- 1Shandong University of Traditional Chinese Medicine, Jinan, China
- 2Department of Anesthesiology, Affiliated Hospital of Shandong University of Traditional Chinese Medicine, Jinan, China
Neostigmine is a clinical cholinesterase inhibitor, that is, commonly used to enhance the function of the cholinergic neuromuscular junction. Recent studies have shown that neostigmine regulates the immune-inflammatory response through the cholinergic anti-inflammatory pathway, affecting perioperative neurocognitive function. This article reviews the relevant research evidence over the past 20 years, intending to provide new perspectives and strategies for the clinical application of neostigmine.
1 Introduction
Neostigmine has become a classic anticholinesterase drug since it was introduced in the 1930s. It inhibits acetylcholinesterase (AChE) activity, produces cholinergic effects, and enhances the transmission function of the neuromuscular junction, helping increase skeletal muscle strength. It is commonly used clinically to antagonize the residual muscle relaxation effect of non-depolarizing muscle relaxants following anesthesia surgery, and is also used to treat myasthenia gravis, postoperative functional flatulence, and urinary retention, etc., (Pohanka, 2012). In recent years, applications to modulate immune-inflammatory response through the cholinergic anti-inflammatory pathway (CAP) and affect neurocognitive function have been reported in turn, marking new progress in the clinical applications of this drug. This paper conducts a review in order to provide new evidence for the clinical application of neostigmine (The methodology of this mini-review is shown in Supplementary Material).
2 Pharmacological overview: cholinergic effect
Neostigmine is a carbamate derivative, belonging to the quaternary ammonium group, and is clinically used as a parasympathetic agent and cholinesterase inhibitor (ChE-Is). Its chemical structure (Kim et al., 2013) is shown in Supplementary Material. The positively charged nitrogen in the neostigmine molecule electrostatically binds to the peripheral anionic site of AChE, whereas the carbamate group in the molecule covalently binds to the serine residue in the catalytic site of the enzyme. Through serine carbamylation, the activity of AChE is reversibly inhibited. As a result, the half-life of ACh in cholinergic synapses is prolonged and the activation of nicotinic/muscarinic cholinergic receptors (nAChRs/mAChRs) is increased, ultimately producing cholinergic effects (Eldufani and Blaise, 2019). In addition, neostigmine-induced inhibition of voltage-gated potassium channels can prolong action potentials in motor neurons and thereby increase ACh release at the neuromuscular junction to increase muscle fiber contraction. Neostigmine can also directly activate postsynaptic nAChRs at the motor endplate (Liu et al., 2022a). The commonly used routes of administration are intravenous and intramuscular injection; neostigmine is difficult to be absorbed orally by the gastrointestinal tract. Due to its structural properties (quaternary amine), the drug has difficulty crossing the blood-brain barrier (BBB) and entering the central nervous system (CNS) (Luo et al., 2018).
3 Conventional application: classical theater
3.1 Muscle strength: increasing
Increasing skeletal muscle strength is the classic application of neostigmine. Neostigmine reduces the activity of AChE, thereby prolonging the half-life of ACh at the neuromuscular junction. As a result, the increased ACh-induced activation of muscle AChRs increases muscle strength. These effects make it an important alternative drug for post-anesthesia residual muscle relaxation (non-depolarizing muscle relaxation) (Fuchs-Buder et al., 2023), myasthenia gravis (Feibel, 2021), acute colonic pseudo-obstruction (Adiamah et al., 2017), constipation (Kapoor, 2008), urinary retention (Cao et al., 2022), neurotoxic snake bites (Anil et al., 2010) and other conditions or diseases that cause muscular weakness due to dysfunction of the cholinergic muscle junction.
However, since the cholinergic effect of neostigmine is limited by the amount of synaptic ACh release, it has a ceiling effect on the enhancement of muscle strength (Blobner et al., 2020). If neostigmine is administered when the neuromuscular function is normal or has fully recovered, abnormal muscle weakness may be observed due to desensitization of nAChRs caused by excessive ACh accumulation at synapses (Naguib and Kopman, 2018). Therefore, neuromuscular function monitoring is important in order to confirm the proper timing of administration (Kopman and Naguib, 2015; Phillips and Stewart, 2018). Neostigmine activates both nAChRs and mAChRs at the same time, thus it must be combined with anticholinergic drugs (such as atropine, etc.) to avoid its muscarinic side effects (arrhythmia, increased secretion, nausea, or vomiting, etc.) under different application purposes (Tajaate et al., 2018).
3.2 Analgesia: adjuvants
Ach is one of multiple neurotransmitters involved in regulating the production and transmission of nociceptive signals in the spinal cord. Physiological (trauma, pain, etc.) or pharmacological stimulation (activation of α2-adrenergic receptors in the spinal cord or opioid receptors in the brain stem) contribute to release of ACh. Cholinergic receptors are present in the superficial and deep dorsal horn of the spinal cord, and are involved in transmission and modulation of nociceptive signals (Eldufani and Blaise, 2019). Clinical application of neostigmine has been widely reported for perioperative adjunctive analgesia (Swain et al., 2017; Prabhakar et al., 2019). It helps to enhance the analgesic effect, prolong the analgesic time, and reduce the consumption of analgesics such as morphine, ketamine and clonidine. The drug is typically injected intrathecally or epidurally (Habib and Gan, 2006), with less use of peripheral blockade. It inhibits the activity of AChE, increasing the concentration of endogenous ACh in spinal cord synapses. Then, through mAChRs, the transmission of nociceptive signals in the spinal dorsal horn are inhibited (Duttaroy et al., 2002; Lauretti, 2015), and nociceptive signaling of various afferent fibers (such as Aβ, C and Aδ fibers) (Buerkle et al., 1998) are modulated, thus inhibiting central sensitization, and increasing the pain threshold (Naguib and Yaksh, 1994). In addition, the release of NO in the spinal cord is promoted, producing analgesic effects (Prabhakar et al., 2019).
However, neostigmine has dose-related side effects when used for adjuvant analgesia, such as nausea and vomiting, which to some extent limit its application (Prabhakar et al., 2019). Further research is needed to optimize the medication regimen.
4 New applications: new arena for label-off usage
4.1 Immune and inflammatory regulation
For a long time, immune and inflammation regulation have not been considered to be the main effects of acetylcholinesterase inhibitors such as neostigmine. However, recent evidence has shed new light on this issue.
The cholinergic anti-inflammatory pathway (CAP) is an important endogenous immunomodulatory mechanism in the body (Borovikova et al., 2000; Hoover, 2017). The local immune-inflammatory signal transmitted to the CNS nucleus tractus solitarius via the vagal afferent nerve is the driving factor of CAP. ACh released from vagal efferent nerve fibers in the inflammatory reflex pathway activates α7nAChRs expressed on immune cells (such as macrophages). Subsequently, the synthesis and release of pro-inflammatory cytokines/mediators such as tumor necrosis factor-α (TNF-α), interleukin-1β (IL-1β), interleukin-6 (IL-6), and high mobility group protein box 1 (HMGB1) are inhibited through PI3K/Akt, JAK2/STAT3, NF-κB, Nrf2/HO-1 and other pathways. Finally, the tissue damage caused by inflammation and oxidative stress is improved (Fodale and Santamaria, 2008). CAP is mainly activated by central cholinergic transmission, electrical stimulation of the vagus nerve, or cholinergic agonists. AChE-Is inhibits AChE to increase the level and duration of ACh, activate α7nAChR and amplify the activity of the CAP system to exert anti-inflammatory effects, thus improving inflammatory response damage (Nizri et al., 2006). Therefore, in terms of pharmacological mechanism, it has the potential to become an immune-inflammatory regulatory drug (Pohanka, 2014). See Figure 1.
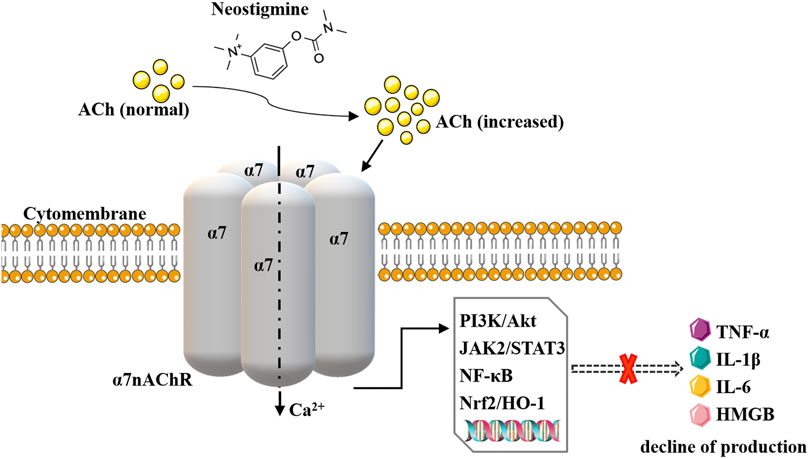
FIGURE 1. Schematic representation of neostigmine acting on CAP. Neostigmine increases the effect of ACh on α7nAChRs and amplifies CAP activity, reducing the levels of pro-inflammatory cytokines such as TNF-α, IL-1β, IL-6, and HMGB1 through PI3K/Akt, NF-kappaB, JAK2/STAT3 and other pathways.
A series of studies over the last 20 years has reported the immune-inflammatory regulatory effects of neostigmine (Miceli and Jacobson, 2003; Akinci et al., 2005; Pollak et al., 2005; Freeling et al., 2008; Hofer et al., 2008; Liu et al., 2012; Sun et al., 2012; Kalb et al., 2013; Li et al., 2014; Schneider et al., 2014; Steinebrunner et al., 2014; Zhou et al., 2014; Qian et al., 2015; Kanashiro et al., 2016; Xu et al., 2016; Abdel-Salam et al., 2018; Herman et al., 2018; Bitzinger et al., 2019; Antunes et al., 2020a; Antunes et al., 2020b; Lyu et al., 2021; El-Tamalawy et al., 2022). Its peripheral administration increases the effect of ACh on α7nAChRs and amplifies CAP activity. Through PI3K/Akt (Antunes et al., 2020a), NF-kappaB (Hofer et al., 2008; Antunes et al., 2020a), JAK2/STAT3 (Xu et al., 2016) and other pathways, it reduces the levels of pro-inflammatory cytokines such as TNF-α, IL-1β, IL-6, and HMGB1 and upregulates activity of anti-inflammatory factors such as IL-10 (Paparini et al., 2015). It can regulate the migration, recruitment, and infiltration of immune cells, inhibiting inflammatory response, oxidative stress injury and apoptosis (Liu et al., 2012). As detailed in Table 1, management with neostigmine (either prophylactic or delayed administration) triggers immunomodulatory effects in a variety of animal models (including arthritis, pancreatitis, colitis, sepsis and organ injury or failure, etc.). Some studies (Liu et al., 2012; Sun et al., 2012; Li et al., 2014; Zhou et al., 2014; Qian et al., 2015; Xu et al., 2016; Lyu et al., 2021) have found that the combination of anisodamine and neostigmine produces good anti-inflammatory effects (better than that of neostigmine alone). The mechanisms are as follows: anisodamine blocks mAChRs to prevent its non-specific parasympathetic side effects (Li et al., 2014), and indirectly enables more endogenous ACh to bind to α7nAChRs (Poupko et al., 2007; Liu et al., 2009; Zhao et al., 2011), ultimately enhancing the effect of CAP (Liu et al., 2009; Xu et al., 2011; Zhao et al., 2011; Zhang et al., 2023).
Contrary to the findings above, however, some studies report that neostigmine does not modulate immune and inflammatory responses (Tyagi et al., 2010; Kox et al., 2011; Leib et al., 2011; Zhang et al., 2016). However, the reasons for the ineffectiveness have not been fully elucidated. Meanwhile, studies have reported that while neostigmine plays an immune-inflammatory regulatory role through CAP (activation of α7nAChRs), parasympathetic side effects may be observed (due to activation of mAChRs) (Akinci et al., 2005), requiring combination with anticholinergic drugs (e.g., anisodamine, the collaborator mentioned above) or dosage control, thus its application is somewhat limited. In addition, the above anti-inflammatory data on neostigmine are mostly nonclinical studies, and only one study reported its clinical anti-inflammatory effect in septic shock patients. Clinical evidence that neostigmine affects other inflammatory diseases is scarce, which limits the external validity and clinical anti-inflammatory application promotion. More large-sample and multi-center clinical studies are required to provide evidence for the regulatory effect of neostigmine on inflammation.
4.2 Perioperative neurocognitive protection
Perioperative neurocognitive disorders (PND) refer to the decline and deterioration of abilities in multiple cognitive domains during the perioperative period, including preoperative cognitive dysfunction, postoperative delirium (POD), and postoperative cognitive dysfunction (POCD). (Evered et al., 2018). The pathological role of CNS inflammatory response caused by anesthesia and surgery in PND has been widely reported (Liu et al., 2022b). Anesthesia and surgery (tissue damage or pathogenic attack) induce activation of the body’s immune-inflammatory system, resulting in a rapid increase in levels of pro-inflammatory cytokines (e.g., IL-1β, TNF-α, and IL-6) in a short period of time, causing peripheral local inflammation. Anesthesia and surgery can also increase the permeability of the BBB (Glumac et al., 2019). Inflammatory factors enter the CNS from the periphery through the BBB (Banks et al., 1995), and at the same time, peripheral inflammatory signals are transmitted to the brain through the afferent nerve. Then immune-related cells (such as astrocytes and microglia) in the hippocampus and other regions of the brain are activated, and pro-inflammatory cytokines such as IL-1β are released, thus causing a central immune-inflammatory response (Needham et al., 2017). The whole process interferes with the activity of neurons and synaptic transmission in the cerebral cortex or hippocampal region of the brain, ultimately affecting perioperative cognitive function (Cibelli et al., 2010; Fidalgo et al., 2011; Liu and Yin, 2018; Liu et al., 2022b). Neostigmine, as the most typical cholinesterase inhibitor in used general anesthesia, is often used to antagonize postoperative residual neuromuscular blockade and is occasionally used as an adjunct to perioperative analgesia. At the same time, cholinergic system activity and CAP activity can be increased through the cholinergic effect of neostigmine. Therefore, whether neostigmine could improve CNS immune-inflammation impairment by increasing cholinergic system activity and through mechanisms such as CAP, and thus be an exposure factor for improving perioperative cognitive function, is a clinical question worth investigating.
4.2.1 Neostigmine on central anti-inflammation and neuro-protection: nonclinical studies
Animal experiments have shown that neostigmine inhibits AChE, increases cholinergic system activity and neurotransmission (Pollak et al., 2005), and then activates CAP, regulates the activation level of CNS immune cells such as microglia and astrocytes, and reduces the expression of pro-inflammatory cytokines such as IL-1β (Kalb et al., 2013), thereby attenuating or delaying the inflammatory response, oxidative stress and neuronal degeneration in the cerebral cortex and hippocampus of the surgical stress model rats (Abdel-Salam et al., 2018), maintaining synaptic plasticity (Tozzi et al., 2015), and finally exerting central immune-inflammatory response regulation and neuroprotective effects (Antunes et al., 2020b). However, the data above only reported the central anti-inflammatory and neuroprotective effects of neostigmine, without quantitative evaluation of neurocognitive function changes in animals.
4.2.2 Neostigmine on perioperative neurocognitive function: clinical studies
An earlier study by Prohovnik et al. (1997) found that intravenous administration of neostigmine (11 μg/kg) exhibited a reversal effect on scopolamine-induced memory deficits in healthy subjects (no difference from the effect of 22 μg/kg of physostigmine, which can be transferred into the CNS). One case report showed that intravenous neostigmine improved patients’ delirium symptoms while treating postoperative acute colonic pseudo-obstruction (Lankarani-Fard and Castle, 2006). Zhu et al. found that the incidence of early postoperative cognitive decline in elderly patients undergoing radical resection of gastrointestinal cancer following intravenous injection of 0.04 mg/kg neostigmine in PACU was significantly lower than in the control group (Zhu et al., 2020). Cozanitis et al. showed that the postoperative Wechsler Memory Scale scores of elderly cataract surgery patients in the neostigmine group were similar to those in the galantamine group (a type of AChE-Is, acting on the CNS, commonly used in neurodegenerative diseases such as Alzheimer’s disease and Parkinson’s disease) (Cozanitis et al., 2012). However, Batistaki et al. came to the opposite conclusion: no significant difference in POCD incidence in middle-aged and elderly surgical patients in the neostigmine group compared with sugammadex (Batistaki et al., 2017); and Liu et al. indicated that application of neostigmine in patients undergoing colon cancer surgery did not reduce the incidence of POD (Liu et al., 2022a). The reasons are speculated as follows: i) Neostigmine is mainly used postoperatively, with rapid half-life elimination and short duration of action (Carron et al., 2016), thus making it difficult to account for the influence of anesthetic surgical factors on the CNS in a short period of time; ii) Neostigmine is often combined with anticholinergic drugs such as atropine to avoid its non-specific parasympathetic adverse reactions, while anticholinergic effects antagonize the cholinergic and CAP effects of neostigmine (Zwart and Vijverberg, 1997; Gonzalez-Rubio et al., 2006). The clinical data above show the potential of neostigmine to improve perioperative neurocognitive function. However, there was heterogeneity in the type of surgery involved in these studies, and the quantitative assessment tools of cognitive function were not consistent. At the same time, some studies did not provide sample size estimation basis. This limited heterogeneity control may therefore introduce bias, and the results need to be viewed with caution.
Many issues need to be explored before the effects of neostigmine on central anti-inflammatory and neuroprotective effects are confirmed. It is generally accepted that drugs need to be present in the central compartment to have CNS effects (Pavlov et al., 2009; Noori et al., 2012; Herman et al., 2019). As a quaternary ammonium compound, however, neostigmine does not readily cross the BBB and stays in the peripheral compartment when delivered via non-central routes of administration. Thus, it is difficult to understand how peripheral administration of neostigmine modulates inflammation in the CNS. Through literature review and collation, the mechanisms are speculated as follows. i) Increased BBB permeability: Anesthesia and surgery, inflammatory response, stress, and other factors increase BBB permeability (Friedman et al., 1996; Beck et al., 2003; Danielski et al., 2018; Glumac et al., 2019). Peripheral neostigmine may enter the CNS through the damaged BBB to function (Zhu et al., 2020). However, the results of current studies on the effect of peripheral administration of neostigmine on central AChE activity under certain specific conditions are controversial (Pollak et al., 2005; Kalb et al., 2013; Zhang et al., 2016; Abdel-Salam et al., 2018; Dubrovskii et al., 2018; Antunes et al., 2020b). Therefore, the available evidence is still inconclusive about whether neostigmine can directly play a corresponding role through the BBB into the CNS in some special cases. ii) Improving peripheral inflammation: Peripheral inflammatory signals are transmitted to the CNS via vagal afferent nerves, while peripheral pro-inflammatory cytokines reach the brain parenchyma through the BBB (due to increased BBB permeability or active transport mechanisms). Both the nervous signals and humoral factors to some extent directly or indirectly cause CNS immune-inflammatory responses (Banks et al., 1995; Needham et al., 2017; Noll et al., 2017). Neostigmine reduces the peripheral inflammatory response level and expression of pro-inflammatory cytokines. Then, the transmission of peripheral inflammatory signals to the central system is attenuated (Herman et al., 2017; Herman et al., 2018), and the CNS is less affected by peripheral pro-inflammatory cytokines passing through the BBB (peripheral pro-inflammatory cytokines need to be enriched to a critical level in order to affect the CNS (Antunes et al., 2020b)), thus reducing the central immune-inflammatory response (Pollak et al., 2005; Steinman, 2010; Kalb et al., 2013) (The relationship between neostigmine and neuroprotective effects is shown in Supplementary Material). iii) Other potentially significant factors: neostigmine may be involved in changes in cognitive function by modulating the peripheral cholinergic cerebrovascular circulation (Kocsis et al., 2014). This view is based on the functional basis of high energy and oxygen consumption in the brain. Contrary to most people’s perception, carbamate compounds such as neostigmine may exert CNS effects in certain circumstances - an important point that should not be ignored in the evaluation of the effects of neostigmine!
5 Conclusion and perspective
Recent studies have shown that the classic cholinergic effect of neostigmine may play a new role in immune-inflammatory regulation and perioperative neurocognitive protection through CAP. However, the function, downstream regulatory targets and transduction pathways of CAP are still not completely clear. Current studies on neostigmine in immune-inflammation regulation and perioperative neurocognitive protection are mostly animal experiments, and more clinical studies are needed for verification. Considering the non-specific parasympathetic side effects of neostigmine under different application purposes, further research on the reasonable medication regimen is required. It is believed that with deep follow-up research, new and comprehensive neostigmine usage strategies will be observed.
Author contributions
SS conceived, designed, and conducted the study, analyzed the data, and wrote the manuscript. XZ, LX, and FW helped design the study and review the manuscript. DZ, LS, and HL helped conceive, design, and conduct the study and analyze the data. FS helped conceive, design, and conduct the study, analyze the data, and write the manuscript. All authors contributed to the article and approved the submitted version.
Funding
This study was supported by the Ji’nan Science and Technology Innovation Development Plan (202134068) and Ji’nan “20 New universities” scientific research leader studio project (202228124).
Acknowledgments
We thank Zhenzhen Gao (Guangdong University of Foreign Studies, Interpreting Studies) for polishing up the language.
Conflict of interest
The authors declare that the research was conducted in the absence of any commercial or financial relationships that could be construed as a potential conflict of interest.
Publisher’s note
All claims expressed in this article are solely those of the authors and do not necessarily represent those of their affiliated organizations, or those of the publisher, the editors and the reviewers. Any product that may be evaluated in this article, or claim that may be made by its manufacturer, is not guaranteed or endorsed by the publisher.
Supplementary material
The Supplementary Material for this article can be found online at: https://www.frontiersin.org/articles/10.3389/fphar.2023.1227496/full#supplementary-material
References
Abdel-Salam, O., Youness, E. R., Esmail, R., Mohammed, N. A., Khadrawy, Y. A., Sleem, A. A., et al. (2018). Protection by neostigmine and atropine against brain and liver injury induced by acute malathion exposure. J. Nanosci. Nanotechnol. 18, 510–521. doi:10.1166/jnn.2018.13933
Adiamah, A., Johnson, S., Ho, A., and Orbell, J. (2017). Neostigmine and glycopyrronium: A potential safe alternative for patients with pseudo-obstruction without access to conventional methods of decompression. Bmj Case Rep. 2017, bcr2017221249. doi:10.1136/bcr-2017-221249
Akinci, S. B., Ulu, N., Yondem, O. Z., Firat, P., Guc, M. O., Kanbak, M., et al. (2005). Effect of neostigmine on organ injury in murine endotoxemia: Missing facts about the cholinergic antiinflammatory pathway. World J. Surg. 29, 1483–1489. doi:10.1007/s00268-005-0073-2
Anil, A., Singh, S., Bhalla, A., Sharma, N., Agarwal, R., and Simpson, I. D. (2010). Role of neostigmine and polyvalent antivenom in Indian common krait (Bungarus caeruleus) bite. J. Infect. Public Health 3, 83–87. doi:10.1016/j.jiph.2010.01.002
Antunes, G. L., Silveira, J. S., Kaiber, D. B., Luft, C., Da, C. M., Marques, E. P., et al. (2020a). Cholinergic anti-inflammatory pathway confers airway protection against oxidative damage and attenuates inflammation in an allergic asthma model. J. Cell. Physiol. 235, 1838–1849. doi:10.1002/jcp.29101
Antunes, G. L., Silveira, J. S., Kaiber, D. B., Luft, C., Dos, S. T., Marques, E. P., et al. (2020b). Neostigmine treatment induces neuroprotection against oxidative stress in cerebral cortex of asthmatic mice. Metab. Brain Dis. 35, 765–774. doi:10.1007/s11011-020-00558-7
Banks, W. A., Kastin, A. J., and Broadwell, R. D. (1995). Passage of cytokines across the blood-brain barrier. Neuroimmunomodulation 2, 241–248. doi:10.1159/000097202
Batistaki, C., Riga, M., Zafeiropoulou, F., Lyrakos, G., Kostopanagiotou, G., and Matsota, P. (2017). Effect of sugammadex versus neostigmine/atropine combination on postoperative cognitive dysfunction after elective surgery. Anaesth. Intensive Care 45, 581–588. doi:10.1177/0310057X1704500508
Beck, K. D., Brennan, F. X., Moldow, R. L., Ottenweller, J. E., Zhu, G., and Servatius, R. J. (2003). Stress interacts with peripheral cholinesterase inhibitors to cause central nervous system effects. Life Sci. 73, 41–51. doi:10.1016/s0024-3205(03)00255-8
Bitzinger, D. I., Gruber, M., Tummler, S., Malsy, M., Seyfried, T., Weber, F., et al. (2019). In vivo effects of neostigmine and physostigmine on neutrophil functions and evaluation of acetylcholinesterase and butyrylcholinesterase as inflammatory markers during experimental sepsis in rats. Mediat. Inflamm. 2019, 8274903. doi:10.1155/2019/8274903
Blobner, M., Hunter, J. M., Meistelman, C., Hoeft, A., Hollmann, M. W., Kirmeier, E., et al. (2020). Use of a train-of-four ratio of 0.95 versus 0.9 for tracheal extubation: An exploratory analysis of POPULAR data. Br. J. Anaesth. 124, 63–72. doi:10.1016/j.bja.2019.08.023
Borovikova, L. V., Ivanova, S., Zhang, M., Yang, H., Botchkina, G. I., Watkins, L. R., et al. (2000). Vagus nerve stimulation attenuates the systemic inflammatory response to endotoxin. Nature 405, 458–462. doi:10.1038/35013070
Buerkle, H., Boschin, M., Marcus, M. A., Brodner, G., Wusten, R., and Van Aken, H. (1998). Central and peripheral analgesia mediated by the acetylcholinesterase-inhibitor neostigmine in the rat inflamed knee joint model. Anesth. Analg. 86, 1027–1032. doi:10.1097/00000539-199805000-00023
Cao, M., Wu, X., and Xu, J. (2022). A systematic review and meta-analysis of neostigmine for urinary retention after surgeries. Transl. Androl. Urol. 11, 190–201. doi:10.21037/tau-22-16
Carron, M., Toniolo, A., and Ori, C. (2016). Prolonged cholinergic effects after the reversal of neuromuscular blockade with neostigmine. J. Clin. Anesth. 28, 85. doi:10.1016/j.jclinane.2015.06.017
Cibelli, M., Fidalgo, A. R., Terrando, N., Ma, D., Monaco, C., Feldmann, M., et al. (2010). Role of interleukin-1beta in postoperative cognitive dysfunction. Ann. Neurol. 68, 360–368. doi:10.1002/ana.22082
Cozanitis, D., Keinonen, M., and Maunuksela, E. L. (2012). Effect on cognition of galanthamine administered for neuromuscular block reversal in octogenarians undergoing cataract surgery. Anaesthesiol. Intensive Ther. 44, 76–80.
Danielski, L. G., Giustina, A. D., Badawy, M., Barichello, T., Quevedo, J., Dal-Pizzol, F., et al. (2018). Brain barrier breakdown as a cause and consequence of neuroinflammation in sepsis. Mol. Neurobiol. 55, 1045–1053. doi:10.1007/s12035-016-0356-7
Dubrovskii, V. N., Shalabodov, A. D., and Belkin, A. V. (2018). Effects of neostigmine and physostigmine on activity of Na(+),K(+)-ATPase in various subdivisions of rat brain. Bull. Exp. Biol. Med. 166, 50–53. doi:10.1007/s10517-018-4287-3
Duttaroy, A., Gomeza, J., Gan, J. W., Siddiqui, N., Basile, A. S., Harman, W. D., et al. (2002). Evaluation of muscarinic agonist-induced analgesia in muscarinic acetylcholine receptor knockout mice. Mol. Pharmacol. 62, 1084–1093. doi:10.1124/mol.62.5.1084
El-Tamalawy, M. M., Soliman, M. M., Omara, A. F., Rashad, A., Ibrahim, O. M., and El-Shishtawy, M. M. (2022). Efficacy and safety of neostigmine adjunctive therapy in patients with sepsis or septic shock: A randomized controlled trial. Front. Pharmacol. 13, 855764. doi:10.3389/fphar.2022.855764
Eldufani, J., and Blaise, G. (2019). The role of acetylcholinesterase inhibitors such as neostigmine and rivastigmine on chronic pain and cognitive function in aging: A review of recent clinical applications. Alzheimers Dement. (N Y) 5, 175–183. doi:10.1016/j.trci.2019.03.004
Evered, L., Silbert, B., Knopman, D. S., Scott, D. A., DeKosky, S. T., Rasmussen, L. S., et al. (2018). Recommendations for the nomenclature of cognitive change associated with anaesthesia and surgery-2018. Br. J. Anaesth. 121, 1005–1012. doi:10.1016/j.bja.2017.11.087
Feibel, R. M. (2021). Henry R. Viets, MD, and the history of myasthenia gravis. Neurology 96, 322–326. doi:10.1212/WNL.0000000000011239
Fidalgo, A. R., Cibelli, M., White, J. P., Nagy, I., Maze, M., and Ma, D. (2011). Systemic inflammation enhances surgery-induced cognitive dysfunction in mice. Neurosci. Lett. 498, 63–66. doi:10.1016/j.neulet.2011.04.063
Fodale, V., and Santamaria, L. B. (2008). Cholinesterase inhibitors improve survival in experimental sepsis: A new way to activate the cholinergic anti-inflammatory pathway. Crit. Care Med. 36, 622–623. doi:10.1097/CCM.0B013E31816297CE
Freeling, J., Wattier, K., LaCroix, C., and Li, Y. F. (2008). Neostigmine and pilocarpine attenuated tumour necrosis factor alpha expression and cardiac hypertrophy in the heart with pressure overload. Exp. Physiol. 93, 75–82. doi:10.1113/expphysiol.2007.039784
Friedman, A., Kaufer, D., Shemer, J., Hendler, I., Soreq, H., and Tur-Kaspa, I. (1996). Pyridostigmine brain penetration under stress enhances neuronal excitability and induces early immediate transcriptional response. Nat. Med. 2, 1382–1385. doi:10.1038/nm1296-1382
Fuchs-Buder, T., Romero, C. S., Lewald, H., Lamperti, M., Afshari, A., Hristovska, A. M., et al. (2023). Peri-operative management of neuromuscular blockade: A guideline from the European society of anaesthesiology and intensive care. Eur. J. Anaesthesiol. 40, 82–94. doi:10.1097/EJA.0000000000001769
Glumac, S., Kardum, G., and Karanovic, N. (2019). Postoperative cognitive decline after cardiac surgery: A narrative review of current knowledge in 2019. Med. Sci. Monit. 25, 3262–3270. doi:10.12659/MSM.914435
Gonzalez-Rubio, J. M., Garcia, D. D. A., Egea, J., Olivares, R., Rojo, J., Gandia, L., et al. (2006). Blockade of nicotinic receptors of bovine adrenal chromaffin cells by nanomolar concentrations of atropine. Eur. J. Pharmacol. 535, 13–24. doi:10.1016/j.ejphar.2006.01.057
Habib, A. S., and Gan, T. J. (2006). Use of neostigmine in the management of acute postoperative pain and labour pain: A review. Cns Drugs 20, 821–839. doi:10.2165/00023210-200620100-00004
Herman, A. P., Skipor, J., Krawczynska, A., Bochenek, J., Wojtulewicz, K., Antushevich, H., et al. (2017). Peripheral inhibitor of AChE, neostigmine, prevents the inflammatory dependent suppression of GnRH/LH secretion during the follicular phase of the estrous cycle. Biomed. Res. Int. 2017, 6823209. doi:10.1155/2017/6823209
Herman, A. P., Skipor, J., Krawczynska, A., Bochenek, J., Wojtulewicz, K., Pawlina, B., et al. (2019). Effect of central injection of neostigmine on the bacterial endotoxin induced suppression of GnRH/LH secretion in ewes during the follicular phase of the estrous cycle. Int. J. Mol. Sci. 20, 4598. doi:10.3390/ijms20184598
Herman, A. P., Tomaszewska-Zaremba, D., Kowalewska, M., Szczepkowska, A., Oleszkiewicz, M., Krawczynska, A., et al. (2018). Neostigmine attenuates proinflammatory cytokine expression in preoptic area but not choroid plexus during lipopolysaccharide-induced systemic inflammation. Mediat. Inflamm. 2018, 9150207. doi:10.1155/2018/9150207
Hofer, S., Eisenbach, C., Lukic, I. K., Schneider, L., Bode, K., Brueckmann, M., et al. (2008). Pharmacologic cholinesterase inhibition improves survival in experimental sepsis. Crit. Care Med. 36, 404–408. doi:10.1097/01.CCM.0B013E31816208B3
Hoover, D. B. (2017). Cholinergic modulation of the immune system presents new approaches for treating inflammation. Pharmacol. Ther. 179, 1–16. doi:10.1016/j.pharmthera.2017.05.002
Kalb, A., von Haefen, C., Sifringer, M., Tegethoff, A., Paeschke, N., Kostova, M., et al. (2013). Acetylcholinesterase inhibitors reduce neuroinflammation and -degeneration in the cortex and hippocampus of a surgery stress rat model. Plos One 8, e62679. doi:10.1371/journal.pone.0062679
Kanashiro, A., Talbot, J., Peres, R. S., Pinto, L. G., Bassi, G. S., Cunha, T. M., et al. (2016). Neutrophil recruitment and articular hyperalgesia in antigen-induced arthritis are modulated by the cholinergic anti-inflammatory pathway. Basic Clin. Pharmacol. Toxicol. 119, 453–457. doi:10.1111/bcpt.12611
Kapoor, S. (2008). Management of constipation in the elderly: Emerging therapeutic strategies. World J. Gastroenterol. 14, 5226–5227. doi:10.3748/wjg.14.5226
Kim, S., Bolton, E. E., and Bryant, S. H. (2013). PubChem3D: Conformer ensemble accuracy. J. Cheminform 5, 1. doi:10.1186/1758-2946-5-1
Kocsis, P., Gyertyan, I., Eles, J., Laszy, J., Hegedus, N., Gajari, D., et al. (2014). Vascular action as the primary mechanism of cognitive effects of cholinergic, CNS-acting drugs, a rat phMRI BOLD study. J. Cereb. Blood Flow. Metab. 34, 995–1000. doi:10.1038/jcbfm.2014.47
Kopman, A. F., and Naguib, M. (2015). Neostigmine: You can't have it both ways. Anesthesiology 123, 231–233. doi:10.1097/ALN.0000000000000678
Kox, M., Pompe, J. C., Peters, E., Vaneker, M., van der Laak, J. W., van der Hoeven, J. G., et al. (2011). α7 nicotinic acetylcholine receptor agonist GTS-21 attenuates ventilator-induced tumour necrosis factor-α production and lung injury. Br. J. Anaesth. 107, 559–566. doi:10.1093/bja/aer202
Lankarani-Fard, A., and Castle, S. C. (2006). Postoperative delirium and Ogilvie's syndrome resolving with neostigmine. J. Am. Geriatr. Soc. 54, 1016–1017. doi:10.1111/j.1532-5415.2006.00759.x
Lauretti, G. R. (2015). The evolution of spinal/epidural neostigmine in clinical application: Thoughts after two decades. Saudi J. Anaesth. 9, 71–81. doi:10.4103/1658-354X.146319
Leib, C., Goser, S., Luthje, D., Ottl, R., Tretter, T., Lasitschka, F., et al. (2011). Role of the cholinergic antiinflammatory pathway in murine autoimmune myocarditis. Circ. Res. 109, 130–140. doi:10.1161/CIRCRESAHA.111.245563
Li, C. H., Zhang, X., Ge, X. L., Huang, X., Zhang, A. Q., and Gu, W. Q. (2014). Effects of combined anisodamine and neostigmine treatment on the inflammatory response and liver regeneration of obstructive jaundice rats after hepatectomy. Biomed. Res. Int. 2014, 362024. doi:10.1155/2014/362024
Liu, A. J., Zang, P., Guo, J. M., Wang, W., Dong, W. Z., Guo, W., et al. (2012). Involvement of acetylcholine-α7nAChR in the protective effects of arterial baroreflex against ischemic stroke. Cns Neurosci. Ther. 18, 918–926. doi:10.1111/cns.12011
Liu, C., Shen, F. M., Le, Y. Y., Kong, Y., Liu, X., Cai, G. J., et al. (2009). Antishock effect of anisodamine involves a novel pathway for activating alpha7 nicotinic acetylcholine receptor. Crit. Care Med. 37, 634–641. doi:10.1097/CCM.0b013e31819598f5
Liu, F., Lin, X., Lin, Y., Deng, X., Guo, Y., Wang, B., et al. (2022a). The effect of neostigmine on postoperative delirium after colon carcinoma surgery: A randomized, double-blind, controlled trial. Bmc Anesthesiol. 22, 267. doi:10.1186/s12871-022-01804-4
Liu, Y., Fu, H., and Wang, T. (2022b). Neuroinflammation in perioperative neurocognitive disorders: From bench to the bedside. Cns Neurosci. Ther. 28, 484–496. doi:10.1111/cns.13794
Liu, Y., and Yin, Y. (2018). Emerging roles of immune cells in postoperative cognitive dysfunction. Mediat. Inflamm. 2018, 6215350. doi:10.1155/2018/6215350
Luo, J., Chen, S., Min, S., and Peng, L. (2018). Reevaluation and update on efficacy and safety of neostigmine for reversal of neuromuscular blockade. Ther. Clin. Risk Manag. 14, 2397–2406. doi:10.2147/TCRM.S179420
Lyu, S. C., Wang, J., Xu, W. L., Wang, H. X., Pan, F., Jiang, T., et al. (2021). Therapeutic effect of combining anisodamine with neostigmine on local scar formation following roux-en-Y choledochojejunostomy in a novel rat model. Front. Pharmacol. 12, 700050. doi:10.3389/fphar.2021.700050
Miceli, P. C., and Jacobson, K. (2003). Cholinergic pathways modulate experimental dinitrobenzene sulfonic acid colitis in rats. Auton. Neurosci. 105, 16–24. doi:10.1016/S1566-0702(03)00023-7
Naguib, M., and Kopman, A. F. (2018). Neostigmine-induced weakness: What are the facts? Anaesthesia 73, 1055–1057. doi:10.1111/anae.14322
Naguib, M., and Yaksh, T. L. (1994). Antinociceptive effects of spinal cholinesterase inhibition and isobolographic analysis of the interaction with mu and alpha 2 receptor systems. Anesthesiology 80, 1338–1348. doi:10.1097/00000542-199406000-00022
Needham, M. J., Webb, C. E., and Bryden, D. C. (2017). Postoperative cognitive dysfunction and dementia: What we need to know and do. Br. J. Anaesth. 119, i115–i125. doi:10.1093/bja/aex354
Nizri, E., Hamra-Amitay, Y., Sicsic, C., Lavon, I., and Brenner, T. (2006). Anti-inflammatory properties of cholinergic up-regulation: A new role for acetylcholinesterase inhibitors. Neuropharmacology 50, 540–547. doi:10.1016/j.neuropharm.2005.10.013
Noll, F., Behnke, J., Leiting, S., Troidl, K., Alves, G. T., Muller-Redetzky, H., et al. (2017). Self-extracellular RNA acts in synergy with exogenous danger signals to promote inflammation. Plos One 12, e0190002. doi:10.1371/journal.pone.0190002
Noori, H. R., Fliegel, S., Brand, I., and Spanagel, R. (2012). The impact of acetylcholinesterase inhibitors on the extracellular acetylcholine concentrations in the adult rat brain: A meta-analysis. Synapse 66, 893–901. doi:10.1002/syn.21581
Paparini, D., Gori, S., Grasso, E., Scordo, W., Calo, G., Perez, L. C., et al. (2015). Acetylcholine contributes to control the physiological inflammatory response during the peri-implantation period. Acta Physiol. (Oxf) 214, 237–247. doi:10.1111/apha.12494
Pavlov, V. A., Parrish, W. R., Rosas-Ballina, M., Ochani, M., Puerta, M., Ochani, K., et al. (2009). Brain acetylcholinesterase activity controls systemic cytokine levels through the cholinergic anti-inflammatory pathway. Brain Behav. Immun. 23, 41–45. doi:10.1016/j.bbi.2008.06.011
Phillips, S., and Stewart, P. A. (2018). Catching a unicorn: Neostigmine and muscle weakness-not neostigmine for all, but quantitative monitoring for everyone. Anesthesiology 129, 381–382. doi:10.1097/ALN.0000000000002295
Pohanka, M. (2012). Acetylcholinesterase inhibitors: A patent review (2008 - present). Expert Opin. Ther. Pat. 22, 871–886. doi:10.1517/13543776.2012.701620
Pohanka, M. (2014). Inhibitors of acetylcholinesterase and butyrylcholinesterase meet immunity. Int. J. Mol. Sci. 15, 9809–9825. doi:10.3390/ijms15069809
Pollak, Y., Gilboa, A., Ben-Menachem, O., Ben-Hur, T., Soreq, H., and Yirmiya, R. (2005). Acetylcholinesterase inhibitors reduce brain and blood interleukin-1beta production. Ann. Neurol. 57, 741–745. doi:10.1002/ana.20454
Poupko, J. M., Baskin, S. I., and Moore, E. (2007). The pharmacological properties of anisodamine. J. Appl. Toxicol. 27, 116–121. doi:10.1002/jat.1154
Prabhakar, A., Lambert, T., Kaye, R. J., Gaignard, S. M., Ragusa, J., Wheat, S., et al. (2019). Adjuvants in clinical regional anesthesia practice: A comprehensive review. Best. Pract. Res. Clin. Anaesthesiol. 33, 415–423. doi:10.1016/j.bpa.2019.06.001
Prohovnik, I., Arnold, S. E., Smith, G., and Lucas, L. R. (1997). Physostigmine reversal of scopolamine-induced hypofrontality. J. Cereb. Blood Flow. Metab. 17, 220–228. doi:10.1097/00004647-199702000-00012
Qian, J., Zhang, J. M., Lin, L. L., Dong, W. Z., Cheng, Y. Q., Su, D. F., et al. (2015). A combination of neostigmine and anisodamine protects against ischemic stroke by activating α7nAChR. Int. J. Stroke 10, 737–744. doi:10.1111/ijs.12458
Schneider, L., Jabrailova, B., Soliman, H., Hofer, S., Strobel, O., Hackert, T., et al. (2014). Pharmacological cholinergic stimulation as a therapeutic tool in experimental necrotizing pancreatitis. Pancreas 43, 41–46. doi:10.1097/MPA.0b013e3182a85c21
Steinebrunner, N., Mogler, C., Vittas, S., Hoyler, B., Sandig, C., Stremmel, W., et al. (2014). Pharmacologic cholinesterase inhibition improves survival in acetaminophen-induced acute liver failure in the mouse. Bmc Gastroenterol. 14, 148. doi:10.1186/1471-230X-14-148
Steinman, L. (2010). Modulation of postoperative cognitive decline via blockade of inflammatory cytokines outside the brain. Proc. Natl. Acad. Sci. U. S. A. 107, 20595–20596. doi:10.1073/pnas.1015282107
Sun, L., Zhang, G. F., Zhang, X., Liu, Q., Liu, J. G., Su, D. F., et al. (2012). Combined administration of anisodamine and neostigmine produces anti-shock effects: Involvement of α7 nicotinic acetylcholine receptors. Acta Pharmacol. Sin. 33, 761–766. doi:10.1038/aps.2012.26
Swain, A., Nag, D. S., Sahu, S., and Samaddar, D. P. (2017). Adjuvants to local anesthetics: Current understanding and future trends. World J. Clin. Cases 5, 307–323. doi:10.12998/wjcc.v5.i8.307
Tajaate, N., Schreiber, J. U., Fuchs-Buder, T., Jelting, Y., and Kranke, P. (2018). Neostigmine-based reversal of intermediate acting neuromuscular blocking agents to prevent postoperative residual paralysis: A systematic review. Eur. J. Anaesthesiol. 35, 184–192. doi:10.1097/EJA.0000000000000741
Tozzi, A., Sclip, A., Tantucci, M., de Iure, A., Ghiglieri, V., Costa, C., et al. (2015). Region- and age-dependent reductions of hippocampal long-term potentiation and NMDA to AMPA ratio in a genetic model of Alzheimer's disease. Neurobiol. Aging 36, 123–133. doi:10.1016/j.neurobiolaging.2014.07.002
Tyagi, E., Agrawal, R., Nath, C., and Shukla, R. (2010). Cholinergic protection via alpha7 nicotinic acetylcholine receptors and PI3K-Akt pathway in LPS-induced neuroinflammation. Neurochem. Int. 56, 135–142. doi:10.1016/j.neuint.2009.09.011
Xu, Z. P., Wang, H., Hou, L. N., Xia, Z., Zhu, L., Chen, H. Z., et al. (2011). Modulatory effect of anisodamine on airway hyper-reactivity and eosinophilic inflammation in a murine model of allergic asthma. Int. Immunopharmacol. 11, 260–265. doi:10.1016/j.intimp.2010.12.001
Xu, Z. Q., Shao, B. Z., Ke, P., Liu, J. G., Liu, G. K., Chen, X. W., et al. (2016). Combined administration of anisodamine and neostigmine rescued acute lethal crush syndrome through α7nAChR-dependent JAK2-STAT3 signaling. Sci. Rep. 6, 37709. doi:10.1038/srep37709
Zhang, J., Zhang, L., Sun, X., Yang, Y., Kong, L., Lu, C., et al. (2016). Acetylcholinesterase inhibitors for alzheimer's disease treatment ameliorate acetaminophen-induced liver injury in mice via central cholinergic system regulation. J. Pharmacol. Exp. Ther. 359, 374–382. doi:10.1124/jpet.116.233841
Zhang, Y., Zou, J., Wan, F., Peng, F., and Peng, C. (2023). Update on the sources, pharmacokinetics, pharmacological action, and clinical application of anisodamine. Biomed. Pharmacother. 161, 114522. doi:10.1016/j.biopha.2023.114522
Zhao, T., Li, D. J., Liu, C., Su, D. F., and Shen, F. M. (2011). Beneficial effects of anisodamine in shock involved cholinergic anti-inflammatory pathway. Front. Pharmacol. 2, 23. doi:10.3389/fphar.2011.00023
Zhou, J. X., Ke, P., Huan, G., Shao, B. Z., and Liu, C. (2014). Combined treatment with anisodamine and neostigmine inhibits joint inflammation in collagen-induced arthritis mice. Cns Neurosci. Ther. 20, 186–187. doi:10.1111/cns.12213
Zhu, B., Sun, D., Yang, L., Sun, Z., Feng, Y., and Deng, C. (2020). The effects of neostigmine on postoperative cognitive function and inflammatory factors in elderly patients - a randomized trial. Bmc Geriatr. 20, 387. doi:10.1186/s12877-020-01793-4
Keywords: α7nAChR, cholinesterase inhibitors, cholinergic anti-inflammatory pathway, inflammation, neostigmine, perioperative cognitive function
Citation: Si S, Zhao X, Su F, Lu H, Zhang D, Sun L, Wang F and Xu L (2023) New advances in clinical application of neostigmine: no longer focusing solely on increasing skeletal muscle strength. Front. Pharmacol. 14:1227496. doi: 10.3389/fphar.2023.1227496
Received: 23 May 2023; Accepted: 28 July 2023;
Published: 04 August 2023.
Edited by:
José Fernando Oliveira-Costa, Secretaria de Saúde do Estado da Bahia, BrazilReviewed by:
Edna Pereira, University of Maryland, United StatesMara Pires, Bahiana School of Medicine and Public Health, Brazil
Copyright © 2023 Si, Zhao, Su, Lu, Zhang, Sun, Wang and Xu. This is an open-access article distributed under the terms of the Creative Commons Attribution License (CC BY). The use, distribution or reproduction in other forums is permitted, provided the original author(s) and the copyright owner(s) are credited and that the original publication in this journal is cited, in accordance with accepted academic practice. No use, distribution or reproduction is permitted which does not comply with these terms.
*Correspondence: Dongbin Zhang, emhhbmdkYnp1bnlpQDE2My5jb20=; Hongxiu Lu, MTUzMDY0MTgzMzBAMTYzLmNvbQ==; Fan Su, Ym9hdHNhaWxAMTI2LmNvbQ==
†These authors have contributed equally to this work