- 1Interdisciplinary Centre of Marine and Environmental Research (CIIMAR), University of Porto, Matosinhos, Portugal
- 2School of Medicine and Biomedical Sciences Abel Salazar (ICBAS), University of Porto, Porto, Portugal
Bioprospecting actinobacterial secondary metabolism from untapped marine sources may lead to the discovery of biotechnologically-relevant compounds. While studying the diversity and bioactive potential of Actinomycetota associated with Codium tomentosum, a green seaweed collected in the northern Portuguese cost, strain CT-F61, identified as Streptomyces violaceoruber, was isolated. Its extracts displayed a strong anticancer activity on breast carcinoma T-47D and colorectal carcinoma HCT116 cells, being effective as well against a panel of human and fish pathogenic bacteria. Following a bioactivity-guided isolation pipeline, a new analogue of the red-pigmented family of the antibiotics prodigiosins, decylprodigiosin (1), was identified and chemically characterized. Despite this family of natural products being well-known for a long time, we report a new analogue and the first evidence for prodigiosins being produced by a seaweed-associated actinomycete.
Introduction
Nature is a wealthy reservoir of biotechnologically-relevant molecules, some of them even labelled as prodigious (i.e., something marvellous), as the family of the blood-red pigmented bacterial alkaloids, prodigiosins. This group of compounds harbours a diverse set of heterocyclic natural products (NPs), with historical evidence dating back to the beginning of the 19th century (Bennett and Bentley, 2000). Surveying the broad spectrum of properties that prodigiosin and prodigiosin-related molecules encode, from antimicrobial, antimalarial, anticancer, algicidal, antiparasitic to antiviral and immunosuppressive (Castro, 1967; Montaner and Prez-Toms, 2003; Lapenda et al., 2015; Zhang et al., 2016; Zhou et al., 2016; Ehrenkaufer et al., 2020), it becomes clear why they have attracted the attention of NPs research programs for so many years. Apart from their value in medical industry, they are also used in food, cosmetics and dye markets as an eco-friendlier and cost effective alternative to synthetic pigments (Paul et al., 2020). Prodigiosins have been reported to be produced by a wide range of Gram-negative and positive bacteria. Examples include members of the Pseudomonadota phylum as Alteromonas rubra, Achromobacter denitrificans, Beneckea gazogenes, Hahella chejuensis, Janthinobacterium lividum, Rugamonas rubra, Zooshikela rubidus, (Harwood, 1978; Gerber and Gauthier, 1979; Austin and Moss, 1986; Schloss et al., 2010; Lee et al., 2011; Pradeep et al., 2014), Pseudoalteromonas (Kawauchi et al., 1997; Klein et al., 2017), Serratia (Williams, 1973; Berg, 2000; Miao et al., 2013; Su et al., 2016; Halder et al., 2020) and Vibrio (Allen et al., 1983; Vitale et al., 2020). Members of the phylum Actinomycetota, considered the most prolific bacterial source of drug-lead chemicals (Bahrami et al., 2022), have also been reported to synthetize prodigiosins. From several species of Streptomyces (S. longisporuber, Streptomyces griseoviridis and S. coelicolor), Actinomadura (Actinomadura madurae and A. pelletieri), and from Streptoverticillium rubrireticuli, undecylprodigiosin, butylcycloheptylprodigiosine, metacycloprodigiosin, nonylprodigiosin, prodigiosin 25-C and prodigiosins R1, R2 and R3 were described, highlighting actinobacterial metabolism richness in the production of these tripyrrole red pigments (Harashima et al., 1967; Gerber, 1969; Gerber, 1975; TSAO et al., 1985; Kawasaki et al., 2008; Kimata et al., 2018; Islan et al., 2022; Kimata et al., 2023). The widespread occurrence of prodigiosins in phylogenetically distant microbes suggests that these molecules may play a significant, albeit uncertain and yet to be fully defined, physiological role. In recent years, marine-sourced Actinomycetota have proved their value as source of relevant chemistry (Girão et al., 2022). Some known prodigiosins and related molecules, exhibiting a wide range of bioactive properties, have been found to be produced by Streptomyces species living in association with sponges (El-Bondkly et al., 2012; Abdelfattah et al., 2019) and inhabiting deep-sea sediments (Song et al., 2015). Also from a marine Streptomyces, two novel spiroaminals, marineosins A and B, obtained from unknown modifications of prodigiosin-like pigment pathways and exhibiting significant anticancer activity, have been discovered (Boonlarppradab et al., 2008). Streptomyces can be found in many places in the marine ecosystem, including in association with seaweeds (Girão et al., 2019), but little is known regarding bioactive NPs biosynthesis as part of such associations. As prodigiosins display algicidal properties, they are able to inhibit and control the growth of various microalgae and cyanobacteria (Zhang et al., 2016; Yang et al., 2017; Zhang et al., 2017; Zhang et al., 2020), by disrupting cell membranes, interfering with photosynthesis, and/or inducting oxidative stress, having been considered good candidates for algal bloom management and aquatic ecosystem restoration. In this work, by exploring the secondary metabolism of the seaweed-associated Streptomyces violaceoruber CT-F61, isolated from the tissues of Codium tomentosum, a new 10-carbon alkyl chain prodigiosin was discovered and chemically characterized. To our knowledge, the presence of bacteria producing prodigiosins living in association with macroalgae has hitherto not been described. We briefly discuss the potential ecological significance of this finding.
Materials and methods
Sampling and bacterial isolation
One specimen of Codium tomentosum was collected in the intertidal rocky northern Portuguese shore (41.309298°; −8.742228°). The macroalga was transported to the laboratory under refrigeration and processed on the same day for Actinomycetota isolation. The seaweed was washed with sterile sea water and macerated using a sterile mortar. To increase the success of actinobacterial isolation, the macerated tissues were incubated in a water bath at 58 °C for 15 min, to limit the incidence of non-spore forming microorganisms and boost the development of slow growing strains. From a tissue sample inoculated in Actinomycete Isolation Agar (AIA)—4 g C3H5NaO2, 0.5 g K2HPO4, 0.2 g Na2CO3, 0.2 g FeSO4, 0.2 g MgSO4 and 0.1 g L-arginine per litre of a 3:2 mixture of deionized water/seawater, supplemented with cycloheximide and nalidixic acid (50 mg L−1; Sigma-Aldrich, MO, United States)—strain CT-F61 was isolated as an axenic culture.
Taxonomic and phylogenetic analysis of Streptomyces violaceoruber CT-F61
Strain CT-F61 was taxonomically identified through 16S rRNA gene sequencing. Biomass of the strain was obtained from a 2-week old culture in AIA liquid medium, and its genomic DNA was extracted using the E. Z.N.A. Bacterial DNA Kit (Omega Bio-Tek, GA, United States). The 16S rRNA gene was amplified by PCR (universal primers 27F and 1492R (Weisburg et al., 1991)) and sequenced as described by Girão et al., 2019 (Girão et al., 2019). The obtained sequences were analysed using Geneious Prime 2023.1 software (Biomatters, Auckland, New Zealand). The taxonomic identification of CT-F61 was established by comparison of its 16S rRNA gene consensus sequence with type strains deposited in the EzBioCloud database (Yoon et al., 2017). To infer the evolutionary relationship between CT-F61 and its closest related species, a phylogenetic tree was built. The 15 consensus sequences closest to CT-F61 (according to ExTaxon database) were selected and aligned, together with CT-F61 16S rRNA gene sequence and B. subtilis NCIB 3610T as an outgroup, using the MUSCLE tool from the Geneious software package. An alignment of 1366 bp was used to construct the phylogenetic tree, applying the Maximum Likelihood method with 1000 bootstraps based on the Tamura-Nei model. Evolutionary analyses were conducted in MEGA X (Kumar et al., 2018).
Bioactivity screenings
Strain CT-F61 was cultured in AIA medium, and its organic extract obtained, following the methodology previously described in Girão et al. (Girão et al., 2019). This crude extract was tested against a panel of human and fish pathogenic microbial strains (Table 1), using the agar-based disc diffusion method. The assay was performed with the turbidity of each test organism adjusted within the 0.5 McFarland standard (OD625 = 0.13) in the corresponding culture media. The suspensions were used to inoculate agar plates by evenly streaking each with a swab dipped in the suspension. In each blank disk (6 mm in diameter, Whatman, UK), placed on the surface of the inoculated plates, 15 µL of the crude extract (1 mg mL-1, dissolved in dimethyl sulfoxide DMSO ≥99.9%; Sigma-Aldrich, MO, United States) were loaded and the plates incubated accordingly to the reference organism requirements (Table 1). Antimicrobial activity was determined by measuring the diameter of the inhibition halo formed around the disc. Positive controls consisted in Enrofloxacin (1 mg mL-1) for all bacterial strains and Nystatin (1 mg mL-1) for the yeast Candida albicans. DMSO was used as negative control for all the microorganisms. The crude extract was tested in triplicate, in two independent experiments. Results are presented as the average diameter of the inhibition halos measured (mm) in each experiment. The cytotoxic activity of CT-F61 crude extract was tested at a concentration of 15 μg mL-1 in monolayer cell cultures of two cancer and one non-cancer cell lines—T-47D cells (breast ductal carcinoma), HCT116 (colorectal carcinoma) and hCMEC/D3 (human brain capillary endothelial cells), respectively—using the MTT [3-(4,5-dimethylthiazol-2-yl)-2,5-diphenyltetrazolium bromide] assay, as previously described (Girão et al., 2019). The crude extract was tested in triplicate, in two independent experiments. Results are presented as percentage of cellular viability relative to the solvent control, after 48 h of exposure. Data from cytotoxic assays (six technical replicates in total per sample) was tested for significant differences compared to the solvent control, and the significance level was set for all tests at p < 0.05. The normality distribution of data was accessed using the Kolmogorov Smirnov test. One-Way ANOVA was applied followed by Dunnett’s post hoc test for parametric data, and Kruskal–Wallis test, followed by Dunn’s multiple comparison test, used for non-parametric data. The apoptotic Staurosporine (Sigma-Aldrich, MO, United States) was used as positive control, at the same concentration as the extracts, and 0.5% DMSO was used as solvent control.
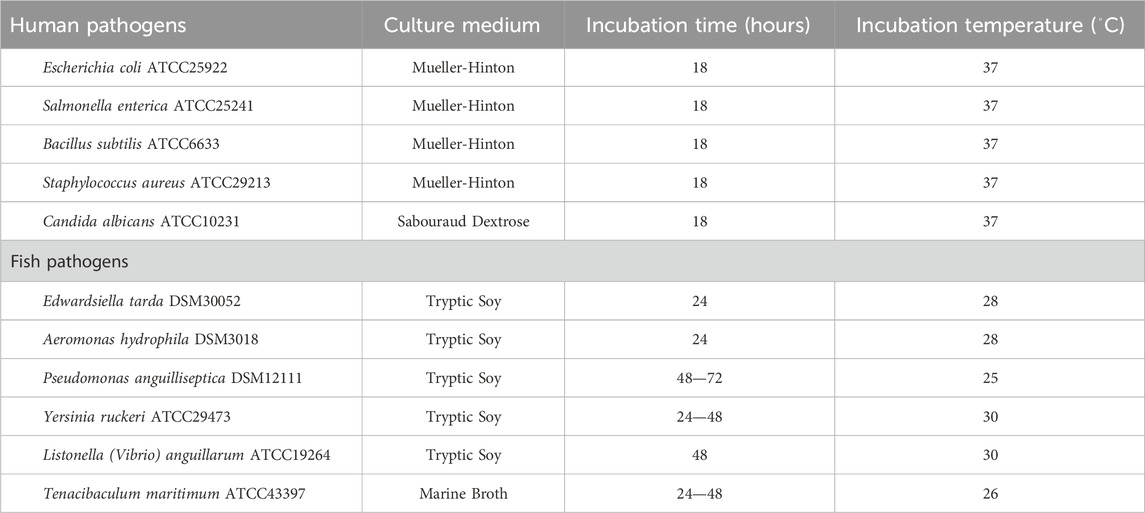
Table 1. Reference strains used in the antimicrobial assay with the indication of the culture medium used, incubation time and temperature.
Decylprodigiosin isolation and structure elucidation
To obtain sufficient amounts of compounds for a bioactivity-guided isolation, strain CT-F61 was cultivated in a larger scale (24 L) using 5 L Erlenmeyer flasks, each containing 2 L of AIA culture medium. A pre-inoculum of 100 mL was prepared in the same culture medium, using a 250 mL Erlenmeyer flask, to inoculate the bigger flasks. After 7 days of incubation, 30 g of Amberlite XAD16N resin (Sigma-Aldrich, MO, United States) were added to each flask and incubation continued for seven additional days. The biomass and resin were recovered by centrifugation (2500g, 5 min), lyophilized, and repeatedly extracted using a mixture of acetone/methanol 1:1 (v/v). After confirming the previously observed biological activities, by testing the obtained organic extract in the formerly described sets of assays, a reverse-phase vacuum liquid chromatography (VLC) of the organic extract was performed using a solvent polarity gradient (Supplementary Table S1) on a glass chromatography column. All resulting fractions were tested for antimicrobial and cytotoxic activities and examined for the presence of unknown molecules using Global Natural Products Social Molecular Networking (GNPS) dereplication tools (Wang et al., 2016), based on liquid chromatography coupled to high-resolution electrospray ionization mass spectrometry (LC-HRESIMS/MS) analysis. LC-HRESIMS/MS analyses were performed on an UltiMate 3000 UHPLC (Thermo Fisher Scientific) system composed of an LPG-3400SD pump, WPS-3000SL autosampler, and VWD-3100 UV/vis detector coupled to a Q Exactive Focus Hybrid Quadrupole-Orbitrap mass spectrometer controlled by Q Exactive Focus Tune 2.9 and Xcalibur 4.1 (Thermo Fisher Scientific, US). For LC-HRESIMS data, full scan mode was used with the capillary voltage set to −3.8 kV, capillary temperature to 300°C, and sheath gas flow rate to 35 units. The active VLC fractions with no hits for known compounds in the GNPS-based dereplication were selected and further subjected to a reverse-phase semi-preparative high-performance liquid chromatography (HPLC) (flow 3 mL min–1, column ACE 10 C18-AR, 250 × 10 mm; Supplementary Table S2). All resulting fractions (Supplementary Figure S1) were tested for bioactivity. Manual dereplication of mass features present in the most active fractions against the Dictionary of Natural Products database (version 32.1—dnp. chemnetbase.com) and the NP Atlas (version 2023_06—npatlas. org) unveiled the presence of an undescribed mass feature m/z 380.2699 [M + H]+. Fractions containing this mass were pooled and an additional purification step targeting this putative new compound was performed in a reverse-phase analytical HPLC (flow 0.8 mL min–1, column ACE Excel 3 Super C18 V19-3214 75 × 4.6 mm; Supplementary Table S3). The chemical structure of this compound was elucidated by comparing its MS/MS data with those of commercial undecylprodigiosin (Wasserman et al., 1966) standard (Abcam, Netherlands). LC-HRESIMS/MS analysis of both molecules was performed by direct injection of each (1.0 mg mL–1, flow 0.005 mL min–1) into the spectrometer, with a 35,000 fwhm resolution, using an isolation window of 1 m/z, loop count of 3, AGC target of 5 × 104, and a collision energy of 35 (arbitrary units). The parent mass of each molecule was selected for fragmentation and the resulting MS/MS spectra fragmentation patterns compared. Additionally, 1H (600 MHz) NMR spectroscopy was used to a more comprehensive understanding of the novel compound molecular structure. The NMR data were acquired in methanol-d4 (CD3OD).
Streptomyces violaceoruber CT-F61 genome sequencing
The previously obtained gDNA of strain CT-F61 was sequenced using Illumina 2 × 250 bp paired-end technology. The identification of the closest reference genomes for reading map was performed using Kraken 2 (Wood et al., 2019), reads quality check was done using BWA-MEM and final assembly was established using SPAdes (Bankevich et al., 2012). DFAST (Tanizawa et al., 2018) was used to determine completeness and contamination. The genome sequence was annotated using the NCBI Prokaryotic Genome Annotation Pipeline and deposited at GenBank under the accession number SAMN37527650. AntiSMASH 6.0 (Blin et al., 2021) was used for the automated analysis and identification of secondary metabolite biosynthetic gene clusters using relaxed detection settings and all extra features selected.
Results and discussion
Streptomyces violaceoruber CT-F61 isolation and taxonomic identification
Our continued efforts in exploring seaweed-associated actinobacterial diversity led to the isolation of Streptomyces violaceoruber CT-F61 from the Chlorophyta Codium tomentosum. From the macroalgae frond tissues, using the selective culture medium AIA, formulated with 40% of seawater, a regular, opaque, white spore forming colony, able to change the culture medium colour from yellowish to dark blue, was isolated from the frond tissues of C. tomentosum, purified, and named as strain CT-F61. According to the EzBioCloud 16S database, strain CT-F61 showed the highest 16S rRNA gene sequence similarity to S. violaceoruber NBRC 12826T, Streptomyces anthocyanicus NBRC 14892T and Streptomyces tricolor NBRC 15461T (all 99.85%), three species that have been recently classified as heterotypic synonyms of S. violaceoruber based on multilocus sequence analysis (Komaki, 2022). Phylogenetic assessment showed that strain CT-F61 clustered with the three type strains mentioned above (Figure 1). Although 16S rRNA gene is traditionally used in bacterial systematics, its resolution might not be sufficient for species identification, especially for genera integrating a large number of species, like the Streptomyces genus. Genome sequencing of strain CT-F61 showed a closest association to S. anthocyanicus JCM 5058, with 99.36% average nucleotide identity (ANI) between the two strains, based on Genome Taxonomy Database (GTDB) taxonomy assignment. Considering all the mentioned aspects, strain CT-F61 was identified as Streptomyces violaceoruber CT-F61.
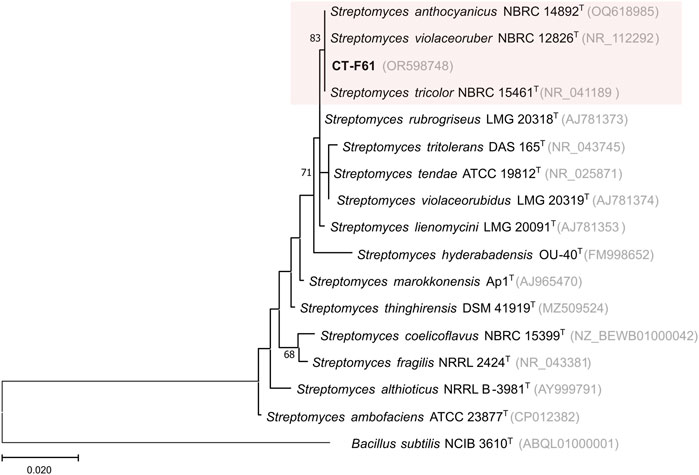
Figure 1. Maximum-likelihood phylogenetic tree, based on 16S rRNA gene sequences (1366 nt), showing the relationship between strain CT-F61 and closest related type species. Accession numbers are indicated in brackets. Values at the nodes indicate bootstrap values higher than 50%, obtained from 1000 resampling events. Bacillus subtilis NCIB 3610T was used as outgroup.
Bioactivity screening
In order to assess the bioactive properties of S. violaceoruber CT-F61, we cultivated this strain in a small scale (SS; 30 mL) with 0.5 g of resin, and tested an organic (acetone/MeOH, 1:1 - both cells and resin material were extracted together) extract from this culture, dissolved in DMSO, against a panel of pathogenic microbial strains and on different human cancer cell lines, using a test concentration of 15 μg mL-1. The extract from this seaweed-associated actinomycete inhibited the growth of the Gram-positive bacteria B. subtilis and Staphylococcus aureus (Figure 2A). Exploring new sources of pharmaceutically-relevant compounds is crucial to address major global crisis as antibiotic resistance, responsible for over 700,000 human deaths annually (Church and McKillip, 2021), or the pressing and lacking-solution cancer pathologies (Ferlay et al., 2021). Additionally, CT-F61 proved to inhibit the growth of the Gram-negative fish pathogens T. maritimum, L. anguillarum and Aeromonas hydrophila. Tenacibaculum maritimum is a bacterial pathogen responsible for tenacibaculosis, an ulcerative disease causing significant mortalities in various marine fish species worldwide, with high economic impact in aquaculture industry (Mabrok et al., 2023). Similar losses occur due to L. anguillarum, agent of vibriosis (Hickey and Lee, 2018), and A. hydrophila that distresses fishes with gastroenteritis and septicemia (Semwal et al., 2023). Molecules encoded in CT-F61 crude were thus found to have potential to address these diseases, a less-explored biotechnological application of prodigiosins. No inhibitory activity was recorded against the growth of the human pathogens E. coli and Salmonella enterica and C. albicans, and the aquaculture-relevant species E. tarda, P. anguilliseptica and Y. ruckeri. In the cancer cell line assays, the extract reduced the viability of breast carcinoma T-47D and colorectal carcinoma HCT116 cell lines by more than 80%, with no deleterious effect on non-cancer cells (Figure 2A).
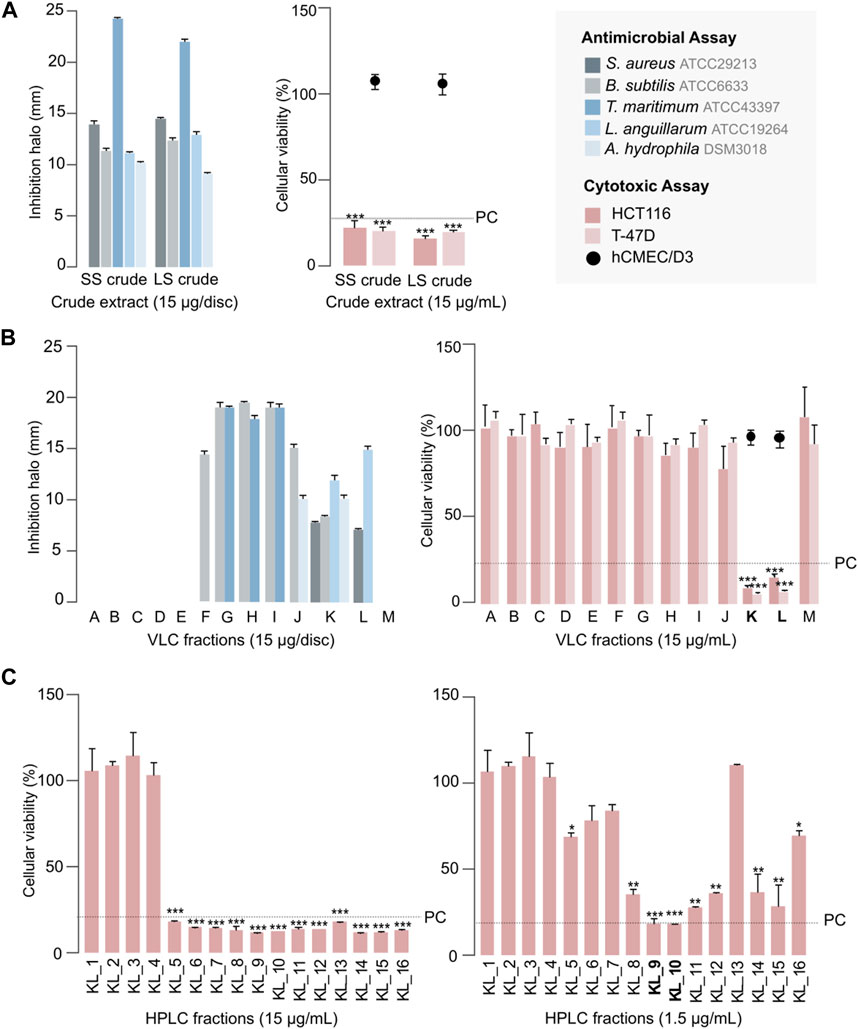
Figure 2. Antimicrobial and cytotoxic activities of CT-F61 crude extract, obtained from the small-scale (SS) and large-scale (LS) cultivation (A), C18 VLC fractions of CT-F61 LS crude extract (B) and CT-F61 KL C18 HPLC fractions tested at 15 μg mL-1 and 1.5 μg mL-1 in HCT116 cell line (C). Antimicrobial results presented as mean of the diameter of the inhibition halos measured from two independent experiments. Only reference strains affected by at least one tested sample are presented. Cytotoxic results presented as percentage of cellular viability after 48 h of exposure, measured as mean from two independent MTT experiments, performed with triplicates to each sample. Significant differences compared to the solvent control (*p < 0.05; **p < 0.01; ***p < 0.001). The percentage of cellular viability for the positive control (PC: Staurporine 15 μg mL-1) is indicated, as well as the samples activity on the non-carcinogenic cell line hCMEC/D3.
Bioactivity-guided isolation and structure elucidation of decylprodigiosin
Dereplication of the CT-F61 organic extract using GNPS Dereplicator, Dereplicator VarQuest, and Dereplicator+ tools (Mohimani et al., 2017; Gurevich et al., 2018; Mohimani et al., 2018) did not lead to the identification of known compounds that could explain the observed biological activity. Thus, we performed large-scale cultivation (LS; 24 L) of the strain, in order to isolate any putative novel bioactive compound from its metabolome. An organic crude extract of 5.7 g, with a similar bioactive profile as the one previously recorded for the SS culture, was obtained (Figure 2A). A set of sequential chromatographic steps was then used to purify the bioactive compounds of interest. All generated fractions were subjected to HRMS dereplication to avoid the isolation of known molecules. The VLC (C18 stationary phase) of the LS crude extract led to 13 fractions (A-M) of decreasing polarity. All fractions were tested for antimicrobial and cytotoxic activities (Figure 2B). Several fractions proved to be effective in inhibiting the growth of the reference bacterial strains, with fraction K being active against all, except T. maritimum. Fractions K and L presented activity on both cancer cell lines tested (p < 0.001), with no effect on the viability of the non-cancer cell line. From all the results recorded, in this work we decided to follow the strong anticancer activity of fractions K and L towards the human cancer cell lines T-47D and HCT116. Fractions K and L were pooled (25.1 mg) and fractionated by C18 semi-preparative HPLC. Sixteen fractions were obtained and tested for cytotoxic activity in HCT116 cell line at 15 μg mL-1 and 1.5 μg mL-1 (Figure 2C). Fractions KL_5 to KL_16 showed strong cytotoxicity when tested at 15 μg mL-1, but at 1.5 μg mL-1 only fractions KL_9 and KL_10 retained strong cytotoxic activity. Despite the initial dereplication step, fractions KL_9 and KL_10 were dereplicated using GNPS tools to investigate if the recorded cytotoxic activity was due to any putative novel compound. From this analysis, two mass features associated to undecylprodigiosin and butylcyclohexylprodigiosin, known members of the prodigiosins family, were detected. Yet, a more detailed manual search using the Dictionary of NP database and NP Atlas revealed that the protonated ion [M + H]+ at m/z 380.2699, detected in the same fractions, was not associated to any described prodigiosin, suggesting that this was a new compound (Figure 3). Is noteworthy to mention that no prodigiosin or related compound was initially detected by GNPS dereplicator tools in the crude extract or VLC fractions, as these were possibly masked by the complex matrix. The presence of undecylprodigiosin and butylcyclohexylprodigiosin could explain the recorded bioactivity, as their anticancer and antibacterial properties are well-recognized. Nonetheless, we decided to focus on the potentially-new prodigiosin analogue. To our knowledge, prodigiosins or related compounds have not been reported so far from strains affiliated to the species S. violaceoruber, S. anthocyanicus or S. tricolor (heterotypic synonyms of S. violaceoruber). Fractions KL_9 and KL_10 were combined (6.8 mg) and processed in a C18 analytical HPLC to further purify the new molecule. Based on the NMR data it was clear that the compound contained typical prodigiosin signals, (δH 7.5–6.20, associated to the pyrrole rings, as well as a large methylene envelope δH 1.29–1.25), but was not pure. Due to the low amount of compound isolated we decided to approach its structure elucidation using MS/MS. A standard of undecylprodigiosin was acquired and a MS/MS fragmentation comparative study was performed (Figure 4A). Using this approach, we could conclude that 1 m/z 380.2699 [M + H]+ differs from undecylprodigiosin on the carbon alkyl chain with a loss of a methylene group (−14 atomic mass units), conserving the aromatic moieties (m/z 238.0971). Based on this 10-carbon alkyl chain feature and the absence of 1H NMR signals pointing towards a terminal isopropyl moiety, this chain is proposed to be linear and the compound was designated as decylprodigiosin (1, Figure 4B). Reported isopropyl-containing alkyl chains in prodigiosins are odd-numbered (van Santen et al., 2022). Additional studies must be performed to characterize the bioactive properties of this novel molecule. To confirm that strain CT-F61 contains the genetic information necessary to produce prodigiosins, we sequenced its genome. The genome data was assembled into one contig with a length of 8 599 857 bp, with in silico G + C content of 72.2 mol%. DFAST results of completeness and contamination were 99.92% and 0.08%, respectively. As predicted, using AntiSMASH we identified a genomic region in the genome of strain CT-F61 in which the entire set of genes associated with the production of undecylprodigiosin (biosynthetic gene cluster from Streptomyces coelicolor A3 (2)—MIBiG accession: BGC0001063) could be found. The even-numbered chain in one could be derived from an odd-numbered starter unit extended by the polyketide synthase machinery involved in the biosynthesis of actinobacterial prodigiosins (Hu et al., 2016).
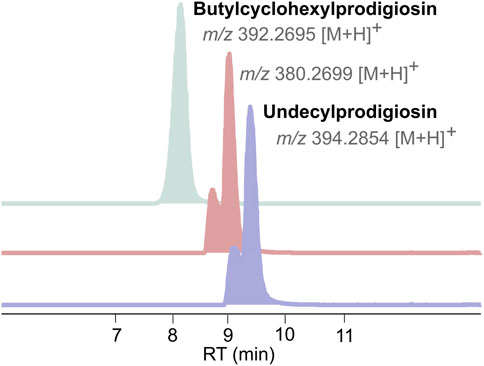
Figure 3. Extracted ion chromatograms (EICs) of Undecylprodigiosin and Butylcyclohexylprodigiosin, detected in KL_9 and KL_10 fractions, alongside with the mass feature corresponding to 1.
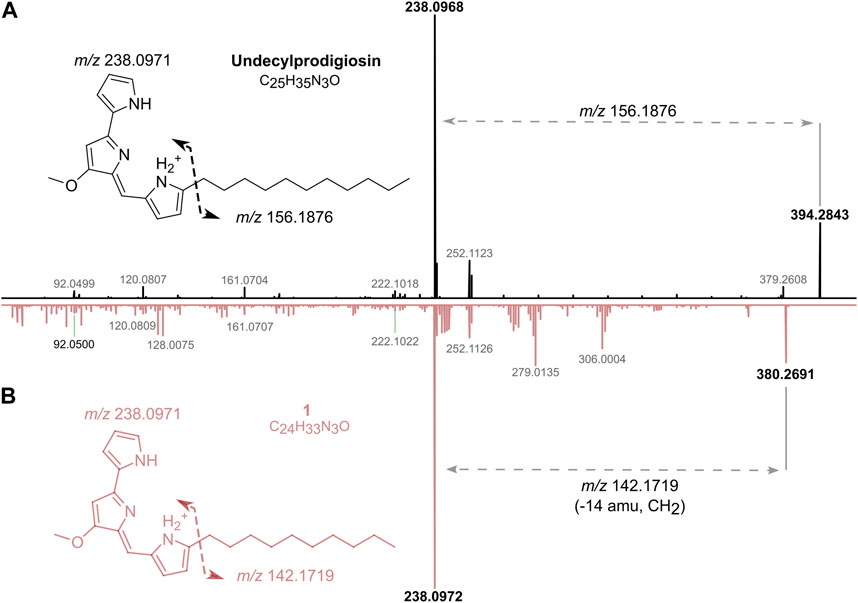
Figure 4. Structure elucidation of one by comparison of HRESIMS/MS spectra of undecylprodigiosin (in black) and one (in pink) (A). Proposed chemical structure of one and molecular formula (B).
Prodigiosins as product of seaweed-associated actinobacterial metabolism
The intricate web of symbiotic relationships in nature can shape entire ecosystems. In aquatic environments, symbiosis plays a key role in entire bionetworks, as, for example, in the coral-algae mutualism supporting a quarter of marine life (Tirichine and Piganeau, 2023). Seaweeds offer a suitable substratum for bacterial life and provide organic nutrients for multiplication and establishment of biofilms. In return, the host benefits from chemicals synthetized by the bacterial communities that can act as growth-promoting substances, quorum sensing signaling molecules or bioactive compounds responsible for their normal morphogenesis, growth and survival (Egan et al., 2013; Singh and Reddy, 2014). One distinctive trait of the red-pigmented family of the antibiotics prodigiosins is their algicidal activity (Zhang et al., 2016; Yang et al., 2017; Zhang et al., 2017; Zhang et al., 2020). In this work we show for the first time that a symbiotic Streptomyces strain, isolated from the tissues of a green macroalgae, is able to produce a wide range of bioactive prodigiosins. Prodigiosin and its family derivatives have been widely studied due to their biotechnological applications. In particular, this NPs family is efficient across several cancer types with low effects against non-malignant tissues, also offering interesting possibilities for combinatorial applications once they can act synergistically and/or additively with other drugs (Manderville, 2001; Montaner and Prez-Toms, 2003; Anwar et al., 2022). Different prodigiosin analogues, with minor modifications on their structures, have shown different modes of action and degrees of cytotoxicity (Kimata et al., 2018). Therefore, the discovery of a new prodigiosin molecule can provide additional insights into the structure-activity relationships within this natural products family. Even without certainty about the ecological role that these compounds play in this marine niche, we hypothesize that prodigiosins may be involved in protecting the host from algal overgrowth. In this symbiotic relationship, the seaweed provides a hospitable environment for Streptomyces to thrive, while the bacterial partner reciprocates by potentially deploying its potent prodigiosins arsenal. Such a natural defense mechanism could prevent excessive algal colonization that otherwise would compete with the seaweed host for vital resources such as sunlight and nutrients. However, more studies should be conducted to test this possibility.
Conclusion
In this work we have explored the bioactive secondary metabolism of the seaweed-associated Streptomyces violaceoruber CT-F61, isolated from the tissues of Codium tomentosum, a green macroalgae from the northern Portuguese shore. We describe the antimicrobial and anticancer properties of the metabolome of this strain, valuable for both human and animal health. We describe the discovery of 1, a new 10-carbon alkyl chain member of the prodigiosin family. To our knowledge, no prodigiosin or prodigiosin-like molecule has been described before from an actinomycete living is symbiosis with a seaweed, proving the value of this ecological niche as a source of novel NPs with biotechnological applications. Additional studies should be performed to allow a better understanding of the bioactivity and ecological role of 1.
Data availability statement
The datasets presented in this study can be found in online repositories. The names of the repository/repositories and accession number(s) can be found below: https://www.ncbi.nlm.nih.gov/genbank/, SFRH/BD/145646/2019 https://www.ncbi.nlm.nih.gov/genbank/, SAMN37527650 https://massive.ucsd.edu/ProteoSAFe/static/massive.jsp, MSV000093436 https://gnps.ucsd.edu/ProteoSAFe/libraries.jsp, CCMSLIB00012176068.
Ethics statement
Ethical approval was not required for the studies on humans in accordance with the local legislation and institutional requirements because only commercially available established cell lines were used.
Author contributions
MG: Conceptualization, Data curation, Investigation, Methodology, Writing–original draft, Writing–review and editing, Formal Analysis. SF: Data curation, Methodology, Writing–review and editing. TM: Methodology, Writing–review and editing, Data curation. RU: Resources, Supervision, Writing–review and editing, Funding acquisition. MC: Funding acquisition, Resources, Supervision, Writing–review and editing. PL: Conceptualization, Data curation, Funding acquisition, Investigation, Resources, Supervision, Validation, Writing–review and editing, Formal Analysis, Methodology, Writing–original draft.
Funding
The author(s) declare financial support was received for the research, authorship, and/or publication of this article. This project has received funding from the European Union’s Horizon 2020 research and innovation programme under grant agreement No 952374 and Ph.D. grant from Foundation for Science and Technology (FCT) SFRH/BD/145646/2019. The authors acknowledge the Strategic Funding UIDB/04423/2020 and UIDP/04423/2020, provided by FCT and the European Regional Development Fund.
Conflict of interest
The authors declare that the research was conducted in the absence of any commercial or financial relationships that could be construed as a potential conflict of interest.
The author(s) declared that they were an editorial board member of Frontiers, at the time of submission. This had no impact on the peer review process and the final decision.
Publisher’s note
All claims expressed in this article are solely those of the authors and do not necessarily represent those of their affiliated organizations, or those of the publisher, the editors and the reviewers. Any product that may be evaluated in this article, or claim that may be made by its manufacturer, is not guaranteed or endorsed by the publisher.
Supplementary material
The Supplementary Material for this article can be found online at: https://www.frontiersin.org/articles/10.3389/fphar.2024.1347485/full#supplementary-material
References
Abdelfattah, M. S., Elmallah, M. I., Ebrahim, H. Y., Almeer, R. S., Eltanany, R. M., and Abdel Moneim, A. E. (2019). Prodigiosins from a marine sponge-associated actinomycete attenuate HCl/ethanol-induced gastric lesion via antioxidant and anti-inflammatory mechanisms. PloS one 14 (6), e0216737. doi:10.1371/journal.pone.0216737
Allen, G. R., Reichelt, J. L., and Gray, P. P. (1983). Influence of environmental factors and medium composition on Vibrio gazogenes growth and prodigiosin production. Appl. Environ. Microbiol. 45 (6), 1727–1732. doi:10.1128/AEM.45.6.1727-1732.1983
Anwar, M. M., Albanese, C., Hamdy, N. M., and Sultan, A. S. (2022). Rise of the natural red pigment ‘prodigiosin’as an immunomodulator in cancer. Cancer Cell Int. 22 (1), 419. doi:10.1186/s12935-022-02815-4
Austin, D. A., and Moss, M. (1986). Numerical taxonomy of red-pigmented bacteria isolated from a lowland river, with the description of a new taxon, Rugamonas rubra gen. nov., sp. nov. Microbiology 132 (7), 1899–1909. doi:10.1099/00221287-132-7-1899
Bahrami, Y., Bouk, S., Kakaei, E., and Taheri, M. (2022). Natural products from Actinobacteria as a potential source of new therapies against colorectal cancer: a review. Front. Pharmacol. 13, 929161. doi:10.3389/fphar.2022.929161
Bankevich, A., Nurk, S., Antipov, D., Gurevich, A. A., Dvorkin, M., Kulikov, A. S., et al. (2012). SPAdes: a new genome assembly algorithm and its applications to single-cell sequencing. J. Comput. Biol. 19 (5), 455–477. doi:10.1089/cmb.2012.0021
Bennett, J., and Bentley, R. (2000). Seeing red: the story of prodigiosin. Adv. Appl. Microbiol. 47, 1–32. doi:10.1016/s0065-2164(00)47000-0
Berg, G. (2000). Diversity of antifungal and plant-associated Serratia plymuthica strains. J. Appl. Microbiol. 88 (6), 952–960. doi:10.1046/j.1365-2672.2000.01064.x
Blin, K., Shaw, S., Kloosterman, A. M., Charlop-Powers, Z., Van Wezel, G. P., Medema, M. H., et al. (2021). antiSMASH 6.0: improving cluster detection and comparison capabilities. Nucleic acids Res. 49 (W1), W29–W35. doi:10.1093/nar/gkab335
Boonlarppradab, C., Kauffman, C. A., Jensen, P. R., and Fenical, W. (2008). Marineosins A and B, cytotoxic spiroaminals from a marine-derived actinomycete. Org. Lett. 10 (24), 5505–5508. doi:10.1021/ol8020644
Castro, A. (1967). Antimalarial activity of prodigiosin. Nature 213 (5079), 903–904. doi:10.1038/213903a0
Church, N. A., and McKillip, J. L. (2021). Antibiotic resistance crisis: challenges and imperatives. Biologia 76 (5), 1535–1550. doi:10.1007/s11756-021-00697-x
Egan, S., Harder, T., Burke, C., Steinberg, P., Kjelleberg, S., and Thomas, T. (2013). The seaweed holobiont: understanding seaweed–bacteria interactions. FEMS Microbiol. Rev. 37 (3), 462–476. doi:10.1111/1574-6976.12011
Ehrenkaufer, G., Li, P., Stebbins, E. E., Kangussu-Marcolino, M. M., Debnath, A., White, C. V., et al. (2020). Identification of anisomycin, prodigiosin and obatoclax as compounds with broad-spectrum anti-parasitic activity. PLoS neglected Trop. Dis. 14 (3), e0008150. doi:10.1371/journal.pntd.0008150
El-Bondkly, A., El-Gendy, M., and Bassyouni, R. H. (2012). Overproduction and biological activity of prodigiosin-like pigments from recombinant fusant of endophytic marine Streptomyces species. Ant. Van Leeuwenhoek 102 (4), 719–734. doi:10.1007/s10482-012-9772-5
Ferlay, J., Colombet, M., Soerjomataram, I., Parkin, D. M., Piñeros, M., Znaor, A., et al. (2021). Cancer statistics for the year 2020: an overview. Int. J. cancer 149 (4), 778–789. doi:10.1002/ijc.33588
Gerber, N. N. (1969). Prodigiosin-like pigments from Actinomadura (nocardia) pelletieri and Actinomadura madurae. Appl. Microbiol. 18 (1), 1–3. doi:10.1128/am.18.1.1-3.1969
Gerber, N. N. (1975). A new prodiginine (prodigiosin-like) pigment from Streptomyces. Antimalarial activity of several prodiginines. J. Antibiotics 28 (3), 194–199. doi:10.7164/antibiotics.28.194
Gerber, N. N., and Gauthier, M. (1979). New prodigiosin-like pigment from Alteromonas rubra. Appl. Environ. Microbiol. 37 (6), 1176–1179. doi:10.1128/AEM.37.6.1176-1179.1979
Girão, M., Ribeiro, I., and Carvalho, M. F. (2022). “Actinobacteria from marine environments: a unique source of natural products,” in Natural products from actinomycetes (Springer), 1–45.
Girão, M., Ribeiro, I., Ribeiro, T., Azevedo, I. C., Pereira, F., Urbatzka, R., et al. (2019). Actinobacteria isolated from Laminaria ochroleuca: a source of new bioactive compounds. Front. Microbiol. 10, 683. doi:10.3389/fmicb.2019.00683
Gurevich, A., Mikheenko, A., Shlemov, A., Korobeynikov, A., Mohimani, H., and Pevzner, P. A. (2018). Increased diversity of peptidic natural products revealed by modification-tolerant database search of mass spectra. Nat. Microbiol. 3 (3), 319–327. doi:10.1038/s41564-017-0094-2
Halder, U., Banerjee, A., Biswas, R., Sharma, A., Pal, S., Adhikary, A., et al. (2020). Production of prodigiosin by a drug-resistant Serratia rubidaea HB01 isolated from sewage. Environ. Sustain. 3 (3), 279–287. doi:10.1007/s42398-020-00115-z
Harashima, K., Tsuchida, N., Tanaka, T., and Nagatsu, J. (1967). Prodigiosin-25 C: isolation and the chemical structure. Agric. Biol. Chem. 31 (4), 481–489. doi:10.1271/bbb1961.31.481
Harwood, C. (1978). Beneckea gazogenes sp. nov., a red, facultatively anaerobic, marine bacterium. Curr. Microbiol. 1 (4), 233–238. doi:10.1007/bf02602849
Hickey, M. E., and Lee, J. L. (2018). A comprehensive review of Vibrio (Listonella) anguillarum: ecology, pathology and prevention. Rev. Aquac. 10 (3), 585–610. doi:10.1111/raq.12188
Hu, D. X., Withall, D. M., Challis, G. L., and Thomson, R. J. (2016). Structure, chemical synthesis, and biosynthesis of prodiginine natural products. Chem. Rev. 116 (14), 7818–7853. doi:10.1021/acs.chemrev.6b00024
Islan, G. A., Rodenak-Kladniew, B., Noacco, N., Duran, N., and Castro, G. R. (2022). Prodigiosin: a promising biomolecule with many potential biomedical applications. Bioengineered 13 (6), 14227–14258. doi:10.1080/21655979.2022.2084498
Kawasaki, T., Sakurai, F., and Hayakawa, Y. (2008). A prodigiosin from the roseophilin producer Streptomyces griseoviridis. J. Nat. Prod. 71 (7), 1265–1267. doi:10.1021/np7007494
Kawauchi, K., Shibutani, K., Yagisawa, H., Kamata, H., Si, N., Anzai, H., et al. (1997). A possible immunosuppressant, cycloprodigiosin hydrochloride, obtained from Pseudoalteromonas denitrificans. Biochem. biophysical Res. Commun. 237 (3), 543–547. doi:10.1006/bbrc.1997.7186
Kimata, S., Matsuda, T., Kawasaki, T., and Hayakawa, Y. (2023). Prodigiosin R3, a new multicyclic prodigiosin formed by prodigiosin cyclization genes in the roseophilin producer. J. Antibiotics 76 (1), 14–19. doi:10.1038/s41429-022-00572-0
Kimata, S., Matsuda, T., Suizu, Y., and Hayakawa, Y. (2018). Prodigiosin R2, a new prodigiosin from the roseophilin producer Streptomyces griseoviridis 2464-S5. J. Antibiotics 71 (3), 393–396. doi:10.1038/s41429-017-0011-1
Klein, A. S., Domröse, A., Bongen, P., Brass, H. U., Classen, T., Loeschcke, A., et al. (2017). New prodigiosin derivatives obtained by mutasynthesis in Pseudomonas putida. ACS Synth. Biol. 6 (9), 1757–1765. doi:10.1021/acssynbio.7b00099
Komaki, H. (2022). Resolution of housekeeping gene sequences used in MLSA for the genus Streptomyces and reclassification of Streptomyces anthocyanicus and Streptomyces tricolor as heterotypic synonyms of Streptomyces violaceoruber. Int. J. Syst. Evol. Microbiol. 72 (5), 005370. doi:10.1099/ijsem.0.005370
Kumar, S., Stecher, G., Li, M., Knyaz, C., and Tamura, K. (2018). MEGA X: molecular evolutionary genetics analysis across computing platforms. Mol. Biol. Evol. 35 (6), 1547–1549. doi:10.1093/molbev/msy096
Lapenda, J., Silva, P., Vicalvi, M., Sena, K., and Nascimento, S. (2015). Antimicrobial activity of prodigiosin isolated from Serratia marcescens UFPEDA 398. World J. Microbiol. Biotechnol. 31, 399–406. doi:10.1007/s11274-014-1793-y
Lee, J. S., Kim, Y.-S., Park, S., Kim, J., Kang, S.-J., Lee, M.-H., et al. (2011). Exceptional production of both prodigiosin and cycloprodigiosin as major metabolic constituents by a novel marine bacterium, Zooshikella rubidus S1-1. Appl. Environ. Microbiol. 77 (14), 4967–4973. doi:10.1128/AEM.01986-10
Mabrok, M., Algammal, A. M., Sivaramasamy, E., Hetta, H. F., Atwah, B., Alghamdi, S., et al. (2023). Tenacibaculosis caused by Tenacibaculum maritimum: updated knowledge of this marine bacterial fish pathogen. Front. Cell. Infect. Microbiol. 12, 1068000. doi:10.3389/fcimb.2022.1068000
Manderville, R. (2001). Synthesis, proton-affinity and anti-cancer properties of the prodigiosin-group natural products. Curr. Med. Chemistry-Anti-Cancer Agents 1 (2), 195–218. doi:10.2174/1568011013354688
Miao, L., Wang, X., Jiang, W., Yang, S., Zhou, H., Zhai, Y., et al. (2013). Optimization of the culture condition for an antitumor bacterium Serratia proteamacula 657 and identification of the active compounds. World J. Microbiol. Biotechnol. 29 (5), 855–863. doi:10.1007/s11274-012-1240-x
Mohimani, H., Gurevich, A., Mikheenko, A., Garg, N., Nothias, L.-F., Ninomiya, A., et al. (2017). Dereplication of peptidic natural products through database search of mass spectra. Nat. Chem. Biol. 13 (1), 30–37. doi:10.1038/nchembio.2219
Mohimani, H., Gurevich, A., Shlemov, A., Mikheenko, A., Korobeynikov, A., Cao, L., et al. (2018). Dereplication of microbial metabolites through database search of mass spectra. Nat. Commun. 9 (1), 4035. doi:10.1038/s41467-018-06082-8
Montaner, B., and Prez-Toms, R. (2003). The prodigiosins: a new family of anticancer drugs. Curr. cancer drug targets 3 (1), 57–65. doi:10.2174/1568009033333772
Paul, T., Bandyopadhyay, T. K., Mondal, A., Tiwari, O. N., Muthuraj, M., and Bhunia, B. (2020). A comprehensive review on recent trends in production, purification, and applications of prodigiosin. Biomass Convers. Biorefinery 12, 1409–1431. doi:10.1007/s13399-020-00928-2
Pradeep, S., Josh, M. S., Balachandran, S., Devi, R. S., Sadasivam, R., Thirugnanam, P., et al. (2014). Achromobacter denitrificans SP1 produces pharmaceutically active 25C prodigiosin upon utilizing hazardous di (2-ethylhexyl) phthalate. Bioresour. Technol. 171, 482–486. doi:10.1016/j.biortech.2014.08.077
Schloss, P. D., Allen, H. K., Klimowicz, A. K., Mlot, C., Gross, J. A., Savengsuksa, S., et al. (2010). Psychrotrophic strain of Janthinobacterium lividum from a cold Alaskan soil produces prodigiosin. DNA Cell Biol. 29 (9), 533–541. doi:10.1089/dna.2010.1020
Semwal, A., Kumar, A., and Kumar, N. (2023). A review on pathogenicity of Aeromonas hydrophila and their mitigation through medicinal herbs in aquaculture. Heliyon 9, e14088. doi:10.1016/j.heliyon.2023.e14088
Singh, R. P., and Reddy, C. (2014). Seaweed–microbial interactions: key functions of seaweed-associated bacteria. FEMS Microbiol. Ecol. 88 (2), 213–230. doi:10.1111/1574-6941.12297
Song, Y., Liu, G., Li, J., Huang, H., Zhang, X., Zhang, H., et al. (2015). Cytotoxic and antibacterial angucycline-and prodigiosin-analogues from the deep-sea derived Streptomyces sp. SCSIO 11594. Mar. drugs 13 (3), 1304–1316. doi:10.3390/md13031304
Su, C., Xiang, Z., Liu, Y., Zhao, X., Sun, Y., Li, Z., et al. (2016). Analysis of the genomic sequences and metabolites of Serratia surfactantfaciens sp. nov. YD25T that simultaneously produces prodigiosin and serrawettin W2. BMC genomics 17 (1), 865–919. doi:10.1186/s12864-016-3171-7
Tanizawa, Y., Fujisawa, T., and Nakamura, Y. (2018). DFAST: a flexible prokaryotic genome annotation pipeline for faster genome publication. Bioinformatics 34 (6), 1037–1039. doi:10.1093/bioinformatics/btx713
Tirichine, L., and Piganeau, G. (2023). Editorial: algal symbiotic relationships in freshwater and marine environments. Front. Media SA 14, 1155759. doi:10.3389/fpls.2023.1155759
Tsao, S.-W., Rudd, B. A., He, X.-G., Chang, C.-J., and Floss, H. G. (1985). Identification of a red pigment from Streptomyces coelicolor A3 (2) as a mixture of prodigiosin derivatives. J. antibiotics 38 (1), 128–131. doi:10.7164/antibiotics.38.128
van Santen, J. A., Poynton, E. F., Iskakova, D., McMann, E., Alsup, T. A., Clark, T. N., et al. (2022). The Natural Products Atlas 2.0: a database of microbially-derived natural products. Nucleic Acids Res. 50 (D1), D1317–D1323. doi:10.1093/nar/gkab941
Vitale, G. A., Sciarretta, M., Palma Esposito, F., January, G. G., Giaccio, M., Bunk, B., et al. (2020). Genomics–metabolomics profiling disclosed marine Vibrio spartinae 3.6 as a producer of a new branched side chain prodigiosin. J. Nat. Prod. 83 (5), 1495–1504. doi:10.1021/acs.jnatprod.9b01159
Wang, M., Carver, J. J., Phelan, V. V., Sanchez, L. M., Garg, N., Peng, Y., et al. (2016). Sharing and community curation of mass spectrometry data with global natural products social molecular networking. Nat. Biotechnol. 34 (8), 828–837. doi:10.1038/nbt.3597
Wasserman, H., Rodgers, G., and Keith, D. (1966). The structure and synthesis of undecylprodigiosin. A prodigiosin analogue from Streptomyces. Chem. Commun. Lond. (22), 825–826. doi:10.1039/c19660000825
Weisburg, W. G., Barns, S. M., Pelletier, D. A., and Lane, D. J. (1991). 16S ribosomal DNA amplification for phylogenetic study. J. Bacteriol. 173 (2), 697–703. doi:10.1128/jb.173.2.697-703.1991
Williams, R. P. (1973). Biosynthesis of prodigiosin, a secondary metabolite of Serratia marcescens. Appl. Microbiol. 25 (3), 396–402. doi:10.1128/am.25.3.396-402.1973
Wood, D. E., Lu, J., and Langmead, B. (2019). Improved metagenomic analysis with Kraken 2. Genome Biol. 20, 257–313. doi:10.1186/s13059-019-1891-0
Yang, K., Chen, Q., Zhang, D., Zhang, H., Lei, X., Chen, Z., et al. (2017). The algicidal mechanism of prodigiosin from Hahella sp. KA22 against Microcystis aeruginosa. Sci. Rep. 7 (1), 7750. doi:10.1038/s41598-017-08132-5
Yoon, S.-H., Ha, S.-M., Kwon, S., Lim, J., Kim, Y., Seo, H., et al. (2017). Introducing EzBioCloud: a taxonomically united database of 16S rRNA gene sequences and whole-genome assemblies. Int. J. Syst. Evol. Microbiol. 67 (5), 1613–1617. doi:10.1099/ijsem.0.001755
Zhang, H., Peng, Y., Zhang, S., Cai, G., Li, Y., Yang, X., et al. (2016). Algicidal effects of prodigiosin on the harmful algae Phaeocystis globosa. Front. Microbiol. 7, 602. doi:10.3389/fmicb.2016.00602
Zhang, H., Wang, H., Zheng, W., Yao, Z., Peng, Y., Zhang, S., et al. (2017). Toxic effects of prodigiosin secreted by Hahella sp. KA22 on harmful alga Phaeocystis globosa. Front. Microbiol. 8, 999. doi:10.3389/fmicb.2017.00999
Zhang, S., Zheng, W., and Wang, H. (2020). Physiological response and morphological changes of Heterosigma akashiwo to an algicidal compound prodigiosin. J. Hazard. Mater. 385, 121530. doi:10.1016/j.jhazmat.2019.121530
Keywords: decylprodigiosin, Streptomyces, seaweed, bioactivity, actinomycete
Citation: Girão M, Freitas S, Martins TP, Urbatzka R, Carvalho MF and Leão PN (2024) Decylprodigiosin: a new member of the prodigiosin family isolated from a seaweed-associated Streptomyces. Front. Pharmacol. 15:1347485. doi: 10.3389/fphar.2024.1347485
Received: 30 November 2023; Accepted: 11 March 2024;
Published: 21 March 2024.
Edited by:
Patrícia Mendonça Rijo, Lusofona University, PortugalReviewed by:
Manigandan Venkatesan, The University of Texas Health Science Center at San Antonio, United StatesXuewei Pan, Jiangnan University, China
Copyright © 2024 Girão, Freitas, Martins, Urbatzka, Carvalho and Leão. This is an open-access article distributed under the terms of the Creative Commons Attribution License (CC BY). The use, distribution or reproduction in other forums is permitted, provided the original author(s) and the copyright owner(s) are credited and that the original publication in this journal is cited, in accordance with accepted academic practice. No use, distribution or reproduction is permitted which does not comply with these terms.
*Correspondence: Mariana Girão, bWFyaWFuYS5tYXJ0aW5zQGNpaW1hci51cC5wdA==