- 1Key Laboratory of Entomology and Pest Control Engineering, College of Plant Protection, Southwest University, Chongqing, China
- 2State Cultivation Base of Crop Stress Biology for Southern Mountainous Land, Southwest University, Chongqing, China
- 3International Joint Laboratory of China-Belgium on Sustainable Crop Pest Control, Academy of Agricultural Sciences, Southwest University, Chongqing, China
Pesticide resistance is a serious problem that poses a major challenge to pest control. One of the most potent resistance mechanisms is the overexpression of genes coding for detoxification enzymes. The expression of detoxification genes is regulated by a series of transcription factors. Previous studies have revealed that the increased expression of detoxification genes contributes to the insecticide tolerance of Bactrocera dorsalis. Our objective was thus to identify the transcription factors involved in this process. Temporal expression profiles showed that the transcription factor MafB and detoxification genes were expressed highly in the fat body. Further analysis showed that the expression of MafB, GSTz2, and CYP473A3 was induced by abamectin. Disruption of the MafB transcription factor through RNA interference decreased the transcript levels of GSTz2 and CYP473A3 and increased the susceptibility to abamectin significantly. Direct silencing of the expression of GSTz2 also increased susceptibility to abamectin, while CYP473A3 did not. In conclusion, these results suggest that the expression of GSTz2 and CYP473A3 was regulated by the transcription factor MafB, and the up-regulation of GSTz2 via MafB decreased the susceptibility of B. dorsalis to abamectin.
Introduction
The oriental fruit fly Bactrocera dorsalis (Hendel) is globally distributed and constitutes one of the most damaging and economically important agricultural pests. In the field control of B. dorsalis, the application of insecticides has led to high levels of insecticide resistance in China as well as Hawaii of United States, which has resulted in severe ecological problems as well as significant economic losses (Hsu et al., 2004; Chou et al., 2010; Jin et al., 2014). Abamectin, a macrocyclic lactones compound, originated from the soil microorganism involving Streptomyces avermitilis, has been commonly used for the control of fruit flies (Jin et al., 2011). It belongs to the family of avermectins (Putter et al., 1981), targeting the glutamate-gated chloride channels (GluCls) and histamine-gated chloride channels (HisCls) (Zheng et al., 2002; McCavera et al., 2007). However, resistance selection analysis of a laboratory-reared strain indicated that B. dorsalis could develop high resistance to abamectin after 36 generations. Furthermore, resistance monitoring showed that field populations of B. dorsalis in China have developed resistance to abamectin (Jin et al., 2011). Therefore, investigating the underlying molecular mechanisms of insecticide resistance is an important prerequisite for the development of resistance management strategies.
Great progress has been made over the last two decades in understanding the molecular mechanisms of insecticide resistance. It has been well reported that decreased target site sensitivity, enhanced metabolic detoxification, and reduced cuticle penetration are the major contributors to resistance (Vontas et al., 2011; Liu, 2015; Vargas et al., 2015). Metabolic resistance refers to the enhanced detoxification and metabolism of insecticides in insects through increasing the expression or activity of detoxification enzymes. Notably, the overexpression of cytochrome P450 monooxygenases (P450s), carboxylesterases (CarEs), and glutathione S-transferases (GSTs) constitutes the most common metabolic resistance mechanism (Wilson, 2001). RNA interference (RNAi) technology can effectively detect the importance of these continuously overexpressed detoxification genes in insect resistance. Interference of the overexpressed genes (CYP6FD2 and CYP6FF1) of Locusta migratoria has led to an increase in deltamethrin toxicity (Guo et al., 2016). In Tetranychus cinnabarinus, P450 genes, such as CYP389B1 and CYP392A26, were overexpressed in a fenpropathrin-resistant strain (Shi et al., 2015). The transcription levels of GSTs (GSTe2, GSTe4, and GSTe9) and CarEs (CarE2, CarE4, and CarE6) in malathion-resistant strains of B. dorsalis were increased, and the silencing of these genes by RNAi could increase the susceptibility to malathion (Wang et al., 2015, 2016; Lu et al., 2016).
To further clarify the regulatory mechanisms of specific genes, the transcription system of xenobiotic detoxification in Drosophila was assessed (Misra et al., 2011). Under stress exposure conditions, Keap1 releases CncC [a basic leucine zipper (bZIP) protein], which translocates to the nucleus, and a heterodimer formed by CncC and sMaf (belonging to the bZIP family) binds upstream of the stress-response genes (XRE) to regulate the expression of antioxidant genes (Nioi et al., 2003; Baird and Dinkova-Kostova, 2011; Misra et al., 2011; Hirotsu et al., 2012). It has been reported that the Cncc–Maf heterodimer is involved in the transcriptional regulation of detoxifying metabolic enzymes. In Drosophila melanogaster, CYP6a2 and CYP6a8 were down-regulated following the knockdown of CncC, which resulted in a decreased DDT tolerance in DDT-resistant strains (Misra et al., 2013). Expression of GSTd1 in D. melanogaster was also reduced after the silencing of CncC and led to the high mortality of paraquat poisoning (Sykiotis and Bohmann, 2008). In Aphis gossypii, CncC regulates Cyp6AD2 to alter gossypol tolerance in a gossypol-resistant strain (Peng et al., 2016).
Oriental fruit flies are polyphagous pests that reduce the yield and quality of fruit, often cause spoilage. The long-term intensive use of pesticides has led to resistance to various insecticides in the species (Brown and Payne, 1988). It was previously indicated that the overexpression of detoxification enzymes constitutes a primary mechanism of resistance in B. dorsalis. In this study, we focused on the function of the transcription factor MafB and its downstream genes, which are involved in the abamectin tolerance of B. dorsalis.
Materials and Methods
Test Insects
Larvae of the flies were originally collected from the infested oranges in Hainan province, China, in 2008. For this study, 60 generations of the flies have been reared. The insects were reared with an artificial diet according to a previously described protocol (Wang et al., 2013). The laboratory strain of the flies was cultured in a growth chamber at 27.5 ± 0.5°C, 75 ± 5% relative humidity, and a photoperiod of 14:10 h (light:dark).
Molecular Cloning
The reference sequence of the MafB cDNA was acquired from NCBI (GenBank accession number, XM_011207424). Primers amplifying the complete open-reading frame (ORF) were designed (Table 1) using Primer Premier 5.0 (Premier Biosoft International, Palo Alto, CA, United States). PrimeSTAR high-fidelity DNA polymerase (Takara, Dalian, China) was used for PCR amplification. The purified PCR product was subcloned into the pGEM-T Easy Vector (Promega, Beijing, China), and the construct was transformed into Trans5α chemically competent cells. Positive clones were sent for sequencing (TransGen Biotech, Beijing, China).
Quantitative Reverse Transcript PCR
RNA was extracted from different tissues, including the Malpighian tubules, fat body, and midgut. TRIzol reagent (Life Technologies, Carlsbad, CA, United States) was used for the RNA isolation with a genome DNA elimination by DNase I (Promega, Madison, WI, United States). The extracted RNA was purified using phenol/chloroform method and dissolved in the RNase-free water. The concentration and the purity were assessed by Nanodrop 1000 (GE Healthcare Bio-sciences, Uppsala, Sweden). The cDNA synthesis was conducted by the PrimeScript 1st Strand cDNA Synthesis Kit (Takara, Dalian, China) according to the manufacturers’ instructions. For each sample, 1 μg of total RNA was used to synthesize cDNA. A serial dilution (fivefold) of the cDNA was employed to determine the amplification efficiency of each primer pair for the target gene. Quantitative Reverse Transcript PCR (RT-qPCR) was performed in 10 μL reaction volumes consisting of 5 μL qPCR Master Mix (Promega, Madison, WI, United States), 0.5 μL each of cDNA and reverse primers (5 μM), and 3.5 μL nuclease-free water. The RT-qPCR reactions were conducted with the following program: initial incubation of 95°C for 2 min, followed by 40 cycles of 95°C for 15 s and 60°C for 30 s. RPS and α-tubulin served as the internal reference genes for normalization of the relative expression levels (Shen et al., 2010). The RT-qPCR was conducted with two technical and four biological replications. The relative expression data were analyzed using Qbase. The expression of MafB and detoxification enzymes in three different tissues of B. dorsalis was log2 transformed. A heat map was generated by HemI. RNAi and abamectin treatment data are presented as the mean ± standard error (SE). All the data were subject to independent t-test by utilizing SPSS 20.0 (SPSS Inc., Chicago, IL, United States).
Phylogenetic Analysis and Identification
MafB protein sequences of dipteran species were obtained from the NCBI web server1 and aligned with the MafB sequences generated in the present study using Clustal (Larkin et al., 2007) and JalView 2.9 (Waterhouse et al., 2009). To infer the evolutionary relationships, the neighbor-joining method was used to construct a phylogenetic tree in MEGA5.05 (Tamura et al., 2011) with 1000 bootstrap replicates. The molecular weight and isoelectric point of MafB were predicted using the online software tool2.
RNAi
A fragment of MafB was amplified by PCR using primers (Table 1) containing the T7 promoter as the template for double-stranded (ds) RNA synthesis. The dsRNA was synthesized using the TranscriptAid T7 High Yield Transcription Kit (Thermo Scientific, Wilmington, DE, United States). About 1.5 μg of dsRNA was injected into the abdomen between the first and second abdominal segments with a Nanoject II Auto-Nanoliter Injector (Drummond Scientific, Broomall, PA, United States). Equivalent injection quantities of the ds green fluorescent protein (dsGFP) served as the control. The RNAi efficiency was determined using randomly collected adults at 24 and 48 h post-injection. At least 60 4-day-old adult flies were used for each treatment and control group. Mortality was less than or equal to 5% after dsRNA injection for each treated and control group.
Abamectin Bioassay
Abamectin (95% purity) was purchased from Bangnong Chemical Company (Guangzhou, China). The bioassay was performed following the knockdown of MafB, CYP437A3, and GSTz2. A 0.5-μL of abamectin [concentration = LC40 (105 μg/mL)] was applied to the pronotum of test insects using a PB-600-1 repeating dispenser (Hamilton Company, Reno, NV, United States). For the dsRNA-injected flies, the bioassay was conducted at 24 h after the injection. At least 60 injected flies were used for the bioassays for each treatment. Mortality of the flies was scored after 24 h of exposure to abamectin. Chi-square (χ2)-test was used to determine the significant difference of the mortality between dsRNA treated and control group by utilizing SPSS 20.0 (SPSS Inc., Chicago, IL, United States). Data are presented as the mean ± SE.
Results
MafB Sequence Analysis
The ORF of MafB was screened out from the transcriptome data of B. dorsalis and confirmed by PCR. The sequence of MafB is accessible in GenBank (XM_011207424). The complete ORF of MafB contains 1323 nucleotides and encodes 440 amino acids. The predicted molecular weight of the MafB protein is 48.71 kD, and the isoelectric point is 6.97. The phylogenetic tree of dipteran MafB protein sequences shows that the MafB proteins are relatively conserved and that the MafB of B. dorsalis is close to the MafB of B. latifrons and B. oleae from Tephritidae (Figure 1). The MafB protein includes one conserved domain (Basic Leucine zipper domain). There is a dimerization domain and a DNA-binding site (DBD) (Figure 1). Thirteen residues consist of DNA-binding sites and 19 are composed of a dimer interface that can form a dimer and bind upstream of the target gene to initiate transcription.
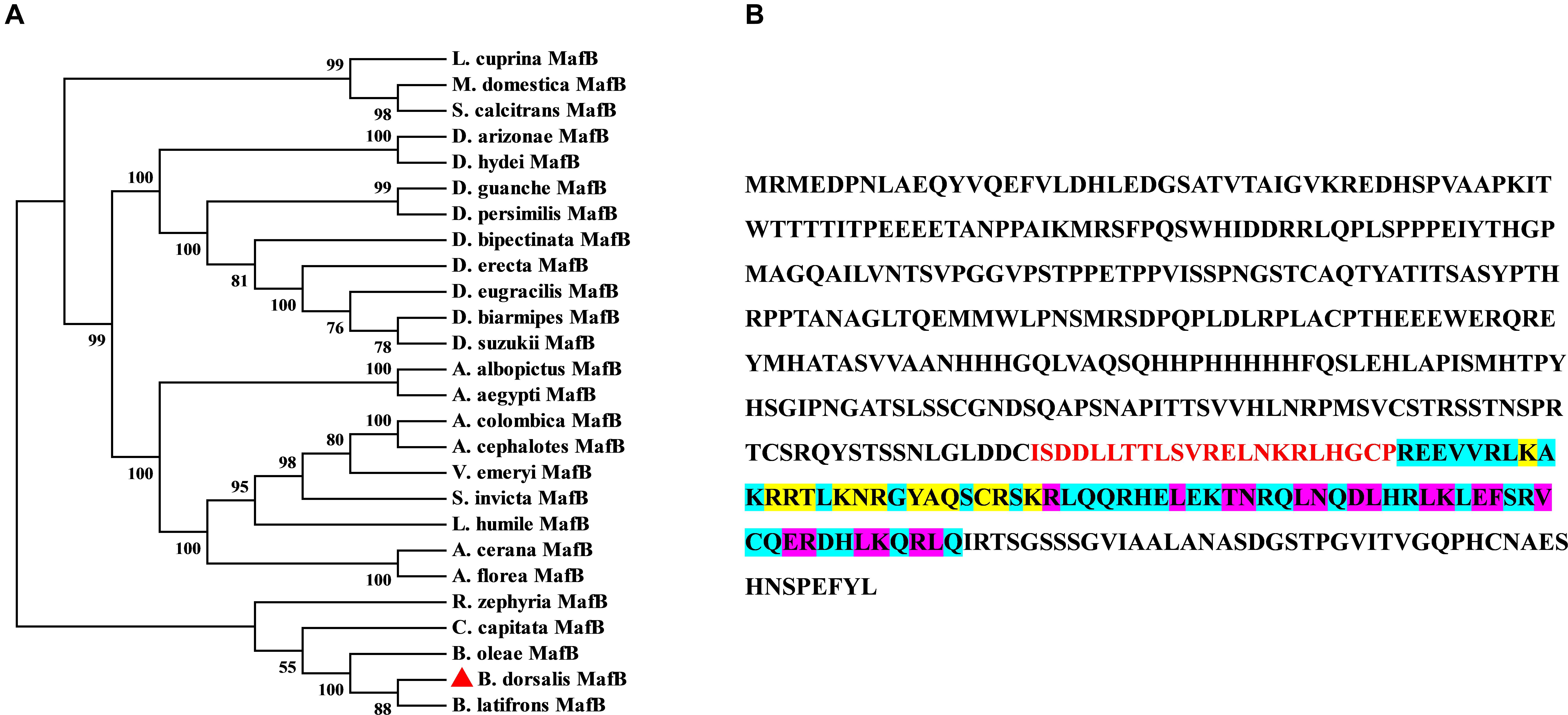
Figure 1. Sequence and phylogenetic tree of MafB. (A) Phylogenetic tree of MafBs. Amino acid sequences of B. dorsalis MafB and dipteran MafB were aligned to forming phylogenetic tree to assess their relationships. MafB of B. dorsalis was indicated by the red rectangle. (B) The amino acid sequence structure of MafB. The basic leucine and zipper (bZIP) domain are indicated by blue background and red color. DNA-binding site and dimer interface domain are indicated by yellow and purple core color, respectively.
Tissue-Specific Expression Profiling
The expression patterns of the transcription factor (MafB) as well as the detoxification enzymes in the three major tissues (midgut, fat body, and Malpighian tubules) associated with insecticide metabolism were analyzed by RT-qPCR (Figure 2). The highest expression of MafB was detected in the fat body, followed by the midgut and then the Malpighian tubules. Based on this characteristic, GSTe6, GSTz2, CYP437A3, CYP4AC4, and aE6, which were highly expressed in the fat body, were screened out. These filtered genes were then used in the following experiments.
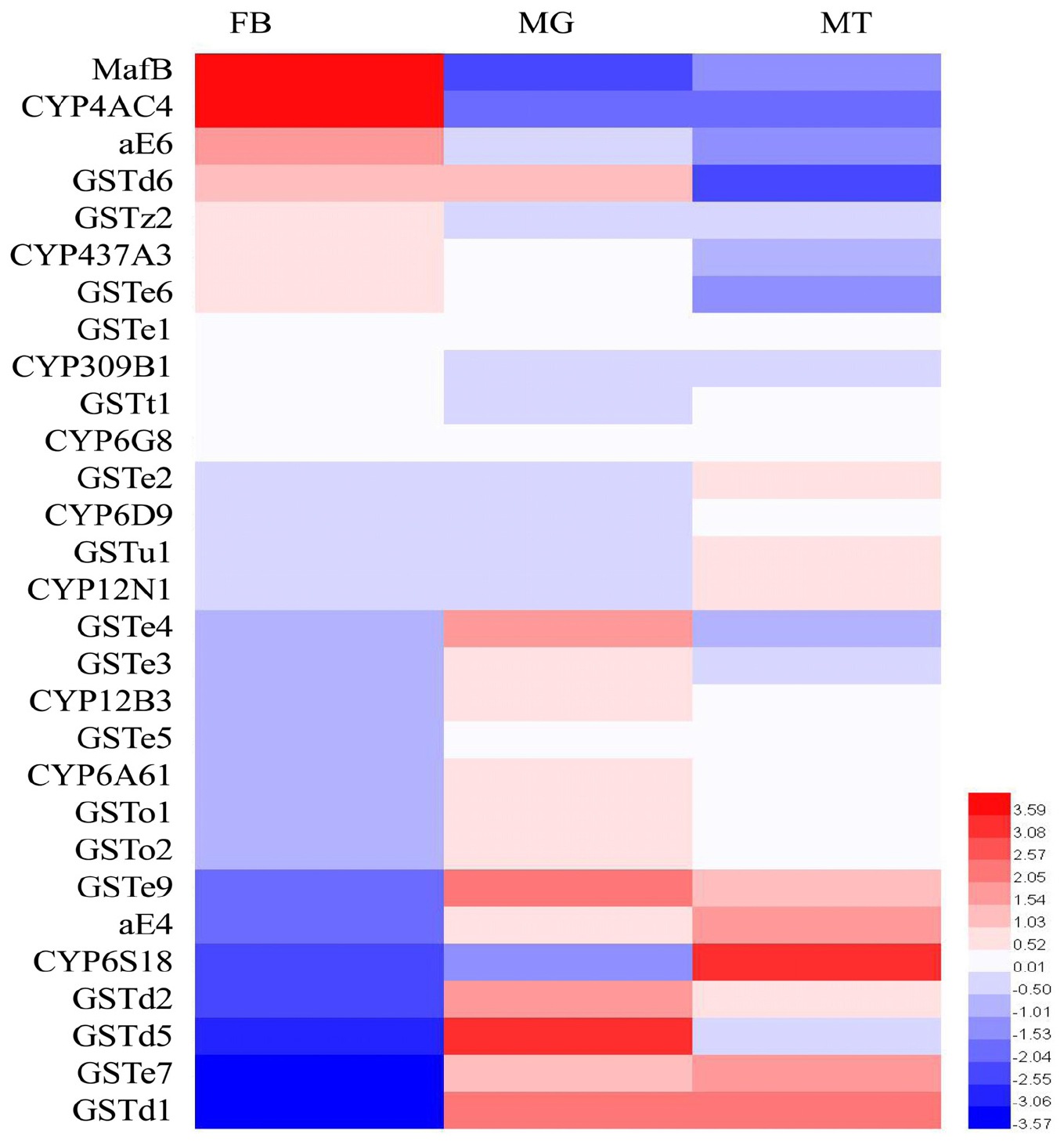
Figure 2. The transcriptional expression profiles of MafB and detoxification enzymes in three different tissues of B. dorsalis. MT, Malpighian tubules; MG, midgut; FB, fat body. The expression of MafB and detoxification enzymes of B. dorsalis was log2 transformed. Different colors represent the degree of expression, red and blue indicate upregulation and downregulation, respectively.
Abamectin-Induced Expression
The relative expressions of MafB and the filtered detoxifying genes in the 4-day-old adult flies under abamectin treatment at three different time points (12, 24, and 36 h) were analyzed by RT-qPCR (Figure 3). The relative expression of MafB in the fat body after treatment was significantly higher than in the control at 12 h (P < 0.05), indicating the rapid response of MafB to abamectin stimulation. The relative expressions of CYP437A3 and GSTz2 in the fat body after 36 h of treatment were significantly increased (P < 0.05). However, the expression of CYP4AC4, aE6, and GSTe6 did not change significantly at any time point following abamectin treatment. CYP437A3 and GSTz2 were thus selected for the subsequent interference experiments.
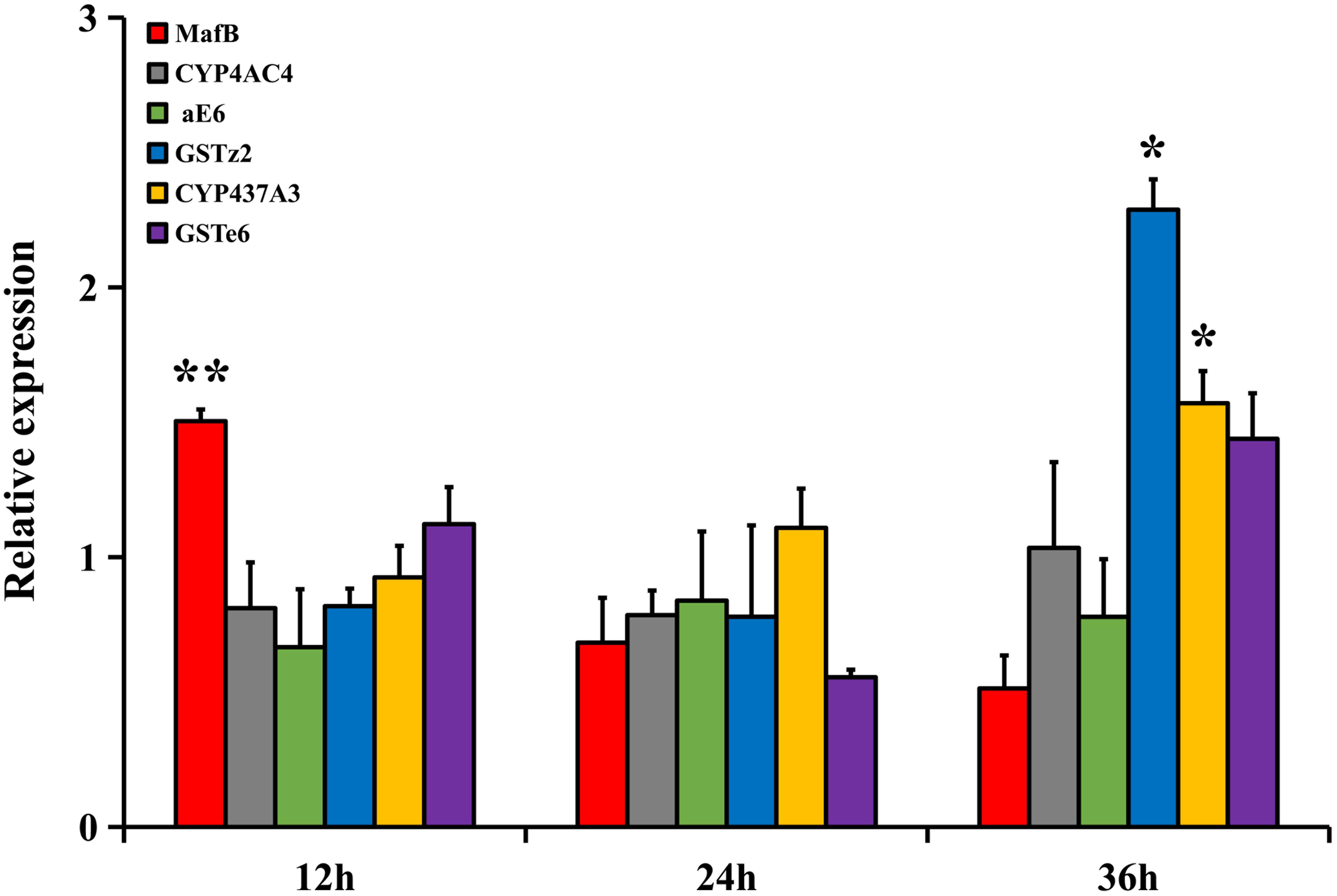
Figure 3. The transcriptional expression profiles of MafB and GSTe6, GSTz2, CYP4AC4, CYP437A3, and aE6 of B. dorsalis in the fat body by abamectin treatment. The data shown are mean ± SE (n = 3). Relative expression was calculated based on the value of acetone. Asterisks (∗ and ∗∗) above the error bars present statistical differences determined by the independent samples t-test with P < 0.05 and P < 0.01, respectively.
RNAi of MafB and Abamectin Susceptibility Test
To examine whether MafB controls the expression of detoxifying genes (including CYP437A3 and GSTz2) and affects the susceptibility of abamectin, RNAi of MafB was conducted. The suppression efficiency of MafB compared to the GFP-injected control was quantified by RT-qPCR (Figure 4A). The transcriptional level of MafB was significantly reduced (88.4% suppressed) than in the control after 24 h (P < 0.05). RNAi of MafB also led to the reduced expression of two detoxification genes (CYP437A3 and GSTz2). The abamectin bioassays showed that RNAi of MafB presented a significantly higher mortality rate (Figure 4B). Under abamectin exposure, the mortalities at 24 h were 37 and 51% in the flies injected with dsGFP and dsMafB, respectively.
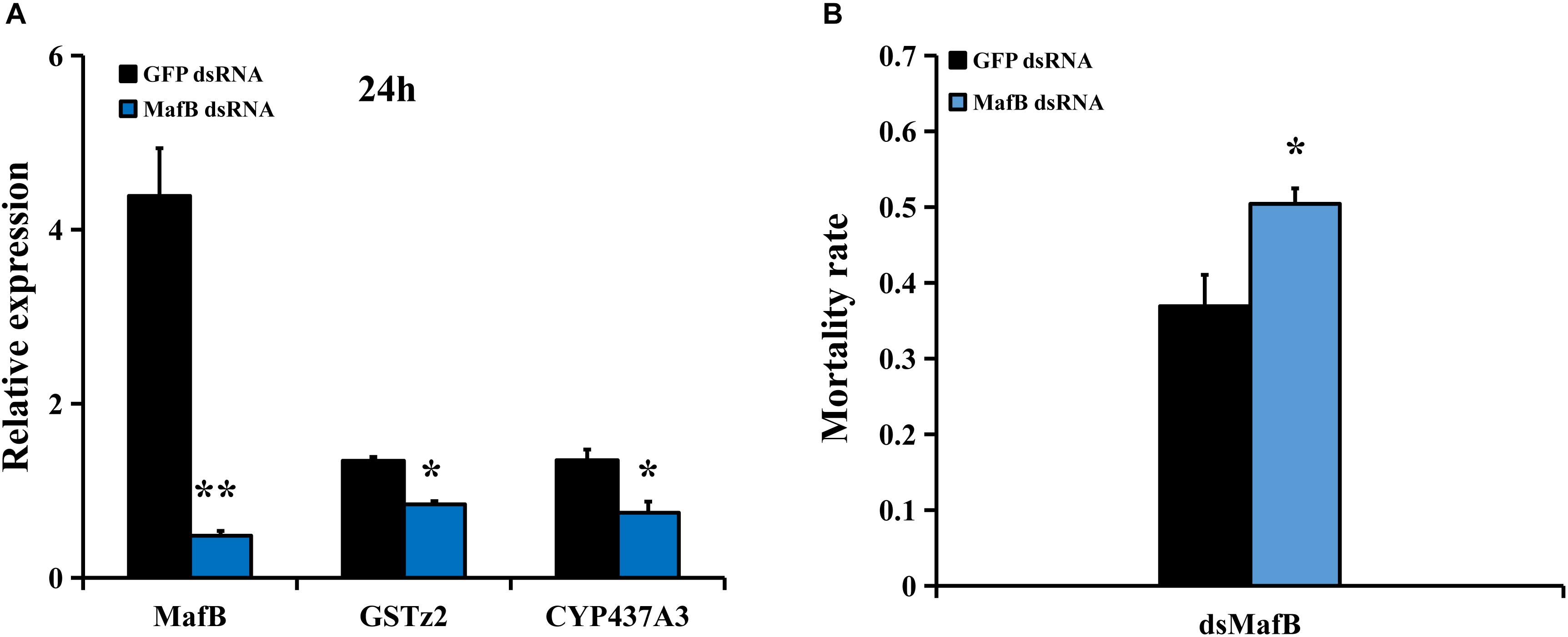
Figure 4. Susceptibility of B. dorsalis to abamectin after MafB knockdown by RNAi. (A) Relative expression levels of MafB after B. dorsalis adults were injected with dsMafB compared to dsGFP, a double-stranded green fluorescent protein RNA. (B) Mortality rate for the dsMafB- and dsGFP-injected flies after abamectin treatment. Asterisks (∗ and ∗∗) above the error bars present statistical differences determined by the independent samples t-test with P < 0.05 and P < 0.01, respectively.
RNAi of GSTz2 and CYP437A3 and Abamectin Susceptibility Test
RNA interference of CYP437A3 and GSTz2 was conducted in B. dorsalis using the same method described above. The mRNA levels of CYP437A3 and GSTz2 were significantly reduced after 24 h (P < 0.05) (Figure 5A). The abamectin bioassay suggested that the RNAi of GSTz2 was associated with a higher mortality rate (Figure 5B). However, the RNAi of CYP437A3 had no effect on mortality. Under exposure to abamectin, the mortalities at 24 h were 47 and 61% in the flies injected with dsGFP and dsGSTz2, respectively.
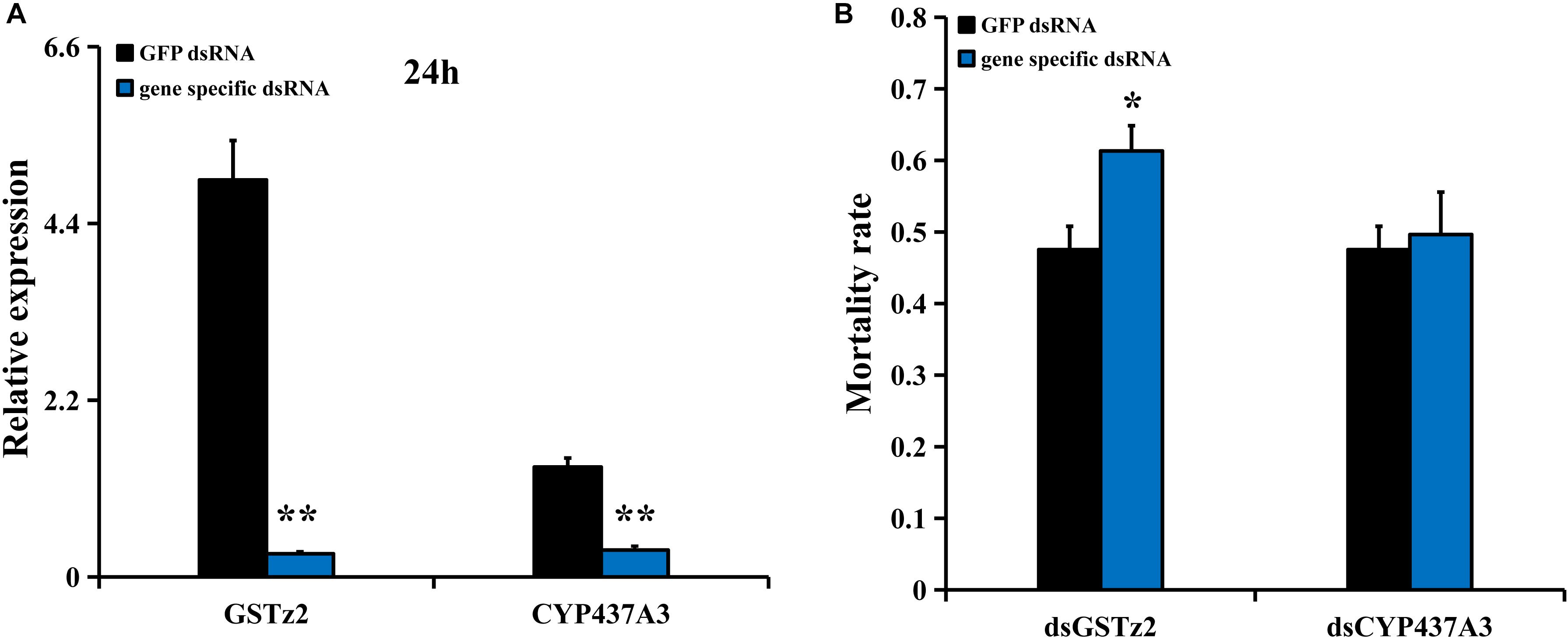
Figure 5. Susceptibility of B. dorsalis to abamectin after GSTz2 and CYP437A3 knockdown by RNAi. (A) Relative expression levels of GSTz2 and CYP437A3 after B. dorsalis adults were injected with dsGSTz2 and dsCYP437A3, respectively, compared to dsGFP, a double-stranded green fluorescent protein RNA. (B) Mortality rate for the dsGSTz2 and dsCYP437A3 and dsGFP-injected flies after abamectin treatment. Asterisks (∗ and ∗∗) above the error bars present statistical differences determined by the independent samples t-test with P < 0.05 and P < 0.01, respectively.
Discussion
The principal objective of this study was to confirm whether MafB functions in the regulation of detoxification genes in B. dorsalis. Although small Maf works as a ligand protein of CncC, it plays an indispensable role in the heterodimer that regulates the detoxification of metabolic enzymes. Without Maf, the regulatory pathway of CncC is invalid. When CncC and Maf were both expressed in Tribolium castaneum, luciferase activity was higher than under the expression of CncC alone in the regulation of CYP6BQ12, CYP6BQ6, CYP6BQ7, and CYP6BQ9 (Kalsi and Palli, 2015). In T. cinnabarinus, luciferase activity was also higher in the presence of both CncC and Maf than in the presence of CncC alone in the regulation of CYP389B1 and CYP392A28 (Shi et al., 2017). In addition, the bZIP nuclear transcription factor family is composed of small Maf (MafG, MafK, and MafF) and large Maf (c-Maf and MafB) proteins that repress as well as activate the transcription of many genes. Notably, large Maf (MafB) and CncC proteins belong to the bZIP family. Large Maf (MafB) proteins are similar to CncC proteins and possess a transcription activity region, binding to antioxidant response elements (AREs) to activate or repress ARE-mediated transcription (Katsuoka and Yamamoto, 2016). It has been documented that large Maf proteins regulate the gene expression of ARE-mediated detoxifying enzymes (Saravanakumar and Jaiswal, 2002). The functional analysis of MafB is thus particularly important in oriental fruit fly.
In order to explore the functions of MafB, the expression of MafB was analyzed. Compared with the midgut and Malpighian tubules, the MafB gene was highly expressed in the fat body and exhibited tissue specificity. The fat body is one of the most important tissues for detoxification metabolism. Numerous P450s express in the fat body, midgut, and Malpighian tubules. Signaling of the CYP4p2 and CYP4s3 genes has been exclusively detected in the fat body via a hybridization technique (Chung et al., 2009). The fat body plays a significant role in metabolic functioning, including in the storage and delivery of energy to satisfy energy demands in insects (Arrese and Soulages, 2010). The high expression in the fat body implies that MafB was involved in detoxification metabolism. Based on the transcripts, CYP4AC4, CYP437A3, aE6, GSTe6, and GSTz2 were highly expressed in the fat body, which suggests a regulatory relationship between MafB and these genes.
Abamectin treatment experiments were then conducted to further explore these regulatory relationships. MafB was significantly expressed at 12 h in the fat body following abamectin treatment, and the downstream target genes (GSTz2 and CYP437A3) were elevated at 36 h, which indicates that MafB responds to abamectin more rapidly and that MafB is likely to regulate downstream genes. However, the mRNA levels of GSTe6, aE6, and CYP4AC4 were not significantly increased at any time following abamectin treatment. RNAi experiments were performed to further evaluate the relationship between MafB and downstream genes (GSTz2 and CYP437A3).
RNA interference was used to disrupt the expression of MafB. MafB knockdown resulted in increased abamectin susceptibility and the down-regulation of CYP437A3 and GSTz2. In a previous experiment, the transcription levels of detoxification genes were suppressed by Maf disruption, including CYP6M2, GSTD1, GSTD3, jheh1, jheh2, and Gnmt, leading to increased susceptibility to DDT, permethrin, and deltamethrin in Anopheles gambiae (Ingham et al., 2017). Furthermore, Maf-S transcription factors were found to control the expression of P450 genes associated with deltamethrin resistance in T. castaneum (Kalsi and Palli, 2015), imidacloprid resistance in Leptinotarsa decemlineata (Kalsi and Palli, 2017), and fenpropathrin resistance in T. cinnabarinus (Shi et al., 2017). The studies indicated that the silencing of Maf results in the down-regulated expression of detoxifying enzyme genes, which further influences metabolism. In this paper, we found that the mRNA levels of GSTz2 and CYP437A3 were also significantly decreased following MafB knockdown. We also demonstrated that the silencing of GSTz2 results in increased susceptibility to abamectin. GSTs are an important group of detoxification enzymes and can be divided into six classes, including epsilon, delta, zeta, theta, omega, and sigma (Ding et al., 2003). GST activity was increased in the fat body and hemolymph under exposure to abamectin in Colorado potato beetle (Tomilova et al., 2016). In pollen beetle Meligethes aeneus, cytochromes P450 and GST made a contribution to deltamethrin exposure (Erban et al., 2017). However, silencing of CYP437A3 did not alter the susceptibility of B. dorsalis to abamectin in the current study, indicating that it is a downstream gene of MafB and may not contribute decisively to abamectin susceptibility.
Conclusion
In conclusion, the results indicated that the expression of GSTz2 was regulated by the transcription factor MafB, which resulted in the susceptibility change of B. dorsalis to abamectin. This study improves our understanding of the regulatory relationship between MafB and detoxification enzymes and also confirms that GSTz2 is an important downstream gene that is involved in abamectin tolerance.
Data Availability
All datasets for this study are included in the manuscript and/or the supplementary files.
Author Contributions
H-BJ and J-JW designed the research and provided the materials and reagents. G-HT, YX, YL, and YY performed all of the experiments. Z-HS and G-HT reared the test insects and collected the data in bioassay. G-HT, YX, G-MS, and H-BJ analyzed the data. G-HT wrote the original draft. H-BJ, J-JW and G-MS edited and modified the manuscript.
Funding
This work was financially supported by the National Natural Science Foundation of China (31572016 and 31772233), the Fok Ying Tung Education Foundation (161029), the Chongqing Young Talents Support Program, the Chongqing Excellent Talents Support Program in University, the Fundamental Research Funds for the Central Universities (XDJK2017A011), and the earmarked fund for Modern Agro-industry (Citrus) Technology Research System (CARS-27) of China.
Conflict of Interest Statement
The authors declare that the research was conducted in the absence of any commercial or financial relationships that could be construed as a potential conflict of interest.
Acknowledgments
We thank LetPub (http://www.letpub.com) for its linguistic assistance during the preparation of this manuscript.
Footnotes
References
Arrese, E. L., and Soulages, J. L. (2010). Insect fat body: energy, metabolism, and regulation. Annu. Rev. Entomol. 55, 207–225. doi: 10.1146/annurev-ento-112408-085356
Baird, L., and Dinkova-Kostova, A. T. (2011). The cytoprotective role of the keap1–Nrf2 pathway. Arch. Toxicol. 85, 241–272. doi: 10.1007/s00204-011-0674-5
Brown, T. M., and Payne, G. T. (1988). Experimental selection for insecticide resistance. J. Econom. Entomol. 81, 49–56. doi: 10.1093/jee/81.1.49
Chou, M. Y., Haymer, D. S., Feng, H. T., Mau, R. F. L., and Hsu, J. C. (2010). Potential for insecticide resistance in populations of Bactrocera dorsalis in hawaii: spinosad susceptibility and molecular characterization of a gene associated with organophosphate resistance. Entomologia Exp. Et Applicata. 134, 296–303. doi: 10.1111/j.1570-7458.2009.00962.x
Chung, H., Sztal, T., Pasricha, S., Sridhar, M., Batterham, P., and Daborn, P. J. (2009). Characterization of Drosophila Melanogaster cytochrome P450 genes. Proc. Nat. Acad. Sci. U. S. A. 106, 5731–5736. doi: 10.1073/pnas.0812141106
Ding, Y., Ortelli, F., Rossiter, L. C., Hemingway, J., and Ranson, H. (2003). The Anopheles gambiae glutathione transferase supergene family: annotation, phylogeny and expression profiles. BMC Genom. 4:35. doi: 10.1186/1471-2164-4-35
Erban, T., Harant, K., Chalupnikova, J., Kocourek, F., and Stara, J. (2017). Beyond the survival and death of the deltamethrin-threatened pollen beetle Meligethes aeneus: an in-depth proteomic study employing a transcriptome database. J. Proteom. 150, 281–289. doi: 10.1016/j.jprot.2016.09.016
Guo, Y., Wu, H., Zhang, X., Ma, E., Guo, Y., Zhu, K. Y., et al. (2016). RNA interference of cytochrome P450 CYP6F subfamily genes affects susceptibility to different insecticides in Locusta migratoria. Pest Manag. Sci. 72, 2154–2165. doi: 10.1002/ps.4248
Hirotsu, Y., Katsuoka, F., Funayama, R., Nagashima, T., Nishida, Y., Nakayama, K., et al. (2012). Nrf2-MafG heterodimers contribute globally to antioxidant and metabolic networks. Nucleic Acids Res. 40, 10228–10239. doi: 10.1093/nar/gks827
Hsu, J. C., Feng, H. T., and Wu, W. J. (2004). Resistance and synergistic effects of insecticides in Bactrocera dorsalis (Diptera: Tephritidae) in taiwan. J. Econom. Entomol. 97, 1682–1688. doi: 10.1603/0022-0493-97.5.1682
Ingham, V. A., Pignatelli, P., Moore, J. D., Wagstaff, S., and Ranson, H. (2017). The transcription factor Maf-S regulates metabolic resistance to insecticides in the malaria vector Anopheles gambiae. BMC Genom. 18:669. doi: 10.1186/s12864-017-4086-7
Jin, T., Liang, G. W., Zeng, L., and Lu, Y. Y. (2014). Detoxification enzymes activities in different Bactrocera dorsalis (Hendel) populations and their relationship with the resistant levels. J. Environ. Entomol. 36, 58–67.
Jin, T., Zeng, L., Lin, Y., Lu, Y., and Liang, G. (2011). Insecticide resistance of the oriental fruit fly, Bactrocera dorsalis (Hendel) (Diptera: Tephritidae), in mainland China. Pest Manag. Sci. 67, 370–376. doi: 10.1002/ps.2076
Kalsi, M., and Palli, S. R. (2015). Transcription factors, CncC and Maf, regulate expression of CYP6BQ genes responsible for deltamethrin resistance in Tribolium castaneum. Insect Biochem. Mole. Biol. 65, 47–56. doi: 10.1016/j.ibmb.2015.08.002
Kalsi, M., and Palli, S. R. (2017). Transcription factor cap n collar C regulates multiple cytochrome P450 genes conferring adaptation to potato plant allelochemicals and resistance to imidacloprid in Leptinotarsa decemlineata (Say). Insect Biochem. Mole. Biol. 83, 1–12. doi: 10.1016/j.ibmb.2017.02.002
Katsuoka, F., and Yamamoto, M. (2016). Small Maf proteins (MafF, MafG, MafK): history, structure and function. Gene 586, 197–205. doi: 10.1016/j.gene.2016.03.058
Larkin, M. A., Blackshields, G., Brown, N. P., Chenna, R., McGettigan, P. A., McWilliam, H., et al. (2007). Clustal W and clustal X version 2.0. Bioinformatics 23, 2947–2948. doi: 10.1093/bioinformatics/btm404
Liu, N. (2015). Insecticide resistance in mosquitoes: impact, mechanisms, and research directions. Annu. Rev. Entomol. 60:537. doi: 10.1146/annurev-ento-010814-020828
Lu, X. P., Wang, L. L., Huang, Y., Dou, W., Chen, C. T., and Wei, D. (2016). The epsilon glutathione s-transferases contribute to the malathion resistance in the oriental fruit fly, bactrocera dorsalis (hendel). Comp. Biochem. Physiol. C Toxicol. Pharmacol. 180, 40–48. doi: 10.1016/j.cbpc.2015.11.001
McCavera, S., Walsh, T. K., and Wolstenholme, A. J. (2007). Nematode ligand-gated chloride channels: an appraisal of their involvement in macrocyclic lactone resistance and prospects for developing molecular markers. Parasitology 134, 1111–1121. doi: 10.1017/s0031182007000042
Misra, J. R., Geanette, L., and Thummel, C. S. (2013). Constitutive activation of the Nrf2/Keap1 pathway in insecticide-resistant strains of Drosophila. Insect Biochem. Mole. Biol. 43, 1116–1124. doi: 10.1016/j.ibmb.2013.09.005
Misra, J. R., Horner, M. A., Geanette, L., and Thummel, C. S. (2011). Transcriptional regulation of xenobiotic detoxification in Drosophila. Genes Dev. 25, 1796–1806. doi: 10.1101/gad.17280911
Nioi, P., McMahon, M., Itoh, K., Yamamoto, M., and Hayes, J. D. (2003). Identification of a novel Nrf2-regulated antioxidant response element (ARE) in the mouse NAD(P) H: quinone oxidoreductase 1 gene: reassessment of the ARE consensus sequence. Biochem. J. 374, 337–348. doi: 10.1042/bj20030754
Peng, T., Pan, Y., Gao, X., Xi, J., Zhang, L., Yang, C., et al. (2016). Cytochrome P450 CYP6DA2 regulated by cap ‘n’collar isoform C (CncC) is associated with gossypol tolerance in Aphis gossypii Glover. Insect Mole. Biol. 25, 450–459. doi: 10.1111/imb.12230
Putter, I., Connell, J. G. M., Preiser, F. A., Haidri, A. A., Ristich, S. S., and Dybas, R. A. (1981). Avermectins: novelinsecticides, acaricides, and nematicides from soil microorganism. Cell. Mole. Life Sci. 37, 963–964. doi: 10.1007/bf01971780
Saravanakumar, D., and Jaiswal, A. K. (2002). c-Maf negatively regulates ARE-mediated detoxifying enzyme genes expression and anti-oxidant induction. Oncogene 21, 5301–5312. doi: 10.1038/sj.onc.1205642
Shen, G. M., Jiang, H. B., Wang, X. N., and Wang, J. J. (2010). Evaluation of endogenous references for gene expression profiling in different tissues of the oriental fruit fly Bactrocera dorsalis (Diptera: Tephritidae). BMC Mole. Biol. 11:76. doi: 10.1186/1471-2199-11-76
Shi, L., Wang, M., Zhang, Y., Shen, G., Di, H., Wang, Y., et al. (2017). The expression of P450 genes mediating fenpropathrin resistance is regulated by CncC and Maf in Tetranychus cinnabarinus (Boisduval). Comp. Biochem. Physiol. C Toxicol. Pharmacol. 198, 28–36. doi: 10.1016/j.cbpc.2017.05.002
Shi, L., Xu, Z. F., Shen, G. M., Song, C. G., Wang, Y., Peng, J. F., et al. (2015). Expression characteristics of two novel cytochrome P450 genes involved in fenpropathrin resistance in Tetranychus cinnabarinus (Boisduval). Pesticide Biochemistry and Physiology. 119, 33–41. doi: 10.1016/j.pestbp.2015.02.009
Sykiotis, G. P., and Bohmann, D. (2008). Keap1/Nrf2 Signaling regulates oxidative stress tolerance and lifespan in Drosophila. Dev. Cell. 14, 76–85. doi: 10.1016/j.devcel.2007.12.002
Tamura, K., Peterson, D., Peterson, N., Stecher, G., Nei, M., and Kumar, S. (2011). MEGA5: molecular evolutionary genetics analysis using maximum likelihood, evolutionary distance, and maximum parsimony methods. Mole. Biol. Evol. 28, 2731–2739. doi: 10.1093/molbev/msr121
Tomilova, O. G., Kryukov, V. Y., Duisembekov, B. A., Yaroslavtseva, O. N., Tyurin, M. V., Kryukova, N. A., et al. (2016). Immune-physiological aspects of synergy between avermectins and the entomopathogenic fungus metarhizium robertsii in colorado potato beetle larvae. J. Invertebrate Pathol. 140, 8–15. doi: 10.1016/j.jip.2016.08.008
Vargas, R. I., Piñero, J. C., and Leblanc, L. (2015). An overview of pest species of Bactrocera Fruit Flies (Diptera: Tephritidae) and the integration of biopesticides with other biological approaches for their management with a focus on the pacific region. Insects 6, 297–318. doi: 10.3390/insects6020297
Vontas, J., Hernandez-Crespo, P., Margaritopoulos, J. T., Ortego, F., Feng, H. T., Mathiopoulos, K. D., et al. (2011). Insecticide resistance in tephritid flies. Pestic. Biochem. Physiol. 100, 199–205. doi: 10.1016/j.pestbp.2011.04.004
Wang, J. J., Wei, D., Dou, W., Hu, F., Liu, W. F., and Wang, J. J. (2013). Toxicities and synergistic effects of several insecticides against the oriental fruit fly (Diptera: Tephritidae). J. Econom. Entomol. 106, 970–978. doi: 10.1603/ec12434
Wang, L. L., Huang, Y., Lu, X. P., Jiang, X. Z., Smagghe, G., Feng, Z. J., et al. (2015). Overexpression of two-esterase genes mediates metabolic resistance to malathion in the oriental fruit fly, Bactrocera dorsalis (Hendel). Insect Mole. Biol. 24, 467–479. doi: 10.1111/imb.12173
Wang, L. L., Lu, X. P., Meng, L. W., Huang, Y., Wei, D., Jiang, H. B., et al. (2016). Functional characterization of an α-esterase gene involving malathion detoxification in Bactrocera dorsalis (Hendel). Pestic. Biochem. Physiol. 130, 44–51. doi: 10.1016/j.pestbp.2015.12.001
Waterhouse, A. M., Procter, J. B., Martin, D. M. A., Clamp, M., and Barton, G. J. (2009). Jalview Version 2-a multiple sequence alignment editor and analysis workbench. Bioinformatics 25, 1189–1191. doi: 10.1093/bioinformatics/btp033
Keywords: oriental fruit fly, transcription factor, MafB, GST, abamectin
Citation: Tang G-H, Xiong Y, Liu Y, Song Z-H, Yang Y, Shen G-M, Wang J-J and Jiang H-B (2019) The Transcription Factor MafB Regulates the Susceptibility of Bactrocera dorsalis to Abamectin via GSTz2. Front. Physiol. 10:1068. doi: 10.3389/fphys.2019.01068
Received: 01 May 2019; Accepted: 05 August 2019;
Published: 20 August 2019.
Edited by:
Bin Tang, Hangzhou Normal University, ChinaReviewed by:
Alvin Kah-Wei Hee, Universiti Putra Malaysia, MalaysiaTomas Erban, Crop Research Institute (CRI), Czechia
Zhaorigetu Hubhachen, Oklahoma State University, United States
Copyright © 2019 Tang, Xiong, Liu, Song, Yang, Shen, Wang and Jiang. This is an open-access article distributed under the terms of the Creative Commons Attribution License (CC BY). The use, distribution or reproduction in other forums is permitted, provided the original author(s) and the copyright owner(s) are credited and that the original publication in this journal is cited, in accordance with accepted academic practice. No use, distribution or reproduction is permitted which does not comply with these terms.
*Correspondence: Hong-Bo Jiang, amhiODM0MkBzd3UuZWR1LmNu