- 1Department of Medical Biochemistry, School of Medicine, Koc University, Sariyer, Istanbul, Turkey
- 2Koç University Research Center for Translational Medicine (KUTTAM), Sariyer, Istanbul, Turkey
Introduction
Glucose-6-phosphate dehydrogenase (G6PD, glucose 6-phosphate: NADP (+) oxidoreductase, EC1.1.1.1.49) enzyme is the first and rate-limiting step in the pentose phosphate pathway (PPP). G6PD is an allosteric enzyme found in monomer, homodimer, and homotetramer forms and catalyzes the production of 6-phosphogluconolactone from glucose-6-phosphate (G6P) (Meng et al., 2022). G6PD maintains the reduction of NADP+ into coenzyme NADPH which is vital for various reductive biosynthetic reactions such as maintaining reduced glutathione (GSH), protection against reactive oxygen species (ROS), and detoxification of xenobiotics (B Tandogan, 2011; Gao et al., 2019). NADPH is used by the glutathione reductase (GR) enzyme to convert oxidized glutathione (GSSG) into reduced glutathione (GSH) which is one of the most powerful antioxidant molecules in the cell. GSH is used by glutathione s-transferase (GST) enzyme to detoxify xenobiotics such as environmental pollutants, drugs, and chemicals used in industrial products (Aydemir et al., 2018, 2019a; 2019b, 2020a).
G6PD activity is tightly regulated via NADPH/NADP+ ratio, extracellular oxidants, and posttranslational modifications such as phosphorylation, acetylation, glycosylation, ubiquitinylation, and glutarylation (Aydemir and Ulusu, 2020a). G6PD is the common player in glycolysis, gluconeogenesis, PPP, and lipid metabolism; also, overexpression of G6PD activity is associated with lipid dysregulation, insulin resistance, increased body weight, and obesity (Park et al., 2005). The increasing incidence of diabetes and obesity is the leading cause of death, and disabilities worldwide are considered a major public health problem (Lin et al., 2020). Excessive exposure to industrial products and environmental pollutants such as processed food and beverages is directly associated with an increased risk of obesity, metabolic disorders, and diabetes (Bhupathiraju and Hu, 2016). A wide variety of synthetic chemicals used in industrial products such as cosmetics, pharmaceuticals, food and beverage packaging, dyes, households, pesticides, and hygiene products interfere with hormone metabolism altering the endocrine system in humans and wildlife; thus, they are called endocrine-disrupting chemicals (EDCs) (Metcalfe et al., 2022).
Since EDCs activate peroxisome proliferating activating receptors (PPARs) and exert adverse effects on hormone metabolism, they dysregulate lipid, glucose, and energy metabolisms and are referred to as obesogens (Darbre, 2017). People are exposed to EDCs daily at various concentrations via inhalation, digestion, and dermal route. After exposure, these chemicals are metabolized by the liver, kidney, gut, and skin esterases; however, some parts accumulate in the body without metabolizing over time (Aydemir et al., 2020). On the other hand, administration of phthalate and butylparaben altered G6PD activity in various tissues and cells, including the liver, kidney, testis, brain, breast, and spleen, according to the literature (Table 1) (Aydemir et al., 2018, 2019b; 2019a, 2020a). Therefore, we suggest that EDCs can adversely affect the G6PD activity associated with increased body weight, obesity, metabolic syndrome, and diabetes.
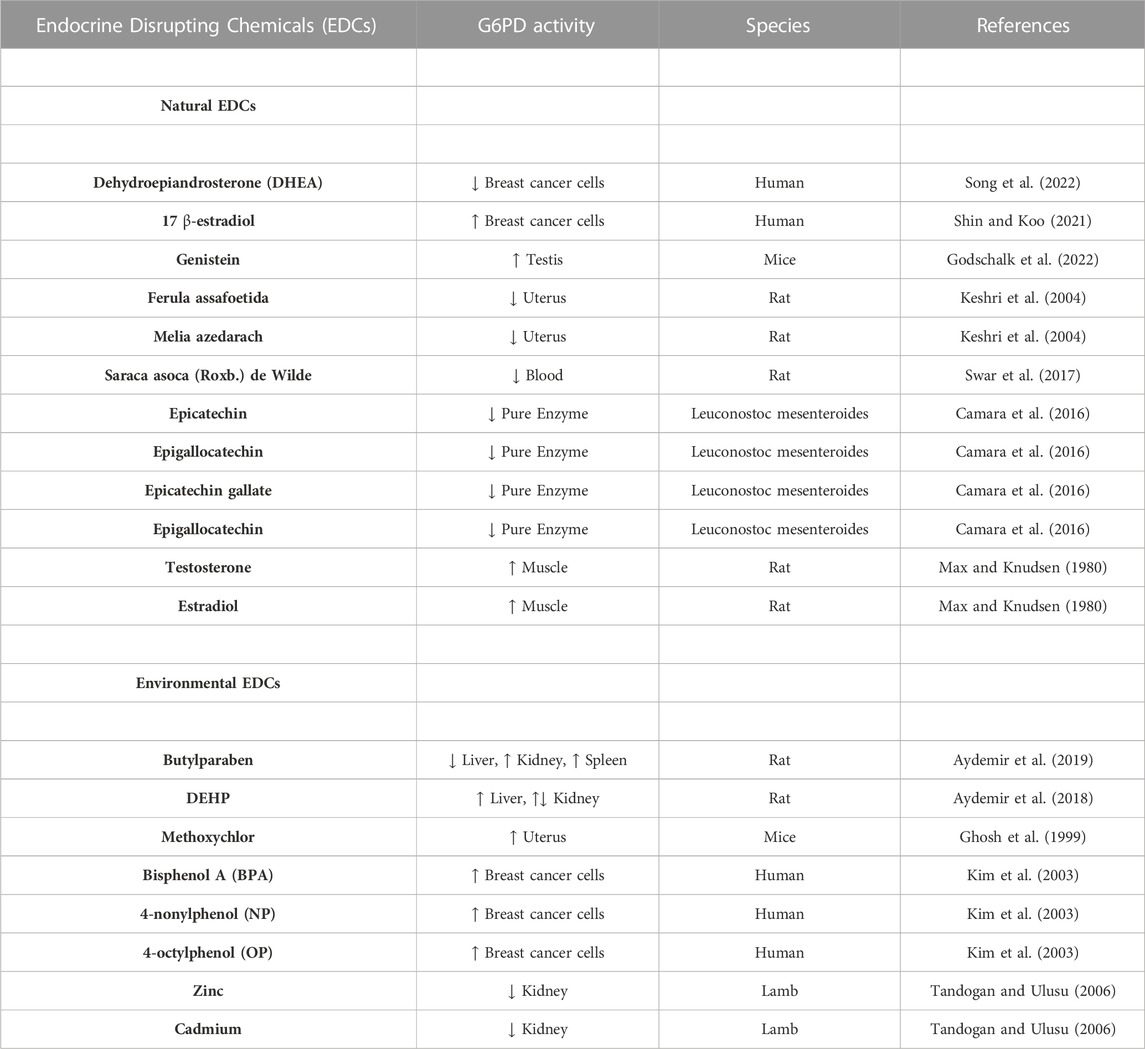
TABLE 1. Impact of the natural and environmental endocrine disrupting chemicals (EDCS) on the G6PD enzyme activity in different species.
Impact of EDCs on the G6PD activity via hormonal regulation
EDCs can be natural or environmental, affecting synthesis, uptake, or hormone-release mechanism in humans and wildlife (Autrup et al., 2020). Natural EDCs can be classified as phytoestrogens and mycoestrogens. Phytoestrogens are the most prominent group mimicking 17 β-estradiol (E2) hormones that can bind estrogen receptors. Increased G6PD activity has been reported in breast cancer cells upon 17 β-estradiol administrations (Monet JD, 1987; Shin and Koo, 2021). Genistein is found in soybeans as the primary dietary source of phytoestrogens, and it increases the G6PD in transgenic mice with lower G6PD activity (Atm-ΔSRI mice) (Zin et al., 2013; Godschalk et al., 2022).
Ferula assafoetida (“Ferula assa-foetida”) belongs to the Umbelliferae family containing terpenoids and affects the estrogen signaling pathway specifically via estrogen receptor α and estrogen receptor β same as phytoestrogens (Ikeda et al., 2002). The extracts of Ferula assafoetida and Chinaberry (“Melia azedarach”) have estrogenic effects, and they cause pregnancy failure when administered to pregnant rats. On the other hand, these extracts exhibit inhibitory effects on the G6PD (Keshri et al., 2004). Saraca asoca (Roxb.) de Wilde, Ashok has estrogenic effects, which is very popular in India; however, dose-dependent G6PD activity increased by using this plant’s extracts, according to the literature (Swar et al., 2017). On the other hand, Epicatechin, epigallocatechin, epicatechin gallate, and epigallocatechin are found in green tea and have anticancer and anti-inflammatory properties. The literature shows they inhibit the G6PD activity (Camara et al., 2016).
G6PD activity is regulated by various hormones (Stanton, 2012), and its activity is considered an indicator of testosterone or estradiol levels. It was proven that injection of the indicated hormones could activate the G6PD activity in a dose-dependent manner (Max and Knudsen, 1980). Xenobiotics, including antibiotics, drugs, and EDCs, can interfere with the secretion, production, metabolism, and transport of estrogen or androgen hormones affecting the G6PD activity. For instance, BPA, dichlorodiphenyltrichloroethane (DDTs), polychlorinated biphenyls (PCBs), and polybrominated diphenyl ethers (PBDEs) have adverse effects on the estrogenic or androgenic pathways associated with reproductive disorders (Amir et al., 2021). Dehydroepiandrosterone (DHEA), androstenolone, is an endogenous steroid hormone precursor. This hormone is produced by the brain, gonads, and adrenal glands and is one of the non-competitive inhibitors of the G6PD in breast cancer cells (Song et al., 2022). Methoxychlor, an insecticide known as an EDC and accepted as a xenoestrogen, can activate the G6PD in mice’s uterus (Ghosh et al., 1999).
Endocrine-disrupting chemicals can cause a metabolic shift in human and wildlife
EDCs, including bisphenol A (BPA), phthalates, perfluoroalkyl substances (PFAS), and aluminum, contributes to obesity via increasing fatty acid storage and appetite (Braun, 2017; Tinkov et al., 2019). EDCs-related obesity may be due to the downregulation of mitochondrial pyruvate carriers (MPC) since, in aerobic conditions, MPC transports pyruvate into mitochondria to be metabolized in the Krebs cycle (Ruiz-Iglesias and Mañes, 2021). Downregulation of MPC results in decreased pyruvate levels in the mitochondria, causing a metabolic shift to the triacylglycerol synthesis associated with obesity (Chen et al., 2018; Hodges et al., 2022). Hypoxia-inducible factor 1α (HIF-α) is one of the essential regulators of the G6PD. EDCs induce HIF-α expression; for instance, BPA can activate HIF-α and vascular endothelial growth factor (VEGF) by triggering the G-protein estrogen receptor (GPER) (Xu et al., 2017; Yang et al., 2021). Both cellular signaling factors, HIF-α and VEGF, can upregulate the G6PD activity (Leopold et al., 2003).
Bisphenols can regulate the nuclear factor erythroid 2–related factor 2 (Nrf2), which controls antioxidant metabolism upon physiological and pathophysiological outcomes of exposure to oxidative stress. Nrf2 transcriptionally induces G6PD activity to upregulate the PPP pathway involved in the antioxidant response of the cell (Salehabadi et al., 2022; H.-C; Yang et al., 2021). Another activator of the G6PD Ataxia-telangiectasia mutated kinase (ATM), a DNA damage-inducible protein kinase that can be activated by BPA (Tichý et al., 2010; Ganesan & Keating, 2016; Xu et al., 2017). 2,3,7,8-Tedtrachlorodibenzo-p-dioxin (TCDD) is one the most toxic EDC with dioxin-like properties inducing TGF-beta1-Smad pathway, oxidative stress, and G6PD activity (Jin et al., 2008; H.-C; Yang et al., 2021). 17beta-estradiol (E2), 4-nonylphenol (NP), and BPA induce the G6PD activity in a concentration-dependent manner in the estrogen-sensitive human breast cancer cell (MCF-7 cells) (Kim et al., 2003).
BPA administration reduces the G6PD activity in erythrocytes leading to hemolysis and morphological changes in the erythrocytes. Thus exposure to EDCs can be life-threatening in G6PD enzyme-deficient individuals (Trivedi et al., 2020). On the other hand, the G6PD was used to explain BPA-mediated diseases in colon cancer cells (SW480), mammary glands, and Sertoli cells because the G6PD was accepted as one of the sensitive biomarkers and can be used for the prediction of BPA-mediated diseases (Ryu et al., 2017). Butylparaben-induced and phthalate-induced oxidative stress via altered G6PD activity caused tissue damage in rats’ liver, kidney, brain, and testis tissue (Aydemir et al., 2020).
Toxic metals such as cadmium lead, silver nitrate, thallium sulfate, cobalt, nitrate, arsenic oxide, and arsenic, and essential trace metals copper, nickel, and manganese are found to be related to G6PD enzyme inhibition and various organ damage associated with the pathogenesis of multiple disorders (Tandogan & Ulusu, 2006; 2007). For instance, cadmium and zinc inhibit the G6PD enzyme in lamb kidneys (Tandogan and Ulusu, 2006). On the other hand, exposure to various EDCs can change trace elements’ homeostasis and affect the activity of antioxidant and detoxification enzymes (Akbay et al., 2004; Aydemir et al., 2018; Aydemir, Öztaşcı, et al., 2019; Aydemir & Ulusu, 2020; Anapali et al., 2022). Metals can interfere with the enzymes’ active site, affect the substrate’s binding to the active site and cause reversible or irreversible enzyme inhibitions. Since heavy metals can denature enzymes by disturbing the native state of proteins’ by destroying secondary and tertiary structures’ disulfide bonds, heavy metal ions are accepted as the most effective inhibitors of the G6PD. Workplace exposure to heavy metals such as lead can cause hematotoxicity, hematopoietic malignancies, and mortality in G6PD-deficient individuals (Cocco, 1998; Cocco et al., 2006). G6PD is vital for all living organisms since the detoxification system relies on NADPH production via G6PD, 6PGD, and IDH enzymes. On the other hand, individuals with G6PD deficiency are more vulnerable to various diseases, primarily oxidative stress-induced disorders, including endocrine, cardiovascular, and metabolic disorders (Tandogan and Ulusu, 2006; Arese et al., 2012; Aydemir et al., 2018; 2019b; 2019a; 2020; Aydemir and Ulusu, 2020).
Conclusion
G6PD enzyme is vital for cell proliferation, production of cellular metabolites, cellular energy, oxidative stress status, and antioxidant response in the cell. G6PD is the rate-limiting enzyme of the PPP responsible for NADPH production. NADPH is vital for various reductive biosynthetic reactions, such as maintaining reduced glutathione (GSH), protecting against reactive oxygen species (ROS), and detoxifying xenobiotics, including drugs, environmental pollutants, and environmental pollutants and EDCs. G6PD activity is tightly regulated via NADPH/NADP+ ratio, extracellular oxidants, and posttranslational modifications such as phosphorylation, acetylation, glycosylation, ubiquitinylation, and glutarylation. Altered G6PD activity is associated with lipid dysregulation, insulin resistance, increased body weight, and obesity since G6PD is the common player in glycolysis, gluconeogenesis, lipid metabolism PPP, and oxidative stress. EDCs are found in most industrial products, including cosmetics, hygiene products, food and beverage packages, toys, and medical devices, and these chemicals interfere with hormone metabolism in humans and wildlife. Since EDCs activate PPARs and adversely affect hormone metabolism, they dysregulate lipid, glucose, and energy metabolisms and are called obesogens. EDCs, including BP, DEHP, BPA, DDT, cadmium, phytoestrogens, PFAS, TCDD, silver nitrate, copper, nickel, manganese, arsenic oxide, and phthalates impair G6PD activity at transcriptional, translational, and posttranslational levels in human and wildlife. Therefore, we suggest that EDCs dysregulate oxidative stress, lipid, and glucose by interfering with G6PD enzyme activity. Individuals with G6PD deficiency can be vulnerable to EDCs-induced adverse health effects, including endocrine, metabolic, and cardiovascular disorders.
Author contributions
NU was responsible for the conceptualization, supervision and writing the original manuscript. DA was responsible for the supporting conceptualization and writing the original manuscript.
Conflict of interest
The authors declare that the research was conducted in the absence of any commercial or financial relationships that could be construed as a potential conflict of interest.
Publisher’s note
All claims expressed in this article are solely those of the authors and do not necessarily represent those of their affiliated organizations, or those of the publisher, the editors and the reviewers. Any product that may be evaluated in this article, or claim that may be made by its manufacturer, is not guaranteed or endorsed by the publisher.
References
Akbay, E., Nuray Ulusu, N., Töröner, F., Ayvaz, G., Taneri, F., Aktürk, M., et al. (2004). Effects of rosiglitazone treatment on the pentose phosphate pathway and glutathione-dependent enzymes in liver and kidney of rats fed a high-fat diet. Curr. Ther. Res. 65, 79–89. doi:10.1016/S0011-393X(04)90007-0
Amir, S., Shah, S. T. A., Mamoulakis, C., Docea, A. O., Kalantzi, O.-I., Zachariou, A., et al. (2021). Endocrine disruptors acting on estrogen and androgen pathways cause reproductive disorders through multiple mechanisms: A review. Int. J. Environ. Res. Public Health 18, 1464. doi:10.3390/ijerph18041464
Anapali, M., Kaya-Dagistanli, F., Akdemir, A. S., Aydemir, D., Ulusu, N. N., Ulutin, T., et al. (2022). Combined resveratrol and vitamin D treatment ameliorate inflammation-related liver fibrosis, ER stress, and apoptosis in a high-fructose diet/streptozotocin-induced T2DM model. Histochem Cell Biol. 158, 279–296. doi:10.1007/s00418-022-02131-y
Arese, P., Gallo, V., Pantaleo, A., and Turrini, F. (2012). Life and death of glucose-6-phosphate dehydrogenase (G6PD) deficient erythrocytes – role of redox stress and band 3 modifications. Transfus. Med. Hemotherapy 39, 328–334. doi:10.1159/000343123
Autrup, H., Barile, F. A., Berry, S. C., Blaauboer, B. J., Boobis, A., Bolt, H., et al. (2020). Human exposure to synthetic endocrine disrupting chemicals (S-EDCs) is generally negligible as compared to natural compounds with higher or comparable endocrine activity: How to evaluate the risk of the S-EDCs? Arch. Toxicol. 94, 2549–2557. doi:10.1007/s00204-020-02800-8
Aydemir, D., Karabulut, G., Gok, M., Barlas, N., and Ulusu, N. N. (2019a). Data the DEHP induced changes on the trace element and mineral levels in the brain and testis tissues of rats. Data Brief. 26, 104526. doi:10.1016/j.dib.2019.104526
Aydemir, D., Karabulut, G., Şimşek, G., Gok, M., Barlas, N., and Ulusu, N. N. (2018). Impact of the di(2-ethylhexyl) phthalate administration on trace element and mineral levels in relation of kidney and liver damage in rats. Biol. Trace Elem. Res. 186, 474–488. doi:10.1007/s12011-018-1331-0
Aydemir, D., Öztaşcı, B., Barlas, N., and Ulusu, N. N. (2019b). Effects of butylparaben on antioxidant enzyme activities and histopathological changes in rat tissues. Arh. Hig. Rada Toksikol. 70, 315–324. doi:10.2478/aiht-2019-70-3342
Aydemir, D., Oztasci, B., Barlas, N., and Ulusu, N. N. (2020). Influence of the butylparaben administration on the oxidative stress metabolism of liver, kidney and spleen. Turk. J. Biochem. 45, 689–694. doi:10.1515/tjb-2020-0048
Aydemir, D., and Ulusu, N. N. (2020). The possible role of the glucose-6-phosphate dehydrogenase enzyme deficiency in the polyneuropathies. J. Basic Clin. Health Sci. 4 (3), 212–217. doi:10.30621/jbachs.2020.1151
B Tandogan, C. S. N. N. U., Sengezer, C., and Ulusu, N. N. (2011). In vitro effects of imatinib on glucose-6-phosphate dehydrogenase and glutathione reductase. Folia Biol. (Praha) 57, 57–64.
Bhupathiraju, S. N., and Hu, F. B. (2016). Epidemiology of obesity and diabetes and their cardiovascular complications. Circ. Res. 118, 1723–1735. doi:10.1161/CIRCRESAHA.115.306825
Braun, J. M. (2017). Early-life exposure to EDCs: Role in childhood obesity and neurodevelopment. Nat. Rev. Endocrinol. 13, 161–173. doi:10.1038/nrendo.2016.186
Camara, M. A., Tian, M., Liu, X., Liu, X., Wang, Y., Yang, J., et al. (2016). Determination of the inhibitory effect of green tea extract on glucose-6-phosphate dehydrogenase based on multilayer capillary enzyme microreactor. Biomed. Chromatogr. 30, 1210–1215. doi:10.1002/bmc.3669
Chen, Y., McCommis, K. S., Ferguson, D., Hall, A. M., Harris, C. A., and Finck, B. N. (2018). Inhibition of the mitochondrial pyruvate carrier by tolylfluanid. Endocrinol 159, 609–621. doi:10.1210/en.2017-00695
Cocco, P., Fadda, D., Atzeri, S., Avataneo, G., Meloni, M., and Flore, C. (2006). Causes of death among lead smelters in relation to the glucose-6-phosphate dehydrogenase polymorphism. Occup. Environ. Med. 64, 414–416. doi:10.1136/oem.2006.028779
Cocco, P. (1998). Occupational lead exposure and screening of glucose-6-phosphate dehydrogenase polymorphism: Useful prevention or nonvoluntary discrimination? Int. Arch. Occup. Environ. Health 71, 148–150. doi:10.1007/s004200050263
Darbre, P. D. (2017). Endocrine disruptors and obesity. Curr. Obes. Rep. 6, 18–27. doi:10.1007/s13679-017-0240-4
Ganesan, S., and Keating, A. F. (2016). Bisphenol A-induced ovotoxicity involves DNA damage induction to which the ovary mounts a protective response indicated by increased expression of proteins involved in DNA repair and xenobiotic biotransformation. Toxicol. Sci. 152, 169–180. doi:10.1093/toxsci/kfw076
Gao, X., Zhao, L., Liu, S., Li, Y., Xia, S., Chen, D., et al. (2019). γ-6-Phosphogluconolactone, a byproduct of the oxidative pentose phosphate pathway, contributes to AMPK activation through inhibition of PP2A. Mol. Cell 76, 857–871. doi:10.1016/j.molcel.2019.09.007
Ghosh, D., Taylor, J. A., Green, J. A., and Lubahn, D. B. (1999). Methoxychlor stimulates estrogen-responsive messenger ribonucleic acids in mouse uterus through a non-estrogen receptor (Non-ER)α and non-erβ mechanism. Endocrinol 140, 3526–3533. doi:10.1210/endo.140.8.6877
Godschalk, R. W. L., Janssen, M. C. M., Vanhees, K., Doorn-Khosrovanivanvan, S. B. W., and Schooten, F.-J. van (2022). Maternal exposure to genistein during pregnancy and oxidative DNA damage in testes of male mouse offspring. Front. Nutr. 9, 904368. doi:10.3389/fnut.2022.904368
Hodges, W. T., Jarasvaraparn, C., Ferguson, D., Griffett, K., Gill, L. E., Chen, Y., et al. (2022). Mitochondrial pyruvate carrier inhibitors improve metabolic parameters in diet-induced obese mice. J. Biol. Chem. 298, 101554. doi:10.1016/j.jbc.2021.101554
Ikeda, K., Arao, Y., Otsuka, H., Nomoto, S., Horiguchi, H., Kato, S., et al. (2002). Terpenoids found in the umbelliferae family act as agonists/antagonists for ER(alpha) and ERbeta: Differential transcription activity between ferutinine-liganded ER(alpha) and ERbeta. Biochem. Biophys. Res. Commun. 291, 354–360. doi:10.1006/bbrc.2002.6446
Jin, M. H., Hong, C. H., Lee, H. Y., Kang, H. J., and Han, S. W. (2008). Enhanced TGF-beta1 is involved in 2,3,7,8-tetrachlorodibenzo-p-dioxin (TCDD) induced oxidative stress in C57BL/6 mouse testis. Toxicol. Lett. 178, 202–209. doi:10.1016/j.toxlet.2008.03.015
Keshri, G., Bajpai, M., Lakshmi, V., Setty, B. S., and Gupta, G. (2004). Role of energy metabolism in the pregnancy interceptive action of Ferula assafoetida and Melia azedarach extracts in rat. Contraception 70, 429–432. doi:10.1016/j.contraception.2004.06.003
Kim, K. B., Seo, K. W., Kim, Y. J., Park, M., Park, C. W., Kim, P. Y., et al. (2003). Estrogenic effects of phenolic compounds on glucose-6-phosphate dehydrogenase in MCF-7 cells and uterine glutathione peroxidase in rats. Chemosphere 50, 1167–1173. doi:10.1016/S0045-6535(02)00628-8
Leopold, J. A., Walker, J., Scribner, A. W., Voetsch, B., Zhang, Y.-Y., Loscalzo, A. J., et al. (2003). Glucose-6-phosphate dehydrogenase modulates vascular endothelial growth factor-mediated angiogenesis. J. Biol. Chem. 278, 32100–32106. doi:10.1074/jbc.M301293200
Lin, X., Xu, Y., Pan, X., Xu, J., Ding, Y., Sun, X., et al. (2020). Global, regional, and national burden and trend of diabetes in 195 countries and territories: An analysis from 1990 to 2025. Sci. Rep. 10, 14790. doi:10.1038/s41598-020-71908-9
Max, S. R., and Knudsen, J. F. (1980). Effect of sex hormones on glucose-6-phosphate dehydrogenase in rat levator ani muscle. Mol. Cell Endocrinol. 17, 111–118. doi:10.1016/0303-7207(80)90123-9
Meng, Q., Zhang, Y., Hao, S., Sun, H., Liu, B., Zhou, H., et al. (2022). Recent findings in the regulation of G6PD and its role in diseases. Front. Pharmacol. 13, 932154. doi:10.3389/fphar.2022.932154
Metcalfe, C. D., Bayen, S., Desrosiers, M., Muñoz, G., Sauvé, S., and Yargeau, V. (2022). An introduction to the sources, fate, occurrence and effects of endocrine disrupting chemicals released into the environment. Environ. Res. 207, 112658. doi:10.1016/j.envres.2021.112658
Monet Jd, T. M. D. N. B. M. B. C., ThoMas, M., DautigNy, N., BraMi, M., and Bader, C. A. (1987). Effects of 17 beta-estradiol and R5020 on glucose-6-phosphate dehydrogenase activity in MCF-7 human breast cancer cells: A cytochemical assay. Cancer Res. 47, 5116–5119.
Park, J., Rho, H. K., Kim, K. H., Choe, S. S., Lee, Y. S., and Kim, J. B. (2005). Overexpression of glucose-6-phosphate dehydrogenase is associated with lipid dysregulation and insulin resistance in obesity. Mol. Cell Biol. 25, 5146–5157. doi:10.1128/MCB.25.12.5146-5157.2005
Ruiz-Iglesias, A., and Mañes, S. (2021). The importance of mitochondrial pyruvate carrier in cancer cell metabolism and tumorigenesis. Cancers (Basel) 13, 1488. doi:10.3390/cancers13071488
Ryu, D.-Y., Rahman, M., and Pang, M.-G. (2017). Determination of highly sensitive biological cell model systems to screen BPA-related health hazards using pathway studio. Int. J. Mol. Sci. 18, 1909. doi:10.3390/ijms18091909
Salehabadi, A., Farkhondeh, T., Harifi-Mood, M. S., Aschner, M., and Samarghandian, S. (2022). Role of Nrf2 in bisphenol effects: A review study. Environ. Sci. Pollut. Res. 29, 55457–55472. doi:10.1007/s11356-022-20996-3
Shin, E., and Koo, J. S. (2021). Glucose metabolism and glucose transporters in breast cancer. Front. Cell Dev. Biol. 9, 728759. doi:10.3389/fcell.2021.728759
Song, J., Sun, H., Zhang, S., and Shan, C. (2022). The multiple roles of glucose-6-phosphate dehydrogenase in tumorigenesis and cancer chemoresistance. Life 12, 271. doi:10.3390/life12020271
Stanton, R. C. (2012). Glucose-6-phosphate dehydrogenase, NADPH, and cell survival. IUBMB Life 64, 362–369. doi:10.1002/iub.1017
Swar, G., Shailajan, S., and Menon, S. (2017). Activity based evaluation of a traditional Ayurvedic medicinal plant: Saraca asoca (Roxb.) de Wilde flowers as estrogenic agents using ovariectomized rat model. J. Ethnopharmacol. 195, 324–333. doi:10.1016/j.jep.2016.11.038
Tandogan, B., and Ulusu, N. N. (2006). Effects of cadmium and zinc ions on purified lamb kidney cortex glucose-6-phosphate dehydrogenase activity. J. Enzyme Inhib. Med. Chem. 21, 225–230. doi:10.1080/14756360500480533
Tandogan, B., and Ulusu, N. N. (2007). The inhibition kinetics of yeast glutathione reductase by some metal ions. J. Enzyme Inhib. Med. Chem. 22, 489–495. doi:10.1080/14756360601162147
Tichý, A., Vávrová, J., Pejchal, J., and Řezáčová, M. (2010). Ataxia-telangiectasia mutated kinase (ATM) as a central regulator of radiation-induced DNA damage response. Acta Medica (Hradec Kralove, Czech Repub. 53, 13–17. doi:10.14712/18059694.2016.57
Tinkov, A. A., Skalnaya, M. G., Aaseth, J., Ajsuvakova, O. P., Aschner, M., and Skalny, A. v. (2019). Aluminium levels in hair and urine are associated with overweight and obesity in a non-occupationally exposed population. J. Trace Elem. Med. Biol. 56, 139–145. doi:10.1016/j.jtemb.2019.08.005
Trivedi, M., Vaidya, D., Patel, C., Prajapati, S., and Bhatt, J. (2020). In silico and in vitro studies to elucidate the role of 1HYN and 1QKI activity in BPA induced toxicity and its amelioration by Gallic acid. Chemosphere 241, 125076. doi:10.1016/j.chemosphere.2019.125076
Xu, F., Wang, X., Wu, N., He, S., Yi, W., Xiang, S., et al. (2017). Bisphenol A induces proliferative effects on both breast cancer cells and vascular endothelial cells through a shared GPER-dependent pathway in hypoxia. Environ. Pollut. 231, 1609–1620. doi:10.1016/j.envpol.2017.09.069
Yang, H.-C., Stern, A., and Chiu, D. T.-Y. (2021). G6PD: A hub for metabolic reprogramming and redox signaling in cancer. Biomed. J. 44, 285–292. doi:10.1016/j.bj.2020.08.001
Keywords: G6PD, endocrine disruptors, enzyme, environmental pollutant, oxidative stress
Citation: Aydemir D and Ulusu NN (2023) The impact of the endocrine-disrupting chemicals on the glucose-6-phosphate dehydrogenase enzyme activity. Front. Pharmacol. 14:1133741. doi: 10.3389/fphar.2023.1133741
Received: 29 December 2022; Accepted: 01 March 2023;
Published: 13 March 2023.
Edited by:
Francesco Sessa, University of Catania, ItalyReviewed by:
Charis Liapi, National and Kapodistrian University of Athens, GreeceRavi Sonkar, Boston University, United States
Copyright © 2023 Aydemir and Ulusu. This is an open-access article distributed under the terms of the Creative Commons Attribution License (CC BY). The use, distribution or reproduction in other forums is permitted, provided the original author(s) and the copyright owner(s) are credited and that the original publication in this journal is cited, in accordance with accepted academic practice. No use, distribution or reproduction is permitted which does not comply with these terms.
*Correspondence: Nuriye Nuray Ulusu, bnVsdXN1QGt1LmVkdS50cg==