013; Yamamoto et al., 2013), that impaired JH biosynthesis. The destruction of the CA for manipulating endogenous JH allowed us to examine the role of JH during different stages.
Juvenile Hormone Biosynthesis
In Drosophila, three sesquiterpenoid products, methyl farnesoate (MF), JH III, and the principal form JH bisepoxide (JHB3), are produced in the CA via the mevalonate pathway (Richard et al., 1989a,b; Bellés et al., 2005; Harshman et al., 2010; Jones et al., 2010; Wen et al., 2015). To date, though the entire JH biosynthetic pathway in Drosophila has not been fully defined, the majority of enzymes have been characterized in the steps from acetyl-CoA to JHs.
Juvenile hormone biosynthesis involves multiple enzymatic catalytic reactions and is conventionally divided into early and late steps (Bellés et al., 2005). The early steps follow the mevalonate pathway to form farnesyl pyrophosphate (FPP). The 3-hydroxy-3-methylglutaryl-CoA synthase (HMG-S), 3-hydroxy-3-methylglutaryl CoA reductase (HMGCR), and Farnesyl diphosphate synthase (FPPS) are important regulatory enzymes in the steps of FPP formation (Bellés et al., 2005). In the late steps, farnesoic acid (FA) is converted to MF, JH III, and JHB3. JH acid methyltransferase (JHAMT) is identified as a rate-limiting enzyme that converts FA and JH acid to MF and JH III in insects via in vitro assays (Shinoda and Itoyama, 2003; Niwa et al., 2008; Defelipe et al., 2011). Interestingly, knockout or overexpression of Jhamt in the Drosophila CA has no effect on MF or JH III biosynthesis but alters JHB3 titer in vivo (Bendena et al., 2011; Wen et al., 2015), suggesting that JHAMT is only responsible for JHB3 biosynthesis in Drosophila. Alternately, JHB3 is synthesized by a P450-mediated epoxidation reaction (Moshitzky and Applebaum, 1995). Cytochrome P450 6g2 (Cyp6g2) has emerged as a promising candidate owing to the performance of in situ hybridization and RNAi experiments (Chung et al., 2009; Christesen et al., 2017), while a Cyp6g2 null allele and a Jhamt Cyp6g2 double mutant are urgently required for the understanding JH biosynthesis in Drosophila.
Regulation of Juvenile Hormone Biosynthesis
Nowadays, compared to the classical insect model in JH earlier research, Drosophila with powerful genetic manipulation has become the leader in the search for the regulatory mechanism of JH biosynthesis (Noriega, 2014). In the past 20 years, much progress has been made in understanding the regulation of JH biosynthesis, and various factors have been identified in Drosophila, such as insulin (Tatar et al., 2001; Tu et al., 2005; Belgacem and Martin, 2007), ecdysteroids (Liu et al., 2018), miRNAs (Qu et al., 2017; Zhang J. et al., 2021), biogenic amines (Chiang et al., 2002; Gruntenko et al., 2005, 2007; Huang et al., 2011), Decapentaplegic (Dpp) (Huang et al., 2011), Ecdysis-triggering hormone (ETH) (Meiselman et al., 2017, 2018), and sex peptide (SP) (Moshitzky et al., 1996; Bontonou et al., 2015; Schwenke and Lazzaro, 2017). In essence, JH biosynthesis by the CA is considered to be controlled at the level of the expression of JH biosynthetic enzymes (Hiruma and Kaneko, 2013). Nevertheless, the transcriptional regulatory mechanism of JH biosynthesis is nearly unknown in other insects, Drosophila might be a useful tool to make breakthroughs in this direction.
In insects, nutrition, via the insulin/insulin-like growth factor (IIS)/target of rapamycin (TOR) signaling pathway, mediates its effect on body size partially by regulating JH biosynthesis at specific points during development (Koyama et al., 2013; Zhu et al., 2020). Mutation of Drosophila insulin receptor (InR) decreases JH biosynthesis (Tatar et al., 2001; Tu et al., 2005). Likewise, CA-specific silencing of InR suppresses Hmgcr expression (Belgacem and Martin, 2007), while ectopic activation of the PI3K is sufficient to promote Jhamt expression and CA cell growth (Zhang J. et al., 2021). In response to starvation, increased levels of ecdysteroids, especially 20-hydroxyecdysone (20E), have been demonstrated to negatively regulate JH biosynthesis (Terashima et al., 2005; Meiselman et al., 2017, 2018). Moreover, inhibition of 20E signaling in the CA leads to elevated JH biosynthesis by upregulating Jhamt and Hmgcr, which, in turn, prevents ecdysone biosynthesis in the PG and 20E-induced metamorphosis (Liu et al., 2018; Zhang et al., 2018).
MicroRNAs are a major group of small endogenous non-coding RNAs that act as post-transcriptional regulators of JH biosynthesis and subsequent JH signaling (Qu et al., 2017, 2018). By using high-throughput sequencing, the expression profiles of Drosophila microRNAs have been identified in the ring gland. In combination with the performance of a two-tiered screening approach, miR-8 has been identified as a positive regulator of CA growth and JH biosynthesis (Zhang J. et al., 2021). In addition, over-expression of Bantam using the CA-specific drivers results in the inhibition of Jhamt expression, a decrease of JH titer, and pupal lethality (Qu et al., 2017; Zhang J. et al., 2021). The research studies on JH biosynthesis regulated by microRNAs are still in the initial stage and worth exploring in the future.
Neurons can directly innervate the CA to affect JH biosynthesis by releasing neurotransmitters, in particular, biogenic amines (Siegmund and Korge, 2001; Chiang et al., 2002; Bendena et al., 2020). For example, dopamine influences JH production (Gruntenko et al., 2005, 2007). Moreover, glutamate binds to and activates N-methyl-D-aspartate (NMDA) receptors in the Drosophila CA (Chiang et al., 2002), and activation of the NMDA signaling in the CA indirectly stimulates JH biosynthesis through Dpp signaling-mediated Jhamt expression (Huang et al., 2011). The regulation of JH production also occurs through the actions of neuropeptides (Bendena et al., 2020). Allatostatin-C (AST-C) acts on its receptor (AST-CR1 and AST-CR2) in the CA to inhibit JH biosynthesis (Wang et al., 2012). Alternatively, the neuropeptide ETH, released by endocrine Inka cells, stimulates JH biosynthesis through action on CA in which the ETH receptor gene is expressed (Meiselman et al., 2017). At the adult stage, the male SP with sperm is transferred to the female during mating, and then SP activates JHB3 production in the CA (Moshitzky et al., 1996; Bontonou et al., 2015; Schwenke and Lazzaro, 2017). However, it should be noted that a subset of neurons directly projected to the adult CA might not participate in the regulation of JH biosynthesis, such as hugin neurons (Mizuno et al., 2021).
Over the years, we have learned nothing about how the expression of JH biosynthetic enzymes is regulated by transcription factor (TF) in Drosophila except for apterous (ap) (Postlethwait and Weiser, 1973; Postlethwait and Jones, 1978; Tompkins, 1990; Altaratz et al., 1991; Ringo et al., 1992; Shtorch et al., 1995). Mutation of ap leads to a decrease in JH titer, delayed maturation of adult fat body, and male courtship defects (Tompkins, 1990; Ringo et al., 1992; Shtorch et al., 1995). For further research, integrative approaches, such as transcriptomics, proteomics, and large-scale genetic screens, are promising to identify more TFs implicated in direct the regulation of JH biosynthetic enzymes.
Juvenile Hormone Degradation
Two JH metabolizing enzymes, JH esterase (JHE), largely present in the hemolymph, and JH epoxide hydrolase (JHEH), found in tissues, have been identified in Drosophila (Campbell et al., 1992; Kethidi et al., 2005; Crone et al., 2007). JHE or JHEH causes hydrolysis of the methyl ester or epoxide moiety of JH resulting in the conversion of JH into JH acid or JH diol, respectively (Kamita and Hammock, 2010). The developmental expression levels are quantified and found that JHE mRNA levels increase during JH III peaks in the hemolymph and decrease during ecdysteroid peaks in the hemolymph (Kethidi et al., 2005), suggesting JHE is also controlled by these hormones. Over-expression of a JHE-binding protein resulted in adult phenotypes is associated with decreased JH (Liu et al., 2008). Thus, the balance between JH biosynthesis and degradation is contributed to the stringent regulation of JH, which is essential for normal insect development and metamorphosis.
Juvenile Hormone Signaling Transduction
Intracellular Receptor
The discovery of gene Methoprene-tolerant (Met) by Wilson and Fabian nearly 35 years ago in Drosophila (Wilson and Fabian, 1986) was a milestone event for understanding JH signaling transduction, although the key features of Met as the JH receptor were underscored in a non-fruit fly model (Konopova and Jindra, 2007). In 1986, the mutation at this locus was obtained by Wilson lab using mutagenesis screen, and they showed that Drosophila with loss of Met is highly resistant to the toxic effects of JH analog methoprene (Wilson and Fabian, 1986). Unlike the lethality by CA ablation (Liu et al., 2009; Riddiford et al., 2010), Met null mutation was viable with subtle defects in phenotypes (Wilson and Fabian, 1986; Ashok et al., 1998; Wilson and Ashok, 1998). It seemed that Met encodes a non-vital protein and another gene appears to function redundantly in JH reception (Wilson and Ashok, 1998).
As the members of the basic helix-loop-helix (bHLH)-Per-Arnt-Sim (PAS) family of TFs, Met is derived from the ancestral gene germ cell-expressed (Gce) (Baumann et al., 2010). Both Met and Gce bind to JH III and MF and JH analogs with high affinity in the PAS-B domain (Shemshedini and Wilson, 1990; Ashok et al., 1998; Miura et al., 2005; Charles et al., 2011; Jindra et al., 2015b; Bittova et al., 2019). They form homodimers or heterodimers and JH reduces this dimerization (Godlewski et al., 2006). Defective phenotypes, such as precocious and enhanced programmed cell death (PCD) and pupal lethality in Met/Gce double mutant, are similar to those found in JH-deficient flies (Liu et al., 2009; Abdou et al., 2011). Importantly, they could be rescued by exogenous JH analog pyriproxyfen in JH-deficient flies but not in Met/Gce double mutant (Abdou et al., 2011). The requirement of direct JH-binding capacity to Met/Gce in vivo for JH action is required for the fly normal development (Jindra et al., 2015b). All findings together demonstrate that Met and Gce mediate the effects of JH as the intracellular JH receptor.
Signaling Transduction
Identification of JH receptor accelerates the research studies on JH intracellular signaling transduction in Drosophila. In detail, Met heterodimerizes with another bHLH-PAS protein Taiman (Tai; steroid response coactivator, SRC or βFtz-F1 Interacting Steroid Receptor Coactivator, FISC) after binding of JH (Li et al., 2011). βFTZ-F1 is also an essential binding protein of Met/Gce for JH signaling (Dubrovsky et al., 2011; Bernardo and Dubrovsky, 2012). As a TF, Met is predominantly localized in the nuclei of cultured cells (Miura et al., 2005; Greb-Markiewicz et al., 2011) and tissues (Pursley et al., 2000). The chaperone heat shock protein 83 (Hsp83) facilitates this Met nuclear import by physically interacting with its PAS-B and bHLH domains (He et al., 2014). Subsequently, Nucleoporin 358 kD (Nup358) promotes the JH-Met-Hsp83 complex to transport into the nucleus dependent on importin β (He et al., 2017). Finally, the Met-cofactors complex binds to the JH response region (JHRR) directly and regulates the expression of JH response genes (He et al., 2014, 2017). The zinc-finger TF Krüppel-homolog 1 (Kr-h1) acts as an early JH-response gene and is recognized as the anti-metamorphosis factor (Minakuchi et al., 2008; Huang et al., 2011; Liu et al., 2018).
Physiological Actions of Juvenile Hormone Intracellular Signaling
Metamorphosis
Juvenile hormone was originally discovered for its capacity to prevent metamorphosis (Wigglesworth, 1934; Riddiford, 2020). The prominent metamorphic events in Drosophila include the destruction of most larval structures and tissue remodeling. 20E orchestrates these diverse cellular events, and JH prevents 20E-induced metamorphosis via the JH receptor and Kr-h1, both of which are critical for the normal development of insects (Jindra et al., 2013, 2015a). As the main organ of the intermediate metabolism of insects, the fat body plays a central role in the integration of hormonal signals to regulate metamorphosis (Li S. et al., 2019). For example, Kr-h1 transduces the JH intracellular signal to repress 20E responsive genes, namely, Broad-complex (Br-C) and ecdysone-inducible proteins E93 (E93), which subsequently inhibit 20E-induced precocious program cell death of the larval fat body (Minakuchi et al., 2008; Abdou et al., 2011; Belles and Santos, 2014; Liu X. et al., 2015). Moreover, precocious fat body cell dissociation was observed in both JH-deficient animals and Met/Gce double-mutant animals (Liu et al., 2009; Abdou et al., 2011). Kr-h1 represses matrix metalloproteinases (Mmps) expression and thus prevents fat body cell dissociation during the larval-prepupal transition (Jia et al., 2017). Likewise, JH signaling prevents the precocious formation of adult organs, such as the optic lobe. JH removal by CA ablation resulted in precocious optic lobe development during the prepupal period whereas JH application suppressed this visual system defect (Riddiford et al., 2010, 2018). The direct and transient repression of Kr-h1 by Orthodenticle (Otd) and Ecdysone receptor (EcR) is required for correct photoreceptor maturation, also exhibiting the anti-metamorphosis activity of Kr-h1 in remodeling neurons (Fichelson et al., 2012). With the generation of genetic tools for JH research (He et al., 2014; Baumann et al., 2017), more functions of JH in target tissues during metamorphosis or other processes will be uncovered.
On the other hand, JH can suppress ecdysone synthesis of the PG in vitro and in vivo (Richard and Gilbert, 1991; Liu et al., 2018; Zhang et al., 2018). Knockdown of Kr-h1 in the PG results in precocious metamorphosis and pupal lethality, implying the direct regulatory function on ecdysone synthesis (Danielsen et al., 2016; Liu et al., 2018). Indeed, JH directly targets PG to inhibit ecdysone biosynthesis by reducing steroidogenesis autoregulation, PG size, and expression of the steroidogenic enzymes (Liu et al., 2018; Zhang et al., 2018). At the epigenetic level, JH impairs polycomb repressive complex 2 (PRC2)-mediated histone H3 lysine 27 (H3K27) methylation and thereby induces hairy expression, and thus inhibits ecdysone biosynthesis by repressing expression of the steroidogenic enzyme to regulate metamorphosis (Yang et al., 2021). The epigenetic regulatory mechanism of JH action will certainly shed light on hormone regulation in animals.
Reproduction
Juvenile hormone evolves as a gonadotrophic hormone (Dubrovsky et al., 2002; Riddiford, 2012; Santos et al., 2019), which has been implicated in vitellogenesis and yolk protein uptake in Drosophila females (Postlethwait and Weiser, 1973; Bownes, 1989; Saunders et al., 1990; Soller et al., 1999; Riddiford, 2012), larval fat body histolysis (Postlethwait and Jones, 1978; Yamamoto et al., 2013), and male accessory gland protein synthesis (Yamamoto et al., 1988; Shemshedini et al., 1990; Wolfner et al., 1997; Wilson et al., 2003).
Previous studies have shown that incomplete ablation of the CA or mutation of Jhamt results in the reduction of JH level with an associated reduction in fecundity and ovary size (Gruntenko et al., 2010; Wen et al., 2015). These reproductive deficiencies are caused by decreases of JH-induced Vg production in the fat body and Vg uptake by the oocytes (Luo et al., 2021) or probably due to reduced germline stem cells (Luo et al., 2020). A recent study reports that the single null mutant of JH receptors, Met27 or Gce2.5K, also shows decreased fecundity but with abnormal egg shape and ovary size gradually increase. Subsequently, a novel mechanism for JH-regulated Drosophila reproduction is uncovered that JH intracellular signaling induces Laminin or Collagen IV gene expressions in ovarian muscle or fat body cells, respectively, which are contributed to the assembly of ovarian muscle extracellular matrix (ECM) that is indispensable for ovarian muscle contraction, then ovarian muscle contraction externally generates a mechanical force to promote ovulation and maintain egg shape (Luo et al., 2021).
Behavior
Besides the roles in metamorphosis and reproduction, JH is also known to play roles in the behaviors of Drosophila. After eclosion, JH regulates the maturation of female receptivity by promoting the production of sex pheromone (Manning, 1966; Ringo et al., 1991; Bilen et al., 2013). As for mature males, knockdown of Jhamt significantly reduced courtship that could be rescued by the application of JH analogs, suggesting the physiological role of JH in male courtship behavior (Wijesekera et al., 2016). Moreover, JH potentiates the sensitivity of a pheromone sensing olfactory receptor OR47b to maximize courtship success (Lin et al., 2016). The activation of Ca2+/calmodulin-dependent protein kinase I (CaMKI) and CREB-binding protein (CBP) enhances the efficacy of JH in male Or47b neurons to modulate pheromone detection and thereby regulate courtship behavior (Sethi et al., 2019). Interestingly, there is a piece of evidence that JH suppresses mating behavior by activating TF cyclic adenosine 3’,5’-monophosphate (cAMP) response element-binding protein 2 (CREB2) in juvenile males (Zhang S. X. et al., 2021), suggesting the complex regulatory function of JH on courtship behavior. Additionally, JH signaling influences the short-term and long-term courtship memory of males by acting on diverse neural circuits (Lee et al., 2017; Lee and Adams, 2021). Furthermore, JH signaling controls sexual dimorphic behaviors, such as locomotion and sleep (Belgacem and Martin, 2007; Wu et al., 2018; Wu B. et al., 2021). Investigating the JH-regulated sexually dimorphic behaviors emerges as a promising direction in JH studies in Drosophila.
Conceivably, the known JH intracellular receptors, Met and Gce, mediate the action of JH on different behaviors but their functions are not fully redundant. For females, JH regulates mating and pheromone production primarily via Met (Bilen et al., 2013). For males, on the one hand, Met is necessary for both normal fertility and courtship behavior through modulating Or47b sensitivity (Wilson et al., 2003; Lin et al., 2016). On the other hand, Met expression in dopaminergic (DA) neurons and mushroom body (MB) γ lobe neurons is essential for courtship short-term and long-term courtship memory, respectively (Lee et al., 2017; Lee and Adams, 2021). However, Gce is dispensable for long-term courtship memory (Lee and Adams, 2021). In addition, Met mutant increases sleep in both males and females, but Gce deletion mutant exhibits sexually dimorphic effects on sleep (Wu et al., 2018, Wu B. et al., 2021). There are lots of possible factors for their different functions, for example, the differentiated subcellular and tissue distribution (Baumann et al., 2017; Kolonko et al., 2020) or the disruption of Met-Gce dimerization by JH (Godlewski et al., 2006; Zhang S. X. et al., 2021).
Juvenile Hormone Membrane Signaling and Action
Juvenile hormone might rapidly exert non-genomic actions through putative plasma membrane receptors in a wide range of insects’ studies (Jindra et al., 2015a). For example, a potential member(s) of the receptor tyrosine kinase (RTK) family might function as the membrane receptor of JH in Diptera insects, namely, Drosophila (Liu P. et al., 2015). This JH-RTK pathway activates the phospholipase C (PLC) pathway, leading to the phosphorylation and activation of calcium/CaMKII and protein kinase C (PKC), which subsequently induce phosphorylation of Met and Tai, thus regulating the activity of JH intracellular signaling (Liu P. et al., 2015; Ojani et al., 2016). However, the study on the JH membrane pathway in Drosophila is very limited. About three decades ago, Yamamoto et al. (1988) showed that JH regulates protein synthesis in the male accessory glands by activating the PKC pathway, implying the existence and importance of JH membrane signaling (Yamamoto et al., 1988). Until 2021, using genetics and quantitative phosphoproteomics methods, Gao et al. (2021) discovered that JH phosphorylated ultraspiracle protein (USP) at Ser35 through the RTK-PLC-PKC pathway to maximize 20E signal transduction even in the absence of JH intracellular signaling (Gao et al., 2021). It will be beneficial to identify the JH membrane receptors and advance our understanding of the complex JH signaling network.
Concluding Remarks
Given that the roles of JH are multidirectional and complex, model organisms, such as Drosophila and other insects, provide an ideal framework to understand the molecular and cellular mechanisms of JH action regulating insect physiology in response to diverse environmental cues. In this review, we have summarized the knowledge that known factors controlling JH biosynthesis, JH signaling transduction, and its essential impacts on physiological outputs focus on its roles in metamorphosis, reproduction, and behaviors (Figure 1). Based on the accessibility of genetic tools and simplicity of genome, the fruit fly D. melanogaster has made great contributions to the field of JH, particularly in the discovery of JH intracellular receptors. Despite that, some questions still need to address in the future.
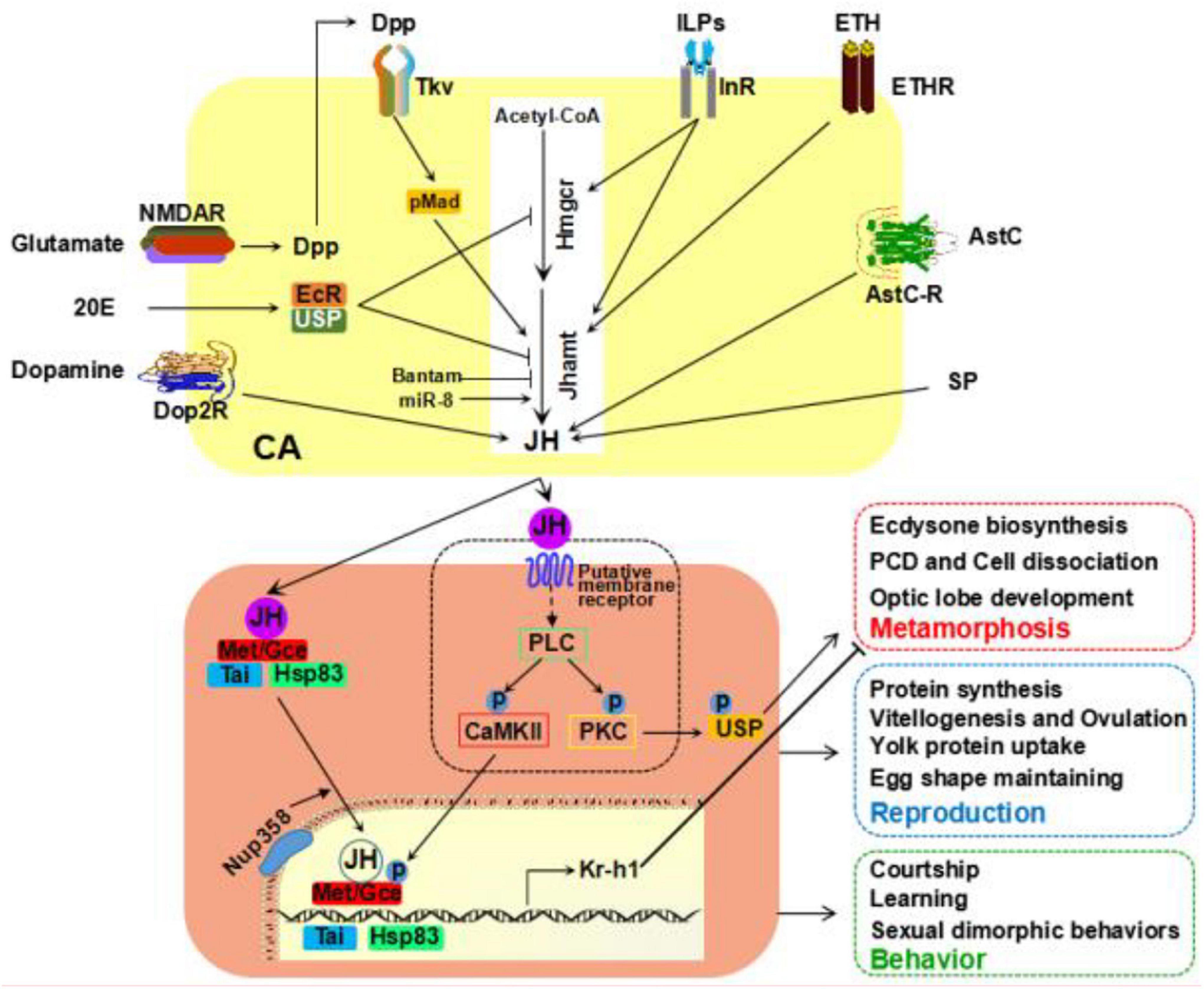
Figure 1. Recent studies on JH studies in Drosophila. Examples of various factors affecting JH biosynthesis in corpus allatum (CA) cells at the larval or adult stage. JH employees both intracellular and membrane receptors for signal transduction. JH signaling plays an essential role in multiple physiological processes. JH, juvenile hormone.
Although JH action has been investigated mostly in the postembryonic development, its embryonic functions remain unclear. Suppression of JH biosynthesis or JH signaling in Bombyx mori or Tribolium castaneum results in minor embryonic developmental defects (Shinoda and Itoyama, 2003; Daimon et al., 2015). Conversely, JH signaling is necessary for embryogenesis in some hemimetabolous species, namely, Blattella germanica (Fernandez-Nicolas and Belles, 2017). It reveals the complexity of JH action on embryonic development in different species. In Drosophila, the larvae can survive up to the end of the larval stage whether they are genetically allatectomized, Met/Gce double mutant, or Jhamt mutant (Liu et al., 2009; Riddiford et al., 2010; Abdou et al., 2011; Wen et al., 2015). It seems that JH signaling is unimportant for embryonic or even early larval development in this species. However, a recent study reports that JH signaling is activated in mid-embryogenesis prior to CA development, and JH is required for migrating germ cells to reach the somatic gonad via a non-canonical pathway (Barton et al., 2021). Despite JH embryonic functions are relatively minor, Drosophila is still a powerful model to explore how JH affects embryonic development.
Our current understanding of JH actions is largely based on phenotypic defects induced by JH treatment or removal, and mutations or RNAi of JH signaling, which have limitations to analyze the JH functions in detail since more putative components of the JH signaling cascades, such as binding proteins and novel targets of JH intracellular receptors, putative JH membrane receptor, and targets of Kr-h1, need to be identified and characterized. Moreover, in Drosophila, Kr-h1 is mainly considered as the transcriptional repressor to antagonize 20E signaling, whereas it also functions as a transcriptional activator in the adult Locusta migratoria by recruiting CBP after phosphorylation (Wu Z. et al., 2021). The evolutionary conservation and more detailed analysis of transcriptional activation activity of Kr-h1 in Drosophila, and post-translational modification (Kim et al., 2021; Wu Z. et al., 2021), will further dissect the JH functions in directing insect development.
Author Contributions
XZ and SNL drafted and wrote the manuscript and figures. SL provided conception, guidance, editing, and support with the manuscript. All authors contributed to the article and reviewed the final manuscript.
Funding
This work was supported by the National Science Foundation of China (Grant Nos. 32070441, 31620103917, and 31930014), the Shenzhen Science and Technology Program (Grant No. 20180411143628272), the National Science Foundation of Guangdong Province (Grant No. 2019A1515011899), and the Science and Technology Plan Project of Guangzhou (Grant No. 202102020572).
Conflict of Interest
The authors declare that the research was conducted in the absence of any commercial or financial relationships that could be construed as a potential conflict of interest.
Publisher’s Note
All claims expressed in this article are solely those of the authors and do not necessarily represent those of their affiliated organizations, or those of the publisher, the editors and the reviewers. Any product that may be evaluated in this article, or claim that may be made by its manufacturer, is not guaranteed or endorsed by the publisher.
References
Abdou, M. A., He, Q., Wen, D., Zyaan, O., Wang, J., Xu, J., et al. (2011). Drosophila Met and Gce are partially redundant in transducing juvenile hormone action. Insect Biochem. Mol. Biol. 41, 938–945. doi: 10.1016/j.ibmb.2011.09.003
Altaratz, M., Applebaum, S. W., Richard, D. S., Gilbert, L. I., and Segal, D. (1991). Regulation of juvenile hormone synthesis in wild-type and apterous mutant Drosophila. Mol. Cell. Endocrinol. 81, 205–216. doi: 10.1016/0303-7207(91)90219-i
Ashburner, M. (1970). Effects of Juvenile Hormone on Adult Differentiation of Drosophila melanogaster. Nature 227, 187–189. doi: 10.1038/227187a0
Ashok, M., Turner, C., and Wilson, T. G. (1998). Insect juvenile hormone resistance gene homology with the bHLH-PAS family of transcriptional regulators. Proc. Natl. Acad. Sci. U.S.A. 95, 2761–2766. doi: 10.1073/pnas.95.6.2761
Barton, L. J., Sanny, J., Dawson, E. P., Nouzova, M., Noriega, F. G., Stadtfeld, M., et al. (2021). Bioactive isoprenoids guide migrating germ cells to the embryonic gonad. bioRxiv 2021 09, 462471. doi: 10.1101/2021.09.30.462471
Baumann, A., Fujiwara, Y., and Wilson, T. G. (2010). Evolutionary divergence of the paralogs Methoprene tolerant (Met) and germ cell expressed (gce) within the genus Drosophila. J. Insect. Physiol. 56, 1445–1455. doi: 10.1016/j.jinsphys.2010.05.001
Baumann, A. A., Texada, M. J., Chen, H. M., Etheredge, J. N., Miller, D. L., Picard, S., et al. (2017). Genetic tools to study juvenile hormone action in Drosophila. Sci. Rep. 7:2132. doi: 10.1038/s41598-017-02264-4
Belgacem, Y. H., and Martin, J. R. (2007). Hmgcr in the corpus allatum controls sexual dimorphism of locomotor activity and body size via the insulin pathway in Drosophila. PLoS One 2:e187. doi: 10.1371/journal.pone.0000187
Bellés, X., Martín, D., and Piulachs, M. D. (2005). The mevalonate pathway and the synthesis of juvenile hormone in insects. Annu. Rev. Entomol. 50, 181–199. doi: 10.1146/annurev.ento.50.071803.130356
Belles, X., and Santos, C. G. (2014). The MEKRE93 (Methoprene tolerant-Krüppel homolog 1-E93) pathway in the regulation of insect metamorphosis, and the homology of the pupal stage. Insect Biochem. Mol. Biol. 52, 60–68. doi: 10.1016/j.ibmb.2014.06.009
Bendena, W. G., Hui, J. H. L., Chin-Sang, I., and Tobe, S. S. (2020). Neuropeptide and microRNA regulators of juvenile hormone production. Gen. Comp. Endocrinol. 295:113507. doi: 10.1016/j.ygcen.2020.113507
Bendena, W. G., Zhang, J., Burtenshaw, S. M., and Tobe, S. S. (2011). Evidence for differential biosynthesis of juvenile hormone (and related) sesquiterpenoids in Drosophila melanogaster. Gen. Comp. Endocrinol. 172, 56–61. doi: 10.1016/j.ygcen.2011.02.014
Bernardo, T. J., and Dubrovsky, E. B. (2012). The Drosophila juvenile hormone receptor candidates methoprene-tolerant (MET) and germ cell-expressed (GCE) utilize a conserved LIXXL motif to bind the FTZ-F1 nuclear receptor. J. Biol. Chem. 287, 7821–7833. doi: 10.1074/jbc.M111.327254
Bilen, J., Atallah, J., Azanchi, R., Levine, J. D., and Riddiford, L. M. (2013). Regulation of onset of female mating and sex pheromone production by juvenile hormone in Drosophila melanogaster. Proc. Natl. Acad. Sci. U.S.A. 110, 18321–18326. doi: 10.1073/pnas.1318119110
Bittova, L., Jedlicka, P., Dracinsky, M., Kirubakaran, P., Vondrasek, J., Hanus, R., et al. (2019). Exquisite ligand stereoselectivity of a Drosophila juvenile hormone receptor contrasts with its broad agonist repertoire. J. Biol. Chem. 294, 410–423. doi: 10.1074/jbc.RA118.005992
Bontonou, G., Shaik, H. A., Denis, B., and Wicker-Thomas, C. (2015). Acp70A regulates Drosophila pheromones through juvenile hormone induction. Insect Biochem. Mol. Biol. 56, 36–49. doi: 10.1016/j.ibmb.2014.11.008
Bownes, M. (1989). The roles of juvenile hormone, ecdysone and the ovary in the control of Drosophila vitellogenesis. J. Insect Physiol. 35, 409–413. doi: 10.1016/0022-1910(89)90115-7
Bryant, P. J., and Sang, J. H. (1968). Drosophila: lethal derangements of metamorphosis and modifications of gene expression caused by juvenile hormone mimics. Nature 220, 393–394. doi: 10.1038/220393a0
Campbell, P. M., Healy, M. J., and Oakeshott, J. G. (1992). Characterisation of juvenile hormone esterase in Drosophila melanogaster. Insect Biochem. Mol. Biol. 22, 665–677. doi: 10.1016/0965-1748(92)90045-G
Charles, J. P., Iwema, T., Epa, V. C., Takaki, K., Rynes, J., and Jindra, M. (2011). Ligand-binding properties of a juvenile hormone receptor. Proc. Natl. Acad. Sci. U.S.A. 108, 21128–21133. doi: 10.1073/pnas.1116123109
Chiang, A. S., Lin, W. Y., Liu, H. P., Pszczolkowski, M. A., Fu, T. F., Chiu, S. L., et al. (2002). Insect NMDA receptors mediate juvenile hormone biosynthesis. Proc. Natl. Acad. Sci. U.S. 99, 37–42. doi: 10.1073/pnas.012318899
Christesen, D., Yang, Y. T., Somers, J., Robin, C., Sztal, T., Batterham, P., et al. (2017). Transcriptome Analysis of Drosophila melanogaster Third Instar Larval Ring Glands Points to Novel Functions and Uncovers a Cytochrome p450 Required for Development. G3 7, 467–479. doi: 10.1534/g3.116.037333
Chung, H., Sztal, T., Pasricha, S., Sridhar, M., Batterham, P., and Daborn, P. J. (2009). Characterization of Drosophila melanogaster cytochrome P450 genes. Proc. Natl. Acad. Sci. U.S. 106, 5731–5736. doi: 10.1073/pnas.0812141106
Crone, E. J., Sutherland, T. D., Campbell, P. M., Coppin, C. W., Russell, R. J., and Oakeshott, J. G. (2007). Only one esterase of Drosophila melanogaster is likely to degrade juvenile hormone in vivo. Insect Biochem. Mol. Biol. 37, 540–549. doi: 10.1016/j.ibmb.2007.02.010
Dai, J. D., and Gilbert, L. I. (1991). Metamorphosis of the corpus allatum and degeneration of the prothoracic glands during the larval-pupal-adult transformation of Drosophila melanogaster: a cytophysiological analysis of the ring gland. Dev. Biol. 144, 309–326. doi: 10.1016/0012-1606(91)90424-2
Daimon, T., Uchibori, M., Nakao, H., Sezutsu, H., and Shinoda, T. (2015). Knockout silkworms reveal a dispensable role for juvenile hormones in holometabolous life cycle. Proc. Natl. Acad. Sci. U.S. 112, E4226–E4235. doi: 10.1073/pnas.1506645112
Danielsen, E. T., Moeller, M. E., Yamanaka, N., Ou, Q., Laursen, J. M., Soenderholm, C., et al. (2016). A Drosophila Genome-Wide Screen Identifies Regulators of Steroid Hormone Production and Developmental Timing. Dev. Cell 37, 558–570. doi: 10.1016/j.devcel.2016.05.015
Defelipe, L. A., Dolghih, E., Roitberg, A. E., Nouzova, M., Mayoral, J. G., Noriega, F. G., et al. (2011). Juvenile hormone synthesis: “esterify then epoxidize” or “epoxidize then esterify”? Insights from the structural characterization of juvenile hormone acid methyltransferase. Insect Biochem. Mol. Biol 41, 228–235. doi: 10.1016/j.ibmb.2010.12.008
Dubrovsky, E. B., Dubrovskaya, V. A., and Berger, E. M. (2002). Juvenile hormone signaling during oogenesis in Drosophila melanogaster. Insect Biochem. Mol. Biol. 32, 1555–1565. doi: 10.1016/s0965-1748(02)00076-0
Dubrovsky, E. B., Dubrovskaya, V. A., Bernardo, T., Otte, V., Difilippo, R., and Bryan, H. (2011). The Drosophila FTZ-F1 nuclear receptor mediates juvenile hormone activation of E75A gene expression through an intracellular pathway. J. Biol. Chem. 286, 33689–33700. doi: 10.1074/jbc.M111.273458
Fernandez-Nicolas, A., and Belles, X. (2017). Juvenile hormone signaling in short germ-band hemimetabolan embryos. Development 144, 4637–4644. doi: 10.1242/dev.152827
Fichelson, P., Brigui, A., and Pichaud, F. (2012). Orthodenticle and Kruppel homolog 1 regulate Drosophila photoreceptor maturation. Proc. Natl. Acad. Sci. U.S. 109, 7893–7898.
Gao, Y., Liu, S., Jia, Q., Wu, L., Yuan, D., Li, E. Y., et al. (2021). Juvenile hormone membrane signaling phosphorylates USP and thus potentiates 20-hydroxyecdysone action in Drosophila. Sci. Bull. 289, 26630–26641. doi: 10.1016/j.scib.2021.06.019
Godlewski, J., Wang, S., and Wilson, T. G. (2006). Interaction of bHLH-PAS proteins involved in juvenile hormone reception in Drosophila. Biochem. Biophys. Res. Commun. 342, 1305–1311. doi: 10.1016/j.bbrc.2006.02.097
Goodman, W. G., and Cusson, M. J. I. E. (2012). “The juvenile hormones,” in Insect Endocrinology, ed. L. I. Gilbert (London: Elsevier), 310–365. doi: 10.1016/B978-0-12-384749-2.10008-1
Greb-Markiewicz, B., Orłowski, M., Dobrucki, J., and Ożyhar, A. (2011). Sequences that direct subcellular traffic of the Drosophila methoprene-tolerant protein (MET) are located predominantly in the PAS domains. Mol. Cell. Endocrinol. 345, 16–26. doi: 10.1016/j.mce.2011.06.035
Gruntenko, N. E., Bogomolova, E. V., Adonyeva, N. V., Karpova, E. K., Menshanov, P. N., Alekseev, A. A., et al. (2012). Decrease in juvenile hormone level as a result of genetic ablation of the corpus allatum cells affects the synthesis and metabolism of stress related hormones in Drosophila. J. Insect Physiol. 58, 49–55. doi: 10.1016/j.jinsphys.2011.09.015
Gruntenko, N. E., Karpova, E. K., Alekseev, A. A., Chentsova, N. A., Bogomolova, E. V., Bownes, M., et al. (2007). Effects of octopamine on reproduction, juvenile hormone metabolism, dopamine, and 20-hydroxyecdysone contents in Drosophila. Arch. Insect Biochem. Physiol. 65, 85–94. doi: 10.1002/arch.20187
Gruntenko, N. E., Karpova, E. K., Alekseev, A. A., Chentsova, N. A., Saprykina, Z. V., Bownes, M., et al. (2005). Effects of dopamine on juvenile hormone metabolism and fitness in Drosophila virilis. J. Insect Physiol. 51, 959–968. doi: 10.1016/j.jinsphys.2005.04.010
Gruntenko, N. E., Wen, D., Karpova, E. K., Adonyeva, N. V., Liu, Y., He, Q., et al. (2010). Altered juvenile hormone metabolism, reproduction and stress response in Drosophila adults with genetic ablation of the corpus allatum cells. Insect Biochem. Mol. Biol. 40, 891–897. doi: 10.1016/j.ibmb.2010.09.001
Harshman, L. G., Song, K. D., Casas, J., Schuurmans, A., Kuwano, E., Kachman, S. D., et al. (2010). Bioassays of compounds with potential juvenoid activity on Drosophila melanogaster: juvenile hormone III, bisepoxide juvenile hormone III and methyl farnesoates. J. Insect Physiol. 56, 1465–1470. doi: 10.1016/j.jinsphys.2010.06.003
He, Q., Wen, D., Jia, Q., Cui, C., Wang, J., Palli, S. R., et al. (2014). Heat shock protein 83 (Hsp83) facilitates methoprene-tolerant (Met) nuclear import to modulate juvenile hormone signaling. J. Biol. Chem. 289, 27874–27885. doi: 10.1074/jbc.M114.582825
He, Q., Zhang, Y., Zhang, X., Xu, D., Dong, W., Li, S., et al. (2017). Nucleoporin Nup358 facilitates nuclear import of Methoprene-tolerant (Met) in an importin (- and Hsp83-dependent manner. Insect Biochem. Mol. Biol. 81, 10–18. doi: 10.1016/j.ibmb.2016.12.005
Hiruma, K., and Kaneko, Y. (2013). Hormonal regulation of insect metamorphosis with special reference to juvenile hormone biosynthesis. Curr. Top. Dev. Biol. 103, 73–100. doi: 10.1016/B978-0-12-385979-2.00003-4
Huang, J., Tian, L., Peng, C., Abdou, M., Wen, D., Wang, Y., et al. (2011). DPP-mediated TGFbeta signaling regulates juvenile hormone biosynthesis by activating the expression of juvenile hormone acid methyltransferase. Development 138, 2283–2291. doi: 10.1242/dev.057687
Jia, Q., Liu, S., Wen, D., Cheng, Y., Bendena, W. G., Wang, J., et al. (2017). Juvenile hormone and 20-hydroxyecdysone coordinately control the developmental timing of matrix metalloproteinase-induced fat body cell dissociation. J. Biol. Chem. 292, 21504–21516. doi: 10.1074/jbc.M117.818880
Jindra, M., Bellés, X., and Shinoda, T. (2015a). Molecular basis of juvenile hormone signaling. Curr. Opin. Insect. Sci. 11, 39–46. doi: 10.1016/j.cois.2015.08.004
Jindra, M., Uhlirova, M., Charles, J. P., Smykal, V., and Hill, R. J. (2015b). Genetic Evidence for Function of the bHLH-PAS Protein Gce/Met As a Juvenile Hormone Receptor. PLoS Genet. 11:e1005394. doi: 10.1371/journal.pgen.1005394
Jindra, M., Palli, S. R., and Riddiford, L. M. (2013). The juvenile hormone signaling pathway in insect development. Annu. Rev. Entomol. 58, 181–204. doi: 10.1146/annurev-ento-120811-153700
Jones, G., Jones, D., Li, X., Tang, L., Ye, L., Teal, P., et al. (2010). Activities of natural methyl farnesoids on pupariation and metamorphosis of Drosophila melanogaster. J. Insect Physiol. 56, 1456–1464. doi: 10.1016/j.jinsphys.2010.06.001
Kamita, S. G., and Hammock, B. D. (2010). Juvenile hormone esterase: biochemistry and structure. J. Pestic. Sci. 35, 265–274. doi: 10.1584/jpestics.R10-09
Kethidi, D. R., Xi, Z., and Palli, S. R. (2005). Developmental and hormonal regulation of juvenile hormone esterase gene in Drosophila melanogaster. J. Insect Physiol. 51, 393–400. doi: 10.1016/j.jinsphys.2004.12.007
Kim, K., Albishi, N. M., and Palli, S. R. (2021). Identification of juvenile hormone-induced posttranslational modifications of methoprene tolerant and Krüppel homolog 1 in the yellow fever mosquito. Aedes Aegypti. J. Proteomics. 242:104257. doi: 10.1016/j.jprot.2021.104257
King, R. C., Aggarwal, S. K., and Bodenstein, D. (1966). The comparative submicroscopic morphology of the ring gland of Drosophila melanogaster during the second and third larval instars. Z. Zellforsch. Mikrosk. Anat. 73, 272–285. doi: 10.1007/BF00334868
Kolonko, M., Bystranowska, D., Taube, M., Kozak, M., Bostock, M., Popowicz, G., et al. (2020). The intrinsically disordered region of GCE protein adopts a more fixed structure by interacting with the LBD of the nuclear receptor FTZ-F1. Cell Commun. Signal. 18:180.
Konopova, B., and Jindra, M. (2007). Juvenile hormone resistance gene Methoprene-tolerant controls entry into metamorphosis in the beetle Tribolium castaneum. Proc. Natl. Acad. Sci. U.S.A. 104, 10488–10493. doi: 10.1073/pnas.0703719104
Koyama, T., Mendes, C. C., and Mirth, C. K. (2013). Mechanisms regulating nutrition-dependent developmental plasticity through organ-specific effects in insects. Front. Physiol. 4:263. doi: 10.3389/fphys.2013.00263
Lee, S. S., and Adams, M. E. (2021). Regulation of Drosophila Long-Term Courtship Memory by Ecdysis Triggering Hormone. Front. Neurosci. 15:670322. doi: 10.3389/fnins.2021.670322
Lee, S. S., Ding, Y., Karapetians, N., Rivera-Perez, C., Noriega, F. G., and Adams, M. E. (2017). Hormonal Signaling Cascade during an Early-Adult Critical Period Required for Courtship Memory Retention in Drosophila. Curr. Biol. 27, 2798–2809.e3. doi: 10.1016/j.cub.2017.08.017
Li, K., Jia, Q., and Li, S. (2019). Juvenile hormone signaling - a mini review. Insect Sci. 26, 600–606. doi: 10.1111/1744-7917.12614
Li, S., Yu, X., and Feng, Q. (2019). Fat Body Biology in the Last Decade. Annu. Rev. Entomol. 64, 315–333. doi: 10.1146/annurev-ento-011118-112007
Li, M., Mead, E. A., and Zhu, J. (2011). Heterodimer of two bHLH-PAS proteins mediates juvenile hormone-induced gene expression. Proc. Natl. Acad. Sci. U.S.A. 108, 638–643. doi: 10.1073/pnas.1013914108
Lin, H. H., Cao, D. S., Sethi, S., Zeng, Z., Chin, J. S. R., Chakraborty, T. S., et al. (2016). Hormonal Modulation of Pheromone Detection Enhances Male Courtship Success. Neuron 90, 1272–1285. doi: 10.1016/j.neuron.2016.05.004
Liu, P., Peng, H.-J., and Zhu, J. (2015). Juvenile hormone-activated phospholipase C pathway enhances transcriptional activation by the methoprene-tolerant protein. Proc. Natl. Acad. Sci. U.S.A. 112, E1871–E1879. doi: 10.1073/pnas.1423204112
Liu, X., Dai, F., Guo, E., Li, K., Ma, L., Tian, L., et al. (2015). 20-Hydroxyecdysone (20E) Primary Response Gene E93 Modulates 20E Signaling to Promote Bombyx Larval-Pupal Metamorphosis. J. Biol. Chem. 290, 27370–27383. doi: 10.1074/jbc.M115.687293
Liu, S., Li, K., Gao, Y., Liu, X., Chen, W., Ge, W., et al. (2018). Antagonistic actions of juvenile hormone and 20-hydroxyecdysone within the ring gland determine developmental transitions in Drosophila. Proc. Natl. Acad. Sci. U.S.A. 115, 139–144. doi: 10.1073/pnas.1716897115
Liu, Y., Sheng, Z., Liu, H., Wen, D., He, Q., Wang, S., et al. (2009). Juvenile hormone counteracts the bHLH-PAS transcription factors MET and GCE to prevent caspase-dependent programmed cell death in Drosophila. Development 136, 2015–2025. doi: 10.1242/dev.033712
Liu, Z., Li, X., Prasifka, J. R., Jurenka, R., and Bonning, B. C. (2008). Overexpression of Drosophila juvenile hormone esterase binding protein results in anti-JH effects and reduced pheromone abundance. Gen. Comp. Endocrinol. 156, 164–172. doi: 10.1016/j.ygcen.2008.01.006
Luo, W., Liu, S., Zhang, W., Yang, L., Huang, J., Zhou, S., et al. (2021). Juvenile hormone signaling promotes ovulation and maintains egg shape by inducing expression of extracellular matrix genes. Proc. Natl. Acad. Sci. U.S.A. 118:e2104461118. doi: 10.1073/pnas.2104461118
Luo, W., Veeran, S., Wang, J., Li, S., Li, K., and Liu, S. N. (2020). Dual roles of juvenile hormone signaling during early oogenesis in Drosophila. Insect Sci. 27, 665–674. doi: 10.1111/1744-7917.12698
Madhavan, K. (1973). Morphogenetic effects of juvenile hormone and juvenile hormone mimics on adult development of Drosophila. J. Insect Physiol. 19, 441–453. doi: 10.1016/0022-1910(73)90119-4
Manning, A. (1966). Corpus allatum and sexual receptivity in female Drosophila melanogaster. Nature 211, 1321–1322. doi: 10.1038/2111321b0
Meiselman, M., Lee, S. S., Tran, R. T., Dai, H., Ding, Y., Rivera-Perez, C., et al. (2017). Endocrine network essential for reproductive success in Drosophila melanogaster. Proc. Natl. Acad. Sci. U.S.A. 114, E3849–E3858. doi: 10.1073/pnas.1620760114
Meiselman, M. R., Kingan, T. G., and Adams, M. E. (2018). Stress-induced reproductive arrest in Drosophila occurs through ETH deficiency-mediated suppression of oogenesis and ovulation. BMC Biol. 16:18. doi: 10.1186/s12915-018-0484-9
Minakuchi, C., Zhou, X., and Riddiford, L. M. (2008). Krüppel homolog 1 (Kr-h1) mediates juvenile hormone action during metamorphosis of Drosophila melanogaster. Mech. Dev. 125, 91–105. doi: 10.1016/j.mod.2007.10.002
Miura, K., Oda, M., Makita, S., and Chinzei, Y. (2005). Characterization of the Drosophila Methoprene -tolerant gene product. Juvenile hormone binding and ligand-dependent gene regulation. FEBS J. 272, 1169–1178. doi: 10.1111/j.1742-4658.2005.04552.x
Mizuno, Y., Imura, E., Kurogi, Y., Shimada-Niwa, Y., Kondo, S., Tanimoto, H., et al. (2021). A population of neurons that produce hugin and express the diuretic hormone 44 receptor gene projects to the corpora allata in Drosophila melanogaster. Dev. Growth Differ. 63, 249–261. doi: 10.1111/dgd.12733
Moshitzky, P., and Applebaum, S. W. (1995). Pathway and regulation of JHIII-Bisepoxide biosynthesis in adult Drosophila melanogaster corpus allatum. Arch. Insect Biochem. Physiol. 30, 225–237. doi: 10.1002/arch.940300211
Moshitzky, P., Fleischmann, I., Chaimov, N., Saudan, P., Klauser, S., Kubli, E., et al. (1996). Sex-peptide activates juvenile hormone biosynthesis in the Drosophila melanogaster corpus allatum. Arch. Insect Biochem. Physiol. 32, 363–374.
Niwa, R., Niimi, T., Honda, N., Yoshiyama, M., Itoyama, K., Kataoka, H., et al. (2008). Juvenile hormone acid O-methyltransferase in Drosophila melanogaster. Insect Biochem. Mol. Biol. 38, 714–720. doi: 10.1016/j.ibmb.2008.04.003
Noriega, F. G. (2014). Juvenile Hormone Biosynthesis in Insects: What Is New. What Do We Know, and What Questions Remain? Int. Sch. Res. Notices 2014:967361. doi: 10.1155/2014/967361
Ojani, R., Liu, P., Fu, X., and Zhu, J. (2016). Protein kinase C modulates transcriptional activation by the juvenile hormone receptor methoprene-tolerant. Insect Biochem. Mol. Biol. 70, 44–52. doi: 10.1016/j.ibmb.2015.12.001
Postlethwait, J. H. (1974). Juvenile hormone and the adult development of Drosophila. Biol. Bull. 147, 119–135. doi: 10.2307/1540573
Postlethwait, J. H., and Jones, G. J. (1978). Endocrine control of larval fat body histolysis in normal and mutant Drosophila melanogaster. J. Exp. Zool. 203, 207–214. doi: 10.1002/jez.1402030204
Postlethwait, J. H., and Weiser, K. (1973). Vitellogenesis induced by juvenile hormone in the female sterile mutant apterous-four in Drosophila melanogaster. Nat. New Biol. 244, 284–285. doi: 10.1038/newbio244284a0
Pursley, S., Ashok, M., and Wilson, T. G. (2000). Intracellular localization and tissue specificity of the Methoprene-tolerant (Met) gene product in Drosophila melanogaster. Insect Biochem. Mol. Biol. 30, 839–845. doi: 10.1016/s0965-1748(00)00056-4
Qu, Z., Bendena, W. G., Nong, W., Siggens, K. W., Noriega, F. G., Kai, Z. P., et al. (2017). MicroRNAs regulate the sesquiterpenoid hormonal pathway in Drosophila and other arthropods. Proc. Biol. Sci. 284:20171827. doi: 10.1098/rspb.2017.1827
Qu, Z., Bendena, W. G., Tobe, S. S., and Hui, J. H. L. (2018). Juvenile hormone and sesquiterpenoids in arthropods: Biosynthesis, signaling, and role of MicroRNA. J. Steroid. Biochem. Mol. Biol. 184, 69–76. doi: 10.1016/j.jsbmb.2018.01.013
Richard, D. S., Applebaum, S. W., and Gilbert, L. I. (1989a). Developmental regulation of juvenile hormone biosynthesis by the ring gland of Drosophila melanogaster. J. Comp. Physiol. B. 159, 383–387. doi: 10.1007/BF00692410
Richard, D. S., Applebaum, S. W., Sliter, T. J., Baker, F. C., Schooley, D. A., Reuter, C. C., et al. (1989b). Juvenile hormone bisepoxide biosynthesis in vitro by the ring gland of Drosophila melanogaster: a putative juvenile hormone in the higher Diptera. Proc. Natl. Acad. Sci. U.S.A. 86, 1421–1425. doi: 10.1073/pnas.86.4.1421
Richard, D. S., and Gilbert, L. I. (1991). Reversible juvenile hormone inhibition of ecdysteroid and juvenile hormone synthesis by the ring gland of Drosophila melanogaster. Experientia 47, 1063–1066. doi: 10.1007/BF01923343
Riddiford, L. M. (2012). How does juvenile hormone control insect metamorphosis and reproduction? Gen. Comp. Endocrinol. 179, 477–484. doi: 10.1016/j.ygcen.2012.06.001
Riddiford, L. M. (2020). Rhodnius, Golden Oil, and Met: A History of Juvenile Hormone Research. Front. Cell Dev. Biol. 8:679. doi: 10.3389/fcell.2020.00679
Riddiford, L. M., and Ashburner, M. (1991). Effects of juvenile hormone mimics on larval development and metamorphosis of Drosophila melanogaster. Gen. Comp. Endocrinol. 82, 172–183. doi: 10.1016/0016-6480(91)90181-5
Riddiford, L. M., Truman, J. W., Mirth, C. K., and Shen, Y. C. (2010). A role for juvenile hormone in the prepupal development of Drosophila melanogaster. Development 137, 1117–1126. doi: 10.1242/dev.037218
Riddiford, L. M., Truman, J. W., and Nern, A. (2018). Juvenile hormone reveals mosaic developmental programs in the metamorphosing optic lobe of Drosophila melanogaster. Biol. Open 7:bio034025. doi: 10.1242/bio.034025
Ringo, J., Werczberger, R., Altaratz, M., and Segal, D. (1991). Female sexual receptivity is defective in juvenile hormone-deficient mutants of the apterous gene of Drosophila melanogaster. Behav. Genet. 21, 453–469. doi: 10.1007/BF01066724
Ringo, J., Werczberger, R., and Segal, D. (1992). Male sexual signaling is defective in mutants of the apterous gene of Drosophila melanogaster. Behav. Genet. 22, 469–487. doi: 10.1007/BF01066616
Rivera-Perez, C., Clifton, M. E., Noriega, F. G., and Jindra, M. (2020). “Juvenile Hormone Regulation and Action,” in Advances in Invertebrate (Neuro)Endocrinology, eds S. Saleuddin and A. B. Lange I (Orchard: Apple Academic Press), 1–77. doi: 10.1201/9781003029861-1
Rivera-Perez, C., Nouzova, M., and Noriega, F. G. (2012). A quantitative assay for the juvenile hormones and their precursors using fluorescent tags. PLoS One 7:e43784. doi: 10.1371/journal.pone.0043784
Sánchez-Higueras, C., Sotillos, S., and Castelli-Gair Hombría, J. (2014). Common origin of insect trachea and endocrine organs from a segmentally repeated precursor. Curr. Biol. 24, 76–81. doi: 10.1016/j.cub.2013.11.010
Santos, C. G., Humann, F. C., and Hartfelder, K. (2019). Juvenile hormone signaling in insect oogenesis. Curr. Opin. Insect Sci. 31, 43–48. doi: 10.1016/j.cois.2018.07.010
Saunders, D. S., Richard, D. S., Applebaum, S. W., Ma, M., and Gilbert, L. I. (1990). Photoperiodic diapause in Drosophila melanogaster involves a block to the juvenile hormone regulation of ovarian maturation. Gen. Comp. Endocrinol. 79, 174–184. doi: 10.1016/0016-6480(90)90102-r
Schwenke, R. A., and Lazzaro, B. P. (2017). Juvenile Hormone Suppresses Resistance to Infection in Mated Female Drosophila melanogaster. Curr. Biol. 27, 596–601. doi: 10.1016/j.cub.2017.01.004
Sethi, S., Lin, H.-H., Shepherd, A. K., Volkan, P. C., Su, C.-Y., and Wang, J. W. (2019). Social Context Enhances Hormonal Modulation of Pheromone Detection in Drosophila. Curr. Biol. 29, 3887–3898.e4. doi: 10.1016/j.cub.2019.09.045
Shemshedini, L., Lanoue, M., and Wilson, T. G. (1990). Evidence for a juvenile hormone receptor involved in protein synthesis in Drosophila melanogaster. J. Biol. Chem. 265, 1913–1918. doi: 10.1016/S0021-9258(19)39917-X
Shemshedini, L., and Wilson, T. G. (1990). Resistance to juvenile hormone and an insect growth regulator in Drosophila is associated with an altered cytosolic juvenile hormone-binding protein. Proc. Natl. Acad. Sci. U.S.A. 87, 2072–2076. doi: 10.1073/pnas.87.6.2072
Shinoda, T., and Itoyama, K. (2003). Juvenile hormone acid methyltransferase: a key regulatory enzyme for insect metamorphosis. Proc. Natl. Acad. Sci. U.S.A. 100, 11986–11991. doi: 10.1073/pnas.2134232100
Shtorch, A., Werczberger, R., and Segal, D. (1995). Genetic and molecular studies of apterous: a gene implicated in the juvenile hormone system of Drosophila. Arch. Insect Biochem. Physiol. 30, 195–209. doi: 10.1002/arch.940300209
Siegmund, T., and Korge, G. (2001). Innervation of the ring gland of Drosophila melanogaster. J. Comp. Neurol. 431, 481–491.
Soller, M., Bownes, M., and Kubli, E. (1999). Control of oocyte maturation in sexually mature Drosophila females. Dev. Biol. 208, 337–351. doi: 10.1006/dbio.1999.9210
Tatar, M., Kopelman, A., Epstein, D., Tu, M. P., Yin, C. M., and Garofalo, R. S. (2001). A mutant Drosophila insulin receptor homolog that extends life-span and impairs neuroendocrine function. Science 292, 107–110. doi: 10.1126/science.1057987
Terashima, J., Takaki, K., Sakurai, S., and Bownes, M. (2005). Nutritional status affects 20-hydroxyecdysone concentration and progression of oogenesis in Drosophila melanogaster. J. Endocrinol. 187, 69–79. doi: 10.1677/joe.1.06220
Tompkins, L. (1990). Effects of the apterous4 mutation on Drosophila melanogaster males’ courtship. J. Neurogenet. 6, 221–227. doi: 10.3109/01677069009107112
Tu, M. P., Yin, C. M., and Tatar, M. (2005). Mutations in insulin signaling pathway alter juvenile hormone synthesis in Drosophila melanogaster. Gen. Comp. Endocrinol. 142, 347–356. doi: 10.1016/j.ygcen.2005.02.009
Wang, C., Zhang, J., Tobe, S. S., and Bendena, W. G. (2012). Defining the contribution of select neuropeptides and their receptors in regulating sesquiterpenoid biosynthesis by Drosophila melanogaster ring gland/corpus allatum through RNAi analysis. Gen. Comp. Endocrinol. 176, 347–353. doi: 10.1016/j.ygcen.2011.12.039
Wen, D., Rivera-Perez, C., Abdou, M., Jia, Q., He, Q., Liu, X., et al. (2015). Methyl farnesoate plays a dual role in regulating Drosophila metamorphosis. PLoS Genet. 11::e1006559. doi: 10.1371/journal.pgen.1005038
Wigglesworth, V. B. (1934). The physiology of ecdysis in Rhodnius prolixus (Hemiptera). II. Factors controlling moulting and metamorphosis. Q. J. Microsc. Sci. 77, 193–221.
Wijesekera, T. P., Saurabh, S., and Dauwalder, B. (2016). Juvenile Hormone Is Required in Adult Males for Drosophila Courtship. PLoS One 11:e0151912. doi: 10.1371/journal.pone.0151912
Wilson, T. G., and Ashok, M. (1998). Insecticide resistance resulting from an absence of target-site gene product. Proc. Natl. Acad. Sci. U.S.A. 95, 14040–14044. doi: 10.1073/pnas.95.24.14040
Wilson, T. G., Demoor, S., and Lei, J. (2003). Juvenile hormone involvement in Drosophila melanogaster male reproduction as suggested by the Methoprene-tolerant(27) mutant phenotype. Insect Biochem. Mol. Biol. 33, 1167–1175. doi: 10.1016/j.ibmb.2003.06.007
Wilson, T. G., and Fabian, J. (1986). A Drosophila melanogaster mutant resistant to a chemical analog of juvenile hormone. Dev. Biol. 118, 190–201. doi: 10.1016/0012-1606(86)90087-4
Wolfner, M. F., Partridge, L., Lewin, S., Kalb, J. M., Chapman, T., and Herndon, L. A. (1997). Mating and hormonal triggers regulate accessory gland gene expression in male Drosophila. J. Insect Physiol. 43, 1117–1123. doi: 10.1016/s0022-1910(97)00062-0
Wu, B., He, L., Xiao, Y., Du, J., Wang, X., and Zhao, Z. (2021). Juvenile hormone receptor MET regulates sleep and neuronal morphology via glial-neuronal crosstalk. J. Genet. Genomics 48, 706–715. doi: 10.1016/j.jgg.2021.04.010
Wu, B., Ma, L., Zhang, E., Du, J., Liu, S., Price, J., et al. (2018). Sexual dimorphism of sleep regulated by juvenile hormone signaling in Drosophila. PLoS Genet. 14:e1007318. doi: 10.1371/journal.pgen.1007318
Wu, Z., Yang, L., Li, H., and Zhou, S. (2021). Krüppel-homolog 1 exerts anti-metamorphic and vitellogenic functions in insects via phosphorylation-mediated recruitment of specific cofactors. BMC Biol. 19:222. doi: 10.1186/s12915-021-01157-3
Yamamoto, K., Chadarevian, A., and Pellegrini, M. (1988). Juvenile hormone action mediated in male accessory glands of Drosophila by calcium and kinase C. Science 239, 916–919. doi: 10.1126/science.3124270
Yamamoto, R., Bai, H., Dolezal, A. G., Amdam, G., and Tatar, M. (2013). Juvenile hormone regulation of Drosophila aging. BMC Biol. 11:85. doi: 10.1186/1741-7007-11-85
Yang, Y., Zhao, T., Li, Z., Qian, W., Peng, J., Wei, L., et al. (2021). Histone H3K27 methylation-mediated repression of Hairy regulates insect developmental transition by modulating ecdysone biosynthesis. Proc. Natl. Acad. Sci. U.S.A. 118, e2101442118. doi: 10.1073/pnas.2101442118
Zhang, J., Wen, D., Li, E. Y., Palli, S. R., Li, S., Wang, J., et al. (2021). MicroRNA miR-8 promotes cell growth of corpus allatum and juvenile hormone biosynthesis independent of insulin/IGF signaling in Drosophila melanogaster. Insect Biochem. Mol. Biol. 136:103611. doi: 10.1016/j.ibmb.2021.103611
Zhang, S. X., Glantz, E. H., Miner, L. E., Rogulja, D., and Crickmore, M. A. (2021). Hormonal control of motivational circuitry orchestrates the transition to sexuality in Drosophila. Sci. Adv. 7:eabg6926. doi: 10.1126/sciadv.abg6926
Zhang, T., Song, W., Li, Z., Qian, W., Wei, L., Yang, Y., et al. (2018). Krüppel homolog 1 represses insect ecdysone biosynthesis by directly inhibiting the transcription of steroidogenic enzymes. Proc. Natl. Acad. Sci. U.S.A. 115, 3960–3965. doi: 10.1073/pnas.1800435115
Keywords: juvenile hormone, corpus allatum, methoprene-tolerant, Drosophila melanogaster, metamorphosis, reproduction, behavior
Citation: Zhang X, Li S and Liu S (2022) Juvenile Hormone Studies in Drosophila melanogaster. Front. Physiol. 12:785320. doi: 10.3389/fphys.2021.785320
Received: 29 September 2021; Accepted: 29 October 2021;
Published: 10 February 2022.
Edited by:
Ya-Nan Zhang, Huaibei Normal University, ChinaReviewed by:
Wen Liu, Huazhong Agricultural University, ChinaPengcheng Liu, Nanjing Agricultural University, China
Guan-Heng Zhu, Sun Yat-sen University, China
Copyright © 2022 Zhang, Li and Liu. This is an open-access article distributed under the terms of the Creative Commons Attribution License (CC BY). The use, distribution or reproduction in other forums is permitted, provided the original author(s) and the copyright owner(s) are credited and that the original publication in this journal is cited, in accordance with accepted academic practice. No use, distribution or reproduction is permitted which does not comply with these terms.
*Correspondence: Suning Liu, liusuning@m.scnu.edu.cn