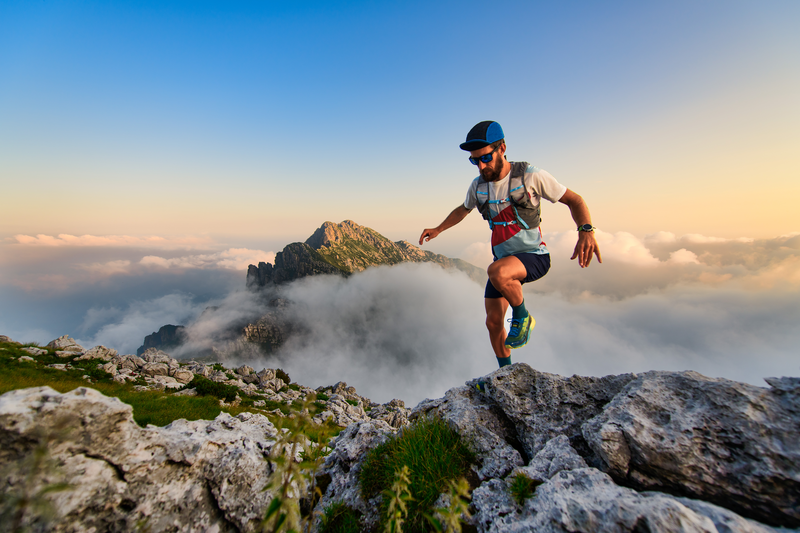
95% of researchers rate our articles as excellent or good
Learn more about the work of our research integrity team to safeguard the quality of each article we publish.
Find out more
ORIGINAL RESEARCH article
Front. Pharmacol. , 01 April 2025
Sec. Inflammation Pharmacology
Volume 16 - 2025 | https://doi.org/10.3389/fphar.2025.1535969
This article is part of the Research Topic Ferroptosis: Intersections, Implications, and Innovations in Programmed Cell Death View all 12 articles
Introduction: Cisplatin-induced acute kidney injury (AKI) is primarily caused by oxidative stress from reactive oxygen species (ROS) accumulation. Developing ROS scavengers presents promising opportunities for preventing and treating this condition by targeting oxidative stress mechanisms.
Methods: This study involves the fabrication of a metal-polyphenol self-assembled nanozyme (Fe@Ba) designed to inhibit ferroptosis through synergistic catalytic actions and antioxidant properties. The nanozyme is constructed using metal-polyphenol coordination-driven nanoprecipitation techniques. Its performance is evaluated in vitro using MTEC cells and in vivo within an AKI model, with assessments of catalytic activities, ROS depletion efficacy, antioxidant effects, and anti-ferroptotic mechanisms.
Results: The Fe@Ba nanozyme demonstrates significant catalase (CAT) and superoxide dismutase (SOD)-like activities upon internalization by MTEC cells, effectively reducing high ROS levels in the AKI model. Baicalein (Ba), a traditional Chinese medicine component in the nanozyme, exhibits strong antioxidant properties, inhibits lipid peroxidation (LPO), upregulates reductive glutathione (GSH), and promotes glutathione peroxidase 4 (GPX4) expression, thereby inhibiting ferroptosis. Fluorescence imaging confirms effective renal accumulation of Cy5.5-labeled Fe@Ba nanozyme. In vivo experiments show the nanozyme reduces inflammation and significantly enhances survival rates in AKI models.
Discussion: This study validates the concept of self-assembling nanozymes for AKI treatment and offers new insights into nanomedicine applications. The Fe@Ba nanozyme's ability to counteract inflammation-related damage and inhibit ferroptosis through multiple mechanisms highlights its therapeutic potential. The successful integration of traditional Chinese medicine components with nanotechnology represents an innovative approach to addressing cisplatin-induced AKI, suggesting broader applications for metal-polyphenol nanozymes in oxidative stress-related kidney diseases.
Acute kidney injury (AKI) is a critical global health issue that rapidly impairs kidney function, often resulting in tubular cell death and inflammation (Li et al., 2023; Jiang et al., 2024; Liu et al., 2020). It is frequently associated with severe illnesses and can be intensified by conditions such as reduced blood flow, sepsis, low blood pressure, and the overuse of certain antibiotics and chemotherapy drugs, including cisplatin (DDP) (Tang et al., 2023; Li et al., 2021a; Zhang et al., 2021a; Li et al., 2021b). DDP-induced AKI is a major clinical complication, and its development is closely tied to the buildup of reactive oxygen species (ROS) (Li et al., 2021a; Dai et al., 2020), which overwhelms the body’s antioxidant defenses (Zhao et al., 2021; Meng et al., 2018; Zhang et al., 2021b). These ROS can damage essential cellular components like lipids (Zhu et al., 2024a; Liu et al., 2015), nucleic acids (Zhu et al., 2023a; Liu et al., 2021), and proteins (Pan et al., 2024; Huang et al., 2024), leading to kidney dysfunction. While treatments like N-acetylcysteine are employed to counteract ROS and mitigate cisplatin-induced AKI, they are swiftly cleared by the immune system (Magner et al., 2022). In addition, antioxidant enzymes such as catalase (CAT) and superoxide dismutase (SOD) are promising candidates for clinical treatment of ROS-induced diseases (Chen et al., 2023; Scholz et al., 2021). Consequently, exploring artificial enzyme systems to address the challenges of scavenging ROS generation, and inhibiting ferroptosis, ultimately preventing cisplatin-induced kidney damage remains a substantial challenge.
Nanozymes, nanomaterials that mimic the functions of enzymes, are gaining recognition as a viable substitute for natural enzymes (Zhang et al., 2022; Zhu et al., 2023b; Jiang et al., 2019; Huang et al., 2019). Their appeal stems from their affordability, customizable catalytic properties, and enhanced stability (Chen and Arnold, 2020; Peng et al., 2024; Zhu et al., 2022; Zhu et al., 2021). Nanozymes have emerged as promising alternatives to natural enzymes, effectively bridging the unique intersection of nanotechnology and biomedicine (Zhu et al., 2023c; Xu et al., 2024; Zhu et al., 2024b). Metal-polyphenol nanozymes have been widely exploited in recent years (Liang et al., 2024a; Liang et al., 2024b). Previous studies have reported that various nanozymes with CAT- and SOD-like activities have been proven effective in treating AKI by neutralizing harmful ROS, which aids in the recovery of kidney function (Wang et al., 2022; Zhang et al., 2021c). While the potential of nanozymes in treating cisplatin-induced AKI is encouraging, several challenges remain (Li et al., 2024). Firstly, their relatively low catalytic efficiency hinders their effectiveness in treating AKI. Secondly, the ongoing process of ROS scavenging by nanozymes is not as effective as needed. Consequently, it is crucial to develop new nanozymes that are highly catalytically efficient and can continuously eliminate ROS, to address the issue of AKI in cancer patients treated with cisplatin.
Herein, we have developed a metal-polyphenol nanozyme, Fe@Ba, which is formed by the interaction of ferric ions (Fe3+) and the antioxidant compound baicalein (Ba) from traditional Chinese medicine. The polyphenol contains phenolic hydroxyl groups, which have more lone pair electrons, while the trivalent iron ion has vacant orbitals. Therefore, the phenolic hydroxyl groups can effectively coordinate with the iron ions to form nanoparticles. Fe@Ba inhibits lipid peroxidation (LPO), a process that contributes to cell death known as ferroptosis, by neutralizing harmful ROS and activating the antioxidant properties of baicalein. Fe@Ba nanozyme mimics the functions of natural enzymes, CAT and SOD, to convert toxic superoxide anions (·O2−) into harmless oxygen, thereby reducing inflammation and preventing ferroptosis. It also increases the expression of glutathione peroxidase 4 (GPX4), a key enzyme in the antioxidant defense system, further inhibiting ferroptosis. Fluorescence imaging reveals that the cyanine 5.5 (Cy5.5)-labeled Fe@Ba nanozyme effectively accumulates in the kidneys. Our in vivo experiments have shown that Fe@Ba can alleviate inflammation and improve survival rates in an AKI model, demonstrating its therapeutic potential. This research provides a proof-of-concept for the development of self-assembling nanozymes and offers new insights into the use of nanomedicine for treating AKI, highlighting their potential to mitigate the negative effects of inflammation.
The synthesis of the Fe@Ba nanozyme was outlined in Scheme 1. We utilized a self-assembly method to combine Fe3+ ions with a metal-organic framework Ba in the presence of polyvinylpyrrolidone (PVP). As depicted in Figure 1A, the resulting Fe@Ba nanozyme, which was uniformly dispersed and approximately 50 nm in size, was directly visualized using transmission electron microscopy (TEM). The hydrodynamic size of the Fe@Ba nanozyme was measured with dynamic light scattering (DLS), as shown in Figure 1B. The zeta potential of Fe@Ba was −16.7 mV.
Scheme 1. Schematic representation of the application of Fe@Ba nanozyme to inhibit ferroptosis in a cisplatin-induced AKI model.
Figure 1. (A) TEM image of Fe@Ba nanozyme. (B) The DLS results of Fe@Ba nanozyme. (C) XPS spectrum of O 1s in Fe@Ba nanozyme. (D) High-resolution XPS spectrum of Fe 2p. (E) XRD spectrum of Fe@Ba nanozyme. (F) The UV-vis spectra of BDP and Fe@Ba nanozyme.
The high-angle annular dark-field scanning transmission electron microscopy (HAADF-STEM) images was employed to observed the element analysis (Supplementary Figure S1). Further analysis was conducted using X-ray photoelectron spectroscopy (XPS) to determine the oxidation states of iron and oxygen in the Fe@Ba nanozyme, with results presented in Figures 1D; Supplementary Figures S2, S3. The O 1s XPS spectrum revealed a peak at a binding energy of 531.4 eV, indicative of the presence of Fe-O bonds, as shown in Figure 1C. The high-resolution Fe 2p XPS spectrum split the Fe 2p peak into two components, corresponding to Fe3+ at 722.9 eV and Fe2+ at 710.1 eV, which are crucial for the nanozyme’s catalytic activity. The X-ray powder diffraction (XRD) spectrum indicated no distinct crystal pattern, suggesting that the iron in the Fe@Ba nanozyme had poor crystallinity, as shown in Figure 1E. Raman spectroscopy was employed to assess the degree of crystallinity, with results in Supplementary Figure S4. Ultraviolet-visible (UV-vis) spectroscopy confirmed the successful assembly of Ba and Fe3+, as illustrated in Figure 1F. Collectively, these findings confirmed the successful fabrication of the Fe@Ba nanozyme.
Motivated by the potential of multivalent iron elements, we investigated the catalytic capabilities of the Fe@Ba nanozyme. We assessed its catalase-like activity by measuring its ability to convert the highly toxic H2O2 into the non-toxic O2, which helps to reduce oxidative stress caused by ROS, as shown in Figure 2A. Furthermore, we determined SOD-like activity of Fe@Ba nanozyme using electron spin resonance (ESR) analysis with the spin-trap reagent 5-tert-butyloxycarbonyl-5-methyl-1-pyrroline N-oxide (BMPO). The distinctive quadrupling peak for BMPO-OOH confirmed the effectiveness of Fe@Ba nanozyme in scavenging superoxide anions, demonstrating the strong SOD mimetic activity, as depicted in Figure 2B. These results confirmed the effectiveness of Fe@Ba nanozyme in reducing ROS and alleviating oxidative stress. AKI is a significant side effect of DDP treatment, closely linked to the accumulation of ROS due to an overactive oxidation system and a compromised antioxidant defense. To evaluate the cellular protective effects of the Fe@Ba nanozyme, we used confocal laser scanning microscopy (CLSM) to observe the uptake of Cy5.5-labeled Fe@Ba nanozyme by DDP-induced cells over time. The increasing red fluorescence signal indicated that the nanozyme had a favorable cellular affinity and could enter mouse tubular epithelial cells (MTEC) cells to exert its therapeutic effects, as seen in Figures 2C, D; Supplementary Figure S5. A colocalization assay revealed that some Fe@Ba nanozyme could escape from lysosomes into the cytoplasm, where it scavenged ROS and exhibited antioxidant capabilities (Figure 2E). These findings indicated that Fe@Ba nanozyme can effectively be internalized by MTEC cells.
Figure 2. (A) The oxygen generation rate of H2O2 catalyzed by Fe@Ba nanozyme was assessed by dissolved oxygen analyzer. (B) ESR spectra of Fe@Ba nanozyme with DMPO as the spin trapper. (C) The CLSM images and (D) corresponding quantification of MTEC cells treated with Cy5.5-labeled Fe@Ba nanozyme. (E) The CLSM images revealed the colocalization of Cy5.5-labeled Fe@Ba nanozyme with the lysosomes of MTEC cells.
The protective impact of the Fe@Ba nanozyme on cells was measured using a cell counting kit 8 (CCK-8), which showed that the nanozyme did not hinder the growth of MTEC cells and was associated with strong antioxidant effects and reduction of ROS, as illustrated in Figure 3A. Importantly, the CCK-8 assay indicated that the Fe@Ba nanozyme significantly improved the MTEC cell viability in a concentration-dependent way, as shown in Figure 3B. The cytoprotective effect of the Fe@Ba nanozyme was also evaluated visually through flow cytometry analysis, with results displayed in Figure 3C; Supplementary Figure S6. These analyses showed that the Fe@Ba nanozyme-treated group had a notably higher cell survival rate compared to groups treated with free baicalein or cisplatin, aligning with the findings from the CCK-8 assay. Moreover, cellular ROS levels were quantified using a ROS probe, 2′,7′-dichlorofluorescin diacetate (DCFH-DA), as illustrated in Figures 3D, E. CLSM revealed that the Fe@Ba nanozyme had a greater capacity to reduce ROS than baicalein alone, attributed to its robust catalytic activities and antioxidant properties. These results confirm that the Fe@Ba nanozyme can effectively lower cellular ROS levels and enhance cell viability.
Figure 3. (A) Cell viability after a 24-h treatment with various concentrations of Fe@Ba nanozyme. (B) Cell viability after a 24-h treatment with various concentrations of Fe@Ba nanozyme in the presence of cisplatin. (C) Flow cytometry measurement of MTEC cells death rate following different treatments. (D) DCF fluorescence and (E) corresponding quantification of MTEC cells subjected to different treatments.
To understand how the Fe@Ba nanozyme can prevent ferroptosis in MTEC cells, we used a fluorescence probe, 5,5′,6,6′-tetrachloro-1,1′,3,3′-tetraethyl-imidacarbocyanine iodide (JC-1), to assess changes in mitochondrial membrane potential (MMP). The fluorescence of probe shifts from green to red as MMP decreases. CLSM images revealed a decrease in red fluorescence and an increase in green fluorescence in the DDP group, indicating MMP damage, as shown in Figure 4A. In contrast, the Fe@Ba nanozyme treatment resulted in a decrease in green fluorescence and an increase in red fluorescence, suggesting significant mitochondrial depolarization. Ferroptosis is marked by mitochondrial damage, and we used the fluorescent probe BODIPY C11581/591 to measure lipid peroxide levels, which change from red to green fluorescence. CLSM images indicated that the Fe@Ba nanozyme significantly reduced green fluorescence and increased red fluorescence, as depicted in Figure 4B, suggesting a reduction in lipid peroxides and thus inhibiting LPO. Furthermore, the Fe@Ba nanozyme was found to enhance glutathione (GSH) levels, which in turn increases the expression of GPX4, a key enzyme that inhibits ferroptosis. Figure 5A shows that the Fe@Ba nanozyme substantially increased GSH levels due to its excellent enzymatic activities and antioxidant capabilities. An immunofluorescence assay was used to measure GPX4 expression, and Figures 5B, C shows that the Fe@Ba nanozyme upregulated GPX4 expression, likely due to its catalytic activities in raising GSH levels. We also measured additional indicators of ferroptosis, malondialdehyde (MDA) and 4-hydroxynonenal (4-HNE), to confirm the inhibitory effect of the Fe@Ba nanozyme on ferroptosis. Figures 5D, E demonstrate that MTEC cells treated with the Fe@Ba nanozyme significantly reduced the levels of these harmful byproducts. These findings suggest that the Fe@Ba nanozyme can effectively neutralize ROS and protect mitochondria from depolarization, potentially offering antioxidant protection in an AKI model. This provides strong evidence for the potential of Fe@Ba nanozyme as an effective inhibitor of ferroptosis.
Figure 4. (A) Confocal images of MTEC cells stained with JC-1 kit following a 24-h treatment with different formulations. (B) Confocal images of MTEC cells stained with C11-BODIPY581/589 following different formulations.
Figure 5. (A) The cellular levels of GSH after different treatments were measured using a DTNB assay kit. (B) Confocal images (C) and the corresponding quantification (f) of GPX4 expression in MTEC cells after different formulations. (D) Quantitative analysis of MDA (E) and 4-HNE levels following different formulations.
The animal study protocol was approved by the Ethical Committee of Fujian Medical University (IACUC FJMU 2024-Y-0291). We used an IVIS imaging system to track the in vivo distribution of the Cy5.5-labeled Fe@Ba nanozyme. The imaging showed that the fluorescence signal at the kidney area increased over time, peaking 24 h after injection, which corresponds to ROS scavenging and ferroptosis inhibition effects of Fe@Ba nanozyme, as seen in Figures 6A, B. The mice were divided into four groups: PBS control, DDP treatment, DDP plus free Ba, and DDP plus Fe@Ba nanozyme. The results, as depicted in Figure 6C, indicated that the Fe@Ba nanozyme group had a significantly extended survival rate compared to the free Ba group, demonstrating the nanozyme’s superior antioxidant performance. We have measured the serum creatinine and blood urea nitrogen of different treatment groups in mice in vivo. As shown in Supplementary Figures SS7, S8, Fe@Ba nanozyme can significantly decrease the levels of serum creatinine, blood urea nitrogen, and inflammatory factor. To further confirm the antioxidant effect of Fe@Ba nanozyme, we performed terminal deoxynucleotidyl transferase-mediated dUTP nick-end labeling (TUNEL) staining on kidney sections. Figures 6D, E; Supplementary Figure S9 show that kidneys treated with the Fe@Ba nanozyme experienced less damage than those treated with free Ba, indicating a significant therapeutic benefit. Immunofluorescence staining confirmed the inhibition of kidney ferroptosis, with notable reductions in ROS and increases in GPX4 expression observed directly in the kidney, as shown in Figures 6F–I and S10-S11. In conclusion, the Fe@Ba nanozyme reduced lipid peroxidation, decreased intracellular oxidative stress, and enhanced GPX4-mediated protection against ferroptosis. Blood biochemistry analysis of mice and H&E staining further confirmed the antioxidant ability of Fe@Ba nanozyme, significantly reducing the levels of blood urea nitrogen (BUN), creatinine (CREA), and renal injury (Supplementary Figures S12, S13). These findings underscore the potential of Fe@Ba nanozyme as an effective inhibitor of ferroptosis for the treatment of AKI.
Figure 6. (A) Fluorescence images (B) corresponding quantification of cisplatin-induced AKI mice at different time points post-injection with Cy5.5-labeled Fe@Ba nanozyme. (C) Kaplan-Meier survival curves of mice following different treatments. (D) TUNEL staining of kidney slices and (E) the corresponding quantification from various groups following a 24-h treatment with different formulations. (F) ROS staining and (G) the corresponding quantification of kidney slices following various formulations. (H) Immunofluorescence staining and (I) the corresponding quantification of GPX4 in kidney slices following various formulations.
We successfully developed a metal-polyphenol self-assembling nanozyme as a ROS scavenger for treating cisplatin-induced AKI. The Fe@Ba nanozyme was synthesized through the interaction between Fe3+ and the antioxidant compound Ba, derived from traditional Chinese medicine. This nanozyme effectively inhibited LPO, a key driver of ferroptosis, by scavenging highly reactive ROS and enhancing antioxidant properties of Ba. Fe@Ba nanozyme exhibited CAT- and SOD-like activities to convert toxic ·O2− into harmless oxygen, thereby reducing inflammation and preventing ferroptosis. Additionally, Fe@Ba nanozyme upregulated the expression of GPX4, further inhibiting ferroptosis. Fluorescence imaging demonstrated that Cy5.5-labeled Fe@Ba nanozyme effectively accumulated in the kidneys. In vivo experiments confirmed that Fe@Ba nanozyme reduced inflammation and improved survival rates in an AKI model, showcasing its therapeutic potential. This research not only validated the concept of self-assembling nanozymes but also offered new insights into the use of nanomedicine for AKI treatment, emphasizing their ability to counteract inflammation-related damage.
The original contributions presented in the study are included in the article/Supplementary Material, further inquiries can be directed to the corresponding authors.
The animal study protocol was approved by the Ethical Committee of Fujian Medical University (IACUC FJMU 2024-Y-0291). The studies were conducted in accordance with the local legislation and institutional requirements. Written informed consent was obtained from the owners for the participation of their animals in this study.
YX: Writing – original draft, Writing – review and editing. HK: Conceptualization, Data curation, Formal Analysis, Investigation, Methodology, Project administration, Writing – original draft, Writing – review and editing. YR: Conceptualization, Writing – original draft, Writing – review and editing. XH: Data curation, Writing – original draft, Writing – review and editing. YZ: Supervision, Writing – original draft, Writing – review and editing. LW: Supervision, Writing – original draft, Writing – review and editing.
The author(s) declare that financial support was received for the research and/or publication of this article. This work was supported by the National Natural Science Foundation of China (82070727 to Lixin Wei), Joint Funds for the innovation of Science and Technology, Fujian province (2020Y9074 to Lixin Wei), and by Fujian provincial health technology project (2020QNA031 to Huimin Kang).
The authors declare that the research was conducted in the absence of any commercial or financial relationships that could be construed as a potential conflict of interest.
The author(s) declare that no Generative AI was used in the creation of this manuscript.
All claims expressed in this article are solely those of the authors and do not necessarily represent those of their affiliated organizations, or those of the publisher, the editors and the reviewers. Any product that may be evaluated in this article, or claim that may be made by its manufacturer, is not guaranteed or endorsed by the publisher.
The Supplementary Material for this article can be found online at: https://www.frontiersin.org/articles/10.3389/fphar.2025.1535969/full#supplementary-material
Chen, K., and Arnold, F. H. (2020). Engineering new catalytic activities in enzymes. Nat. Catal. 3 (3), 203–213. doi:10.1038/s41929-019-0385-5
Chen, Q., Nan, Y., Yang, Y., Xiao, Z., Liu, M., Huang, J., et al. (2023). Nanodrugs alleviate acute kidney injury: manipulate RONS at kidney. Bioact. Mater. 22, 141–167. doi:10.1016/j.bioactmat.2022.09.021
Dai, Y., Zhu, Y., Cheng, J., Shen, J., Huang, H., Liu, M., et al. (2020). Nitric oxide-releasing platinum(IV) prodrug efficiently inhibits proliferation and metastasis of cancer cells. Chem. Commun. 56 (90), 14051–14054. doi:10.1039/D0CC05422D
Huang, L., Chen, J., Gan, L., Wang, J., and Dong, S. (2019). Single-atom nanozymes. Sci. Adv. 5 (5), eaav5490. doi:10.1126/sciadv.aav5490
Huang, W., Tian, Y., Ma, J., Wei, P., Du, C., Zhang, X., et al. (2024). Neutrophil membrane-based biomimetic metal-polyphenol self-assembled nanozyme for the targeting treatment of early brain injury following subarachnoid hemorrhage. Chem. Eng. J. 498, 155643. doi:10.1016/j.cej.2024.155643
Jiang, D., Ni, D., Rosenkrans, Z. T., Huang, P., Yan, X., and Cai, W. (2019). Nanozyme: new horizons for responsive biomedical applications. Chem. Soc. Rev. 48 (14), 3683–3704. doi:10.1039/C8CS00718G
Jiang, W., Hou, X., Qi, Y., Wang, Z., Liu, Y., Gao, X. J., et al. (2024). pH-activatable pre-nanozyme mediated H2S delivery for endo-exogenous regulation of oxidative stress in acute kidney injury. Adv. Sci. 11 (18), 2303901. doi:10.1002/advs.202303901
Li, L., Liu, X., Liu, G., Xu, S., Hu, G., and Wang, L. (2024). Valence-engineered catalysis-selectivity regulation of molybdenum oxide nanozyme for acute kidney injury therapy and post-cure assessment. Nat. Commun. 15 (1), 8720. doi:10.1038/s41467-024-53047-1
Li, L., Shen, Y., Tang, Z., Yang, Y., Fu, Z., Ni, D., et al. (2023). Engineered nanodrug targeting oxidative stress for treatment of acute kidney injury. Exploration 3 (6), 20220148. doi:10.1002/EXP.20220148
Li, L., Zhu, Y., Liu, M., Jin, D., Zhang, L., Cheng, J., et al. (2021a). Conjugation of oxaliplatin with PEGylated-nanobody for enhancing tumor targeting and prolonging circulation. J. Inorg. Biochem. 223, 111553. doi:10.1016/j.jinorgbio.2021.111553
Li, L., Zhu, Y., Liu, M., Jin, D., Zhang, L., Cheng, J., et al. (2021b). Conjugation of oxaliplatin with PEGylated-nanobody for enhancing tumor targeting and prolonging circulation. J. Inorg. Biochem. 223, 111553. doi:10.1016/j.jinorgbio.2021.111553
Liang, X., Ding, L., Ma, J., Li, J., Cao, L., Liu, H., et al. (2024b). Enhanced mechanical strength and sustained drug release in carrier-free silver-coordinated anthraquinone natural antibacterial anti-inflammatory hydrogel for infectious wound healing. Adv. Healthc. Mater. 13 (23), 2400841. doi:10.1002/adhm.202400841
Liang, X., Liu, H., Chen, H., Peng, X., Li, Z., Teng, M., et al. (2024a). Rhein-based Pickering emulsion for hepatocellular carcinoma: shaping the metabolic signaling and immunoactivation in transarterial chemoembolization. Aggregate 5 (4), e552. doi:10.1002/agt2.552
Liu, D., Shu, G., Jin, F., Qi, J., Xu, X., Du, Y., et al. (2020). ROS-responsive chitosan-SS31 prodrug for AKI therapy via rapid distribution in the kidney and long-term retention in the renal tubule. Sci. Adv. 6 (41), eabb7422. doi:10.1126/sciadv.abb7422
Liu, L., Zhang, K., Sandoval, H., Yamamoto, S., Jaiswal, M., Sanz, E., et al. (2015). Glial lipid droplets and ROS induced by mitochondrial defects promote neurodegeneration. Cell 160 (1), 177–190. doi:10.1016/j.cell.2014.12.019
Liu, M., Zhu, Y., Jin, D., Li, L., Cheng, J., and Liu, Y. (2021). Hemin-caged ferritin acting as a peroxidase-like nanozyme for the selective detection of tumor cells. Inorg. Chem. 60 (19), 14515–14519. doi:10.1021/acs.inorgchem.1c01863
Magner, K., Ilin, J. V., Clark, E. G., Kong, J. W. Y., Davis, A., and Hiremath, S. (2022). Meta-analytic techniques to assess the association between N-acetylcysteine and acute kidney injury after contrast administration: a systematic review and meta-analysis. JAMA Netw. Open 5 (7), e2220671. doi:10.1001/jamanetworkopen.2022.20671
Meng, X.-M., Ren, G.-L., Gao, L., Yang, Q., Li, H.-D., Wu, W.-F., et al. (2018). NADPH oxidase 4 promotes cisplatin-induced acute kidney injury via ROS-mediated programmed cell death and inflammation. Lab. Investig. 98 (1), 63–78. doi:10.1038/labinvest.2017.120
Pan, Y., Cheng, J., Zhu, Y., Zhang, J., Fan, W., and Chen, X. (2024). Immunological nanomaterials to combat cancer metastasis. Chem. Soc. Rev. 53 (12), 6399–6444. doi:10.1039/D2CS00968D
Peng, C., Pang, R., Li, J., and Wang, E. (2024). Current advances on the single-atom nanozyme and its bioapplications. Adv. Mater. 36 (10), 2211724. doi:10.1002/adma.202211724
Scholz, H., Boivin, F. J., Schmidt-Ott, K. M., Bachmann, S., Eckardt, K.-U., Scholl, U. I., et al. (2021). Kidney physiology and susceptibility to acute kidney injury: implications for renoprotection. Nat. Rev. Nephrol. 17 (5), 335–349. doi:10.1038/s41581-021-00394-7
Tang, C., Livingston, M. J., Safirstein, R., and Dong, Z. (2023). Cisplatin nephrotoxicity: new insights and therapeutic implications. Nat. Rev. Nephrol. 19 (1), 53–72. doi:10.1038/s41581-022-00631-7
Wang, K., Zhang, Y., Mao, W., Feng, W., Lu, S., Wan, J., et al. (2022). Engineering ultrasmall ferroptosis-targeting and reactive oxygen/nitrogen species-scavenging nanozyme for alleviating acute kidney injury. Adv. Funct. Mater. 32 (10), 2109221. doi:10.1002/adfm.202109221
Xu, R., Zhang, S., Wang, P., Zhang, R., Lin, P., Wang, Y., et al. (2024). Nanozyme-based strategies for efficient theranostics of brain diseases. Coord. Chem. Rev. 501, 215519. doi:10.1016/j.ccr.2023.215519
Zhang, D.-Y., Liu, H., He, T., Younis, M. R., Tu, T., Yang, C., et al. (2021b). Biodegradable self-assembled ultrasmall nanodots as reactive oxygen/nitrogen species scavengers for theranostic application in acute kidney injury. Small 17 (8), 2005113. doi:10.1002/smll.202005113
Zhang, D.-Y., Liu, H., Younis, M. R., Lei, S., Yang, C., Lin, J., et al. (2021c). Ultrasmall platinum nanozymes as broad-spectrum antioxidants for theranostic application in acute kidney injury. Chem. Eng. J. 409, 127371. doi:10.1016/j.cej.2020.127371
Zhang, D.-Y., Younis, M. R., Liu, H., Lei, S., Wan, Y., Qu, J., et al. (2021a). Multi-enzyme mimetic ultrasmall iridium nanozymes as reactive oxygen/nitrogen species scavengers for acute kidney injury management. Biomaterials 271, 120706. doi:10.1016/j.biomaterials.2021.120706
Zhang, S., Li, Y., Sun, S., Liu, L., Mu, X., Liu, S., et al. (2022). Single-atom nanozymes catalytically surpassing naturally occurring enzymes as sustained stitching for brain trauma. Nat. Commun. 13 (1), 4744. doi:10.1038/s41467-022-32411-z
Zhao, M., Wang, Y., Li, L., Liu, S., Wang, C., Yuan, Y., et al. (2021). Mitochondrial ROS promote mitochondrial dysfunction and inflammation in ischemic acute kidney injury by disrupting TFAM-mediated mtDNA maintenance. Theranostics 11 (4), 1845–1863. doi:10.7150/thno.50905
Zhu, Y., Ding, C., Fang, W., Li, T., Yan, L., Tian, Y., et al. (2024b). Metal-polyphenol self-assembled nanodots for NIR-II fluorescence imaging-guided chemodynamic/photodynamic therapy-amplified ferroptosis. Acta Biomater. 185, 361–370. doi:10.1016/j.actbio.2024.07.017
Zhu, Y., Gong, P., Wang, J., Cheng, J., Wang, W., Cai, H., et al. (2023a). Amplification of lipid peroxidation by regulating cell membrane unsaturation to enhance chemodynamic therapy. Angew. Chem. Int. Ed. 62 (12), e202218407. doi:10.1002/anie.202218407
Zhu, Y., Jin, D., Liu, M., Dai, Y., Li, L., Zheng, X., et al. (2022). Oxygen self-supply engineering-ferritin for the relief of hypoxia in tumors and the enhancement of photodynamic therapy efficacy. Small. 2022 2022/04/01 18 (15), 2200116. doi:10.1002/smll.202200116
Zhu, Y., Liao, Y., Zou, J., Cheng, J., Pan, Y., Lin, L., et al. (2023c). Engineering single-atom nanozymes for catalytic biomedical applications. Small. 2023 2023/07/01 19 (30), 2300750. doi:10.1002/smll.202300750
Zhu, Y., Niu, X., Wu, T., Cheng, J., Zou, J., Pan, Y., et al. (2024a). Metal-phenolic nanocatalyst rewires metabolic vulnerability for catalytically amplified ferroptosis. Chem. Eng. J. 485, 150126. doi:10.1016/j.cej.2024.150126
Zhu, Y., Wang, W., Cheng, J., Qu, Y., Dai, Y., Liu, M., et al. (2021). Stimuli-Responsive manganese single-atom nanozyme for tumor therapy via integrated cascade reactions. Angew. Chem. Int. Ed. 60 (17), 9480–9488. doi:10.1002/anie.202017152
Keywords: nanozyme, acute kidney injury, ferroptosis, cisplatin, reactive oxygen species
Citation: Xiong Y, Kang H, Rao Y, Huang X, Zhu Y and Wei L (2025) Metal-phenolic nanozyme as a ferroptosis inhibitor for alleviating cisplatin-induced acute kidney injury. Front. Pharmacol. 16:1535969. doi: 10.3389/fphar.2025.1535969
Received: 28 November 2024; Accepted: 24 March 2025;
Published: 01 April 2025.
Edited by:
Patrice X. Petit, Centre National de la Recherche Scientifique (CNRS), FranceReviewed by:
Hongwei Cheng, University of Macau, ChinaCopyright © 2025 Xiong, Kang, Rao, Huang, Zhu and Wei. This is an open-access article distributed under the terms of the Creative Commons Attribution License (CC BY). The use, distribution or reproduction in other forums is permitted, provided the original author(s) and the copyright owner(s) are credited and that the original publication in this journal is cited, in accordance with accepted academic practice. No use, distribution or reproduction is permitted which does not comply with these terms.
*Correspondence: Yang Zhu, emh1eWFuZ0BtYWlsLnVzdGMuZWR1LmNu; Lixin Wei, bGl4aW53ZWk2NkBmam11LmVkdS5jbg==
†These authors have contributed equally to this work
Disclaimer: All claims expressed in this article are solely those of the authors and do not necessarily represent those of their affiliated organizations, or those of the publisher, the editors and the reviewers. Any product that may be evaluated in this article or claim that may be made by its manufacturer is not guaranteed or endorsed by the publisher.
Research integrity at Frontiers
Learn more about the work of our research integrity team to safeguard the quality of each article we publish.