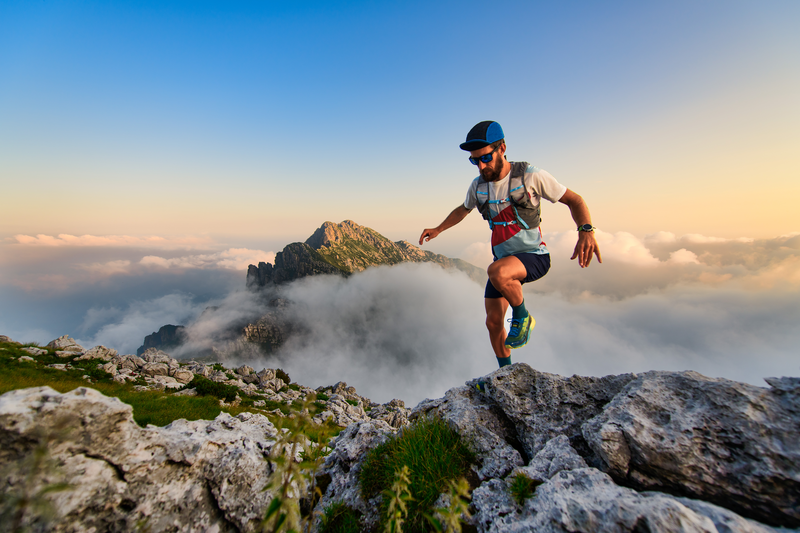
95% of researchers rate our articles as excellent or good
Learn more about the work of our research integrity team to safeguard the quality of each article we publish.
Find out more
SYSTEMATIC REVIEW article
Front. Pharmacol. , 11 December 2024
Sec. Experimental Pharmacology and Drug Discovery
Volume 15 - 2024 | https://doi.org/10.3389/fphar.2024.1473599
This article is part of the Research Topic Nano Pharmaceutical Materials Design and Therapeutic Application View all 7 articles
Objective: The objective of this study was to analyze the impact of nanotechnology on the treatment and recovery of spinal cord injury (SCI), a condition that has profound global effects on physical and psychological health.
Methods: We utilized the Web of Science Core Collection to obtain bibliometric data. With the tools such as VOSviewer and CiteSpace, we conducted a comprehensive review of 422 relevant publications to identify research trends and influential works in the field of nanotechnology applied to SCI.
Results: The analysis revealed significant contributions from both China, Sweden and the United States, and pinpointed inflammation, apoptosis, and nano-drug delivery as the primary areas of focus in current research, with emerging trends evident in recent literature.
Conclusion: Nanotechnology hold great potential to revolutionize the treatment of SCI through targeted therapeutics and modulation of pathological processes. This study provided valuable insights into the evolving landscape of SCI research, underscoring the importance of continuous innovation and interdisciplinary collaboration.
SCI denotes the structural and functional impairment of the spinal cord resulting from an external force (McDonald and Sadowsky, 2002). The pathophysiological cascade of SCI encompasses primary and secondary injuries (Eli et al., 2021). The primary injury corresponds to the immediate trauma at the moment of impact, which may include compression, laceration, or severance. Subsequently, secondary injury ensues as a consequence of a complex interplay of biochemical, immunological, and cellular reactions following the primary insult (Fan et al., 2018). These reactions involve inflammation, free radical generation, cellular apoptosis, and glial scar formation, which collectively exacerbate the initial damage. Worldwide, the annual incidence of SCI ranges from 40 to 80 cases per million individuals, predominantly affecting young and middle-aged adults, with a higher prevalence in males than in females. The leading causes of SCI include traffic accidents, falls, sports-related injuries, and acts of violence. Incidence rates vary across different regions and are influenced by factors such as the level of economic development, traffic accident frequency, and lifestyle choices (Kumar et al., 2018; Ding et al., 2022; Liu Y. et al., 2023). The current management of SCI is categorized into acute and rehabilitation phases, tailored to the stage of injury and recovery (Karsy and Hawryluk, 2019). Acute management strategies involve pharmacological interventions, like the administration of corticosteroids (e.g., methylprednisolone) to mitigate inflammation and cellular damage, along with surgical interventions and supportive care, including respiratory support, nutritional therapy, and complication prevention. Rehabilitation protocols encompass physical therapy modalities, such as exercise and balance training, as well as functional electrical stimulation to enhance or restore motor capabilities. Occupational therapy is employed to foster maximum independence in activities of daily living, while psychological therapy provides counseling and support to aid patients in adapting to their altered circumstances (Harvey, 2016). Beyond these established clinical treatments, experimental approaches are being investigated in laboratory settings, including stem cell therapies and biomaterial-based interventions. The application of stem cells in the treatment of spinal cord injury is also of great significance (Venkatesh et al., 2019; Katari et al., 2024). Despite progress in SCI understanding and management, the search for innovative treatments is essential. Nanotechnology is a science that focuses on manipulating and engineering matter at the nanoscale. The definition of nanoparticles usually refers to particles within the nanoscale range (typically between 1 and 1,000 nm). However, in actual applications, a wider range of particles is sometimes included, especially when they exhibit nano-effects. Under a broader definition, nanoparticles can cover a range from 1 nm to several hundred nanometers or even thousands of nanometers. Particles within this range are still considered to possess special properties at the nanoscale. Generally, the smaller the size of the nanoparticle, the more pronounced its surface and quantum effects, particularly when the size is below 100 nm, making its properties even more prominent. Its innovative applications in this realm have brought tremendous potential to the fields of medicine and biotechnology. The classification of nanotechnology can vary in many ways. The simplest method is based on the basic properties of nanoparticles, which can be divided into organic and inorganic nanoparticles. Both types of nanoparticles are widely used in the field of spinal cord injury. Each type has its own characteristics; for instance, inorganic nanoparticles, such as cerium oxide nanoparticles, have significant effects in combating oxidative stress (Behroozi et al., 2022). Organic nanoparticles, such as liposomes and polymer nanoparticles, are more commonly used in drug delivery systems, especially in applications related to targeted drug delivery and cellular regeneration (Wang X. et al., 2021). Based on different applications of nanotechnology, it can also be categorized into drug delivery applications, selective targeting, and imaging/diagnostics. Nanotechnology has been used to develop more effective drug delivery systems. For example, nanoparticles can be used to deliver drugs directly to the site of a disease, reducing damage to healthy tissues and improving therapeutic efficacy. Nanotechnology has also enabled selective targeting applications by designing specific types of nanoparticles that can recognize and bind to particular cell receptors or disease markers. This enhances the precision of drug delivery, allowing treatment to focus on diseased cells while minimizing the impact on healthy cells (Sabourian et al., 2023; Khan et al., 2020). Additionally, applications of nanotechnology in biotechnology include the use of nanosensors for early detection and diagnosis of diseases, and the enhancement of biomedical imaging techniques with nanomaterials, allowing doctors to observe and understand the inside of the human body more accurately. These applications not only improve the precision and efficiency of treatments but also open up new possibilities for personalized medicine and precision therapy, with the potential to greatly improve human healthcare in the future. Nanotechnology offers promising avenues for SCI treatment, highlighted by the field of nanotechnology (Zimmermann et al., 2021; Gao et al., 2021; Bayda et al., 2019; Imran et al., 2023; Alzate-Correa et al., 2022; Santamaría et al., 2023; Saeedi et al., 2019). This discipline explores nanoscale materials, showing significant potential across various sectors, particularly in SCI, where it promises to transform diagnosis, treatment, and rehabilitation strategies.
This bibliometric analysis examines the current trends in nanotechnology for SCI, analyzing research volume, geographic distribution, key researchers, institutions, and citation patterns (Shang et al., 2023). It aims to provide a detailed view of this field’s growth, spotlighting influential studies and emerging directions for future research. This work underscores nanotechnology’s critical role in advancing SCI treatment, and recovery, fostering further innovation and offering hope to those affected by SCI.
In this article, we selected Web of Science Core Collection (WoSCC) as the source of bibliometric data in the field of nanotechnology for SCI research.
The specific retrieval method has been mentioned in previously published articles (Shang et al., 2023; Zhang et al., 2023; Wang et al., 2024). To put it simply, the data was downloaded and retrieved by two authors on the same day. The search was conducted in the WoSCC database using the following search formula: TS = nano* and [(TS = spinal cord injury) or (TS = spinal cord injuries)]. We only included articles in English and limited the article types to reviews and monographs. A total of 1,367 articles were initially included. Inclusion criteria: Only articles or reviews that focus on nanotechnology in spinal cord injury research will be considered. Papers that only briefly mentioned nanotechnology and spinal cord injury in their abstracts were excluded. Two individuals reviewed the titles, abstracts, and full texts to exclude papers that were not relevant to the topic, resulting in a final inclusion of 422 papers for this study.
In this article, we utilized five softwares of VOSviewer, CiteSpace, Bibliometrix, SCImago, and GraphPad Prism to conduct bibliometric visual analysis. Specifically, we utilize VOSviewer, CiteSpace, and Bibliometrix to process and analyze the data downloaded from WoSCC. Use VOSviewer to analyze and extract information and build visualization maps of countries, institutions, authors, keywords. Use CiteSpace to construct a keyword busrt visualization graph. Use Bibliometrix analysis to extract information on the number of publications and citations. Use SCImago to build a visualization map of collaborations between countries, institutions, and authors. Use GraphPad Prism software to build visualization graphs of the number of publications and citations. The specific procedures were described in previous articles (Zhang et al., 2023; Wang et al., 2024).
Based on our search and literature inclusion process (Figure 1), a total of 422 publications were included in our study, of which 381 were articles and 41 were reviews. In the timespan of this research field from 2002 to 2023, a total of 2,293 authors from 155 countries and regions belonging to 1,582 organizations participated in research in this field. Related research was published in 206 journals, and the rate of international cooperation was 28.67%, with each article being cited an average of 26.78 times. As shown in Figure 2, since 2002, this field has gradually attracted the attention of researchers. After 2018, the number of published articles has increased rapidly, with an average annual growth rate of 19.75%. 2022 will be the year with the largest number of published articles, reaching 61 articles. The 422 articles in this field received a total of 10,788 citations, and the average citations per article were 26.93. Conducting an in-depth analysis of the top 100 cited documents, we found that these documents accounted for 74.8% of the total citations.
China and the United States are the countries with the largest number of publications in this field. Figures 3A,B illustrate the global publication situation and cooperation between countries. China published 195 articles, followed by the United States with 125 articles. In particular, in 2019, the volume of articles published by China surged rapidly, closely followed by the United States (Figure 3C). Figure 3D demonstrates the proportion of the number of articles published by the top 10 countries. It is worth noting that although the United States does not have the highest number of publications, articles from the United States receive the highest number of citations. The Average Citation Per Publication represents the level of attention a published article receives in the field. In the Average Citation Per Publication category, Israel ranks first, the United States ranks 12th, and China ranks 28th (Table 1; Supplementary Table S1). This indicates that even though China has the highest number of publications, it does not receive as much attention as Israel and the United States.
Figure 3. The visualization of countries on research of nanotechnology in SCI. (A, B) Top 5 countries by number of publications over time (C) Proportion of articles published by the top 10 countries (D).
A total of 1,582 organizations participated in this research area. Figure 4A shows the 10 organizations that have made the most contributions in this field, namely Jinzhou Medical University, Zhejiang University, University of Tehran Medical Science, Purdue University, University of Hong Kong, Uppsala University, Soochow University, Jinan University, Sichuan University, and Sun Yat Sen University. Among these ten organizations, seven are from China, one is from the United States, one is from Iran, and one is from Sweden. Figure 4B displays the collaboration between organizations that publish a larger number of articles. Supplementary Figure S1 illustrates the overlay situation of articles published by organizations. In the research field, early articles were primarily published by Purdue University, Northwestern University, MIT, University of Hong Kong, and other institutions, while recent articles were mainly published by the University of Chinese Academy of Science and other affiliated schools.
Figure 4. The top 10 institutions with the most publications and their cooperative relationships (A, B).
A total of 206 research papers on nanotechnology in SCI were published in 18 journals that each published more than 5 papers. Figure 2 in the supplementary material shows the density visualization of journal in this field. Table 2 presents the top 10 journals with the highest number of articles. It is evident that among these top 10 journals, biomaterials have the highest number of articles, reaching up to 12. The quality of journals in this field was relatively high, with 6 journals belonging to Journal Citation Reports (JCR) Q1 and 4 journals having an impact factor of over 10.
A total of 2,293 authors participated in the publication of papers in this field. Table 3 presents the top 10 authors with the highest number of published papers, namely Sharma HS, Sharma A, Muresanu DF, Lafuente JV, Mei XF, Tian ZR, Patnaik R, Forloni G, Papa S, and Rossi F. Supplementary Figure S3 shows the annual publication status of these top ten authors. It was noteworthy that the top four authors with the most published articles were all affiliated with Uppsala University in Switzerland, followed by Italy. There were two authors from the United States and China. Figure 5A and B shows the cooperation among the authors, revealing close cooperative relationships between teams from Italy, Sweden, and the United States.
The Figure 6 displays the subject category bursts. The blue line represents the time interval, while the red line segment indicates the span of citation bursts, including the start and end years. The Figure 6 showcases the top 15 terms with the highest burst intensity during different periods. The subject category bursts evolved from “Surgery” (2007) to “Neurosciences” (2015), “Multidisciplinary Sciences” (2016), “Pharmacology and Pharmacy” (2017), “Cell Biology” (2017); and from “Physics. Condensed Matter” (2008), further developed into “Biochemistry and Molecular Biology” (2019). This progression indicates that research on nanotechnology in spinal cord injury has shifted from fields like neurosurgery and fundamental physics to disciplines such as pharmacology, cell biology, or biochemistry. For instance, the use of nanomedicine as a therapeutic approach for spinal cord injury, where Prussian blue nanocatalysts combined with zinc ions can improve the microenvironment of spinal cord injury (Gao et al., 2024); or the loading of traditional drugs (Paclitaxel, Cerebrolysin) onto nanoparticles to enhance delivery methods (Zhang et al., 2022; Sahib et al., 2020). Additionally, research on nanomaterials as carriers for cell therapy, such as adipose-derived stem cells loaded in nanogels to improve motor function after spinal cord injury (Li J. et al., 2022).
At a more refined level, we have revealed the research trends in the field of nanotechnology for spinal cord injury throughout the entire timespan (2002–2023) by examining keyword bursts. As shown in the Figure 7, the top 25 keywords with the highest degree of burst are displayed. “Edema Formation” is the earliest keyword burst, persisting from 2007 to 2015, reaching a burst value of 4.2. This indicates that during this phase, researchers primarily focused on the role of nanotechnology in eliminating spinal tissue edema following spinal cord injury. “Blood-Spinal Cord Barrier” reached a burst value of 4.37 from 2009 to 2013, signifying that at this stage, using nanotechnology to breach the blood-spinal cord barrier and enable therapeutic agents to reach the injury site was a hot research topic. In addition to the blood-spinal cord barrier, several other hotspots were observed in the keyword bursts, which I categorize into: tissue engineering material types, target cell types, tissue engineering material properties, and biological behaviors. “Peptide Nanofiber Scaffold,” “Electrospinning,” and “Iron Oxide Nanoparticle” reached bursts in 2009–2010, 2011–2018, and 2015–2018, respectively. These three types of nanomaterials for tissue engineering were among the more extensively studied material categories at the time. “Neuron” and “Schwann Cell” were keywords that burst in 2013 and 2014, respectively, indicating that these two types of cells received significant attention from researchers around 2013–2018. Keywords related to the properties of tissue engineering materials, such as “Local Delivery,” “Gene Delivery,” “Transplantation,” and “Controlled Release,” experienced bursts during the 2015–2018 period. Additionally, cellular biological behaviors like “Axon Regeneration,” “Apoptosis,” and “Neurogenesis” have burst in recent years. This perspective suggests that in the initial stages, researchers focused on the application of nanotechnology in spinal cord injury treatment as a means to eliminate spinal edema and to penetrate the blood-spinal cord barrier. With the advancement of research techniques and shifts in thinking, the focus of researchers has gradually transitioned to studying different types of nanomaterials, targeting various cells, and utilizing the advantageous properties of multiple materials to achieve the goal of improving cellular biological behaviors.
Keywords exhibit strong intrinsic correlations, and certain keywords can form different clusters based on their affinity. Identifying these clusters can more intuitively delineate the various subfields of nanotechnology research in spinal cord injury. As shown in Figure 8A, keyword clustering can be categorized into four groups: different nanomaterials (#0 Nanofiber, #9 Cerium Oxide), cell-related therapies (#3 Cells, #5 Cell Therapy, #7 Extracellular Matrix, #8 Extracellular Vesicles), therapeutic targets (#2 Oxidative Stress, #4 Blood-Spinal Cord Barrier), and therapeutic goals (#1 Nerve Regeneration) as well as #6 Central Nervous System Injury. #0 Nanofiber and #6 Central Nervous System Injury are the main themes in this field, while #1 Nerve Regeneration is the goal of all research, and thus these three keyword clusters are present throughout almost the entire research process. #2 Oxidative Stress is a key pathophysiological process in secondary injury following spinal cord injury. Treatments targeting oxidative stress have been a hot research topic in recent years. #4 Blood-Spinal Cord Barrier is a major limitation for traditional pharmacological treatments of spinal cord injury. Large molecular drugs are unable to penetrate the blood-spinal cord barrier, but nanomedicines, after years of development, have demonstrated improved capabilities to cross this barrier (Khan et al., 2020; Xie et al., 2023). In recent years, traditional nanofibers for spinal cord injury treatment have shown certain limitations. However, #7 Extracellular Matrix, #8 Extracellular Vesicles, and the novel #9 Cerium Oxide nanoparticles have shown greater therapeutic significance for spinal cord injury (Figure 8B).
The Y-axis represents the frequency of occurrence.
Citation analysis is a method utilized to analyze the influence of a paper. The greater the number of citations a paper receives, the higher its influence within the academic world. Supplementary Table S2 presents the top 10 most cited articles. All ten articles have garnered over 100 citations, with the first and second-ranked articles amassing more than 500 citations. In this field, there were a total of 18,357 documents, out of which 17 have been cited more than 20 times (Supplementary Table S3).
Research on nanotechnology in SCI has primarily progressed through three stages. The first stage spanned from 2002 to 2009, marking the nascent phase of the field, characterized by a scattering of published articles. The second stage was from 2010 to 2018. The number of papers published in this stage increased slowly. The third stage, from 2019 to the present, has witnessed a rapid surge in publications (Figure 2). In this domain, China and the United States, two of the most technologically advanced countries in the world, have the highest number of publications globally. Notably, the volume of papers from China has grown swiftly after 2019, surpassing that of the United States. However, the most prolific and influential team in this field was from another country Sweden and affiliated with Uppsala University, including prominent members such as Sharma Hs, Sharma A, Muresanu Df, Lafuente Jv, and Patnaik R, et al. In this field, the most cited paper was “Auto-catalytic ceria nanoparticles offer neuroprotection to adult rat spinal cord neurons,” published by Das et al. (2007). This paper discussed the potent antioxidant and neuroprotective properties of auto-catalytic ceria nanoparticles, which could prevent ischemic SCI and offer neuroprotective effects, showing promising application potential in SCI treatment. The second most cited paper was “Self-assembling nanofibers inhibit glial scar formation and promote axon elongation after spinal cord injury “ by Tysseling-Mattiace et al. (2008). This research focused on self-assembling peptide amphiphile nanofibers, which could inhibit the differentiation of neural stem cells into glial cells and promote the regeneration of neural axons. In vivo, these nanofibers could reduce astrocytes and increase the proportion of oligodendrocytes. The third most cited article, “Magnetic resonance tracking of transplanted stem cells in rat brain and spinal cord (Syková and Jendelová, 2006),” described the use of iron oxide nanoparticles as markers for stem cell therapy in SCI and brain injury. The fourth-ranked paper “Nano hemostat solution: immediate hemostasis at the nanoscale (Ellis-Behnke et al., 2006),” which introduced a novel nanopeptide capable of stopping bleeding in internal organs, including the spinal cord. The fifth-ranked article, “Reknitting the injured spinal cord by self-assembling peptide nanofiber scaffold (Guo et al., 2007),” presented a self-assembled nanofiber scaffold that served as a growth platform for neural progenitor cells and Schwann cells, providing a three-dimensional environment for axon growth, with transplantation into SCI sites promoting functional recovery. The article ranked 6th was “Squalenoyl adenosine nanoparticles provide neuroprotection after stroke and spinal cord injury (Gaudin et al., 2014),” which showed that squalenoyl adenosine nanoparticles could have neuroprotective effects on rats with SCI and stroke, indicating promising application prospects. The seventh-ranked article, “Axonal alignment and enhanced neuronal differentiation of neural stem cells on graphene-nanoparticle hybrid structures (Solanki et al., 2013),” discussed the combined effect of graphene nanoparticles on the differentiation of neural stem cells. The structural characteristics of graphene can affect axonal arrangement, while nanoparticles can promote the differentiation of neural stem cells. The article ranked 8th was “Nanoparticle-mediated local delivery of Methylprednisolone after spinal cord injury (Kim et al., 2009).” Methylprednisolone pulse therapy has been the main method for the treatment of SCI, but high-dose injections may cause side effects in other organs. This paper introduced a new type of methylprednisolone nanoparticle that could be locally injected at SCI site, slowly releasing the drug for improved efficacy over systemic high-dose shock therapy and minimizing systemic side effects. The article ranked 9th was “Flexible and stretchable nanowire-coated fibers for optoelectronic probing of spinal cord circuits (Lu et al., 2017),” which covered a nanofiber capable of detecting spinal cord electrical signals during rehabilitation exercises for SCI. The article ranked 10th was Transplantation of Nanostructured Composite Scaffolds Results in the Regeneration of Chronically Injured Spinal Cords (Gelain et al., 2011). After SCI, large cavities and cysts might appear in the injured area, and nerve axons could not pass through the large cystic area. This article described a system comprising electrospun fibers and peptides. Transplanting this nano-drug-delivery system into the SCI area could result in re-covering of the spinal cord cyst area with a nerve cell nuclear matrix, significantly enhancing overall motor function scores.
Co-cited articles indicated that two articles were cited together by other articles. Commonly cited articles represented the foundational articles and knowledge background in this field. Supplementary Table S3 shows the top ten papers with the highest number of common citations. Articles ranked 2 (Kim et al., 2009) and 4 (Tysseling-Mattiace et al., 2008) functioned as highly cited articles, which were introduced in the previous section. Among these ten articles, four articles simply introduced information related to SCI. One article introduced the Basso-Beattie-Bresnahan (BBB) score, which is the most commonly used scoring method for rat SCI models. This article also has the highest number of citations (Basso et al., 1995). Three articles respectively discussed the epidemiology, pathological changes, prognosis (Ahuja et al., 2017), treatment methods (Thuret et al., 2006), and targets (Silver and Miller, 2004) of SCI. Additionally, there were two articles that introduce the application and therapeutic effect of combining methylprednisolone, a commonly used drug for SCI, with nanoparticles in SCI. These studies found that sustained release of methylprednisolone could lead to better therapeutic effects (Chvatal et al., 2008; Cerqueira et al., 2013). The remaining two articles describe the potential application of two different nanomaterials in SCI (Silva et al., 2004; Shi et al., 2010).
The inflammatory response following SCI was characterized by several key events.
Recruitment of immune cells: Following an injury, immune cells such as macrophages and neutrophils are promptly attracted to the site of damage. These immune cells play a crucial role in clearing debris and pathogens, but their activation can also contribute to inflammation (Brockie et al., 2024; Lv et al., 2024; Li C. et al., 2022). Release of pro-inflammatory cytokines tumor necrosis factor (TNF)-α (Cao et al., 2022), interleukin (IL)-1β (Müller et al., 2023), and IL-6 (Shipman et al., 2023), were released as part of the immune response. These cytokines can activate intracellular signaling pathways, including those involved in apoptosis (Ding et al., 2023). Activation of signaling pathways: The inflammatory environment can activate specific signaling pathways within cells. For example, TNF-α has been known to engage the extrinsic apoptotic pathway, leading to cell death (Zhou et al., 2021). This activation of apoptotic pathways can result in the loss of neurons and other essential cells within the spinal cord, thereby exacerbating the severity of the injury (Weng et al., 2023).
Conversely, apoptosis itself could trigger or intensify the inflammatory response through various mechanisms. Release of damage-associated molecular patterns (DAMPs): When cells undergo apoptosis, they release intracellular components and molecules known as DAMPs. DAMPs act as danger signals, alerting the immune system to the presence of cell damage or death (Lyu et al., 2023). Immune cell activation: DAMPs released during apoptosis can activate nearby immune cells, such as dendritic cells and macrophages. This activation stimulates the immune cells to release pro-inflammatory cytokines and chemokines, further promoting an inflammatory environment (Li and Lan, 2023). Amplification of inflammation: The continued presence of DAMPs and the release of pro-inflammatory factors can exacerbate the inflammatory milieu at the injury site (Das et al., 2024). This leads to the sustained recruitment of more immune cells to the site and the perpetuation of the inflammatory response, potentially causing further tissue damage.
The interplay between apoptosis and inflammation in SCI was a complex and dynamic process. Inflammatory triggers can induce apoptosis in damaged cells, contributing to tissue loss. Conversely, apoptosis can stimulate an inflammatory response by releasing danger signals, leading to the recruitment of more immune cells and the persistence of inflammation. Understanding these mechanisms is crucial for developing targeted therapeutic interventions, including nanotechnology approaches, to modulate this crosstalk and promote improved outcomes in patients with SCI.
Nanotechnology has shown promise in modulating apoptosis and inflammation in the SCI process (Luo W. et al., 2023). Nanotechnology, which harnesses the power of nanoparticles for therapeutic purposes, holds great potential in modulating the intricate interplay between apoptosis and inflammation following SCI. Jaffer et al. (2023) found that injecting antioxidant nanoparticles into the spinal cord injury site activated microglia to differentiate in an anti-inflammatory direction. This process inhibited nerve cell apoptosis in the injured area.
Nanoparticles can be meticulously designed and customized to transport a wide array of therapeutic agents, ranging from drugs (Yin et al., 2023) and growth factors (Xia et al., 2021; Zhu et al., 2016) to anti-inflammatory compounds. The CC-chemokine ligand 2 (CCL2) - CC-chemokine receptor 2 (CCR2) axis is an inflammatory therapeutic target in SCI (Geng et al., 2022). Encapsulating drugs in the cell membrane of macrophages overexpressing CCR2 can promote targeted delivery of drugs to the injured area and reduce the infiltration of inflammatory cells and neuronal apoptosis (Gu et al., 2023). One of the difficulties in treating SCI is the efficiency with which drugs cross the blood-spinal cord barrier. Improving drug penetration into the blood-spinal cord barrier is one of the current design concepts of nanotechnology. R2KC peptide (Wu et al., 2023), and choline (Bao et al., 2023) are applied to the surface of nanotechnology to enhance their ability to penetrate the blood-spinal cord barrier. Click chemistry is applied in the preparation of nanotechnology. By incorporating peptides such as isoleucyl-lysyl-valyl-alanyl-valine (IKVAV) (Zeng et al., 2023) and DA7R (Ruan et al., 2023) onto the surface of exosomes derived from M2 macrophages using click chemistry methods, this novel nano-preparation can be targeted and transported to the damaged area to reduce the inflammatory response in the affected region. This tailored approach enables the precise delivery of these agents to the injured site within the spinal cord, thereby minimizing off-target effects and significantly enhancing therapeutic efficacy.
In the context of SCI, specific nanoparticles have been engineered to serve as powerful allies. They can act as scavengers of inflammation, effectively neutralizing pro-inflammatory molecules and modulating the behavior of immune cells. In the current study, drugs such as curcumin (Xiong W. et al., 2023), metformin (Su et al., 2023; Liu X. et al., 2023), and rapamycin (Shen et al., 2023) were encapsulated in nanomaterials. This drug delivery system further promotes the polarization of macrophages towards the M2 phenotype and reduces the infiltration of inflammatory factors related to the injured area. In addition to metallic nanomaterials, exosomes have also been used to deliver anti-inflammatory drugs. Incorporating non-coding RNAs such as miR-672-5p (Zhou et al., 2022) or specific proteins (Li et al., 2020) into exosomes, or overexpressing certain proteins like LRRC75A-AS1 (Xing et al., 2024), miR-216a-5p (Xue et al., 2023) miR-9-5p (He et al., 2022), miR-137 (Shao et al., 2023), and other methods, can improve inflammatory symptoms in SCI areas. Meanwhile, Zinc Oxide and tubastatin are dedicated to promoting cell survival and stimulating tissue regeneration (Lin et al., 2021; Liao et al., 2022). These nanoparticles deliver neuroprotective factors and facilitate the repair of damaged tissues, helping to counteract both apoptosis and inflammation.
One of the remarkable attributes of nanotechnology is its adaptability. Nanoparticles can be finely tuned to respond to specific cues within the injury microenvironment. For instance, they can be designed to release therapeutic agents in response to indicators of inflammation or changes in pH levels. The nano-drug delivery system of chitosan and poly acrylic acid designed by Sabourian et al. (2023) can decompose in the acidic environment of the injured area, allowing the drug to enter the cell nucleus and exert a regulatory effect. Qian et al. (2023) synthesized curcumin on pH-responsive nanomicelles, which can be released in the damaged area to exert anti-inflammatory effects. This dynamic responsiveness helps maintain a more conducive and supportive environment for the healing process, addressing the challenges posed by both apoptosis and inflammation. In the microenvironment of SCI, high concentrations of reactive oxygen species (ROS) severely limit the therapeutic effect of drugs. Removing ROS from the microenvironment is an important therapeutic approach. Liu et al. (Liu D. et al., 2023) applied cerium oxide nanoparticles to the treatment of SCI and found that this treatment can reduce ROS in the environment and improve the survival and differentiation of transplanted neural stem cells. Ma et al. (2023) used the ROS scavenging effect of Polydopamine to improve the efficacy of neurotrophic factors in the injured area. Nanozymes have attracted significant attention due to their unique structure and catalytic effect in improving the microenvironment of SCI areas. Shen et al. (2023) used Prussian blue nanozyme to target the SCI area, effectively clearing ROS while improving inflammation and apoptosis-induced neural damage. Xiong T. et al. (2023) discovered that the nanozyme Mn3O4 has a higher substrate affinity than ordinary antioxidant enzymes and shows superior ROS scavenging effects in SCI Figure 9.
Nanotechnology emerges as a promising avenue for tackling the complex interplay between apoptosis and inflammation in SCI. By offering precise targeting, inflammation mitigation, and promotion of the microenvironment, nanotechnology stands at the forefront of innovative approaches to enhance recovery and reduce the impact of these interconnected processes.
In addition to studying the effects of different nanotechnology on SCI, the mechanism of drug action is also a research focus in this field. Inflammatory signaling pathways are crucial targets for the treatment of SCI. Macrophage polarization can be regulated by inhibiting these pathways. Sesamol-loaded nanomicelles have been shown to inhibit apoptosis and pro-inflammatory factors through the NF-κB signaling pathway (Wang N. et al., 2021). Human umbilical cord mesenchymal stem cells target TLR4/NF-κB p65 to inhibit IL-1β, IL-6, TNFα and other cytokines (Wang et al., 2023). Stem cell exosomes can promote microglial M2 polarization by activating the nuclear factor erythroid 2-related factor 2 (Nrf2)/heme oxygenase-1 (HO-1) (Luo Y. et al., 2023) and suppressor of cytokine signaling 3 (SOCS3)/Janus Kinase 2 (JAK2)/signal transducer and activator of transcription 3 (STAT3) (Yuan et al., 2023) inflammatory signaling pathways, thereby promoting neurological recovery. Angiogenesis is another important target for treating SCI. New blood vessels can provide necessary nutrients for repairing the injured area. Exosomes in cerebrospinal fluid can promote angiogenesis in the injured area through activation of the phosphoinositide 3-kinase (PI3K)/protein kinase B (AKT) signaling pathway (Li et al., 2023),while exosomes derived from M2 macrophages can promote vascular regeneration through the hypoxia-inducible factor (HIF)-1α/vascular endothelial-derived growth factor (VEGF) signaling pathway (Huang et al., 2022). TGF-betaR2 (Zhu et al., 2021) and nerve growth factor (NGF)/tropomyosin receptor kinase A (TrkA) (Zhao et al., 2021) are important targets for nanotechnology in promoting nerve regeneration.
This study had the following advantages: (1) It was the first bibliometric study on nanotechnology in SCI research, providing a systematic and comprehensive overview of the current research status in this field. It highlighted the most important articles, the most active research teams, and current research hotspots.; (2) It provided a visually appealing and easily understandable summary in the form of a picture. However, this study also had certain limitations: (1) Only English-language documents were included, potentially excluding relevant studies published in other languages. Some documents may have been unintentionally missed during the study selection process. (2) Although there is a lot of basic research in this field, clinical trials are still rare. Some clinical trials have found that the combination of stem cells and scaffolds has good effects in patients with spinal cord injuries (Xiao et al., 2018). More clinical research is needed in the future to enrich this field.
This bibliometric analysis provided a comprehensive overview of the significant progress achieved in nanotechnology research, specifically within the field of SCI. The findings demonstrated a considerable increase in global research output, particularly in the past decade. Furthermore, the analysis revealed a collaborative and geographically diverse research landscape, with noteworthy contributions from institutions in China, the United States, and Europe. The presence of certain organizations and authors indicated a solid knowledge base, while the examination of citations highlighted the influential works that have shaped current trends. Within the realm of SCI treatment, the intersection with nanotechnology was characterized by a specific focus on addressing inflammation and apoptosis. Research efforts were primarily directed towards the development of nanotechnology capable of precise targeting, inflammation mitigation, and promotion of a conducive microenvironment for healing. Despite certain limitations, such as the restriction to English-language documents, the trends and insights of this study offered a valuable framework for future research directions, emphasizing the need for continued innovation and interdisciplinary collaboration to overcome the challenges presented by SCI. Future research should pay more attention to the following: How to combine nanomaterials with new treatment methods such as gene therapy and stem cell therapy, how to further improve the targeting and therapeutic efficiency of nanomedicines, the long-term biocompatibility of nanomaterials, the control mechanism of drug release, and the obstacles to large-scale clinical applications.
The raw data supporting the conclusions of this article will be made available by the authors, without undue reservation.
XG: Conceptualization, Writing–original draft, Writing–review and editing. SZ: Conceptualization, Funding acquisition, Investigation, Writing–original draft, Writing–review and editing. WM: Writing–original draft, Writing–review and editing.
The author(s) declare that no financial support was received for the research, authorship, and/or publication of this article.
Thanks to Figdraw for providing a picture painting platform.
The authors declare that the research was conducted in the absence of any commercial or financial relationships that could be construed as a potential conflict of interest.
All claims expressed in this article are solely those of the authors and do not necessarily represent those of their affiliated organizations, or those of the publisher, the editors and the reviewers. Any product that may be evaluated in this article, or claim that may be made by its manufacturer, is not guaranteed or endorsed by the publisher.
The Supplementary Material for this article can be found online at: https://www.frontiersin.org/articles/10.3389/fphar.2024.1473599/full#supplementary-material
Ahuja, C. S., Wilson, J. R., Nori, S., Kotter, M. R. N., Druschel, C., Curt, A., et al. (2017). Traumatic spinal cord injury. Nat. Rev. Dis. Prim. 3, 17018. doi:10.1038/nrdp.2017.18
Alzate-Correa, D., Lawrence, W. R., Salazar-Puerta, A., Higuita-Castro, N., and Gallego-Perez, D. (2022). Nanotechnology-driven cell-based therapies in regenerative medicine. Aaps J. 24 (2), 43. doi:10.1208/s12248-022-00692-3
Bao, T., Li, N., Chen, H., Zhao, Z., Fan, J., Tao, Y., et al. (2023). Drug-loaded zwitterion-based nanomotors for the treatment of spinal cord injury. ACS Appl. Mater Interfaces 15 (27), 32762–32771. doi:10.1021/acsami.3c05866
Basso, D. M., Beattie, M. S., and Bresnahan, J. C. (1995). A sensitive and reliable locomotor rating scale for open field testing in rats. J. Neurotrauma 12 (1), 1–21. doi:10.1089/neu.1995.12.1
Bayda, S., Adeel, M., Tuccinardi, T., Cordani, M., and Rizzolio, F. (2019). The history of nanoscience and nanotechnology: from chemical-physical applications to nanomedicine. Molecules 25 (1), 112. doi:10.3390/molecules25010112
Behroozi, Z., Rahimi, B., Hamblin, M. R., Nasirinezhad, F., Janzadeh, A., and Ramezani, F. (2022). Injection of cerium oxide nanoparticles to treat spinal cord injury in rats. J. Neuropathol. Exp. Neurol. 81 (8), 635–642. doi:10.1093/jnen/nlac026
Brockie, S., Zhou, C., and Fehlings, M. G. (2024). Resident immune responses to spinal cord injury: role of astrocytes and microglia. Neural Regen. Res. 19 (8), 1678–1685. doi:10.4103/1673-5374.389630
Cao, H., Ji, X., Wang, Q., Guan, X., Wei, W., and Ying, L. (2022). PTBP-1 and TNF-α/NF-κB are involved in repair mechanisms of human umbilical cord mesenchymal stem cell transplantation in mice with spinal cord injury. Am. J. Transl. Res. 14 (7), 4443–4456.
Cerqueira, S. R., Oliveira, J. M., Silva, N. A., Leite-Almeida, H., Ribeiro-Samy, S., Almeida, A., et al. (2013). Microglia response and in vivo therapeutic potential of methylprednisolone-loaded dendrimer nanoparticles in spinal cord injury. Small 9 (5), 738–749. doi:10.1002/smll.201201888
Chvatal, S. A., Kim, Y. T., Bratt-Leal, A. M., Lee, H., and Bellamkonda, R. V. (2008). Spatial distribution and acute anti-inflammatory effects of Methylprednisolone after sustained local delivery to the contused spinal cord. Biomaterials 29 (12), 1967–1975. doi:10.1016/j.biomaterials.2008.01.002
Das, M., Patil, S., Bhargava, N., Kang, J. F., Riedel, L. M., Seal, S., et al. (2007). Auto-catalytic ceria nanoparticles offer neuroprotection to adult rat spinal cord neurons. Biomaterials 28 (10), 1918–1925. doi:10.1016/j.biomaterials.2006.11.036
Das, N., Mukherjee, S., Das, A., Gupta, P., Bandyopadhyay, A., and Chattopadhyay, S. (2024). Intra-tumor ROS amplification by melatonin interferes in the apoptosis-autophagy-inflammation-EMT collusion in the breast tumor microenvironment. Heliyon 10 (1), e23870. doi:10.1016/j.heliyon.2023.e23870
Ding, Q., Gao, H., Hu, X., and Gao, W. (2023). HGFIN deficiency exacerbates spinal cord injury by promoting inflammation and cell apoptosis through regulation of the PI3K/AKT signaling pathway. Adv. Clin. Exp. Med. 33, 929–940. doi:10.17219/acem/174004
Ding, W., Hu, S., Wang, P., Kang, H., Peng, R., Dong, Y., et al. (2022). Spinal cord injury: the global incidence, prevalence, and disability from the global burden of disease study 2019. Spine (Phila Pa 1976) 47 (21), 1532–1540. doi:10.1097/brs.0000000000004417
Eli, I., Lerner, D. P., and Ghogawala, Z. (2021). Acute traumatic spinal cord injury. Neurol. Clin. 39 (2), 471–488. doi:10.1016/j.ncl.2021.02.004
Ellis-Behnke, R. G., Liang, Y. X., Tay, D. K., Kau, P. W. F., Schneider, G. E., Zhang, S., et al. (2006). Nano hemostat solution: immediate hemostasis at the nanoscale. Nanomedicine 2 (4), 207–215. doi:10.1016/j.nano.2006.08.001
Fan, B., Wei, Z., Yao, X., Shi, G., Cheng, X., Zhou, X., et al. (2018). Microenvironment imbalance of spinal cord injury. Cell Transpl. 27 (6), 853–866. doi:10.1177/0963689718755778
Gao, J., Chen, S., Lin, S., and Mei, X. (2024). Prussian blue analogues improves the microenvironment after spinal cord injury by regulating Zn. Int. Immunopharmacol. 131, 111868. doi:10.1016/j.intimp.2024.111868
Gao, J., Khang, M., Liao, Z., Detloff, M., and Lee, J. S. (2021). Therapeutic targets and nanomaterial-based therapies for mitigation of secondary injury after spinal cord injury. Nanomedicine (Lond) 16 (22), 2013–2028. doi:10.2217/nnm-2021-0113
Gaudin, A., Yemisci, M., Eroglu, H., Lepetre-Mouelhi, S., Turkoglu, O. F., Dönmez-Demir, B., et al. (2014). Squalenoyl adenosine nanoparticles provide neuroprotection after stroke and spinal cord injury. Nat. Nanotechnol. 9 (12), 1054–1062. doi:10.1038/nnano.2014.274
Gelain, F., Panseri, S., Antonini, S., Cunha, C., Donega, M., Lowery, J., et al. (2011). Transplantation of nanostructured composite scaffolds results in the regeneration of chronically injured spinal cords. ACS Nano 5 (1), 227–236. doi:10.1021/nn102461w
Geng, H., Chen, L., Tang, J., Chen, Y., and Wang, L. (2022). The role of CCL2/CCR2 Axis in cerebral ischemia-reperfusion injury and treatment: from animal experiments to clinical trials. Int. J. Mol. Sci. 23 (7), 3485. doi:10.3390/ijms23073485
Gu, C., Geng, X., Wu, Y., Dai, Y., Zeng, J., Wang, Z., et al. (2023). Engineered macrophage membrane-coated nanoparticles with enhanced CCR2 expression promote spinal cord injury repair by suppressing neuroinflammation and neuronal death. Small 20, e2305659. doi:10.1002/smll.202305659
Guo, J., Su, H., Zeng, Y., Liang, Y. X., Wong, W. M., Ellis-Behnke, R. G., et al. (2007). Reknitting the injured spinal cord by self-assembling peptide nanofiber scaffold. Nanomedicine 3 (4), 311–321. doi:10.1016/j.nano.2007.09.003
Harvey, L. A. (2016). Physiotherapy rehabilitation for people with spinal cord injuries. J. Physiother. 62 (1), 4–11. doi:10.1016/j.jphys.2015.11.004
He, X., Zhang, J., Guo, Y., Yang, X., Huang, Y., and Hao, D. (2022). Exosomal miR-9-5p derived from BMSCs alleviates apoptosis, inflammation and endoplasmic reticulum stress in spinal cord injury by regulating the HDAC5/FGF2 axis. Mol. Immunol. 145, 97–108. doi:10.1016/j.molimm.2022.03.007
Huang, J. H., He, H., Chen, Y. N., Liu, Z., Romani, M. D., Xu, Z. Y., et al. (2022). Exosomes derived from M2 macrophages improve angiogenesis and functional recovery after spinal cord injury through HIF-1α/VEGF Axis. Brain Sci. 12 (10), 1322. doi:10.3390/brainsci12101322
Imran, H., Tang, Y., Wang, S., Yan, X., Liu, C., Guo, L., et al. (2023). Optimized dox drug deliveries via chitosan-mediated nanoparticles and stimuli responses in cancer chemotherapy: a review. Molecules 29 (1), 31. doi:10.3390/molecules29010031
Jaffer, H., Andrabi, S. S., Petro, M., Kuang, Y., Steinmetz, M. P., and Labhasetwar, V. (2023). Catalytic antioxidant nanoparticles mitigate secondary injury progression and promote functional recovery in spinal cord injury model. J. Control Release 364, 109–123. doi:10.1016/j.jconrel.2023.10.028
Karsy, M., and Hawryluk, G. (2019). Modern medical management of spinal cord injury. Curr. Neurol. Neurosci. Rep. 19 (9), 65. doi:10.1007/s11910-019-0984-1
Katari, V., Pasupuleti, S. K., Mullick, M., Lekkala, V. K. R., and Sen, D. (2024). Editorial: advanced neural stem cell therapies for spinal cord injury. Front. Pharmacol. 15, 1469535. doi:10.3389/fphar.2024.1469535
Khan, T. I., Hemalatha, S., and Waseem, M. (2020). Promising role of nano-encapsulated drugs for spinal cord injury. Mol. Neurobiol. 57 (4), 1978–1985. doi:10.1007/s12035-019-01862-9
Kim, Y. T., Caldwell, J. M., and Bellamkonda, R. V. (2009). Nanoparticle-mediated local delivery of Methylprednisolone after spinal cord injury. Biomaterials 30 (13), 2582–2590. doi:10.1016/j.biomaterials.2008.12.077
Kumar, R., Lim, J., Mekary, R. A., Rattani, A., Dewan, M. C., Sharif, S. Y., et al. (2018). Traumatic spinal injury: global epidemiology and worldwide volume. World Neurosurg. 113, e345–e363. doi:10.1016/j.wneu.2018.02.033
Li, C., Qin, T., Jin, Y., Hu, J., Yuan, F., Cao, Y., et al. (2023). Cerebrospinal fluid-derived extracellular vesicles after spinal cord injury promote vascular regeneration via PI3K/AKT signaling pathway. J. Orthop. Transl. 39, 124–134. doi:10.1016/j.jot.2023.02.001
Li, C., Xiong, W., Wan, B., Kong, G., Wang, S., Wang, Y., et al. (2022b). Role of peripheral immune cells in spinal cord injury. Cell Mol. Life Sci. 80 (1), 2. doi:10.1007/s00018-022-04644-0
Li, J., Ji, Z., Wang, Y., Li, T., Luo, J., and Li, J. (2022a). Human adipose-derived stem cells combined with nano-hydrogel promote functional recovery after spinal cord injury in rats. Biol. (Basel) 11 (5), 781. doi:10.3390/biology11050781
Li, L., Zhang, Y., Mu, J., Chen, J., Zhang, C., Cao, H., et al. (2020). Transplantation of human mesenchymal stem-cell-derived exosomes immobilized in an adhesive hydrogel for effective treatment of spinal cord injury. Nano Lett. 20 (6), 4298–4305. doi:10.1021/acs.nanolett.0c00929
Li, Q., and Lan, P. (2023). Activation of immune signals during organ transplantation. Signal Transduct. Target Ther. 8 (1), 110. doi:10.1038/s41392-023-01377-9
Liao, S., Liu, Y., Kong, Y., Shi, H., Xu, B., Tang, B., et al. (2022). A bionic multichannel nanofiber conduit carrying Tubastatin A for repairing injured spinal cord. Mater Today Bio 17 (Dec 15), 100454. doi:10.1016/j.mtbio.2022.100454
Lin, S., Zhao, H. S., Xu, C., Zhou, Z. P., Wang, D. H., Chen, S. R., et al. (2021). Bioengineered zinc oxide nanoparticle-loaded hydrogel for combinative treatment of spinal cord transection. Front. Bioeng. Biotechnol. 9, 796361. doi:10.3389/fbioe.2021.796361
Liu, D., Lu, G., Shi, B., Ni, H., Wang, J., Qiu, Y., et al. (2023c). ROS-scavenging hydrogels synergize with neural stem cells to enhance spinal cord injury repair via regulating microenvironment and facilitating nerve regeneration. Adv. Healthc. Mater 12 (18), e2300123. doi:10.1002/adhm.202300123
Liu, X., Sun, J., Du, J., An, J., Li, Y., Hu, Y., et al. (2023b). Encapsulation of selenium nanoparticles and metformin in macrophage-derived cell membranes for the treatment of spinal cord injury. ACS Biomater. Sci. Eng. 9 (10), 5709–5723. doi:10.1021/acsbiomaterials.3c01009
Liu, Y., Yang, X., He, Z., Li, J., Li, Y., and Wu, Y. (2023a). Spinal cord injury: global burden from 1990 to 2019 and projections up to 2030 using Bayesian age-period-cohort analysis. Front. Neurol. 14, 1304153. doi:10.3389/fneur.2023.1304153
Lu, C., Park, S., Richner, T. J., Derry, A., Brown, I., Hou, C., et al. (2017). Flexible and stretchable nanowire-coated fibers for optoelectronic probing of spinal cord circuits. Sci. Adv. 3 (3), e1600955. doi:10.1126/sciadv.1600955
Luo, W., Li, Y., Xiang, C., Aizawa, T., Niu, R., Wang, Y., et al. (2023a). Nanomaterials as therapeutic agents to modulate astrocyte-mediated inflammation in spinal cord injury. Mater Today Bio 23, 100888. doi:10.1016/j.mtbio.2023.100888
Luo, Y., He, Y. Z., Wang, Y. F., Xu, Y. X., and Yang, L. (2023b). Adipose-derived mesenchymal stem cell exosomes ameliorate spinal cord injury in rats by activating the Nrf2/HO-1 pathway and regulating microglial polarization. Folia Neuropathol. 61 (3), 326–335. doi:10.5114/fn.2023.130455
Lv, J., Wang, Z., Wang, B., Deng, C., Wang, W., and Sun, L. (2024). S100A9 induces macrophage M2 polarization and immunomodulatory role in the lesion site after spinal cord injury in rats. Mol. Neurobiol. 61, 5525–5540. doi:10.1007/s12035-024-03920-3
Lyu, Y., Wang, T., Huang, S., and Zhang, Z. (2023). Mitochondrial damage-associated molecular patterns and metabolism in the regulation of innate immunity. J. Innate Immun. 15 (1), 665–679. doi:10.1159/000533602
Ma, J., Li, J., Wang, X., Li, M., Teng, W., and Tao, Z. (2023). GDNF-loaded polydopamine nanoparticles-based anisotropic scaffolds promote spinal cord repair by modulating inhibitory microenvironment. Adv. Healthc. Mater 12 (8), e2202377. doi:10.1002/adhm.202202377
McDonald, J. W., and Sadowsky, C. (2002). Spinal-cord injury. Lancet 359 (9304), 417–425. doi:10.1016/s0140-6736(02)07603-1
Müller, N., Scheld, M., Voelz, C., Gasterich, N., Zhao, W., Behrens, V., et al. (2023). Lipocalin-2 deficiency diminishes canonical NLRP3 inflammasome formation and IL-1β production in the subacute phase of spinal cord injury. Int. J. Mol. Sci. 24 (10), 8689. doi:10.3390/ijms24108689
Qian, T., Li, Z., Shang, L., Huang, S., Li, G., Zheng, W., et al. (2023). pH/temperature responsive curcumin-loaded micelle nanoparticles promote functional repair after spinal cord injury in rats via modulation of inflammation. Tissue Eng. Regen. Med. 20 (6), 879–892. doi:10.1007/s13770-023-00567-4
Ruan, H., Li, Y., Wang, C., Jiang, Y., Han, Y., and Li, Y. (2023). Click chemistry extracellular vesicle/peptide/chemokine nanocarriers for treating central nervous system injuries. Acta Pharm. Sin. B 13 (5), 2202–2218. doi:10.1016/j.apsb.2022.06.007
Sabourian, P., Frounchi, M., Kiani, S., Mashayekhan, S., Kheirabadi, M. Z., Heydari, Y., et al. (2023). Targeting reactive astrocytes by pH-responsive ligand-bonded polymeric nanoparticles in spinal cord injury. Drug Deliv. Transl. Res. 13 (6), 1842–1855. doi:10.1007/s13346-023-01300-3
Saeedi, M., Eslamifar, M., Khezri, K., and Dizaj, S. M. (2019). Applications of nanotechnology in drug delivery to the central nervous system. Biomed. Pharmacother. 111, 666–675. doi:10.1016/j.biopha.2018.12.133
Sahib, S., Sharma, A., Menon, P. K., Muresanu, D. F., Castellani, R. J., Nozari, A., et al. (2020). Cerebrolysin enhances spinal cord conduction and reduces blood-spinal cord barrier breakdown, edema formation, immediate early gene expression and cord pathology after injury. Prog. Brain Res. 258, 397–438. doi:10.1016/bs.pbr.2020.09.012
Santamaría, E., Maestro, A., and González, C. (2023). Use of double gelled microspheres to improve release control of cinnamon-loaded nanoemulsions. Molecules 29 (1), 158. doi:10.3390/molecules29010158
Shang, J., Jiang, C., Cai, J., Chen, Z., Jin, S., Wang, F., et al. (2023). Knowledge mapping of macrophage in spinal cord injury: a bibliometric analysis. World Neurosurg. 180, e183–e197. doi:10.1016/j.wneu.2023.09.022
Shao, Y., Wang, Q., Liu, L., Wang, J., and Wu, M. (2023). Alleviation of spinal cord injury by MicroRNA 137-overexpressing bone marrow mesenchymal stem cell-derived exosomes. Tohoku J. Exp. Med. 259 (3), 237–246. doi:10.1620/tjem.2022.J118
Shen, K., Li, X., Huang, G., Yuan, Z., Xie, B., Chen, T., et al. (2023). High rapamycin-loaded hollow mesoporous Prussian blue nanozyme targets lesion area of spinal cord injury to recover locomotor function. Biomaterials 303, 122358. doi:10.1016/j.biomaterials.2023.122358
Shi, Y., Kim, S., Huff, T. B., Borgens, R. B., Park, K., Shi, R., et al. (2010). Effective repair of traumatically injured spinal cord by nanoscale block copolymer micelles. Nat. Nanotechnol. 5 (1), 80–87. doi:10.1038/nnano.2009.303
Shipman, H., Monsour, M., Foley, M. M., Marbacher, S., Croci, D. M., and Bisson, E. F. (2023). IL-6 in spinal cord injury: could immunomodulation replace immunosuppression in the management of acute traumatic spinal cord injuries? J. Neurol. Surg. A Cent. Eur. Neurosurg.
Silva, G. A., Czeisler, C., Niece, K. L., Beniash, E., Harrington, D. A., Kessler, J. A., et al. (2004). Selective differentiation of neural progenitor cells by high-epitope density nanofibers. Science 303 (5662), 1352–1355. doi:10.1126/science.1093783
Silver, J., and Miller, J. H. (2004). Regeneration beyond the glial scar. Nat. Rev. Neurosci. 5 (2), 146–156. doi:10.1038/nrn1326
Solanki, A., Chueng, S. T., Yin, P. T., Kappera, R., Chhowalla, M., and Lee, K. B. (2013). Axonal alignment and enhanced neuronal differentiation of neural stem cells on graphene-nanoparticle hybrid structures. Adv. Mater 25 (38), 5477–5482. doi:10.1002/adma.201302219
Su, X., Jing, X., Jiang, W., Li, M., Liu, K., Teng, M., et al. (2023). Curcumin-Containing polyphosphazene nanodrug for Anti-Inflammation and nerve regeneration to improve functional recovery after spinal cord injury. Int. J. Pharm. 642, 123197. doi:10.1016/j.ijpharm.2023.123197
Syková, E., and Jendelová, P. (2006). Magnetic resonance tracking of transplanted stem cells in rat brain and spinal cord. Neurodegener. Dis. 3 (1-2), 62–67. doi:10.1159/000092095
Thuret, S., Moon, L. D., and Gage, F. H. (2006). Therapeutic interventions after spinal cord injury. Nat. Rev. Neurosci. 7 (8), 628–643. doi:10.1038/nrn1955
Tysseling-Mattiace, V. M., Sahni, V., Niece, K. L., Birch, D., Czeisler, C., Fehlings, M. G., et al. (2008). Self-assembling nanofibers inhibit glial scar formation and promote axon elongation after spinal cord injury. J. Neurosci. 28 (14), 3814–3823. doi:10.1523/JNEUROSCI.0143-08.2008
Venkatesh, K., Ghosh, S. K., Mullick, M., Manivasagam, G., and Sen, D. (2019). Spinal cord injury: pathophysiology, treatment strategies, associated challenges, and future implications. Cell Tissue Res. 377 (2), 125–151. doi:10.1007/s00441-019-03039-1
Wang, F., Zhang, S., Xu, Y., He, W., Wang, X., He, Z., et al. (2024). Mapping the landscape: a bibliometric perspective on autophagy in spinal cord injury. Med. Baltim. 103 (29), e38954. doi:10.1097/MD.0000000000038954
Wang, N., Yu, H., Song, Q., Mao, P., Li, K., and Bao, G. (2021b). Sesamol-loaded stearic acid-chitosan nanomicelles mitigate the oxidative stress-stimulated apoptosis and induction of pro-inflammatory cytokines in motor neuronal of the spinal cord through NF-ĸB signaling pathway. Int. J. Biol. Macromol. 186 (Sep 1), 23–32. doi:10.1016/j.ijbiomac.2021.06.171
Wang, X., Wu, J., Liu, X., Tang, K., Cheng, L., Li, J., et al. (2021a). Engineered liposomes targeting the gut-CNS Axis for comprehensive therapy of spinal cord injury. J. Control Release 331, 390–403. doi:10.1016/j.jconrel.2021.01.032
Wang, X., Yang, Y., Li, W., Hao, M., and Xu, Y. (2023). Umbilical mesenchymal stem cell-derived exosomes promote spinal cord functional recovery through the miR-146b/TLR4 -mediated NF-κB p65 signaling pathway in rats. Biochem. Biophys. Rep. 35, 101497. doi:10.1016/j.bbrep.2023.101497
Weng, J., Wang, L., Wang, K., Su, H., Luo, D., Yang, H., et al. (2023). Tauroursodeoxycholic acid inhibited apoptosis and oxidative stress in H(2)O(2)-induced BMSC death via modulating the nrf-2 signaling pathway: the therapeutic implications in a rat model of spinal cord injury. Mol. Neurobiol. 61, 3753–3768. doi:10.1007/s12035-023-03754-5
Wu, F., Wang, P., Wei, X., Yang, Y., Al Mamun, A., Zhang, X., et al. (2023). Barrier-penetrating liposome targeted delivery of basic fibroblast growth factor for spinal cord injury repair. Mater Today Bio 18, 100546. doi:10.1016/j.mtbio.2023.100546
Xia, N., Gao, Z., Hu, H., Li, D., Zhang, C., Mei, X., et al. (2021). Nerve growth factor loaded macrophage-derived nanovesicles for inhibiting neuronal apoptosis after spinal cord injury. J. Biomater. Appl. 36 (2), 276–288. doi:10.1177/08853282211025912
Xiao, Z., Tang, F., Zhao, Y., Han, G., Yin, N., Li, X., et al. (2018). Significant improvement of acute complete spinal cord injury patients diagnosed by a combined criteria implanted with NeuroRegen scaffolds and mesenchymal stem cells. Cell Transpl. 27 (6), 907–915. doi:10.1177/0963689718766279
Xie, Y., Sun, Y., Liu, Y., Zhao, J., Liu, Q., Xu, J., et al. (2023). Targeted delivery of RGD-cd146(+)cd271(+) human umbilical cord mesenchymal stem cell-derived exosomes promotes blood-spinal cord barrier repair after spinal cord injury. ACS Nano 17 (18), 18008–18024. doi:10.1021/acsnano.3c04423
Xing, X., Xu, P., Xing, X., Xu, Z., Huang, Z., Li, Z., et al. (2024). Effects of ADSC-derived exosome lrrc75a-AS1 on anti-inflammatory function after SCI. Appl. Biochem. Biotechnol. 196, 5920–5935. doi:10.1007/s12010-023-04836-9
Xiong, T., Yang, K., Zhao, T., Zhao, H., Gao, X., You, Z., et al. (2023b). Multifunctional integrated nanozymes facilitate spinal cord regeneration by remodeling the extrinsic neural environment. Adv. Sci. (Weinh) 10 (7), e2205997. doi:10.1002/advs.202205997
Xiong, W., Tian, H., Li, Z., Peng, Z., and Wang, Y. (2023a). Curcumin-primed umbilical cord mesenchymal stem cells-derived extracellular vesicles improve motor functional recovery of mice with complete spinal cord injury by reducing inflammation and enhancing axonal regeneration. Neurochem. Res. 48 (5), 1334–1346. doi:10.1007/s11064-022-03832-5
Xue, H., Ran, B., Li, J., Wang, G., Chen, B., and Mao, H. (2023). Bone marrow mesenchymal stem cell exosomes-derived microRNA-216a-5p on locomotor performance, neuronal injury, and microglia inflammation in spinal cord injury. Front. Cell Dev. Biol. 11, 1227440. doi:10.3389/fcell.2023.1227440
Yin, X., Lin, S., Xiong, Y., Zhang, P., and Mei, X. (2023). Biomimetic nanoplatform with anti-inflammation and neuroprotective effects for repairing spinal cord injury in mice. Mater Today Bio 23, 100836. doi:10.1016/j.mtbio.2023.100836
Yuan, F., Peng, W., Yang, Y., Xu, J., Liu, Y., Xie, Y., et al. (2023). Endothelial progenitor cell-derived exosomes promote anti-inflammatory macrophages via SOCS3/JAK2/STAT3 axis and improve the outcome of spinal cord injury. J. Neuroinflammation 20 (1), 156. doi:10.1186/s12974-023-02833-7
Zeng, J., Gu, C., Sun, Y., and Chen, X. (2023). Engineering of M2 macrophages-derived exosomes via click chemistry for spinal cord injury repair. Adv. Healthc. Mater 12 (11), e2203391. doi:10.1002/adhm.202203391
Zhang, S., Shang, J., Gu, Z., Gu, X., Wang, F., Hu, X., et al. (2023). Global research trends and hotspots on tendon-derived stem cell: a bibliometric visualization study. Front. Bioeng. Biotechnol. 11, 1327027. doi:10.3389/fbioe.2023.1327027
Zhang, X., Xiong, W., Kong, G., Zhen, Y., Zeng, Q., Wang, S., et al. (2022). Paclitaxel-incorporated nanoparticles improve functional recovery after spinal cord injury. Front. Pharmacol. 13, 957433. doi:10.3389/fphar.2022.957433
Zhao, F., Zou, X., Li, S., He, J., Xi, C., Tang, Q., et al. (2021). BmK NSPK, a potent potassium channel inhibitor from scorpion buthus martensii karsch, promotes neurite outgrowth via NGF/TrkA signaling pathway. Toxins (Basel) 13 (1), 33. doi:10.3390/toxins13010033
Zhou, Q., Jin, H., Shi, N., Gao, S., Wang, X., Zhu, S., et al. (2021). Inhibit inflammation and apoptosis of pyrroloquinoline on spinal cord injury in rat. Ann. Transl. Med. 9 (17), 1360. doi:10.21037/atm-21-1951
Zhou, Z., Li, C., Bao, T., Zhao, X., Xiong, W., Luo, C., et al. (2022). Exosome-shuttled miR-672-5p from anti-inflammatory microglia repair traumatic spinal cord injury by inhibiting AIM2/ASC/Caspase-1 signaling pathway mediated neuronal pyroptosis. J. Neurotrauma 39 (15-16), 1057–1074. doi:10.1089/neu.2021.0464
Zhu, R., Zhu, X., Zhu, Y., Wang, Z., He, X., Wu, Z., et al. (2021). Immunomodulatory layered double hydroxide nanoparticles enable neurogenesis by targeting transforming growth factor-β receptor 2. ACS Nano 15 (2), 2812–2830. doi:10.1021/acsnano.0c08727
Zhu, S. P., Wang, Z. G., Zhao, Y. Z., Wu, J., Shi, H. X., Ye, L. B., et al. (2016). Gelatin nanostructured lipid carriers incorporating nerve growth factor inhibit endoplasmic reticulum stress-induced apoptosis and improve recovery in spinal cord injury. Mol. Neurobiol. 53 (7), 4375–4386. doi:10.1007/s12035-015-9372-2
Keywords: spinal cord injury, nanotechnology, inflammation, bibliometric, CiteSpace
Citation: Gu X, Zhang S and Ma W (2024) Bibliometric analysis of nanotechnology in spinal cord injury: current status and emerging frontiers. Front. Pharmacol. 15:1473599. doi: 10.3389/fphar.2024.1473599
Received: 31 July 2024; Accepted: 27 November 2024;
Published: 11 December 2024.
Edited by:
lili Jin, Yanbian University, ChinaReviewed by:
Venkatesh Katari, University of Toledo, United StatesCopyright © 2024 Gu, Zhang and Ma. This is an open-access article distributed under the terms of the Creative Commons Attribution License (CC BY). The use, distribution or reproduction in other forums is permitted, provided the original author(s) and the copyright owner(s) are credited and that the original publication in this journal is cited, in accordance with accepted academic practice. No use, distribution or reproduction is permitted which does not comply with these terms.
*Correspondence: WeiHu Ma, d2VpaHVtYUAxMjYuY29t
†These authors share first authorship
Disclaimer: All claims expressed in this article are solely those of the authors and do not necessarily represent those of their affiliated organizations, or those of the publisher, the editors and the reviewers. Any product that may be evaluated in this article or claim that may be made by its manufacturer is not guaranteed or endorsed by the publisher.
Research integrity at Frontiers
Learn more about the work of our research integrity team to safeguard the quality of each article we publish.