- 1Photobiological Dermatology Laboratory, Medical Research Center, Department of Dermatology and Medicine, Faculty of Medicine, University of Málaga, Málaga, Spain
- 2Departament of Medicine and Medical Specialities, University of Alcalá, Alcalá de Henares, Spain
Background and Objective: We are exposed to solar radiation from early childhood, so learning different photoprotection strategies is fundamental. As a primary prevention tool, knowledge of the solar ultraviolet radiation to which we are exposed is fundamental. There are devices based on the color change of photochromic substances when exposed to UV radiation which are widely used as a tool for children’s play. The aim was to assess both quantitatively and qualitatively the effectiveness of the use of these devices under different conditions of simulated sun exposure.
Materials and Methods: Ten commercial UV-indicating photochromic devices plus three pure photochromic substances were used. Photochromic devices belong to the spirooxazine family of molecules. They were exposed to different rates of UV radiation under a solar UV-visible simulator, and their velocity, degree of response, and color reversal time were analyzed both visually and objectively using a colorimeter based on three-dimensional CIE L*a*b* color space values. Activation response was also evaluated under different UV high-energy visible light wavelengths using different cut-off filters. Finally, the devices were exposed under high UV irradiance (corresponding to UVI of 10) and interposing sunscreens of different sun protection factors in order to evaluate their potentials as indicators for sunscreen re-application.
Results: All the devices and pure substances offered color change almost immediately under simulated solar radiation. From very low irradiances (UVI >3), they saturated color, and it was not possible to observe and measure color change. The time to color reversal took longer than activation (from 60 s to obtain initial values). When devices were exposed to a UVI of 10 with topical sunscreen overlay, color was observed after 1 min irradiation even under SPF of 50+ sunscreen. Photochromic devices were activated under both UVA and high energy visible light.
Conclusion: UV radiation indicators using photochromic substances which, although possible awareness-raising elements, are of limited use as indicators of UV radiation intensity and dosimetry. They are useful elements for detecting the presence/absence of UV radiation and do not distinguish between different UV radiation spectral bands. Moreover, their incorporation as photoprotective elements used by minors could lead to exposures that are not objectively controlled.
Introduction
According to data from the Spanish State Meteorological Agency, there are on average around 3,000 h of potential sun exposure per year in Spain and other southern European countries (Sánchez-Laulhé Ollero, 2021). This, together with social and cultural aspects, contributes to a high lifetime sun exposure (Aguilera, 2019). The lack of awareness of the risks of so much sun exposure is one of the most important health problems today (Gilaberte and González, 2010).
Both radiometers and spectroradiometers are used to determine the solar radiation to which we are daily exposed; these offer measurements such as the UV index (UVI), a measure established by the WHO to determine the levels of solar radiation with erythematic potential to which we may be exposed (Pérez Ferriols et al., 2014; ISO/CIE 17166, 2019).
At a social level, knowledge of the current UVI, as a tool for controlling this overexposure, can be discovered on the internet or by various smartphone applications (Turner et al., 2020). Easier tools developed to raise awareness about our exposure to the sun include UV indicator strips, based on photochromic substances, or substances that change color when exposed to UV radiation and reverse in the dark (Wang et al., 2018; Saric-Bosanac et al., 2019). Such UV detectors are based on photochromic chemical compounds which can absorb ultraviolet radiation between 280 and 400 nm and change their absorbance in the visible region of the spectrum—mainly red, violet, and blue. Molecules that exhibit photochromic properties, including azobenzene, spiropyran, spirooxazine, naftopyranes, diarylethene, or fulgide structures are the most widespread (Coelho, 2006; Tian and Zhang, 2016; Han et al., 2022; Kozlenko et al., 2023). These substances were introduced 1976 using polysulphone bands for dosimetry studies in medicine, sports, and education and for use in skin stickers, helmets, and hats (Challoner et al., 1976; Davis et al., 1976; Downs et al., 2019; Downs et al., 2020; Henning et al., 2022). Research on these substances is mainly focused on promoting awareness of the use of photoprotection tools by quickly observing the changes in UV radiation to which we are exposed at any given time. Spirooxazines are easy-to-handle and low-cost photochromic substances, and are extensively used as UV indicators in wristbands, fabrics, detectors in sunglasses, merchandising items, and even as elements in tattoo inks (Goudjil, 1996; Goudjil, 1998; Carrol, 1999; Kurz et al., 2020). The reversible color change of spirooxazines is observed after a closed spiro form absorbs UV radiation and opens into a merocyanine form (Tian and Zhang, 2016; Han et al., 2022). Recently, they have been used as detectors to promote the reapplication of sunscreens, being incorporated into stickers placed on the skin (Hacker et al., 2019; Horsham et al., 2020a). These could become very useful for teaching children about sun exposure (Garnacho Saucedo et al., 2020), although they could also promote uncontrolled overexposure to the sun if used as toys, which has recently raised doubt about their usefulness in photodermatological forums.
The main objective of this research was the qualitative and quantitative analysis of the color change performance of a set of photochromic devices based on spirooxazines, as well as the analysis of these pure component substances to assess their potential usefulness as UV exposure indicators.
Materials and methods
A comparative experimental analysis between different UV-indicator wristbands, cards, and stickers and pure photochromic substances was carried out. Ten UV-indicator devices and a sample of three photochromic substances in red, purple, and blue, which are the substances that make up the majority of devices in commercial use, were used. The devices were acquired as gift samples, purchased from specific photoprotection education websites, and the pure photochromic substances were purchased from Hali Industries Co. (Changzhou, China). No indications of the compositions of the photochromic substances were shown in the different devices since no specific regulation is applied to these elements in their commercialization (Han et al., 2022). After requesting information from suppliers, only the provider of pure photochromic substances answered us with information about their composition. The pure photochromic substances acquired were 1-photochromic blue dye 6′-(indolin-1-yl)-1,3,3-trimethylspiro[indoline-2,3′-naphtho[2,1-b][1,4]oxazine], 2-photochromic purple-violet dye (1,3,3-trimethyl-6'-(piperidin-1-yl)spiro[indoline-2,3′-naphtho [2,1-b][1,4]oxazine]), and 3-photochromic red dye ((1,3,3-trimethylindolino-6'-(1-piperidinyl) spironaphthooxazine). More information on the compounds of the formula was the use of plyyoxymethyleneamine as a stabilization photochromic dye and styrene maleic anhydremonomethyl-maleate polymer as support material. We received no answer from suppliers in three cases and responses about protection under trade secrecy were given in another three cases. Only in three cases of UV cards and wristbands indicated to us that they use the Technocolor Purple 3—another name for the red and purple pure spirooxacines. One company referred to us to the patents US5581090A and US5914197A regarding the use of spirooxazines. (Goudjil, 1996; Goudjil, 1998).
The following indicator element type samples were analyzed with red/purple spirooxazines.
1. Bracelet of silicone UV test “Euromelanoma”. Seritec
2. ISDIN UV tester. Isdin
3. Kids Sun Watch. IPS
4. Silicone bracelet UV solar reactive. Zhongyepei. China
5. Smart Sun. Bluemarionge Health
6. UV Test Card. HUXUAN. China
7. Quantadose multi UV wavelength photochromic card
8. UV detection stickers. Sunny Patch
9. Photochromic substance Halli HLPC—1 (Red) Hali Industries Co. Changzhou, China
10. Photochromic substance Halli HLPC—2 (Purple)
11. UVI Evo Advanced Protection. IPS
12. Hönle test UV strip
13. Photochromic substance Halli HLPC—3 (Blue)
UV exposure and color change measurement and observation
This study’s objective was to qualitatively and quantitatively determine the color change response of the devices under different UV exposure conditions. An Oriel 300 W solar simulator (Newport Co. Cleveland, Ohio, United States) was used as the UV-visible light source (290–750 nm). In order to avoid any temperature effect on photochromic responses, the solar simulator included a constantly refrigerated water column in order to avoid any infrared radiation that could increase temperature at the output level (see Figure 1 on the position of the Teflon tubes for the water refrigeration system). Thus, the temperature at the site of exposure was a constant 21–22 °C. The spectral distribution as well as the UV-visible irradiance of the solar simulator was characterized using a Macam SR9910-V7 double monochromator spectroradiometer (Irradian Co., Scotland, United Kingdom) with an Ulbrich integrating sphere (Figure 1), calibrated at the National Institute of Aerospatial Technologies of Spain against a NIST-certified calibrated halogen lamp (1000 W −50 cm distance). The spectral distribution of the full UV-visible radiation (290–750 nm) emitted by the solar simulator is shown in Figure 2. The maximum irradiance measured at 3 cm from the lamp output was 435 W m-2 in the interval of 290–750 nm and 57.6 W m-2 of total UV in the interval of 290–400. This irradiance corresponds to midday mean summer solar irradiance in latitudes of south Spain (36oN) (Figure 2). To obtain the different UVI values to which the photochromic devices were exposed, the erythemally weighted irradiance of the light source was characterized from the physical energy units per wavelength weighted by its erythematic potential according to ISO/CIE 17166:2019 (ISO/CIE 17166, 2019). The final values were expressed in terms of UV index (UVI) as explained by the WHO to make solar radiation with a erythematic effect more understandable (WHO Team, 2003). To obtain different UVI values in which samples were exposed, the spectroradiometer sensor was situated gradually at different distances from the light source (from 5.5 cm at UV of 10–40 cm at UVI of 0.25) (Table 1). The color grade of the photochromic devices was determined using a DermaLab Combo Series colorimeter (Cortex Technology ApS., Denmark) associated with calculation software (Figure 1). This device provides color values in three-dimensional space based on the L*a*b system proposed by the Commission Internationale de l'Éclairage (CIE) (Smith and Guild, 1931; van der Wal et al., 2013). Basically, since color is a subjective perception and interpretation, color space is widely used because it consistently correlates numerical color values with human visual perception. L* indicates lightness and a* and b* are the chromatic coordinates: a* = red/green coordinates (+a indicates red, −a indicates green) and b* = yellow/blue coordinates (+b indicates yellow, −b indicates blue). In this study, the chromatic coordinate a* (positive values indicating red intensity) was used for most of the red/purple samples, while the chromatic coordinate b* was used for blue photochromic (negative values indicating blue intensity).
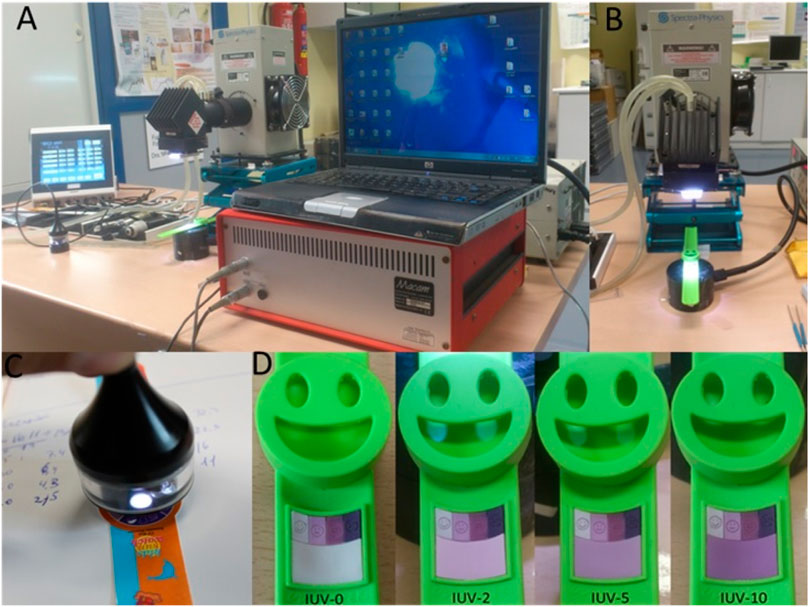
Figure 1. (A) Image of the lighting system with solar simulator, spectral measurement system, and colorimeter. (B) Illumination of photochromic wristbands under solar simulator. (C) Color intensity measurement. (D) Purple color of a photochromic wristband under different UVI levels.
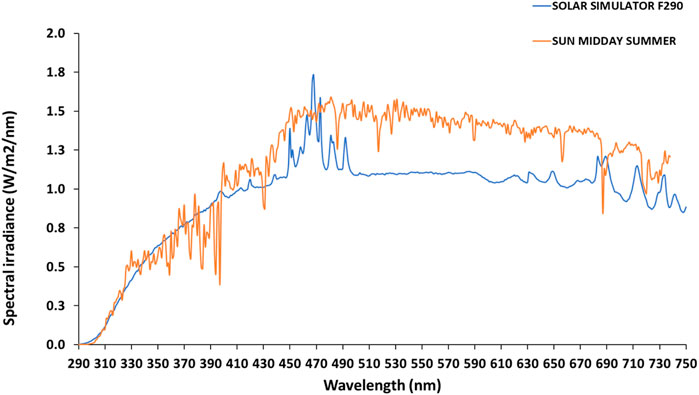
Figure 2. Spectral characteristics of transmitted UV-visible radiation (290–750 nm) from an Oriel solar simulator using a cut-off filter of 290 nm compared with the sun at midday on a mean summer day in Málaga (Spain). Solar spectrum was measured at midday (14:00 local time) in the second week of June 2022 using a MACAM spectroradiometer of double monochromator combined with an Ulbricht integrating sphere.

Table 1. Values corresponding to total UV irradiance, corresponding erythematic irradiance, UVI at each of the distances between the emission source, and the place where the photochromic strips are placed. In colors, the UVI levels corresponding to the WHO recommendations are green-low; yellow-medium; orange-high; violet-extreme.
Protocol
Five types of experiment were carried out to analyze the potential use of devices based on photochromic substances. Each experiment was performed in triplicate, and measurements were repeated twice per device (wristbands, UV detector, stickers, and pure photochromic substances) (Figure 3).
1. Activation time under very high UVI. Each UV device as well as pure photochromic substances were exposed to a constant UV irradiance of 57.6 W m-2, corresponding to a WHO Very High UVI value of 10 for 120 s, taking color measurements (a* or b* depending the color substance) during the test after 0, 7, 15, 30, 60, and 120 s.
2. Activation level by UVI value. Each device was exposed to different UVI values of 0, 0.25, 0.5, 1, 2, 3, 5, 7, and 10 for 60 s. The different UVI values were obtained by placing the photochromic devices at different distances from the emitting source of the solar simulator (Table 1).
3. Color reversal time. After color saturation under UVI of 10, each wristband was kept in darkness (samples and colorimeter sensor were placed into a closed box) and color measurements were made in same position of each UV device at 0, 15, 30, 60, and 120 s after exposure.
4. Activation level against different sunscreen application with different sun protection factors (SPF). An experiment was conducted to assess the potential of these devices as indicators of when the topical sunscreen should be reapplied. Two photochromic devices were used for the test: 1) a UV test card Huxuan (China) and 2) a Smart Sun band (Bluemarionge Health). For this purpose, a standard methacrylate plate (Schoemberg, Hamburg, Germany), used in the UVA sun protection test according to ISO 24443:2021 (ISO 24443, 2021) as skin simulating support, was placed on each photochromic band, and different sunscreen formulas were tested. These were the standard topical sunscreen P8 (expected sun protection factor of 60) from ISO 24444:2019 (ISO 24444, 2019) and one commercial sunscreen anthelios 50+ dermo-pediatrics gel ester from Laroche Posay (L'Oreal Co. Paris, France). Both products were applied at different concentrations diluted with the product excipient to obtain different mixtures with different SPF levels. They were diluted to 0% (excipient only), 12.5%, 25%, 50%, and 100% and applied on methacrylate plate at a concentration of 1.3 mg cm-2 following as per ISO 24443:2021. In vitro SPF values were 3.5, 5.9, 14.8, 29.6, and 59.2 for P8 formula and SPF of 4.1, 6.9, 17.3, 34.7, and 69.3 for Laroche Posay dermo-pediatrics sunscreen. The color level under different SPF combinations was measured after 60 s under UV irradiance of the solar simulator corresponding to a UVI of 10 (total UV irradiance of 57.6 W m-2).
5. Wavelength effect on activation level. In order to analyze the action spectrum of the spirooxazine-based photochromic substances activated by UV and visible light, one red UV detector card device as well as the pure red and blue photochromic substances (four replicas for each) were exposed under a solar simulator to a constant UV irradiance of 57.6 W m-2 (corresponding to a UVI value of 10). Different wavelength exposures were obtained using a set of different cut-off filters 290–450 nm (305, 310, 320, 330, 355, 370, 400, 420, and 450 nm Schott AG, Mainz, Germany). The values represent the approximate 50% transmission wavelength of each cut-off filter. The spectral characteristics of full and filtered UV and blue light by different cut-off filters is shown in Figure 3. In order to obtain the same light doses for all experiments, time exposure depended on the total transmitted light from 290 to 750 nm. Thus, exposure under filter with 50% transmission wavelength of 290 nm was 20 s and was gradually increased depending on the total irradiance measured (290-20 s, 305-21 s, 310-22 s, 320-23 s, 330-25 s, 355-25 s, 370-25 s, 400-28s, 420-30 s, 450-32 s).
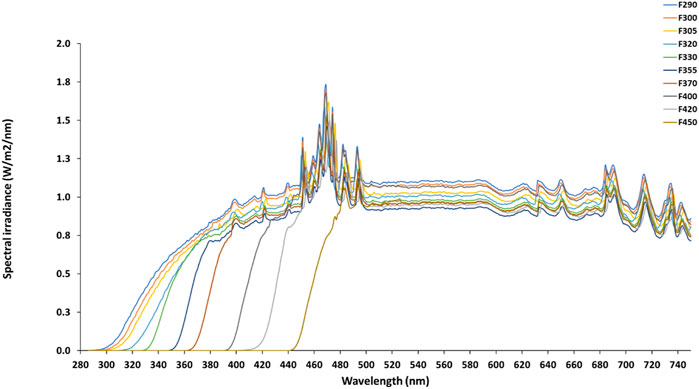
Figure 3. Spectral characteristics of the transmitted UV-visible radiation (290-750nm) from an Oriel solar simulator using a set of different cut-off filters used to determine wavelength activation of photochromic substances.
Results
Activation time
The activation response time of the different devices, photochromic wristbands, UV cards, UV stickers, and pure photochromic substances with respect to maximum value in relative units is represented in Figure 4. The response of most of the photochromic bands and pure photochromic red/purple substances was very fast, with color saturation of 7–15 s after illuminating the different as well as pure photochromic substances (Figure 4A). Only two wristbands needed 30 s to reach their maximum (Isdin Sun watch and Quantadose multi UV). The results were similar for the blue photochromic substances, with very fast activations reaching color saturation b* 7 s after illumination under simulated solar UV (Figure 4B).
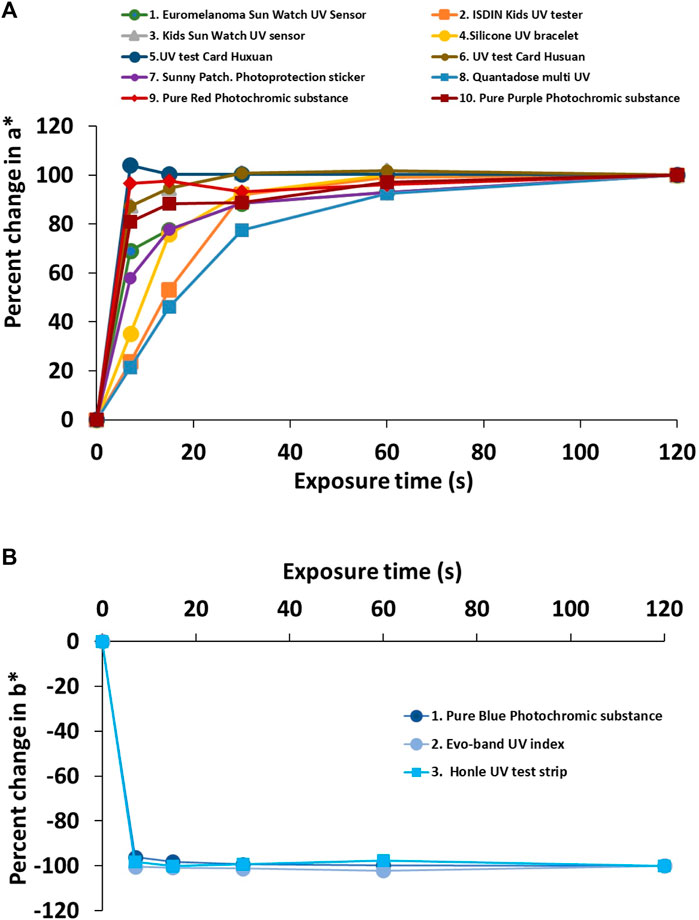
Figure 4. Change in a* (green to red) and b* (blue to yellow), relative to the maximum a* and b* values (of the CIE Lab color space) with respect to exposure time under a solar simulated total UV irradiance corresponding to an UVI value of 10. (A) Bracelets, stickers, UV cards, and pure red and purple photochromic substances (color space a* with positive values). (B) Blue UV cards and photochromic substance (color space b* with negative values). Standard deviation of mean values is less than 5% (data not shown).
Color reversion
Color reversion in the dark after irradiation took much longer than color saturation under a high UVI index of 10 (Figure 5A in relative units with respect to maximum values time 0). An exponential a* color decay pattern was observed for the color reversion of photochromic devices and pure substances. In the case of the pure photochromic substances, both red and purple, the decay was gradual, up to 120 s, while the other photochromic bands and bracelets were near baseline at 60 s. A similar effect was observer for blue devices and pure photochromic substances (Figure 5B).
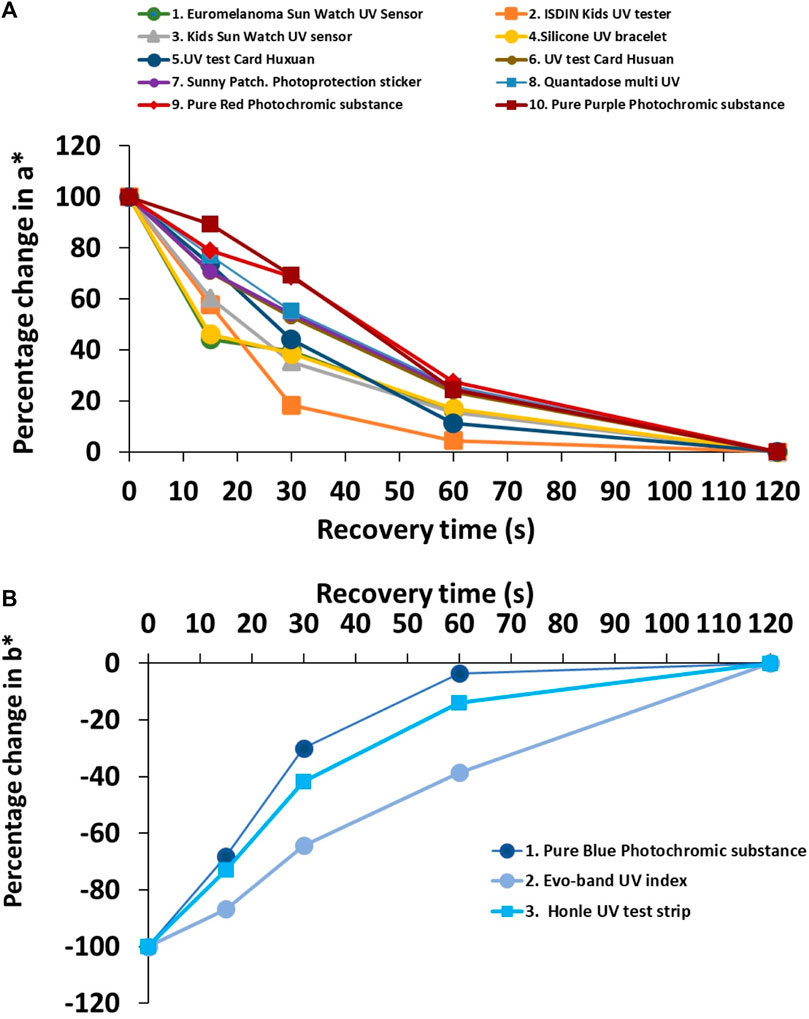
Figure 5. Change in a* (green to red) and b* (blue to yellow) (in relative units with respect to the initial maximum value of activation of the CIE Lab color space) in the dark after exposing several bracelets to a solar simulated total UV irradiance corresponding to an UVI value of 10. (A) Bracelets, stickers, UV cards, and pure red and purple photochromic substances (color space a* with positive values). (B) Blue UV cards and photochromic substance (color space b* with negative values). Standard deviation of mean values less than 5% (data not shown).
Color activation under different UVI values
The color response of the different devices, photochromic wristbands, UV cards, UV stickers, and pure photochromic was analyzed against exposure to different UVI levels (Figure 6 in relative units with respect to maximum values). All bands and pure photochromic substances showed color saturation (a* and b* values of the CIE Lab color space) when exposed to a very low UVI of 2–3 (Figure 6A). Only wristband 3 gradually increased the color level up to a UVI of 10. When color changes were analyzed by eye observation, the a* and b* color changes detected by the colorimeter were no longer visible to the human eye after 15 s under irradiances corresponding to a UV index of 2 and over (Figure 7).
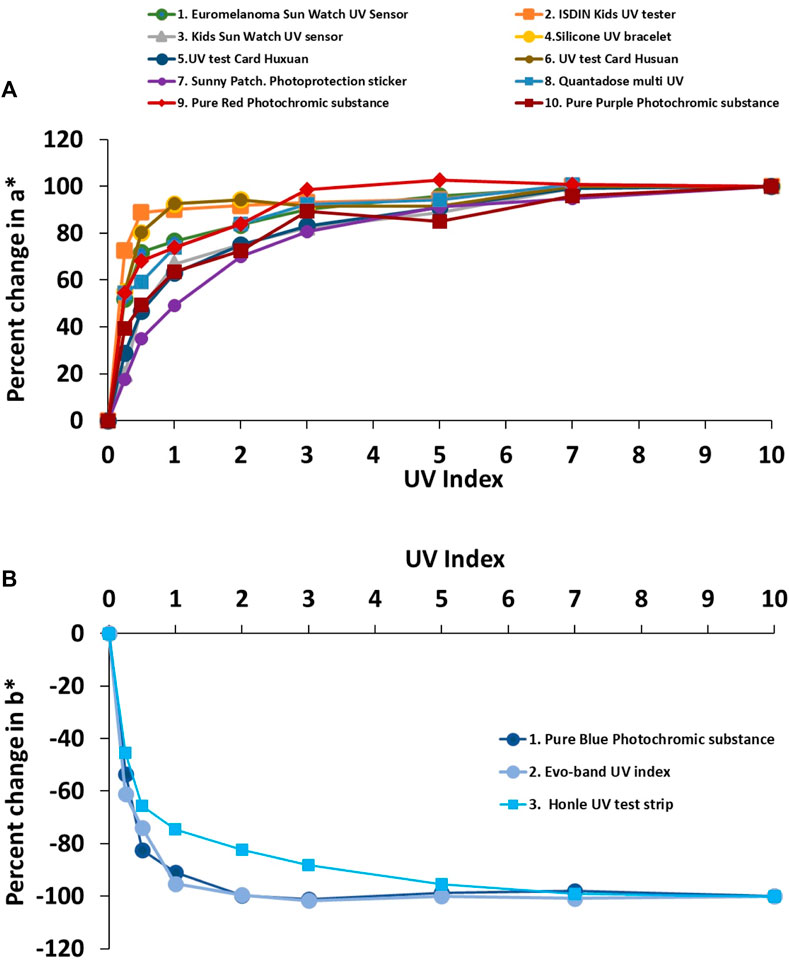
Figure 6. Change in a* (green to red) and b* (blue to yellow) (from the CIE Lab color space) with increasing solar simulated total UV irradiance corresponding to an UVI increment from 0 to 10. (A) Bracelets, stickers, UV cards, and pure red and purple photochromic substances (color space a* with positive values). (B) Blue UV cards and photochromic substance (color space b* with negative values). Standard deviation of mean values is less than 5% (data not shown).
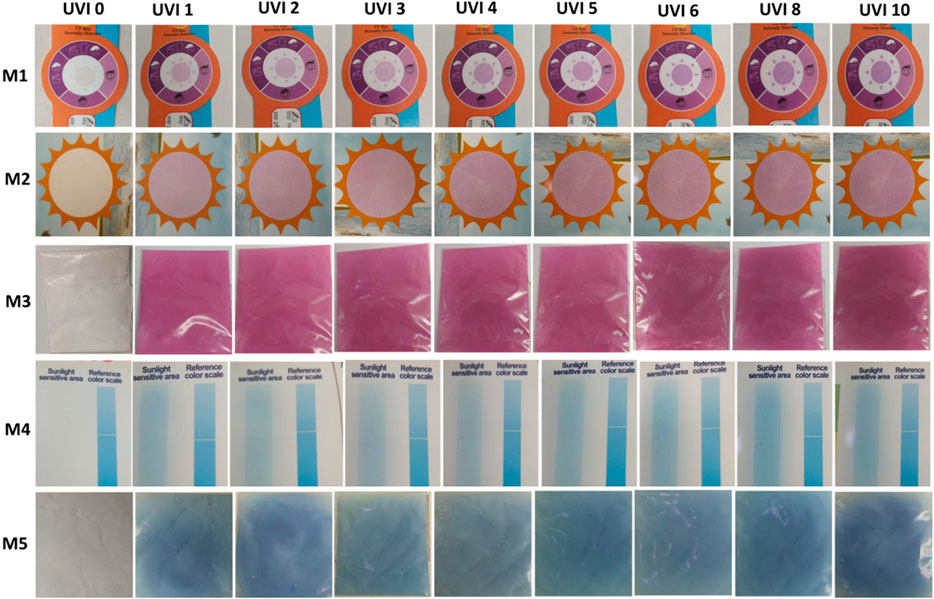
Figure 7. Photograph of the photochromic substances and two examples of UV detector devices after 15 s of exposure under different UV-visible irradiance corresponding to UVI from 1 to 10. M1 corresponds to a Kid Sun Watch (IPS), M2: UV Test Card (Huxuan), M3 Red pure photochromic substance, M4: UVI Evo Advance Protection (IPS) and M5: Pure blue photochromic substance.
The time necessary for activation of the two examples of photochromic substances as well as a red (Sunny patch photoprotection sticker) and blue (Hönle UV test strip) under different UV-visible irradiances corresponding to different UVI (from 1 to 10) is shown in Figures 8A–D. In all cases a gradual increase in color can be observed following a potential model of longer saturation times under lower UV-visible irradiance corresponding to lower UVI values. UVI over 5 required around 10 s for saturation. In the case of lower irradiances, saturation took 30 s. Similar results were observed in the case of blue devices. Differences observed in the velocity of saturation reflects the difficulty in determining a fixed time for using the photochromic devices as dosimeters, especially by simple observation as observed in the devices’ photos.
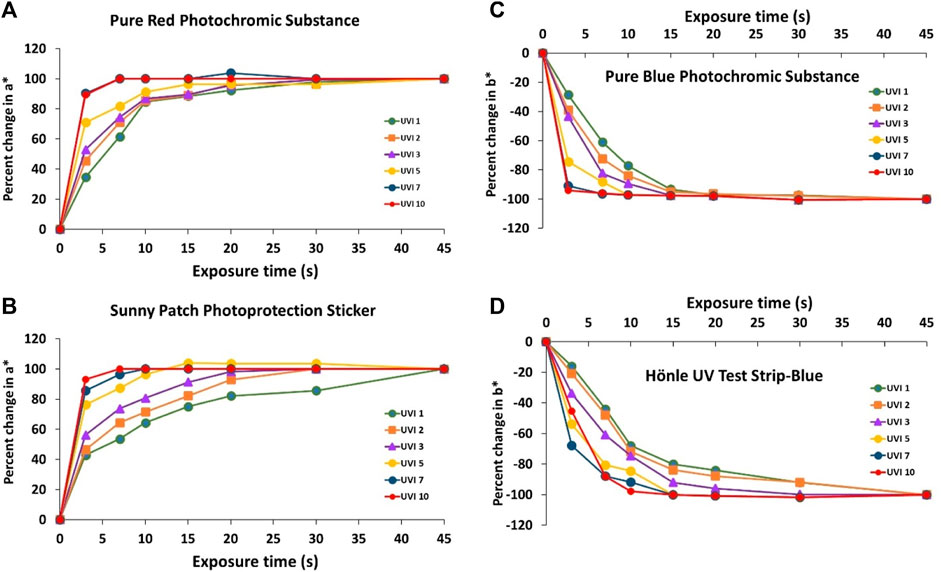
Figure 8. Time necessary for color activation measured in terms of a* (green to red) and b* (blue to yellow) (from the CIE Lab color space) in terms of relative values with respect to the maximum value, with increasing solar simulated total UV-visible irradiance corresponding to an UVI increment from 0 to 10. (A) Red photochromic substances (color space a* with positive values). (B) Sunny patch photoprotection sticker (red photochromic), (C) pure blue photochromic substance, and (D) Hönle UV test strip blue.
Activation under different sun protection factor sunscreens
To analyze spirooxazine-based photochromic substances as detectors for sunscreen reapplication, a photochromic detection band, the UV test card Huxuan red, a Sunny Patch photoprotection sticker (used as a sunscreen re-application photochromic device), and the pure red photochromic substance were used. Figure 9 shows the color changes of photochromic devices under PMMA plates with different SPF measured after 60 s under 57.6 W m-2 of UV, corresponding to a UVI of 10. In all cases, the increase in SPF produced a gradual exponential decrease in color level a* from the SPF of 0, or untreated plate which saturated the UV band, sticker, and pure red photochromic product with color. Although there was a gradual decrease in the degree of color, pink/red was visible to the naked eye, and, from SPF 17 for the standard P8 and 20 for the pediatric sunscreen, no further decrease was apparent to the naked eye. It could also be observed that even at protection factors 60 and 69 (high SPF of both sunscreens), after 1 min of exposure there was a UVI of 10. Only the Sunny patch photoprotection sticker showed no color change after 1 min under a UVI of 10. However, a value of a* color of 15 was again observed under SPF 30 under P8 sunscreen standard and, in cases of dermo-pediatrics SPF 50+ sunscreen, very low values of a* color were observed from SPF 17. Results indicate that photochromic substances are very sensitive to UV, and it was possible to observe color changes under SPF values sufficient for good photoprotection for skin that could lead to unnecessary reapplication of sunscreens in normal sun exposure.
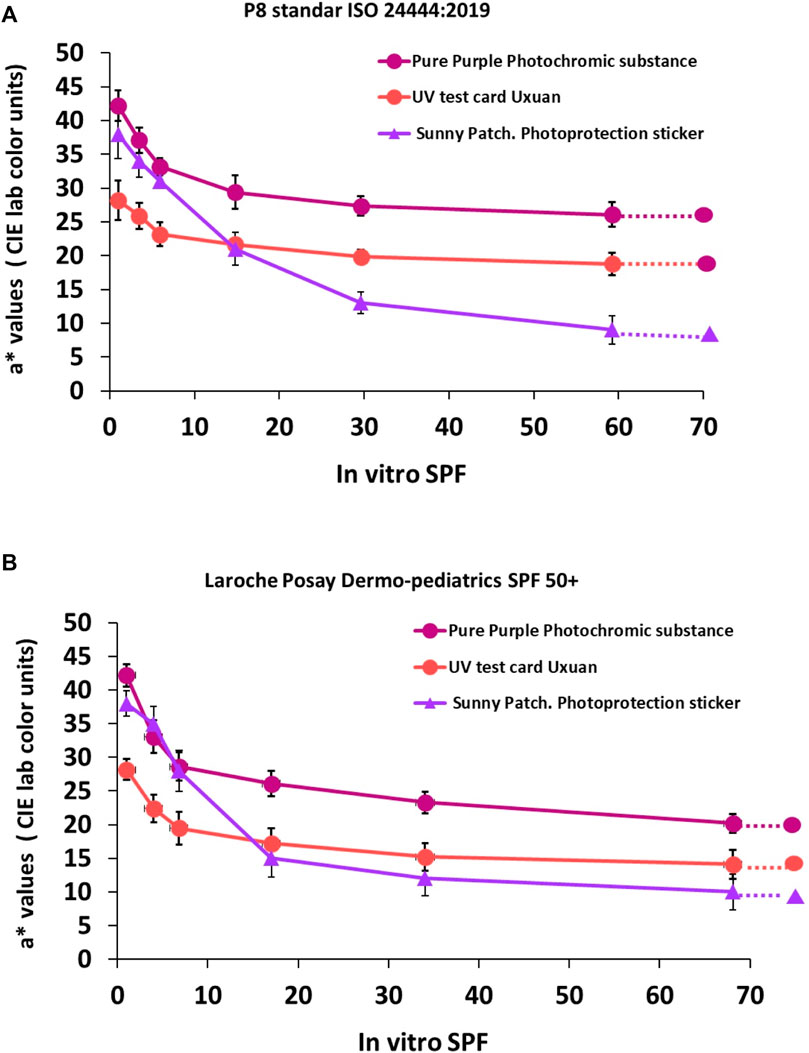
Figure 9. Change in a* color values (of the CIE Lab color space) in the dark after exposing one UV detector test card and the red pure photochromic substance to different sun protection factors (SPF) after application of (A) P8 reference of the ISO 2444:2019 and (B) a pediatric SPF 50+ commercial sunscreen. The exposure time was 1 min under an irradiance of total solar simulated UV radiation of 45.7 W m-2, corresponding to an UVI of 10 after ponderation with respect to erythema action spectrum. The dashed lines indicate the color level when the pure photochromic substance or each device was non-irradiated (similar to that obtained when irradiated under highest SPF).
Finally, in order to analyze how the exclusion of parts of the UV-visible spectrum affects the activation of photochromic substances, some red and blue devices and pure substances were irradiated under a series of long-pass filters with a range of cut-off wavelengths; the results are shown in Figures 10–12. Both the pure red and red UV card as well as the pure photochromic substance as examples were analyzed, and the color reached after 20–25 s under cut-off wavelengths under 370 nm and did not vary (Figures 10, 11). The maximum color of the pigment activation was visually observed under the different cut-off filters with respect to the half of the irradiated surface not covered by the filters, as shown in the device photos. The color level after activation was gradually decreased for cut-off wavelengths of 370–400 nm, and exposure over 450 nm still showed a faint but significant color level of the photochromic substances after just 32 s of irradiation. Thus, spirooxazines as photochromic substances can be activated not only by UV radiation but also by high-energy visible radiation. Similar results were observed for blue devices (Figures 10–12).
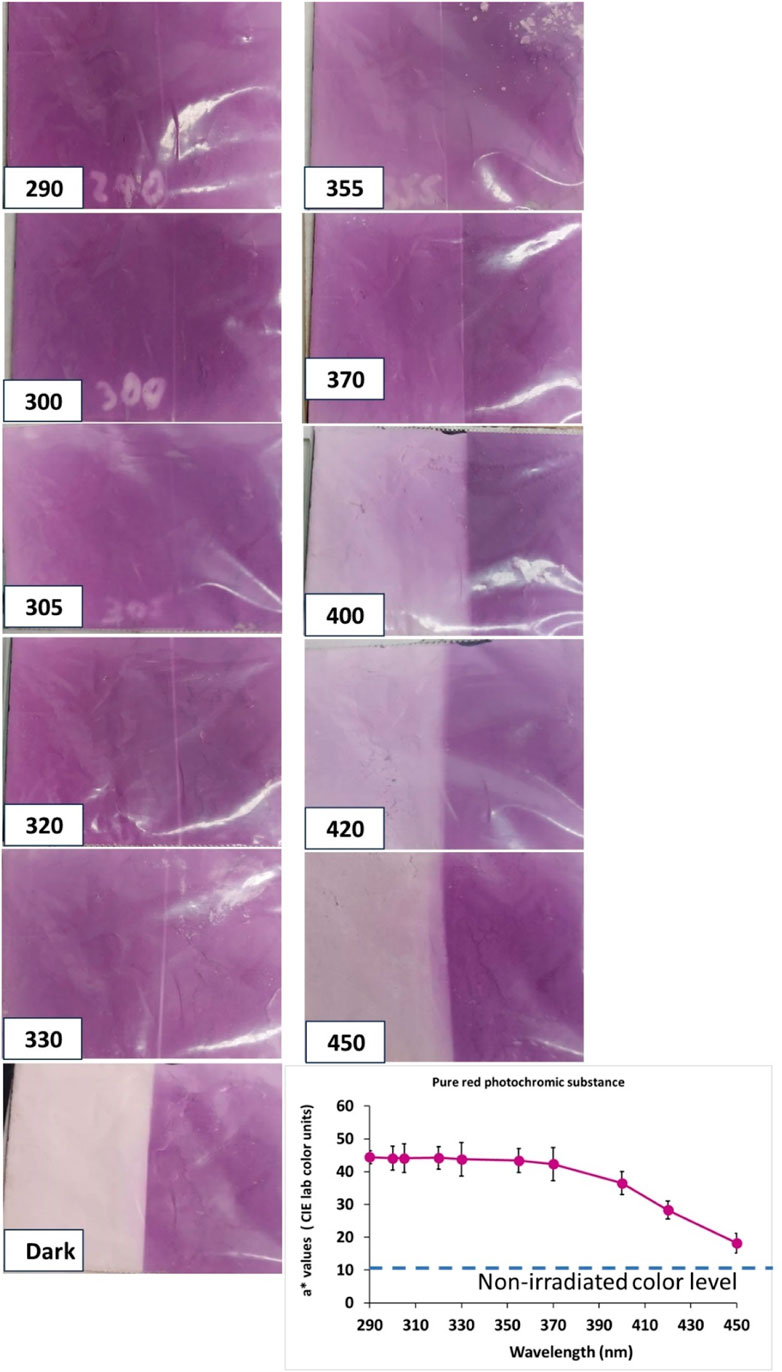
Figure 10. Photographs as well as the figure of the change in a* color values (of the CIE Lab color space) after exposure of 30–35 seg under total UV-visible radiation corresponding to an UVI of 10 across different cut-off filters. Each wavelength indicates the approximate 50% transmission wavelength of each cut-off filter. Substances, included in a UV transparent plastic bag, were directly exposed to the solar simulator, and half of the surface was covered by the different cut-off filters. Non-irradiated half of the substance was made covering with a black PMMA piece.
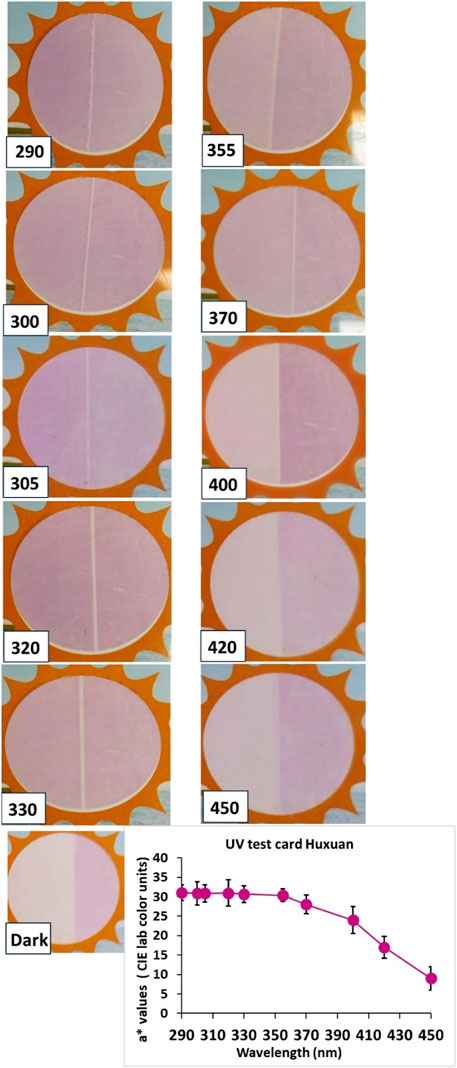
Figure 11. Photographs as well as the figure of the change in a* color values (of the CIE Lab color space) after exposure of 30–35 seg under total UV-visible radiation corresponding to an UVI of 10 across different cut-off filters. Each wavelength indicates the approximate 50% transmission wavelength of each cut-off filter. UV detectors were directly exposed to the solar simulator and half of the surface was covered by the different cut-off filters. Non-irradiated half of the UV detector was made covered with a black PMMA piece.
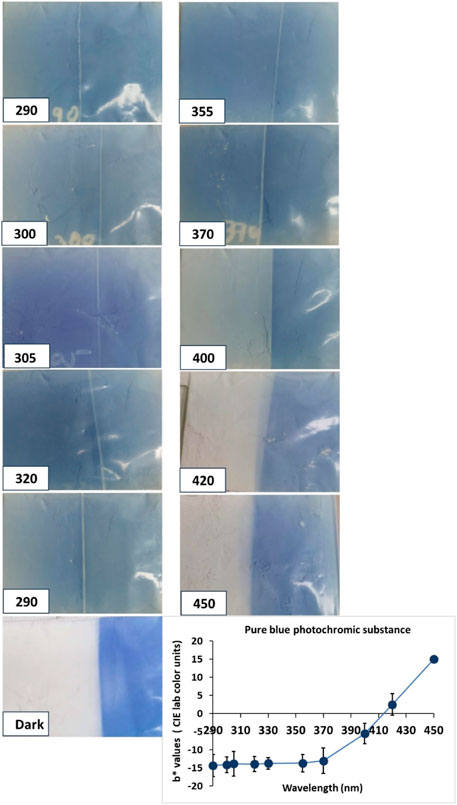
Figure 12. Photographs as well as the figure of the change in b* color values (of the CIE Lab color space) after exposure of 30–35 seg under total UV-visible radiation corresponding to an UVI of 10 across different cut-off filters. Each wavelength indicates the approximate 50% transmission wavelength of each cut-off filter. Substances, included into a UV transparent plastic bag, were directly exposed to the solar simulator and half of the surface was covered by the different cut-off filters. The non-irradiated half of the substance was made covered with a black PMMA piece.
Discussion
Protection against excessive exposure to solar UV radiation should begin in childhood due to high exposure. Both because of our social learning environment and the physiological need for vitamin D synthesis, sun exposure in children is high from an early age. It is in this learning process that tools such as UV radiation indicator bracelets, like those analyzed in this study, could be particularly useful in establishing good habits (Boldeman et al., 2004; Blázquez-Sánchez et al., 2021; Huh et al., 2021; Thoonen et al., 2021).
Previous results of this research group have shown experience with the use of bio-dosimeters (Biologically weighting UV dosimetry VioSpor, 2023; Horsham et al., 2020b), which at the research level have demonstrated great potential as biosensors. We have used these to characterize the daily exposures of athletes in Olympic competition (Gutiérrez-Manzanedo et al., 2023) or for occupational exposures of personnel at risk due to high sun exposure (physical education teachers, lifeguards, construction workers, and street cleaners) (Castro-Maqueda et al., 2019; de Troya Martín et al., 2021). However, in this study we have attempted to address the potential of spirooxazine films, which are widely used as UV detectors and as dosimeters (Thieden et al., 2000; Henning et al., 2022) due to their ease of use, reversibility of measurement, and low cost. Other bands of photochromic substances have been designed to indicate when photoprotection should be reapplied and even allow the application of the cream on the bracelet to indicate when the sunscreen is no longer effective (Horsham et al., 2020b). The results obtained in this work confirm these facts, as these bracelets are good indicators of UV presence/absence but do not offer potential utility as radiation level sensors or dosimeters. Horsham et al. (2020c) demonstrated that the use of these bracelets (presence of red color change) led to attitudinal changes in a population of 663 adolescents participating in a study of photoprotection and sun exposure behavior during an outdoor festival on the Gold Coast in Australia. These indicator bracelets prompted the use of sunglasses and topical sunscreen, although the decrease in sunburn was not significant.
The positive aspect of these widely used photochromic substances is that they require a very short activation time under the sun to show the assumed amount of radiation to which we would be exposed, while in total darkness, which would be ideal for their return to the pre-exposure state, it would take no more than 1 min for most of the substances to reverse, as demonstrated in the present work.
A limitation of the UVI indicator bracelets is their response to the light intensity to which we are exposed. From colorimetric analysis as well as from visual observation of the photochromic substances of different red and blue devices, no gradual increase in color depending on light intensity could be observed. In terms of total solar simulated UV radiation corresponding to UVI values of 2, the photochromic substances are saturated in almost all the devices and pure photochromic substances. This UVI of 2 corresponds to maximum values for winter days at our latitude. Therefore, we have an indicator of the level of exposure to UV radiation that offers the same color value at 10:00 intensities on a summer day (UVI of 2) as at 14:00 (UVI of 9–10) (Aguilera et al., 2020). When a deeper analysis regarding the potential as UV detectors of some example devices and pure red and blue photochromic substances was performed, the color activation under different cut-off filters again showed an important conclusion (Figures 10–12). All devices and pure substances showed similar color activation when UV radiation was cut off at 370 nm and under, with color saturation reached after activation in less than 25 s and with a lower level but significant activation when solar radiation was cut off over 400 nm. No significant added effect was found when UVB was included with UVA in the cut-off filter of 290 nm. When photochromic devices were exposed to only UVB (exposure under 5.8 W m-2 of a narrow Band UVB phototherapy lamp (Philips PL-S 9 W/01, Philips, Netherlands) with a peak at 311 nm), only faint activation was observed after 120 s (data not shown). A full color change should have occurred if the devices were responding to erythemal irradiance since this exposure has a UVI >10. Thus, the activation under solar simulated UV-visible radiation was mainly due to UVA radiation. It is therefore difficult to accurately correlate color changes with UVI as many suppliers indicate that UVI (related to erythemal wavelengths) depends mainly on UVB radiation. It is clear that when solar radiation increases to higher UVI values, solar UVA irradiance also increases, and we could correlate the activation of photochromic devices with respect to increased total UV radiation and, therefore, the UVI. However, the atmospheric conditions that affect UVI (clouds, ozone, aerosols) cannot be objectively quantified with photochromic devices because solar spectral characteristics change in these atmospheric conditions. Since photochromic-based devices respond to UV long wavelengths, and these longer wavelengths reach earth surface at much higher irradiances, the devices would be more informative as total UV dosimeters (mainly UVA radiation) than as UVI detectors.
A similar limitation applies to the use of spirooxazine-based photochromic substances as detectors for the reapplication of sunscreen (Hacker et al., 2019; Horsham et al., 2020a). A priori, a color change of the sticker placed on the skin and covered by sunscreen could give us an idea of when to re-apply the sunscreen. We found that when sunscreen with SPF over 60 was applied over the photochromic devices, the color was not altered over 1 min of exposure. However, when the same sunscreen was diluted with excipient in order to reach SPF of 30 and lower, color changes could be observed very quickly. This means that a user, only by simply observing the color of the photochromic device, can make a decision to reapply sunscreen even when it offers an SPF of 30 and does not really require reapplication. Again, the photochromic substances are very sensitive to UV, even when there is enough sunscreen on the skin and a user could decide to unnecessarily reapply it. Sunscreens with very high SPF ensure a long time of photoprotection on the skin. We should (based on color changes even at time interval of few minutes) follow international recommendations of reapplication every 2 h or when skin conditions have been altered (swimming, sport, skin friction) as the best way to protect against solar overexposure.
The photochromic responses of spirooxazine to temperature could also lead to color changes that not only depend on UV presence (di Nunzio et al., 2008). Thus, all our experiments have been done under controlled temperature conditions of around 21–22 °C; however, in normal use conditions, this temperature control does not exist, especially under elevated temperature exposure in summer. This could affect final readings of photochromic color change not only due to UV radiation.
In conclusion, wristbands, UV stickers, and UV detection cards based on photochromic substances as spirooxazine can serve as fast indicators of the presence/absence of UV, which, when translated into educational terms, could be useful for raising awareness among children of the importance of sun protection. Other uses such as UV level indication or objective sunscreen reapplication indication are limited, given our results. In addition, the lack of information associated with the use of such devices offered as promotional items increases the lack of objective control of UV exposure; therefore, the use of these color-changing devices should be limited to certain uses and not be used on a generalized basis.
Data availability statement
The raw data supporting the conclusion of this article will be made available by the authors without undue reservation.
Author contributions
JA: Conceptualization, Data curation, Investigation, Methodology, Validation, Writing–original draft. DC-G: Data curation, Investigation, Methodology, Validation, Writing–review and editing. CS-R: Data curation, Investigation, Methodology, Writing–review and editing. SG: Supervision, Validation, Writing–review and editing. RB-G: Supervision, Validation, Writing–review and editing. EH-C: Supervision, Validation, Writing–review and editing. MdG: Investigation, Project administration, Supervision, Writing–review and editing.
Funding
The authors declare that financial support was received for the research, authorship, and/or publication of this article. This research is part of the project funded by the State Programs of Generation of Knowledge and Scientific and Technological Strengthening of the System from the Spanish Ministry of Science and Innovation, Grant/Award Number: PID 2020- 117224RB-100. This work is part of the research of the Institute of Biomedicine of Málaga (IBIMA) and the Junta de Andalucía working group CTS-162.
Conflict of interest
The authors declare that the research was conducted in the absence of any commercial or financial relationships that could be construed as a potential conflict of interest.
Publisher’s note
All claims expressed in this article are solely those of the authors and do not necessarily represent those of their affiliated organizations, or those of the publisher, the editors and the reviewers. Any product that may be evaluated in this article, or claim that may be made by its manufacturer, is not guaranteed or endorsed by the publisher.
References
Aguilera, J. (2019). Nociones fundamentales de Fotobiología. En: Escalas J. Fotodermatología. 2nd Edn. (Madrid: Editorial Médica Panamericana), 3–11.
Aguilera, J., de Gálvez, M. V., Aguilera, P., de Troya, M., and Gilaberte, Y.en representación del Grupo Español de Fotobiología de la AEDV (2020). Recommendations on sun exposure and photoprotection following easing of the COVID-19 pandemic lockdown: Spanish photobiology group of the Spanish academy of dermatology and venerology (AEDV). Actas Dermosifiliogr. Engl. Ed. 111, 799–801. doi:10.1016/j.ad.2020.06.001
Biologically weighting UV dosimetry VioSpor (2023). Biologically weighting UV-dosimetry VioSpor the UV-sensitive “artificial skin”. Available at: http://www.biosense.de/v-e.htm (Accessed April 15, 2023).
Blázquez-Sánchez, N., Rivas-Ruiz, F., Bueno-Fernández, S., Fernández-Morano, M. T., Arias-Santiago, S., Rodríguez-Martínez, A., et al. (2021). Photoprotection habits, attitudes, and knowledge among school communities in the Costa del sol (Spain). Eur. J. Public Health. 31, 508–514. doi:10.1093/eurpub/ckab010
Boldeman, C., Dal, H., and Wester, U. (2004). Swedish pre-school children’s UVR exposure - a comparison between two outdoor environments. Photodermatol. Photoimmunol. Photomed. 20, 2–8. doi:10.1111/j.1600-0781.2004.00069.x
Castro-Maqueda, G., Gutierrez-Manzanedo, J. V., Fernandez-Santos, J. R., Linares-Barrios, M., and Troya Martín, M. (2019). Sun protection habits and sun exposure of physical education teachers in the south of Spain. Photochem. Photobiol. 95, 1468–1472. doi:10.1111/php.13147
Challoner, A. V., Corless, D., Davis, A., Deane, G. H., Diffey, B. L., Gupta, S. P., et al. (1976). Personnel monitoring of exposure to ultraviolet radiation. Clin. Exp. Dermatol. 1, 175–179. doi:10.1111/j.1365-2230.1976.tb01413.x
Coelho, P. J. (2006). Study of the photochromic behaviour of a naphthopyran: a simple experiment illustrative of the photochromic phenomenon. Quimica Nova 3, 607.
Davis, A., Deane, G. H. W., and Diffey, B. L. (1976). Possible dosimeter for ultraviolet radiation. Nature 261, 169–170. doi:10.1038/261169a0
de Troya Martín, M., Blázquez Sánchez, N., García Harana, C., Alarcón Leiva, M. C., Aguilera Arjona, J., Rivas Ruiz, F., et al. (2021). Beach lifeguards' sun exposure and sun protection in Spain. Saf. Health Work 12, 244–248. doi:10.1016/j.shaw.2020.10.003
di Nunzio, M. R., Gentili, P. L., Romani, A., and Favaro, G. (2008). Photochromic, thermochromic, and fluorescent spirooxazines and naphthopyrans: a spectrokinetic and thermodynamic study. Chemphyschem 4, 768–775. doi:10.1002/cphc.200700789
Downs, N. J., Axelsen, T., Parisi, A. V., Schouten, P. W., and Dexter, B. R. (2020). Measured UV exposures of ironman, sprint and olympic-distance triathlon competitors. Atmosphere 11, 440. doi:10.3390/atmos11050440
Downs, N. J., Igoe, D. P., Parisi, A. V., Taylor, O., Lazzaroni, S. L., Rawlings, A., et al. (2019). Seasonal minimum and maximum solar ultraviolet exposure measurements of classroom teachers residing in tropical north queensland, Australia. Photochem. Photobiol. 95, 1083–1093. doi:10.1111/php.13081
Garnacho Saucedo, G. M., Salido Vallejo, R., and Moreno Giménez, J. C. (2020). Effects of solar radiation and an update on photoprotection. Pediatr (Engl Ed) 92 (92), 377.e1–377. doi:10.1016/j.anpedi.2020.04.014
Gilaberte, Y., and González, S. (2010). Update on photoprotection. Actas Dermosifiliogr. 101, 659–672. doi:10.1016/s1578-2190(10)70696-x
Gutiérrez-Manzanedo, J. V., Vaz Pardal, C., Blázquez-Sánchez, N., De Gálvez, M. V., Aguilera-Arjona, J., González-Montesinos, J., et al. (2023). Ultraviolet exposure of competitors during a Tokyo olympic sailing regatta test event. Photodermatol. Photoimmunol. Photomed. 39, 325–331. doi:10.1111/phpp.12839
Hacker, E., Horsham, C., Ford, H., Hartel, G., Olsen, C. M., Pandeya, N., et al. (2019). UV detection stickers can assist people to reapply sunscreen. Prev. Med. Balt. 124, 67–74. doi:10.1016/j.ypmed.2019.05.005
Han, F., Wang, T., Liu, G., Liu, H., Xie, X., Wei, Z., et al. (2022). Materials with tunable optical properties for wearable epidermal sensing in health monitoring. Adv. Mater 34 (26), e2109055. doi:10.1002/adma.202109055
Henning, A. J., Downs, N., and Vanos, J. K. (2022). Wearable ultraviolet radiation sensors for research and personal use. Int. J. Biometeorol. 66, 627–640. doi:10.1007/s00484-021-02216-8
Horsham, C., AntrobusJ, O. C. M., Ford, H., Abernethy, D., and Hacker, E. (2020c). Testing wearable UV sensors to improve sun protection in young adults at an outdoor festival: field study. JMIR Mhealth Uhealth 8, e21243. doi:10.2196/21243
Horsham, C., Ford, H., and Hacker, E. (2020a). Promoting sunscreen use in adolescents playing outdoor sports using UV detection stickers. Prev. Med. Rep. 19, 101166. doi:10.1016/j.pmedr.2020.101166
Horsham, C., Ford, H., and Hacker, E. (2020b). Promoting sunscreen use in adolescents playing outdoor sports using UV detection stickers. Prev. Med. Rep. 19, 101166. doi:10.1016/j.pmedr.2020.101166
Huh, J., Lee, K. J., Roldan, W., Castro, Y., Kshirsagar, S., Rastogi, P., et al. (2021). Making of mobile SunSmart: Co-designing a just-in-time sun protection intervention for children and parents. Int. J. Behav. Med. 28, 768–778. doi:10.1007/s12529-021-09987-9
ISO 24444 (2019). Cosmetics — sun protection test methods — in vivo determination of the sun protection factor (SPF). 2st Edn. International Standard Organization.
ISO 24443 (2021). Cosmetics —determination of sunscreen UVA photoprotection in vitro. 2nd Edn. International Standard published.
Kozlenko, A. S., Ozhogin, I. V., Pugachev, A. D., Lukyanova, M. B., El-Sewify, I. M., and Lukyanov, B. S. (2023). A modern look at spiropyrans: from single molecules to Smart materials. Top. Curr. Chem. (Cham) 381, 8. doi:10.1007/s41061-022-00417-2
Kurz, W., Yetisen, A. L., Kaito, M. V., Fuchter, M. J., Elsner, M., Koch, A. W., et al. (2020). UV-Sensitive wearable devices for colorimetric monitoring of UV exposure. Adv. Opti. Mater 8, 1901969. doi:10.1002/adom.201901969
Pérez Ferriols, A., Aguilera, J., Argila, D., Barnadas, M. A., Cabo, X., Carrrascosa, J. M., et al. (2014). Determination of minimal erythema dose and anomalous reactions to UVA radiation by skin phototype. Actas Dermosifiliogr. 105, 780–788. doi:10.1016/j.ad.2014.05.001
Sánchez -Laulhé Ollero, J. M. (2021) “Informe sobre el estado del clima de España 2020,” in Agencia Estatal de Meteorología, 10.
Saric-Bosanac, S. S., Clark, A. K., Nguyen, V., Pan, A., Chang, F. Y., Li, C. S., et al. (2019). Quantification of ultraviolet (UV) radiation in the shade and in direct sunlight. Dermatol. Online J. 25, 13030. doi:10.5070/d3257044801
Smith, T., and Guild, J. (1931). The C.I.E. colorimetric standards and their use. Trans. Opt. Soc., 33.
Thieden, E., Agren, M. S., and Wulf, H. C. (2000). The wrist is a reliable body site for personal dosimetry of ultraviolet radiation. Photodermatol. Photoimmunol. Photomed. 16, 57–61. doi:10.1034/j.1600-0781.2000.d01-4.x
Thoonen, K., van Osch, L., Crutzen, R., de Vries, H., and Schneider, F. (2021). Identification of relevant sociocognitive determinants explaining multiple parental sun protection behaviors. Health Educ. Behav. 31, 392–404. doi:10.1177/10901981211010434
H. Tian, and J. Zhang (2016). (Wiley-VCH Verlag GmbH & Co).Photochromic materials: preparation, properties and applications.
Turner, J., Igoe, D., Parisi, A. V., McGonigle, A. J., Amar, A., and Wainwright, L. (2020). A review on the ability of smartphones to detect ultraviolet (UV) radiation and their potential to be used in UV research and for public education purposes. Sci. .Total Environ. 706, 135873. doi:10.1016/j.scitotenv.2019.135873
van der Wal, M. B. A., Bloemen, M., Verhaegen, P., Tuinebreijer, W., de Vet, H., van Zuijlen, P. P., et al. (2013). Objective color measurements: clinimetric performance of three devices on normal skin and scar tissue. J. Burn Care Res. 34, 187–e194. doi:10.1097/BCR.0b013e318264bf7d
Wang, J., Jeevarathinam, A. S., Jhunjhunwala, A., Ren, H., Lemaster, J., Luo, Y., et al. (2018). A wearable colorimetric dosimeter to monitor sunlight exposure. Adv. Mater. Technol. 3, 1800037. doi:10.1002/admt.201800037
Keywords: indicator bracelets, photochromic substances, photoprotection, ultraviolet radiation, children game
Citation: Aguilera J, Cárdenas-González D, Sánchez-Roldán C, González S, Bosch-García R, Herrera-Ceballos E and de Gálvez MV (2024) Qualitative and quantitative analysis of colorimetric response in different UV solar radiation level indicator bracelets based on photochromic substances. Front. Photobiol. 2:1281474. doi: 10.3389/fphbi.2024.1281474
Received: 22 August 2023; Accepted: 05 July 2024;
Published: 08 August 2024.
Edited by:
Patrick J. Neale, Smithsonian Environmental Research Center (SI), United StatesReviewed by:
Ravindra Sonajirao Kale, Louisiana State University, United StatesMichael Gorka, The Pennsylvania State University (PSU), United States
Copyright © 2024 Aguilera, Cárdenas-González, Sánchez-Roldán, González, Bosch-García, Herrera-Ceballos and de Gálvez. This is an open-access article distributed under the terms of the Creative Commons Attribution License (CC BY). The use, distribution or reproduction in other forums is permitted, provided the original author(s) and the copyright owner(s) are credited and that the original publication in this journal is cited, in accordance with accepted academic practice. No use, distribution or reproduction is permitted which does not comply with these terms.
*Correspondence: J. Aguilera, amFndWlsZXJhQHVtYS5lcw==