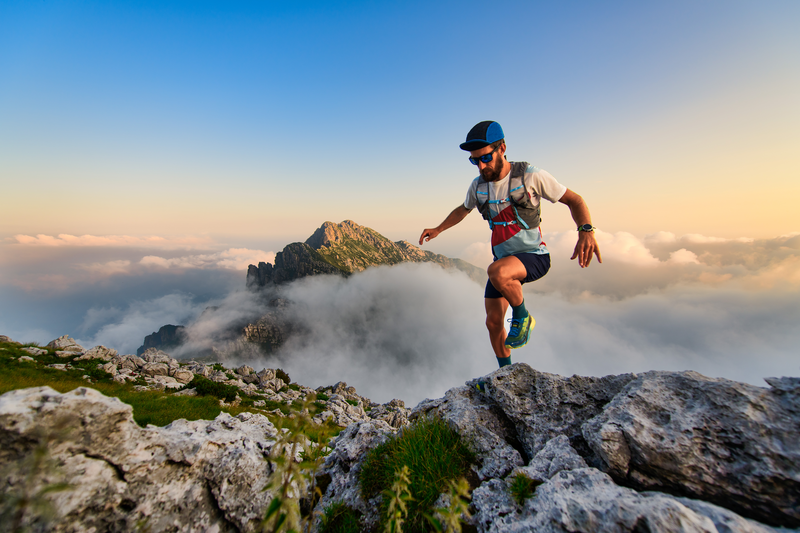
95% of researchers rate our articles as excellent or good
Learn more about the work of our research integrity team to safeguard the quality of each article we publish.
Find out more
REVIEW article
Front. Pharmacol. , 06 December 2024
Sec. Inflammation Pharmacology
Volume 15 - 2024 | https://doi.org/10.3389/fphar.2024.1509172
As a mechanism of cell death, ferroptosis has gained popularity since 2012. The process is distinguished by iron toxicity and phospholipid accumulation, in contrast to autophagy, apoptosis, and other cell death mechanisms. It is implicated in the advancement of multiple diseases across the body. Researchers currently know that osteosarcoma, osteoporosis, and other orthopedic disorders are caused by NRF2, GPX4, and other ferroptosis star proteins. The effective relief of osteoarthritis symptoms from deterioration has been confirmed by clinical treatment with multiple ferroptosis inhibitors. At the same time, it should be reminded that the mechanisms involved in ferroptosis that regulate orthopedic diseases are not currently understood. In this manuscript, we present the discovery process of ferroptosis, the mechanisms involved in ferroptosis, and the role of ferroptosis in a variety of orthopedic diseases. We expect that this manuscript can provide a new perspective on clinical diagnosis and treatment of related diseases.
Cell death is a crucial mechanism by which organisms maintain homeostasis and equilibrium in their systems. Currently, the predominant focus of research is on programmed cell death (PCD). The content includes autophagy, apoptosis, and various other cell death mechanisms (Fuchs and Steller, 2015; Bertheloot et al., 2021). PCD is controlled by multiple factors, including genetics, signaling pathways, and the cellular environment (Jacobson et al., 1997). These factors influence the development and outcome of systemic immunity, biochemistry, and disease (Su et al., 2015; Tower, 2015). Ferroptosis is distinct from more conventional forms of cell death since it relies on lipid peroxidation and intracellular iron buildup as its primary triggers (Chen F. et al., 2024). The extensive inquiry into PCD has been prompted by these two characteristics. There are noticeable necrotic alterations and morphological differences between ferroptosis and other forms of cell death (Lei et al., 2022). Additionally, the most notable physical features are alterations to the mitochondrial architecture, including a decreased body size, increased density, reduced or absent cristae, and damaged outer membranes (Dixon et al., 2012; Stockwell et al., 2017).
Currently, the majority of studies on ferroptosis mortality mostly investigate its association with cancer, neurological disorders and blood-related conditions (Yan et al., 2021). They are closely associated with lipid metabolism, reactive oxygen species (ROS) and hypoxia, and iron metabolism, all of which involve metabolic pathways related to ferroptosis (Deng et al., 2024; Qiu et al., 2020). Musculoskeletal disorders (MSKs) are the leading cause of long-term disability globally. In some cases, these diseases may progress to the point of causing dysfunction or even death. Presently, numerous studies have been published regarding the impact of ferroptosis on the development and outcome of orthopedic diseases. Therefore, it is essential to consolidate the understanding of the causes and clinical management strategies for iron deficiency in the field of orthopedic diseases.
The notion of ferroptosis was first proposed in 2012 by Dixon et al. (Dixon et al., 2012). In the 1950s, researchers discovered specific changes in the morphology of cystine-deficient cells (Eagle, 1955a; Eagle, 1955b). It was also revealed that the addition of cysteine did not alter the morphological changes of cells triggered by cystine deficiency, which may be mainly due to the difference in intracellular absorption mechanisms between cystine and cysteine (Eagle, 1959; Eagle et al., 1961). Cystine deficiency leads to reduced glutathione and cell death (Bannai et al., 1977). In the liver necrosis model, which is now thought to be caused by ferroptosis, the addition of glutathione and cysteine protects tissues from injury (Mitchell et al., 1973). These studies link cystine, cysteine, and glutathione. By introducing α-tocopherol, an antioxidant, it can be accomplished to reverse cell death caused by cystine deprivation, even without an increase in glutathione levels (Bannai et al., 1977). The findings of this investigation provide further proof that the formation of ROS is a causative component in cell death (Hirschhorn and Stockwell, 2019).
Subsequent investigations have shown that the prevention of such forms of cell death may be achieved by introducing iron chelators and lipophilic antioxidants (Ratan et al., 1994; Ratan et al., 1996; Wang et al., 2004). Glutathione peroxidase 4 (GPX4), discovered in 1982, showed its capacity to suppress iron-catalyzed lipid peroxidation in microsomes. The foremost function of GPX4 is to safeguard phosphatidylcholine-containing liposomes and biofilms against destruction caused by peroxidation (Ursini et al., 1985; Ursini et al., 1982). It is regarded as a crucial enzyme inhibitor that regulates ferroptosis cell death (Arai et al., 1999; Hurst et al., 2001). This protective effect is assumed to be due to GPX4’s ability to shield cells from oxidative stress-induced cell turnover (Yagi et al., 1996). The notion of non-apoptotic types of cell death was first introduced by much research on non-apoptotic caspase-independent cell death, characterized by necrosis-like morphology (Borner and Monney, 1999; Fiers et al., 1999; Loscalzo, 2008).
Stoxwell’s laboratory conducted a screening of deadly chemicals that specifically targeted cells harboring oncogenic mutations HRAS as big and small T oncoproteins. Due to its evident capacity to eliminate RAS and small T-transformed cells, it was designated as “erastin” (Dolma et al., 2003; Yang and Stockwell, 2008). Nevertheless, no discernible indicators of programmed cell death were seen after the administration of erastin to the tumor cells. Iron chelators and lipophilic antioxidants were successful in preventing the fatal effects of erastin. This finding indicates that erastin has the ability to trigger a kind of cell death that is not associated with apoptosis (Dolma et al., 2003; Yagoda et al., 2007). Another study also discovered that RAS synthetic lethal 3 (RSL3) induces a non-apoptotic form of cell death that is reliant on iron. Subsequent experiments by Dixon and Stockwell confirmed that erastin acts by inhibiting cystine/glutamate reverse transporter (SystemXc-), which reduces the cysteine-dependent synthesis of reduced glutathione (GSH). This is the first explanation of the mechanism of ferroptosis induction. According to these findings, the term ferroptosis was coined in 2012 to describe this iron-dependent, non-apoptotic form of cell death induced by erastin and RSL3 (Dixon et al., 2012; Dixon et al., 2014) (Figure 1).
Figure 1. Timeline for development of ferroptosis. Each time point has critical findings on the mechanism of ferroptosis. These findings have led to the refinement of ferroptosis theories as distinct from other modes of ferroptosis. ACSL4: Acyl-CoA synthetase long-chain family member 4; ATM: ataxia telangiectasia mutated; BH4: tetrahydrobiopterin; CD8+: a human leukocyte differentiation antigen; CoQ10: Ubiquinone-10; CST1: Cystatin SN; FSP1: ferroptosis suppressor protein 1; GCH1: Guanosine triphosphate cyclohydrolase-1; GPX4: Glutathione Peroxidase 4; HPCAL1: hippocalcin like 1; LPCAT3: Lysophosphatidylcholine acyltransferase 3; NCOA4: Nuclear Receptor Coactivator 4; NrF2: Nuclear factor erythroid 2-related factor 2; OTUD5:OTU Deubiquitinase 5; PL-PUFA2S: phospholipids with two polyunsaturated fatty acyl tails; PUFA: polyunsaturated fatty acid; RSL3: RAS synthetic lethal 3; System Xc-: A Cystine/Glutamate Reverse Transport System; TMEM164: Transmembrane Protein 164.
This review provides an overview of the molecular mechanisms underlying ferroptosis, the connection between ferroptosis and other forms of cell death, the development of ferroptosis in orthopedic diseases, and protocols to target ferroptosis for the treatment of orthopedic diseases. This review aims to enhance the ability of clinicians to provide more accurate guidance in clinical diagnosis and treatment options.
Ferroptosis differs from other modes of cell death mainly because of its iron concentration dependence as well as lipid peroxidation. In this process, iron metabolism disorders, antioxidant system imbalance as well as mitochondrial dysfunction are involved in the progression of cellular ferroptosis. We will address in this section the mechanisms by which ferroptosis occurs, including the mode of iron metabolism in organisms, the effect of iron on lipid peroxidation, and the role of the antioxidant system during ferroptosis. And we will introduce how ferroptosis occurs in cells from the perspective of lipid metabolism, energy metabolism, and amino acid transport. We also introduce the perspectives of inflammation, hypoxia and epigenetics to further describe the mechanism of ferroptosis (Figure 2).
Figure 2. Mechanistic map of ferroptosis occurring in cells. A variety of key molecules, pathways and related reactions are involved in ferroptosis when cells undergo ferroptosis, of which abnormal iron metabolism and lipid peroxidation are key factors in the development of ferroptosis. ·OH: hydroxyl radical; ALOX: Arachidonate Lipoxygenase; CoQ10H2: reduced form of coenzyme Q10; DMT1: divalent metal transporter 1; FPN: ferroportin; GSH: Glutathione, Reduced; GSR: Glutathione-Disulfide Reductase; GSSG: Glutathione, Oxidized; HO-1: Heme oxygenase-1; LIP: labile iron pool; LPCAT3: Lysophosphatidylcholine Acyltransferase 3; NADP+: Nicotinamide adenine dinucleoside phosphate; NADPH: nicotinamide adenine dinucleotide phosphate; PLOO·: Phospholipid hydrogen peroxide radical; PUFA-CoA: polyunsaturated fatty acid- Ubiquinone; PUFA-PL: phospholipids containing polyunsaturated fatty acids; PUFA-PL-OOH: PUFA phospholipid hydroperoxides; ROS: reactive oxygen species; SLC3A2: Solute Carrier Family 3 Member 2; SLC7A11: Solute Carrier Family 7 Member 11; STEAP3: Six-Transmembrane Epithelial Antigen Of Prostate 3; TCA: tricarboxylic acid cycle; TF: transferrin; TFR1: transferrin receptor 1.
The human body has approximately 3–5 g of iron, which is primarily found in red blood cells and hemoglobin (Wang and Pantopoulos, 2011). The tight management of iron absorption from the meal in the duodenum is a vital mechanism for maintaining homeostasis (Hentze et al., 2010). Mammals experience iron loss by detachment or bleeding from mucosal and skin cells (Chen et al., 2023). Therefore, the regulation of iron levels at the systemic level involves maintaining a balance between iron supply, utilization, and loss (Hentze et al., 2010; Muckenthaler et al., 2017).
Systemic iron metabolism involves the co-participation and co-regulation of multiple pathways. Iron is transported through the blood stream to juvenile erythrocytes and most tissues using transferrin (Tf) (Weichhart, 2024). The reticuloendothelial system can also participate in iron metabolism in vivo as an iron reservoir (Comità et al., 2024). Among them, cytochrome b in the duodenum has a strong regulatory effect on iron (Lane et al., 2015). Ferric reductase is coupled to divalent metal transporter 1 (DMT1) or divalent cation transporter (DCT1) to transport Fe2+ across the apical membrane oxygenase promotes inorganic iron release from heme in macrophages and enterocytes (Cain and Smith, 2021). Hepcidin binds to ferroportin (FPN), thereby controlling iron transport into the plasma (Wang and Babitt, 2019).
Cells possess a comprehensive mechanism for regulating the absorption and metabolism of iron, in comparison to systemic iron metabolism. The plasma membrane exerts strict regulation over the transportation of iron between cells. Cells adhere to transferrin receptor 1 (TfR1) and obtain iron from Tf (Anderson and Vulpe, 2009). Each Tf molecule is able to bind two Fe3+ (Chua et al., 2007). The Tf-TfR1 complex is internalized and acidified in endosomes via receptor-mediated endocytosis, facilitating Fe3+ release (Dautry-Varsat et al., 1983; Bali et al., 1991). Fe3+ is then reduced to Fe2+ via six-transmembrane epithelial antigen of the prostate 3 (STEAP3). Fe2+ enters the cytoplasm via DMT1 or transient receptor potential protein (TRPML1) (Fleming et al., 1998; Ohgami et al., 2006). The Tf-TfR1 complex then breaks down and enters the next cycle. Non-transferrin bound iron (NTBI) can similarly contribute significantly to cellular iron uptake. When the ability of plasma Tf to bind iron is overloaded, NTBI can rapidly enter the cell to replace the function of Tf (Craven et al., 1987; Wright et al., 1986). The labile iron pool (LIP) refers to a collection of iron that has a low molecular weight and is weakly bound to chelating agents. Iron that is taken up through Ff-dependent or independent pathways enters the labile LIP (Kruszewski, 2003). Furthermore, in situations where cellular iron levels are insufficient, iron regulatory protein 1 and iron regulatory protein 2 attach to stem-loop structures known as iron response elements (IREs) in messenger RNAs that encode various proteins involved in iron metabolism (Meneghini, 1997; West and Oates, 2008; Nemeth et al., 2004).
Iron overload is a condition that occurs due to various factors. Iron overload is typically categorized as either primary or secondary (Cherukuri et al., 2005). The main component of secondary iron load is the iron that builds up because of transfusions used to treat disorders of the red blood cell system (Larrick and Hyman, 1984). β-thalassemia can involve both increased absorption of iron and an excess of iron from transfusions (Anderson, 2007). Primary iron overload is characterized by the increased absorption of excessive iron due to the enhanced reabsorption function of the small intestine. Diseases related typically exhibit low levels of hepcidin in the bloodstream in relation to the amount of iron in the body (Uchida et al., 1983; Hentze et al., 2004).
When the binding capacity of plasma Tf is excessive, the free iron concentration in plasma increases, NTBI increases rapidly (Bernstein, 1987; Trenor et al., 2000). The result of this process is the gradual accumulation of iron in these organs, which leads to toxicity (Dickinson and Connor, 1994; Beutler et al., 2000; Asada-Senju et al., 2002). The formation of highly ROS is initiated by labile cellular iron (Craven et al., 1987). ROS are injurious, nevertheless cells possess multiple defense mechanisms to minimize ROS accumulation (Finch, 1994). When the body’s defense mechanisms reach max capacity, they can result in cell destruction. Lipid peroxidation is caused by ROS attacking cell and organelle membranes (Craven et al., 1987).
Ferroptosis characterized by elevated levels of lipid peroxidation and a lack of ability to remove lipid peroxides (Dixon et al., 2012; Stockwell, 2022; Yang et al., 2016). Because phospholipids (PL) in mammalian cell membranes contain large amounts of PUFAs, PLs are the major sites of lipid peroxidation (Li et al., 2024a). Lipid peroxidation is categorized into three distinct stages: initiation, propagation, and termination (Yan et al., 2021). The initiation process removes hydrogen atoms from allylic carbons in PUFAs by ROS, RNS, and RLS, forming lipid radicals. Hydroxyl and hydroperoxyl radicals are generated through the Fenton reaction involving ferrous iron and hydrogen peroxide. Lipid peroxidation can be initiated by reactive nitrogen species (RNS) like peroxynitrite, reacting with nitric oxide and superoxide. Lipid radicals undergo oxygen-to-peroxyl reactions, producing lipid peroxides that can be converted by GPX4 or broken down into reactive lipid species (RLS), such as 4-HNE and MDA, perpetuating lipid peroxidation and triggering cellular signaling.
Phospholipid peroxidation, reliant on iron, leads to membrane degradation and cell death (Liang et al., 2022). PL’s two fatty acyl chains (sn-1 and sn-2) contribute to its chemical diversity, with sn-1 having SFA or MUFA, and sn-2 having SFA, MUFA, or PUFA (Harayama and Riezman, 2018). PUFA-PLs are the substrate for PL peroxides. They can be converted to PLOOH enzymatically or non-enzymatically. Normally, GPX4 reduces PLOOH to phosphatidyl alcohols, terminating the Fenton reaction (Ursini et al., 1982; Seiler et al., 2008).
PUFA-PLs are more prone to ferroptosis due to their susceptibility as substrates for PL peroxidation (Doll et al., 2017; Kagan et al., 2017). PL may undergo peroxidation through non-enzymatic reactions, facilitated by metals like iron. Intracellular iron can become labile and react with H2O2, initiating the Fenton reaction which converts Fe2+ and Fe3+ ions and H2O2 into reactive radicals and lipid peroxidation.
If neutralization is slow, PLOOH can engage in an iron-catalyzed Fenton reaction, forming lipid radicals, which can spread oxidation to nearby PUFA-PL molecules (Chen et al., 2020; Geng et al., 2018; Gao et al., 2016; Hou et al., 2016). Iron-dependent enzymatic reactions, like those catalyzed by lipoxygenases (LOXs), which possess non-heme iron, play a crucial role in the peroxidation of phospholipid membranes, influencing ferroptosis regulation (Liang et al., 2022; Kuhn et al., 2015).
15-LOX facilitates conversion of PE and plays a role in peroxidizing membrane phospholipids (Erba et al., 2024). This conversion is very selective and specific, resulting in the catalysis of arachidonoyl (AA) and adrenoyl (AdA) groups (Stoyanovsky et al., 2019). GPX4 is crucial to lipid peroxidation. GPX4 converts poisonous PLOOH to harmless PLOH (Ursini et al., 1982). Phospholipids/neutral lipid hydroperoxides are reduced to their hydroxyl derivatives by GPX4 (Björnstedt et al., 1995; Imai, 2004; Imai and Nakagawa, 2003). nhibiting GPX4 activity promotes PL peroxidation, PLOOH buildup, and ferroptosis (Friedmann Angeli et al., 2014; Yang et al., 2014).
As indicated, ROS have significance to several cell death processes. ROS greatly impact lipid peroxidation, which is the hallmark of ferroptosis. DNA, protein and lipid can react with ROS (Halliwell and J, 2022). Lipid peroxidation occurs when oxygen free radicals attack PUFAs, resulting in the generation of lipid free radicals. These radicals then proceed to target neighboring PUFAs and membrane proteins, ultimately leading to membrane lipid peroxidation (Yang et al., 2016; Liang et al., 2022; Que et al., 2018). ROS generated via the Finton reaction catalyzed by iron (Lei et al., 2019; Toyokuni et al., 2017). ROS-PUFA reactions cause lipid peroxidation. Oxidative stress triggered by ROS in lipids leads to cell damage (Rochette et al., 2022).
SystemXc-is a transporter that is located on the cell membrane. The transportation and exchange of cystine can be facilitated by System Xc-, which also allows for the transport of glutamate (Fantone et al., 2024). Cystine enters cells and is reduced to cysteine, which combines with glutamate and glycine to form GSH. System Xc-controls GSH production through disulfide bonds between solute carrier family 7 member 11 (SLC7A11) and solute carrier family 3 member 2 (SLC3A2) (Liu et al., 2020a; Jiang and Sun, 2024). SLC7A11 facilitates cystine to glutamate transfer in a 1:1 ratio (Fantone et al., 2024). Cysteine is subsequently reduced to cysteine by thioredoxin reductase 1 (TrxR1) (Conrad and Sato, 2012; Mandal et al., 2010). SLC3A2 plays a crucial role in regulating the transport function of SLC7A11 and ensuring its stability (Nakamura et al., 1999).
Glutamate, cysteine, and glycine form GSH, with glutamate-cysteine synthase and glutathione synthase as catalysts for its production (Bansal and Simon, 2018; Forman et al., 2009). Within the cell, most of the space is occupied by reduced glutathione (Diaz-Vivancos et al., 2015). Glutathione disulfide (GSSG) is the most common oxidized form of glutathione. Both reduced and oxidized forms of glutathione are capable of undergoing interconversion (Niu et al., 2021). The progression of ferroptosis is contingent upon the levels of GSH((Guo et al., 2021)). GSH is an antioxidant that neutralizes ROS, inhibits lipid peroxides, and maintains cellular redox equilibrium by adjusting NADP/NADPH and GSH/GSSG ratios (Diaz-Vivancos et al., 2015; Sahoo et al., 2022; Zhong et al., 2022). GSH acts as a cofactor for GPX4, preventing lipid peroxidation and ferroptosis by converting LOOH into LOH (Jia et al., 2020).
Cysteine in GPX’s protein superfamily redox residues catalyzes redox processes (Flohé et al., 2022; Xie et al., 2023). GPX4 can prevent lipid oxidation and biofilm destruction by using GSH’s reducing equivalent (Ursini et al., 1982; Nishida et al., 2022). GPX4 reduces intracellular peroxides, especially phospholipid hydroperoxides (Ursini et al., 1982; Thomas et al., 1990). GPX4 is most typically expressed in the cytosol, followed by mitochondrial and nuclear classes. Apoptosis resistance is mostly due to mitochondrial GPX4 (Liang et al., 2009). GPX4 is one of the main regulators performing ferroptosis. Gpx4 safeguards mitochondria from peroxide damage (Liu et al., 2024). GPX4 loss or malfunction causes intracellular peroxide buildup and ferroptosis (Xie et al., 2023). GSH functions as a crucial cofactor for GPX4, inhibiting lipid peroxidation and ferroptosis through the reduction of LOOH to LOH (Li FJ. et al., 2022).
Glucose serves as a primary energy source, transformed into pyruvate through glycolysis, then entering the tricarboxylic acid (TCA) cycle and oxidative phosphorylation (OXPHOS) (Liu J. et al., 2021; Nie et al., 2020). During anoxia, the enzyme lactate dehydrogenase catalyzes the conversion of pyruvate into lactate (Sonveaux et al., 2008). Glucose 6-phosphate can also produce NADPH and biosynthetic precursors through the pentose phosphate pathway (PPP) (Lee et al., 2017; Martinez-Outschoorn et al., 2017). NADPH plays a crucial role in the transformation of oxidized glutathione into reduced glutathione (Dong et al., 2015; Liu et al., 2019). Mitochondria play a crucial role in energy metabolism and are responsible for generating ROS (Ashton et al., 2018; Zong et al., 2016). The occurrence of ferroptosis is closely linked to energy metabolism.
Energy metabolism involves electron transfer for glucose-dependent systems, with electrons transferred to oxygen, generating ROS (Chen and Zweier, 2014; Corbet et al., 2016; Förstermann et al., 2017). Blocking TCA cycle in tumor cells disrupts electron transfer, reducing ROS levels and preventing ferroptosis (Gao et al., 2015; Gao et al., 2019a). Ferroptosis is linked to TCA cycle intermediates and enzymes. Glutamine relates to iron-induced apoptosis, while glutamine synthase 2 facilitates iron-mediated programmed cell death (Suzuki et al., 2022). α-Ketoglutarate, a TCA cycle intermediate, induces iron-dependent cell death like glutamine. Citrate synthase and acyl-CoA synthesis family member 2 control the production of fatty acids and impact the process of lipid peroxidation (Gao et al., 2019a). Pyruvate dehydrogenase kinase 4 prevents cell death caused by iron by inhibiting pyruvate dehydrogenase, while malic enzyme 1 deficiency in synovial sarcoma tumors makes cells vulnerable to ferroptosis (Song et al., 2021). Deficiency of malic enzyme 1 in tumor models of synovial sarcoma renders cells vulnerable to ferroptosis (Brashears et al., 2022). Fumarate hydratase inactivation causes cell death in hereditary leiomyomatosis and renal cell cancer, linked to GPX4 inactivation (Kerins et al., 2018). Mutations occur in isocitrate dehydrogenases (IDH1 and IDH2) in cancer cells (Gbyli et al., 2022; Varn et al., 2022). Based on this premise, cancer cells are susceptible to erastin-induced ferroptosis (Kim H. et al., 2020). IDH1 can additionally suppress GPX4 expression and facilitate GSH depletion, thereby triggering ferroptosis (Wang et al., 2019).
PPP is an energy metabolism branch that produces NADPH, essential for fatty acid synthesis and preventing ferroptosis by converting GSSG to GSH. Ferroptosis inducers decrease NADPH activity (Shimada et al., 2016). NADPH facilitates cystine uptake through SLC7A11, converting it to cysteine for GSH generation (Shu et al., 2020; Liu X. et al., 2021). Thioredoxin (Trx) helps trigger ferroptosis by being a redox pathway. Blocking Trx’s activity triggers ferroptosis. NADPH helps convert oxidized Trx to its reduced form (Sun et al., 2001). NADPH can also enzymatically convert coenzyme Q10 (CoQ10) into CoQ10-H2, resulting in inhibiting lipid peroxidation (Bersuker et al., 2019; Doll et al., 2019). Ferroptosis triggers PPP-NADPH production, boosting GSH, Trx, and CoQ10-H2 antioxidant effects.
Iron deficiency is linked to inflammation, triggering production of inflammatory molecules that stimulate lipid peroxidation (Chen X. et al., 2021; Chen Y. et al., 2022; Sun et al., 2020). Ferroptosis is linked to inflammatory signaling pathways. Triggering ferroptosis with erastin or RSL3 activates the JAK-STAT pathway through IFN-γ in tumor cells (Barrat et al., 2019; Kong et al., 2021). The JAK2-STAT3 signaling pathway positively correlates with hepcidin expression, influencing systemic iron metabolism through regulation (Yang L. et al., 2020; Ren et al., 2021; Kowdley et al., 2021).
The NF-κB pathway is associated with the activation of inflammation and the innate immune response (Hoesel and Schmid, 2013). Ferroptosis is mediated by the NF-κB signaling pathway, which is activated by ferroptosis inducer RSL3, regulating System Xc− transmission and interacting with heme oxygenase 1 to metabolize heme and ferrous iron (Pulkkinen et al., 2011; Ryter, 2021). NF-κB signaling promotes ferritin heavy chain 1(FTH1) expression in response to TNF-α (Kou et al., 2013). Ferritin light chain expression (FTL) increases in lipopolysaccharide (LPS)-stimulated macrophages, limiting NF-κB signaling and reducing TNF-α, IL-1β, and inflammation (Zarjou et al., 2019).
Inflammasomes have a strong correlation with lipid peroxidation. NLRP3 inflammasomes upregulated in response to GPX4 inhibition, linked to lipid peroxidation (Xie et al., 2022). Inhibition of NLRP3 reverses oxidative stress in a lipid peroxidation model (Orecchioni et al., 2022). Suppressing NLRP3 led to increased GPX4, elevated GSH levels, and reduced phospholipid peroxides (Meihe et al., 2021). The cGAS-STING pathway interacts with ferroptosis, inducing oxidative stress and STING translocation. STING inhibition decreases ferroptosis sensitivity (Li C. et al., 2021). Ferroptosis from high iron diet and GPX4 deficiency can cause pancreatic cancer in mouse models, affecting cGAS-STING signaling (Dai et al., 2020).
The MAPK pathway triggers ferroptosis through inflammatory activation, inducing pro-inflammatory cytokines, suppressing GPX4 and System Xc−, and leading to neuroinflammation and cell death (Zhu et al., 2021). Excessive iron activates ERK1/2 and p38, causing oxidative stress and peroxide formation through the MAPK pathway (Salama and Kabel, 2020; Ikeda et al., 2019). Application of antioxidants resulted in the inhibition of the MAPK pathway and a reduction in peroxide concentration (Fu et al., 2018; Cavdar et al., 2020).
Hypoxia is a physiological reaction that occurs due to various internal or external conditions (Tano and Gollasch, 2014; Chen G. et al., 2022; McClelland and Scott, 2019). Within the hypoxic environment, some distinct signaling pathways are triggered, mostly through the mediation of hypoxia-inducible factor (HIF) (Schito and Semenza, 2016; Kaelin and Ratcliffe, 2008). Oxidative stress increases under hypoxia and ROS accumulation is the main cause of ferroptosis (Honda et al., 2019). The HIFs family has three isoforms: HIF-1, HIF-2, and HIF-3. HIF-1 forms in extreme oxygen deprivation, while HIF-2 forms in moderate oxygen deprivation (Koh and Powis, 2012; Cowman and Koh, 2022).
The HIFs family has three isoforms: HIF-1, HIF-2, and HIF-3. HIF-1 forms in extreme oxygen deprivation, while HIF-2 forms in moderate oxygen deprivation (Zheng X. et al., 2023). The inhibiting impact of this substance affects both normal cells and malignant cells (Kumar and Choi, 2015; Pan et al., 2021). HIF-1α prevents ferroptosis by stabilizing SLC7A11 and activating hypoxia response elements that control glutathione metabolism (Hu et al., 2022; Lin Z. et al., 2022). HIF-2α acts as a positive regulator of ferroptosis (Johansson et al., 2017; Singhal et al., 2021). HIF-2α triggers ferroptosis by activating genes like ACSL4 Cigarette smoke exposure boosts HIF-2α, leading to myotube apoptosis (Zhang L. et al., 2022). Further investigation is needed to investigate the diametrically opposite effects of HIF-1α and HIF-2α.
α-tocopherol is a free radical-trapping antioxidant that blocks peroxidation propagation and inhibits phospholipid peroxide accumulation (Burton and Ingold, 1981; Traber and Atkinson, 2007; Ingold and Pratt, 2014). By competitively binding PUFAs and scavenging hydroxyl radicals, α-tocopherol has been demonstrated to block the action of LOX, hence preventing ferroptosis (Feng and Stockwell, 2018; Angeli et al., 2017). In vitro studies have shown thatα-tocopherol can protect GPX4 knockout mice from ferroptosis (Kagan et al., 2017; Friedmann Angeli et al., 2014). α-tocopherol can synergize with GPX4 to inhibit lipid peroxidation (Carlson et al., 2016; Wortmann et al., 2013).
NRF2 is a transcription factor that controls cellular antioxidant reactions and the effects of oxidative stress (Kajarabille and Latunde-Dada, 2019). Reducing the expression of NRF2 enhances vulnerability to ferroptosis (Xie et al., 2016). NRF2 additionally governs SLC7A11 (Rosell et al., 2023). NRF2 regulates iron/ferroheme metabolism at the metabolic level and governs the activity of FTL and FTH1, which are crucial proteins involved in iron metabolism (Agyeman et al., 2012; Harada et al., 2011; Kerins and Ooi, 2018). NRF2 is also involved in regulating NADPH, GSH and GPX4 synthesis (Jiang et al., 2024; Luchkova et al., 2024).
Epigenetics is a dynamic process that includes DNA methylation, histone modification, and non-coding RNA (ncRNA) regulation, which allows gene expression to change without changing the DNA sequence (Wang N. et al., 2023; Cavalli and Heard, 2019). In addition to being impacted by traditional signaling pathways, ferroptosis-related genes are also controlled by epigenetic processes.
Gene expression is determined by the structure of the chromosome. Through acetylation, methylation, and ubiquitination, histone modification controls the structure of DNA (Yang M. et al., 2020). It is regulated by the bromodomain-containing protein (BRD) family, histone acetyltransferases (HATs), and histone deacetylases (HDACs) (Sabari et al., 2017). BRD4 suppression or inhibitors led to ferroptosis in tumor cells, suppressing GPX4 and System Xc− expression (Sui et al., 2019). Histone acetylation stimulates transcription, while deacetylation inhibits transcription (Barneda-Zahonero and Parra, 2012). NRF2 recruits P300/CBP-associated factor (PCAF), which regulates ferroptosis through H3K9ac levels, controlling SLC7A11 expression (Chung et al., 2019). HDACs inhibit epithelial-mesenchymal transition (EMT) markers’ expression in cancer cells, resulting in ferroptosis initiation (Liu L. et al., 2021; Lee J. et al., 2020). Histone methylation regulates transcription by modifying H3 and H4 histone N-terminal lysine/arginine residues (Yi et al., 2017a; Yi et al., 2017b). Histone methyltransferases are enzymes that add methyl groups to specific sites on histone proteins, including H3K4 and H3K9 (Li et al., 2019). He elevated expression of GPX4 in cancerous cells could be attributed to the augmented abundance of H3K4me3 in its promoter region (Ma M. et al., 2022). Increasing H3K4me3 abundance upregulates Acyl-CoA Synthetase, suppressing ferroptosis (Zhang et al., 2020). The expression of SLC7A11 is tied to histone 2A ubiquitination and histone 2B ubiquitination (Ling et al., 2022; Horniblow et al., 2022).
DNA methylation plays a crucial role in the ferroptosis process as it regulates the synthesis of PUFAs and levels of ROS (Jiang et al., 2017; Lee JY. et al., 2020). DNA methylation represses gene activity related to lipid peroxidation, preventing ferroptosis, and inactivates GPX4 promoter causing cellular ferroptosis (Zhang J. et al., 2022; Ling et al., 2022). Methylation is controlled by ferroptosis, which occurs in iron-rich environments and affects NRF2(213).
MiRNAs regulate epigenetic inheritance by binding to 3′UTRs and interfering with mRNA translation (Zhang and Liu, 2021). Research has shown that miRNAs target proteins that regulate iron metabolism, leading to ferroptosis (Li X. et al., 2021; Wei et al., 2021). In addition, miRNAs can also inhibit System Xc− and GPX4 expression to promote ferroptosis (Fan et al., 2021; Xu et al., 2020; Deng et al., 2021; Yadav et al., 2021; Ding et al., 2020). Researchers focus on Circular RNAs (circRNAs), which counteract miRNA inhibition on GPX4 expression through endogenous competition (Xu et al., 2020; Chen W. et al., 2021). CircRNA can also act as a sponge for miRNA, resulting in the upregulation of SLC7A11 expression (Wu et al., 2021). Long non-coding RNAs (lncRNAs) can directly interact with the p53 gene and trigger ferroptosis via the p53-GPX4 axis (Chen C. et al., 2021). Additionally, lncRNAs can indirectly facilitate ferroptosis by promoting apoptosis (Wang Z. et al., 2021).
Multiple mechanisms are involved in the epigenetic regulation of ferroptosis, as stated in conclusion. In addition, there are inextricable connections between its systems, which are consistent areas that require additional research.
Lysosomes are attached to autolysosomes during autophagy in order to facilitate cellular turnover and metabolism. The breakdown of internal metabolic activities during autophagy results in the creation of autophagosomes (Luo and Tao, 2020; Zhou et al., 2020). There is growing evidence that ferroptosis requires autophagy’s involvement (Mizushima and Levine, 2020). T Ferroptosis-inducing medications can cause GPX4 degradation by autophagy, which is subsequently mediated by the enzyme acid sphingomyelinase, which is essential for the metabolism of sphingolipids (Thayyullathil et al., 2021). Autophagy causes the amount of free iron in the bodies of mice with subarachnoid hemorrhage to increase, which leads to ferroptosis of free iron in their bodies grow, which ultimately results in ferroptosis (Patil et al., 2021). A few results that are comparable to one another demonstrate that autophagy increases ferritin degradation (Hou et al., 2016; Liu et al., 2020b; Tian et al., 2020; Ma et al., 2017).
Pyroptosis is an inflammatory form of cell death that relies on the involvement of caspases (Chen et al., 2019). It is characterized by a similar pattern to ferroptosis, involving membrane damage, accompanied by ROS accumulation and iron dependence (Xu R. et al., 2021). ROS, via iron-dependent activation, facilitate caspase-related pathways, leading to the degradation of ferritin and the initiation of pyroptosis (Zhou et al., 2018).
Cuproptosis is characterized by an aberrant metabolism of copper ions. High levels of copper ions can lead to protein toxicity, which can ultimately result in the death of cells (Tsvetkov et al., 2022). Mitochondria link ferroptosis with cuproptosis. Mitochondria play a significant role in the production of ROS and directly contribute to cell death caused by iron. Additionally, in the mitochondrial TCA cycle, the process of glutaminolysis, which leads to a shortage of cysteine, is also responsible for cell death caused by iron (Gao et al., 2019b). Observations revealed morphological alterations in mitochondria affected by cuproptosis, with mitochondrial respiration and acting a significant part in this process (Tang et al., 2022). Furthermore, GSH serves as a central point linking iron toxicity with copper toxicity. A study has proven that the drugs sorafenib and erastin, which are ferroptosis inducers, increase cell death in primary hepatocellular carcinoma cells when combined with a copper ionophore (Wang W. et al., 2023). This effect is achieved by decreasing the synthesis of GSH. GSH also forms a complex with copper to decrease the buildup of copper within cells (Liu and Chen, 2024).
Apoptosis in programmed cell death is one of the most intensively studied. Initiation of apoptosis activates caspase (Yuan and Ofengeim, 2024). Caspases can cause cells to form apoptotic typical morphology, such as nuclear lysis and rounding of the cell shape (Rajagopalan et al., 2024). Ferroptosis was discovered as a novel programmed cell death independent of apoptosis because it caused cell death without caspase activation and could not be reversed by caspase inhibitors (Wu P. et al., 2023). But recent studies have found an inextricable link between iron death and apoptosis. For example, p53 apoptosis stimulating protein inhibitor (iASPP) inhibits p53-induced apoptosis, while iASPP also plays an anti-ROS role, thereby promoting Nrf2 accumulation and nuclear metastasis (Li et al., 2020). And Nrf2 is a protective mechanism against iron death. Perez et al. found that iron death is mutually exclusive with apoptosis (Perez et al., 2020). In addition, erastin induced p53 to promote apoptosis in A549 lung cancer cells (Huang et al., 2018). Erastin can also induce oxidative stress and cause caspase-9-dependent cell death (Huo et al., 2016).
The relationship between ferroptosis and disease is a hot research direction in recent years. The development of skeletal system disorders has also been widely documented to be strongly associated with iron death. In addition, multiple regulatory mechanisms of ferroptosis also play a critical role in the progression of skeletal system diseases. Therefore, we will discuss how ferroptosis affects the occurrence and outcome of bone diseases in this section, and try to enrich clinical treatment strategies through the exploration of the mechanism of iron death in seven bone diseases (Figure 3).
Figure 3. Schematic representation of the mechanisms by which ferroptosis regulates seven skeletal diseases. ATF3: Activating Transcription Factor 3; CircBLNK: circular RNA; DEPDC5: DEP Domain Containing 5; DFO: Deferoxamine; ECM: Extracellular matrix; ERS: Endoplasmic Reticulum Stress; GRP78: Glucose regulatory protein 78; GSK-3β: Glycogen Synthase Kinase 3 Beta; Hif-2α: Hypoxia Inducible Factor 2 Subunit Alpha; HMOX1: Heme Oxygenase 1; IL-1β: Interleukin 1 Beta; MAPK: Mitogen-Activated Protein Kinase; MSU: monosodium urate; NLRP3: NLR Family Pyrin Domain Containing 3; NOX: nitrogen oxides; NP: Nucleus pulposus; OB: Osteoblast; OC: osteoclast; RANKL: Receptor Activator of Nuclear Factor-κB Ligand; Runx2: RUNX Family Transcription Factor 3; Sirt3: Sirtuin 3; TNF-α: Tumor Necrosis Factor Alpha; UA: urine acid; UPR: unfolded protein response; USP11: Ubiquitin Specific Peptidase 11; XO: xanthine oxidase; ZEB1: Zinc Finger E-Box Binding Homeobox 1.
Chondrocytes are the only cells that constitute articular cartilage and act to be responsible for the metabolism of the extracellular matrix (ECM) (Pettenuzzo et al., 2023). Studies have demonstrated that chondrocytes, which are affected by inflammation, tend to accumulate ROS and have modified expression of ferroptosis-related proteins in models of OA. More precisely, the expression of GPX4 and SLC7A11 in chondrocytes was reduced when inflammation was triggered by IL-1β and by creating a simulated iron overload environment. Utilizing ferroptosis inhibitors resulted in a decrease in ROS levels and a reduction in cytotoxicity (Yao et al., 2021). Another study revealed that inflammatory stimuli can disturb the iron equilibrium in chondrocytes. The expression of TfR1 was upregulated while the expression of FPN was downregulated in chondrocytes after treatment with IL-1β and TNF-α. An excess amount of iron in cartilage leads to the destruction of cartilage, resulting in oxidative stress and damage to the mitochondria (Jing et al., 2021).
From the aforementioned investigations, it is evident that there is a strong correlation between the occurrence of OA, characterized mostly by cartilage destruction, and iron depletion. Oa is a closely related disease to age and a disease that is easily disabling in chronic diseases. Inflammation, cartilage degeneration, and synovial hyperplasia can be seen in OA. Abnormal iron metabolism can directly contribute to OA by causing inflammation, in addition to the indirect damage to cartilage that is commonly associated with the condition (Burton et al., 2020). Elevated ferritin levels are one of the risk factors for OA. Imaging analysis showed a positive correlation between ferritin levels and the severity of arthritis (Cai et al., 2021; Kennish et al., 2014). One element affecting older patients’ morbidity is gender. Research has shown that OA affects older women on more occasions than older males, and that this difference is related to postmenopausal estrogen levels (Ko and Kim, 2020). Nonetheless, ferritin levels are negatively connected with estrogen levels, and iron levels in the serum of postmenopausal women are 200%–300% higher than those of non-menopausal women, according to current research. Because of this distinction, postmenopausal women experience a much higher prevalence than do men (Park et al., 2012; Zhang et al., 2001; Ke et al., 2021). There is a substantial correlation between blood problems and OA, according to a Mendelian randomization study (Xu J. et al., 2022). Hemophiliac individuals have an increase in red blood cells in the joint space because of continuous methemoglobin release and prolonged bleeding, which causes iron accumulation and ferroptosis (van Vulpen et al., 2018; Bhat et al., 2015). Patients with hemochromatosis who have OA have also been observed to accumulate iron in the joint space; dysmorphic cartilage has been restored after excess iron has been removed (Richette et al., 2010; Heiland et al., 2010).
The mechanism of OA resulting from ferroptosis involves inhibition of antioxidant pathways. In OA, inhibition of GPX4 and SLC3A2 expression has been shown (Liu H. et al., 2022; Miao et al., 2022). ECM degrades when GPX4 expression is suppressed (Miao et al., 2022). Type II collagen (collagen II) was also expressed in ECM by ferroptosis inhibitors, and this process was reversed after ferroptosis inhibitor treatment (Yao et al., 2021). Activation of Nrf2 can also prevent ferroptosis (Xu C. et al., 2022). In addition, HIF-2α stimulates lipid peroxidation and suppresses GPX4 and SLC7A11 to facilitate chondrocyte ferroptosis (Zhou et al., 2021). Piezo1, a mechanosensitive ion channel, is also involved in iron metabolism in OA by inhibiting the GSH-GPX4 axis (Wang S. et al., 2022; Ma et al., 2021). Overall, ferroptosis in OA involves multiple aspects. Among them, inflammation and antioxidant inhibition are the main causes of OA.
AS is a rheumatic bone disease caused by inflammation. The location of AS is centered on the sacroiliac joint and affects the surrounding joints (Thomas and Brown, 2010; Rostom et al., 2010). The main symptom of AS is inflammatory spinal pain, accompanied by bone erosion and ligamentous osteophytes (Tam et al., 2010). Although ferroptosis caused by lipid peroxidation is rarely reported in AS, it is not difficult to find from the study of specific serum proteomics and metabolome student markers in AS patients that the main reasons affecting ferroptosis in AS are iron levels and oxidative stress response. Studies have reported that serum TfR1 levels are low in AS patients, while platelet iron content remains high (Fischer et al., 2012; Feltelius et al., 1986). Decreased antioxidant capacity is one of the characteristics of AS. A study on serum oxidation and antioxidation in AS patients highlighted decreased antioxidant capacity and increased oxidative stress index in AS patients (Karakoc et al., 2007). AS patients with metabolic syndrome are more susceptible to oxidative stress (Pishgahi et al., 2020). GPX, which is closely associated with ferroptosis, was found to have decreased expression in mouse models of AS (Feng et al., 2020; Dong, 2018).
GA is characterized by hyperuricemia and urate deposition (Zhang Y. et al., 2022). Abnormal iron metabolism and antioxidant imbalance are one of the pathogeneses of GA. A Mendelian randomization study revealed a relationship between ferritin and risk of gout. This study is the first to demonstrate a positive association between serum ferritin and the risk and frequency of gout (Fatima et al., 2018). In a study on the association between markers of iron status and the risk of hyperuricemia in Chinese adults, the researchers found a positive correlation between serum ferritin, transferrin and hyperuricemia (Li et al., 2018). The same results were found in another US National Health and Nutrition Examination Survey (Ghio et al., 2005). Maintaining close to iron deficiency levels has also been demonstrated as a protective factor in GA (Facchini, 2003). Xanthine oxidase (XO) is the only source of urate. XO enhances its activity when combined with iron (Jomova et al., 2024; Maiti et al., 2022). A direct relationship between iron and uric acid is therefore concluded.
The autoimmune illness RA is typified by progressive bone damage and synovial hyperplasia. Teratogenicity in RA is caused by pannus development, chronic inflammation, and bone loss. Recent research has demonstrated that characteristics unique to ferroptosis can also be seen in RA, and these discoveries have established a connection between RA and ferroptosis. Treatment for RA may be improved by having a better understanding of the general process of ferroptosis in RA.
Abnormal iron metabolism is the first element leading to ferroptosis in RA. A Mendelian randomization study of genetic data from a large genome-wide association study of 257,953 individuals suggests that individuals with genes associated with higher iron levels may have a lower risk of RA (Wu, 2024). Another Mendelian randomization trial yielded similar results, showing a negative correlation between iron intake and RA (Wang et al., 2024a). According to a clinical investigation, RA patients had considerably lower serum iron levels and higher serum TFR values (Stefanova et al., 2018). Peripheral blood iron levels are lower in patients with severe RA (Wu et al., 2022).
Another important factor in the progression of RA is ROS, which has been shown to rise approximately fivefold in the mitochondria from whole blood and monocytes of RA patients. The main manifestations of ROS include increased oxidative stress and decreased antioxidant levels (Ferreira et al., 2021). Oxidative stress occurs when there is an imbalance between ROS production and the body‘s antioxidant defenses. In RA, elevated ROS levels were observed in synovial fluid, blood, and affected joint tissues. These ROS contribute to joint inflammation and injury by promoting activation of pro-inflammatory signaling pathways, inducing cytokine production, and enhancing proliferation of fibroblast-like synoviocytes (FLS). The resulting inflammatory and oxidative damage leads to degeneration of cartilage and bone, which are hallmark features of RA (Mueller et al., 2021). The immune system plays a crucial role in RA, where T cells, B cells, and macrophages contribute to a chronic inflammatory state. Oxidative stress can affect the function and survival of these immune cells. For example, ROS can modulate T cell activation and differentiation, skewing immune responses toward more inflammatory features (Hassan et al., 2011). High levels of inflammation trigger lipid peroxidation, and excess ROS also generate pannus at home, and such circulatory effects accelerate the progression of RA (Phull et al., 2018; Zhou et al., 2012). During RA, macrophages release a significant number of inflammatory factors. TNF-α has the most intricate role among them all. One the one hand, as previously mentioned, TNF-α can increase inflammation by inducing ROS generation through NADPH oxidase (Latchoumycandane et al., 2012). TNF-α has been observed to stimulate GSH production and cystine absorption, however. Long-term TNF exposure can prevent NADPH Oxidase (NOX) from producing ROS, shielding FLS from ferroptosis (Wu et al., 2022).
The endoplasmic reticulum (ER) is the major organelle responsible for protein synthesis and lipid metabolism in eukaryotic cells. ER stress (ERS) refers to a series of pathological conditions such as overload of folding mechanisms and disruption of redox balance that occur during protein synthesis in the ER (Tabas and Ron, 2011). The unfolded protein response (UPR) is a compensatory response initiated in the ERS state (Walter and Ron, 2011). Evidence suggests that in the setting of RA, inflammation provokes ERS and proteins are synthesized via UPR (Park et al., 2014). ERS shares signaling pathways with ferroptosis, which makes the role of ERS have to be considered when studying ferroptosis in RA (Li C. et al., 2023). Glucose-regulated protein 78 (GRP78) promotes aberrant protein breakdown under UPR in the ERS state (Grootjans et al., 2016). GRP78-specific antibodies were identified in 63% of RA patients (Bläss et al., 2001). ERS and increased GRP78 expression can be brought on by ferroptosis inducers. P53 functions as a tumor suppressor gene in ferroptosis and ERS. It has been discovered that p53, which induces cell cycle arrest to promote chondrocyte death, is substantially expressed in RA patients (Ghosh et al., 2012; Takatori et al., 2014; Hong et al., 2017).
The skeleton of the human body is always in a state of dynamic balance. Osteoblasts (OBs) and osteoclasts (OCs) are key players in this balance and occupy approximately 90% of the skeletal composition (Cui et al., 2022). Different cells are used to differentiate OBs from OCs. Bone marrow-derived mesenchymal stem cells (BMSCs) are the source of OBs, which is a component of bone production. Originating from the monocyte/macrophage lineage of hematopoietic cells, OCs is the main factor for bone resorption and is abundant in mitochondria and lysosomes (Boyce, 2013; Kim JM. et al., 2020; Sommerfeldt and Rubin, 2001). An imbalance between the production and resorption of bone results in OP, a metabolic bone disease that increases the risk of fractures. Ferroptosis is implicated in the pathophysiology of OP, according to numerous studies (Yang et al., 2022).
BMSCs serve as precursors for the generation of OBs (Lin et al., 2019). Runt-related transcription factor 2 (Runx2) regulates BMSCs to promote differentiation into OBs under normal physiological conditions. Other transcription factors that regulate differentiation into OBs include alkaline phosphatase (ALP) and osteocalcin (OCN) (Komori, 2022). The accumulation of body iron leads to an increase in ferritin expression in BMSCs, which inhibits transcription factors crucial for osteoblast development (Balogh et al., 2016). Similar ones have now been observed in a series of experiments: ferroptosis downregulates the OBs phenotype and promotes OBs death (Xu P. et al., 2022; Ma et al., 2020); inhibition of PI3K-Akt-mTOR prevented ferroptosis in BMSCs and upregulated Runx2 and ALP expression (Lan et al., 2022a); and several closely related pathways, including GPX4 and Nrf2, can regulate ferroptosis in OBs (Messer et al., 2009). The OBs ferroptosis process is also influenced by other gene expressions. In an in vitro investigation, iron mortality was noted in OBs treated with ferric ammonium citrate, and the genes TfR1 and DMT1, which are in charge of cellular iron uptake, were discovered to be overexpressed (Luo et al., 2022). In MC3T3-E1 cells, excess iron ions also increased the expression of the apoptosis gene and NOX4 (Tian et al., 2016).
OCs differentiate under the influence of activating the receptor activator of the nuclear factor-κB (RANK)-RANK ligand (RANKL) pathway. RANK-RANKL is a specific representation of OCs and reflects OCs number and activity. Research indicates that increased RANKL expression during iron overload conditions stimulates the development of OCs and ultimately results in OP (Yang J. et al., 2020; Ma J. et al., 2022). RANKL-induced differentiation of OCs involves ferroptosis, and the mechanism by which RANKL-induced ferroptosis in OCs is mediated is ferritin autophagy. For OCs to survive, intracellular iron levels are consequently essential. In addition, this study discovered that HIF-1α can effectively prevent osteopenia-related osteopenia by blocking ferritin autophagy (Ni et al., 2021).
Hematological diseases are also one of the causes of OP involved in ferroptosis. For example, people with hemochromatosis are more likely to have osteopenia, and some of them even develop OP, which is closely related to iron accumulation (Baschant et al., 2022). Osteoporotic fractures are more common in thalassemia patients when their bodies’ ability to excrete iron is compromised by prolonged, frequent blood transfusions and iron buildup. Studies of a similar nature have verified a positive correlation between the frequency of blood transfusions and the risk of fracture (Ekbote et al., 2021; Dede et al., 2016).
There are two types of OPs: primary and secondary. Primary OP mostly refers to senile OP and postmenopausal osteoporosis (PMOP), while secondary OP is primarily Diabetic osteoporosis (DOP) and glucocorticoid osteoporosis (GIOP). Abnormal glucose and lipid metabolism may be the pathogenesis of ferroptosis in OBs in DOP (Chen et al., 2023). The researchers discovered that reduced GPX4 expression in DOP bone tissue in mice led to cellular ferroptosis. It was also verified that turning on the Nrf2/Heme Oxygenase-1 (HO-1) pathway may undo this outcome (Ma et al., 2020). Osteocyte mortality in DOP was successfully prevented by focusing on ferroptosis or HO-1, which broke the vicious cycle between HO-1 activation and lipid peroxidation (Yang et al., 2022). Mitochondrial ferritin (FtMt) is generally considered a tool for mitochondria to regulate free iron content. In the DOP model, high expression of FtMt reduced iron-induced lipid peroxidation, whereas its expression is known to instead cause mitophagy in OBs (Wang et al., 2022b). Increased serum ferritin and decreased GPX4 expression were also observed in the mouse DOP model. Therefore, high glucose environment not only inhibits osteoblast expression, exacerbates trabecular degeneration, osteopenia, but also activates ferroptosis-related gene expression and inhibits the antioxidant system (Lin Y. et al., 2022).
The pathogenesis of PMOP is closely related to estrogen. Iron plays an important role in PMOP. In a study of 728 postmenopausal women, iron was found to be an important risk factor for the onset of PMOP (Okyay et al., 2013). Postmenopausal bone loss might be addressed by taking dietary iron supplements within reasonable bounds (Wylenzek et al., 2024). HIF-1α specific inhibitors have also been found to prevent bone loss in ovariectomy (OVX). Continuous steroid hormone administration impairs osteoblast differentiation activity and lowers antioxidant system capacity, which results in GIOP (Yang et al., 2021a). Patients receiving long-term steroid therapy are more likely to have trabecular bone destruction and osteoporotic fractures. Decreased expression activity of GPX4 and the System Xc− was found in the high-dose dexamethasone-induced GIOP model (Lu et al., 2019).
Based on these findings, we conclude that there is a close relationship between OP pathogenesis and iron metabolism, and the mechanism also involves lipid peroxidation and oxidative stress. Modulation of osteoblast and osteoclast ferroptosis is a potential treatment option for OP.
Osteosarcoma (OS) is a malignant bone tumor arising from mesenchymal cells and is mostly primary (Yu and Yao, 2024). OS can lead to persistent joint pain, limited mobility, and even susceptibility to lung metastases (Zhang et al., 2018). Epidemiological adjustment reveals that the incidence of OS is closely related to age and ethnicity. Primary OS is more common in men and occurs in the long bones of the lower extremities, with large variations in incidence across ethnic groups (Mirabello et al., 2009). In addition, the age of onset tends to be more in adolescents, which may be the result of rapid skeletal growth during adolescence, and OS is therefore more likely to occur at the ends of long bones in adolescents (Mirabello et al., 2011).
Studies have shown that ferroptosis can inhibit OS progression and reduce OS chemoresistance. The status of the System Xc−-GSH-GPX4 axis is critical in OS progression. By inhibiting the demethylation of H3K9me3 at the SLC7A11 promoter region in the System Xc−, the ability of the System Xc− to prevent ferroptosis can be reduced (Chen M. et al., 2021). Osteosarcoma cells are resistant to ferroptosis because of a decrease in P53, one of the tumor suppressor genes. P53 binding to SLC7A11 is inhibited and is the main cause (Wang and Pan, 2023). Zinc finger structure E-box-binding homeobox 1 (ZEB1) is involved in lipid metabolism in vivo. Overexpressed ZEB1 leads to ROS accumulation (Liu et al., 2023). In transcriptomic experiments, mitochondria from the knockdown ZEB1 group showed ferroptosis-like changes and were involved in the ferroptosis process in OS (Jiacong et al., 2023).
NcRNAs are also involved in the process of ferroptosis. It was shown that genes inhibiting ferroptosis were repressed following miR-206 overexpression in OS cell lines, whereas genes promoting ferroptosis were increased than expression (Li L. et al., 2023). MiR-188-3p targets GPX4 and its expression is reduced in OS tissues, which contributes directly to ferroptosis in OS (Li Z. et al., 2023). LncRNAs can modulate OS resistance and resist OS metastasis (Argenziano et al., 2021). Despite the fact that circRNAs development and cognition are still in their infancy, there is evidence linking circRNAs to tumor metastasis and progression (Zhang et al., 2019). It has been shown that circBLNK and GPX4 are significantly upregulated in OS tissues, promote OS progression, and avoid OS cell ferroptosis (Li Z. et al., 2023).
As a degenerative disease, IVDD is the main cause of cervical and low back pain, and about 80% of low back pain is related to IVDD (Li et al., 2024b). Nucleus pulposus (NP) and annulus fibrosus (AF) are the main components of the intervertebral disc and are the main responsible for intervertebral disc function (Le Maitre et al., 2015). Although IVDD is an age-related degenerative disease, current studies suggest that ferroptosis plays an important role in it.
Ferroptosis is involved in IVDD through multiple pathways. The first is to affect normal physiological function by interfering with iron metabolism. Patients’ disc tissue showed reduced expression of FTH (Yang et al., 2021b). Additionally, there was an iron-dose dependent degeneration of cartilage endplates (Wang W. et al., 2022). When FPN is dysfunctional in IVDD, intracellular iron is excessive, which aggravates ferroptosis-induced IVDD (Lu et al., 2021). Some small molecule compounds, such as amino acids, enzymes, and transcription factors, which target the regulation of lipid metabolism and anti-oxidation are also involved in the process of ferroptosis in IVDD. Homocysteine (Hcy), derived from methionine and cysteine, is an important substance in cellular physiology (Mudd et al., 1985). Hcy can cause many musculoskeletal diseases through cellular ferroptosis (Koh et al., 2006; Fayfman et al., 2009). Epidemiological investigation, hyperhomocysteinemia is an important risk factor for IVDD. Inhibition of GPX4 methylation prevented Hcy-guided oxidative stress and ferroptosis (Fan et al., 2023). Activating transcription factor 3 (ATF3) is a member of the ATF/CREB family of transcription factors responsible for regulating signaling pathways and cellular metabolism (Rohini et al., 2018). It has now been demonstrated that ATF3 is a positive regulator of ferroptosis (Wang et al., 2020). In tumor cells, ATF3 can inhibit System Xc− expression, and inhibit GPX4 and induce ferroptosis (Wang Y. et al., 2021). Results of a bioinformatics experiment revealed that ATF3 gene differences were located in the fourth place in the hub ferroptosis gene ranking in the spinal cord injury model (Gupta et al., 2023). And clinical observations have also found that ATF3 is highly expressed in IVDD, and the mechanism is through the inhibition of SLC7A11 and SOD2 (Li Y. et al., 2022).
Angiogenesis of vascularized granulation tissue is a major feature of IVDD, and much neovascularization is also responsible for keyboard tissue degeneration. Angiogenic vascularized granulation tissue is a major feature of IVDD, and many new vessels are found in NP and are also responsible for disc tissue degeneration (Xiao et al., 2020). As such, hemoglobin numbers were significantly higher in NP than in other surrounding tissues. When IVDD occurs, the iron content in NP is too high, which is the main cause of aggravated ferroptosis (Shan et al., 2021). Notably, HO-1 has a dual regulatory role in ferroptosis. As an antioxidant, it can inhibit ferroptosis, but at the same time, it is characterized by iron concentration dependence, which activates ferroptosis at high iron content (Fang et al., 2019; Adedoyin et al., 2018). A simultaneous increase in HO-1 and iron accumulation was found in the rat IVDD model (Zhang et al., 2021), and clinical studies have also confirmed increased HO-1 expression in NP(358). Such results are strongly associated with neovascularization. As one of the regulatory heme transcription factors, BTB Domain And CNC Homolog 1 (BACH1) expression is closely associated with IVDD. In vivo experiments confirmed that knockdown of BACH1 could increase GPX4 and SLC7A11 expression in IVDD, thereby inhibiting ferroptosis (Yao et al., 2023). Sirtuin 3 (Sirt3) is a critical regulator of ROS. High expression of ubiquitin-specific protease 11 (USP11) alleviates ferroptosis caused by oxidative stress. Sirt3 has been found to increase oxidative stress and induce ferroptosis to promote IVDD, while USP11 can bind to Sirt3 and stabilize Sirt3 to slow IVDD progression (Zhu et al., 2023a).
Epigenetics also regulates ferroptosis in IVDD. MiR-10A-5p mediated overexpression of IL-6R in disc cartilage is responsible for ferroptosis in IVDD (Bin et al., 2021). Circ0072464 downregulation and miR-431 upregulation were observed in IVDD, and this result triggered high NRF2 expression, thereby promoting NP proliferation to alleviate IVDD and improve prognosis (Yu et al., 2022). MiR-672-3p promotes ferroptosis during spinal cord injury by downregulating ferroptosis suppressor protein 1 (FSP1) (Wang F. et al., 2022). As a competitive RNA for miR-5627-5p, lncGm36569 induced upregulation of FSP1 to alleviate ferroptosis.
According to the characteristics of ferroptosis and its mechanism of action in bone diseases, it is necessary to find suitable and efficient treatment options for bone diseases caused by ferroptosis. According to the previous discussion, it is known that antioxidant disorders and iron metabolism disorders are the underlying mechanisms of ferroptosis, so therapeutic regimens targeting these two aspects are worthy of in-depth study. Currently relevant treatment options have been reported several times, but an overview of their overall is lacking. Therefore, we try to summarize the current treatment ideas through the content of this part, hoping to help the experimental and clinical application.
Cartilage damage is a cardinal feature of OA. As mentioned above, cartilage damage is caused by nothing more than abnormal ROS production and iron metabolism. Regulation of iron metabolism can affect chondrocyte survival. Iron chelators are drugs that are effective against diseases caused by iron accumulation. Iron load is a marker of ferroptosis, so rational use of iron chelators is an effective treatment to cope with ferroptosis. For clinical application, three iron chelators—Deferoxamine (DFO), deferasirox (DFX), and deferiprone (DFP)—have been approved (Mobarra et al., 2016). DFO is an iron chelator approved by the US Food and Drug Administration. DFO was shown to prevent IL-1β-induced upregulation of matrix metallopeptidase 13 (MMP13), and this result also indirectly confirmed that iron is involved in chondrocyte apoptosis in OA (Jing et al., 2020). DFO can also reverse MMP13 activation triggered by erastin, an ferroptosis inducer, and reduce protection against chondrocyte injury by promoting NRF2 pathway activation (Guo et al., 2022). In addition, after DFO treatment, cartilage under hypoxia showed higher ultimate tensile strength and pyridinoline (a collagen protein of mature articular cartilage), although the aim of this finding was to evaluate the mechanical properties of new cartilage, it can also be seen from the results that regulating iron content has far-reaching significance for cartilage tissue (Otarola et al., 2022). In a mouse model, it was found that by intra-articular injection of ferrostatin-1 (an ferroptosis inhibitor), the NRF2 system is activated, attenuating IL-1β-induced ROS accumulation and relieving chondrocyte breakdown, which is a new way to treat OA (250). Another animal experiment also demonstrated that mitochondrial morphology was restored in chondrocytes undergoing ferroptosis after joint injection of ferrostatin-1 and astaxanthin, and collagen II was upregulated due to attenuation of IL-1β (Wang et al., 2022e). Hypoxic environment impacts the body in a complex state, as mentioned above. Several recent studies have focused on the key role of HIF in OA progression (Gonzalez et al., 2018). D-mannose, an isomer of glucose, has been reported to inhibit LPS-induced IL-1β production, which is considered an effective treatment for OA (Torretta et al., 2020). Recent studies have confirmed that D-mannose can inhibit HIF-1α-mediated ferroptosis in chondrocytes (Zhou et al., 2012). HIF-2α is also a non-negligible factor in OA progression. Relevant studies have confirmed that HIF-2α can lead to cartilage destruction by affecting the expression of genes responsible for metabolism in chondrocytes (Saito et al., 2010; Yang et al., 2010; Yang et al., 2015). D-mannose can inhibit HIF-2α to reduce the sensitivity of chondrocytes to ferroptosis (Zhou et al., 2021). Moreover, D-mannose inhibited OA degeneration brought on by IL-1β in rat chondrocytes by triggering autophagy via the AMPK pathway (Lin Z. et al., 2021). Some medicinal ingredients from traditional Chinese medicine are also widely used to treat OA. Icariin, the main component of herb Epimedium, has been demonstrated to reduce the expression of IL-1β, MMP, and GRP78, and its mechanism of action is to activate the System Xc−/GPX4 pathway to inhibit ferroptosis (Luo and Zhang, 2021; Pan et al., 2017). Stigmasterol, the main component of Achyranthes bidentata, also acts on IL-1β to reduce its chondrocyte damage and regulates ferroptosis through sterol regulatory elements combined with transcription factor 2 (Mo et al., 2021). Intra-articular injection of Cardamonin, one of the extracts of ginger, also inhibited IL-1β-mediated cartilage explanation and regulated ferroptosis through the p53 pathway (Gong et al., 2023).
There are few reports on the treatment of AS in ferroptosis, but a number of studies have dominated the prediction of ferroptosis genes closely related to AS. With these predicted genes as primary target points, therapeutic strategies for AS can be identified. Li et al. constructed a protein network of ferroptosis and AS and collected gene expression profiles of AS patients through the GEO database, and concluded that DNA Damage Inducible Transcript 3 and Heat Shock Protein Family B (Small) Member 1 are target genes for inducing ferroptosis in AS cells after enrichment analysis (Li Q. et al., 2022). Another analysis identified Small Ubiquitin Like Modifier 2 and NADH:Ubiquinone Oxidoreductase Subunit S4 as hub genes for ferroptosis in AS cells by constructing differential gene and protein networks (Rong et al., 2022). Dong et al. screened Chloride Intracellular Channel 4 and Tripartite Motif Containing 21 (TRIM21) as key genes in ferroptosis-regulated AS by using a disease prediction model for differential genes involved in cell death, with TRIM21 expression elevated in male patients (Dong et al., 2024). It has been established that acrylamide raises the risk of AS. Several cancers are caused by aflatoxin, which is produced when food is heated. Additionally, it can raise the risk of AS by causing autophagy-dependent ferroptosis. It is therefore advised to limit the sources of acrylamide in meals (Wang H. et al., 2023).
Inflammation and ROS are key factors in the pathogenesis of GA and the mechanism of ferroptosis. Targeting ROS-NLRP3 for GA is the primary strategy. Multiple ROS-NLRP3 blockers have been demonstrated to treat GA (Zhang et al., 2024). Multiple natural medicines have proven effective in treating GA. Carvacrol blocked ROS-NLRP3-mediated inflammation, decreased oxidative stress, and decreased uric acid levels in GA patients (Riaz et al., 2022). The natural flavonoid compound rutin was demonstrated to inhibit ROS production and inhibit ROS-NLRP3 inflammatory activation thereby improving joint swelling in a quail GA model (Wu H. et al., 2023). The non-coding RNA lncRNA ZNF883 is one of the key genes identified as ferroptosis leading to GA (Shao et al., 2024). Targeting lncRNA Zinc Finger Protein 883 to treat GA is therefore a drug worth investigating. Targeted modulation of XO is another way. DEP Domain Containing 5 (DEPDC5) subunit deficiency can lead to increased XO and ROS accumulation, which leads to ferroptosis (Li S. et al., 2024). Targeting DEPDC5 pharmaceuticals may be one way to treat GA. Drugs observed from models in which non-ferroptosis leads to GA also decrease XO levels, suggesting that these are potential agents for the treatment of GA. Artemisia argyi essential oil could decrease XO and upregulate GPX4 in the hepatic ferroptosis model induced by bisphenol A (Cui et al., 2023). Empagliflozin, a selective inhibitor of sodium-glucose cotransporter 2, alleviated doxorubicin-induced myocardial ferroptosis and decreased XO expression (Quagliariello et al., 2021). The results of transcriptomic and metabolomic analysis showed that exposure to PM2. Five environment reduced antioxidant capacity, increased XO expression, and ultimately led to ferroptosis in mice. Avoiding PM2. Five is therefore also one of the ways to prevent GA resulting from ferroptosis (Shi et al., 2022).
Inhibition of synovial hyperplasia to restore synovial homeostasis is an effective treatment for RA (Sandhu and Thelma, 2022). Antioxidant dysregulation due to FLS is a risk factor for RA. Promoting ferroptosis in FLS has therefore emerged as a way to treat RA. Imidazolone erastin (IKE) and the TNF antagonist etanercept induced ferroptosis in FLS and reduced RA symptoms (Wu et al., 2022). Glycine can promote FLS ferroptosis through S-adenosylmethionine mediated methylation of the GPX4 promoter (Ling et al., 2022). Asiatic acid can die from its FLS iron by increasing Fe2+ (Sun et al., 2024). Quercetin is a natural flavonoid, and cells treated by quercetin not only showed inhibition of FLS proinflammatory ability, but also showed that caspase-8 levels, a marker of ferroptosis, could be reduced (Zheng Q. et al., 2023). In addition to natural medicines, drugs targeting FLS modulation have now been developed and proven effective. Cathepsin B is a protease involved in joint injury and is highly expressed in articular cartilage in RA. Its inhibitor CA-074Me inhibited FLS proliferation and promoted FLS ferroptosis (Luo et al., 2024). Sulfasalazine, as a treatment for AS, has been shown to promote ferroptosis in FLS in RA (Zhao et al., 2024). Several differential gene analyses yielded genes involved in regulating ferroptosis in FLS. Several differential gene analyses yielded genes involved in regulating ferroptosis in FLS. Wang et al. analyzed eight ferroptosis genes associated with RA, of which TIMP Metallopeptidase Inhibitor 1 was significantly expressed in FLS (Wang et al., 2024b). Jing et al. identified SLC2A3 as highly expressed in FLS by bioinformatics methods and machine learning algorithms and experimentally verified that FLS treated with RSL3 exhibited SLC2A3 downregulation and underwent ferroptosis (Xiang et al., 2023). Therefore, it is well documented to treat differential genes. Nuclear Receptor Coactivator 4 (NCOA4) mediates LPS-induced ferroptosis in FLS and targeting NCOA4 may be an effective strategy for the treatment of RA (Wang Y. et al., 2024).
In addition to targeting FLS, ways to interfere with ferroptosis for RA have also been mostly reported. An injectable gel composed of folic acid-functionalized polydopamine and leonurine (Leon) inhibits joint inflammation caused by macrophages and protects cartilage from ferroptosis (Lv et al., 2024). It is a novel way to treat RA by carrying Fe3O4 and sulfasalazine by using macrophages as carriers (Ruan et al., 2024). In this experiment, macrophage carriers could be transported to sites of RA inflammation guided by inflammatory factors and under near-infrared light irradiation, Fe3O4 converted light energy to heat energy. This synergistic effect, instead, predisposes inflammatory cells and proliferating synovium to ferroptosis, thereby achieving the effect of treating RA.
BMSCs are an important source of OBs and OCs differentiation is influenced by RANKL. When OP develops, OBs differentiation is inhibited, and OCs differentiation is enhanced. Targeting BMSCs and RANKL is therefore a way to treat OP. Ferroptosis has been mostly reported to affect BMSCs and RANKL, and OP based on ferroptosis is a hot area of current research.
As a naturally occurring phenolic chemical, Picein enhances BMSCs’ capacity for osteogenic differentiation while reducing oxidative stress caused by erastin via the Nrf2/HO-1/GPX4 pathway (Huang et al., 2024a). Overexpression of Crystallin Alpha B (CRYAB) increased OCN and Runx2 expression and increased ALP activity in BMSCs. Further experiments verified that CRYAB could interact with FTH1, inhibit ferroptosis of BMSCs, and promote osteogenic differentiation (Tian et al., 2024). BMSCs induced by high glucose and high fat environments exhibited bone degradation and ferroptosis, but this was reversed by poliumoside. When poliumoside was used in the T2DOP mouse model, increased bone mineral density and GPX4 expression were observed in the distal femur of mice, which also confirmed its effectiveness with cellular experiments (Xu et al., 2024). Based on a DNA tetrahedral nanoparticle involved in curcumin, tFNA-Cur, could inhibit ferroptosis in BMSCs and promote osteogenic differentiation in diabetic environment through Nrf2/GPX4 pathway (Li et al., 2024d). Ebselen is a selenium-containing organic drug molecule that can act as a mimetic of GPX. The experiment verified that Ebselen improved the ferroptosis and osteogenic differentiation inhibition status of BMSCs induced by LPS (Huang Z. et al., 2023). Engeletin, as an endogenous antioxidant, can promote osteogenic differentiation of BMSCs and upregulate osteogenesis-related proteins, which has achieved the effect of counteracting ferroptosis (Huang L. et al., 2023). Tocopherol as an antioxidant can reduce oxidative stress in BMSCs, promote osteogenesis-related protein expression, and inhibit ferroptosis in BMSCs (Lan et al., 2022b). Vitamin K2 has been used clinically to prevent OP. In cell experiments, vitamin K2 reversed ferroptosis and upregulated osteogenic marker expression in BMSCs under high glucose conditions (Jin et al., 2023).
In addition, novel medical devices have been developed to target the inhibition of osteogenic differentiation caused by ferroptosis. Targeted regulation of BMSCs metabolic status is a critical point to promote osteoblast differentiation. Therapeutic protocols targeting BMSCs metabolism were therefore designed and experimentally confirmed. Yang designed a titanium implant coated with caffeic acid and DFO. Titanium implants were implanted into the femoral epiphysis of OVX rats, and it was observed that titanium implants promoted new bone formation after 1 month. Its mechanism of action is to reduce the lipid peroxidation level of BMSCs by activating the KEAP1/NRF2/HMOX1 pathway and to activate and promote the SLC7A11/GSH/GPX4 axis to inhibit BMSCs ferroptosis (Yang Y. et al., 2024). Bone cement is commonly used to treat OP fractures. However, its cytotoxic properties make it likely to have an impact on osteogenic differentiation. A composite PDT-TCP-SE based on polylactide-based copolymer (PDT), β-tricalcium phosphate (β-TCP), and selenium nanoparticles (SeNPs) was developed to replace the application of traditional bone cements. It was found that PDT-TCP-SE could protect BMSCs from erastin-induced ferroptosis through Sirt1/Nrf2/GPX4 antioxidant pathway and had the effect of regulating new osteogenesis at OP fracture site (Huang et al., 2024b).
Saikosaponin A, a component of the natural medicine Bupleurum falcatum, can inhibit RANKL-induced OCs production and inhibit the Nrf2/SCL7A11/GPX4 axis to promote ferroptosis in OCs (Li TQ. et al., 2024). Zoledronic acid is a bisphosphonate that blocks ferrostatin-1 ‘s ability to induce osteoclast death. Moreover, the expression of ferroptosis-related expression in OCs treated with zoledronic acid was significantly increased, and these results indicated that zoledronic acid could puncture osteoclast ferroptosis (Qu et al., 2021). Further studies with zoledronic acid have found that it is through inhibition of the RANKL signaling pathway that OCs production is inhibited and bone loss is relieved (Wang B. et al., 2022). Artemisinin has been shown to downregulate RANKL-induced differentiation of OCs and has been used in place of treating bone loss caused by OCs. Because of the high iron content in OCs, the mechanism of action of artemisinin was identified as possibly associated with ferroptosis (Zhang, 2020).
Osteocyte metabolism affects OP caused by ferroptosis is rarely reported, however, as the most abundant cells in bone, regulating osteocyte metabolism is undoubtedly a worthwhile attempt. Activation of Activating Transcription Factor 2 (ATF2) has been found to induce ferroptosis in osteocytes, a phenomenon that is inextricably linked to age-related bone loss. AFT2 expression was suppressed and OP progression was slowed by administration of JY-2, a novel Forkhead Box O1 inhibitor (Yin et al., 2024). Eldecalcitol is an orally active vitamin D analogue (Sanford and McCormack, 2011). Eldecalcitol showed protection against bone in OVX mice induced by D-galactose. Further cell experiments confirmed that Eldecalcitol alleviated D-galactose-triggered ferroptosis, inhibited lipid peroxide accumulation, and enhanced GPX4 expression in MLO-Y4 cells (Fu et al., 2024).
In conclusion, ferroptosis can accelerate OP progression by affecting the osteogenic differentiation of BMSCs, target inhibition of ferroptosis in BMSCs, or promote ferroptosis in OCs induced by RANKL, which is a strategy worth applying for the treatment of OP.
The treatment regimen of OS is consistent with conventional cancer treatment, that is, surgery, radiotherapy, chemotherapy and other modalities. However, due to its high incidence of lung metastasis, drug resistance and other characteristics, resulting in OS treatment effect is unsatisfactory. Induction of ferroptosis in tumor cells is currently the focus of treatment options, which also brings new perspectives for the treatment of OS. Multiple agents giving mechanisms of ferroptosis have been demonstrated to inhibit OS progression. EF24, an analog of curcumin, induced osteosarcoma cell death, the outcome of which was reversed by ferrostatin-1. In-depth studies have confirmed that it can increase MDA levels, ROS levels and increase intracellular iron content. HMOX1 expression was upregulated in a dose-dependent manner and promoted ferroptosis in osteosarcoma (Lin H. et al., 2021). The combination of ursolic acid and cisplatin induced intracellular overload of Fe3+, resulting in ferroptosis in osteosarcoma cells (Tang et al., 2021). Tipazamine can inhibit the expression of GPX4 and SLC7A11 under hypoxia and thus induce ferroptosis (Shi et al., 2021). Sulfasalazine and miR-1287-5p mimics could inhibit GPX4 to promote ferroptosis in osteosarcoma cells (Xu Z. et al., 2021; Liu J. et al., 2022). Bavachin, as a flavonoid compound, can promote ferroptosis in osteosarcoma cells by up-regulating p53, and down-regulating SLC7A11 and GPX4 (Luo et al., 2021). Several novel nanomedicines also exert a role in promoting ferroptosis in OS cells (Fu et al., 2021; Wang Y. et al., 2022). Ferroptosis is also involved in reducing drug resistance in osteosarcoma. miR-1287-5p rendered osteosarcoma cells more sensitive to cisplatin (Xu Z. et al., 2021). The same result was found in osteosarcoma cells after inhibition of Lysine Demethylase 4A expression (Chen M. et al., 2021). Combination of erastin, RSL3 and STAT3 inhibitors also increased sensitivity to cisplatin (Liu and Wang, 2019).
NP cell reduction or death is the main cause of IVDD. Several studies have now been directed at inhibiting NP ferroptosis to maintain its normal physiological state. Shu et al. determined that Tinoridine could rescue RSL3-induced ferroptosis in NP cells by increasing Nrf2 expression by screening nonsteroidal anti-inflammatory drugs (Yang S. et al., 2024). Fisetin is involved in the regulation of the Nrf2/HO-1 pathway, thereby inhibiting NP ferroptosis (Li C. et al., 2024). Hesperidin can enhance Nrf2 expression and inhibit NF-κB, thereby alleviating ferroptosis resulting from oxidative stress in NP (Zhu et al., 2023b). HIF-1α promotes translation of SLC7A11 and reduces NP ferroptosis under hypoxia by inducing expression of the m6A reading protein YTHDF1 (Lu et al., 2024). DNA methyltransferase inhibitors prevented puncture-induced IVDD and protected NP from ferroptosis (Chen J. et al., 2024). In vivo experiments confirmed that targeting the miR-874-3p/ATF3 axis could modulate NP ferroptosis and is an effective way to treat IVDD (Wang X. et al., 2024). Circ-STC2 is a critical circRNAs involved in IVDD (Chang et al., 2021). In cell experiments against Circ-STC2, Circ-STC2 was found to be highly expressed in IVDD tissues. Knockdown of Circ-STC2 promoted NP cell viability and prevented from suffering ferroptosis (Xiong et al., 2023). A hydrogel containing SLC7A11-modRNA inhibited ferroptosis in NP cells by local injection, and the rate of SLC7A11-modRNA release positively correlated with IVDD severity (Gao et al., 2024).
Ferroptosis, a programmed cell death that is significantly different from other modes of cell death, is currently the focus of research in the field of disease mechanisms. The mechanism of ferroptosis mainly involves lipid peroxidation, iron accumulation and antioxidant system, which makes it independent of the mode of cell death. Because of this, diseases associated with ferroptosis can be found in various tissues and organs of the human body. The occurrence of bone disease is closely related to the physiological status of bone. Abnormal physiological metabolism of cells in bone tissue can cause various types of bone diseases. Ferroptosis can be involved in any ring of metabolism in cells. Multiple core regulators involved in ferroptosis, such as GSH, GPX4, System Xc−, Nrf2, and ROS, have also been demonstrated to be involved in the development of skeletal diseases.
However, it is undeniable that although iron death has been increasingly studied in the field of orthopedics, it still faces non-negligible challenges and limitations in clinical application. Iron death may have different effects on osteoblasts and osteoclasts, either protecting bone health or exacerbating bone loss under certain conditions. This dual effect makes it difficult to precisely regulate the degree and balance of ferroptosis in treatment. Current studies on iron death mostly focus on tumor cells and other non-skeletal tissues, and there is still a lack of in-depth studies targeting skeletal-related cells such as osteoblasts, osteoclasts, and chondrocytes. Susceptibility to ferroptosis and mechanisms may differ between cell types. Accumulation of iron in skeletal cells may lead to cellular dysfunction or even induce apoptosis, which is not conducive to bone health. In addition, regulation of iron metabolism by exogenous means may lead to altered systemic iron load and trigger adverse effects in other organs and tissues, such as iron accumulation in the liver and heart. In addition, the response to ferroptosis regulation may vary widely between individual patients, and different bone disease types (e.g., osteoarthritis versus osteoporosis) and disease duration stages respond differently to iron death interventions. Therefore, how to develop a precise ferroptosis regulation program according to the individual condition of patients is a major challenge for future application.
In this paper, we try to summarize the current research progress of ferroptosis in detail by elaborating the development process of ferroptosis, the intrinsic mechanism, and the relationship with other cell death modes. The mechanism of ferroptosis in many bone diseases is also listed. Finally, the current research on the treatment of bone diseases by affecting ferroptosis is summarized. Because ferroptosis involves a wide range of fields and complex mechanisms, research on the effects of ferroptosis on bone disease remains to be continued. We hope to provide efficient and safe treatment modalities and preventive strategies for clinical as well as scientific research through this paper and further research in the future.
GH: Writing–original draft. YuL: Writing–original draft. LL: Writing–original draft. YH: Writing–original draft. YQ: Writing–original draft. YaL: Writing–original draft. RZ: Writing–original draft. BW: Writing–original draft. QG: Writing–original draft, Writing–review and editing. ZH: Writing–original draft, Writing–review and editing. HY: Writing–original draft, Writing–review and editing.
The author(s) declare that no financial support was received for the research, authorship, and/or publication of this article.
The authors declare that the research was conducted in the absence of any commercial or financial relationships that could be construed as a potential conflict of interest.
The author(s) declare that no Generative AI was used in the creation of this manuscript.
All claims expressed in this article are solely those of the authors and do not necessarily represent those of their affiliated organizations, or those of the publisher, the editors and the reviewers. Any product that may be evaluated in this article, or claim that may be made by its manufacturer, is not guaranteed or endorsed by the publisher.
Adedoyin, O., Boddu, R., Traylor, A., Lever, J. M., Bolisetty, S., George, J. F., et al. (2018). Heme oxygenase-1 mitigates ferroptosis in renal proximal tubule cells. Am. J. Physiol. Ren. Physiol. 314 (5), F702-F714–f14. doi:10.1152/ajprenal.00044.2017
Agyeman, A. S., Chaerkady, R., Shaw, P. G., Davidson, N. E., Visvanathan, K., Pandey, A., et al. (2012). Transcriptomic and proteomic profiling of Keap1 disrupted and sulforaphane-treated human breast epithelial cells reveals common expression profiles. Breast Cancer Res. Treat. 132 (1), 175–187. Epub 20110520. doi:10.1007/s10549-011-1536-9
Anderson, G. J. (2007). Mechanisms of iron loading and toxicity. Am. J. Hematol. 82 (12 Suppl. l), 1128–1131. doi:10.1002/ajh.21075
Anderson, G. J., and Vulpe, C. D. (2009). Mammalian iron transport. Cell Mol. Life Sci. 66 (20), 3241–3261. Epub 20090531. doi:10.1007/s00018-009-0051-1
Angeli, J. P. F., Shah, R., Pratt, D. A., and Conrad, M. (2017). Ferroptosis inhibition: mechanisms and opportunities. Trends Pharmacol. Sci. 38 (5), 489–498. Epub 20170328. doi:10.1016/j.tips.2017.02.005
Arai, M., Imai, H., Koumura, T., Yoshida, M., Emoto, K., Umeda, M., et al. (1999). Mitochondrial phospholipid hydroperoxide glutathione peroxidase plays a major role in preventing oxidative injury to cells. J. Biol. Chem. 274 (8), 4924–4933. doi:10.1074/jbc.274.8.4924
Argenziano, M., Tortora, C., Pota, E., Di Paola, A., Di Martino, M., Di, L. C., et al. (2021). Osteosarcoma in children: not only chemotherapy. Pharm. (Basel) 14 (9), 923. Epub 20210913. doi:10.3390/ph14090923
Asada-Senju, M., Maeda, T., Sakata, T., Hayashi, A., and Suzuki, T. (2002). Molecular analysis of the transferrin gene in a patient with hereditary hypotransferrinemia. J. Hum. Genet. 47 (7), 355–359. doi:10.1007/s100380200049
Ashton, T. M., McKenna, W. G., Kunz-Schughart, L. A., and Higgins, G. S. (2018). Oxidative phosphorylation as an emerging target in cancer therapy. Clin. Cancer Res. 24 (11), 2482–2490. Epub 20180202. doi:10.1158/1078-0432.Ccr-17-3070
Bali, P. K., Zak, O., and Aisen, P. (1991). A new role for the transferrin receptor in the release of iron from transferrin. Biochemistry 30 (2), 324–328. doi:10.1021/bi00216a003
Balogh, E., Tolnai, E., Nagy, B., Nagy, B., Balla, G., Balla, J., et al. (2016). Iron overload inhibits osteogenic commitment and differentiation of mesenchymal stem cells via the induction of ferritin. Biochim. Biophys. Acta 1862 (9), 1640–1649. Epub 20160607. doi:10.1016/j.bbadis.2016.06.003
Bannai, S., Tsukeda, H., and Okumura, H. (1977). Effect of antioxidants on cultured human diploid fibroblasts exposed to cystine-free medium. Biochem. Biophys. Res. Commun. 74 (4), 1582–1588. doi:10.1016/0006-291x(77)90623-4
Bansal, A., and Simon, M. C. (2018). Glutathione metabolism in cancer progression and treatment resistance. J. Cell Biol. 217 (7), 2291–2298. Epub 20180618. doi:10.1083/jcb.201804161
Barneda-Zahonero, B., and Parra, M. (2012). Histone deacetylases and cancer. Mol. Oncol. 6 (6), 579–589. Epub 20120827. doi:10.1016/j.molonc.2012.07.003
Barrat, F. J., Crow, M. K., and Ivashkiv, L. B. (2019). Interferon target-gene expression and epigenomic signatures in health and disease. Nat. Immunol. 20 (12), 1574–1583. Epub 20191119. doi:10.1038/s41590-019-0466-2
Baschant, U., Altamura, S., Steele-Perkins, P., Muckenthaler, M. U., Spasić, M. V., Hofbauer, L. C., et al. (2022). Iron effects versus metabolic alterations in hereditary hemochromatosis driven bone loss. Trends Endocrinol. Metab. 33 (9), 652–663. Epub 20220720. doi:10.1016/j.tem.2022.06.004
Bernstein, S. E. (1987). Hereditary hypotransferrinemia with hemosiderosis, a murine disorder resembling human atransferrinemia. J. Lab. Clin. Med. 110 (6), 690–705.
Bersuker, K., Hendricks, J. M., Li, Z., Magtanong, L., Ford, B., Tang, P. H., et al. (2019). The coq Oxidoreductase Fsp1 acts parallel to Gpx4 to inhibit ferroptosis. Nature 575 (7784), 688–692. Epub 20191021. doi:10.1038/s41586-019-1705-2
Bertheloot, D., Latz, E., and Franklin, B. S. (2021). Necroptosis, pyroptosis and apoptosis: an intricate game of cell death. Cell Mol. Immunol. 18 (5), 1106–1121. Epub 20210330. doi:10.1038/s41423-020-00630-3
Beutler, E., Gelbart, T., Lee, P., Trevino, R., Fernandez, M. A., and Fairbanks, V. F. (2000). Molecular characterization of a case of atransferrinemia. Blood 96 (13), 4071–4074. doi:10.1182/blood.v96.13.4071.h8004071_4071_4074
Bhat, V., Olmer, M., Joshi, S., Durden, D. L., Cramer, T. J., Barnes, R. F., et al. (2015). Vascular remodeling underlies rebleeding in hemophilic arthropathy. Am. J. Hematol. 90 (11), 1027–1035. Epub 20151008. doi:10.1002/ajh.24133
Bin, S., Xin, L., Lin, Z., Jinhua, Z., Rui, G., and Xiang, Z. (2021). Targeting mir-10a-5p/il-6r Axis for reducing il-6-induced cartilage cell ferroptosis. Exp. Mol. Pathol. 118, 104570. Epub 20201107. doi:10.1016/j.yexmp.2020.104570
Björnstedt, M., Hamberg, M., Kumar, S., Xue, J., and Holmgren, A. (1995). Human thioredoxin reductase directly reduces lipid hydroperoxides by nadph and selenocystine strongly stimulates the reaction via catalytically generated selenols. J. Biol. Chem. 270 (20), 11761–11764. doi:10.1074/jbc.270.20.11761
Bläss, S., Union, A., Raymackers, J., Schumann, F., Ungethüm, U., Müller-Steinbach, S., et al. (2001). The stress protein bip is overexpressed and is a major B and T cell target in rheumatoid arthritis. Arthritis Rheum. 44 (4), 761–771. doi:10.1002/1529-0131(200104)44:4<761::Aid-anr132>3.0.Co;2-s
Borner, C., and Monney, L. (1999). Apoptosis without caspases: an inefficient molecular guillotine? Cell Death Differ. 6 (6), 497–507. doi:10.1038/sj.cdd.4400525
Boyce, B. F. (2013). Advances in the regulation of osteoclasts and osteoclast functions. J. Dent. Res. 92 (10), 860–867. Epub 20130801. doi:10.1177/0022034513500306
Brashears, C. B., Prudner, B. C., Rathore, R., Caldwell, K. E., Dehner, C. A., Buchanan, J. L., et al. (2022). Malic enzyme 1 absence in synovial sarcoma shifts antioxidant system dependence and increases sensitivity to ferroptosis induction with acxt-3102. Clin. Cancer Res. 28 (16), 3573–3589. doi:10.1158/1078-0432.Ccr-22-0470
Burton, G. W., and Ingold, K. U. (1981). Autoxidation of biological molecules. 1. Antioxidant activity of vitamin E and related chain-breaking phenolic antioxidants in vitro. J. Am. Chem. Soc. 103 (21), 6472–6477. doi:10.1021/ja00411a035
Burton, L. H., Radakovich, L. B., Marolf, A. J., and Santangelo, K. S. (2020). Systemic iron overload exacerbates osteoarthritis in the strain 13 Guinea pig. Osteoarthr. Cartil. 28 (9), 1265–1275. Epub 20200703. doi:10.1016/j.joca.2020.06.005
Cai, C., Hu, W., and Chu, T. (2021). Interplay between iron overload and osteoarthritis: clinical significance and cellular mechanisms. Front. Cell Dev. Biol. 9, 817104. Epub 20220114. doi:10.3389/fcell.2021.817104
Cain, T. J., and Smith, A. T. (2021). Ferric iron reductases and their contribution to unicellular ferrous iron uptake. J. Inorg. Biochem. 218, 111407. doi:10.1016/j.jinorgbio.2021.111407
Carlson, B. A., Tobe, R., Yefremova, E., Tsuji, P. A., Hoffmann, V. J., Schweizer, U., et al. (2016). Glutathione peroxidase 4 and vitamin E cooperatively prevent hepatocellular degeneration. Redox Biol. 9, 22–31. Epub 20160526. doi:10.1016/j.redox.2016.05.003
Cavalli, G., and Heard, E. (2019). Advances in epigenetics link genetics to the environment and disease. Nature 571 (7766), 489–499. Epub 20190724. doi:10.1038/s41586-019-1411-0
Cavdar, Z., Oktan, M. A., Ural, C., Calisir, M., Kocak, A., Heybeli, C., et al. (2020). Renoprotective effects of alpha lipoic acid on iron overload-induced kidney injury in rats by suppressing nadph oxidase 4 and P38 mapk signaling. Biol. Trace Elem. Res. 193 (2), 483–493. Epub 20190425. doi:10.1007/s12011-019-01733-3
Chang, H., Wang, H., Yang, X., You, K., Jiang, M., Cai, F., et al. (2021). Comprehensive profile analysis of differentially expressed circrnas in glucose deprivation-induced human Nucleus pulposus cell degeneration. Biomed. Res. Int. 2021, 4770792. Epub 20210702. doi:10.1155/2021/4770792
Chen, C., Huang, Y., Xia, P., Zhang, F., Li, L., Wang, E., et al. (2021c). Long noncoding rna Meg3 mediates ferroptosis induced by oxygen and glucose deprivation combined with hyperglycemia in rat brain microvascular endothelial cells, through modulating the P53/gpx4 Axis. Eur. J. Histochem 65 (3), 3224. Epub 20210930. doi:10.4081/ejh.2021.3224
Chen, F., Kang, R., Tang, D., and Liu, J. (2024a). Ferroptosis: principles and significance in health and disease. J. Hematol. Oncol. 17 (1), 41. Epub 20240606. doi:10.1186/s13045-024-01564-3
Chen, G., Wu, K., Li, H., Xia, D., and He, T. (2022b). Role of hypoxia in the tumor microenvironment and targeted therapy. Front. Oncol. 12, 961637. Epub 20220923. doi:10.3389/fonc.2022.961637
Chen, H., Han, Z., Wang, Y., Su, J., Lin, Y., Cheng, X., et al. (2023). Targeting ferroptosis in bone-related diseases: facts and perspectives. J. Inflamm. Res. 16, 4661–4677. Epub 20231018. doi:10.2147/jir.S432111
Chen, J., Yang, X., Li, Q., Ma, J., Li, H., Wang, L., et al. (2024b). Inhibiting DNA methyltransferase Dnmt3b confers protection against ferroptosis in Nucleus pulposus and ameliorates intervertebral disc degeneration via upregulating Slc40a1. Free Radic. Biol. Med. 220, 139–153. Epub 20240503. doi:10.1016/j.freeradbiomed.2024.05.007
Chen, K. W., Demarco, B., Heilig, R., Shkarina, K., Boettcher, A., Farady, C. J., et al. (2019). Extrinsic and intrinsic apoptosis activate pannexin-1 to drive Nlrp3 inflammasome assembly. Embo J. 38 (10), e101638. Epub 20190322. doi:10.15252/embj.2019101638
Chen, M., Jiang, Y., and Sun, Y. (2021d). Kdm4a-Mediated histone demethylation of Slc7a11 inhibits cell ferroptosis in osteosarcoma. Biochem. Biophys. Res. Commun. 550, 77–83. Epub 20210306. doi:10.1016/j.bbrc.2021.02.137
Chen, P. H., Wu, J., Ding, C. C., Lin, C. C., Pan, S., Bossa, N., et al. (2020). Kinome screen of ferroptosis reveals a novel role of atm in regulating iron metabolism. Cell Death Differ. 27 (3), 1008–1022. Epub 20190718. doi:10.1038/s41418-019-0393-7
Chen, W., Fu, J., Chen, Y., Li, Y., Ning, L., Huang, D., et al. (2021b). Circular rna Circkif4a facilitates the malignant progression and suppresses ferroptosis by sponging mir-1231 and upregulating Gpx4 in papillary thyroid cancer. Aging (Albany NY) 13 (12), 16500–16512. Epub 20210621. doi:10.18632/aging.203172
Chen, X., Kang, R., Kroemer, G., and Tang, D. (2021a). Ferroptosis in infection, inflammation, and immunity. J. Exp. Med. 218 (6), e20210518. Epub 2021/05/13. doi:10.1084/jem.20210518
Chen, Y., Yi, X., Huo, B., He, Y., Guo, X., Zhang, Z., et al. (2022a). Brd4770 functions as a novel ferroptosis inhibitor to protect against aortic dissection. Pharmacol. Res. 177, 106122. Epub 20220208. doi:10.1016/j.phrs.2022.106122
Chen, Y. R., and Zweier, J. L. (2014). Cardiac mitochondria and reactive oxygen species generation. Circ. Res. 114 (3), 524–537. doi:10.1161/circresaha.114.300559
Cherukuri, S., Potla, R., Sarkar, J., Nurko, S., Harris, Z. L., and Fox, P. L. (2005). Unexpected role of ceruloplasmin in intestinal iron absorption. Cell Metab. 2 (5), 309–319. doi:10.1016/j.cmet.2005.10.003
Chua, A. C., Graham, R. M., Trinder, D., and Olynyk, J. K. (2007). The regulation of cellular iron metabolism. Crit. Rev. Clin. Lab. Sci. 44 (5-6), 413–459. doi:10.1080/10408360701428257
Chung, S., Kim, S., Son, M., Kim, M., Koh, E. S., Shin, S. J., et al. (2019). Inhibition of P300/cbp-associated factor attenuates renal tubulointerstitial fibrosis through modulation of nf-kb and Nrf2. Int. J. Mol. Sci. 20 (7), 1554. Epub 20190328. doi:10.3390/ijms20071554
Comità, S., Falco, P., Mezzanotte, M., Vujić Spasić, M., and Roetto, A. (2024). Lack of hfe and Tfr2 in macrophages impairs iron metabolism in the spleen and the bone marrow. Int. J. Mol. Sci. 25 (17), 9142. doi:10.3390/ijms25179142
Conrad, M., and Sato, H. (2012). The oxidative stress-inducible cystine/glutamate antiporter, system X (C) (-): cystine supplier and beyond. Amino Acids 42 (1), 231–246. Epub 20110316. doi:10.1007/s00726-011-0867-5
Corbet, C., Pinto, A., Martherus, R., Santiago de Jesus, J. P., Polet, F., and Feron, O. (2016). Acidosis drives the reprogramming of fatty acid metabolism in cancer cells through changes in mitochondrial and histone acetylation. Cell Metab. 24 (2), 311–323. doi:10.1016/j.cmet.2016.07.003
Cowman, S. J., and Koh, M. Y. (2022). Revisiting the Hif switch in the tumor and its immune microenvironment. Trends Cancer 8 (1), 28–42. Epub 20211104. doi:10.1016/j.trecan.2021.10.004
Craven, C. M., Alexander, J., Eldridge, M., Kushner, J. P., Bernstein, S., and Kaplan, J. (1987). Tissue distribution and clearance kinetics of non-transferrin-bound iron in the hypotransferrinemic mouse: a rodent model for hemochromatosis. Proc. Natl. Acad. Sci. U. S. A. 84 (10), 3457–3461. doi:10.1073/pnas.84.10.3457
Cui, J., Shibata, Y., Zhu, T., Zhou, J., and Zhang, J. (2022). Osteocytes in bone aging: advances, challenges, and future perspectives. Ageing Res. Rev. 77, 101608. Epub 20220311. doi:10.1016/j.arr.2022.101608
Cui, W., Zhou, H., Zhang, J., Zhang, J., Wu, D., Rong, Y., et al. (2023). Hepatoprotective effect of artemisia argyi essential oil on bisphenol a-induced hepatotoxicity via inhibition of ferroptosis in mice. Environ. Toxicol. 38 (10), 2416–2428. Epub 20230622. doi:10.1002/tox.23877
Dai, E., Han, L., Liu, J., Xie, Y., Zeh, H. J., Kang, R., et al. (2020). Ferroptotic damage promotes pancreatic tumorigenesis through a tmem173/sting-dependent DNA sensor pathway. Nat. Commun. 11 (1), 6339. Epub 20201211. doi:10.1038/s41467-020-20154-8
Dautry-Varsat, A., Ciechanover, A., and Lodish, H. F. (1983). Ph and the recycling of transferrin during receptor-mediated endocytosis. Proc. Natl. Acad. Sci. U. S. A. 80 (8), 2258–2262. doi:10.1073/pnas.80.8.2258
Dede, A. D., Trovas, G., Chronopoulos, E., Triantafyllopoulos, I. K., Dontas, I., Papaioannou, N., et al. (2016). Thalassemia-associated osteoporosis: a systematic review on treatment and brief overview of the disease. Osteoporos. Int. 27 (12), 3409–3425. Epub 20160808. doi:10.1007/s00198-016-3719-z
Deng, L., Tian, W., and Luo, L. (2024). Application of natural products in regulating ferroptosis in human diseases. Phytomedicine 128, 155384. Epub 20240128. doi:10.1016/j.phymed.2024.155384
Deng, S. H., Wu, D. M., Li, L., Liu, T., Zhang, T., Li, J., et al. (2021). Mir-324-3p reverses cisplatin resistance by inducing gpx4-mediated ferroptosis in lung adenocarcinoma cell line A549. Biochem. Biophys. Res. Commun. 549, 54–60. Epub 20210301. doi:10.1016/j.bbrc.2021.02.077
Diaz-Vivancos, P., de Simone, A., Kiddle, G., and Foyer, C. H. (2015). Glutathione--Linking cell proliferation to oxidative stress. Free Radic. Biol. Med. 89, 1154–1164. Epub 20151103. doi:10.1016/j.freeradbiomed.2015.09.023
Dickinson, T. K., and Connor, J. R. (1994). Histological analysis of selected brain regions of hypotransferrinemic mice. Brain Res. 635 (1-2), 169–178. doi:10.1016/0006-8993(94)91436-2
Ding, C., Ding, X., Zheng, J., Wang, B., Li, Y., Xiang, H., et al. (2020). Mir-182-5p and mir-378a-3p regulate ferroptosis in I/R-Induced renal injury. Cell Death Dis. 11 (10), 929. Epub 20201028. doi:10.1038/s41419-020-03135-z
Dixon, S. J., Lemberg, K. M., Lamprecht, M. R., Skouta, R., Zaitsev, E. M., Gleason, C. E., et al. (2012). Ferroptosis: an iron-dependent form of nonapoptotic cell death. Cell 149 (5), 1060–1072. doi:10.1016/j.cell.2012.03.042
Dixon, S. J., Patel, D. N., Welsch, M., Skouta, R., Lee, E. D., Hayano, M., et al. (2014). Pharmacological inhibition of cystine-glutamate exchange induces endoplasmic reticulum stress and ferroptosis. Elife 3, e02523. Epub 20140520. doi:10.7554/eLife.02523
Doll, S., Freitas, F. P., Shah, R., Aldrovandi, M., da Silva, M. C., Ingold, I., et al. (2019). Fsp1 is a glutathione-independent ferroptosis suppressor. Nature 575 (7784), 693–698. Epub 20191021. doi:10.1038/s41586-019-1707-0
Doll, S., Proneth, B., Tyurina, Y. Y., Panzilius, E., Kobayashi, S., Ingold, I., et al. (2017). Acsl4 dictates ferroptosis sensitivity by shaping cellular lipid composition. Nat. Chem. Biol. 13 (1), 91–98. Epub 20161114. doi:10.1038/nchembio.2239
Dolma, S., Lessnick, S. L., Hahn, W. C., and Stockwell, B. R. (2003). Identification of genotype-selective antitumor agents using synthetic lethal chemical screening in engineered human tumor cells. Cancer Cell 3 (3), 285–296. doi:10.1016/s1535-6108(03)00050-3
Dong, B. (2018). Protective effects of sinomenine against ankylosing spondylitis and the underlying molecular mechanisms. Med. Sci. Monit. 24, 3631–3636. Epub 20180531. doi:10.12659/msm.907589
Dong, L. H., Li, L., Song, Y., Duan, Z. L., Sun, S. G., Lin, Y. L., et al. (2015). Traf6-Mediated Sm22α K21 ubiquitination promotes G6pd activation and nadph production, contributing to gsh homeostasis and vsmc survival in vitro and in vivo. Circ. Res. 117 (8), 684–694. Epub 20150819. doi:10.1161/circresaha.115.306233
Dong, T., Li, X., Yu, W., Liu, Y., and Yang, J. (2024). Identification of sex-specific biomarkers related to programmed cell death and analysis of immune cells in ankylosing spondylitis. Sci. Rep. 14 (1), 15358. Epub 20240704. doi:10.1038/s41598-024-65745-3
Eagle, H. (1955a). The specific amino acid requirements of a human carcinoma cell (stain hela) in tissue culture. J. Exp. Med. 102 (1), 37–48. doi:10.1084/jem.102.1.37
Eagle, H. (1955b). Nutrition needs of mammalian cells in tissue culture. Science 122 (3168), 501–514. doi:10.1126/science.122.3168.501
Eagle, H. (1959). Amino acid metabolism in mammalian cell cultures. Science 130 (3373), 432–437. doi:10.1126/science.130.3373.432
Eagle, H., Piez, K. A., and Oyama, V. I. (1961). The biosynthesis of cystine in human cell cultures. J. Biol. Chem. 236, 1425–1428. doi:10.1016/s0021-9258(18)64190-0
Ekbote, V., Padidela, R., Khadilkar, V., Ramanan, V., Maheshwari, A., Mughal, Z., et al. (2021). Increased prevalence of fractures in inadequately transfused and chelated Indian children and young adults with beta thalassemia major. Bone 143, 115649. Epub 20200918. doi:10.1016/j.bone.2020.115649
Erba, F., Mei, G., Minicozzi, V., Sabatucci, A., Di Venere, A., and Maccarrone, M. (2024). Conformational dynamics of lipoxygenases and their interaction with biological membranes. Int. J. Mol. Sci. 25 (4), 2241. Epub 20240213. doi:10.3390/ijms25042241
Facchini, F. S. (2003). Near-iron deficiency-induced remission of gouty arthritis. Rheumatol. Oxf. 42 (12), 1550–1555. Epub 20030627. doi:10.1093/rheumatology/keg402
Fan, C., Chu, G., Yu, Z., Ji, Z., Kong, F., Yao, L., et al. (2023). The role of ferroptosis in intervertebral disc degeneration. Front. Cell Dev. Biol. 11, 1219840. Epub 20230727. doi:10.3389/fcell.2023.1219840
Fan, K., Huang, W., Qi, H., Song, C., He, C., Liu, Y., et al. (2021). The egr-1/mir-15a-5p/gpx4 Axis regulates ferroptosis in acute myocardial infarction. Eur. J. Pharmacol. 909, 174403. Epub 20210731. doi:10.1016/j.ejphar.2021.174403
Fang, X., Wang, H., Han, D., Xie, E., Yang, X., Wei, J., et al. (2019). Ferroptosis as a target for protection against cardiomyopathy. Proc. Natl. Acad. Sci. U. S. A. 116 (7), 2672–2680. Epub 20190128. doi:10.1073/pnas.1821022116
Fantone, S., Piani, F., Olivieri, F., Rippo, M. R., Sirico, A., Di Simone, N., et al. (2024). Role of slc7a11/xct in ovarian cancer. Int. J. Mol. Sci. 25 (1), 587. Epub 20240102. doi:10.3390/ijms25010587
Fatima, T., McKinney, C., Major, T. J., Stamp, L. K., Dalbeth, N., Iverson, C., et al. (2018). The relationship between ferritin and urate levels and risk of gout. Arthritis Res. Ther. 20 (1), 179. Epub 20180815. doi:10.1186/s13075-018-1668-y
Fayfman, M., Niu, J., Zhang, Y. Q., Felson, D. T., Sack, B., Aliabadi, P., et al. (2009). The relation of plasma homocysteine to radiographic knee osteoarthritis. Osteoarthr. Cartil. 17 (6), 766–771. Epub 20081130. doi:10.1016/j.joca.2008.11.015
Feltelius, N., Lindh, U., Venge, P., and Hällgren, R. (1986). Ankylosing spondylitis: a chronic inflammatory disease with iron overload in granulocytes and platelets. Ann. Rheum. Dis. 45 (10), 827–831. doi:10.1136/ard.45.10.827
Feng, H., and Stockwell, B. R. (2018). Unsolved mysteries: how does lipid peroxidation cause ferroptosis? PLoS Biol. 16 (5), e2006203. doi:10.1371/journal.pbio.2006203
Feng, X., Yang, Q., Wang, C., Tong, W., and Xu, W. (2020). Punicalagin exerts protective effects against ankylosing spondylitis by regulating nf-κb-Th17/jak2/stat3 signaling and oxidative stress. Biomed. Res. Int. 2020, 4918239. Epub 20200923. doi:10.1155/2020/4918239
Ferreira, H. B., Melo, T., Paiva, A., and Domingues, M. D. R. (2021). Insights in the role of lipids, oxidative stress and inflammation in rheumatoid arthritis unveiled by new trends in lipidomic investigations. Antioxidants (Basel) 10 (1), 45. Epub 20210102. doi:10.3390/antiox10010045
Fiers, W., Beyaert, R., Declercq, W., and Vandenabeele, P. (1999). More than one way to die: apoptosis, necrosis and reactive oxygen damage. Oncogene 18 (54), 7719–7730. doi:10.1038/sj.onc.1203249
Finch, C. (1994). Regulators of iron balance in humans [see comments]. Blood 84 (6), 1697–1702. doi:10.1182/blood.v84.6.1697.bloodjournal8461697
Fischer, R., Trudgian, D. C., Wright, C., Thomas, G., Bradbury, L. A., Brown, M. A., et al. (2012). Discovery of candidate serum proteomic and metabolomic biomarkers in ankylosing spondylitis. Mol. Cell Proteomics 11 (2), M111.013904. Epub 20111013. doi:10.1074/mcp.M111.013904
Fleming, M. D., Romano, M. A., Su, M. A., Garrick, L. M., Garrick, M. D., and Andrews, N. C. (1998). Nramp2 is mutated in the anemic belgrade (B) rat: evidence of a role for Nramp2 in endosomal iron transport. Proc. Natl. Acad. Sci. U. S. A. 95 (3), 1148–1153. doi:10.1073/pnas.95.3.1148
Flohé, L., Toppo, S., and Orian, L. (2022). The glutathione peroxidase family: discoveries and mechanism. Free Radic. Biol. Med. 187, 113–122. doi:10.1016/j.freeradbiomed.2022.05.003
Forman, H. J., Zhang, H., and Rinna, A. (2009). Glutathione: overview of its protective roles, measurement, and biosynthesis. Mol. Asp. Med. 30 (1-2), 1–12. Epub 20080830. doi:10.1016/j.mam.2008.08.006
Förstermann, U., Xia, N., and Li, H. (2017). Roles of vascular oxidative stress and nitric oxide in the pathogenesis of atherosclerosis. Circ. Res. 120 (4), 713–735. doi:10.1161/circresaha.116.309326
Friedmann Angeli, J. P., Schneider, M., Proneth, B., Tyurina, Y. Y., Tyurin, V. A., Hammond, V. J., et al. (2014). Inactivation of the ferroptosis regulator Gpx4 triggers acute renal failure in mice. Nat. Cell Biol. 16 (12), 1180–1191. Epub 20141117. doi:10.1038/ncb3064
Fu, J., Li, T., Yang, Y., Jiang, L., Wang, W., Fu, L., et al. (2021). Activatable nanomedicine for overcoming hypoxia-induced resistance to chemotherapy and inhibiting tumor growth by inducing collaborative apoptosis and ferroptosis in solid tumors. Biomaterials 268, 120537. Epub 20201117. doi:10.1016/j.biomaterials.2020.120537
Fu, S., Lv, R., Wang, L., Hou, H., Liu, H., and Shao, S. (2018). Resveratrol, an antioxidant, protects spinal cord injury in rats by suppressing mapk pathway. Saudi J. Biol. Sci. 25 (2), 259–266. Epub 20161104. doi:10.1016/j.sjbs.2016.10.019
Fu, Y. F., Guo, Y. X., Xia, S. H., Zhou, T. T., Zhao, Y. C., Jia, Z. H., et al. (2024). Eldecalcitol protected osteocytes against ferroptosis of D-gal-induced senescent mlo-Y4 cells and ovariectomized mice. Exp. Gerontol. 189, 112408. Epub 20240323. doi:10.1016/j.exger.2024.112408
Fuchs, Y., and Steller, H. (2015). Live to die another way: modes of programmed cell death and the signals emanating from dying cells. Nat. Rev. Mol. Cell Biol. 16 (6), 329–344. Epub 20150520. doi:10.1038/nrm3999
Gao, M., Monian, P., Pan, Q., Zhang, W., Xiang, J., and Jiang, X. (2016). Ferroptosis is an autophagic cell death process. Cell Res. 26 (9), 1021–1032. Epub 20160812. doi:10.1038/cr.2016.95
Gao, M., Monian, P., Quadri, N., Ramasamy, R., and Jiang, X. (2015). Glutaminolysis and transferrin regulate ferroptosis. Mol. Cell 59 (2), 298–308. Epub 20150709. doi:10.1016/j.molcel.2015.06.011
Gao, M., Yi, J., Zhu, J., Minikes, A. M., Monian, P., Thompson, C. B., et al. (2019a). Role of mitochondria in ferroptosis. Mol. Cell 73 (2), 354–363. Epub 20181220. doi:10.1016/j.molcel.2018.10.042
Gao, M., Yi, J., Zhu, J., Minikes, A. M., Monian, P., Thompson, C. B., et al. (2019b). Role of mitochondria in ferroptosis. Mol. cell 73 (2), 354–363. doi:10.1016/j.molcel.2018.10.042
Gao, T., Xu, G., Ma, T., Lu, X., Chen, K., Luo, H., et al. (2024). Ros-responsive injectable hydrogel loaded with slc7a11-modrna inhibits ferroptosis and mitigates intervertebral disc degeneration in rats. Adv. Healthc. Mater 13, e2401103. Epub 20240501. doi:10.1002/adhm.202401103
Gbyli, R., Song, Y., Liu, W., Gao, Y., Biancon, G., Chandhok, N. S., et al. (2022). In vivo anti-tumor effect of parp inhibition in idh1/2 mutant mds/aml resistant to targeted inhibitors of mutant idh1/2. Leukemia 36 (5), 1313–1323. Epub 20220310. doi:10.1038/s41375-022-01536-x
Geng, N., Shi, B. J., Li, S. L., Zhong, Z. Y., Li, Y. C., Xua, W. L., et al. (2018). Knockdown of ferroportin accelerates erastin-induced ferroptosis in neuroblastoma cells. Eur. Rev. Med. Pharmacol. Sci. 22 (12), 3826–3836. doi:10.26355/eurrev_201806_15267
Ghio, A. J., Ford, E. S., Kennedy, T. P., and Hoidal, J. R. (2005). The association between serum ferritin and uric acid in humans. Free Radic. Res. 39 (3), 337–342. doi:10.1080/10715760400026088
Ghosh, A. P., Klocke, B. J., Ballestas, M. E., and Roth, K. A. (2012). Chop potentially Co-operates with Foxo3a in neuronal cells to regulate puma and bim expression in response to Er stress. PLoS One 7 (6), e39586. Epub 20120628. doi:10.1371/journal.pone.0039586
Gong, Z., Wang, Y., Li, L., Li, X., Qiu, B., and Hu, Y. (2023). Cardamonin alleviates chondrocytes inflammation and cartilage degradation of osteoarthritis by inhibiting ferroptosis via P53 pathway. Food Chem. Toxicol. 174, 113644. doi:10.1016/j.fct.2023.113644
Gonzalez, F. J., Xie, C., and Jiang, C. (2018). The role of hypoxia-inducible factors in metabolic diseases. Nat. Rev. Endocrinol. 15 (1), 21–32. doi:10.1038/s41574-018-0096-z
Grootjans, J., Kaser, A., Kaufman, R. J., and Blumberg, R. S. (2016). The unfolded protein response in immunity and inflammation. Nat. Rev. Immunol. 16 (8), 469–484. Epub 20160627. doi:10.1038/nri.2016.62
Guo, W., Li, K., Sun, B., Xu, D., Tong, L., Yin, H., et al. (2021). Dysregulated glutamate transporter Slc1a1 propels cystine uptake via Xc(-) for glutathione synthesis in lung cancer. Cancer Res. 81 (3), 552–566. Epub 20201123. doi:10.1158/0008-5472.Can-20-0617
Guo, Z., Lin, J., Sun, K., Guo, J., Yao, X., Wang, G., et al. (2022). Deferoxamine alleviates osteoarthritis by inhibiting chondrocyte ferroptosis and activating the Nrf2 pathway. Front. Pharmacol. 13, 791376. Epub 20220314. doi:10.3389/fphar.2022.791376
Gupta, U., Ghosh, S., Wallace, C. T., Shang, P., Xin, Y., Nair, A. P., et al. (2023). Increased Lcn2 (lipocalin 2) in the rpe decreases autophagy and activates inflammasome-ferroptosis processes in a mouse model of dry amd. Autophagy 19 (1), 92–111. Epub 2022/04/28. doi:10.1080/15548627.2022.2062887
Halliwell, B., and J, M. C. G. (2022). Reprint of: oxygen free radicals and iron in relation to biology and medicine: some problems and concepts. Arch. Biochem. Biophys. 726, 109246. Epub 20220606. doi:10.1016/j.abb.2022.109246
Harada, N., Kanayama, M., Maruyama, A., Yoshida, A., Tazumi, K., Hosoya, T., et al. (2011). Nrf2 regulates ferroportin 1-mediated iron efflux and counteracts lipopolysaccharide-induced ferroportin 1 mrna suppression in macrophages. Arch. Biochem. Biophys. 508 (1), 101–109. Epub 20110212. doi:10.1016/j.abb.2011.02.001
Harayama, T., and Riezman, H. (2018). Understanding the diversity of membrane lipid composition. Nat. Rev. Mol. Cell Biol. 19 (5), 281–296. Epub 20180207. doi:10.1038/nrm.2017.138
Hassan, S. Z., Gheita, T. A., Kenawy, S. A., Fahim, A. T., El-Sorougy, I. M., and Abdou, M. S. (2011). Oxidative stress in systemic lupus erythematosus and rheumatoid arthritis patients: relationship to disease manifestations and activity. Int. J. Rheum. Dis. 14 (4), 325–331. Epub 20110721. doi:10.1111/j.1756-185X.2011.01630.x
Heiland, G. R., Aigner, E., Dallos, T., Sahinbegovic, E., Krenn, V., Thaler, C., et al. (2010). Synovial immunopathology in haemochromatosis arthropathy. Ann. Rheum. Dis. 69 (6), 1214–1219. Epub 20091123. doi:10.1136/ard.2009.120204
Hentze, M. W., Muckenthaler, M. U., and Andrews, N. C. (2004). Balancing acts: molecular control of mammalian iron metabolism. Cell 117 (3), 285–297. doi:10.1016/s0092-8674(04)00343-5
Hentze, M. W., Muckenthaler, M. U., Galy, B., and Camaschella, C. (2010). Two to tango: regulation of mammalian iron metabolism. Cell 142 (1), 24–38. doi:10.1016/j.cell.2010.06.028
Hirschhorn, T., and Stockwell, B. R. (2019). The development of the concept of ferroptosis. Free Radic. Biol. Med. 133, 130–143. Epub 20180928. doi:10.1016/j.freeradbiomed.2018.09.043
Hoesel, B., and Schmid, J. A. (2013). The complexity of nf-κb signaling in inflammation and cancer. Mol. Cancer 12, 86. Epub 20130802. doi:10.1186/1476-4598-12-86
Honda, T., Hirakawa, Y., and Nangaku, M. (2019). The role of oxidative stress and hypoxia in renal disease. Kidney Res. Clin. Pract. 38 (4), 414–426. doi:10.23876/j.krcp.19.063
Hong, S. H., Lee, D. H., Lee, Y. S., Jo, M. J., Jeong, Y. A., Kwon, W. T., et al. (2017). Molecular crosstalk between ferroptosis and apoptosis: emerging role of Er stress-induced P53-independent puma expression. Oncotarget 8 (70), 115164–115178. Epub 20171208. doi:10.18632/oncotarget.23046
Horniblow, R. D., Pathak, P., Balacco, D. L., Acharjee, A., Lles, E., Gkoutos, G., et al. (2022). Iron-mediated epigenetic activation of Nrf2 targets. J. Nutr. Biochem. 101, 108929. Epub 20211223. doi:10.1016/j.jnutbio.2021.108929
Hou, W., Xie, Y., Song, X., Sun, X., Lotze, M. T., Zeh, H. J., et al. (2016). Autophagy promotes ferroptosis by degradation of ferritin. Autophagy 12 (8), 1425–1428. Epub 20160531. doi:10.1080/15548627.2016.1187366
Hu, P., Xu, Y., Jiang, Y., Huang, J., Liu, Y., Wang, D., et al. (2022). The mechanism of the imbalance between proliferation and ferroptosis in pulmonary artery smooth muscle cells based on the activation of Slc7a11. Eur. J. Pharmacol. 928, 175093. doi:10.1016/j.ejphar.2022.175093
Huang, C., Yang, M., Deng, J., Li, P., Su, W., and Jiang, R. (2018). Upregulation and activation of P53 by erastin-induced reactive oxygen species contribute to cytotoxic and cytostatic effects in A549 lung cancer cells. Oncol. Rep. 40 (4), 2363–2370. Epub 20180720. doi:10.3892/or.2018.6585
Huang, L., Bian, M., Lu, S., Wang, J., Yu, J., Jiang, L., et al. (2023b). Engeletin alleviates erastin-induced oxidative stress and protects against ferroptosis via nrf2/keap1 pathway in bone marrow mesenchymal stem cells. Tissue Cell 82, 102040. Epub 20230214. doi:10.1016/j.tice.2023.102040
Huang, L., Wang, J., Yu, J., Bian, M., Xiang, X., Han, G., et al. (2024a). Picein alleviates oxidative stress and promotes bone regeneration in osteoporotic bone defect by inhibiting ferroptosis via Nrf2/Ho-1/Gpx4 pathway. Environ. Toxicol. 39 (7), 4066–4085. Epub 20240510. doi:10.1002/tox.24239
Huang, L., Zhang, S., Bian, M., Xiang, X., Xiao, L., Wang, J., et al. (2024b). Injectable, anti-collapse, adhesive, plastic and bioactive bone graft substitute promotes bone regeneration by moderating oxidative stress in osteoporotic bone defect. Acta Biomater. 180, 82–103. Epub 20240415. doi:10.1016/j.actbio.2024.04.016
Huang, Z., Chen, G., Wu, H., Huang, X., Xu, R., Deng, F., et al. (2023a). Ebselen restores peri-implantitis-induced osteogenic inhibition via suppressing bmscs ferroptosis. Exp. Cell Res. 427 (2), 113612. Epub 20230426. doi:10.1016/j.yexcr.2023.113612
Huo, H., Zhou, Z., Qin, J., Liu, W., Wang, B., and Gu, Y. (2016). Erastin disrupts mitochondrial permeability transition pore (mptp) and induces apoptotic death of colorectal cancer cells. PLoS One 11 (5), e0154605. Epub 20160512. doi:10.1371/journal.pone.0154605
Hurst, R., Korytowski, W., Kriska, T., Esworthy, R. S., Chu, F. F., and Girotti, A. W. (2001). Hyperresistance to cholesterol hydroperoxide-induced peroxidative injury and apoptotic death in a tumor cell line that overexpresses glutathione peroxidase isotype-4. Free Radic. Biol. Med. 31 (9), 1051–1065. doi:10.1016/s0891-5849(01)00685-2
Ikeda, Y., Satoh, A., Horinouchi, Y., Hamano, H., Watanabe, H., Imao, M., et al. (2019). Iron accumulation causes impaired myogenesis correlated with mapk signaling pathway inhibition by oxidative stress. Faseb J. 33 (8), 9551–9564. Epub 20190530. doi:10.1096/fj.201802724RR
Imai, H. (2004). Biological significance of lipid hydroperoxide and its reducing enzyme, phospholipid hydroperoxide glutathione peroxidase, in mammalian cells. Yakugaku Zasshi 124 (12), 937–957. doi:10.1248/yakushi.124.937
Imai, H., and Nakagawa, Y. (2003). Biological significance of phospholipid hydroperoxide glutathione peroxidase (phgpx, Gpx4) in mammalian cells. Free Radic. Biol. Med. 34 (2), 145–169. doi:10.1016/s0891-5849(02)01197-8
Ingold, K. U., and Pratt, D. A. (2014). Advances in radical-trapping antioxidant chemistry in the 21st century: a kinetics and mechanisms perspective. Chem. Rev. 114 (18), 9022–9046. doi:10.1021/cr500226n
Jacobson, M. D., Weil, M., and Raff, M. C. (1997). Programmed cell death in animal development. Cell 88 (3), 347–354. doi:10.1016/s0092-8674(00)81873-5
Jia, M., Qin, D., Zhao, C., Chai, L., Yu, Z., Wang, W., et al. (2020). Redox homeostasis maintained by Gpx4 facilitates sting activation. Nat. Immunol. 21 (7), 727–735. Epub 20200615. doi:10.1038/s41590-020-0699-0
Jiacong, H., Qirui, Y., Haonan, L., Yichang, S., Yan, C., and Keng, C. (2023). Zoledronic acid induces ferroptosis by upregulating por in osteosarcoma. Med. Oncol. 40 (5), 141. Epub 20230410. doi:10.1007/s12032-023-01988-w
Jiang, X., Yu, M., Wang, W. K., Zhu, L. Y., Wang, X., Jin, H. C., et al. (2024). The regulation and function of Nrf2 signaling in ferroptosis-activated cancer therapy. Acta Pharmacol. Sin. 45 (11), 2229–2240. Epub 20240717. doi:10.1038/s41401-024-01336-2
Jiang, Y., Mao, C., Yang, R., Yan, B., Shi, Y., Liu, X., et al. (2017). Egln1/C-Myc induced lymphoid-specific helicase inhibits ferroptosis through lipid metabolic gene expression changes. Theranostics 7 (13), 3293–3305. Epub 20170723. doi:10.7150/thno.19988
Jiang, Y., and Sun, M. (2024). Slc7a11: the achilles heel of tumor? Front. Immunol. 15, 1438807. Epub 20240708. doi:10.3389/fimmu.2024.1438807
Jin, C., Tan, K., Yao, Z., Lin, B. H., Zhang, D. P., Chen, W. K., et al. (2023). A novel anti-osteoporosis mechanism of Vk2: interfering with ferroptosis via ampk/sirt1 pathway in type 2 diabetic osteoporosis. J. Agric. Food Chem. 71 (6), 2745–2761. Epub 20230131. doi:10.1021/acs.jafc.2c05632
Jing, X., Du, T., Li, T., Yang, X., Wang, G., Liu, X., et al. (2021). The detrimental effect of iron on oa chondrocytes: importance of pro-inflammatory cytokines induced iron influx and oxidative stress. J. Cell Mol. Med. 25 (12), 5671–5680. Epub 20210503. doi:10.1111/jcmm.16581
Jing, X., Lin, J., Du, T., Jiang, Z., Li, T., Wang, G., et al. (2020). Iron overload is associated with accelerated progression of osteoarthritis: the role of Dmt1 mediated iron homeostasis. Front. Cell Dev. Biol. 8, 594509. Epub 20210105. doi:10.3389/fcell.2020.594509
Johansson, E., Grassi, E. S., Pantazopoulou, V., Tong, B., Lindgren, D., Berg, T. J., et al. (2017). Cd44 interacts with hif-2α to modulate the hypoxic phenotype of perinecrotic and perivascular glioma cells. Cell Rep. 20 (7), 1641–1653. doi:10.1016/j.celrep.2017.07.049
Jomova, K., Alomar, S. Y., Alwasel, S. H., Nepovimova, E., Kuca, K., and Valko, M. (2024). Several lines of antioxidant defense against oxidative stress: antioxidant enzymes, nanomaterials with multiple enzyme-mimicking activities, and low-molecular-weight antioxidants. Arch. Toxicol. 98 (5), 1323–1367. Epub 20240314. doi:10.1007/s00204-024-03696-4
Kaelin, W. G., and Ratcliffe, P. J. (2008). Oxygen sensing by metazoans: the central role of the Hif hydroxylase pathway. Mol. Cell 30 (4), 393–402. doi:10.1016/j.molcel.2008.04.009
Kagan, V. E., Mao, G., Qu, F., Angeli, J. P., Doll, S., Croix, C. S., et al. (2017). Oxidized arachidonic and adrenic pes navigate cells to ferroptosis. Nat. Chem. Biol. 13 (1), 81–90. Epub 20161114. doi:10.1038/nchembio.2238
Kajarabille, N., and Latunde-Dada, G. O. (2019). Programmed cell-death by ferroptosis: antioxidants as mitigators. Int. J. Mol. Sci. 20 (19), 4968. doi:10.3390/ijms20194968
Karakoc, M., Altindag, O., Keles, H., Soran, N., and Selek, S. (2007). Serum oxidative-antioxidative status in patients with ankylosing spondilitis. Rheumatol. Int. 27 (12), 1131–1134. doi:10.1007/s00296-007-0352-3
Ke, Y., Dai, X., Xu, D., Liang, J., Yu, Y., Cao, H., et al. (2021). Features and outcomes of elderly rheumatoid arthritis: does the age of onset matter? A comparative study from a single center in China. Rheumatol. Ther. 8 (1), 243–254. Epub 20201214. doi:10.1007/s40744-020-00267-8
Kennish, L., Attur, M., Oh, C., Krasnokutsky, S., Samuels, J., Greenberg, J. D., et al. (2014). Age-dependent ferritin elevations and hfe C282y mutation as risk factors for symptomatic knee osteoarthritis in males: a longitudinal cohort study. BMC Musculoskelet. Disord. 15, 8. Epub 20140108. doi:10.1186/1471-2474-15-8
Kerins, M. J., Milligan, J., Wohlschlegel, J. A., and Ooi, A. (2018). Fumarate hydratase inactivation in hereditary leiomyomatosis and renal cell cancer is synthetic lethal with ferroptosis induction. Cancer Sci. 109 (9), 2757–2766. Epub 20180720. doi:10.1111/cas.13701
Kerins, M. J., and Ooi, A. (2018). The roles of Nrf2 in modulating cellular iron homeostasis. Antioxid. Redox Signal 29 (17), 1756–1773. Epub 20170921. doi:10.1089/ars.2017.7176
Kim, H., Lee, J. H., and Park, J. W. (2020a). Down-regulation of Idh2 sensitizes cancer cells to erastin-induced ferroptosis. Biochem. Biophys. Res. Commun. 525 (2), 366–371. Epub 20200221. doi:10.1016/j.bbrc.2020.02.093
Kim, J. M., Lin, C., Stavre, Z., Greenblatt, M. B., and Shim, J. H. (2020b). Osteoblast-osteoclast communication and bone homeostasis. Cells 9 (9), 2073. Epub 20200910. doi:10.3390/cells9092073
Ko, S. H., and Kim, H. S. (2020). Menopause-associated lipid metabolic disorders and foods beneficial for postmenopausal women. Nutrients 12 (1), 202. doi:10.3390/nu12010202
Koh, J. M., Lee, Y. S., Kim, Y. S., Kim, D. J., Kim, H. H., Park, J. Y., et al. (2006). Homocysteine enhances bone resorption by stimulation of osteoclast formation and activity through increased intracellular ros generation. J. Bone Min. Res. 21 (7), 1003–1011. doi:10.1359/jbmr.060406
Koh, M. Y., and Powis, G. (2012). Passing the baton: the Hif switch. Trends Biochem. Sci. 37 (9), 364–372. Epub 20120718. doi:10.1016/j.tibs.2012.06.004
Komori, T. (2022). Whole aspect of Runx2 functions in skeletal development. Int. J. Mol. Sci. 23 (10), 5776. doi:10.3390/ijms23105776
Kong, R., Wang, N., Han, W., Bao, W., and Lu, J. (2021). Ifnγ-mediated repression of system Xc(-) drives vulnerability to induced ferroptosis in hepatocellular carcinoma cells. J. Leukoc. Biol. 110 (2), 301–314. doi:10.1002/jlb.3ma1220-815rrr
Kou, X., Jing, Y., Deng, W., Sun, K., Han, Z., Ye, F., et al. (2013). Tumor necrosis factor-α attenuates starvation-induced apoptosis through upregulation of ferritin heavy chain in hepatocellular carcinoma cells. BMC Cancer 13, 438. Epub 20130925. doi:10.1186/1471-2407-13-438
Kowdley, K. V., Gochanour, E. M., Sundaram, V., Shah, R. A., and Handa, P. (2021). Hepcidin signaling in health and disease: ironing out the details. Hepatol. Commun. 5 (5), 723–735. Epub 20210326. doi:10.1002/hep4.1717
Kruszewski, M. (2003). Labile iron pool: the main determinant of cellular response to oxidative stress. Mutat. Res. 531 (1-2), 81–92. doi:10.1016/j.mrfmmm.2003.08.004
Kuhn, H., Banthiya, S., and van Leyen, K. (2015). Mammalian lipoxygenases and their biological relevance. Biochim. Biophys. Acta 1851 (4), 308–330. Epub 20141012. doi:10.1016/j.bbalip.2014.10.002
Kumar, H., and Choi, D.-K. (2015). Hypoxia inducible factor pathway and physiological adaptation: a cell survival pathway? Mediat. Inflamm. 2015 (1), 584758. doi:10.1155/2015/584758
Lan, D., Qi, S., Yao, C., Li, X., Liu, H., Wang, D., et al. (2022a). Quercetin protects rat bmscs from oxidative stress via ferroptosis. J. Mol. Endocrinol. 69 (3), 401–413. Epub 20220824. doi:10.1530/jme-22-0086
Lan, D., Yao, C., Li, X., Liu, H., Wang, D., Wang, Y., et al. (2022b). Tocopherol attenuates the oxidative stress of bmscs by inhibiting ferroptosis through the pi3k/akt/mtor pathway. Front. Bioeng. Biotechnol. 10, 938520. Epub 20220817. doi:10.3389/fbioe.2022.938520
Lane, D. J., Bae, D. H., Merlot, A. M., Sahni, S., and Richardson, D. R. (2015). Duodenal cytochrome B (dcytb) in iron metabolism: an update on function and regulation. Nutrients 7 (4), 2274–2296. Epub 20150331. doi:10.3390/nu7042274
Larrick, J. W., and Hyman, E. S. (1984). Acquired iron-deficiency anemia caused by an antibody against the transferrin receptor. N. Engl. J. Med. 311 (4), 214–218. doi:10.1056/nejm198407263110402
Latchoumycandane, C., Marathe, G. K., Zhang, R., and McIntyre, T. M. (2012). Oxidatively truncated phospholipids are required agents of tumor necrosis factor α (TNFα)-induced apoptosis. J. Biol. Chem. 287 (21), 17693–17705. Epub 20120320. doi:10.1074/jbc.M111.300012
Lee, J., You, J. H., Kim, M. S., and Roh, J. L. (2020a). Epigenetic reprogramming of epithelial-mesenchymal transition promotes ferroptosis of head and neck cancer. Redox Biol. 37, 101697. doi:10.1016/j.redox.2020.101697
Lee, J. Y., Nam, M., Son, H. Y., Hyun, K., Jang, S. Y., Kim, J. W., et al. (2020b). Polyunsaturated fatty acid biosynthesis pathway determines ferroptosis sensitivity in gastric cancer. Proc. Natl. Acad. Sci. U. S. A. 117 (51), 32433–32442. Epub 20201207. doi:10.1073/pnas.2006828117
Lee, W. C., Guntur, A. R., Long, F., and Rosen, C. J. (2017). Energy metabolism of the osteoblast: implications for osteoporosis. Endocr. Rev. 38 (3), 255–266. doi:10.1210/er.2017-00064
Lei, G., Zhuang, L., and Gan, B. (2022). Targeting ferroptosis as a vulnerability in cancer. Nat. Rev. Cancer 22 (7), 381–396. Epub 20220325. doi:10.1038/s41568-022-00459-0
Lei, P., Bai, T., and Sun, Y. (2019). Mechanisms of ferroptosis and relations with regulated cell death: a review. Front. Physiol. 10, 139. Epub 20190226. doi:10.3389/fphys.2019.00139
Le Maitre, C. L., Binch, A. L., Thorpe, A. A., and Hughes, S. P. (2015). Degeneration of the intervertebral disc with new approaches for treating low back pain. J. Neurosurg. Sci. 59 (1), 47–61. Epub 20141125.
Li, C., Liu, J., Hou, W., Kang, R., and Tang, D. (2021a). Sting1 promotes ferroptosis through mfn1/2-dependent mitochondrial fusion. Front. Cell Dev. Biol. 9, 698679. Epub 20210614. doi:10.3389/fcell.2021.698679
Li, C., Sun, S., Zhuang, Y., Luo, Z., Ji, G., and Liu, Z. (2023a). Ctsb nuclear translocation facilitates DNA damage and lysosomal stress to promote retinoblastoma cell death. Mol. Biotechnol. 66, 2583–2594. Epub 2024/01/02. doi:10.1007/s12033-023-01042-0
Li, C., Zhang, Y., Deng, Y., Chen, Y., Wu, C., Zhao, X., et al. (2024f). Fisetin suppresses ferroptosis through Nrf2 and attenuates intervertebral disc degeneration in rats. Eur. J. Pharmacol. 964, 176298. Epub 20231223. doi:10.1016/j.ejphar.2023.176298
Li, F. J., Long, H. Z., Zhou, Z. W., Luo, H. Y., Xu, S. G., and Gao, L. C. (2022a). System X(C) (-)/Gsh/Gpx4 Axis: an important antioxidant system for the ferroptosis in drug-resistant solid tumor therapy. Front. Pharmacol. 13, 910292. Epub 20220829. doi:10.3389/fphar.2022.910292
Li, L., Zhang, Y., Gao, Y., Hu, Y., Wang, R., Wang, S., et al. (2023b). Lncsnhg14 promotes Nutlin3a resistance by inhibiting ferroptosis via the mir-206/Slc7a11 Axis in osteosarcoma cells. Cancer Gene Ther. 30 (5), 704–715. Epub 20230104. doi:10.1038/s41417-022-00581-z
Li, Q., Chen, Z., Yang, C., Wang, L., Ma, J., He, T., et al. (2022c). Role of ferroptosis-associated genes in ankylosing spondylitis and immune cell infiltration. Front. Genet. 13, 948290. Epub 20221111. doi:10.3389/fgene.2022.948290
Li, R., Wei, X., and Jiang, D. S. (2019). Protein methylation functions as the posttranslational modification switch to regulate autophagy. Cell Mol. Life Sci. 76 (19), 3711–3722. Epub 20190619. doi:10.1007/s00018-019-03161-x
Li, S., Ouyang, X., Sun, H., Jin, J., Chen, Y., Li, L., et al. (2024c). Depdc5 protects Cd8(+) T cells from ferroptosis by limiting mtorc1-mediated purine catabolism. Cell Discov. 10 (1), 53. Epub 20240520. doi:10.1038/s41421-024-00682-z
Li, T. Q., Liu, Y., Feng, C., Bai, J., Wang, Z. R., Zhang, X. Y., et al. (2024e). Saikosaponin a attenuates osteoclastogenesis and bone loss by inducing ferroptosis. Front. Mol. Biosci. 11, 1390257. Epub 20240724. doi:10.3389/fmolb.2024.1390257
Li, X., He, T., Yu, K., Lu, Q., Alkasir, R., Guo, G., et al. (2018). Markers of iron status are associated with risk of hyperuricemia among Chinese adults: nationwide population-based study. Nutrients 10 (2), 191. Epub 20180209. doi:10.3390/nu10020191
Li, X., Si, W., Li, Z., Tian, Y., Liu, X., Ye, S., et al. (2021b). Mir-335 promotes ferroptosis by targeting ferritin heavy chain 1 in in vivo and in vitro models of Parkinson's disease. Int. J. Mol. Med. 47 (4), 61. doi:10.3892/ijmm.2021.4894
Li, Y., Cai, Z., Ma, W., Bai, L., Luo, E., and Lin, Y. (2024d). A DNA tetrahedron-based ferroptosis-suppressing nanoparticle: superior delivery of curcumin and alleviation of diabetic osteoporosis. Bone Res. 12 (1), 14. Epub 20240229. doi:10.1038/s41413-024-00319-7
Li, Y., Cao, Y., Xiao, J., Shang, J., Tan, Q., Ping, F., et al. (2020). Inhibitor of apoptosis-stimulating protein of P53 inhibits ferroptosis and alleviates intestinal ischemia/reperfusion-induced acute lung injury. Cell Death Differ. 27 (9), 2635–2650. Epub 20200318. doi:10.1038/s41418-020-0528-x
Li, Y., Pan, D., Wang, X., Huo, Z., Wu, X., Li, J., et al. (2022b). Silencing Atf3 might delay tbhp-induced intervertebral disc degeneration by repressing npc ferroptosis, apoptosis, and ecm degradation. Oxid. Med. Cell Longev. 2022, 4235126. Epub 20220415. doi:10.1155/2022/4235126
Li, Y., Zhang, H., Yang, F., Zhu, D., Chen, S., Wang, Z., et al. (2024a). Mechanisms and therapeutic potential of disulphidptosis in cancer. Cell Prolif., e13752. Epub 20241001. doi:10.1111/cpr.13752
Li, Y., Zhang, H., Zhu, D., Yang, F., Wang, Z., Wei, Z., et al. (2024b). Notochordal cells: a potential therapeutic option for intervertebral disc degeneration. Cell Prolif. 57 (2), e13541. Epub 20230911. doi:10.1111/cpr.13541
Li, Z., Luo, Y., Wang, C., Han, D., and Sun, W. (2023c). Circular rna circblnk promotes osteosarcoma progression and inhibits ferroptosis in osteosarcoma cells by sponging mir-188-3p and regulating Gpx4 expression. Oncol. Rep. 50 (5), 192. doi:10.3892/or.2023.8629
Liang, D., Minikes, A. M., and Jiang, X. (2022). Ferroptosis at the intersection of lipid metabolism and cellular signaling. Mol. Cell 82 (12), 2215–2227. Epub 20220406. doi:10.1016/j.molcel.2022.03.022
Liang, H., Yoo, S. E., Na, R., Walter, C. A., Richardson, A., and Ran, Q. (2009). Short form glutathione peroxidase 4 is the essential isoform required for survival and somatic mitochondrial functions. J. Biol. Chem. 284 (45), 30836–30844. Epub 20090910. doi:10.1074/jbc.M109.032839
Lin, H., Chen, X., Zhang, C., Yang, T., Deng, Z., Song, Y., et al. (2021b). Ef24 induces ferroptosis in osteosarcoma cells through Hmox1. Biomed. Pharmacother. 136, 111202. Epub 20210114. doi:10.1016/j.biopha.2020.111202
Lin, Y., Shen, X., Ke, Y., Lan, C., Chen, X., Liang, B., et al. (2022b). Activation of osteoblast ferroptosis via the mettl3/ask1-P38 signaling pathway in high glucose and high fat (Hghf)-Induced diabetic bone loss. Faseb J. 36 (3), e22147. doi:10.1096/fj.202101610R
Lin, Z., He, H., Wang, M., and Liang, J. (2019). Microrna-130a controls bone marrow mesenchymal stem cell differentiation towards the osteoblastic and adipogenic fate. Cell Prolif. 52 (6), e12688. Epub 20190926. doi:10.1111/cpr.12688
Lin, Z., Miao, J., Zhang, T., He, M., Zhou, X., Zhang, H., et al. (2021a). D-mannose suppresses osteoarthritis development in vivo and delays il-1β-induced degeneration in vitro by enhancing autophagy activated via the ampk pathway. Biomed. Pharmacother. 135, 111199. Epub 20210102. doi:10.1016/j.biopha.2020.111199
Lin, Z., Song, J., Gao, Y., Huang, S., Dou, R., Zhong, P., et al. (2022a). Hypoxia-induced hif-1α/lncrna-pman inhibits ferroptosis by promoting the cytoplasmic translocation of Elavl1 in peritoneal dissemination from gastric cancer. Redox Biol. 52, 102312. doi:10.1016/j.redox.2022.102312
Ling, H., Li, M., Yang, C., Sun, S., Zhang, W., Zhao, L., et al. (2022). Glycine increased ferroptosis via sam-mediated Gpx4 promoter methylation in rheumatoid arthritis. Rheumatol. Oxf. 61 (11), 4521–4534. doi:10.1093/rheumatology/keac069
Liu, H., Deng, Z., Yu, B., Liu, H., Yang, Z., Zeng, A., et al. (2022a). Identification of Slc3a2 as a potential therapeutic target of osteoarthritis involved in ferroptosis by integrating bioinformatics, clinical factors and experiments. Cells 11 (21), 3430. Epub 20221030. doi:10.3390/cells11213430
Liu, J., Guo, Z. N., Yan, X. L., Huang, S., Ren, J. X., Luo, Y., et al. (2020b). Crosstalk between autophagy and ferroptosis and its putative role in ischemic stroke. Front. Cell Neurosci. 14, 577403. doi:10.3389/fncel.2020.577403
Liu, J., Lou, C., Zhen, C., Wang, Y., Shang, P., and Lv, H. (2022b). Iron plays a role in sulfasalazine-induced ferroptosis with autophagic flux blockage in K7m2 osteosarcoma cells. Metallomics 14 (5), mfac027. doi:10.1093/mtomcs/mfac027
Liu, J., Pan, Z., Tong, B., Wang, C., Yang, J., Zou, J., et al. (2023). Artesunate protects against ocular fibrosis by suppressing fibroblast activation and inducing mitochondria-dependent ferroptosis. Faseb J. 37 (6), e22954. Epub 2023/05/09. doi:10.1096/fj.202201867R
Liu, J., Peng, Y., Shi, L., Wan, L., Inuzuka, H., Long, J., et al. (2021a). Skp2 dictates cell cycle-dependent metabolic oscillation between glycolysis and tca cycle. Cell Res. 31 (1), 80–93. Epub 20200715. doi:10.1038/s41422-020-0372-z
Liu, J., Tang, D., and Kang, R. (2024). Targeting Gpx4 in ferroptosis and cancer: chemical strategies and challenges. Trends Pharmacol. Sci. 45 (8), 666–670. Epub 20240611. doi:10.1016/j.tips.2024.05.006
Liu, J., Xia, X., and Huang, P. (2020a). Xct: a critical molecule that links cancer metabolism to redox signaling. Mol. Ther. 28 (11), 2358–2366. Epub 20200902. doi:10.1016/j.ymthe.2020.08.021
Liu, L., Li, Y., Cao, D., Qiu, S., Li, Y., Jiang, C., et al. (2021c). Sirt3 inhibits gallbladder cancer by induction of akt-dependent ferroptosis and blockade of epithelial-mesenchymal transition. Cancer Lett. 510, 93–104. Epub 20210416. doi:10.1016/j.canlet.2021.04.007
Liu, N., and Chen, M. (2024). Crosstalk between ferroptosis and cuproptosis: from mechanism to potential clinical application. Biomed. & Pharmacother. 171, 116115. doi:10.1016/j.biopha.2023.116115
Liu, Q., and Wang, K. (2019). The induction of ferroptosis by impairing stat3/nrf2/gpx4 signaling enhances the sensitivity of osteosarcoma cells to cisplatin. Cell Biol. Int. 43 (11), 1245–1256. Epub 20190718. doi:10.1002/cbin.11121
Liu, R., Li, W., Tao, B., Wang, X., Yang, Z., Zhang, Y., et al. (2019). Tyrosine phosphorylation activates 6-phosphogluconate dehydrogenase and promotes tumor growth and radiation resistance. Nat. Commun. 10 (1), 991. Epub 20190301. doi:10.1038/s41467-019-08921-8
Liu, X., Zhang, Y., Zhuang, L., Olszewski, K., and Gan, B. (2021b). Nadph debt drives redox bankruptcy: slc7a11/xct-mediated cystine uptake as a double-edged sword in cellular redox regulation. Genes Dis. 8 (6), 731–745. Epub 20201125. doi:10.1016/j.gendis.2020.11.010
Loscalzo, J. (2008). Membrane redox state and apoptosis: death by peroxide. Cell Metab. 8 (3), 182–183. doi:10.1016/j.cmet.2008.08.004
Lu, J., Yang, J., Zheng, Y., Chen, X., and Fang, S. (2019). Extracellular vesicles from endothelial progenitor cells prevent steroid-induced osteoporosis by suppressing the ferroptotic pathway in mouse osteoblasts based on bioinformatics evidence. Sci. Rep. 9 (1), 16130. Epub 20191106. doi:10.1038/s41598-019-52513-x
Lu, S., Song, Y., Luo, R., Li, S., Li, G., Wang, K., et al. (2021). Ferroportin-dependent iron homeostasis protects against oxidative stress-induced Nucleus pulposus cell ferroptosis and ameliorates intervertebral disc degeneration in vivo. Oxid. Med. Cell Longev. 2021, 6670497. Epub 20210210. doi:10.1155/2021/6670497
Lu, X., Li, D., Lin, Z., Gao, T., Gong, Z., Zhang, Y., et al. (2024). Hif-1α-Induced expression of the M6a reader Ythdf1 inhibits the ferroptosis of Nucleus pulposus cells by promoting Slc7a11 translation. Aging Cell 23, e14210. Epub 20240523. doi:10.1111/acel.14210
Luchkova, A., Mata, A., and Cadenas, S. (2024). Nrf2 as a regulator of energy metabolism and mitochondrial function. FEBS Lett. 598 (17), 2092–2105. Epub 20240808. doi:10.1002/1873-3468.14993
Luo, C., and Tao, L. (2020). The function and mechanisms of autophagy in traumatic brain injury. Adv. Exp. Med. Biol. 1207, 635–648. doi:10.1007/978-981-15-4272-5_46
Luo, C., Xu, W., Tang, X., Liu, X., Cheng, Y., Wu, Y., et al. (2022). Canonical wnt signaling works downstream of iron overload to prevent ferroptosis from damaging osteoblast differentiation. Free Radic. Biol. Med. 188, 337–350. Epub 20220623. doi:10.1016/j.freeradbiomed.2022.06.236
Luo, H., and Zhang, R. (2021). Icariin enhances cell survival in lipopolysaccharide-induced synoviocytes by suppressing ferroptosis via the xc-/gpx4 Axis. Exp. Ther. Med. 21 (1), 72. Epub 20201125. doi:10.3892/etm.2020.9504
Luo, L., Chen, H., Xie, K., Xiang, J., Chen, J., and Lin, Z. (2024). Cathepsin B serves as a potential prognostic biomarker and correlates with ferroptosis in rheumatoid arthritis. Int. Immunopharmacol. 128, 111502. doi:10.1016/j.intimp.2024.111502
Luo, Y., Gao, X., Zou, L., Lei, M., Feng, J., and Hu, Z. (2021). Bavachin induces ferroptosis through the Stat3/P53/slc7a11 Axis in osteosarcoma cells. Oxid. Med. Cell Longev. 2021, 1783485. Epub 20211018. doi:10.1155/2021/1783485
Lv, H., Yang, H., Duan, Y., Yan, C., Li, G., Zhao, G., et al. (2024). S-(N,N-Diethyldithiocarbamoyl)-N-Acetyl-L-Cysteine for the treatment of non-small cell lung cancer through regulating nf-κb signaling pathway without neurotoxicity. J. drug Target., 1–16. Epub 2024/07/04. doi:10.1080/1061186x.2024.2374037
Ma, H., Wang, X., Zhang, W., Li, H., Zhao, W., Sun, J., et al. (2020). Melatonin suppresses ferroptosis induced by high glucose via activation of the Nrf2/Ho-1 signaling pathway in type 2 diabetic osteoporosis. Oxid. Med. Cell Longev. 2020, 9067610. Epub 20201204. doi:10.1155/2020/9067610
Ma, J., Wang, A., Zhang, H., Liu, B., Geng, Y., Xu, Y., et al. (2022b). Iron overload induced osteocytes apoptosis and led to bone loss in hepcidin(-/-) mice through increasing sclerostin and rankl/opg. Bone 164, 116511. Epub 20220804. doi:10.1016/j.bone.2022.116511
Ma, M., Kong, P., Huang, Y., Wang, J., Liu, X., Hu, Y., et al. (2022a). Activation of mat2a-acsl3 pathway protects cells from ferroptosis in gastric cancer. Free Radic. Biol. Med. 181, 288–299. Epub 20220216. doi:10.1016/j.freeradbiomed.2022.02.015
Ma, S., Dielschneider, R. F., Henson, E. S., Xiao, W., Choquette, T. R., Blankstein, A. R., et al. (2017). Ferroptosis and autophagy induced cell death occur independently after siramesine and lapatinib treatment in breast cancer cells. PLoS One 12 (8), e0182921. Epub 20170821. doi:10.1371/journal.pone.0182921
Ma, S., Dubin, A. E., Zhang, Y., Mousavi, S. A. R., Wang, Y., Coombs, A. M., et al. (2021). A role of Piezo1 in iron metabolism in mice and humans. Cell 184 (4), 969–982.e13. Epub 20210210. doi:10.1016/j.cell.2021.01.024
Maiti, B. K., Maia, L. B., and Moura, J. J. G. (2022). Sulfide and transition metals - a partnership for life. J. Inorg. Biochem. 227, 111687. Epub 20211205. doi:10.1016/j.jinorgbio.2021.111687
Mandal, P. K., Seiler, A., Perisic, T., Kölle, P., Banjac Canak, A., Förster, H., et al. (2010). System X(C)- and thioredoxin reductase 1 cooperatively rescue glutathione deficiency. J. Biol. Chem. 285 (29), 22244–22253. Epub 20100512. doi:10.1074/jbc.M110.121327
Martinez-Outschoorn, U. E., Peiris-Pagés, M., Pestell, R. G., Sotgia, F., and Lisanti, M. P. (2017). Cancer metabolism: a therapeutic perspective. Nat. Rev. Clin. Oncol. 14 (1), 11–31. Epub 20160504. doi:10.1038/nrclinonc.2016.60
McClelland, G. B., and Scott, G. R. (2019). Evolved mechanisms of aerobic performance and hypoxia resistance in high-altitude natives. Annu. Rev. Physiol. 81, 561–583. Epub 20180926. doi:10.1146/annurev-physiol-021317-121527
Meihe, L., Shan, G., Minchao, K., Xiaoling, W., Peng, A., Xili, W., et al. (2021). The ferroptosis-nlrp1 inflammasome: the vicious cycle of an adverse pregnancy. Front. Cell Dev. Biol. 9, 707959. doi:10.3389/fcell.2021.707959
Meneghini, R. (1997). Iron homeostasis, oxidative stress, and DNA damage. Free Radic. Biol. Med. 23 (5), 783–792. doi:10.1016/s0891-5849(97)00016-6
Messer, J. G., Kilbarger, A. K., Erikson, K. M., and Kipp, D. E. (2009). Iron overload alters iron-regulatory genes and proteins, down-regulates osteoblastic phenotype, and is associated with apoptosis in fetal rat calvaria cultures. Bone 45 (5), 972–979. Epub 20090728. doi:10.1016/j.bone.2009.07.073
Miao, Y., Chen, Y., Xue, F., Liu, K., Zhu, B., Gao, J., et al. (2022). Contribution of ferroptosis and Gpx4's dual functions to osteoarthritis progression. EBioMedicine 76, 103847. Epub 20220202. doi:10.1016/j.ebiom.2022.103847
Mirabello, L., Pfeiffer, R., Murphy, G., Daw, N. C., Patiño-Garcia, A., Troisi, R. J., et al. (2011). Height at diagnosis and birth-weight as risk factors for osteosarcoma. Cancer Causes Control 22 (6), 899–908. Epub 20110405. doi:10.1007/s10552-011-9763-2
Mirabello, L., Troisi, R. J., and Savage, S. A. (2009). Osteosarcoma incidence and survival rates from 1973 to 2004: data from the surveillance, epidemiology, and end results program. Cancer 115 (7), 1531–1543. doi:10.1002/cncr.24121
Mitchell, J. R., Jollow, D. J., Potter, W. Z., Gillette, J. R., and Brodie, B. B. (1973). Acetaminophen-induced hepatic necrosis. Iv. Protective role of glutathione. J. Pharmacol. Exp. Ther. 187 (1), 211–217.
Mizushima, N., and Levine, B. (2020). Autophagy in human diseases. N. Engl. J. Med. 383 (16), 1564–1576. doi:10.1056/NEJMra2022774
Mo, Z., Xu, P., and Li, H. (2021). Stigmasterol alleviates interleukin-1beta-induced chondrocyte injury by down-regulatingsterol regulatory element binding transcription factor 2 to regulateferroptosis. Bioengineered 12 (2), 9332–9340. doi:10.1080/21655979.2021.2000742
Mobarra, N., Shanaki, M., Ehteram, H., Nasiri, H., Sahmani, M., Saeidi, M., et al. (2016). A review on iron chelators in treatment of iron overload syndromes. Int. J. Hematol. Oncol. Stem Cell Res. 10 (4), 239–247.
Muckenthaler, M. U., Rivella, S., Hentze, M. W., and Galy, B. (2017). A red carpet for iron metabolism. Cell 168 (3), 344–361. doi:10.1016/j.cell.2016.12.034
Mudd, S. H., Skovby, F., Levy, H. L., Pettigrew, K. D., Wilcken, B., Pyeritz, R. E., et al. (1985). The natural history of homocystinuria due to cystathionine beta-synthase deficiency. Am. J. Hum. Genet. 37 (1), 1–31.
Mueller, A. L., Payandeh, Z., Mohammadkhani, N., Mubarak, S. M. H., Zakeri, A., Alagheband Bahrami, A., et al. (2021). Recent advances in understanding the pathogenesis of rheumatoid arthritis: new treatment strategies. Cells 10 (11), 3017. Epub 20211104. doi:10.3390/cells10113017
Nakamura, E., Sato, M., Yang, H., Miyagawa, F., Harasaki, M., Tomita, K., et al. (1999). 4f2 (Cd98) heavy chain is associated covalently with an amino acid transporter and controls intracellular trafficking and membrane topology of 4f2 heterodimer. J. Biol. Chem. 274 (5), 3009–3016. doi:10.1074/jbc.274.5.3009
Nemeth, E., Tuttle, M. S., Powelson, J., Vaughn, M. B., Donovan, A., Ward, D. M., et al. (2004). Hepcidin regulates cellular iron efflux by binding to ferroportin and inducing its internalization. Science 306 (5704), 2090–2093. Epub 20041028. doi:10.1126/science.1104742
Ni, S., Yuan, Y., Qian, Z., Zhong, Z., Lv, T., Kuang, Y., et al. (2021). Hypoxia inhibits rankl-induced ferritinophagy and protects osteoclasts from ferroptosis. Free Radic. Biol. Med. 169, 271–282. Epub 20210422. doi:10.1016/j.freeradbiomed.2021.04.027
Nie, H., Ju, H., Fan, J., Shi, X., Cheng, Y., Cang, X., et al. (2020). O-glcnacylation of Pgk1 coordinates glycolysis and tca cycle to promote tumor growth. Nat. Commun. 11 (1), 36. Epub 20200107. doi:10.1038/s41467-019-13601-8
Nishida, X. da S. T., Friedmann Angeli, J. P., and Ingold, I. (2022). Gpx4: old lessons, new features. Biochem. Soc. Trans. 50 (3), 1205–1213. doi:10.1042/BST20220682
Niu, B., Liao, K., Zhou, Y., Wen, T., Quan, G., Pan, X., et al. (2021). Application of glutathione depletion in cancer therapy: enhanced ros-based therapy, ferroptosis, and chemotherapy. Biomaterials 277, 121110. Epub 20210830. doi:10.1016/j.biomaterials.2021.121110
Ohgami, R. S., Campagna, D. R., McDonald, A., and Fleming, M. D. (2006). The steap proteins are metalloreductases. Blood 108 (4), 1388–1394. Epub 20060411. doi:10.1182/blood-2006-02-003681
Okyay, E., Ertugrul, C., Acar, B., Sisman, A. R., Onvural, B., and Ozaksoy, D. (2013). Comparative evaluation of serum levels of main minerals and postmenopausal osteoporosis. Maturitas 76 (4), 320–325. Epub 20130822. doi:10.1016/j.maturitas.2013.07.015
Orecchioni, M., Kobiyama, K., Winkels, H., Ghosheh, Y., McArdle, S., Mikulski, Z., et al. (2022). Olfactory receptor 2 in vascular macrophages drives atherosclerosis by nlrp3-dependent il-1 production. Science 375 (6577), 214–221. Epub 20220113. doi:10.1126/science.abg3067
Otarola, G. A., Hu, J. C., and Athanasiou, K. A. (2022). Ion modulatory treatments toward functional self-assembled neocartilage. Acta Biomater. 153, 85–96. Epub 20220914. doi:10.1016/j.actbio.2022.09.022
Pan, L., Zhang, Y., Chen, N., and Yang, L. (2017). Icariin regulates cellular functions and gene expression of osteoarthritis patient-derived human fibroblast-like synoviocytes. Int. J. Mol. Sci. 18 (12), 2656. Epub 20171208. doi:10.3390/ijms18122656
Pan, Z., Ma, G., Kong, L., and Du, G. (2021). Hypoxia-inducible factor-1: regulatory mechanisms and drug development in stroke. Pharmacol. Res. 170, 105742. doi:10.1016/j.phrs.2021.105742
Park, S. K., Ryoo, J. H., Kim, M. G., and Shin, J. Y. (2012). Association of serum ferritin and the development of metabolic syndrome in middle-aged Korean men: a 5-year follow-up study. Diabetes Care 35 (12), 2521–2526. Epub 20120828. doi:10.2337/dc12-0543
Park, Y. J., Yoo, S. A., and Kim, W. U. (2014). Role of endoplasmic reticulum stress in rheumatoid arthritis pathogenesis. J. Korean Med. Sci. 29 (1), 2–11. Epub 20131226. doi:10.3346/jkms.2014.29.1.2
Patil, N., Walsh, P., Carrabre, K., Holmberg, E. G., Lavoie, N., Dutton, J. R., et al. (2021). Regionally specific human pre-oligodendrocyte progenitor cells produce both oligodendrocytes and neurons after transplantation in a chronically injured spinal cord rat model after glial scar ablation. J. Neurotrauma 38 (6), 777–788. Epub 20210108. doi:10.1089/neu.2020.7009
Perez, M. A., Magtanong, L., Dixon, S. J., and Watts, J. L. (2020). Dietary lipids induce ferroptosis in caenorhabditiselegans and human cancer cells. Dev. Cell 54 (4), 447–454. Epub 20200710. doi:10.1016/j.devcel.2020.06.019
Pettenuzzo, S., Arduino, A., Belluzzi, E., Pozzuoli, A., Fontanella, C. G., Ruggieri, P., et al. (2023). Biomechanics of chondrocytes and chondrons in healthy conditions and osteoarthritis: a review of the mechanical characterisations at the microscale. Biomedicines 11 (7), 1942. doi:10.3390/biomedicines11071942
Phull, A. R., Nasir, B., Haq, I. U., and Kim, S. J. (2018). Oxidative stress, consequences and ros mediated cellular signaling in rheumatoid arthritis. Chem. Biol. Interact. 281, 121–136. Epub 20171216. doi:10.1016/j.cbi.2017.12.024
Pishgahi, A., Abolhasan, R., Danaii, S., Amanifar, B., Soltani-Zangbar, M. S., Zamani, M., et al. (2020). Immunological and oxidative stress biomarkers in ankylosing spondylitis patients with or without metabolic syndrome. Cytokine 128, 155002. Epub 20200124. doi:10.1016/j.cyto.2020.155002
Pulkkinen, K. H., Ylä-Herttuala, S., and Levonen, A. L. (2011). Heme oxygenase 1 is induced by mir-155 via reduced Bach1 translation in endothelial cells. Free Radic. Biol. Med. 51 (11), 2124–2131. Epub 20110917. doi:10.1016/j.freeradbiomed.2011.09.014
Qiu, Y., Cao, Y., Cao, W., Jia, Y., and Lu, N. (2020). The application of ferroptosis in diseases. Pharmacol. Res. 159, 104919. doi:10.1016/j.phrs.2020.104919
Qu, X., Sun, Z., Wang, Y., and Ong, H. S. (2021). Zoledronic acid promotes osteoclasts ferroptosis by inhibiting fbxo9-mediated P53 ubiquitination and degradation. PeerJ 9, e12510. Epub 20211216. doi:10.7717/peerj.12510
Quagliariello, V., De Laurentiis, M., Rea, D., Barbieri, A., Monti, M. G., Carbone, A., et al. (2021). The sglt-2 inhibitor empagliflozin improves myocardial strain, reduces cardiac fibrosis and pro-inflammatory cytokines in non-diabetic mice treated with doxorubicin. Cardiovasc Diabetol. 20 (1), 150. Epub 20210723. doi:10.1186/s12933-021-01346-y
Que, X., Hung, M. Y., Yeang, C., Gonen, A., Prohaska, T. A., Sun, X., et al. (2018). Oxidized phospholipids are proinflammatory and proatherogenic in hypercholesterolaemic mice. Nature 558 (7709), 301–306. Epub 20180606. doi:10.1038/s41586-018-0198-8
Rajagopalan, K., Selvan Christyraj, J. D., Chelladurai, K. S., Kalimuthu, K., Das, P., Chandrasekar, M., et al. (2024). Understanding the molecular mechanism of regeneration through apoptosis-induced compensatory proliferation studies - updates and future aspects. Apoptosis 29 (9-10), 1399–1414. Epub 20240406. doi:10.1007/s10495-024-01958-1
Ratan, R. R., Lee, P. J., and Baraban, J. M. (1996). Serum deprivation inhibits glutathione depletion-induced death in embryonic cortical neurons: evidence against oxidative stress as a final common mediator of neuronal apoptosis. Neurochem. Int. 29 (2), 153–157. doi:10.1016/0197-0186(95)00115-8
Ratan, R. R., Murphy, T. H., and Baraban, J. M. (1994). Macromolecular synthesis inhibitors prevent oxidative stress-induced apoptosis in embryonic cortical neurons by shunting cysteine from protein synthesis to glutathione. J. Neurosci. 14 (7), 4385–4392. doi:10.1523/jneurosci.14-07-04385.1994
Ren, F., Yang, Y., Wu, K., Zhao, T., Shi, Y., Song, M., et al. (2021). The effects of dandelion polysaccharides on iron metabolism by regulating hepcidin via jak/stat signaling pathway. Oxid. Med. Cell Longev. 2021, 7184760. Epub 20210102. doi:10.1155/2021/7184760
Riaz, M., Al, K. L. T., Atzaz, N., Alattar, A., Alshaman, R., Shah, F. A., et al. (2022). Carvacrol alleviates hyperuricemia-induced oxidative stress and inflammation by modulating the nlrp3/nf-κb pathwayt. Drug Des. Devel Ther. 16, 1159–1170. Epub 20220422. doi:10.2147/dddt.S343978
Richette, P., Eymard, C., Deberg, M., Vidaud, D., de Kerguenec, C., Valla, D., et al. (2010). Increase in type ii collagen turnover after iron depletion in patients with hereditary haemochromatosis. Rheumatol. Oxf. 49 (4), 760–766. Epub 20100122. doi:10.1093/rheumatology/kep429
Rochette, L., Dogon, G., Rigal, E., Zeller, M., Cottin, Y., and Vergely, C. (2022). Lipid peroxidation and iron metabolism: two corner stones in the homeostasis control of ferroptosis. Int. J. Mol. Sci. 24 (1), 449. Epub 20221227. doi:10.3390/ijms24010449
Rohini, M., Haritha Menon, A., and Selvamurugan, N. (2018). Role of activating transcription factor 3 and its interacting proteins under physiological and pathological conditions. Int. J. Biol. Macromol. 120 (Pt A), 310–317. Epub 20180823. doi:10.1016/j.ijbiomac.2018.08.107
Rong, T., Jia, N., Wu, B., Sang, D., and Liu, B. (2022). New insights into the regulatory role of ferroptosis in ankylosing spondylitis via consensus clustering of ferroptosis-related genes and weighted gene Co-expression network analysis. Genes (Basel) 13 (8), 1373. doi:10.3390/genes13081373
Rosell, R., Jain, A., Codony-Servat, J., Jantus-Lewintre, E., Morrison, B., Ginesta, J. B., et al. (2023). Biological insights in non-small cell lung cancer. Cancer Biol. Med. 20 (7), 500–518. doi:10.20892/j.issn.2095-3941.2023.0108
Rostom, S., Dougados, M., and Gossec, L. (2010). New tools for diagnosing spondyloarthropathy. Jt. Bone Spine 77 (2), 108–114. Epub 20100212. doi:10.1016/j.jbspin.2009.12.005
Ruan, L., Cai, X., Qian, R., Bei, S., Wu, L., Cao, J., et al. (2024). Live macrophages loaded with Fe(3)O(4) and sulfasalazine for ferroptosis and photothermal therapy of rheumatoid arthritis. Mater Today Bio 24, 100925. Epub 20231220. doi:10.1016/j.mtbio.2023.100925
Ryter, S. W. (2021). Heme oxgenase-1, a cardinal modulator of regulated cell death and inflammation. Cells 10 (3), 515. Epub 20210228. doi:10.3390/cells10030515
Sabari, B. R., Zhang, D., Allis, C. D., and Zhao, Y. (2017). Metabolic regulation of gene expression through histone acylations. Nat. Rev. Mol. Cell Biol. 18 (2), 90–101. Epub 20161207. doi:10.1038/nrm.2016.140
Sahoo, B. M., Banik, B. K., Borah, P., and Jain, A. (2022). Reactive oxygen species (ros): key components in cancer therapies. Anticancer Agents Med. Chem. 22 (2), 215–222. doi:10.2174/1871520621666210608095512
Saito, T., Fukai, A., Mabuchi, A., Ikeda, T., Yano, F., Ohba, S., et al. (2010). Transcriptional regulation of endochondral ossification by hif-2alpha during skeletal growth and osteoarthritis development. Nat. Med. 16 (6), 678–686. Epub 20100523. doi:10.1038/nm.2146
Salama, S. A., and Kabel, A. M. (2020). Taxifolin ameliorates iron overload-induced hepatocellular injury: modulating pi3k/akt and P38 mapk signaling, inflammatory response, and hepatocellular regeneration. Chem. Biol. Interact. 330, 109230. Epub 20200820. doi:10.1016/j.cbi.2020.109230
Sandhu, G., and Thelma, B. K. (2022). New druggable targets for rheumatoid arthritis based on insights from synovial biology. Front. Immunol. 13, 834247. Epub 20220221. doi:10.3389/fimmu.2022.834247
Sanford, M., and McCormack, P. L. (2011). Eldecalcitol: a review of its use in the treatment of osteoporosis. Drugs 71 (13), 1755–1770. doi:10.2165/11206790-000000000-00000
Schito, L., and Semenza, G. L. (2016). Hypoxia-inducible factors: master regulators of cancer progression. Trends Cancer 2 (12), 758–770. Epub 20161116. doi:10.1016/j.trecan.2016.10.016
Seiler, A., Schneider, M., Förster, H., Roth, S., Wirth, E. K., Culmsee, C., et al. (2008). Glutathione peroxidase 4 senses and translates oxidative stress into 12/15-lipoxygenase dependent- and aif-mediated cell death. Cell Metab. 8 (3), 237–248. doi:10.1016/j.cmet.2008.07.005
Shan, L., Xu, X., Zhang, J., Cai, P., Gao, H., Lu, Y., et al. (2021). Increased hemoglobin and heme in maldi-tof ms analysis induce ferroptosis and promote degeneration of herniated human Nucleus pulposus. Mol. Med. 27 (1), 103. Epub 20210908. doi:10.1186/s10020-021-00368-2
Shao, Z. C., Sun, W. K., Deng, Q. Q., Cheng, L., Huang, X., Hu, L. K., et al. (2024). Identification of key lncrnas in gout under copper death and iron death mechanisms: a study based on cerna network analysis and random forest algorithm. Mol. Biotechnol. Epub 20240312. doi:10.1007/s12033-024-01099-5
Shi, F., Zhang, Z., Cui, H., Wang, J., Wang, Y., Tang, Y., et al. (2022). Analysis by transcriptomics and metabolomics for the proliferation inhibition and dysfunction through redox imbalance-mediated DNA damage response and ferroptosis in male reproduction of mice and Tm4 sertoli cells exposed to Pm(2.5). Ecotoxicol. Environ. Saf. 238, 113569. Epub 20220502. doi:10.1016/j.ecoenv.2022.113569
Shi, Y., Gong, M., Deng, Z., Liu, H., Chang, Y., Yang, Z., et al. (2021). Tirapazamine suppress osteosarcoma cells in part through Slc7a11 mediated ferroptosis. Biochem. Biophys. Res. Commun. 567, 118–124. Epub 20210617. doi:10.1016/j.bbrc.2021.06.036
Shimada, K., Hayano, M., Pagano, N. C., and Stockwell, B. R. (2016). Cell-line selectivity improves the predictive power of pharmacogenomic analyses and helps identify nadph as biomarker for ferroptosis sensitivity. Cell Chem. Biol. 23 (2), 225–235. Epub 20160204. doi:10.1016/j.chembiol.2015.11.016
Shu, W., Baumann, B. H., Song, Y., Liu, Y., Wu, X., and Dunaief, J. L. (2020). Ferrous but not ferric iron sulfate kills photoreceptors and induces photoreceptor-dependent rpe autofluorescence. Redox Biol. 34, 101469. Epub 2020/05/05. doi:10.1016/j.redox.2020.101469
Singhal, R., Mitta, S. R., Das, N. K., Kerk, S. A., Sajjakulnukit, P., Solanki, S., et al. (2021). Hif-2α activation potentiates oxidative cell death in colorectal cancers by increasing cellular iron. J. Clin. investigation 131 (12), e143691. doi:10.1172/JCI143691
Sommerfeldt, D. W., and Rubin, C. T. (2001). Biology of bone and how it orchestrates the form and function of the skeleton. Eur. Spine J. 10, S86–S95. doi:10.1007/s005860100283
Song, X., Liu, J., Kuang, F., Chen, X., Zeh, H. J., Kang, R., et al. (2021). Pdk4 dictates metabolic resistance to ferroptosis by suppressing pyruvate oxidation and fatty acid synthesis. Cell Rep. 34 (8), 108767. doi:10.1016/j.celrep.2021.108767
Sonveaux, P., Végran, F., Schroeder, T., Wergin, M. C., Verrax, J., Rabbani, Z. N., et al. (2008). Targeting lactate-fueled respiration selectively kills hypoxic tumor cells in mice. J. Clin. Invest 118 (12), 3930–3942. Epub 20081120. doi:10.1172/jci36843
Stefanova, K. I., Delcheva, G. T., Maneva, A. I., Batalov, A. Z., Geneva-Popova, M. G., Karalilova, R. V., et al. (2018). Pathobiochemical mechanisms relating iron homeostasis with parameters of inflammatory activity and autoimmune disorders in rheumatoid arthritis. Folia Med. Plovdiv. 60 (1), 124–132. doi:10.1515/folmed-2017-0068
Stockwell, B. R. (2022). Ferroptosis turns 10: emerging mechanisms, physiological functions, and therapeutic applications. Cell 185 (14), 2401–2421. doi:10.1016/j.cell.2022.06.003
Stockwell, B. R., Friedmann Angeli, J. P., Bayir, H., Bush, A. I., Conrad, M., Dixon, S. J., et al. (2017). Ferroptosis: a regulated cell death nexus linking metabolism, redox biology, and disease. Cell 171 (2), 273–285. doi:10.1016/j.cell.2017.09.021
Stoyanovsky, D. A., Tyurina, Y. Y., Shrivastava, I., Bahar, I., Tyurin, V. A., Protchenko, O., et al. (2019). Iron catalysis of lipid peroxidation in ferroptosis: regulated enzymatic or random free radical reaction? Free Radic. Biol. Med. 133, 153–161. Epub 20180912. doi:10.1016/j.freeradbiomed.2018.09.008
Su, Z., Yang, Z., Xu, Y., Chen, Y., and Yu, Q. (2015). Apoptosis, autophagy, necroptosis, and cancer metastasis. Mol. Cancer 14, 48. Epub 20150221. doi:10.1186/s12943-015-0321-5
Sui, S., Zhang, J., Xu, S., Wang, Q., Wang, P., and Pang, D. (2019). Ferritinophagy is required for the induction of ferroptosis by the bromodomain protein Brd4 inhibitor (+)-Jq1 in cancer cells. Cell Death Dis. 10 (5), 331. Epub 20190415. doi:10.1038/s41419-019-1564-7
Sun, M., Wang, Q., Huang, J., Sun, Q., Yu, Q., Liu, X., et al. (2024). Asiatic acid induces ferroptosis of Ra-fls via the nrf2/hmox1 pathway to relieve inflammation in rheumatoid arthritis. Int. Immunopharmacol. 137, 112394. Epub 20240608. doi:10.1016/j.intimp.2024.112394
Sun, Q. A., Kirnarsky, L., Sherman, S., and Gladyshev, V. N. (2001). Selenoprotein Oxidoreductase with specificity for thioredoxin and glutathione systems. Proc. Natl. Acad. Sci. U. S. A. 98 (7), 3673–3678. Epub 20010320. doi:10.1073/pnas.051454398
Sun, Y., Chen, P., Zhai, B., Zhang, M., Xiang, Y., Fang, J., et al. (2020). The emerging role of ferroptosis in inflammation. Biomed. Pharmacother. 127, 110108. doi:10.1016/j.biopha.2020.110108
Suzuki, S., Venkatesh, D., Kanda, H., Nakayama, A., Hosokawa, H., Lee, E., et al. (2022). Gls2 is a tumor suppressor and a regulator of ferroptosis in hepatocellular carcinoma. Cancer Res. 82 (18), 3209–3222. doi:10.1158/0008-5472.Can-21-3914
Tabas, I., and Ron, D. (2011). Integrating the mechanisms of apoptosis induced by endoplasmic reticulum stress. Nat. Cell Biol. 13 (3), 184–190. doi:10.1038/ncb0311-184
Takatori, H., Kawashima, H., Suzuki, K., and Nakajima, H. (2014). Role of P53 in systemic autoimmune diseases. Crit. Rev. Immunol. 34 (6), 509–516. doi:10.1615/critrevimmunol.2014012193
Tam, L. S., Gu, J., and Yu, D. (2010). Pathogenesis of ankylosing spondylitis. Nat. Rev. Rheumatol. 6 (7), 399–405. Epub 20100601. doi:10.1038/nrrheum.2010.79
Tang, D., Chen, X., and Kroemer, G. (2022). Cuproptosis: a copper-triggered modality of mitochondrial cell death. Cell Res. 32 (5), 417–418. doi:10.1038/s41422-022-00653-7
Tang, Z., Dong, H., Li, T., Wang, N., Wei, X., Wu, H., et al. (2021). The synergistic reducing drug resistance effect of cisplatin and ursolic acid on osteosarcoma through a multistep mechanism involving ferritinophagy. Oxid. Med. Cell Longev. 2021, 5192271. Epub 20211221. doi:10.1155/2021/5192271
Tano, J. Y., and Gollasch, M. (2014). Hypoxia and ischemia-reperfusion: a bik contribution? Am. J. Physiol. Heart Circ. Physiol. 307 (6), H811–H817. Epub 20140711. doi:10.1152/ajpheart.00319.2014
Thayyullathil, F., Cheratta, A. R., Alakkal, A., Subburayan, K., Pallichankandy, S., Hannun, Y. A., et al. (2021). Acid sphingomyelinase-dependent autophagic degradation of Gpx4 is critical for the execution of ferroptosis. Cell Death Dis. 12 (1), 26. Epub 20210107. doi:10.1038/s41419-020-03297-w
Thomas, G. P., and Brown, M. A. (2010). Genomics of ankylosing spondylitis. Discov. Med. 10 (52), 263–271.
Thomas, J. P., Geiger, P. G., Maiorino, M., Ursini, F., and Girotti, A. W. (1990). Enzymatic reduction of phospholipid and cholesterol hydroperoxides in artificial bilayers and lipoproteins. Biochim. Biophys. Acta 1045 (3), 252–260. doi:10.1016/0005-2760(90)90128-k
Tian, B., Li, X., Li, W., Shi, Z., He, X., Wang, S., et al. (2024). Cryab suppresses ferroptosis and promotes osteogenic differentiation of human bone marrow stem cells via binding and stabilizing Fth1. Aging (Albany NY) 16 (10), 8965–8979. Epub 20240522. doi:10.18632/aging.205851
Tian, Q., Wu, S., Dai, Z., Yang, J., Zheng, J., Zheng, Q., et al. (2016). Iron overload induced death of osteoblasts in vitro: involvement of the mitochondrial apoptotic pathway. PeerJ 4, e2611. Epub 20161108. doi:10.7717/peerj.2611
Tian, Y., Lu, J., Hao, X., Li, H., Zhang, G., Liu, X., et al. (2020). Fth1 inhibits ferroptosis through ferritinophagy in the 6-ohda model of Parkinson's disease. Neurotherapeutics 17 (4), 1796–1812. Epub 20200921. doi:10.1007/s13311-020-00929-z
Torretta, S., Scagliola, A., Ricci, L., Mainini, F., Di Marco, S., Cuccovillo, I., et al. (2020). D-mannose suppresses macrophage il-1β production. Nat. Commun. 11 (1), 6343. Epub 20201211. doi:10.1038/s41467-020-20164-6
Tower, J. (2015). Programmed cell death in aging. Ageing Res. Rev. 23 (Pt A), 90–100. Epub 20150408. doi:10.1016/j.arr.2015.04.002
Toyokuni, S., Ito, F., Yamashita, K., Okazaki, Y., and Akatsuka, S. (2017). Iron and thiol redox signaling in cancer: an exquisite balance to escape ferroptosis. Free Radic. Biol. Med. 108, 610–626. Epub 20170419. doi:10.1016/j.freeradbiomed.2017.04.024
Traber, M. G., and Atkinson, J. (2007). Vitamin E, antioxidant and nothing more. Free Radic. Biol. Med. 43 (1), 4–15. doi:10.1016/j.freeradbiomed.2007.03.024
Trenor, C. C., Campagna, D. R., Sellers, V. M., Andrews, N. C., and Fleming, M. D. (2000). The molecular defect in hypotransferrinemic mice. Blood 96 (3), 1113–1118. doi:10.1182/blood.v96.3.1113
Tsvetkov, P., Coy, S., Petrova, B., Dreishpoon, M., Verma, A., Abdusamad, M., et al. (2022). Copper induces cell death by targeting lipoylated tca cycle proteins. Science. 375(6586), 1254–1261. doi:10.1126/science.abf0529
Uchida, T., Akitsuki, T., Kimura, H., Tanaka, T., Matsuda, S., and Kariyone, S. (1983). Relationship among plasma iron, plasma iron turnover, and reticuloendothelial iron release. Blood 61 (4), 799–802. doi:10.1182/blood.v61.4.799.799
Ursini, F., Maiorino, M., and Gregolin, C. (1985). The selenoenzyme phospholipid hydroperoxide glutathione peroxidase. Biochim. Biophys. Acta 839 (1), 62–70. doi:10.1016/0304-4165(85)90182-5
Ursini, F., Maiorino, M., Valente, M., Ferri, L., and Gregolin, C. (1982). Purification from pig liver of a protein which protects liposomes and biomembranes from peroxidative degradation and exhibits glutathione peroxidase activity on phosphatidylcholine hydroperoxides. Biochim. Biophys. Acta 710 (2), 197–211. doi:10.1016/0005-2760(82)90150-3
van Vulpen, L. F. D., Holstein, K., and Martinoli, C. (2018). Joint disease in haemophilia: pathophysiology, pain and imaging. Haemophilia 24 (Suppl. 6), 44–49. doi:10.1111/hae.13449
Varn, F. S., Johnson, K. C., Martinek, J., Huse, J. T., Nasrallah, M. P., Wesseling, P., et al. (2022). Glioma progression is shaped by genetic evolution and microenvironment interactions. Cell 185 (12), 2184–2199.e16. Epub 20220531. doi:10.1016/j.cell.2022.04.038
Walter, P., and Ron, D. (2011). The unfolded protein response: from stress pathway to homeostatic regulation. Science 334 (6059), 1081–1086. doi:10.1126/science.1209038
Wang, B., Zhan, Y., Yan, L., and Hao, D. (2022f). How zoledronic acid improves osteoporosis by acting on osteoclasts. Front. Pharmacol. 13, 961941. Epub 20220825. doi:10.3389/fphar.2022.961941
Wang, C. Y., and Babitt, J. L. (2019). Liver iron sensing and body iron homeostasis. Blood 133 (1), 18–29. Epub 20181106. doi:10.1182/blood-2018-06-815894
Wang, F., Li, J., Zhao, Y., Guo, D., Liu, D., Chang, S., et al. (2022d). Mir-672-3p promotes functional recovery in rats with contusive spinal cord injury by inhibiting ferroptosis suppressor protein 1. Oxid. Med. Cell Longev. 2022, 6041612. Epub 20220221. doi:10.1155/2022/6041612
Wang, H., Li, J., Follett, P. L., Zhang, Y., Cotanche, D. A., Jensen, F. E., et al. (2004). 12-Lipoxygenase plays a key role in cell death caused by glutathione depletion and arachidonic acid in rat oligodendrocytes. Eur. J. Neurosci. 20 (8), 2049–2058. doi:10.1111/j.1460-9568.2004.03650.x
Wang, H., Tang, Z., Liu, S., Xie, K., and Zhang, H. (2023c). Acrylamide induces human chondrocyte cell death by initiating autophagy-dependent ferroptosis. Exp. Ther. Med. 25 (6), 246. Epub 20230412. doi:10.3892/etm.2023.11945
Wang, H., Wu, Q., Qu, P., Wang, S., Du, S., Peng, Z., et al. (2024a). Diet affects inflammatory arthritis: a mendelian randomization study of 30 dietary patterns causally associated with inflammatory arthritis. Front. Nutr. 11, 1426125. Epub 20240717. doi:10.3389/fnut.2024.1426125
Wang, H., Zhang, M., Hu, Y., He, J., Zhong, Y., Dai, Y., et al. (2024b). Deciphering the role of ferroptosis in rheumatoid arthritis: synovial transcriptome analysis and immune infiltration correlation. Heliyon 10 (13), e33648. Epub 20240626. doi:10.1016/j.heliyon.2024.e33648
Wang, J., and Pantopoulos, K. (2011). Regulation of cellular iron metabolism. Biochem. J. 434 (3), 365–381. doi:10.1042/bj20101825
Wang, L., Liu, Y., Du, T., Yang, H., Lei, L., Guo, M., et al. (2020). Atf3 promotes erastin-induced ferroptosis by suppressing system Xc. Cell Death Differ. 27 (2), 662–675. Epub 20190704. doi:10.1038/s41418-019-0380-z
Wang, L., and Pan, S. (2023). The regulatory effects of P53 on the typical and atypical ferroptosis in the pathogenesis of osteosarcoma: a systematic review. Front. Genet. 14, 1154299. Epub 20230329. doi:10.3389/fgene.2023.1154299
Wang, N., Ma, T., and Yu, B. (2023a). Targeting epigenetic regulators to overcome drug resistance in cancers. Signal Transduct. Target Ther. 8 (1), 69. Epub 20230217. doi:10.1038/s41392-023-01341-7
Wang, S., Li, W., Zhang, P., Wang, Z., Ma, X., Liu, C., et al. (2022a). Mechanical overloading induces gpx4-regulated chondrocyte ferroptosis in osteoarthritis via Piezo1 channel facilitated calcium influx. J. Adv. Res. 41, 63–75. Epub 20220111. doi:10.1016/j.jare.2022.01.004
Wang, T. X., Liang, J. Y., Zhang, C., Xiong, Y., Guan, K. L., and Yuan, H. X. (2019). The oncometabolite 2-hydroxyglutarate produced by mutant Idh1 sensitizes cells to ferroptosis. Cell Death Dis. 10 (10), 755. Epub 20191007. doi:10.1038/s41419-019-1984-4
Wang, W., Jing, X., Du, T., Ren, J., Liu, X., Chen, F., et al. (2022c). Iron overload promotes intervertebral disc degeneration via inducing oxidative stress and ferroptosis in endplate chondrocytes. Free Radic. Biol. Med. 190, 234–246. Epub 20220815. doi:10.1016/j.freeradbiomed.2022.08.018
Wang, W., Lu, K., Jiang, X., Wei, Q., Zhu, L., Wang, X., et al. (2023b). Ferroptosis inducers enhanced cuproptosis induced by copper ionophores in primary liver cancer. J. Exp. & Clin. Cancer Res. 42 (1), 142. doi:10.1186/s13046-023-02720-2
Wang, X., Liu, Z., Peng, P., Gong, Z., Huang, J., and Peng, H. (2022e). Astaxanthin attenuates osteoarthritis progression via inhibiting ferroptosis and regulating mitochondrial function in chondrocytes. Chem. Biol. Interact. 366, 110148. Epub 20220907. doi:10.1016/j.cbi.2022.110148
Wang, X., Ma, H., Sun, J., Zheng, T., Zhao, P., Li, H., et al. (2022b). Mitochondrial ferritin deficiency promotes osteoblastic ferroptosis via mitophagy in type 2 diabetic osteoporosis. Biol. Trace Elem. Res. 200 (1), 298–307. Epub 20210216. doi:10.1007/s12011-021-02627-z
Wang, X., Wang, Q., Li, G., Xu, H., Liu, B., Yuan, B., et al. (2024d). Identifying the protective effects of mir-874-3p/atf3 Axis in intervertebral disc degeneration by single-cell rna sequencing and validation. J. Cell Mol. Med. 28 (12), e18492. doi:10.1111/jcmm.18492
Wang, Y., Ding, H., Zheng, Y., Wei, X., Yang, X., Wei, H., et al. (2024c). Alleviated ncoa4-mediated ferritinophagy protected Ra flss from ferroptosis in lipopolysaccharide-induced inflammation under hypoxia. Inflamm. Res. 73 (3), 363–379. Epub 20240108. doi:10.1007/s00011-023-01842-9
Wang, Y., Quan, F., Cao, Q., Lin, Y., Yue, C., Bi, R., et al. (2021b). Quercetin alleviates acute kidney injury by inhibiting ferroptosis. J. Adv. Res. 28, 231–243. Epub 20200722. doi:10.1016/j.jare.2020.07.007
Wang, Y., Zhang, L., Zhao, G., Zhang, Y., Zhan, F., Chen, Z., et al. (2022g). Homologous targeting nanoparticles for enhanced pdt against osteosarcoma hos cells and the related molecular mechanisms. J. Nanobiotechnology 20 (1), 83. Epub 20220217. doi:10.1186/s12951-021-01201-y
Wang, Z., Chen, X., Liu, N., Shi, Y., Liu, Y., Ouyang, L., et al. (2021a). A nuclear long non-coding rna Linc00618 accelerates ferroptosis in a manner dependent upon apoptosis. Mol. Ther. 29 (1), 263–274. Epub 20200920. doi:10.1016/j.ymthe.2020.09.024
Wei, D., Ke, Y. Q., Duan, P., Zhou, L., Wang, C. Y., and Cao, P. (2021). Microrna-302a-3p induces ferroptosis of non-small cell lung cancer cells via targeting ferroportin. Free Radic. Res. 55 (7), 821–830. Epub 20210806. doi:10.1080/10715762.2021.1947503
Weichhart, T. (2024). Transferrin: the iron transporter takes control. Blood 144 (1), 9–10. doi:10.1182/blood.2024024731
West, A. R., and Oates, P. S. (2008). Mechanisms of heme iron absorption: current questions and controversies. World J. Gastroenterol. 14 (26), 4101–4110. doi:10.3748/wjg.14.4101
Wortmann, M., Schneider, M., Pircher, J., Hellfritsch, J., Aichler, M., Vegi, N., et al. (2013). Combined deficiency in glutathione peroxidase 4 and vitamin E causes multiorgan thrombus formation and early death in mice. Circ. Res. 113 (4), 408–417. Epub 20130614. doi:10.1161/circresaha.113.279984
Wright, T. L., Brissot, P., Ma, W. L., and Weisiger, R. A. (1986). Characterization of non-transferrin-bound iron clearance by rat liver. J. Biol. Chem. 261 (23), 10909–10914. doi:10.1016/s0021-9258(18)67473-3
Wu, B. (2024). Genetically predicted iron status is a causal risk of rheumatoid arthritis: a mendelian randomization study. Glob. Med. Genet. 11 (4), 270–277. Epub 20240829. doi:10.1055/s-0044-1789259
Wu, H., Wang, Y., Huang, J., Li, Y., Lin, Z., and Zhang, B. (2023b). Rutin ameliorates gout via reducing xod activity, inhibiting ros production and Nlrp3 inflammasome activation in quail. Biomed. Pharmacother. 158, 114175. Epub 20221230. doi:10.1016/j.biopha.2022.114175
Wu, J., Feng, Z., Chen, L., Li, Y., Bian, H., Geng, J., et al. (2022). Tnf antagonist sensitizes synovial fibroblasts to ferroptotic cell death in collagen-induced arthritis mouse models. Nat. Commun. 13 (1), 676. Epub 20220203. doi:10.1038/s41467-021-27948-4
Wu, P., Li, C., Ye, D. M., Yu, K., Li, Y., Tang, H., et al. (2021). Circular rna Circepsti1 accelerates cervical cancer progression via mir-375/409-3p/515-5p-slc7a11 Axis. Aging (Albany NY) 13 (3), 4663–4673. Epub 20210202. doi:10.18632/aging.202518
Wu, P., Zhang, X., Duan, D., and Zhao, L. (2023a). Organelle-specific mechanisms in crosstalk between apoptosis and ferroptosis. Oxid. Med. Cell Longev. 2023, 3400147. Epub 20230105. doi:10.1155/2023/3400147
Wylenzek, F., Bühling, K. J., and Laakmann, E. (2024). A systematic review on the impact of nutrition and possible supplementation on the deficiency of vitamin complexes, iron, omega-3-fatty acids, and lycopene in relation to increased morbidity in women after menopause. Arch. Gynecol. Obstet. 310 (4), 2235–2245. Epub 20240627. doi:10.1007/s00404-024-07555-6
Xiang, J., Chen, H., Lin, Z., Chen, J., and Luo, L. (2023). Identification and experimental validation of ferroptosis-related gene Slc2a3 is involved in rheumatoid arthritis. Eur. J. Pharmacol. 943, 175568. Epub 20230202. doi:10.1016/j.ejphar.2023.175568
Xiao, Z. F., Su, G. Y., Hou, Y., Chen, S. D., Zhao, B. D., He, J. B., et al. (2020). Mechanics and biology interact in intervertebral disc degeneration: a novel composite mouse model. Calcif. Tissue Int. 106 (4), 401–414. Epub 20200107. doi:10.1007/s00223-019-00644-8
Xie, S. S., Deng, Y., Guo, S. L., Li, J. Q., Zhou, Y. C., Liao, J., et al. (2022). Endothelial cell ferroptosis mediates monocrotaline-induced pulmonary hypertension in rats by modulating Nlrp3 inflammasome activation. Sci. Rep. 12 (1), 3056. Epub 20220223. doi:10.1038/s41598-022-06848-7
Xie, Y., Hou, W., Song, X., Yu, Y., Huang, J., Sun, X., et al. (2016). Ferroptosis: process and function. Cell Death Differ. 23 (3), 369–379. Epub 20160122. doi:10.1038/cdd.2015.158
Xie, Y., Kang, R., Klionsky, D. J., and Tang, D. (2023). Gpx4 in cell death, autophagy, and disease. Autophagy 19 (10), 2621–2638. doi:10.1080/15548627.2023.2218764
Xiong, L., Li, X., Hua, X., and Qian, Z. (2023). Circ-Stc2 promotes the ferroptosis of Nucleus pulposus cells via targeting mir-486-3p/tfr2 Axis. J. Orthop. Surg. Res. 18 (1), 518. Epub 20230721. doi:10.1186/s13018-023-04010-1
Xu, C., Ni, S., Xu, N., Yin, G., Yu, Y., Zhou, B., et al. (2022b). Theaflavin-3,3'-Digallate inhibits erastin-induced chondrocytes ferroptosis via the nrf2/gpx4 signaling pathway in osteoarthritis. Oxid. Med. Cell Longev. 2022, 3531995. doi:10.1155/2022/3531995
Xu, C. Y., Xu, C., Xu, Y. N., Du, S. Q., Dai, Z. H., Jin, S. Q., et al. (2024). Poliumoside protects against type 2 diabetes-related osteoporosis by suppressing ferroptosis via activation of the nrf2/gpx4 pathway. Phytomedicine 125, 155342. Epub 20240107. doi:10.1016/j.phymed.2024.155342
Xu, J., Zhang, S., Tian, Y., Si, H., Zeng, Y., Wu, Y., et al. (2022a). Genetic causal association between iron status and osteoarthritis: a two-sample mendelian randomization. Nutrients 14 (18), 3683. Epub 20220906. doi:10.3390/nu14183683
Xu, P., Lin, B., Deng, X., Huang, K., Zhang, Y., and Wang, N. (2022c). Vdr activation attenuates osteoblastic ferroptosis and senescence by stimulating the nrf2/gpx4 pathway in age-related osteoporosis. Free Radic. Biol. Med. 193 (Pt 2), 720–735. Epub 20221117. doi:10.1016/j.freeradbiomed.2022.11.013
Xu, Q., Zhou, L., Yang, G., Meng, F., Wan, Y., Wang, L., et al. (2020). Circil4r facilitates the tumorigenesis and inhibits ferroptosis in hepatocellular carcinoma by regulating the mir-541-3p/gpx4 Axis. Cell Biol. Int. 44 (11), 2344–2356. Epub 20200831. doi:10.1002/cbin.11444
Xu, R., Yang, J., Qian, Y., Deng, H., Wang, Z., Ma, S., et al. (2021a). Ferroptosis/pyroptosis dual-inductive combinational anti-cancer therapy achieved by transferrin decorated nanomof. Nanoscale Horiz. 6 (4), 348–356. Epub 20210309. doi:10.1039/d0nh00674b
Xu, Z., Chen, L., Wang, C., Zhang, L., and Xu, W. (2021b). Microrna-1287-5p promotes ferroptosis of osteosarcoma cells through inhibiting Gpx4. Free Radic. Res. 55 (11-12), 1119–1129. Epub 20220117. doi:10.1080/10715762.2021.2024816
Yadav, P., Sharma, P., Sundaram, S., Venkatraman, G., Bera, A. K., and Karunagaran, D. (2021). Slc7a11/xct is a target of mir-5096 and its restoration partially rescues mir-5096-mediated ferroptosis and anti-tumor effects in human breast cancer cells. Cancer Lett. 522, 211–224. Epub 20210924. doi:10.1016/j.canlet.2021.09.033
Yagi, K., Komura, S., Kojima, H., Sun, Q., Nagata, N., Ohishi, N., et al. (1996). Expression of human phospholipid hydroperoxide glutathione peroxidase gene for protection of host cells from lipid hydroperoxide-mediated injury. Biochem. Biophys. Res. Commun. 219 (2), 486–491. doi:10.1006/bbrc.1996.0260
Yagoda, N., von Rechenberg, M., Zaganjor, E., Bauer, A. J., Yang, W. S., Fridman, D. J., et al. (2007). Ras-raf-mek-dependent oxidative cell death involving voltage-dependent anion channels. Nature 447 (7146), 864–868. doi:10.1038/nature05859
Yan, H. F., Zou, T., Tuo, Q. Z., Xu, S., Li, H., Belaidi, A. A., et al. (2021). Ferroptosis: mechanisms and links with diseases. Signal Transduct. Target Ther. 6 (1), 49. Epub 20210203. doi:10.1038/s41392-020-00428-9
Yang, J., Dong, D., Luo, X., Zhou, J., Shang, P., and Zhang, H. (2020c). Iron overload-induced osteocyte apoptosis stimulates osteoclast differentiation through increasing osteocytic rankl production in vitro. Calcif. Tissue Int. 107 (5), 499–509. Epub 20200929. doi:10.1007/s00223-020-00735-x
Yang, L., Wang, H., Yang, X., Wu, Q., An, P., Jin, X., et al. (2020a). Auranofin mitigates systemic iron overload and induces ferroptosis via distinct mechanisms. Signal Transduct. Target Ther. 5 (1), 138. Epub 20200731. doi:10.1038/s41392-020-00253-0
Yang, M., Luo, H., Yi, X., Wei, X., and Jiang, D. S. (2020b). The epigenetic regulatory mechanisms of ferroptosis and its implications for biological processes and diseases. MedComm 4 (3), e267. Epub 20230522. doi:10.1002/mco2.267
Yang, R. Z., Xu, W. N., Zheng, H. L., Zheng, X. F., Li, B., Jiang, L. S., et al. (2021a). Exosomes derived from vascular endothelial cells antagonize glucocorticoid-induced osteoporosis by inhibiting ferritinophagy with resultant limited ferroptosis of osteoblasts. J. Cell Physiol. 236 (9), 6691–6705. Epub 20210216. doi:10.1002/jcp.30331
Yang, R. Z., Xu, W. N., Zheng, H. L., Zheng, X. F., Li, B., Jiang, L. S., et al. (2021b). Involvement of oxidative stress-induced annulus fibrosus cell and Nucleus pulposus cell ferroptosis in intervertebral disc degeneration pathogenesis. J. Cell Physiol. 236 (4), 2725–2739. Epub 20200906. doi:10.1002/jcp.30039
Yang, S., Kim, J., Ryu, J. H., Oh, H., Chun, C. H., Kim, B. J., et al. (2010). Hypoxia-inducible factor-2alpha is a catabolic regulator of osteoarthritic cartilage destruction. Nat. Med. 16 (6), 687–693. Epub 20100523. doi:10.1038/nm.2153
Yang, S., Ryu, J. H., Oh, H., Jeon, J., Kwak, J. S., Kim, J. H., et al. (2015). Nampt (visfatin), a direct target of hypoxia-inducible factor-2α, is an essential catabolic regulator of osteoarthritis. Ann. Rheum. Dis. 74 (3), 595–602. Epub 20131217. doi:10.1136/annrheumdis-2013-204355
Yang, S., Zhu, Y., Shi, Y., Su, S., Liang, H., Li, S., et al. (2024b). Screening of nsaids library identifies tinoridine as a novel ferroptosis inhibitor for potential intervertebral disc degeneration therapy. Free Radic. Biol. Med. 221, 245–256. Epub 20240526. doi:10.1016/j.freeradbiomed.2024.05.040
Yang, W. S., Kim, K. J., Gaschler, M. M., Patel, M., Shchepinov, M. S., and Stockwell, B. R. (2016). Peroxidation of polyunsaturated fatty acids by lipoxygenases drives ferroptosis. Proc. Natl. Acad. Sci. U. S. A. 113 (34), E4966–E4975. Epub 20160809. doi:10.1073/pnas.1603244113
Yang, W. S., SriRamaratnam, R., Welsch, M. E., Shimada, K., Skouta, R., Viswanathan, V. S., et al. (2014). Regulation of ferroptotic cancer cell death by Gpx4. Cell 156 (1-2), 317–331. doi:10.1016/j.cell.2013.12.010
Yang, W. S., and Stockwell, B. R. (2008). Synthetic lethal screening identifies compounds activating iron-dependent, nonapoptotic cell death in oncogenic-ras-harboring cancer cells. Chem. Biol. 15 (3), 234–245. doi:10.1016/j.chembiol.2008.02.010
Yang, Y., Lin, Y., Wang, M., Yuan, K., Wang, Q., Mu, P., et al. (2022). Targeting ferroptosis suppresses osteocyte glucolipotoxicity and alleviates diabetic osteoporosis. Bone Res. 10 (1), 26. Epub 20220309. doi:10.1038/s41413-022-00198-w
Yang, Y., Zhang, X., Yang, Y., Gao, P., Fan, W., Zheng, T., et al. (2024a). A two-pronged approach to inhibit ferroptosis of mscs caused by the iron overload in postmenopausal osteoporosis and promote osseointegration of titanium implant. Bioact. Mater 41, 336–354. Epub 20240725. doi:10.1016/j.bioactmat.2024.07.024
Yao, B., Cai, Y., Wan, L., Deng, J., Zhao, L., Wang, W., et al. (2023). Bach1 promotes intervertebral disc degeneration by regulating hmox1/gpx4 to mediate oxidative stress, ferroptosis, and lipid metabolism in Nucleus pulposus cells. J. Gene Med. 25 (6), e3488. Epub 20230402. doi:10.1002/jgm.3488
Yao, X., Sun, K., Yu, S., Luo, J., Guo, J., Lin, J., et al. (2021). Chondrocyte ferroptosis contribute to the progression of osteoarthritis. J. Orthop. Transl. 27, 33–43. Epub 20201217. doi:10.1016/j.jot.2020.09.006
Yi, X., Jiang, X., Li, X., and Jiang, D. S. (2017b). Histone lysine methylation and congenital heart disease: from bench to bedside (review). Int. J. Mol. Med. 40 (4), 953–964. Epub 20170830. doi:10.3892/ijmm.2017.3115
Yi, X., Tao, Y., Lin, X., Dai, Y., Yang, T., Yue, X., et al. (2017a). Histone methyltransferase Setd2 is critical for the proliferation and differentiation of myoblasts. Biochim. Biophys. Acta Mol. Cell Res. 1864 (4), 697–707. Epub 20170124. doi:10.1016/j.bbamcr.2017.01.012
Yin, Y., Chen, G. J., Yang, C., Wang, J. J., Peng, J. F., Huang, X. F., et al. (2024). Osteocyte ferroptosis induced by atf3/tfr1 contributes to cortical bone loss during ageing. Cell Prolif. 57, e13657. Epub 20240519. doi:10.1111/cpr.13657
Yu, S., and Yao, X. (2024). Advances on immunotherapy for osteosarcoma. Mol. Cancer 23 (1), 192. Epub 20240909. doi:10.1186/s12943-024-02105-9
Yu, X., Xu, H., Liu, Q., Wang, Y., Wang, S., Lu, R., et al. (2022). Circ_0072464 shuttled by bone mesenchymal stem cell-secreted extracellular vesicles inhibits Nucleus pulposus cell ferroptosis to relieve intervertebral disc degeneration. Oxid. Med. Cell Longev. 2022, 2948090. Epub 20220629. doi:10.1155/2022/2948090
Yuan, J., and Ofengeim, D. (2024). A guide to cell death pathways. Nat. Rev. Mol. Cell Biol. 25 (5), 379–395. Epub 20231218. doi:10.1038/s41580-023-00689-6
Zarjou, A., Black, L. M., McCullough, K. R., Hull, T. D., Esman, S. K., Boddu, R., et al. (2019). Ferritin light chain confers protection against sepsis-induced inflammation and organ injury. Front. Immunol. 10, 131. Epub 20190204. doi:10.3389/fimmu.2019.00131
Zhang, F., and Liu, H. (2021). Identification of ferroptosis-associated genes exhibiting altered expression in pulmonary arterial hypertension. Math. Biosci. Eng. 18 (6), 7619–7630. doi:10.3934/mbe.2021377
Zhang, J. (2020). The osteoprotective effects of artemisinin compounds and the possible mechanisms associated with intracellular iron: a review of in vivo and in vitro studies. Environ. Toxicol. Pharmacol. 76, 103358. Epub 20200227. doi:10.1016/j.etap.2020.103358
Zhang, J., Gao, M., Niu, Y., and Sun, J. (2022b). From Dnmt1 degrader to ferroptosis promoter: drug repositioning of 6-thioguanine as a ferroptosis inducer in gastric cancer. Biochem. Biophys. Res. Commun. 603, 75–81. Epub 20220306. doi:10.1016/j.bbrc.2022.03.026
Zhang, L., Li, D., Chang, C., and Sun, Y. (2022a). Myostatin/Hif2α-Mediated ferroptosis is involved in skeletal muscle dysfunction in chronic obstructive pulmonary disease. Int. J. Chronic Obstr. Pulm. Dis. 17, 2383–2399. doi:10.2147/COPD.S377226
Zhang, S., Li, D., Fan, M., Yuan, J., Xie, C., Yuan, H., et al. (2024). Mechanism of reactive oxygen species-guided immune responses in gouty arthritis and potential therapeutic targets. Biomolecules 14 (8), 978. Epub 20240809. doi:10.3390/biom14080978
Zhang, X., Sui, S., Wang, L., Li, H., Zhang, L., Xu, S., et al. (2020). Inhibition of tumor propellant glutathione peroxidase 4 induces ferroptosis in cancer cells and enhances anticancer effect of cisplatin. J. Cell Physiol. 235 (4), 3425–3437. Epub 20190925. doi:10.1002/jcp.29232
Zhang, Y., Chen, S., Yuan, M., Xu, Y., and Xu, H. (2022c). Gout and diet: a comprehensive review of mechanisms and management. Nutrients 14 (17), 3525. Epub 20220826. doi:10.3390/nu14173525
Zhang, Y., Han, S., Kong, M., Tu, Q., Zhang, L., and Ma, X. (2021). Single-cell rna-seq analysis identifies unique chondrocyte subsets and reveals involvement of ferroptosis in human intervertebral disc degeneration. Osteoarthr. Cartil. 29 (9), 1324–1334. Epub 20210706. doi:10.1016/j.joca.2021.06.010
Zhang, Y., Li, J., Wang, Y., Jing, J., and Li, J. (2019). The roles of circular rnas in osteosarcoma. Med. Sci. Monit. 25, 6378–6382. Epub 20190825. doi:10.12659/msm.915559
Zhang, Y., Xu, L., Nevitt, M. C., Aliabadi, P., Yu, W., Qin, M., et al. (2001). Comparison of the prevalence of knee osteoarthritis between the elderly Chinese population in Beijing and whites in the United States: the Beijing osteoarthritis study. Arthritis Rheum. 44 (9), 2065–2071. doi:10.1002/1529-0131(200109)44:9<2065::Aid-art356>3.0.Co;2-z
Zhang, Y., Yang, J., Zhao, N., Wang, C., Kamar, S., Zhou, Y., et al. (2018). Progress in the chemotherapeutic treatment of osteosarcoma. Oncol. Lett. 16 (5), 6228–6237. Epub 20180912. doi:10.3892/ol.2018.9434
Zhao, C., Yu, Y., Yin, G., Xu, C., Wang, J., Wang, L., et al. (2024). Sulfasalazine promotes ferroptosis through akt-erk1/2 and P53-slc7a11 in rheumatoid arthritis. Inflammopharmacology 32 (2), 1277–1294. Epub 20240226. doi:10.1007/s10787-024-01439-6
Zheng, Q., Wang, D., Lin, R., Chen, Y., Xu, Z., and Xu, W. (2023b). Quercetin is a potential therapy for rheumatoid arthritis via targeting caspase-8 through ferroptosis and pyroptosis. J. Inflamm. Res. 16, 5729–5754. Epub 20231201. doi:10.2147/jir.S439494
Zheng, X., Liang, Y., and Zhang, C. (2023a). Ferroptosis regulated by hypoxia in cells. Cells 12 (7), 1050. doi:10.3390/cells12071050
Zhong, W., Guo, F., Chen, F., Law, M. K., Lu, J., Shao, D., et al. (2022). A multifunctional oxidative stress nanoamplifier with ros amplification and gsh exhaustion for enhanced chemodynamic therapy. Front. Pharmacol. 13, 1044083. Epub 20221110. doi:10.3389/fphar.2022.1044083
Zhou, B., Liu, J., Kang, R., Klionsky, D. J., Kroemer, G., and Tang, D. (2020). Ferroptosis is a type of autophagy-dependent cell death. Seminars cancer Biol. 66, 89–100. Epub 2019/03/19. doi:10.1016/j.semcancer.2019.03.002
Zhou, B., Zhang, J. Y., Liu, X. S., Chen, H. Z., Ai, Y. L., Cheng, K., et al. (2018). Tom20 senses iron-activated ros signaling to promote melanoma cell pyroptosis. Cell Res. 28 (12), 1171–1185. Epub 20181004. doi:10.1038/s41422-018-0090-y
Zhou, X., Zheng, Y., Sun, W., Zhang, Z., Liu, J., Yang, W., et al. (2021). D-mannose alleviates osteoarthritis progression by inhibiting chondrocyte ferroptosis in a hif-2α-dependent manner. Cell Prolif. 54 (11), e13134. Epub 20210925. doi:10.1111/cpr.13134
Zhou, Y., Zhang, Q., Stephens, O., Heuck, C. J., Tian, E., Sawyer, J. R., et al. (2012). Prediction of cytogenetic abnormalities with gene expression profiles. Blood 119 (21), e148–e150. Epub 20120410. doi:10.1182/blood-2011-10-388702
Zhu, J., Sun, R., Sun, K., Yan, C., Jiang, J., Kong, F., et al. (2023a). The Deubiquitinase Usp11 ameliorates intervertebral disc degeneration by regulating oxidative stress-induced ferroptosis via deubiquitinating and stabilizing Sirt3. Redox Biol. 62, 102707. Epub 20230420. doi:10.1016/j.redox.2023.102707
Zhu, J., Sun, R., Yan, C., Sun, K., Gao, L., Zheng, B., et al. (2023b). Hesperidin mitigates oxidative stress-induced ferroptosis in Nucleus pulposus cells via nrf2/nf-κb Axis to protect intervertebral disc from degeneration. Cell Cycle 22 (10), 1196–1214. Epub 20230413. doi:10.1080/15384101.2023.2200291
Zhu, K., Zhu, X., Sun, S., Yang, W., Liu, S., Tang, Z., et al. (2021). Inhibition of Tlr4 prevents hippocampal hypoxic-ischemic injury by regulating ferroptosis in neonatal rats. Exp. Neurol. 345, 113828. Epub 20210731. doi:10.1016/j.expneurol.2021.113828
Keywords: lipid peroxidation, antioxidant system, iron metabolism, orthopedic disorders, mechanisms, therapy
Citation: Huo G, Lin Y, Liu L, He Y, Qu Y, Liu Y, Zhu R, Wang B, Gong Q, Han Z and Yin H (2024) Decoding ferroptosis: transforming orthopedic disease management. Front. Pharmacol. 15:1509172. doi: 10.3389/fphar.2024.1509172
Received: 10 October 2024; Accepted: 22 November 2024;
Published: 06 December 2024.
Edited by:
Qi Feng, First Affiliated Hospital of Zhengzhou University, ChinaReviewed by:
Feng Zhang, Nanjing University of Chinese Medicine, ChinaCopyright © 2024 Huo, Lin, Liu, He, Qu, Liu, Zhu, Wang, Gong, Han and Yin. This is an open-access article distributed under the terms of the Creative Commons Attribution License (CC BY). The use, distribution or reproduction in other forums is permitted, provided the original author(s) and the copyright owner(s) are credited and that the original publication in this journal is cited, in accordance with accepted academic practice. No use, distribution or reproduction is permitted which does not comply with these terms.
*Correspondence: Qing Gong, MjcyNjMyNjM1QHFxLmNvbQ==; Zhongyu Han, aHp5Y3p5MTk5N0AxNjMuY29t; Hongbing Yin, MzU2NDEyODA4MEBxcS5jb20=
†These authors have contributed equally to this work
Disclaimer: All claims expressed in this article are solely those of the authors and do not necessarily represent those of their affiliated organizations, or those of the publisher, the editors and the reviewers. Any product that may be evaluated in this article or claim that may be made by its manufacturer is not guaranteed or endorsed by the publisher.
Research integrity at Frontiers
Learn more about the work of our research integrity team to safeguard the quality of each article we publish.