- 1Department of Hepatopancreaticobiliary Surgery, China-Japan Union Hospital, Jilin University, Changchun, China
- 2Department of Clinical Laboratory, Second Hospital of Jilin University, Changchun, China
- 3Department of Endodontics, Hospital of Stomatology, Jilin University, Changchun, China
Metabolic dysfunction-associated steatotic liver disease (MASLD) has become the most prevalent chronic liver disease worldwide, but effective treatments are still lacking. Metabolic disorders such as iron overload, glycolysis, insulin resistance, lipid dysregulation, and glutaminolysis are found to induce liver senescence and ferroptosis, which are hot topics in the research of MASLD. Recent studies have shown that Hippo–YAP1/TAZ pathway is involved in the regulations of metabolism disorders, senescence, ferroptosis, inflammation, and fibrosis in MASLD, but their complex connections and contrast roles are also reported. In addition, therapeutics based on the Hippo–YAP1/TAZ pathway hold promising for MASLD treatment. In this review, we highlight the regulation and molecular mechanism of the Hippo–YAP1/TAZ pathway in MASLD and summarize potential therapeutic strategies for MASLD by regulating Hippo–YAP1/TAZ pathway.
1 Introduction
Metabolic dysfunction-associated steatotic liver disease (MASLD) is a metabolic disorder that can develop into metabolic dysfunction-associated steatohepatitis (MASH) which can lead to liver fibrosis, cirrhosis, and even cancer (Lazarus et al., 2022). High-fat obesity is the most prevalent cause of MASLD, affecting 20%–30% of the global population. Metabolic disorders such as iron overload, glycolysis, insulin resistance, lipid dysregulation, and glutaminolysis are the basis of MASLD (Chen et al., 2020). However, effective treatments for MASLD are still lacking, and underlying molecular mechanisms remain unclear.
The Hippo pathway is first discovered to regulate cell density and organ size through its downstream effectors, Yes-associated protein 1 (YAP1) and transcriptional coactivator with PDZ-binding motif (TAZ) (Russell and Camargo, 2022). Hippo-YAP1/TAZ pathway can regulate the inflammation of macrophages or the fibrogenic program of hepatocytes by binding to the TEA domain (TEAD) family (Mia and Manvendra, 2022). In addition, Hippo–YAP1/TAZ pathway has been recently found to regulate metabolic disorders, which induce senescence and ferroptosis, which are hot toptics in the research of MASLD (Nguyen-Lefebvre et al., 2021). Cellular senescence, a hallmark of aging, is characterized by a stable arrest of the cell cycle and a secretion of pro-inflammatory proteins known as senescence-associated secretory phenotypes (SASP), which promote liver fibrosis (López-Otín et al., 2013). Ferroptosis, a new manner of cell death characterized by iron-dependent phospholipid peroxidation, is also found to regulate cell death and inflammation in the pathological process of MASLD (Ogrodnik et al., 2019; Minamino et al., 2009). However, the research of Hippo–YAP1/TAZ pathway in MASLD are still in its infancy and their contrast roles on senescence, ferroptosis, inflammation, and fibrosis are also found.
In this review, we highlight the regulation and mechanism of the Hippo-YAP1/TAZ pathway in metabolism disorders, cellular senescence, ferroptosis, inflammation, and fibrosis on the progression of MASLD, which may offer new insights for developing effective treatment strategies for MASLD.
2 Hippo-YAP1/TAZ signaling pathway
Hippo signaling is first identified to regulate organ growth (Moya and Halder, 2019; Ma et al., 2015). Hippo signaling senses extracellular mechanical stimuli and triggers a kinase cascade, the mammalian sterile 20-like kinase 1/2 (MST1/2), and large tumor suppressor 1 and 2 (LATS1/2). MST1/2 phosphorylates its adaptor protein salvador 1 (SAV1) to facilitates its interaction and phosphorylation with LATS1/2. MST1/2 also phosphorylates MOB kinase activator 1A/B (MOB1A/B), enabling MOB1A/B to bind the auto-inhibitory region of LATS1/2 and promote full LATS1/2 activation (Moya and Halder, 2019; Ma et al., 2015; Hansen et al., 2015). The phosphorylation of LATS1/2 can further phosphorylate YAP1 and TAZ, leading to their retention in the cytoplasm and subsequent proteasomal degradation (Hansen et al., 2015). Inhibition of YAP1 and TAZ phosphorylation facilitate them to bind with TEAD as transcriptional coactivators, thus regulating various cellular behaviors (d’Angelo et al., 2019).
Mitogen-Activated Protein Kinase 4 (MAP4K) family, 4 Fat molecules (FAT1-4), and thousand and one kinases (TAOK1/2/3), have been found to phosphorylate LATS1/2 directly, or through activation of the MST1/2 kinases, respectively (Yu and Guan, 2013; Angus et al., 2012; Xiao et al., 2011; Boggiano et al., 2011; Poon et al., 2011; Meng et al., 2015; Zheng et al., 2015). In contrast, striatin-interacting phosphatase and kinase (STRIPAK) complex was found to inactivate MST1/2 (Zheng Y. et al., 2017; Bae et al., 2017), while G protein-coupled receptors (GPCRs) family was found to inhibit LATS1/2 activity, thus inhibiting the phosphorylation of YAP1/TAZ and promoting their nuclear translocation to activate transcription factors (Hansen et al., 2015; Feng et al., 2014; Yu et al., 2014; Cai et al., 2018; Zhao et al., 2012). Notably, many other post-translational modifications such as O-GlcNAcylation are also reported to affect YAP1 phosphorylation by interrupting the LATS1/2 interaction (Zhang et al., 2017a).
3 Pathological regulations of metabolic disorders on MASLD
Metabolic disorders including iron, glucose, and lipid dysregulations are the basis of MASLD. For example, the imbalance of iron homeostasis has been shown to correlate with obesity and the development of MASLD (Folgueras et al., 2018). Dietary iron intake correlated with the increased prevalence of MASLD in a dose-responsive manner (Alferink et al., 2019). Pathological iron overload can catalyze the generation of reactive oxygen species (ROS) through the Fenton reaction, contributing to chronic liver disease (Nakamura et al., 2019; Lunova et al., 2014). In addition, insulin resistance, enhanced glycolysis activity, increased lactic acid, and abnormal lipid accumulation are also found to increase the inflammatory cytokines, and cause liver damage and fibrosis in MASLD or MASH (Ye et al., 2016; Reyes-Gordillo et al., 2017).
Metabolic disorders have been found to induce senescence, which is a permanent cessation of the cell proliferation cycle, induced by internal or external factors (Kuilman et al., 2010). For example, palmitic acid (PA) can promote iron overload and induce senescence of hepatocytes (Qi et al., 2021). Senescent cells exhibit aberrant oxidative stress, excessive production of pro-inflammatory cytokines known as SASP, and mitochondrial dysfunction, which contribute to the progression of MASLD (Dabravolski et al., 2021). Inhibition of hepatocyte senescence could improve the pathological process of MASLD (Ogrodnik et al., 2017).
Metabolic disorders such as iron and lipid homeostasis impairments have been found to promote MASLD development by inducing ferroptosis, which is a new manner of cell death characterized by iron-dependent phospholipid peroxidation (Kuilman et al., 2010). Iron-export protein ferroportin 1 is downregulated, and iron-import proteins DMT1, transferrin receptor 1 (TfR1), and ferritin are upregulated in MASLD (Philpott, 2020; Mancias et al., 2014; Liu et al., 2022; Mitsuyoshi et al., 2009). Iron overload has been shown to exacerbate liver injury in a dose-responsive manner in a phase 2 trial of MASLD (Beaton et al., 2013). Similarly, a high-iron diet for 8 weeks can induce mouse liver ferroptosis and damage (Yu et al., 2020). Ferroptosis initiates cell death and inflammation at the onset of MASH by directly increasing the expression of prostaglandin endoperoxide synthase 2, which accelerates the metabolism of arachidonic acid and promotes inflammation (Tsurusaki et al., 2019). Inhibition of ferroptosis alleviates hepatic inflammatory responses and steatosis (Qi et al., 2020). Ferritinophagy is involved in regulating iron metabolism and ferroptosis (Lunova et al., 2014). Nuclear receptor coactivator 4 (NCOA4) is required for the delivery of ferritin to the lysosome via autophagosomes. Deferoxamine (DFO), an iron-chelating agent, inhibits lipid accumulation in the liver of MASH mice induced by ferroptosis inducer RSL3 (Shao and Chung, 2024).
4 Regulation of Hippo-YAP1/TAZ pathway in MASLD
Metabolites are also found to regulate the Hippo-YAP1/TAZ pathway. For example, elevated glucose levels enhance YAP1 activity by promoting O-GlcNAc modification or inhibiting adenosine monophosphate-activated kinase (AMPK) (Zhang et al., 2017b; Peng et al., 2017). In contrast, glucose starvation increased Hippo pathway phosphorylation and YAP1/TAZ cytoplasmic localization via activation of AMPK (Wang et al., 2015a). G-protein-coupled receptor (GPCR) signaling has also been discovered to control Hippo and YAP1/TAZ signaling (Yu et al., 2012). Glucagon or phospholipids such as lysophosphatidic acid can increase LATS1/2 activity and YAP1 phosphorylation by activating GPCR (Yu et al., 2013). Cholesterol-induced activation of TAZ is linked to lipid dysregulation and MASLD pathogenesis in mice (Wang et al., 2020). Mechanically, high-level cholesterol activates and stabilizes TAZ by triggering the inositol trisphosphate receptor (IP3R)-calcium-RhoA pathway in AML12 cells (Wang et al., 2020).
The Hippo-YAP1/TAZ pathway is also found to regulate metabolic disorders. Moreover, the Hippo-YAP1/TAZ pathway is involved in the regulation of senescence and ferroptosis, inflammation, and fibrosis caused by metabolic disorders in the MASLD (Sztolsztener et al., 2020).
4.1 Regulation of the Hippo-YAP1/TAZ pathway in metabolic disorders in MASLD
Hippo signaling has been reported to regulate metabolic abnormalities in MASLD (Minamino et al., 2009). For example, YAP1 can modulate iron concentration in MASLD patients by affecting TfR1 and ferritinophagy (Protchenko et al., 2021; Zhu et al., 2021a). NCOA4 is one of the specific mediators of ferritinophagy that degrade ferritin, which is critical for iron homeostasis (Philpott, 2020; Mancias et al., 2014). In addition, YAP1 is also found to suppress gluconeogenesis, a normal process in which the liver produces glucose in the fasted state (Hu et al., 2017). Mechanically, YAP1 represses the transcription of peroxisome proliferator-activated receptor gamma co-activator-1 alpha (Pgc1α), which plays an important role in gluconeogenesis (Hu et al., 2017). YAP1 can also drive glycolysis partially through the regulation of the glucose transporter glucose transporter 3 (GLUT3), which promotes glucose uptake (He et al., 2021). Moreover, YAP1 was found to induce glycolysis by up-regulating long noncoding RNA BCAR4, which was reported to increase the expression of hexokinase 2 (Zheng X. et al., 2017). Key metabolic enzyme AMPK regulates glucose metabolism by directly phosphorylating YAP1 (Wang et al., 2015b). Hippo-YAP1/TAZ signaling was also found to promote glucose metabolism by up-regulating insulin receptor substrate 2 (Irs2) and protein kinase B (Akt) (Wang C. et al., 2016). YAP1 can also activate Foxm1, which is a crucial regulator of insulin resistance and lipid metabolism and contributes to the progression of MASLD (Jeong et al., 2018). Moreover, Hippo signaling is involved in lipid metabolism by regulating the expression of MST1, which inhibits cholesterol accumulation by reducing constitutive sterol regulatory-element binding protein (SREBP) activity (Aylon et al., 2016; Geng et al., 2016). Consistently, SREBF2 promotes cholesterol and fatty acid synthesis by driving the expressions of HMGCR and FASN (Geng et al., 2016; Romani et al., 2019). Long non-coding RNA LncARSR is found to promote the accumulation of fat in the liver by targeting YAP1 in MASLD by potentiating IRS2/AKT activity. In addition, YAP1 is found to increase glutamine levels in zebrafish liver by directly promoting the expression of glula and glulb (Cox et al., 2016). Furthermore, YAP1 promotes glutaminolysis and myofibroblastic features in the hematopoietic stem cells (HSCs) of patients and mice with acute or chronic fibrosis (Du et al., 2018).
4.2 Regulation of the Hippo-YAP1/TAZ pathway in senescence and ferroptosis in MASLD
Hippo-YAP1/TAZ pathway is involved in the regulation of liver senescence. The YAP1 level is reduced and the iron level is increased in LO2 cells treated with PA. Over-expression of YAP1 can mitigate iron overload and alleviate hepatocyte senescence by inhibiting ferritinophagy (Qi et al., 2021). It is favored that over-expression of NCOA4 largely aggravates lipid droplet deposition and cellular senescence in PA-treated LO2 cells (Qi et al., 2021). LncRNA MAYA is also increased in PA-treated LO2 cells and high-fat diet (HFD)-induced hepatic steatosis in MASLD mice. Suppression of MAYA alleviates iron overload and cellular senescence by up-regulating YAP1 expression (Yuan et al., 2021). Selectively inhibition of YAP1 in HSCs induces senescence, but decreases liver injury and fibrosis by promoting ferroptosis resistance (Du et al., 2023).
The Hippo-YAP1/TAZ pathway can affect many essential ferroptosis pathways, such as the cystine/glutathione (GSH)/glutathione peroxidase 4 (GPX4) axis, the guanosine triphosphate cyclohydrolase 1 (GCH1)/tetrahydrobiopterin (BH4)/dihydrofolate reductase (DHFR) axis, and the ferroptosis suppressor protein 1 (FSP1)/coenzyme Q (CoQ) axis (Zheng and Conrad, 2020). Moreover, YAP1 increased the iron concentration in hepatocellular carcinoma cells (HCC) through transcriptional elevation of TfR1 via its O-GlcNAcylation (Zhu et al., 2021b). Downregulation of YAP1 partially reversed Fibroblast growth factor 21 (FGF21)-mediated ferroptosis of HCC (Xia et al., 2024). TAZ is also reported to mediate ferroptosis by indirectly regulating the expression of the ROS-generating nicotinamide adenine dinucleotide phosphate oxidass (NOX) in ovarian cancer cells (Yang et al., 2020). The p53/YAP1 axis is essential for lipid peroxidation and ferroptosis induced by cytoglobin, a heme-binding protein, that is critical for maintaining redox homeostasis within cells (Ye et al., 2021). However, YAP1/TAZ is also found to inhibit lipid peroxidation and ferroptosis by up-regulating the expressions of solute carrier family 7 member 11 (SLC7A11) and lipoxygenase 3 (Chalasani et al., 2012; Qin et al., 2021). Consistently, YAP1 knockdown aggravated liver injury and inflammation, as well as accelerated hepatocyte ferroptosis induced by cecal ligation and puncture (Wang et al., 2022). Increase in YAP1 nuclear translocation can inhibit ferroptosis of HSCs in carbon tetrachloride (CCl4)-induced mouse liver fibrosis model (Zhu et al., 2024).
Evidence regarding the role of YAP1/TAZ in senescence and ferroptosis within the liver remains controversial. Nevertheless, the regulatory mechanisms of YAP1/TAZ in senescence and ferroptosis have not been thoroughly in the context of the MASLD.
4.3 Regulation of the Hippo-YAP1/TAZ pathway in inflammation and fibrosis of MASLD
YAP1 activation was found to suppress the infiltration of macrophages and neutrophils and reduce the activation of HSCs and liver fibrosis (Liu et al., 2019). YAP1 was increased in macrophages derived from the livers of human cirrhotic patients or CCl4-induced mice (Feng et al., 2024). YAP1 acts as a coactivator for β-catenin, which regulates its target gene X-box binding protein 1, resulting in decreased NOD-like receptor protein 3/caspase-1 activity in macrophages isolated from mouse livers (Wu et al., 2021). It was favored that MST1 can promote reactive oxygen species (ROS)-induced pyroptosis accompanied by decreased YAP1 level in mouse pancreatic cancer model (Cui et al., 2019). MST1/2 depletion in liver macrophages enhanced liver inflammation and fibrosis accompanied by increased YAP1 level in MASLD (Zhang J. et al., 2024). Pharmacological inhibition of YAP1 in KCs has been shown to attenuate the expression of inflammatory cytokines and liver damage in NASH mice (Song et al., 2020). Mechanically, YAP and TAZ are found to promote the production of inflammatory cytokines in MASH mice by enhancing the secretion of Indian hedgehogs (Song et al., 2020; Wang X. et al., 2016). YAP1/TAZ is also reported to promote inflammation in metabolically stressed hepatocytes by up-regulating the expression of cysteine-rich angiogenic inducer 61 (CYR61) in mice (Mooring et al., 2020). The transcription factor Nrf2 mitigates YAP1-mediated inflammation by reducing reactive oxygen species (ROS) and MST1/2 phosphorylation (Wang et al., 2019). Notably, chronic inflammation also prolongs YAP1/TAZ activation in murine MASLD models and liver biopsies from patients with MASLD (Hyun et al., 2021). Pro-inflammatory cytokines including TNFα can directly modulate hepatocyte YAP1 activity in a dose-dependent manner (Zhao et al., 2020), suggesting that the interplay between YAP1/TAZ and inflammatory signaling is complex.
In addition, YAP1/TAZ can directly activate a fibrogenic program of HSCs via regulation of proliferation (Swiderska-Syn et al., 2016). YAP1-positive ductular liver cells were correlated with pro-fibrogenic factors (Tgfβ1, Ctgf, and phosphor-SMAD2) in MASH (Machado et al., 2015). Integrin β1 is required for activating pro-fibrotic hepatocytes by promoting YAP1 expression and its nuclear localization (Martin et al., 2016). The pharmacological blockade of YAP1 with verteporfin prevented collagen formation in cultured mouse HSCs (Martin et al., 2016). Omega-3 polyunsaturated fatty acids have been reported to inhibit the proliferation and activation of HSCs by degradating YAP1/TAZ (Zhang et al., 2016). It is favored that YAP1 promotes the proliferation of cardiac fibroblasts and their transdifferentiation to myofibroblasts in a murine dilated cardiomyopathy model (Jin et al., 2019). Notably, hepatocyte proliferation is generally beneficial for repopulating tissues and YAP1/TAZ is critical for liver regeneration by regulating neurofibromatosis-2 (NF2), which is a negative regulator of liver regeneration (Benhamouche et al., 2010). Inhibiting YAP1/TAZ rescues hepatocyte- and cholangiocyte-overgrowth phenotypes of Nf2-knockout mice (Benhamouche et al., 2010). Pharmacological inhibition or genetic deletion of MST1/2 enhances liver regeneration (Lu et al., 2018). Loss of integrin-linked kinase increased YAP1 expression and liver regeneration following partial hepatectomy (Apte et al., 2009). Precise consequences of YAP1/TAZ activity depend on the overall health of the liver micro-environment (Miyamura et al., 2017).
5 Treatment targeting Hippo-YAP1/TAZ pathway in MASLD
By focusing on the YAP1/TAZ pathway, new pharmacological treatments or MASLD might be found. Si-Ni-San is one of the representative formulas for treating patients with MASLD and is used to reduce lipid droplet deposition and YAP1 expression in the liver in mice (Wang et al., 2021). Lian-Mei-Yin, a traditional Chinese medicine formula, has been used for treating liver disorders by inhibiting YAP1-mediated Foxm1 activation, which is crucial for the occurrence and development of MASLD in zebrafish and mice (Zhang P. et al., 2024). Curcumol is an ingredient extracted from the volatile oil of a traditional Chinese herb zedoary turmeric and inhibits hepatocyte senescence by targeting YAP1/NCOA4 in MASLD (Sorrentino et al., 2014). Statins have been widely used to reduce cholesterol levels in patients with hypercholesterolemia and have recently been identified to inhibit YAP1/TAZ through Rho-mediated modulation of actin cytoskeleton, indicating that statins might be used for treating MASLD (Wang et al., 2015c). In addition, hepatocyte-specific N-acetylgalactosamine-conjugated siRNA targeting TAZ is sufficient to ameliorate HFD-induced liver fibrosis in mice (Liu-Chittenden et al., 2012). Furthermore, verteporfin, MYF-01–37, and XMU-MP-1 have been discovered to interact with YAP1 with TEAD, suggested that they might be used to treat MASLD in the future (Martin et al., 2016; Liu-Chittenden et al., 2012; Kurppa et al., 2020; Fan et al., 2016).
6 Conclusion and perspectives
In this review, we highlight the regulations and mechanisms of Hippo- YAP1/TAZ signaling in MASLD by focusing on metabolism, senescence, ferroptosis, inflammation, and fibrosis. Metabolic disorders such as iron overload, glycolysis, insulin resistance, lipid dysregulation, and glutaminolysis can induce senescence, ferroptosis, inflammation, and fibrosis to promote the development of MASLD via regulation of Hippo-YAP1/TAZ pathway (Figure 1). The drugs targeting Hippo-YAP1/TAZ signaling might be used to treat MASLD in the future. However, the interplay between YAP1/TAZ and inflammation or fibrosis is complex, precise consequences of YAP1/TAZ activity depend on the overall health of the liver micro-environment, which need further investigation.
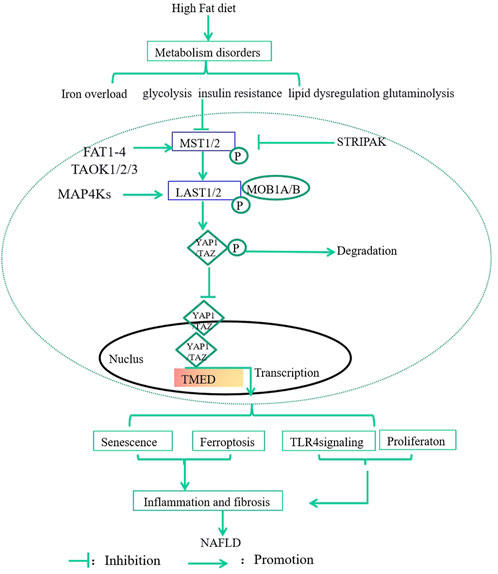
Figure 1. The effects and mechanisms of Hippo-YAP1/TAZ Pathway in MASLD. Metabolic disorders such as iron overload, glycolysis, insulin resistance, lipid dysregulation, and glutaminolysis can induce senescence, ferroptosis, inflammation, and fibrosis to promote the development of MASLD via regulation of Hippo-YAP1/TAZ Pathway. MST1/2, 20-like kinase 1/2; LATS1/2, large tumor suppressor 1 and 2; P, phosphorylation; MAP4K, Mitogen-Activated Protein Kinase 4; MOB1A/B, MOB kinase activator 1A/B; TAOK, thousand and one kinases; GPCRs, G protein-coupled receptors; STRIPAK, striatin-interacting phosphatase and kinase; FAT; Fat molecule.
Author contributions
WX: Writing–original draft. DS: Funding acquisition, Investigation, Resources, Writing–review and editing. JH: Formal Analysis, Writing–review and editing. XM: Conceptualization, Funding acquisition, Writing–review and editing.
Funding
The author(s) declare that financial support was received for the research, authorship, and/or publication of this article. This work was supported by the Science and Technology Project of Jilin Province Financial Department (jcsz2023481-18) and Jilin Provincial Natural Science Foundation (20200201601JC).
Conflict of interest
The authors declare that the research was conducted in the absence of any commercial or financial relationships that could be construed as a potential conflict of interest.
Generative AI statement
The author(s) declare that no Generative AI was used in the creation of this manuscript.
Publisher’s note
All claims expressed in this article are solely those of the authors and do not necessarily represent those of their affiliated organizations, or those of the publisher, the editors and the reviewers. Any product that may be evaluated in this article, or claim that may be made by its manufacturer, is not guaranteed or endorsed by the publisher.
References
Alferink, L. J. M., Kiefte-de Jong, J. C., Erler, N. S., Veldt, B. J., Schoufour, J. D., de Knegt, R. J., et al. (2019). Association of dietary macronutrient composition and non-alcoholic fatty liver disease in an ageing population: the Rotterdam Study. Gut 68 (6), 1088–1098. doi:10.1136/gutjnl-2017-315940
Angus, L., Moleirinho, S., Herron, L., Sinha, A., Zhang, X., Niestrata, M., et al. (2012). Willin/FRMD6 expression activates the Hippo signaling pathway kinases in mammals and antagonizes oncogenic YAP. Oncogene 31, 238–250. doi:10.1038/onc.2011.224
Apte, U., Gkretsi, V., Bowen, W. C., Mars, W. M., Luo, J.-H., Donthamsetty, S., et al. (2009). Enhanced liver regeneration following changes induced by hepatocyte-specific genetic ablation of integrin-linked kinase. Hepatology 50, 844–851. doi:10.1002/hep.23059
Aylon, Y., Gershoni, A., Rotkopf, R., Biton, I. E., Porat, Z., Koh, A. P., et al. (2016). The LATS2 tumor suppressor inhibits SREBP and suppresses hepatic cholesterol accumulation. Genes Dev. 30, 786–797. doi:10.1101/gad.274167.115
Bae, S. J., Ni, L., Osinski, A., Tomchick, D. R., Brautigam, C. A., and Luo, X. (2017). SAV1 promotes Hippo kinase activation through antagonizing the PP2A phosphatase STRIPAK. eLife 6, e30278. doi:10.7554/eLife.30278
Beaton, M., Chakrabarti, S., Levstik, M., Speechley, M., Marotta, P., and Adams, P. (2013). Phase II clinical trial of phlebotomy for non-alcoholic fatty liver disease. Aliment. Pharmacol. Ther. 37, 720–729. doi:10.1111/apt.12255
Benhamouche, S., Curto, M., Saotome, I., Gladden, A. B., Liu, C.-H., Giovannini, M., et al. (2010). Nf2/Merlin controls progenitor homeostasis and tumorigenesis in the liver. Genes Dev. 24, 1718–1730. doi:10.1101/gad.1938710
Boggiano, J. C., Vanderzalm, P. J., and Fehon, R. G. (2011). Tao-1 phosphorylates Hippo/MST kinases to regulate the Hippo-Salvador-Warts tumor suppressor pathway. Dev. Cell. 21, 888–895. doi:10.1016/j.devcel.2011.08.028
Cai, J., Song, X., Wang, W., Watnick, T., Pei, Y., Qian, F., et al. (2018). A RhoA-YAP-c-Myc signaling axis promotes the development of polycystic kidney disease. Genes Dev. 32, 781–793. doi:10.1101/gad.315127.118
Chalasani, N., Younossi, Z., Lavine, J. E., Diehl, A. M., Brunt, E. M., Cusi, K., et al. (2012). The diagnosis and management of non-alcoholic fatty liver disease: practice guideline by the American association for the study of liver diseases, American college of gastroenterology, and the American gastroenterological association. Hepatology 55 (6), 2005–2023. doi:10.1002/hep.25762
Chen, Z., Tian, R., She, Z., Cai, J., and Li, H. (2020). Role of oxidative stress in the pathogenesis of nonalcoholic fatty liver disease. Free Radic. Biol. Med. 152, 116–141. doi:10.1016/j.freeradbiomed.2020.02.025
Cox, A. G., Hwang, K. L., Brown, K. K., Evason, K., Beltz, S., Tsomides, A., et al. (2016). Yap reprograms glutamine metabolism to increase nucleotide biosynthesis and enable liver growth. Nat. Cell Biol. 18, 886–896. doi:10.1038/ncb3389
Cui, J., Zhou, Z., Yang, H., Jiao, F., Li, N., Gao, Y., et al. (2019). MST1 suppresses pancreatic cancer progression via ROS-induced pyroptosis. Mol. Cancer Res. 17 (6), 1316–1325. doi:10.1158/1541-7786.MCR-18-0910
Dabravolski, S. A., Bezsonov, E. E., and Orekhov, A. N. (2021). The role of mitochondria dysfunction and hepatic senescence in NAFLD development and progression. Biomed. Pharmacother. 142, 112041. doi:10.1016/j.biopha.2021.112041
d’Angelo, M., Benedetti, E., Tupone, M. G., Catanesi, M., Castelli, V., Antonosante, A., et al. (2019). The role of stiffness in cell reprogramming: a potential role for biomaterials in inducing tissue regeneration. Cells 8, 1036. doi:10.3390/cells8091036
Du, K., Hyun, J., Premont, R. T., Choi, S. S., Michelotti, G. A., Swiderska-Syn, M., et al. (2018). Hedgehog-YAP signaling pathway regulates glutaminolysis to control activation of hepatic stellate cells. Gastroenterology 154, 1465–1479. doi:10.1053/j.gastro.2017.12.022
Du, K., Maeso-Díaz, R., Oh, S. H., Wang, E., Chen, T., Pan, C., et al. (2023). Targeting YAP-mediated HSC death susceptibility and senescence for treatment of liver fibrosis. Hepatology 77 (6), 1998–2015. doi:10.1097/HEP.0000000000000326
Fan, F., He, Z., Kong, L. L., Chen, Q., Yuan, Q., Zhang, S., et al. (2016). Pharmacological targeting of kinases MST1 and MST2 augments tissue repair and regeneration. Sci. Transl. 8, 352ra108. doi:10.1126/scitranslmed.aaf2304
Feng, X., Degese, M. S., Iglesias-Bartolome, R., Vaque, J. P., Molinolo, A. A., Rodrigues, M., et al. (2014). Hippo-independent activation of YAP by the GNAQ uveal melanoma oncogene through a trio-regulated Rho GTPase signaling circuitry. Cancer Cell 25, 831–845. doi:10.1016/j.ccr.2014.04.016
Feng, X., Liu, H., Sheng, Y., Li, J., Guo, J., Song, W., et al. (2024). Yinchen gongying decoction mitigates CCl4-induced chronic liver injury and fibrosis in mice implicated in inhibition of the FoxO1/TGF-β1/Smad2/3 and YAP signaling pathways. J. Ethnopharmacol. 327, 117975. doi:10.1016/j.jep.2024.117975
Folgueras, A., Freitas-Rodríguez, S., Ramsay, A., Garabaya, C., Rodríguez, F., Velasco, G., et al. (2018). Matriptase-2 deficiency protects from obesity by modulating iron homeostasis. Nat. Commun. 9, 1350. doi:10.1038/s41467-018-03853-1
Geng, C., Zhang, Y., Gao, Y., Tao, W., Zhang, H., Liu, X., et al. (2016). Mst1 regulates hepatic lipid metabolism by inhibiting Sirt1 ubiquitination in mice. Biochem. Biophys. Res. Commun. 471, 444–449. doi:10.1016/j.bbrc.2016.02.059
Hansen, C. G., Moroishi, T., and Guan, K. L. (2015). YAP and TAZ: a nexus for Hippo signaling and beyond. Trends Cell Biol. 25, 499–513. doi:10.1016/j.tcb.2015.05.002
He, Z., Chen, D., Wu, J., Sui, C., Deng, X., Zhang, P., et al. (2021). Yes associated protein 1 promotes resistance to 5-fluorouracil in gastric cancer by regulating GLUT3-dependent glycometabolism reprogramming of tumor-associated macrophages. Arch. Biochem. Biophys. 702, 108838. doi:10.1016/j.abb.2021.108838
Hu, Y., Shin, D.-J., Pan, H., Lin, Z., Dreyfuss, J. M., Camargo, F. D., et al. (2017). YAP suppresses gluconeogenic gene expression through PGC1α. Hepatology 66, 2029–2041. doi:10.1002/hep.29373
Hyun, J., Al Abo, M., Dutta, R. K., Oh, S. H., Xiang, K., Zhou, X., et al. (2021). Dysregulation of the ESRP2-NF2-YAP/TAZ axis promotes hepatobiliary carcinogenesis in metabolic dysfunction-associated steatotic liver disease. J. Hepatol. 75 (3), 623–633. doi:10.1016/j.jhep.2021.04.033
Jeong, S. H., Kim, H. B., Kim, M. C., Lee, J. M., Lee, J.-H., Kim, J. H., et al. (2018). Hippo-mediated suppression of IRS2/AKT signaling prevents hepatic steatosis and liver cancer. J. Clin. Investig. 128, 1010–1025. doi:10.1172/JCI95802
Jin, B., Zhu, J., Shi, H.-M., Wen, Z.-C., and Wu, B.-W. (2019). YAP activation promotes the transdifferentiation of cardiac fibroblasts to myofibroblasts in matrix remodeling of dilated cardiomyopathy. Br. J. Med. Biol. Res. 52, e7914. doi:10.1590/1414-431X20187914
Kuilman, T., Michaloglou, C., Mooi, W. J., and Peeper, D. S. (2010). The essence of senescence. Dev. 24, 2463–2479. doi:10.1101/gad.1971610
Kurppa, K. J., Liu, Y., To, C., Zhang, T., Fan, M., Vajdi, A., et al. (2020). Treatment-induced tumor dormancy through YAP-mediated transcriptional reprogramming of the apoptotic pathway. Cancer Cell 37, 104–122. doi:10.1016/j.ccell.2019.12.006
Lazarus, J. V., Mark, H. E., Anstee, Q. M., Arab, J. P., Batterham, R. L., Castera, L., et al. (2022). Advancing the global public health agenda for NAFLD: a consensus statement. Nat. Rev. Gastroenterol. Hepatol. 19, 60–78. doi:10.1038/s41575-021-00523-4
Liu, C., Chen, Y., Zhang, Z., Xie, J., Yu, C., Xu, L., et al. (2022). Iron status and NAFLD among European populations: a bidirectional two-sample Mendelian randomization study. Nutrients 14, 5237. doi:10.3390/nu14245237
Liu, Y., Lu, T., Zhang, C., Xu, J., Xue, Z., Busuttil, R. W., et al. (2019). Activation of YAP attenuates hepatic damage and fibrosis in liver ischemia-reperfusion injury. J. Hepatol. 71, 719–730. doi:10.1016/j.jhep.2019.05.029
Liu-Chittenden, Y., Huang, B., Shim, J. S., Chen, Q., Lee, S.-J., Anders, R. A., et al. (2012). Genetic and pharmacological disruption of the TEAD-YAP complex suppresses the oncogenic activity of YAP. Genes Dev. 26, 1300–1305. doi:10.1101/gad.192856.112
López-Otín, C., Blasco, M. A., Partridge, L., Serrano, M., and Kroemer, G. (2013). The hallmarks of aging. Cell 153 (6), 1194–1217. doi:10.1016/j.cell.2013.05.039
Lu, L., Finegold, M. J., and Johnson, R. L. (2018). Hippo pathway coactivators Yap and Taz are required to coordinate mammalian liver regeneration. Exp. Mol. Med. 50, e423. doi:10.1038/emm.2017.205
Lunova, M., Goehring, C., Kuscuoglu, D., Mueller, K., Chen, Y., Walther, P., et al. (2014). Hepcidin knockout mice fed with iron-rich diet develop chronic liver injury and liver fibrosis due to lysosomal iron overload. J. Hepatol. 61, 633–641. doi:10.1016/j.jhep.2014.04.034
Ma, Y., Yang, Y., Wang, F., Wei, Q., and Qin, H. (2015). Hippo-YAP signaling pathway: a new paradigm for cancer therapy. Int. J. Cancer. 137, 2275–2286. doi:10.1002/ijc.29073
Machado, M. V., Michelotti, G. A., Pereira, T. A., Xie, G., Premont, R., Cortez-Pinto, H., et al. (2015). Accumulation of duct cells with activated YAP parallels fibrosis progression in non-alcoholic fatty liver disease. J. Hepatol. 63 (4), 962–970. doi:10.1016/j.jhep.2015.05.031
Mancias, J. D., Wang, X., Gygi, S. P., Harper, J. W., and Kimmelman, A. C. (2014). Quantitative proteomics identifies NCOA4 as the cargo receptor mediating ferritinophagy. Nature 509, 105–109. doi:10.1038/nature13148
Martin, K., Pritchett, J., Llewellyn, J., Mullan, A. F., Athwal, V. S., Dobie, R., et al. (2016). PAK proteins and YAP-1 signalling downstream of integrin beta-1 in myofibroblasts promote liver fibrosis. Nat. Commun. 7, 12502. doi:10.1038/ncomms12502
Meng, Z., Moroishi, T., Mottier-Pavie, V., Plouffe, S. W., Hansen, C. G., Hong, A. W., et al. (2015). MAP4K family kinases act in parallel to MST1/2 to activate LATS1/2 in the Hippo pathway. Nat. Commun. 6, 8357. doi:10.1038/ncomms9357
Mia, M. M., and Manvendra, K. S. (2022). New insights into Hippo/YAP signaling in fibrotic diseases. Cells 11 (13), 2065. doi:10.3390/cells11132065
Minamino, T., Orimo, M., Shimizu, I., Kunieda, T., Yokoyama, M., Ito, T., et al. (2009). A crucial role for adipose tissue p53 in the regulation of insulin resistance. Nat. Med. 15 (9), 1082–1087. doi:10.1038/nm.2014
Mitsuyoshi, H., Yasui, K., Harano, Y., Endo, M., Tsuji, K., Minami, M., et al. (2009). Analysis of hepatic genes involved in the metabolism of fatty acids and iron in nonalcoholic fatty liver disease. Hepatol. Res. 39, 366–373. doi:10.1111/j.1872-034X.2008.00464.x
Miyamura, N., Hata, S., Itoh, T., Tanaka, M., Nishio, M., Itoh, M., et al. (2017). Erratum: YAP determines the cell fate of injured mouse hepatocytes in vivo. Nat. Commun. 8, 16146. doi:10.1038/ncomms16146
Mooring, M., Fowl, B. H., Lum, S. Z. C., Liu, Y., Yao, K., Softic, S., et al. (2020). Hepatocyte stress increases expression of yes-associated protein and transcriptional coactivator with PDZ-binding motif in hepatocytes to promote parenchymal inflammation and fibrosis. Hepatology 71 (5), 1813–1830. doi:10.1002/hep.30928
Moya, I. M., and Halder, G. (2019). Hippo-YAP/TAZ signalling in organ regeneration and regenerative medicine. Nat. Rev. Mol. Cell Biol. 20, 211–226. doi:10.1038/s41580-018-0086-y
Nakamura, T., Naguro, I., and Ichijo, H. (2019). Iron homeostasis and iron-regulated ROS in cell death, senescence and human diseases. Biochim. Biophys. Acta Gen. Subj. 1863, 1398–1409. doi:10.1016/j.bbagen.2019.06.010
Nguyen-Lefebvre, A. T., Selzner, N., Wrana, J. L., and Bhat, M. (2021). The Hippo pathway: a master regulator of liver metabolism, regeneration, and disease. FASEB J. 35 (5), e21570. doi:10.1096/fj.202002284RR
Ogrodnik, M., Miwa, S., Tchkonia, T., Tiniakos, D., Wilson, C. L., Lahat, A., et al. (2017). Cellular senescence drives age-dependent hepatic steatosis. Nat. Commun. 8, 15691. doi:10.1038/ncomms15691
Ogrodnik, M., Salmonowicz, H., and Gladyshev, V. N. (2019). Integrating cellular senescence with the concept of damage accumulation in aging: relevance for clearance of senescent cells. Aging Cell 18 (1), e12841. doi:10.1111/acel.12841
Peng, C., Zhu, Y., Zhang, W., Liao, Q., Chen, Y., Zhao, X., et al. (2017). Regulation of the Hippo-YAP pathway by glucose sensor O-GlcNAcylation. Mol. Cell 68, 591–604. doi:10.1016/j.molcel.2017.10.010
Philpott, C. C. (2020). Iron on the move: mobilizing liver iron via NCOA4. Blood 136, 2604–2605. doi:10.1182/blood.2020007971
Poon, C. L., Lin, J. I., Zhang, X., and Harvey, K. F. (2011). The sterile 20-like kinase Tao-1 controls tissue growth by regulating the Salvador-Warts-Hippo pathway. Dev. Cell. 21, 896–906. doi:10.1016/j.devcel.2011.09.012
Protchenko, O., Baratz, E., Jadhav, S., Li, F., Shakoury-Elizeh, M., Gavrilova, O., et al. (2021). Iron chaperone poly rC binding protein 1 protects mouse liver from lipid peroxidation and steatosis. Hepatology 73, 1176–1193. doi:10.1002/hep.31328
Qi, J., Kim, J., Zhou, Z., Lim, C., and Kim, B. (2020). Ferroptosis affects the progression of nonalcoholic steatohepatitis via the modulation of lipid peroxidation-mediated cell death in mice. Am. J. Pathol. 190, 68–81. doi:10.1016/j.ajpath.2019.09.011
Qi, X., Song, A., Ma, M., Wang, P., Zhang, X., Lu, C., et al. (2021). Curcumol inhibits ferritinophagy to restrain hepatocyte senescence through YAP/NCOA4 in non-alcoholic fatty liver disease. Cell Prolif. 54 (9), e13107. doi:10.1111/cpr.13107
Qin, Y., Pei, Z., Feng, Z., Lin, P., Wang, S., Li, Y., et al. (2021). Oncogenic activation of YAP signaling sensitizes ferroptosis of hepatocellular carcinoma via ALOXE3-mediated lipid peroxidation accumulation. Front. Cell Dev. Biol. 9, 751593. doi:10.3389/fcell.2021.751593
Reyes-Gordillo, K., Shah, R., and Muriel, P. (2017). Oxidative stress and inflammation in hepatic diseases: current and future therapy. Oxid. Med. Cell Longev. 2017, 3140673. doi:10.1155/2017/3140673
Romani, P., Brian, I., Santinon, G., Pocaterra, A., Audano, M., Pedretti, S., et al. (2019). Extracellular matrix mechanical cues regulate lipid metabolism through Lipin-1 and SREBP. Nat. Cell Biol. 21, 338–347. doi:10.1038/s41556-018-0270-5
Russell, J. O., and Camargo, F. D. (2022). Hippo signalling in the liver: role in development, regeneration and disease. Nat. Rev. Gastroenterol. Hepatol. 19 (5), 297–312. doi:10.1038/s41575-021-00571-w
Shao, T., and Chung, R. T. (2024). Ironing out MAFLD: therapeutic targeting of liver ferroptosis. Cell Metab. 36 (10), 2167–2169. doi:10.1016/j.cmet.2024.09.005
Song, K., Kwon, H., Han, C., Chen, W., Zhang, J., Ma, W., et al. (2020). Yes-associated protein in Kupffer cells enhances the production of proinflammatory cytokines and promotes the development of nonalcoholic steatohepatitis. Hepatology 72, 72–87. doi:10.1002/hep.30990
Sorrentino, G., Ruggeri, N., Specchia, V., Cordenonsi, M., Mano, M., Dupont, S., et al. (2014). Metabolic control of YAP and TAZ by the mevalonate pathway. Nat. Cell Biol. 16, 357–366. doi:10.1038/ncb2936
Swiderska-Syn, M., Xie, G., Michelotti, G. A., Jewell, M. L., Premont, R. T., Syn, W. K., et al. (2016). Hedgehog regulates yes-associated protein 1 in regenerating mouse liver. Hepatology 64, 232–244. doi:10.1002/hep.28542
Sztolsztener, K., Chabowski, A., Ewa Harasim-Symbor, E., Bielawiec, P., and Konstantynowicz-Nowicka, K. (2020). Arachidonic acid as an early indicator of inflammation during non-alcoholic fatty liver disease development. Biomolecules 10 (8), 1133. doi:10.3390/biom10081133
Tsurusaki, S., Tsuchiya, Y., Koumura, T., Nakasone, M., Sakamoto, T., Matsuoka, M., et al. (2019). Hepatic ferroptosis plays an important role as the trigger for initiating inflammation in nonalcoholic steatohepatitis. Cell Death Dis. 10 (6), 449. doi:10.1038/s41419-019-1678-y
Wang, C., Jeong, K., Jiang, H., Guo, W., Gu, C., Lu, Y., et al. (2016a). YAP/TAZ regulates the insulin signaling via IRS1/2 in endometrial cancer. Am. J. cancer Res. 6 (5), 996–1010.
Wang, J., Zhu, Q., Li, R., Zhang, J., Ye, X., and Li, X. (2022). YAP1 protects against septic liver injury via ferroptosis resistance. Cell Biosci. 12 (1), 163. doi:10.1186/s13578-022-00902-7
Wang, P., Geng, J., Gao, J., Zhao, H., Li, J., Shi, Y., et al. (2019). Macrophage achieves self-protection against oxidative stress-induced ageing through the Mst-Nrf2 axis. Nat. Commun. 10 (1), 755. doi:10.1038/s41467-019-08680-6
Wang, S., Tang, C., Zhao, H., Shen, P., Lin, C., Zhu, Y., et al. (2021). Network pharmacological Analysis and experimental validation of the mechanisms of action of Si-Ni-san against liver fibrosis. Front. Pharmacol. 12, 656115. doi:10.3389/fphar.2021.656115
Wang, W., Li, N., Li, X., Tran, M. K., Han, X., and Chen, J. (2015c). Tankyrase inhibitors target YAP by stabilizing angiomotin family proteins. Cell Rep. 13, 524–532. doi:10.1016/j.celrep.2015.09.014
Wang, W., Xiao, Z. D., Li, X., Aziz, K. E., Gan, B., Johnson, R. L., et al. (2015b). AMPK modulates Hippo pathway activity to regulate energy homeostasis. Nat. Cell Biol. 17 (4), 490–499. doi:10.1038/ncb3113
Wang, W., Xiao, Z. D., Li, X., Aziz, K. E., Gan, B., Johnson, R. L., et al. (2015a). AMPK modulates Hippo pathway activity to regulate energy homeostasis. Nat. Cell Biol. 17, 490–499. doi:10.1038/ncb3113
Wang, X., Cai, B., Yang, X., Sonubi, O. O., Zheng, Z., Ramakrishnan, R., et al. (2020). Cholesterol stabilizes TAZ in hepatocytes to promote experimental non-alcoholic steatohepatitis. Cell Metab. 31, (969–986. doi:10.1016/j.cmet.2020.03.010
Wang, X., Zheng, Z. E., Caviglia, J. M., Corey, K. E., Herfel, T. M., Cai, B., et al. (2016b). Hepatocyte TAZ/WWTR1 promotes inflammation and fibrosis in nonalcoholic steatohepatitis. Cell metab. 24 (6), 848–862. doi:10.1016/j.cmet.2016.09.016
Wu, Q., Guo, J., Liu, Y., Zheng, Q., Li, X., Wu, C., et al. (2021). YAP drives fate conversion and chemoresistance of small cell lung cancer. Sci. Adv. 7 (40), eabg1850. doi:10.1126/sciadv.abg1850
Xia, J., Fu, B., Wang, Z., Wen, G., Gu, Q., Chen, D., et al. (2024). MVP enhances FGF21-induced ferroptosis in hepatocellular carcinoma by increasing lipid peroxidation through regulation of NOX4. Clin. Transl. Sci. 17 (8), e13910. doi:10.1111/cts.13910
Xiao, L., Chen, Y., Ji, M., and Dong, J. (2011). KIBRA regulates Hippo signaling activity via interactions with large tumor suppressor kinases. J. Biol. Chem. 286, 7788–7796. doi:10.1074/jbc.M110.173468
Yang, W. H., Huang, Z., Wu, J., Ding, C. C., Murphy, S. K., and Chi, J. T. (2020). A TAZ-ANGPTL4-NOX2 Axis regulates ferroptotic cell death and chemoresistance in epithelial ovarian cancer. Mol. Cancer Res. 18 (1), 79–90. doi:10.1158/1541-7786.MCR-19-0691
Ye, J. H., Chao, J., Chang, M. L., Peng, W. H., Cheng, H. Y., Liao, J. W., et al. (2016). Pentoxifylline ameliorates non-alcoholic fatty liver disease in hyperglycaemic and dyslipidaemic mice by upregulating fatty acid β-oxidation. Sci. Rep. 6 (33102), 33102. doi:10.1038/srep33102
Ye, S., Xu, M., Zhu, T., Chen, J., Shi, S., Jiang, H., et al. (2021). Cytoglobin promotes sensitivity to ferroptosis by regulating p53-YAP1 axis in colon cancer cells. J. Cell Mol. Med. 25 (7), 3300–3311. doi:10.1111/jcmm.16400
Yu, F. X., and Guan, K. L. (2013). The Hippo pathway: regulators and regulations. Genes Dev. 27, 355–371. doi:10.1101/gad.210773.112
Yu, F.-X., Luo, J., Mo, J.-S., Liu, G., Kim, Y. C., Meng, Z., et al. (2014). Mutant Gq/11 promote uveal melanoma tumorigenesis by activating YAP. Cancer Cell 25, 822–830. doi:10.1016/j.ccr.2014.04.017
Yu, F.-X., Zhang, Y., Park, H. W., Jewell, J. L., Chen, Q., Deng, Y., et al. (2013). Protein kinase A activates the Hippo pathway to modulate cell proliferation and differentiation. Genes Dev. 27, 1223–1232. doi:10.1101/gad.219402.113
Yu, F.-X., Zhao, B., Panupinthu, N., Jewell, J. L., Lian, I., Wang, L. H., et al. (2012). Regulation of the Hippo-YAP pathway by G-protein-coupled receptor signaling. Cell 150, 780–791. doi:10.1016/j.cell.2012.06.037
Yu, Y., Jiang, Li, Wang, H., Shen, Z., Qi, C., Zhang, P., et al. (2020). Hepatic transferrin plays a role in systemic iron homeostasis and liver ferroptosis. Blood, J. Am. Soc. Hematol. 136 (6), 726–739. doi:10.1182/blood.2019002907
Yuan, P., Qi, X., Song, A., Ma, M., Zhang, X., Lu, C., et al. (2021). LncRNA MAYA promotes iron overload and hepatocyte senescence through inhibition of YAP in non-alcoholic fatty liver disease. J. Cell. Mol. Med. 25 (15), 7354–7366. doi:10.1111/jcmm.16764
Zhang, J., Chen, W., Song, K., Song, K., Kolls, J., and Wu, T. (2024a). YAP activation in liver macrophages via depletion of MST1/MST2 enhances liver inflammation and fibrosis in MASLD. FASEB J. 38 (17), e70026. doi:10.1096/fj.202400813RR
Zhang, K., Chang, Y., Shi, Z., Han, X., Han, Y., Yao, Q., et al. (2016). ω-3 PUFAs ameliorate liver fibrosis and inhibit hepatic stellate cells proliferation and activation by promoting YAP/TAZ degradation. Sci. Rep. 6, 30029. doi:10.1038/srep30029
Zhang, P., Cao, J., Liang, X., Su, Z., Zhang, B., Wang, Z., et al. (2024b). Lian-Mei-Yin formula alleviates diet-induced hepatic steatosis by suppressing Yap1/FOXM1 pathway-dependent lipid synthesis. Acta Biochim. Biophys. Sin. (Shanghai) 56 (4), 621–633. doi:10.3724/abbs.2024025
Zhang, X., Qiao, Y., Wu, Q., Chen, Y., Zou, S., Liu, X., et al. (2017a). The essential role of YAP O-GlcNAcylation in high-glucose-stimulated liver tumorigenesis. Nat. Commun. 8, 15280. doi:10.1038/ncomms15280
Zhang, X., Qiao, Y., Wu, Q., Chen, Y., Zou, S., Liu, X., et al. (2017b). The essential role of YAP O-GlcNAcylation in high-glucose-stimulated liver tumorigenesis. Nat. Commun. 8, 15280. doi:10.1038/ncomms15280
Zhao, B., Li, L., Wang, L., Wang, C.-Y., Yu, J., and Guan, K.-L. (2012). Cell detachment activates the Hippo pathway via cytoskeleton reorganization to induce anoikis. Genes Dev. 26, 54–68. doi:10.1101/gad.173435.111
Zhao, S., Jiang, J., Jing, Y., Liu, W., Yang, X., Hou, X., et al. (2020). The concentration of tumor necrosis factor-α determines its protective or damaging effect on liver injury by regulating Yap activity. Cell Death Dis. 11, 70. doi:10.1038/s41419-020-2264-z
Zheng, J., and Conrad, M. (2020). The metabolic underpinnings of ferroptosis. Cell Metab. 32, 920–937. doi:10.1016/j.cmet.2020.10.011
Zheng, X., Han, H., Liu, G.-P., Ma, Y.-X., Pan, R.-L., Sang, L. J., et al. (2017b). LncRNA wires up Hippo and Hedgehog signaling to reprogramme glucose metabolism. EMBO J. 36, 3325–3335. doi:10.15252/embj.201797609
Zheng, Y., Liu, B., Wang, L., Lei, H., Pulgar Prieto, K. D., and Pan, D. (2017a). Homeostatic control of Hpo/MST kinase activity through autophosphorylation-dependent recruitment of the STRIPAK PP2A phosphatase complex. Cell Rep. 21, 3612–3623. doi:10.1016/j.celrep.2017.11.076
Zheng, Y., Wang, W., Liu, B., Deng, H., Uster, E., and Pan, D. (2015). Identification of Happyhour/MAP4K as alternative Hpo/Mst-like kinases in the Hippo kinase cascade. Dev. Cell. 34, 642–655. doi:10.1016/j.devcel.2015.08.014
Zhu, G., Murshed, A., Li, H., Ma, J., Zhen, N., Ding, M., et al. (2021a). O-GlcNAcylation enhances sensitivity to RSL3-induced ferroptosis via the YAP/TFRC pathway in liver cancer. Cell Death Discov. 7 (1), 83. doi:10.1038/s41420-021-00468-2
Zhu, G., Murshed, A., Li, H., Ma, J., Zhen, N., Ding, M., et al. (2021b). O-GlcNAcylation enhances sensitivity to RSL3-induced ferroptosis via the YAP/TFRC pathway in liver cancer. Cell Death Discov. 7 (1), 83. doi:10.1038/s41420-021-00468-2
Keywords: Hippo-YAP1/TAZ pathway, metabolism, senescence, ferroptosis, inflammation, fibrosis, MASLD
Citation: Xuan W, Song D, Hou J and Meng X (2025) Regulation of Hippo-YAP1/TAZ pathway in metabolic dysfunction-associated steatotic liver disease. Front. Pharmacol. 16:1505117. doi: 10.3389/fphar.2025.1505117
Received: 02 October 2024; Accepted: 07 January 2025;
Published: 23 January 2025.
Edited by:
Anna Maria Giudetti, University of Salento, ItalyReviewed by:
Vicente Sánchez-Valle, National Polytechnic Institute of Mexico (CINVESTAV), MexicoFeng Zhang, Nanjing University of Chinese Medicine, China
Copyright © 2025 Xuan, Song, Hou and Meng. This is an open-access article distributed under the terms of the Creative Commons Attribution License (CC BY). The use, distribution or reproduction in other forums is permitted, provided the original author(s) and the copyright owner(s) are credited and that the original publication in this journal is cited, in accordance with accepted academic practice. No use, distribution or reproduction is permitted which does not comply with these terms.
*Correspondence: Xiuping Meng, bWVuZ3hwQGpsdS5lZHUuY24=