- 1College of Traditional Chinese Medicine, Shandong University of Traditional Chinese Medicine, Jinan, China
- 2Department of Respiration, Affiliated Hospital of Shandong University of Traditional Chinese Medicine, Jinan, China
Fritillaria cirrhosa D. Don and F. thunbergii Miq. belong to the genus Fritillaria within the Liliaceae family. They are used in traditional Chinese medicines that are often administered in clinical settings as they have notable effects on cough, bronchitis, pneumonia, lung injury, cancer, and other diseases. In this review, we focus on the history, origin, similarities, and differences in efficacy, chemical composition, and pharmacological outcomes of the drugs obtained from F. cirrhosa (FRC) and F. thunbergii (FRT). We list various valuable pharmacological effects of FRC and FRT, including antitussive, expectorant, anti-inflammatory, antioxidant, and anticancer effects. Thus, this review offers a basis for the medical application of and further research into the pharmacological impacts of these two drugs. We believe that new drugs derived from the phytoconstituents of F. cirrhosa and F. thunbergii that have specific therapeutic properties can be developed in the future.
1 Introduction
Fritillaries are plants that belong to the genus Fritillaria, within the lily family (Liliaceae). The drugs obtained from these plants are called beimu (hereafter referred to as FR). The two most used species of fritillaries for medicinal purposes are Fritillaria cirrhosa D. Don, also known as Sichuan Fritillaria, and F. thunbergii Miq., also known as Bulb of Thunberg Fritillary (Nile et al., 2021). The drugs obtained from these two species are called FRC and FRT, respectively. As a commonly used traditional Chinese medicine (TCM), FR has a long history of use. There is great global demand for wild F. cirrhosa, which is traded in considerable quantities in China, Hong Kong, Taiwan, Canada, Malaysia, Singapore, Korea, and Europe. The demand is particularly high in China, where it exceeds the supply, leading to a price increase of 500 USD per kilogram for F. cirrhosa between 2002 and 2017 (Cunningham et al., 2018). The present study is the first to review the chemical composition and pharmacological effects of both FRC and FRT. It highlights the similarities between FRC and FRT concerning their pharmacological effects, providing a basis for further research into their medicinal application.
1.1 Historical use of fritillary in medicine
FR was first recorded in Shen Nong’s Materia Medica (25–200 AD), where it was listed as a middle-grade herbal medicine, based on toxicity and medicinal effect. The author, Tao Hongjing said, “The shape is like a collection of shellfish, hence the name Fritillaria.” According to the Treatise on Febrile Diseases and Miscellaneous Diseases written by Zhang Zhongjing in 200–210 AD, referred to as the ancestor of the prescription book, Fritillaria species have been utilized to reduce phlegm. In these initial works, FRC and FRT were not clearly distinguished; they were both first differentiated in the late Ming dynasty. “Chuan Fritillaria” was first mentioned in Materia Medica in Southern Yunnan (1463 AD). In the Collected Statements on the Herbal Foundation (1624 AD), Fritillaria species were further classified and compared, with the final proposition that F. cirrhosa and F. tubei (later called F. thunbergii Miq.) are different (Qing et al., 2005). Fritillaria thunbergii was first mentioned in the Description of Materia Medica published in 1691 AD. Thereafter, Benjing Fengyuan (1695 AD) and Benjing Congxin (1757 AD) described the clear distinction between FRC and FRT. A Supplement to Compendium of Materia Medica (1765 AD) explains the basic differences in therapeutic effects between the two. More than 20 medical records of FRC and FRT use are described in the Clinical Guide Medical Records published in 1746 AD (Li et al., 2020). Knowledge about the medicinal value of FR also spread to other East Asian countries and was documented in the Medical Heart Formula (Japan, 982 AD) and Oriental Medicine Treasures (Korea, 1611 AD) (Wang et al., 2021). Currently, medicines containing FR are sold commercially in Japan and Korea for a variety of uses. Furthermore, FR is also widely used as an herbal remedy in other Asian cultures, such as in the Indian Ayurvedic system and Arabian or Unani medicine in Central and Western Asia. However, the Fritillaria species used in these systems are different from the ones used in China. The historical use of FR as a medicine is further described in Table 1.
1.2 Origins and anatomical characteristics of Fritillaria cirrhosa and Fritillaria thunbergii
To date, 165 FR species have been identified worldwide and found in the temperate zone of the Northern hemisphere (Paudel et al., 2021). FRC and FRT medicines are described as two different entities in the Chinese Pharmacopoeia (Chinese Pharmacopoeia Commission, 2015); however, they share commonalities on a biological basis. From a botanical viewpoint, both FRC and FRT are obtained from the desiccated bulbs of plants belonging to the Liliaceae family. FRC is the desiccated bulb of F. cirrhosa, F. przewalskii Maxim., F. unibracteata Hsiao et K.C. Hsia, or F. delavayi Franch., which typically grow under bushes and in woods, fields, wetlands such as streams and seashores, valleys, and rock clefts (Chen and Helen, 2000). Crude plant extracts utilized as medication are mainly obtained from the Gansu (southern), Qinghai, Ningxia, Shaanxi (Qinling), and Shanxi (south) regions in China. Regarding FRT, in Nepal, the Kingdom of Bhutan, Japan, and other countries, dried bulbs are obtained locally from F. thunbergii plants that grow in the shade of lower slopes or in bamboo woodlands. In China, the bulbs are obtained from plants that grow mainly in the Jiangsu (southern), Zhejiang (northern), and Hunan regions. FRC and FRT can be distinguished based on origin, morphological characteristics, and chemical composition (Chinese Pharmacopoeia Commission, 2015). FRC and FRT have different attributes (Figures 1A,B), which can be examined via microscopic observation, thin layer chromatography, polymerase chain reaction-restriction fragment length polymorphism, and content determination. Some researchers have also used matrix-assisted laser desorption/ionization to identify FRs (Wang et al., 2020). According to the Chinese Pharmacopoeia, the total alkaloid content of the dry product of FRC, which is calculated by quantifying sibemrine (C27H43NO3), should be above 0.05%. Similarly, the total peimine (C27H45NO3) and peiminine (C27H43NO3) content of the dry product of FRT should be above 0.08% (Chinese Pharmacopoeia Commission, 2015).
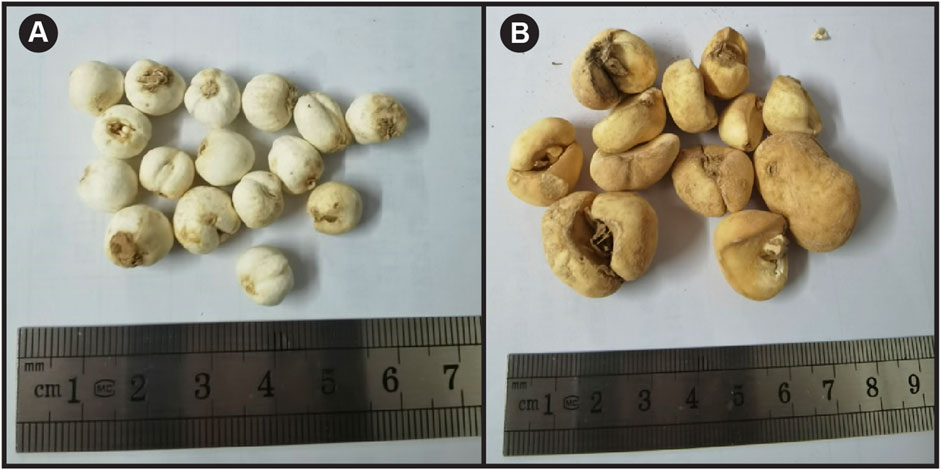
FIGURE 1. Photographs of the dried bulbs of (A) Fritillaria cirrhosa D. Don and (B) Fritillaria thunbergii Miq.
As shown in Figure 1A, FRC is either conical or nearly spherical, with a height of 0.3–0.8 cm and a diameter of 0.3–0.9 cm. The surface of FRC surface is off-white in color, and the external scale leaves have two petals that are incredibly divergent in size. The large petal folds tightly around the small one, and the unfolded portion of the large petal is crescent-shaped. It is commonly said that holding the flower is like holding the moon in one’s arms. One to two small-scale leaves are present within the flower, with obtuse apices that are round or slightly pointed. The bottom of the bulb is flat and slightly concave with a gray-brown bulb disk at the center; occasionally, fibrous roots remain on the bulb. FRC is hard, brittle, and slightly bitter and has a slight smell and a white cross-section that is rich in powder.
As shown in Figure 1B, the single-lobed scale leaf on the external layer of the FRT bulb is slightly crescent in shape, 1–2 cm long, and 2–3.5 cm wide. The external surface is grayish to light yellow, while the internal surface is white or light brown, with white powder. The bulb is hard, brittle, easy to break, and slightly bitter and has a minor smell and a white to yellow-white section that is rich in powder. Fritillaria thunbergii slices are oval or quasi-circular with different sizes. They are typically 1.5–3.5 cm long, 1–2 cm wide, and 0.2–0.4 cm thick. The outer skin is yellowish-brown or gray-brown when slightly shrunken or pale yellow when relatively smooth. The cut surface bulges slightly and is grayish-white or is flat and pinkish-white. The slices are brittle and rich in white flour.
1.3 Similarities and differences in the efficacy of FRC and FRT
FRC and FRT are clinical TCMs that are widely used in the treatment of cough and respiratory diseases (Nile et al., 2021). Both FRC and FRT are classified as heat-clearing and phlegm-resolving drugs. FRC tastes bitter, is slightly cold in nature, and acts on the lung and heart meridians (Hu et al., 2020). It dissipates heat and humidifies the lung, thereby loosening phlegm and relieving cough and asthma. It is generally used for treating dry cough with lung heat, dry cough with less phlegm, tuberculosis caused by yin deficiency, blood in sputum, lung carbuncles, etc. (Liu et al., 2020a). FRC holds clinical significance for diverse pulmonary illnesses, including pneumonia and acute lung injury (Liu et al., 2020a). FRT is bitter, cold in nature, and acts on the lung and heart meridians. It dissipates heat, resolves phlegm, relieves cough, and detoxifies and dispels carbuncles. It can be used to treat cough, bronchitis, hypertension, bacterial infection, and other illnesses caused by wind-heat and phlegm-heat (Cui et al., 2018). In addition, FRT may be used to treat tumoral masses, whereas FRC has little effect on them, Therefore, FRT has been incorporated in some TCM formulations for treating cancer (Hao et at., 2013). Furthermore, FRT can also be used to treat drug-resistant leukemia (Wang et al., 2013). Furthermore, FRC is sweet and moisturizing; it can moisten the lungs and relieve cough, and is specifically appropriate for treating chronic cough caused by internal injury, dry phlegm, and hot phlegm. On the other hand, FRT is strongly bitter and efficient in clearing heat-phlegm and reducing lung-qi. Moreover, its ability to dispel knots and carbuncles is stronger than that of FRC. The differences between the properties of FRC and FRT are summarized in Table 2.
1.4 Differences in the chemical composition of FRC and FRT
FRC and FRT have comparable origins, similar chemical compositions, and are together known as FR (Wu et al., 2018). Researchers have used several methods including high-performance liquid chromatography, quadrupole time-of-flight mass spectrometry, and near-infrared spectroscopy to investigate the chemical composition of FR (Meng et al., 2015; Mohammat et al., 2017). Modern pharmacochemical and pharmacological research has already proven that the primary additives that contribute to the biological potency of FR consist of isosteroidal alkaloids, steroidal alkaloids, non-alkaloids, terpenoids, and steroidal saponins responsible for antitussive activity (Lin et al., 2001; Hu et al., 2018; Wang et al., 2021). Of the 140 compounds isolated from FR, most were isosteroidal alkaloids (72.7%), followed by non-alkaloids (15.8%) and steroidal alkaloids (11.5%) (Lin et al., 2001). Differences in the place of origin and growing conditions also affect the contents of medicinal plants to a certain extent. Studies have shown that light-emitting diodes affect the steroidal alkaloid contents in fritillaries. Furthermore, red- and infrared-light exposure increases fritillary alkaloid content (Chen C. C. et al., 2020). Fritillaria cirrhosa is found in the wild and is also grown under artificial illumination, whereas F. thunbergii is primarily grown under artificial lights. Owing to the high demand for FRC in the pharmaceutical market, wild F. cirrhosa has been collected in large amounts and is now listed as a Grade III protected species (Zhang et al., 2010). Additionally, different harvesting periods and processing methods affect the quality and quantity of active ingredients within cultivated Fritillaria. The best time to harvest this herb is during the wilting period, when the total alkaloid content is at its highest (0.088–0.218%). Drying after washing also ensures quality and improves productivity (Ma et al., 2021).
The primary chemical constituents of FRC are isosteroidal alkaloids, including F. cirrhosa ketones, verticillin ketones, verticillin, and fritillary alkaloids (Liu et al., 2020a). Clear experimental evidence has proven the antitussive and expectorant effects of FRC-derived alkaloids, crinoids (C5), and isovitamin (C6) (Wang et al., 2012). Moreover, amide alkaloids are also found in FRC (Liu et al., 2014). According to the Chinese Pharmacopoeia, sibemidine content is mainly used as the quality control standard of FRC.
FRT comprises diverse chemical elements, including flavonoids, essential oils, saponins, and alkaloids. FRT and its bulbs are mainly composed of alkaloids, essential oils, diterpenes, polysaccharides, amino acids, nucleosides, sterols, lignans, and fatty acids. According to the Chinese Pharmacopoeia, the contents of fritillium A and fritillium B are mainly used as the quality control standard of FRT. The main chemical components of FRC and FRT are illustrated in Figure 2. The chemical structures of sibemine, fritillium A, and fritillium B are illustrated in Figure 3.
2 Pharmacological effects of FRC and FRT
2.1 Pharmacological effects of FRC
Cough suppression, sputum removal, anti-asthmatic, anti-inflammatory, antioxidant, and anticancer properties are some of the pharmacological properties of FRC described in the available literature (Wang et al., 2012; Wang et al., 2016c; Chen T. et al., 2020).
2.1.1 Antitussive and expectorant effects
Cough is one of the most common ailments for which people seek medical help; however, owing to the side effects of narcotic cough suppressants, the treatment options for severe cough remain unsatisfactory (Dicpinigaitis, 2015). FRC has been used to treat cough in China for over 2,000 years. Alkaloids, such as chuanbeione and progesterone isolated from F. chinensis can drastically prolong cough latency, reduce cough frequency in mice, and enhance the production of phenol crimson in the windpipe of mice (Wang et al., 2011). Active alkaloid-chemical sites, such as 17-βH, 22-αH, and 20-OH may also play essential roles in mediating the antitussive and expectorant properties of FRC (Wang et al., 2011). Four alkaloids in F. cirrhosa, namely, imperialine, imperialine N-oxide, isoverticine, and isoverticine N-oxide can dramatically inhibit cough in mice and exert an apparent antitussive effect (Wang et al., 2012). Of these alkaloids, imperialine and isortyline exhibited antitussive effects in a dose-dependent manner (Wang et al., 2012). The alkaloids present in F. cirrhosa can notably increase the excretion of phenol pink within the trachea of mice, which is a method for evaluating expectoration, thereby indicating that F. cirrhosa can relieve cough and reduce phlegm (Wang et al., 2011; Wang et al., 2012). Moreover, FRC extract increased cough latency and suppressed cough frequency in mice (Xu et al., 2019). As an anti-inflammatory compound, FRC extract also prevented the development of ear edema and enhanced the output of phenol red into the trachea of mice (Xu et al., 2019). Thus, the pharmacological outcomes of FRC in terms of relieving cough, resolving phlegm, and reversing inflammation have been demonstrated. Crude alkaloid (10−9–10−5 g/ml) and aqueous extracts (10−7–10−3 g/ml) of FRC relaxed isolated trachea and bronchi of rats subjected to carbachol-induced precontraction, in a concentration-dependent manner (Wu et al., 2018).
2.1.2 Alleviation of acute lung injury and resistance to fibrosis
Acute lung injury is usually caused by direct or indirect alveolar injury, often resulting in acute respiratory distress syndrome (Hughes and Beasley, 2017). Consequently, mortality from acute lung injury is extremely high. It has been shown that inflammatory chemokines, particularly interleukin-6 (IL-6) and tumor necrosis factor-α (TNF-α), play an important role in the onset of acute lung injury (Hu et al., 2015; Rahimi et al., 2017). Wu and his collaborators showed that FRC-derived alkaloids attenuated lipopolysaccharide (LPS)-induced acute lung injury in mice with a correlated reduction in IL-6 and TNF-α levels (Wu et al., 2018). Peimine, which is found in FRC, also ameliorates acute lung injury in mice by inhibiting the expression of TNF-α, IL-1β, IL-6, and IL-8, and further reducing lipid raft formation in alveolar epithelial cells, which is beneficial in alleviating pulmonary fibrosis (Guo et al., 2013; Du et al., 2020).
2.1.3 Anti-asthmatic effect
Asthma is a long-term inflammatory disease of the respiratory tract that adversely impacts the lives of children and elderly persons (Hamid et al., 2003). Experiments show that FRC can reduce nitric oxide (NO), TNF-α, IL-1, IL-6, and malondialdehyde (MDA) levels, increase the activity of superoxide dismutase (SOD), and possibly inhibit matrix metalloproteinase-2 (MMP-2), matrix metalloproteinase-9 (MMP-9), and matrix metalloproteinase-2 (MMP-2). Tissue inhibitor of metalloproteinase-1 (TIMP-1) inhibits airway remodeling in asthmatic mice Histopathological experiments have proved that imperialine contained within FRC, can substantially reduce the percentage of neutrophils in bronchoalveolar lavage fluid as well as in peripheral blood and inhibit TGF-β1 protein expression in lung tissue by correcting TIMP-1/MMP-9 imbalance. Imperialine alleviates the pathological damage and reduces bronchiolar stenosis substantially (Wang et al., 2016a). An in vivo study on asthmatic mice demonstrated the strong inhibitory effect of FRC on airway inflammation (Yeum et al., 2007). The aqueous extract of FRC inhibited the expression of Th2 cytokines (IL-4, IL-5, and IL-13), reduced IgE and eosinophil aggregation, and increased IFN-γ production in the bronchoalveolar fluid (Yeum et al., 2007).
2.1.4 Anti-inflammatory effect
Peimine obtained from FRC exhibits considerable anti-inflammatory effects in vivo by inhibiting LPS-mediated inflammation. Experiments show that peimine inhibits the production of LPS-induced inflammatory cytokines by blocking the MAPKs and NF-κB signaling pathways (Yi et al., 2013). Peiminine can substantially lessen LPS-induced expression of many pro-inflammatory cytokines, including TNF-α, IL-6, cyclooxygenase-2, and inducible nitric oxide synthase, by suppressing the phosphorylation of protein kinase B (AKT) and NF-κB p65 (Chen et al., 2018). Verticinone or imperialine, also found in FRC, can dose-dependently inhibit the production of nitric oxide, expression of both nitric oxide synthase and cyclooxygenase-2, and production of pro-inflammatory cytokines such as TNF-α and IL-1β (Wu et al., 2015). The total alkaloid extract of FRC inhibited paw edema caused by carrageenan and granuloma caused by cotton pellets (Wu et al., 2015). Moreover, FRC-derived edpetiline can upregulate the expression of IL-4 and IL-10, which are cytokines that exhibit powerful anti-inflammatory effects (Zhang et al., 2021). Five alkaloids from FRC exert anti-inflammatory effects by inhibiting LPS-induced phosphorylation of MAPK signaling pathway in RAW264.7 macrophages. As this pathway comprises the anti-inflammatory mediators extracellular signal-regulated kinase (ERK1/2), p38 MAPK, and c-Jun N-terminal kinase (JNK), FRC may be an alternative drug for treating inflammation (Liu et al., 2020b). Some studies have shown that FRC can also relieve gastritis, resist fever and improve memory (Paudel et al., 2021).
2.1.5 Antioxidant effect
Oxidative stress is linked to numerous diseases, including cancer, cardiovascular disease, Parkinson’s disease, Alzheimer’s disease, chronic obstructive pulmonary disease, and rheumatoid arthritis (Pizzino et al., 2017). FRC may serve as a promising therapeutic option for preventing illnesses related to oxidative stress. FRC-derived vitexinone, verticine, imperialine-3-β-D-glucoside, delavir, pemixin, and imperialine reduce the generation of reactive oxygen species, increase the production of glutathione, and promote the expression of heme oxygenase (HO-1), which induces NF-erythroid factor 2-related factor 2 (Nrf2) nuclear translocation, which in turn, is associated with Nrf2 expression upregulation (Liu et al., 2020a). Edpetiline, which is also found in FRC, was shown to reduce the level of reactive oxygen species generated during inflammation, thereby diminishing oxidative stress (Zhang et al., 2021).
2.1.6 Analgesic effect
Verticinone is an alkaloid extracted from FRC, that can inhibit the torsional response triggered by acetic acid; 3 mg/kg verticinone results in a torsional response inhibition of up to 66.2%, which is higher than that induced by 200 mg/kg aspirin (Xu et al., 2011). An analgesic assessment of neuropathic pain revealed that verticinone is more stable than morphine in terms of its analgesic effect (Xu et al., 2011). These studies suggest that verticinone exerts good analgesic effects on both inflammatory and neuropathic pain, with little dependency induction. Thus, verticinone exhibits great potential as an analgesic.
2.1.7 Antiviral effect
FRC extract exerts an antiviral effect by inhibiting the viral replication cycle. It is most effective against H1N1 influenza virus and does not cause toxic reactions in vivo and in vitro. In comparison with oseltamivir, FRC extract is notably less toxic and safer. The use of FRC within 24 h after viral infection resulted in higher survival rates and lower weight loss in mice (Kim et al., 2020).
2.1.8 Anticancer effect
Cancer is the leading cause of death worldwide, with high morbidity and mortality (Jemal et al., 2010). Consequently, it poses a great risk to human health and economic and social development. Despite the approval of several new antitumor drugs every year, most cancer treatments remain limited (Paulson et al., 2013). Interestingly, the aqueous extract of FRC induces apoptosis in cancer cells via immunoregulation mediated by signal transducer and activator of transcription (STAT) 1 and 4 (Li et al., 2020). Moreover, experimental studies have confirmed that FRC-derived imperialine can reliably and safely exert anticancer effects on non-small cell lung cancer and anti-inflammatory effects on non-small cell lung cancer tumors both in vivo and in vitro (Lin et al., 2020). Furthermore, peiminine mediates cell cycle arrest by inhibiting Akt/glycogen synthase kinase 3 beta (GSk3β) and AMP-activated protein kinase (AMPK)/autophagy-activating kinase (ULK1) signaling, which leads to reduced autophagic flux, thereby slowing tumor growth down (Zhao et al., 2018). An in vivo study demonstrated that the alkaloids in FRC retarded the expansion of Lewis lung tumors in rats (Wang et al., 2014) by inhibiting tumor angiogenesis and stimulating apoptosis in cancer cells. The mechanism of action may involve the contribution of total alkaloids in FRC to the downregulation of caspase-3 and endothelial cell adhesion molecule-1 (Wang et al., 2014). Chuanbeinone, which is present in FRC, showed significant antitumor activity in vivo. This component lowered the expression of the anti-apoptotic protein B-cell lymphoma 2 (Bcl-2) and increased the expression of the pro-apoptotic Bcl-2-associated X protein (Bax) and caspase-3, thereby promoting the apoptosis of tumor cells (Wang et al., 2016b). Zheng and his collaborators reported the accumulation of various amino acids such as glutamine in a peiminine-treated colorectal cancer cell line (HCT-116), which may reflect changes in glutathione balance, suggesting that peiminine could impair redox homeostasis in cancer cells (Zheng et al., 2017). It also induced cell death in HCT-116 cells by modulating the production of metabolites such as glucose, glutamine, and oleic acid to alter the autophagic flux. A previous study revealed that FRC extracts block endometrial cancer growth by downregulating the TGF-β/SMAD signaling pathway (Bokhari and Syed, 2015). FRC-derived verticinone inhibits the proliferation of malignant oral keratin-forming cells in a dose- and time-dependent manner, mainly by arresting the G0 and G1 cell cycle and inducing apoptosis (Yun et al., 2008). Verticinone also inhibits the proliferation of human promyelocytic leukemia HL-60 cells by inducing cellular differentiation (Pae et al., 2002).
Part of the anti-inflammatory and antioxidant mechanisms of FRC are showed in Figure 4.
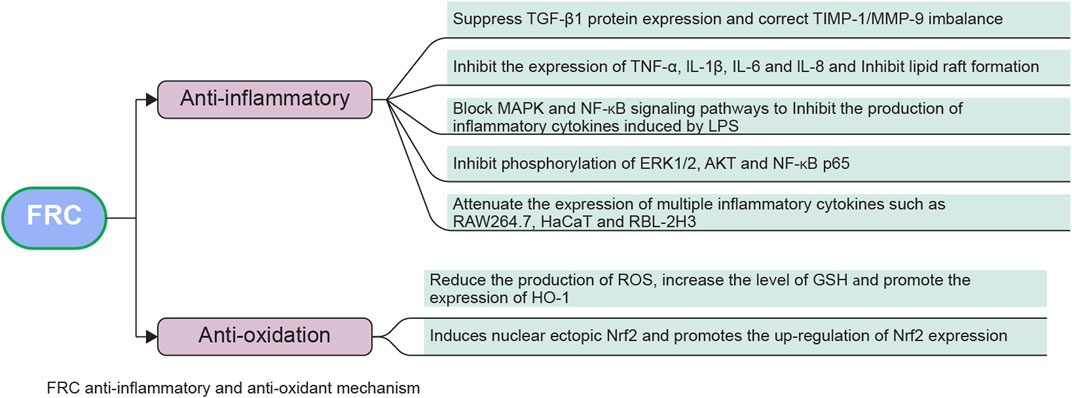
FIGURE 4. Anti-inflammatory and antioxidant mechanism of FRC. Abbreviations: TGF-β1, transforming growth factor β1; IL-1β, interleukin-1β; TIMP-1, matrix metalloproteinase inhibitor-1; MMP-9, matrix metalloproteinase-9; IL-6, interleukin-6; IL-8, interleukin-8; MAPK, mitogen-activated protein kinase; TNF-α, tumor necrosis factor-α; NF-κB, nuclear factor-activated B cells enhanced κ-light chain; LPS, lipopolysaccharide; ERK1/2, extracellular signal-regulated protein kinase 1/2; RAW264.7, monocyte/macrophage-like cell line; AKT, protein kinase B; NF-kB p65, nuclear factor/K gene binding nuclear factor antibody 65; HaCat, human immortalized keratinocyte cell line; ROS, reactive oxygen species; GSH, glutathione; HO-1, heme oxygenase-1; RBL-2H3, rat basophilic leukemia cell line; Nrf2, nuclear factor erythroid factor 2-related factor 2.
2.2 Pharmacological effects of FRT
Similar to FRC, FRT has a history of being used as medicine for over 2,000 years in China. Pharmacological studies have shown that FRT and its bulbs possess a broad spectrum of bioactivity, including antitussive, expectorant, antiulcer, anti-inflammatory, antioxidant, anticancer, neuroprotective, and analgesic activities (Nile et al., 2021).
2.2.1 Cough suppression and expectoration
Two in vivo studies that investigated the cough suppressant effect of FRT showed a significant reduction in cough frequency (15 ± 7.6/5 min) and a prolonged period of remission (73.65 ± 43.02 t/s) after the oral administration of FRT-micronized powder in guinea pigs (Yan X. et al., 2012; Yan Z. et al., 2012). In addition, phenol red expectorant tests showed that FRT has a good expectorant effect and reduces sputum production significantly (Wang et al., 1993; Yan X. et al., 2012; Yan Z. et al., 2012; Qi et al., 2017).
2.2.2 Anti-inflammatory effect
FRT has been applied in Chinese medicine since ancient times to treat inflammatory diseases such as bronchitis or pneumonia. Its ability to reduce inflammation is one of its most important pharmacological effects. Soengbeisine, ebeiedine, and verticine extracted from FRT can inhibit the expression and production of the mucin gene MUC5AC in the human respiratory epithelium, which is induced by epidermal growth factor (EGF), phorbol 12-myristate 13-acetate, or TNF-α (Kim et al., 2016). This is corroborated by the conventional use of FRT as a drug treatment for a broad range of inflammatory lung diseases. FRT-derived isoverticine (C6), puqiedine (C12), 2-monopalmitin (C13), zhebeiresinol (C14), and N-demethylpuqietinone were shown to reduce NF-κB expression in the human embryonic kidney cell line HEK293 and thus exert anti-inflammatory effects (Zhou et al., 2017). FRT extract significantly inhibited IL-6, IL-8, and TNF-α production in human mast cells (HMC-1) and attenuated the phosphorylation of three MAPK signaling channels (ERK, JNK, p38/MAPK) and NF-κB expression, thereby decreasing passive cutaneous anaphylaxis response in rats, indicating its inhibitory impact on anaphylaxis (Cho et al., 2011; Park et al., 2017). Peiminine was shown to inhibit both Nav1.7 and Kv1.3 ion channels, similar to lidocaine, suggesting its potential anti-inflammatory and analgesic effects (Xu et al., 2016).
Part of the anti-inflammatory mechanism of peimine is shown in Figure 5.
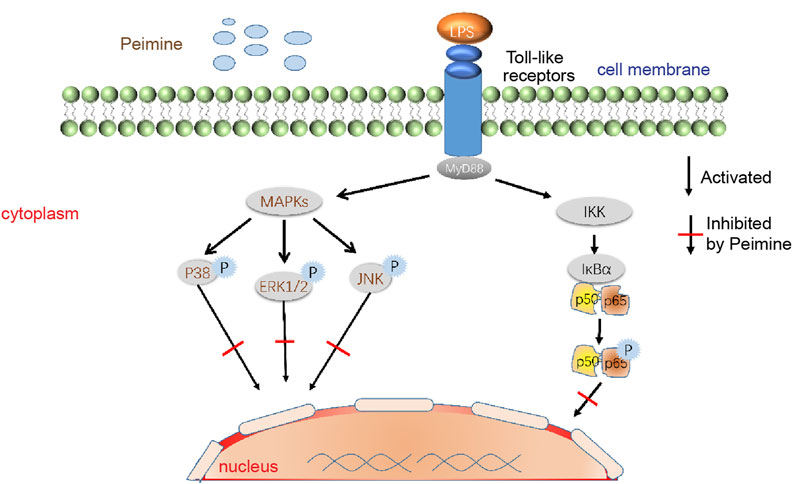
FIGURE 5. Modulation of MAPK and NF-κB signaling pathways by peimine. Abbreviations: LPS, lipopolysaccharide; IKK, inhibitor of nuclear factor kappa-B kinase; P, phosphorylation; MAPKs, mitogen-activated protein kinases; MyD88, myeloid differentiation factor 88; NF-κB, nuclear factor kappa-B; ERK, extracellular signal-regulated kinase; JNK, c-Jun N-terminal kinase. IκBα are significant members of the IκB (NF-κB inhibitor) clan, while NF-κB p50 and p65 are the most delegated dimer complexes in the nuclear transcription clan.
2.2.3 Antioxidant effect
By performing 2,2-diphenyl-1-picrylhydrazyl (DPPH) and 2,2′-azino-bis(3-ethylbenzothiazoline-6-sulfonic acid) (ABTS) free-radical scavenging tests and trivalent reduction activity assay, Ruan and others discovered that crude extracts and alkaloids derived from FRT exert significant antioxidant activity (Ruan et al., 2016). It was also found that polysaccharides extracted from FRT presented antioxidant effects at a concentration of 1 mg/ml (Ma, 2014). These results suggest that FRT has potential antioxidant activities; however, further research is needed to confirm this inference.
2.2.4 Anticancer effect
Different studies have shown that FRT possesses antitumoral effects (Li et al., 2013; Liu et al., 2015). FRT was shown to reverse multidrug-resistance phenotypes in A549 lung adenocarcinoma (Li et al., 2013), HepG2 hepatocellular carcinoma (Liu et al., 2015), and MCF-7 breast cancer cells. The anticancer properties of FRT were also identified in two in vivo and in vitro experimental studies. FRT-derived peiminine inhibits cancer cell growth and movement and induces apoptosis by increasing the intracellular concentration of Ca2+ and phosphorylation induction of calcium/calmodulin-dependent protein kinase II (CaMKII) and JNK (Tan et al., 2020). Although FRT shows good promise as an antitumoral agent, more research on this topic is urgently needed.
Part of anti-cancer effect of FRT are showed in Figure 6.
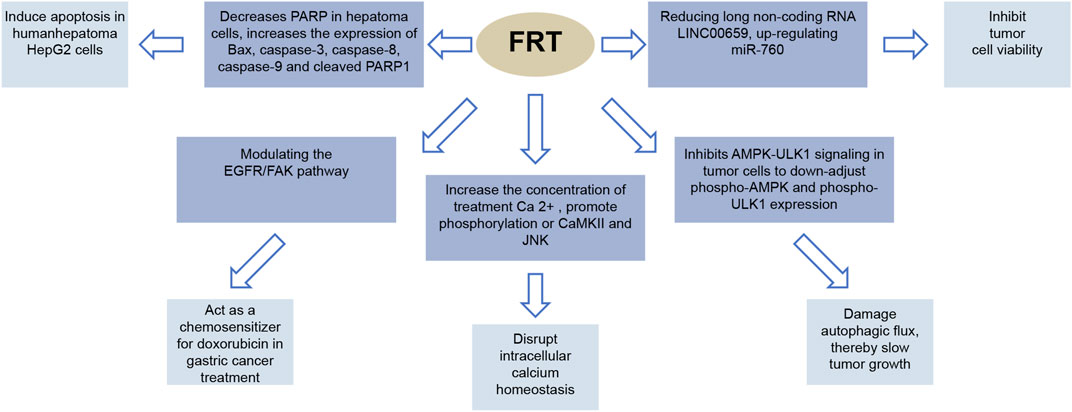
FIGURE 6. Anticancer effect of FRT. PARP (poly-ADP ribose polymerase) is a DNA repair leaven. It plays an important role in DNA damage repair and apoptosis. Bax is a pro-apoptotic protein member of the Bcl-2 family. EGFR is a member of the epidermal growth promoter receptor (HER) clan. FAK (focal adherent kinase) is a tyrosine kinase that is a local adhesion spot kinase. CaMKII is a calcium/calmodulin-dependent protein kinase II. JNK, also known as stress-activated protein kinase, plays an important role in a variety of physiological and pathological processes, including cell cycle, reproduction, apoptosis, and cellular stress.
2.2.5 Other pharmacological effects
FRT-derived peiminine attenuates bleomycin-induced pulmonary fibrotic damage and infection by reducing the levels of circulating INF-γ and inhibiting MAPK/ERK signaling pathways (Guo et al., 2013). In a recent study, peiminine was found to reduce 6-hydroxydopamine-induced degeneration of dopaminergic nerve cells in the midbrain substantia nigra and enhance PTEN induced kinase 1 (PINK1)/parkin expression in order to alleviate apoptosis-related protein in the TGF-β signaling pathway (ARTS)-induced degradation of the X-linked apoptosis inhibitor, resulting in the inhibition of 6-hydroxydopamine-induced apoptosis and thus providing new insight that will be useful for the treatment of Parkinson’s disease (Hsu et al., 2021). Moreover, studies have shown that NF-κB and ERK1/2 signaling pathways are possible targets of peimine. Peimine was described to attenuate bone loss from an ovariectomized mouse by inhibiting the NFATc1, ERK, and NF-κB signaling pathways, thus suppressing the receptor activator of nuclear factor kappa-Β ligand (RANKL), which is involved in osteoclastogenesis (Zhu et al., 2021). In vitro experiments have shown that FRT-derived verticinone has a hypoglycemic effect mediated by increased insulin secretion, glucose uptake, and inhibition of carbohydrate hydrolase activity (Boojar et al., 2020). Another study has shown that fritillin A obtained from FRT inhibited the proliferation and stimulated apoptosis of KG-1a human acute myeloid leukemia cells (Zhang and Chen, 2015). Verticinone inhibits the activity of angiotensin converting enzyme, suggesting that F. thunbergii also has an antihypertensive impact (Oh et al., 2003).
2.2.6 Common pharmacological effects
Because FRC and FRT have a high degree of similarity in terms of composition (Figure 2), they have comparable pharmacological effects, mainly in terms of anti-inflammatory and antitumoral properties.
The primary chemical constituents exhibiting anti-inflammatory effects are peimine and peiminine, which are common to both FRC and FRT. Peimine inhibits IL-1β-induced inflammation in mice chondrocytes by hindering the MAPK pathway (Chen et al., 2019). It also inhibits MAPK phosphorylation and NF-κB expression in mast cells, thus decreasing pro-inflammatory cytokine production (Park et al., 2017). Peimine further targets and inhibits nicotinic acetylcholine receptors with high affinity and may also be responsible for the anti-inflammatory properties of FR (Alberola-Die et al., 2021). Additionally, peiminine attenuates the expression of various inflammatory cytokines in RAW264.7, HaCaT, and RBL-2H3 cells (Lim et al., 2018). Peiminine inhibits the phosphorylation of NF-κB, AKT, ERK1/2, and p38 signaling pathways in vivo and in vitro, thus protecting against LPS-induced mammary-gland inflammation (Gong et al., 2018).
The main chemical constituents exhibiting antitumoral effects are also peiminine and peimine. Peiminine mediates cell cycle arrest by inhibiting Akt/GSk3β and AMPK-ULK1 signaling in glioblastoma multiforme, resulting in the suppression of autophagy, thereby inhibiting glioma growth (Zhao et al., 2018). Peiminine was further shown to reduce the expression of the long non-coding RNA LINC00659, which leads to upregulated miR-760 expression and ultimately inhibited colorectal cancer cell viability (Li et al., 2021). Peiminine also decreases poly (ADP-ribose) polymerase (PARP) activity in hepatoma cells, and increases the expression of Bax, caspase-3, caspase-8, caspase-9, and cleaved PARP1, ultimately leading to apoptosis (Chao et al., 2019). It can also act as a chemosensitizer for doxorubicin in gastric cancer treatment by modulating the EGFR/FAK pathway (Tang et al., 2018). Peimine was also shown to induce cancer cell apoptosis by disrupting intracellular calcium homeostasis via the Ca2+/CaMKII/JNK pathway (Tan et al., 2020).
3 Conclusion
In summary, we analyzed the places of origin, history of development, efficacy, chemical composition, and pharmacological effects of FRC and FRT, which are very similar in origin and composition and have very similar pharmacological effects. This study provides a basis for the clinical use of both herbal medicines. However, herbal medicines have multiple components and targets. As the pharmacological effects of many components have not been studied in depth, further research into the pharmacological mechanisms of each component is urgently needed. The components exhibit many pharmacological properties and thus show great potential in the development of new drugs. FRC and FRT are generally considered to be non-toxic herbal medicines that are free from side effects. Nevertheless, because few toxicological studies have been conducted on these two drugs, more research is needed to establish their clinical safety accurately.
Data availability statement
The original contributions presented in the study are included in the article/Supplementary Material, further inquiries can be directed to the corresponding author.
Author contributions
FW wrote the manuscript. FW, YS, and CW drew the tables and graphs. MT and XL provided some revisions. All authors contributed to the article and approved the submitted version.
Funding
This review was supported by the National Natural Science Foundation of China (No. 82004285).
Acknowledgments
We would like to thank Editage (www.editage.cn) for English language editing.
Conflict of interest
The authors declare that the research was conducted in the absence of any commercial or financial relationships that could be construed as a potential conflict of interest.
Publisher’s note
All claims expressed in this article are solely those of the authors and do not necessarily represent those of their affiliated organizations, or those of the publisher, the editors and the reviewers. Any product that may be evaluated in this article, or claim that may be made by its manufacturer, is not guaranteed or endorsed by the publisher.
References
Alberola-Die, A., Encinar, J. A., Cobo, R., Fernández-Ballester, G., González-Ros, J. M., Ivorra, I., et al. (2021). Peimine, an anti-inflammatory compound from Chinese herbal extracts, modulates muscle-type nicotinic receptors. Int. J. Mol. Sci. 22, 11287. doi:10.3390/ijms222011287
Bokhari, A. A., and Syed, V. (2015). Inhibition of transforming growth factor-β (TGF-β) signaling by Scutellaria baicalensis and Fritillaria cirrhosa extracts in endometrial cancer. J. Cell. Biochem. 116, 1797–1805. doi:10.1002/jcb.25138
Boojar, F. M. A., Aghaei, R., and Boojar, M. M. A. (2020). Data on possible in vitro anti-diabetic effects of verticinone on β-TC6 pancreatic and C2C12 skeletal muscle cells. Data Brief. 28, 104828. doi:10.1016/j.dib.2019.104828
Chao, X., Wang, G., Tang, Y., Dong, C., Li, H., Wang, B., et al. (2019). The effects and mechanism of peiminine-induced apoptosis in human hepatocellular carcinoma HepG2 cells. PLOS ONE 14, e0201864. doi:10.1371/journal.pone.0201864
Chen, C. C., Lee, M. R., Wu, C. R., Ke, H. J., Xie, H. M., Tsay, H. S., et al. (2020a). LED lights affecting morphogenesis and isosteroidal alkaloid contents in Fritillaria cirrhosa D. Don—an important Chinese medicinal herb. Plants 9, 1351. doi:10.3390/plants9101351
Chen, G., Liu, J., Jiang, L., Ran, X., He, D., Li, Y., et al. (2018). Peiminine protects dopaminergic neurons from inflammation-induced cell death by inhibiting the ERK1/2 and NF-κB signalling pathways. Int. J. Mol. Sci. 19, 821. doi:10.3390/ijms19030821
Chen, K., Lv, Z. T., Zhou, C. H., Liang, S., Huang, W., Wang, Z. G., et al. (2019). Peimine suppresses interleukin-1β-induced inflammation via MAPK downregulation in chondrocytes. Int. J. Mol. Med. 43, 2241–2251. doi:10.3892/ijmm.2019.4141
Chen, S., and Helen, V. (2000). in Fritillaria L., Flora of China (24). Editors Z. Y. Wu, and P. H. Raven (Beijing: Science Press).
Chen, T., Zhong, F., Yao, C., Chen, J., Xiang, Y., Dong, J., et al. (2020b). A systematic review on traditional uses, sources, phytochemistry, Pharmacology, pharmacokinetics, and toxicity of fritillariae cirrhosae bulbus. Evid. Based. Complement. Altern. Med. 2020, 1536534. doi:10.1155/2020/1536534
Chinese Pharmacopoeia Commission (2015). Chinese Pharmacopoeia (1). Beijing, China: China Medical Science Press, 191–193.
Cho, I. H., Lee, M. J., Kim, J. H., Han, N. Y., Shin, K. W., Sohn, Y., et al. (2011). Fritillaria ussuriensis extract inhibits the production of inflammatory cytokine and MAPKs in mast cells. Biosci. Biotechnol. Biochem. 75, 1440–1445. doi:10.1271/bbb.110076
Cui, M. C., Chen, S. J., Wang, H. H., Li, Z. H., Chen, H. J., Chen, Y., et al. (2018). Metabolic profiling investigation of Fritillaria thunbergii Miq. by gas chromatography–mass spectrometry. J. Food Drug Anal. 26, 337–347. doi:10.1016/j.jfda.2016.10.003
Cunningham, A. B., Brinckmann, J. A., Pei, S. J., Luo, P., Schippmann, U., Long, X., et al. (2018). High altitude species, high profits: Can the trade in wild harvested fritillaria cirrhosa (Liliaceae) be sustained? J. Ethnopharmacol. 223, 142–151. doi:10.1016/j.jep.2018.05.004
Dicpinigaitis, P. V. (2015). Clinical perspective—cough: An unmet need. Curr. Opin. Pharmacol. 22, 24–28. doi:10.1016/j.coph.2015.03.001
Du, B., Cao, L., Wang, K., Miu, J., Yao, L., Xu, Z., et al. (2020). Peiminine attenuates acute lung injury induced by LPS through inhibiting lipid rafts formation. Inflammation 43, 1110–1119. doi:10.1007/s10753-020-01198-w
Gong, Q., Li, Y., Ma, H., Guo, W., Kan, X., Xu, D., et al. (2018). Peiminine protects against lipopolysaccharide-induced mastitis by inhibiting the AKT/NF-κB, ERK1/2 and p38 signaling pathways. Int. J. Mol. Sci. 19, 2637. doi:10.3390/ijms19092637
Guo, H., Ji, F., Liu, B., Chen, X., He, J., and Gong, J. (2013). Peiminine ameliorates bleomycin-induced acute lung injury in rats. Mol. Med. Rep. 7, 1103–1110. doi:10.3892/mmr.2013.1312
Hamid, Q., Tulic’, M. K., Liu, M. C., and Moqbel, R. (2003). Inflammatory cells in asthma: Mechanisms and implications for therapy. J. Allergy Clin. Immunol. 111, S5–S12. doi:10.1067/mai.2003.22
Hao, D. C., Gu, X. J., Xiao, P. G., and Peng, Y. (2013). Phytochemical and biological research of Fritillaria medicine resources. Chin. J. Nat. Med. 11, 330–344. doi:10.1016/S1875-5364(13)60050-3
Hsu, Y. L., Hung, H. S., Tsai, C. W., Liu, S. P., Chiang, Y. T., Kuo, Y. H., et al. (2021). Peiminine reduces ARTS-mediated degradation of XIAP by modulating the PINK1/parkin pathway to ameliorate 6-hydroxydopamine toxicity and α-synuclein accumulation in Parkinson’s disease models in vivo and in vitro. Int. J. Mol. Sci. 22, 10240. doi:10.3390/ijms221910240
Hu, H., Jiang, Y., Li, X., Lou, J., Zhang, Y., He, X., et al. (2020). The microcirculatory characteristics of the heart and lung meridians: Study protocol clinical trial (SPIRIT Compliant). Med. Baltim. 99, e19594. doi:10.1097/MD.0000000000019594
Hu, J., Zhang, Y., Dong, L., Wang, Z., Chen, L., Liang, D., et al. (2015). Design, synthesis, and biological evaluation of novel quinazoline derivatives as anti-inflammatory agents against lipopolysaccharide-induced acute lung injury in rats. Chem. Biol. Drug Des. 85, 672–684. doi:10.1111/cbdd.12454
Hu, Z., Zong, J. F., Yili, A., Yu, M. H., Aisa, H. A., and Hou, A. J. (2018). Isosteroidal alkaloids from the bulbs of Fritillaria tortifolia. Fitoterapia 131, 112–118. doi:10.1016/j.fitote.2018.10.019
Hughes, K. T., and Beasley, M. B. (2017). Pulmonary manifestations of acute lung injury: More than just diffuse alveolar damage. Arch. Pathol. Lab. Med. 141, 916–922. doi:10.5858/arpa.2016-0342-RA
Jemal, A., Center, M. M., DeSantis, C., and Ward, E. M. (2010). Global patterns of cancer incidence and mortality rates and trends. Cancer Epidemiol. Biomarkers Prev. 19, 1893–1907. doi:10.1158/1055-9965.EPI-10-0437
Kim, E. J., Yoon, Y. P., Woo, K. W., Kim, J. H., Min, S. Y., Lee, H. J., et al. (2016). Verticine, ebeiedine and suchengbeisine isolated from the bulbs of Fritillaria thunbergii Miq. inhibited the gene expression and production of MUC5AC mucin from human airway epithelial cells. Phytomedicine 23, 95–104. doi:10.1016/j.phymed.2015.12.016
Kim, M., Nguyen, D. V., Heo, Y., Park, K. H., Paik, H. D., and Kim, Y. B. (2020). Antiviral activity of Fritillaria thunbergii extract against human influenza virus H1N1 (PR8) in vitro, in ovo and in vivo. J. Microbiol. Biotechnol. 30, 172–177. doi:10.4014/jmb.1908.08001
Li, J., Qin, Y., Wang, W., Yang, K., and Zhang, M. (2021). Peiminine inhibits the progression of colorectal cancer through up-regulating miR-760 via declining the expression of long noncoding RNA LINC00659. Anticancer. Drugs 32, 148–156. doi:10.1097/CAD.0000000000000981
Li, R., Zhang, Y., Wang, Y., Huang, K., Yang, Q., Zhang, T., et al. (2020). Aqueous extract of Fritillariae cirrhosae induces cellular apoptosis through activation of STATs-mediated immunomodulation. J. Ethnopharmacol. 261, 112338. doi:10.1016/j.jep.2019.112338
Li, Z. H., An, C., Hu, K. W., Zhou, K., Duan, H. H., and Tang, M. K. (2013). Multidrug resistance reversal activity of total alkaloid from Fritillaria thunbergii on cisplatin-resistant human lung adenocarcinoma A549/DDP cells. Chin. J. Pharmacol. Toxicol. 27, 315–320. doi:10.3867/j.issn.1000-3002.2013.03.002
Lim, J. M., Lee, B., Min, J. H., Kim, E. Y., Kim, J. H., Hong, S., et al. (2018). Effect of peiminine on DNCB-induced atopic dermatitis by inhibiting inflammatory cytokine expression in vivo and in vitro. Int. Immunopharmacol. 56, 135–142. doi:10.1016/j.intimp.2018.01.025
Lin, G., Li, P., Li, S. L., and Chan, S. W. (2001). Chromatographic analysis of Fritillaria isosteroidal alkaloids, the active ingredients of Beimu, the antitussive traditional Chinese medicinal herb. J. Chromatogr. A 935, 321–338. doi:10.1016/s0021-9673(01)01258-4
Lin, Q., Qu, M., Patra, H. K., He, S., Wang, L., Hu, X., et al. (2020). Mechanistic and therapeutic study of novel antitumor function of natural compound imperialine for treating non-small cell lung cancer. J. Ethnopharmacol. 247, 112283. doi:10.1016/j.jep.2019.112283
Liu, J., Peng, C., He, C. J., Liu, J. L., He, Y. C., Guo, L., et al. (2014). New amino butenolides from the bulbs of Fritillaria unibracteata. Fitoterapia 98, 53–58. doi:10.1016/j.fitote.2014.07.009
Liu, S., Yang, T., Ming, T. W., Gaun, T. K. W., Zhou, T., Wang, S., et al. (2020a). Isosteroid alkaloids from Fritillaria cirrhosa bulbus as inhibitors of cigarette smoke-induced oxidative stress. Fitoterapia 140, 104434. doi:10.1016/j.fitote.2019.104434
Liu, S., Yang, T., Ming, T. W., Gaun, T. K. W., Zhou, T., Wang, S., et al. (2020b). Isosteroid alkaloids with different chemical structures from Fritillariae cirrhosae bulbus alleviate LPS-induced inflammatory response in RAW 264.7 cells by MAPK signaling pathway. Int. Immunopharmacol. 78, 106047. doi:10.1016/j.intimp.2019.106047
Liu, W., Zou, F., and Li, D. (2015). Studies on P-glycoprotein inhibitor of multidrug tumor in Bulbus fritillariae Thunbergii. Chin. J. Surg. Integr. Tradit. West. Med. 21, 379–382. doi:10.3969/j.issn.1007-6948.2015.04.013
Ma, B., Ma, J., Li, B., Tao, Q., Gan, J., and Yan, Z. (2021). Effects of different harvesting times and processing methods on the quality of cultivated Fritillaria cirrhosa D. Don. Food Sci. Nutr. 9, 2853–2861. doi:10.1002/fsn3.2241
Ma, W. (2014). Anti-oxidant activity of polysaccharide from Fritillaria thunbergii Bulbus in vitro. Chin. Arch. Tradit. Chin. Med. 32, 1191–1193. doi:10.13193/j.issn.1673-7717.2014.05.078
Meng, Y., Wang, S., Cai, R., Jiang, B., and Zhao, W. (2015). Discrimination and content analysis of fritillaria using near infrared spectroscopy. J. Anal. Methods Chem. 2015, 752162. doi:10.1155/2015/752162
Mohammat, A., Yili, A., and Aisa, H. A. (2017). Rapid quantification and quantitation of alkaloids in Xinjiang Fritillaria by ultraperformance liquid chromatography-quadrupole time-of-flight mass spectrometry. Molecules 22, 719. doi:10.3390/molecules22050719
Nile, S. H., Su, J., Wu, D., Wang, L., Hu, J., Sieniawska, E., et al. (2021). Fritillaria thunbergii Miq. (Zhe beimu): A review on its traditional uses, phytochemical profile and pharmacological properties. Food Chem. Toxicol. 153, 112289. doi:10.1016/j.fct.2021.112289
Oh, H., Kang, D. G., Lee, S., Lee, Y., and Lee, H. S. (2003). Angiotensin converting enzyme (ACE) inhibitory alkaloids from Fritillaria ussuriensis. Planta Med. 69, 564–565. doi:10.1055/s-2003-40659
Pae, H. O., Oh, H., Choi, B. M., Oh, G. S., Paik, S. G., Jeong, S., et al. (2002). Differentiation-inducing effects of verticinone, an isosteroidal alkaloid isolated from the bulbus of Fritillaria ussuriensis, on human promyelocytic leukemia HL-60 cells. Biol. Pharm. Bull. 25, 1409–1411. doi:10.1248/bpb.25.1409
Park, J. H., Lee, B., Kim, H. K., Kim, E. Y., Kim, J. H., Min, J. H., et al. (2017). Peimine inhibits the production of proinflammatory cytokines through regulation of the phosphorylation of NF-κB and MAPKs in HMC-1 cells. Pharmacogn. Mag. 13, S359–S364. doi:10.4103/0973-1296.210173
Paudel, H. R., Bussmann, R. W., Paniagua-Zambrana, N. Y., and Joshi, L. R. (2021). “Fritillaria cirrhosa D. Don. LILIACEAE,” in Ethnobotany of the himalayas. Ethnobotany of mountain regions. Editors R. M. Kunwar, H. Sher, and R. W. Bussmann (Cham: Springer). doi:10.1007/978-3-030-45597-2_105-3
Paudel, H. R., Timsina, B. P., Kunwar, R. M., Bhandari, G. S., and Poudel, P. (2021). Fritillaria crassicaulis (Liliaceae)―A new record for the Flora of Nepal. J. Jpn. Bot. 96 (5), 292–293. doi:10.51033/jjapbot.96_5_11123
Paulson, A. S., Tran Cao, H. S. T., Tempero, M. A., and Lowy, A. M. (2013). Therapeutic advances in pancreatic cancer. Gastroenterology 144, 1316–1326. doi:10.1053/j.gastro.2013.01.078
Pizzino, G., Irrera, N., Cucinotta, M., Pallio, G., Mannino, F., Arcoraci, V., et al. (2017). Oxidative stress: Harms and benefits for human health. Oxid. Med. Cell. Longev. 2017, 8416763. doi:10.1155/2017/8416763
Qi, Y., Liao, B., Xu, C., Huangfu, Z., and Chen, J. (2017). Effects of peimine on cell viability and apoptosis of multidrug resistant human leukemia cells. Shandong Med. J. 57, 17–20. doi:10.3969/j.issn.1002-266X.2017.26.005
Qing, Y., Shu-qin, N., Xiao-Gang, W., Lan-fang, L., and Lu-qi, H. (2005). Experiment studies on antitumor effect in vivo and in vitro of Aconitum carmichaelii Debx. and Fritillaria thunbergii Miq. used singly or matched. Chin. J. Exp. Tradit. Med. Formulae. 11, 25–28. doi:10.13422/j.cnki.syfjx.2005.04.013
Rahimi, V. B., Shirazinia, R., Fereydouni, N., Zamani, P., Darroudi, S., Sahebkar, A. H., et al. (2017). Comparison of honey and dextrose solution on post-operative peritoneal adhesion in rat model. Biomed. Pharmacother. 92, 849–855. doi:10.1016/j.biopha.2017.05.114
Ruan, X., Yang, L., Cui, W. X., Zhang, M. X., Li, Z. H., Liu, B., et al. (2016). Optimization of supercritical fluid extraction of total alkaloids, peimisine, peimine and peiminine from the bulb of Fritillaria thunbergii Miq, and evaluation of anti-oxidant activities of the extracts. Materials 9, 524. doi:10.3390/ma9070524
Tan, H., Zhang, G., Yang, X., Jing, T., Shen, D., and Wang, X. (2020). Peimine inhibits the growth and motility of prostate cancer cells and induces apoptosis by disruption of intracellular calcium homeostasis through Ca2+/CaMKII/JNK pathway. J. Cell. Biochem. 121, 81–92. doi:10.1002/jcb.28870
Tang, Q., Wang, Y., Ma, L., Ding, M., Li, T., Nie, Y., et al. (2018). Peiminine serves as an adriamycin chemosensitizer in gastric cancer by modulating the EGFR/FAK pathway. Oncol. Rep. 39, 1299–1305. doi:10.3892/or.2018.6184
Wang, D., Du, Q., Li, H., and Wang, S. (2016a). The isosteroid alkaloid imperialine from bulbs of Fritillaria cirrhosa mitigates pulmonary functional and structural impairment and suppresses inflammatory response in a COPD-like rat model. Mediat. Inflamm. 2016, 4192483. doi:10.1155/2016/4192483
Wang, D., Li, Z., Zhang, L., Atanasov, A. G., and Wang, S. (2016b). Characterisation of the isosteroidal alkaloid chuanbeinone from bulbus of Fritillaria pallidiflora as novel antitumor agent in vitro and in vivo. Planta Med. 82, 195–204. doi:10.1055/s-0035-1558156
Wang, D., Wang, S., Chen, X., Xu, X., Zhu, J., Nie, L., et al. (2012). Antitussive, expectorant and anti-inflammatory activities of four alkaloids isolated from Bulbus of Fritillaria wabuensis. J. Ethnopharmacol. 139, 189–193. doi:10.1016/j.jep.2011.10.036
Wang, D., Wang, S., Feng, Y., Zhang, L., Li, Z., Ma, J., et al. (2014). Antitumor effects of Bulbus fritillariae cirrhosae on Lewis lung carcinoma cells in vitro and in vivo. Ind. Crops Prod. 54, 92–101. doi:10.1016/j.indcrop.2013.12.054
Wang, D., Yang, J., Du, Q., Li, H., and Wang, S. (2016c). The total alkaloid fraction of bulbs of Fritillaria cirrhosa displays anti-inflammatory activity and attenuates acute lung injury. J. Ethnopharmacol. 193, 150–158. doi:10.1016/j.jep.2016.08.009
Wang, D., Zhu, J., Wang, S., Wang, X., Ou, Y., Wei, D., et al. (2011). Antitussive, expectorant and anti-inflammatory alkaloids from bulbus fritillariae cirrhosae. Fitoterapia 82, 1290–1294. doi:10.1016/j.fitote.2011.09.006
Wang, J., Chen, X. Y., Lai, Z. L., Zhang, Y. Y., and Hospital, D. (2013). Clinical observation on effects of adjuvant chemotherapy with fritillary Thunbergii bulb complex granules on symptoms of refractory acute leukemia patients. Beijing Zhong Yi Yao Xue Bao 32, 833–835. doi:10.16025/j.1674-1307.2013.11.032
Wang, L., Han, C., and Wang, P. (1993). Pharmacodynamic comparison on antitussive and expectorant effects between Fritillaria anhuiensis SC Chen et SP Yin, Fritillariae cirrhosae Bulbus and Fritillariae thunbergii Bulbus. Anhui Med. J. 14, 57–58.
Wang, Y., Hou, H., Ren, Q., Hu, H., Yang, T., and Li, X. (2021). Natural drug sources for respiratory diseases from fritillaria: Chemical and biological analyses. Chin. Med. 1640, 40. doi:10.1186/s13020-021-00450-1
Wang, Z., Xie, H., Ren, J., Chen, Y., Li, X., Chen, X., et al. (2020). Metabolomic approach for rapid differentiation of Fritillaria bulbs by matrix-assisted laser desorption/ionization mass spectrometry and multivariate statistical analysis. J. Pharm. Biomed. Anal. 185, 113177. doi:10.1016/j.jpba.2020.113177
Wu, K., Mo, C., Xiao, H., Jiang, Y., Ye, B., and Wang, S. (2015). Imperialine and verticinone from bulbs of Fritillaria wabuensis inhibit pro-inflammatory mediators in LPS-stimulated RAW 264.7 macrophages. Planta Med. 81, 821–829. doi:10.1055/s-0035-1546170
Wu, X., Chan, S. W., Ma, J., Li, P., Shaw, P. C., and Lin, G. (2018). Investigation of association of chemical profiles with the tracheobronchial relaxant activity of Chinese medicinal herb Beimu derived from various Fritillaria species. J. Ethnopharmacol. 210, 39–46. doi:10.1016/j.jep.2017.08.027
Xu, F., Xu, S., Wang, L., Chen, C., Zhou, X., Lu, Y., et al. (2011). Antinociceptive efficacy of verticinone in murine models of inflammatory pain and paclitaxel induced neuropathic pain. Biol. Pharm. Bull. 34, 1377–1382. doi:10.1248/bpb.34.1377
Xu, J., Zhao, W., Pan, L., Zhang, A., Chen, Q., Xu, K., et al. (2016). Peimine, a main active ingredient of Fritillaria, exhibits anti-inflammatory and pain suppression properties at the cellular level. Fitoterapia 111, 1–6. doi:10.1016/j.fitote.2016.03.018
Xu, Y., Ming, T. W., Gaun, T. K. W., Wang, S., and Ye, B. (2019). A comparative assessment of acute oral toxicity and traditional pharmacological activities between extracts of Fritillaria cirrhosae Bulbus and Fritillaria pallidiflora Bulbus. J. Ethnopharmacol. 238, 111853. doi:10.1016/j.jep.2019.111853
Yan, X., Tong, Z., Luo, Y., Tang, L., Wu, S., Yang, J., et al. (2012a). Pharmacodynamic comparison of Fritillaria unibracteata, Fritillaria unibracteata var. wabensis and Fritillaria thunbergii cultivated. Chin. J. Exp. Tradit. Med. Formulae. 18, 244–248. doi:10.13422/j.cnki.syfjx.2012.10.072
Yan, Z., Luo, Y., Li, Z., Tang, L., Wu, S., Yan, X., et al. (2012b). Comparative studies on antiussive effect between Fritillaria unibracteata Hisao et K C Hisa and Fritillaria thunbergii Miq. with chemical stimulation induced cough method. Lishizhen Med. Mat. Med. Res. 23, 2522–2525. doi:10.3969/j.issn.1008-0805.2012.10.060
Yeum, H. S., Lee, Y. C., Kim, S. H., Roh, S. S., Lee, J. C., and Seo, Y. B. (2007). Fritillaria cirrhosa, Anemarrhena asphodeloides, Lee-Mo-Tang and cyclosporine a inhibit ovalbumin-induced eosinophil accumulation and Th2-mediated bronchial hyperresponsiveness in a murine model of asthma. Basic Clin. Pharmacol. Toxicol. 100, 205–213. doi:10.1111/j.1742-7843.2007.00043.x
Yi, P. F., Wu, Y. C., Dong, H. B., Guo, Y., Wei, Q., Zhang, C., et al. (2013). Peimine impairs pro-inflammatory cytokine secretion through the inhibition of the activation of NF-κB and MAPK in LPS-induced RAW264.7 macrophages. Immunopharmacol. Immunotoxicol. 35, 567–572. doi:10.3109/08923973.2013.822508
Yun, Y. G., Jeon, B. H., Lee, J. H., Lee, S. K., Lee, H. J., Jung, K. H., et al. (2008). Verticinone induces cell cycle arrest and apoptosis in immortalized and malignant human oral keratinocytes. Phytother. Res. 22, 416–423. doi:10.1002/ptr.2345
Zhang, D. Q., Gao, L. M., and Yang, Y. P. (2010). Genetic diversity and structure of a traditional Chinese medicinal plant species, Fritillaria cirrhosa (Liliaceae) in southwest China and implications for its conservation. Biochem. Syst. Ecol. 38, 236–242. doi:10.1016/j.bse.2009.12.029
Zhang, H., Xu, X., Liu, Z., Sun-Waterhouse, D., Wang, J., Ma, C., et al. (2021). Effects of edpetiline from Fritillaria on inflammation and oxidative stress induced by LPS stimulation in RAW264.7 macrophages. Acta Biochim. Biophys. Sin. 53, 229–237. doi:10.1093/abbs/gmaa160
Zhang, Y., and Chen, X. Y. (2015). Inhibition of AML-KG-1a cell proliferation and induction of apoptosis by bemetin. China Oncol. 24, 325–329. doi:10.11735/j.issn.1004-0242.2015.04.A015
Zhao, B., Shen, C., Zheng, Z., Wang, X., Zhao, W., Chen, X., et al. (2018). Peiminine inhibits glioblastoma in vitro and in vivo through cell cycle arrest and autophagic flux blocking. Cell. Physiol. biochem. 51, 1566–1583. doi:10.1159/000495646
Zheng, Z., Xu, L., Zhang, S., Li, W., Tou, F., He, Q., et al. (2017). Peiminine inhibits colorectal cancer cell proliferation by inducing apoptosis and autophagy and modulating key metabolic pathways. Oncotarget 8, 47619–47631. doi:10.18632/oncotarget.17411
Zhou, M., Ma, X., Ding, G., Wang, Z., Liu, D., Tong, Y., et al. (2017). Comparison and evaluation of antimuscarinic and anti-inflammatory effects of five Bulbus fritillariae species based on UPLC-Q/TOF integrated dual-luciferase reporter assay, PCA and ANN analysis. J. Chromatogr. B Anal. Technol. Biomed. Life Sci. 1042, 60–69. doi:10.1016/j.jchromb.2016.12.012
Keywords: Fritillaria cirrhosa, Fritillaria thunbergii Miq., pharmacological action, efficacy, chemical composition
Citation: Wu F, Tian M, Sun Y, Wu C and Liu X (2022) Efficacy, chemical composition, and pharmacological effects of herbal drugs derived from Fritillaria cirrhosa D. Don and Fritillaria thunbergii Miq.. Front. Pharmacol. 13:985935. doi: 10.3389/fphar.2022.985935
Received: 19 July 2022; Accepted: 22 November 2022;
Published: 30 November 2022.
Edited by:
Anthony Booker, University of Westminster, United KingdomReviewed by:
Kunming Qin, Jiangsu Ocean Universiity, ChinaHem Raj Paudel, National Herbarium and Plant Laboratories, Nepal
Copyright © 2022 Wu, Tian, Sun, Wu and Liu. This is an open-access article distributed under the terms of the Creative Commons Attribution License (CC BY). The use, distribution or reproduction in other forums is permitted, provided the original author(s) and the copyright owner(s) are credited and that the original publication in this journal is cited, in accordance with accepted academic practice. No use, distribution or reproduction is permitted which does not comply with these terms.
*Correspondence: Xue Liu, TGl1eHVlc3p5QDEyNi5jb20=