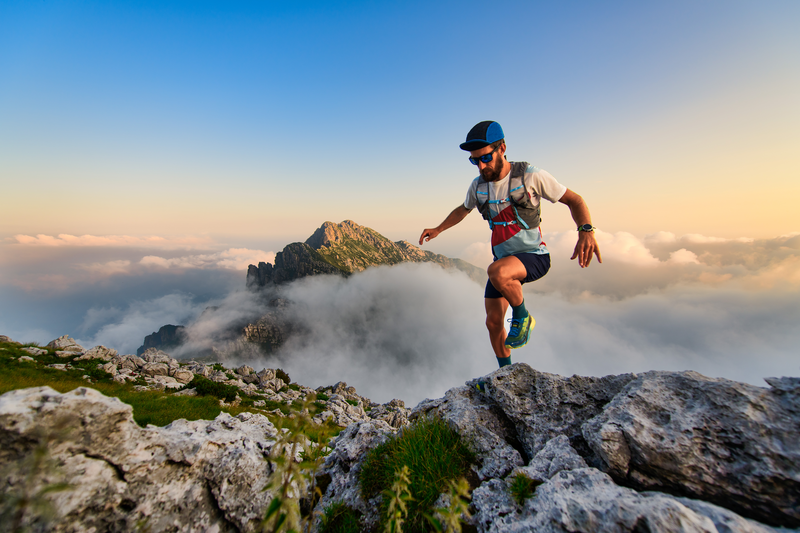
95% of researchers rate our articles as excellent or good
Learn more about the work of our research integrity team to safeguard the quality of each article we publish.
Find out more
ORIGINAL RESEARCH article
Front. Pharmacol. , 09 January 2025
Sec. Drug Metabolism and Transport
Volume 15 - 2024 | https://doi.org/10.3389/fphar.2024.1507915
Background: Dabigatran etexilate (DABE), a prodrug of dabigatran (DAB), is a direct thrombin inhibitor used to prevent ischemic stroke and thromboembolism during atrial fibrillation. The effect of genetic polymorphisms on its metabolism, particularly UGT2B15, has not been extensively explored in humans. This study aimed to investigate the effects of UGT2B15, ABCB1, and CES1 polymorphisms on the pharmacokinetics of DAB and its acylglucuronide metabolites in healthy subjects.
Methods: A total of 124 healthy males were genotyped for UGT2B15, ABCB1, and CES1 polymorphisms. After a single 150 mg dose of DABE, plasma concentrations of total and free DAB, as well as dabigatran acylglucuronide (DABG) were measured using LC-MS/MS. Pharmacokinetic parameters were analyzed using non-compartmental methods, and statistical comparisons were conducted between the genotype groups.
Results: UGT2B15 c.253G>T significantly affected free DAB pharmacokinetics, with a lower Tmax and oral clearance in TT genotype (n = 28, p < 0.05). For DABG, Cmax was significantly higher in GG genotypes (n = 32, 42.3 ± 16.3 ng/mL) compared to that in GT (n = 64, 32.4 ± 20.5 ng/mL) and TT (29.7 ± 17.1 ng/mL) genotypes. Similarly, the AUCall of DABG was highest in GG genotypes (327 ± 148.3 ng h·mL-1), followed by GT (238.7 ± 166.5 ng h·mL-1) and TT (223.3 ± 165.4 ng h·mL-1) genotypes (p < 0.05). The metabolite-to-parent ratios (m/p ratios) for Cmax and AUCall were significantly higher in GG and GT genotypes than that in TT genotype. ABCB1 and CES1 polymorphisms had no significant impact on the pharmacokinetics of DAB or DABG.
Conclusion: UGT2B15 polymorphisms were associated with difference in DAB glucuronidation and pharmacokinetics in healthy male participants.
Dabigatran etexilate (DABE), is a prodrug of dabigatran (DAB), a competitive direct thrombin inhibitor widely used in the treatment and prevention of ischemic stroke, atrial fibrillation, thrombus formation, and systemic embolism (Schellong, 2015; Feuring and van Ryn, 2016; Antonijevic et al., 2017; Blair and Keating, 2017). Routine drug monitoring is typically not required for direct oral anticoagulants, including DABE, because of their predictable pharmacokinetics (Härtig et al., 2020). The standard recommended dosage of DABE is 110 or 150 mg twice daily (Connolly et al., 2009; López-López et al., 2017), with adjustments based on individual-factors such as renal function, body weight, age, concurrent use of P-glycoprotein inhibitors, and bleeding risk (Gong and Kim, 2013; Ferri et al., 2022).
As an ester prodrug, DABE undergoes two sequential activation steps to form its active drug, DAB. Initially, DABE is metabolized to dabigatran ethyl ester (M2) by carboxylesterase 2 (CES2) in the intestine (Blech et al., 2008; Antonijevic et al., 2017; Laizure et al., 2022). Subsequently, M2 is converted to DAB by CES1 in the liver. DAB is further metabolized to dabigatran acylglucuronide (DABG) by uridine 5-diphospho (UDP)-glucuronosyltransferase (UGT) enzymes in the liver, with glucuronidation of the carboxylate moiety being the predominant metabolic pathway in humans (Ebner et al., 2010). Among the UGTs, UGT2B15 has been suggested to be the major isoform responsible for DAB glucuronidation (Ebner et al., 2010; Moj et al., 2019).
Given the complexity of the enzymes and transporters involved in DAB metabolism, genetic polymorphisms that affect the function and expression of these enzymes and transporters may contribute to inter-individual variability in DAB metabolism. Several studies have evaluated the clinical impact of ABCB1 and CES1 single nucleotide polymorphisms (SNPs) on DAB metabolism and pharmacokinetics (Ji et al., 2021); however, the data generally suggest only minor effects on DAB metabolism (Dimatteo et al., 2016; Ji et al., 2021). The effect of UGT2B15 SNPs on DAB metabolism, particularly the its impact on DABG formation, has not been extensively explored in humans (Ebner et al., 2010). Given that DABG is a pharmacologically active metabolite, genetic variations affecting its concentration may potentially influence the overall anticoagulant efficacy.
This study primarily aimed to investigate the effect of UGT2B15 on the pharmacokinetics of DAB in humans, while also considering the roles of ABCB1 and CES1 SNPs to provide a more comprehensive understanding of genetic variability’s impact on DABG formation.
This study enrolled 124 male subjects with a mean (±S.D.) age of 25.9 ± 3.7 years (range: 19–38 years), mean weight of 73.1 ± 8.6 kg (range: 54.4–91 kg), and mean height of 175 ± 5.2 cm (range: 160–191 cm). All participants were confirmed to be healthy by a physician through a detailed physical examination, 12-lead electrocardiography, serum biochemistry, hematology, and urinalysis. Exclusion criteria included history or evidence of a hepatic, renal, gastrointestinal, or hematologic abnormality, any other acute or chronic disease, or an allergy to any drug. All subjects were non-smokers, not taking any medication, and provided written informed consent. The study protocol was approved by the Institutional Review Board (IRB) of Anam Hospital, Korea University, Korea (IRB No. 2023AN0054).
To determine the ABCB1, CES1, and UGT2B15 genotypes, blood samples were collected from each participant and stored at −20°C until DNA extraction. Genomic DNA was isolated from the peripheral leukocytes. All individuals were genotyped for the c.1236C>T (rs1128503), c.2677C>T(A) (rs2032582), c.3435C>T (rs1045642), and c.2482-2236G>A (rs4148738) alleles of ABCB1 polymorphisms, c.1168–33A>C (rs2244613) and c.257 + 885T>C (rs8192935) alleles of CES1 polymorphisms, and c.253G>T (rs1902023) alleles of UGT2B15 polymorphism through pyrosequencing methods using a PyroMark (Biotage, Uppsala, Sweden), as described previously (Kim et al., 2013a; Kim et al., 2013b; Kim et al., 2014; Park et al., 2022).
Following an overnight fast, subjects were administered a single oral dose of 150 mg DABE (Pradaxa; Boehringher Ingelheim, Germany) with 240 mL of water. Blood samples were collected in EDTA tubes (Vacutainer; Becton Dickinson, Franklin Lakes, NJ, United States) immediately before drug administration (baseline) and at 0.5, 1, 1.5, 2, 2.5, 3, 4, 6, 8, 10, 12, 24, and 48 h post-administration. Plasma was separated by centrifugation (1977 g, 4°C, 15 min) and the samples were stored at −70°C awaiting analysis.
Plasma concentrations of DAB were determined using a slightly modified version of a previously validated LC-MS/MS method. The concentration difference between total and free DAB, determined through a deconjugation process, was used to estimate DAB glucuronide levels. This approach followed the method described in the previously literature (Blech et al., 2008). A total of 100 μL of plasma sample was added to a glass tube containing 10 μL of the internal standard, dabigatran-d4 (350 ng/mL). For total DAB determination, 20 μL of potassium hydroxide was added (this step was omitted for free DAB). The mixture was shaken for 15 s, followed by the addition of 400 μL of acetonitrile. The mixture was then vortexed for 1 min, and the organic phase was transferred to a clean glass tube and evaporated to dryness under nitrogen gas flow. The residue was reconstituted with 300 μL of 30% methanol with 1% formic acid. A 3-μL aliquot of this solution was injected onto the LC-MS/MS system which was equipped with a Unison Phenyl column (3 μm, 100 mm × 2.0 mm; Imtakt Corp., Kyoto, Japan). The mobile phase consisted of 10 mM ammonium formate (0.2% formic acid) and methanol in a 60:40 volume ratio at a constant flow rate of 0.2 mL/min. Quantification was performed using multiple reaction monitoring mode, with transitions of m/z 472.2→289.1 for DAB and 476.2→293.1 for the internal standard. A linear calibration curve for DAB, ranging from 0.5 to 350 ng/mL, was established, with regression correlation coefficients exceeding 0.9999. Both intra- and inter-day coefficients of variation were maintained below 5%. The concentration difference between total and free DAB was attributed to the DABG concentration.
The pharmacokinetic parameters for total and free DAB, as well as DABG, were determined using non-compartmental analysis with WinNonlin software (version 8.5.1; Pharsight Corp., Mountain View, CA, United States). Maximum plasma concentration (Cmax) and the time to reach Cmax (Tmax) were estimated directly from the raw data. The total area under the plasma concentration-time curve (AUCall) was calculated using the linear trapezoidal rule. Oral clearance (CL/F) of DAB was estimated using the dose/AUC method. The metabolite-to-parent ratio (m/p ratio) was calculated by dividing the Cmax and AUCall of DABG by those of free DAB. The Cmax ratio was calculated by dividing the Cmax of the metabolite by that of the parent, and the AUC ratio was calculated by dividing the AUCall of the metabolite by that of the parent.
The data were expressed as the mean ± standard deviation (SD) in the text and tables. Statistical comparisons between genotype groups (ABCB1, CES1, and UGT2B15) were performed using one-way analysis of variance (ANOVA) or, where appropriate, Kruskal–Wallis one-way ANOVA by rank test. Pharmacokinetic parameters were compared according to each genotype. These tests were chosen based on the normality of the data distribution, which was assessed prior to ANOVA. Multiple post hoc comparisons were performed using the normality test to identify significant differences between specific groups. Geometric mean ratios (GMRs) for Cmax and AUCall were calculated to compare pharmacokinetic parameters among genotypes. GMRs and their 90% confidence intervals were derived using logarithmic transformations, and statistical significance was assessed via t-tests. Heterozygote mutant and homozygote mutant genotypes were analyzed individually, and they were also grouped together to compare against the wild-type genotype, as genetic variations in these genotypes can affect the function of transporters or enzymes involved in drug metabolism. Demographic variables including age, body weight, and height, were used as covariates. However, the interactions between genotype and these covariate were not statistically significant. Data analysis was performed using SAS 9.2 for Windows. Statistical significance was set at p < 0.05.
This study analyzed the genotype distributions and allelic frequencies of ABCB1, CES1, and UGT2B15 polymorphisms in 124 Korean subjects, along with demographic data such as age, height, and weight (Table 1). The mean age of participants was 25.0 ± 3.7 years, with a mean body weight of 73.1 ± 8.6 kg and height of 175.0 ± 5.2 cm. No significant demographic differences were observed between the genotype groups, except for ABCB1 c.2677G>T(A) polymorphism, which exhibited five distinct genotype groups and significantly deviation from Hardy-Weinberg equilibrium (p = 0.0258), probably due to greater genetic variability among the observed genotypes.
Table 1. Genotype frequencies of ABCB1, CES1, and UGT2B15 genetic polymorphisms in 124 Korean subjects and associated demographic data (Chi-square and P-values calculated based on Hardy-Weinberg Equilibrium [HWE]).
The pharmacokinetics of free DAB, including Tmax, Cmax, AUCall, half-life, and CL/F, were evaluated in relation to the genotypes of ABCB1, CES1, and UGT2B15 polymorphisms (Table 2). The UGT2B15 c.253G>T polymorphism was associated with significant differences in the Tmax (p = 0.0479) and CL/F (p = 0.0171). Subjects with the GG genotype exhibited slightly longer Tmax (2.5 ± 0.8 h) compared with the GT (2.2 ± 0.7 h) and TT (2.4 ± 0.6 h) genotypes, but with borderline significance (p = 0.047). Wild type versus heterozygote/homozygote mutant anaylsis was also not statistically significant. Although CL/F appeared lower in the TT genotype group (177.2 ± 81.5 L/h) compared to the GT (247.9 ± 155.5 L/h) and GG (185.8 ± 90.9 L/h) groups, there was no consistent allelic dose-effect relationship observed to suggest a clear genotype-related trend. No significant differences in DAB pharmacokinetics were observed in the ABCB1 and CES1 polymorphisms. Additionally, no significant differences in GMR values for DAB were observed among the UGT2B15 genotypes (Table 3).
Table 2. Comparisons of pharmacokinetic variables of free DAB, DABG, and metabolite-to-parent ratios (m/p ratios) by UGT2B15 genetic polymorphisms.
Table 3. Geometric Mean Ratio (GMR) Comparisons of Cmax and AUCall for DAB, DABG, and metabolite-to-parent Ratios (m/p ratios) by UGT2B15 genetic polymorphisms (GT and TT were compared to GG, and TT was compared to GT).
The UGT2B15 c.253G>T polymorphism significantly affected the pharmacokinetics of DABG (Table 2, 3; Figures 1–3). Subjects with the GG genotype exhibited higher Cmax and AUCall values than those with GT or TT genotypes (p = 0.0188 and p = 0.0207, respectively). GMR analyses revealed significantly lower Cmax and AUCall values in the TT genotype compared to the GG genotype (Cmax GMR: 0.70, p = 0.0059; AUCall GMR: 0.65, p = 0.004). Similarly, GT vs. TT comparisons showed reductions in both Cmax (GMR: 0.72, p = 0.0264) and AUCall (GMR: 0.67, p = 0.0111). These findings indicate that the UGT2B15 c.253G>T polymorphism plays a critical role in DABG metabolism, potentially affecting drug exposure. No significant differences were observed for the ABCB1 and CES1 polymorphisms.
Figure 1. Concentration-time profiles of DAB and DABG for the UGT2B15 genotype (rs1902023): CC (wild-type), CA (heterozygote mutant), and AA (homozygote mutant). (A) Free DAB plasma concentrations (ng/mL); (B) log10-transformed free DAB plasma concentrations (log10 [ng/mL]); (C) DABG plasma concentrations (ng/mL); (D) log10-transformed DABG plasma concentrations (log10 [ng/mL]).
Figure 2. Concentration-time profiles of free DAB (A, B) and DABG (C, D) stratified by UGT2B15 genotype (rs1902023) groups: GG (wild-type), GT (heterozygote mutant), and TT (homozygote mutant). Data are presented as mean ± SD for plasma concentrations (A, C) and log10-transformed plasma concentrations (B, D) for each genotype group.
Figure 3. Comparisons of (A) Cmax of DABG, (B) Cmax of free DAB, (C) m/p ratio for Cmax, (D) AUCall of DABG, (E) AUCall of free DAB, and (F) m/p ratio for AUCall according to UGT2B15 genotype (rs1902023). Data are stratified by genotype groups: GG (wild-type), GT (heterozygote mutant), and TT (homozygote mutant). * indicates p < 0.05.
The UGT2B15 c.253G>T polymorphism was also associated with significant differences in the m/p ratio of DABG (Table 2, 3; Figure 3). Specifically, significant differences were observed in the Cmax (p = 0.0217) and AUCall (p = 0.0003) values between the GG, GT, and TT genotypes. GMR analyses revealed significantly lower m/p ratio values for both Cmax and AUCall in the TT genotype compared to the GG genotype (Cmax GMR: 0.57, p < 0.0001, AUCall GMR: 0.51, p < 0.0001). Similarly, GT vs. TT comparisons demonstrated reductions in Cmax (GMR: 0.57, p < 0.0001) and AUCall (GMR: 0.51, p < 0.0001). These findings suggest a genotype-dependent effect on the conversion of DAB to its acylglucuronide form, highlighting the potential influence of UGT2B15 polymorphism on DAB metabolism.
This study demonstrated that a UGT2B15 polymorphism, specifically c.253G>T, significantly influences the pharmacokinetics of DABG and the m/p ratio, supporting the hypothesis that genetic variability in UGT2B15 plays a key role in DAB metabolism. DAB undergoes glucuronidation primarily by UGT2B15, which converts the active form of the drug into its acylglucuronide metabolite (Ebner et al., 2010).
Our data showed that individuals with the GG genotype had significantly higher DABG concentrations than those with the GT or TT genotypes, suggesting that this polymorphism influenced both the rate (Cmax) and extent (AUCall) of glucuronidation. Specifically, the Cmax of DABG was 42.3 ± 16.3 ng/mL in the GG genotypes, compared with 32.4 ± 20.5 ng/mL in the GT and 29.7 ± 17.1 ng/mL in the TT genotypes (p < 0.05) (Table 2; Figures 1, 2). The AUCall of DABG followed a similar trend, with values of 327 ± 148.3 ng h·mL-1 in the GG genotypes, 238.7 ± 166.5 ng h·mL-1 in the GT genotypes, and 223.3 ± 165.4 ng h·mL-1 in the TT genotypes, highlighting the significant impact of the UGT2B15 c.253G>T polymorphism on glucuronidation efficiency. Furthermore, the observed differences in the m/p ratios provide additional evidence to support this hypothesis. The m/p ratios for both Cmax and AUCall were significantly higher in the GG and GT genotypes than in the TT genotype (Table 2; Figure 3), indicating more efficient conversion of DAB to its acylglucuronide form in the GG and GT genotypes. Conversely, the reduced m/p ratio among individuals with the TT genotype suggests that this polymorphism impairs the conversion of DAB to its acylglucuronide form, further confirming the role of UGT2B15 in DAB metabolism. A lower m/p ratio in TT genotypes (indicative of less extensive metabolism) aligns with the hypothesis that the UGT2B15 polymorphism diminishes enzyme function, resulting in reduced glucuronidation capacity. Indeed, the role of UGT2B15 in sipoglitazar glucuronidation activity was experimentally demonstrated previously (Nishhara, 2013). The UGT2B15 variant exhitibed 2-fold reduction in intrinsic clearance for sipoglitazar when compared to the wild-type. Taken together, our results suggest that UGT2B15 is responsible for DAB glucuronidation, and the UGT2B15 polymorphism in humans likely decreases DABG formation due to the loss of function associated with this mutation.
In contrast, ABCB1 and CES1 polymorphisms did not significantly affect DAB metabolism in this study Supplementary Tables S1-S3. No statistically significant differences in the pharmacokinetic parameters were observed between the ABCB1 and CES1 genotypes, suggesting that these genetic variations do not play a major role in DAB metabolism.
This study had several limitations. First, it was conducted on healthy adult males, which may limit the generalizability of the findings to broader patient populations, including females, older individuals, and those with comorbid conditions. However, limiting the study to specific demographic variables allowed for the control of potential confounders (Park et al., 2021). Second, this study only examined the effects of a single-dose administration of DAB, leaving the impact of UGT2B15 polymorphisms on long-term treatment and real-world clinical settings remain to be determined. Third, although we identified the effects of genetic polymorphisms on DAB metabolism, we did not assess the clinical outcomes associated with these genetic variations, such as bleeding risk or therapeutic efficacy. Future studies should investigate these clinical endpoints to provide a more comprehensive understanding of the effects of UGT2B15 polymorphisms.
In conclusion, this study provides preliminary evidence that the UGT2B15 c.253G>T polymorphism may influence the pharmacokinetics of DABG in humans, particularly in glucuronidation and the m/p ratio, suggesting a potential role for genetic variability in individual responses to DAB therapy. However, further studies are necessary to assess their potential impact on clinical outcomes and to evaluate the generalizability of these findings to the broader population.
The original contributions presented in the study are publicly available. This data can be found here: https://doi.org/10.6084/m9.figshare.28040075.v1.
The studies involving humans were approved by Institutional Review Board (IRB) of Anam Hospital, Korea University. The studies were conducted in accordance with the local legislation and institutional requirements. The participants provided their written informed consent to participate in this study.
J-WP: Conceptualization, Formal Analysis, Investigation, Methodology, Software, Writing–original draft, Writing–review and editing. J-MK: Data curation, Formal Analysis, Investigation, Methodology, Software, Validation, Writing–review and editing. YYB: Data curation, Formal Analysis, Validation, Writing–review and editing. K-AK: Formal Analysis, Project administration, Resources, Validation, Visualization, Writing–review and editing. SY: Funding acquisition, Resources, Supervision, Writing–original draft, Writing–review and editing. J-YP: Conceptualization, Data curation, Formal Analysis, Investigation, Methodology, Project administration, Resources, Software, Supervision, Validation, Visualization, Writing–original draft, Writing–review and editing.
The author(s) declare that financial support was received for the research, authorship, and/or publication of this article. This work was supported by Korea University grant.
The authors declare that the research was conducted in the absence of any commercial or financial relationships that could be construed as a potential conflict of interest.
The author(s) declare that no Generative AI was used in the creation of this manuscript.
All claims expressed in this article are solely those of the authors and do not necessarily represent those of their affiliated organizations, or those of the publisher, the editors and the reviewers. Any product that may be evaluated in this article, or claim that may be made by its manufacturer, is not guaranteed or endorsed by the publisher.
The Supplementary Material for this article can be found online at: https://www.frontiersin.org/articles/10.3389/fphar.2024.1507915/full#supplementary-material
Antonijevic, N. M., Zivkovic, I. D., Jovanovic, L. M., Matic, D. M., Kocica, M. J., Mrdovic, I. B., et al. (2017). Dabigatran - metabolism, pharmacologic properties and drug interactions. Curr. Drug Metab. 18, 622–635. doi:10.2174/1389200218666170427113504
Blair, H. A., and Keating, G. M. (2017). Dabigatran etexilate: a review in nonvalvular atrial fibrillation. Drugs 77, 331–344. doi:10.1007/s40265-017-0699-z
Blech, S., Ebner, T., Ludwig-Schwellinger, E., Stangier, J., and Roth, W. (2008). The metabolism and disposition of the oral direct thrombin inhibitor, dabigatran, in humans. Drug Metab. Dispos. 36, 386–399. doi:10.1124/dmd.107.019083
Connolly, S. J., Ezekowitz, M. D., Yusuf, S., Eikelboom, J., Oldgren, J., Parekh, A., et al. (2009). Dabigatran versus warfarin in patients with atrial fibrillation. N. Engl. J. Med. 361, 1139–1151. doi:10.1056/NEJMoa0905561
Dimatteo, C., D’Andrea, G., Vecchione, G., Paoletti, O., Cappucci, F., Tiscia, G. L., et al. (2016). Pharmacogenetics of dabigatran etexilate interindividual variability. Thromb. Res. 144, 1–5. doi:10.1016/j.thromres.2016.05.025
Ebner, T., Wagner, K., and Wienen, W. (2010). Dabigatran acylglucuronide, the major human metabolite of dabigatran: in vitro formation, stability, and pharmacological activity. Drug Metab. Dispos. 38, 1567–1575. doi:10.1124/dmd.110.033696
Ferri, N., Colombo, E., Tenconi, M., Baldessin, L., and Corsini, A. (2022). Drug-drug interactions of direct oral anticoagulants (DOACs): from pharmacological to clinical practice. Pharmaceutics 14, 1120. doi:10.3390/pharmaceutics14061120
Feuring, M., and van Ryn, J. (2016). The discovery of dabigatran etexilate for the treatment of venous thrombosis. Expert Opin. Drug Discov. 11, 717–731. doi:10.1080/17460441.2016.1188077
Gong, I. Y., and Kim, R. B. (2013). Importance of pharmacokinetic profile and variability as determinants of dose and response to dabigatran, Rivaroxaban, and apixaban. Can. J. Cardiol. 29 (Suppl. ment), S24–S33. doi:10.1016/j.cjca.2013.04.002
Härtig, F., Poli, S., Ebner, M., Birschmann, I., Kuhn, J., Ziemann, U., et al. (2020). Monitoring of low dabigatran concentrations: diagnostic performance at clinically relevant decision thresholds. J. Thromb. Thrombolysis. 49, 457–467. doi:10.1007/s11239-019-01981-z
Ji, Q., Zhang, C., Xu, Q., Wang, Z., Li, X., and Lv, Q. (2021). The impact of ABCB1 and CES1 polymorphisms on dabigatran pharmacokinetics and pharmacodynamics in patients with atrial fibrillation. Br. J. Clin. Pharmacol. 87, 2247–2255. doi:10.1111/bcp.14646
Kim, K. A., Joo, H. J., Lee, H. M., and Park, J. Y. (2013a). SLCO2B1 genetic polymorphisms in a Korean population: pyrosequencing analyses and comprehensive comparison with other populations. Mol. Biol. Rep. 40, 4211–4217. doi:10.1007/s11033-013-2502-x
Kim, K. A., Song, W. G., Lee, H. M., Joo, H. J., and Park, J. Y. (2013b). Effect of P2Y1 and P2Y12 genetic polymorphisms on the ADP-induced platelet aggregation in a Korean population. Thromb. Res. 132, 221–226. doi:10.1016/j.thromres.2013.06.020
Kim, K. A., Song, W. G., Lee, H. M., Joo, H. J., and Park, J. Y. (2014). Multiplex Pyrosequencing method to determine CYP2C9*3, VKORC1*2, and CYP4F2*3 polymorphisms simultaneously: its application to a Korean population and comparisons with other ethnic groups. Mol. Biol. Rep. 41, 7305–7312. doi:10.1007/s11033-014-3617-4
Laizure, S. C., Chen, F., Farrar, J. E., Ali, D., Yang, B., and Parker, R. B. (2022). Carboxylesterase-2 plays a critical role in dabigatran etexilate active metabolite formation. Drug Metab. Pharmacokinet. 47, 100479. doi:10.1016/j.dmpk.2022.100479
López-López, J. A., Sterne, J. A. C., Thom, H. H. Z., Higgins, J. P. T., Hingorani, A. D., Okoli, G. N., et al. (2017). Oral anticoagulants for prevention of stroke in atrial fibrillation: systematic review, network meta-analysis, and cost effectiveness analysis. BMJ 359, j5058. doi:10.1136/bmj.j5058
Moj, D., Maas, H., Schaeftlein, A., Hanke, N., Gómez-Mantilla, J. D., and Lehr, T. (2019). A comprehensive whole-body physiologically based pharmacokinetic model of dabigatran etexilate, dabigatran and dabigatran glucuronide in healthy adults and renally impaired patients. Clin. Pharmacokinet. 58, 1577–1593. doi:10.1007/s40262-019-00776-y
Nishhara, M. (2013). UDP-glucuronosyltransferase 2B15 (UGT2B15) is the major enzyme responsible for sipoglitazar glucuronidation in humans: retrospective identification of the UGT isoform by in vitro analysis and the effect of UGT2B15*2 mutation. Drug Metab. Pharmacokinet. doi:10.2133/dmpk.dmpk-13-rg-004
Park, J. W., Chung, H., Kim, K. A., Kim, J. M., Park, I. H., Lee, S., et al. (2021). ABCG2 single nucleotide polymorphism affects imatinib pharmacokinetics in lower alpha-1-acid glycoprotein levels in humans. Front. Pharmacol. 12, 658039. doi:10.3389/fphar.2021.658039
Park, J. W., Park, I. H., Kim, J. M., Noh, J. H., Kim, K. A., and Park, J. Y. (2022). Rapid detection of FMO3 single nucleotide polymorphisms using a Pyrosequencing method. Mol. Med. Rep. 25, 48. doi:10.3892/mmr.2021.12564
Keywords: dabigatran, UGT2B15, genetic polymorphism, pharmacokinetics, dabigatran acylglucuronide
Citation: Park J-W, Kim J-M, Bang YY, Kim K-A, Yu S and Park J-Y (2025) UGT2B15 single nucleotide polymorphism reduces dabigatran acylglucuronide formation in humans. Front. Pharmacol. 15:1507915. doi: 10.3389/fphar.2024.1507915
Received: 08 October 2024; Accepted: 02 December 2024;
Published: 09 January 2025.
Edited by:
Hong Shen, Bristol Myers Squibb, United StatesReviewed by:
Stephen Fowler, Roche Innovation Center Basel, SwitzerlandCopyright © 2025 Park, Kim, Bang, Kim, Yu and Park. This is an open-access article distributed under the terms of the Creative Commons Attribution License (CC BY). The use, distribution or reproduction in other forums is permitted, provided the original author(s) and the copyright owner(s) are credited and that the original publication in this journal is cited, in accordance with accepted academic practice. No use, distribution or reproduction is permitted which does not comply with these terms.
*Correspondence: Ji-Young Park, anlwYXJrMjFAa29yZWEuYWMua3I=; Sungwook Yu, c29uZzR5dUBrb3JlYS5hYy5rcg==
†These authors have contributed equally to this work
Disclaimer: All claims expressed in this article are solely those of the authors and do not necessarily represent those of their affiliated organizations, or those of the publisher, the editors and the reviewers. Any product that may be evaluated in this article or claim that may be made by its manufacturer is not guaranteed or endorsed by the publisher.
Research integrity at Frontiers
Learn more about the work of our research integrity team to safeguard the quality of each article we publish.