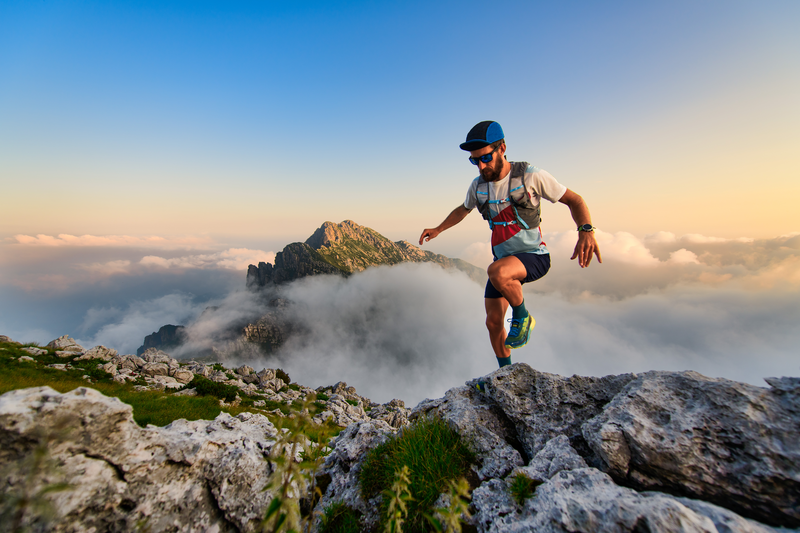
94% of researchers rate our articles as excellent or good
Learn more about the work of our research integrity team to safeguard the quality of each article we publish.
Find out more
MINI REVIEW article
Front. Pharmacol. , 01 June 2022
Sec. Pharmacology of Ion Channels and Channelopathies
Volume 13 - 2022 | https://doi.org/10.3389/fphar.2022.911704
This article is part of the Research Topic Targeting Pumps, Channels and Transporters for the Treatments of Vascular, Cardiovascular and Kidney Diseases View all 6 articles
Hydrogen sulfide (H2S) has been highlighted as an important gasotransmitter in mammals. A growing number of studies have indicated that H2S plays a key role in the pathophysiology of vascular diseases and physiological vascular homeostasis. Alteration in H2S biogenesis has been reported in a variety of vascular diseases and H2S supplementation exerts effects of vasodilation. Accumulating evidence has shown vascular potassium channels activation is involved in H2S-induced vasodilation. This review aimed to summarize and discuss the role of H2S in the regulation of vascular tone, especially by interaction with different vascular potassium channels and the underlying mechanisms.
Hydrogen sulfide (H2S) has been recognized as the third gasotransmitter following nitric oxide and carbon monoxide. Endogenous H2S is mainly produced by enzyme pathways in vivo, including cystathionine γ-lyase (CES), cystathionine β-synthase (CBS), cysteine aminotransferase and 3-mercaptopyruvate sulfurtransferase (3-MST) (Olas, 2015). In the vascular system, H2S is principally synthesized by CES (Szabo, 2012). Biogenesis of H2S or downregulation of H2S pathway has been found in a variety of vascular diseases, such as hypertension, atherosclerosis, diabetes and pulmonary hypertension (Barton and Meyer, 2019; Dillon et al., 2021; Dorofeyeva et al., 2021; Roubenne et al., 2021). H2S plays an important role in regulation vascular homeostasis, such as angiogenesis, proliferation of vascular smooth muscle cells, leukocyte adhesion, oxidative stress and vascular dilation (Wang et al., 2015). The mechanisms underlying H2S induced vascular dilation are not completely understood.
Potassium channels are important determinant of vascular tone (Lorigo et al., 2020; Burkhoff et al., 2021). Activation of potassium channels in vascular cells leads to membrane hyperpolarization and subsequent vasodilatation. Five types of potassium channels have been recognized in vascular cells, including voltage-dependent potassium channels (KV), Ca2+-activated potassium channels (KCa), ATP-sensitive potassium channels (KATP), inward rectifier potassium channels (Kir), and tandem two-pore potassium channels (K2P) (Dogan et al., 2019). Accumulating evidence has shown vascular potassium channels activation is involved in H2S-induced vasodilation (Li et al., 2021; Marinko et al., 2021; Wen et al., 2021). However, the interaction of H2S with different vascular potassium channels and the underlying mechanisms have not been reviewed systematically. This review aimed to summarize and discuss the effects of H2S in the regulation of vascular tone, especially by interaction with different vascular potassium channels and the underlying mechanisms.
Biogenesis of H2S or downregulation of H2S has been found in a variety of vascular diseases. Plasma H2S levels were significantly lower in ever-treated hypertensive patients compared with normal subjects, and were higher in patients with well-controlled blood pressure than in patients with grade 2 and 3 hypertension, suggesting that the reduction of endogenous H2S plays a role in the pathophysiology of hypertension (Sun et al., 2007). The H2S generating enzymes, CSE and 3-MPST were significantly reduced in cutaneous tissue of hypertension adults (Greaney et al., 2017); CSE and CBS expression in lymphocytes were reduced of hypertensive patients (Cui et al., 2020). In patients with portal hypertension, plasma H2S was significantly lower than that in normal subjects, and the level of H2S was negatively correlated with the severity of the disease (Wang et al., 2014). In patients with pulmonary hypertension, plasma level of H2S was decreased (Sun et al., 2014) and negatively correlated with pulmonary artery diameter (Liao et al., 2021). The increase of H2S predicted lower pulmonary artery diameter (Liao et al., 2021). Thus, H2S may be involved in these vascular diseases, providing a new method for the diagnosis and treatment of these vascular diseases.
Accumulating evidence indicated endogenous H2S or H2S supplementation may prevent the development of the vascular diseases. 8 weeks administration of NaHS, a H2S donor, lowered tail artery pressure in spontaneously hypertensive rats (SHR) (Sun et al., 2015). Tain et al. (2018) also reported early short-term NaHS treatment prevented the development of hypertension in SHRs. Exogenous supply of H2S reduced pulmonary hypertension (Chunyu et al., 2003). The intravenous injections of H2S donors, Na2S and NaHS, decreased systemic arterial pressure in the anesthetized rats (Yoo et al., 2015). The predominantly effect of H2S or the H2S donors on regulation of vascular tone is vasodilation, although H2S has vascular constrictive effects in certain conditions (Orlov et al., 2017). NaHS produced vasorelaxation in different vessel beds, including aorta (Köhn et al., 2012), coronary artery (Hedegaard et al., 2014), carotid artery (Centeno et al., 2019), and mesenteric small artery (White et al., 2013). GYY4137, a slow-releasing H2S donor, induced concentration-dependent relaxation in rat mesenteric arteries (Abramavicius et al., 2021) and bovine posterior ciliary artery (Chitnis et al., 2013). N-phenylthiourea (PTU) and N, N′-diphenylthiourea (DPTU) compounds, showing long-lasting H2S donation, promoted vasodilation in rat aortic rings (Citi et al., 2020). Novel H2S donors, AP67 and AP72, also elicited a dose-dependent relaxation in phenylephrine precontracted-bovine posterior ciliary arteries vessels (Kulkarni-Chitnis et al., 2015). L-cysteine, the substrate for CSE, caused a vasodilation in mouse mesenteric artery (Hart, 2020). However, the mechanisms of H2S induced-vasodilation are not fully understood. It may be closely related to vascular potassium channels.
Potassium channels play important roles in regulating of membrane potential and vascular tone. There are many studies having been carried out on H2S-induced vasodilation via activation of potassium channels in different vascular beds. Modulation of potassium channel activity by H2S donors may be a novel treatment for vascular diseases. This review presents the effects and mechanisms of H2S-induced vasodilation via activation of potassium channels, including ATP-sensitive potassium channels (KATP), calcium activated potassiumchannels (KCa) and voltage dependent potassium channels (KV).
The active KATP channel structurally is a hetero-octamer of four Kir6 subunits at the center and four sulfonylurea receptor (SUR) subunits surrounded (Li et al., 2017). Kir6 forms the channel pore and SURs are regulatory subunits (Alquisiras-Burgos et al., 2022). Activation of KATP channels can induce membrane hyperpolarization of the vascular smooth muscle cell, and then lead to the vasodilation.
H2S acted as a KATP channel regulator. Glibenclamide, a KATP channel blocker, significantly inhibited vasodilation-induced by NaHS in carotid arteries of diabetic rabbits (Centeno et al., 2019). H2S was involved in the anticontractile effect of perivascular adiposetissue on aorta of hypertensive pregnant rat and the anticontractile effect was eliminated by KATP blocker (Souza-Paula et al., 2020). NaHS decreases the myogenic response of cerebral arterioles and this effect was partially mediated by KATP channels (Liu et al., 2012). Na2S-induced relaxation of human umbilical vein was also impaired by glibenclamide (Mohammed et al., 2017). Maximum vadilation of constricted mouse aorta elicited by NaHS was unaffected by removal of the endothelium but significantly attenuated by glibenclamide (Al-Magableh and Hart, 2011). In addition to Na2S and NaHS, other H2S donors, such as DPTU, caused vasodilation in rat aorta and was abolished by KATP blocker (Citi et al., 2020). ZYZ-803, a novel H2S and NO conjugated donor, relaxed the aortic contraction induced by PE and the effect was suppressed by glibenclamide (Wu et al., 2016). Glibenclamide significantly attenuated the dilation effect induced by AP67 and AP72 (H2S donors) (Kulkarni-Chitnis et al., 2015) and GYY4137 (Chitnis et al., 2013) respectively on bovine ciliary arteries. H2S plays a role in the vasodilation in response to the reagents, such as moringa oleifera leaves (Aekthammarat et al., 2020) and sulforaphane (Parfenova et al., 2020), and their vasodilation effects was also reduced by glibenclamide. These data suggested an important role of KATP in H2S-induced vasodilation and the possible mechanisms are discussed as below.
First, H2S directly activated KATP channel. H2S increased the whole-cell currents and the single channel open probability of KATP channels. Inhibition of endogenous H2S production reduced whole-cell KATP currents in mesenteric artery smooth muscle cells (Tang et al., 2005). Second, KATP channel can be sulfhydrated by H2S. H2S-mediated sulfhydration and hyperpolarization are absent in cells overexpressing C43S mutant Kir 6.1. Mutating the site of sulfhydration (Kir 6.1 C43S) inhibited H2S-induced hyperpolarization. Sulfhydration augments KATP activity by enhancing Kir 6.1-PIP2 binding and reducing Kir 6.1-ATP binding (Mustafa et al., 2011). Third, H2S-induced vasodilation by activating KATP is mediated by its subunits. Liang et al. (2011) reported glibenclamide partially reversed H2S induced potassium currents in piglet cerebral arterial smooth muscle cells and H2S-induced vasodilation was much smaller in cerebral arteries of SUR2 subunit knockout mice than in wild type mice. Additionaly, H2S promoted vasodilation in SHR, partly through upregulating the expression of Kir6.1 and SUR2B subunits of KATP. H2S inhibited phosphorylation of forkhead transcription factors FOXO1 and FOXO3a, stimulated their nuclear translocation, and enhanced their binding with Kir6.1 and SUR2B promoters (Sun et al., 2015). However, several studies indicated KATP channel had no effect in hydrogen sulfide mediated vasodilation, as KATP blocker did not attenuate H2S-induced vasodilation (Streeter et al., 2012; White et al., 2013; Kutz et al., 2015), suggesting other potassium channels are involved in H2S-induced vascular regulation.
According to the conductance, calcium activated potassium channels are divided into three groups: the large conductance calcium-activated potassium channels (BK, 100–300pS), the intermediate conductance calcium-activated potassium channels (IK, 25–100pS) and the small conductance calcium-activated potassium channels (SK, 2–25pS) (Qian et al., 2014). BK channel is a tetramer consist of α-subunit and β-subunit (Salkoff et al., 2006). The β-subunits has four isoforms (β1–β4) and the β1-subunits are primarily expressed in vascular smooth muscle cells (Lee and Cui, 2010; Wu and Marx, 2010).
Iberiotoxin, a BK channel specific inhibitor, reduced maximal relaxation of human saphenous vein induced by NaHS (Marinko et al., 2021), and Na2S produced dilation of mouse coronary arteries (Chai et al., 2015). Exogenous H2S dilated mesenteric arteries of intermittent hypoxia rats, requires BK channels (Jackson-Weaver et al., 2011). Blockers of BK channel also inhibited GYY4137 induced relaxations in rat mesenteric arteries (Abramavicius et al., 2021).
The activation of BK channel by H2S is mainly mediated by the following two mechanisms. First, H2S activates BK channels by increasing channel currents. BK current density was 91 ± 42 pA/pF under baseline condition while 201 ± 31 pA/pF after exposure to Na2S at testing potential 150 mV in mouse coronary arterial smooth muscle cells (Chai et al., 2015). Second, BK channel was activated by Ca2+influx increase induced by H2S. Naik et al. (2016) reported Na2S increased sulfhydration of transient receptor potential vanilloid type 4 channels in aortic endothelium cells. Activation of transient receptor potential vanilloid type 4 channels by H2S caused Ca2+ influx, resulting in BK channels activation in endothelial cells and subsequent endothelial hyperpolarization, and vascular dilation. Furthermore, the activation of endothelial BK channels by H₂S can also increase Ca2+ sparks in mesenteric artery smooth muscle cells, thus resulted in vasodilation (Jackson-Weaver et al., 2013).
IK and SK channels are also distributed in vascular cells (Liu et al., 2018). H2S-induced vasodilation was partly inhibited by selective IK and SK channel inhibitor charybdotoxin and SK channel inhibitor apamin. H2S stimulated IK and SK in endothelial cells, leading to hyperpolarization and vasodilation (Mustafa et al., 2011). Cheng et al. (2004) also reported charybdotoxin/apamin-sensitive K+ channels in endothelium cells had an important role in H2S effect on rat mesenteric artery. The expression of SK2.3 was decrease in CSE knockout mice or by CSE inhibitor, and was upregulated by H₂S (Tang et al., 2013). Expression of IK was enhanced in carotid arteries from diabetic rabbits, and the relaxant action of NaHS was significantly inhibited by charybdotoxin and 4-amynopiridine in diabetic arteries (Centeno et al., 2019). Thus, H₂S-induced vasodilation was partly through IK and SK.
Kv channels is a diverse family of outwardly rectifying potassium channels (including KV1–KV12) (Hua et al., 2022). Each KV channel comprises a α-subunit and an ancillary β-subunit. α-subunits form the ion conducting pore and β-subunits modulate the activity of α-subunits. KV channels are major determinants of membrane potential and play a key role in regulating vascular dilation (Rhee and Rusch, 2018). And the distribution of KV1, KV7, and KV9.3 are reported in vascular smooth muscle cells of different vessel beds (Nieves-Cintrón et al., 2018).
NaHS relaxed carotid arteries precontracted by phenylephrine of diabetic rabbits, while 4-amynopiridine, a KV channel blocker, significantly inhibited the relaxant effect of NaHS, suggesting a role of KV in H2S induce vasodilation in diabetes (Centeno et al., 2019). Vasodilation to NaHS was also inhibited by blocking KV channels in mouse mesenteric arteries (Hart, 2020), but only a significant shift to the right of the concentration-response curve of NaHS without affecting maximum dilation in the aorta (Al-Magableh and Hart, 2011).
KV7 channel, encoded by KCNQ gene, is thought as a powerful mechanism to H2S induced vasorelaxation. NaHS produced concentration-dependent vasorelaxation, which was blocked by XE991, a KV7 channel inhibitor. Perivascular adipose tissue released H2S, which play a major role in control of vascular tone via KV7 channel (Schleifenbaum et al., 2010). XE991 also inhibited H₂S-induced vasodilatation of porcine coronary arteries (Hedegaard et al., 2014) and murine aortas (Köhn et al., 2012). GYY4137-induced relaxation was also inhibited by KV7 blocker (Abramavicius et al., 2021). The mechanism may be related to persulfidation of KV7 channels by CBS-derived H2S (Vellecco et al., 2020).
Taken together, H2S is involved in vascular diseases, such as hypertension, diabetes, pulmonary hypertension and so on. H2S causes vasodilation in numerous vascular beds and regulates vascular tone by interaction with different vascular potassium channels, including KATP, KCa, and KV channels via the following different mechanisms. H2S can directly activated vascular KATP and BK channels and increased sulfhydration of KATP and KV7 channels. H2S promoted vasodilation in partly through upregulating the expression of Kir6.1 and SUR2B subunits of KATP, or BK channel activation by increased Ca2+influx. Thus, H2S may present a potential therapeutic target on vascular diseases. However, the vasorelaxant effect of H2S in different vessel beds is complex. More studies are needed to focus on the activation mechanisms of H2S on potassium channels, and novel, low-releasing H2S donors are required for treatment in the future.
X-YL wrote the manuscript. R-XW and L-LQ edited the manuscript. All authors read and approved the final manuscript.
This study was supported in part by grants from the National Natural Science Foundation of China (Nos. 82000317 to X-YL and 81770331 to R-XW), Top Talent Support Program for Young and Middle-aged People of Wuxi Health Committee (BJ2020018 to L-LQ) and Research Foundation from Wuxi Health Commission for the Youth (Q202034 to L-LQ).
The authors declare that the research was conducted in the absence of any commercial or financial relationships that could be construed as a potential conflict of interest.
All claims expressed in this article are solely those of the authors and do not necessarily represent those of their affiliated organizations, or those of the publisher, the editors and the reviewers. Any product that may be evaluated in this article, or claim that may be made by its manufacturer, is not guaranteed or endorsed by the publisher.
Thanks to all the peer reviewers for their opinions and suggestions.
Abramavicius, S., Petersen, A. G., Renaltan, N. S., Prat-Duran, J., Torregrossa, R., Stankevicius, E., et al. (2021). GYY4137 and Sodium Hydrogen Sulfide Relaxations Are Inhibited by L-Cysteine and KV7 Channel Blockers in Rat Small Mesenteric Arteries. Front. Pharmacol. 12, 613989. doi:10.3389/fphar.2021.613989
Aekthammarat, D., Tangsucharit, P., and Pannangpetch, P. (2020). Hydrogen Sulfide as a Mediator of Endothelium-dependent Relaxation Evoked by Moringa Oleifera Leaf Extract in Mesenteric Arterial Beds Isolated from L-NAME Hypertensive Rats. J. Complement. Integr. Med. 18, 287–293. doi:10.1515/jcim-2020-0060
Al-Magableh, M. R., and Hart, J. L. (2011). Mechanism of Vasorelaxation and Role of Endogenous Hydrogen Sulfide Production in Mouse Aorta. Naunyn. Schmiedeb. Arch. Pharmacol. 383, 403–413. doi:10.1007/s00210-011-0608-z
Alquisiras-Burgos, I., Franco-Pérez, J., Rubio-Osornio, M., and Aguilera, P. (2022). The Short Form of the SUR1 and its Functional Implications in the Damaged Brain. Neural. Regen. Res. 17, 488–496. doi:10.4103/1673-5374.320967
Barton, M., and Meyer, M. R. (2019). HuR-ry up: How Hydrogen Sulfide Protects against Atherosclerosis. Circulation 139, 115–118. doi:10.1161/CIRCULATIONAHA.118.036854
Burkhoff, D., Rich, S., Pollesello, P., and Papp, Z. (2021). Levosimendan-induced Venodilation Is Mediated by Opening of Potassium Channels. Esc. Heart. Fail. 8, 4454–4464. doi:10.1002/ehf2.13669
Centeno, J. M., López-Morales, M. A., Aliena-Valero, A., Jover-Mengual, T., Burguete, M. C., Castelló-Ruiz, M., et al. (2019). Potassium Channels Contribute to the Increased Sensitivity of the Rabbit Carotid Artery to Hydrogen Sulfide in Diabetes. Eur. J. Pharmacol. 853, 33–40. doi:10.1016/j.ejphar.2019.03.019
Chai, Q., Lu, T., Wang, X. L., and Lee, H. C. (2015). Hydrogen Sulfide Impairs Shear Stress-Induced Vasodilation in Mouse Coronary Arteries. Pflugers. Arch. 467, 329–340. doi:10.1007/s00424-014-1526-y
Cheng, Y., Ndisang, J. F., Tang, G., Cao, K., and Wang, R. (2004). Hydrogen Sulfide-Induced Relaxation of Resistance Mesenteric Artery Beds of Rats. Am. J. Physiol. Heart. Circ. Physiol. 287, H2316–H2323. doi:10.1152/ajpheart.00331.2004
Chitnis, M. K., Njie-Mbye, Y. F., Opere, C. A., Wood, M. E., Whiteman, M., and Ohia, S. E. (2013). Pharmacological Actions of the Slow Release Hydrogen Sulfide Donor GYY4137 on Phenylephrine-Induced Tone in Isolated Bovine Ciliary Artery. Exp. Eye. Res. 116, 350–354. doi:10.1016/j.exer.2013.10.004
Chunyu, Z., Junbao, D., Dingfang, B., Hui, Y., Xiuying, T., and Chaoshu, T. (2003). The Regulatory Effect of Hydrogen Sulfide on Hypoxic Pulmonary Hypertension in Rats. Biochem. Biophys. Res. Commun. 302, 810–816. doi:10.1016/s0006-291x(03)00256-0
Citi, V., Martelli, A., Bucci, M., Piragine, E., Testai, L., Vellecco, V., et al. (2020). Searching for Novel Hydrogen Sulfide Donors: The Vascular Effects of Two Thiourea Derivatives. Pharmacol. Res. 159, 105039. doi:10.1016/j.phrs.2020.105039
Cui, C., Fan, J., Zeng, Q., Cai, J., Chen, Y., Chen, Z., et al. (2020). CD4+ T-Cell Endogenous Cystathionine γ Lyase-Hydrogen Sulfide Attenuates Hypertension by Sulfhydrating Liver Kinase B1 to Promote T Regulatory Cell Differentiation and Proliferation. Circulation 142, 1752–1769. doi:10.1161/CIRCULATIONAHA.119.045344
Dillon, G. A., Stanhewicz, A. E., Serviente, C., Greaney, J. L., and Alexander, L. M. (2021). Hydrogen Sulfide-dependent Microvascular Vasodilation Is Improved Following Chronic Sulfhydryl-Donating Antihypertensive Pharmacotherapy in Adults with Hypertension. Am. J. Physiol. Heart. Circ. Physiol. 321, H728–H734. doi:10.1152/ajpheart.00404.2021
Dogan, M. F., Yildiz, O., Arslan, S. O., and Ulusoy, K. G. (2019). Potassium Channels in Vascular Smooth Muscle: a Pathophysiological and Pharmacological Perspective. Fundam. Clin. Pharmacol. 33, 504–523. doi:10.1111/fcp.12461
Dorofeyeva, N. A., Korkach, I. P., Kutsyk, O. E., and Sagach, V. F. (2021). Modulation of Hydrogen Sulfide Synthesis Improves Heart Function and Endothelium-dependent Vasorelaxation in Diabetes. Can. J. Physiol. Pharmacol. 99, 549–555. doi:10.1139/cjpp-2020-0302
Greaney, J. L., Kutz, J. L., Shank, S. W., Jandu, S., Santhanam, L., and Alexander, L. M. (2017). Impaired Hydrogen Sulfide-Mediated Vasodilation Contributes to Microvascular Endothelial Dysfunction in Hypertensive Adults. Hypertension 69, 902–909. doi:10.1161/HYPERTENSIONAHA.116.08964
Hart, J. L. (2020). Vasorelaxation Elicited by Endogenous and Exogenous Hydrogen Sulfide in Mouse Mesenteric Arteries. Naunyn Schmiedeb. Arch. Pharmacol. 393, 551–564. doi:10.1007/s00210-019-01752-w
Hedegaard, E. R., Nielsen, B. D., Kun, A., Hughes, A. D., Krøigaard, C., Mogensen, S., et al. (2014). KV 7 Channels Are Involved in Hypoxia-Induced Vasodilatation of Porcine Coronary Arteries. Br. J. Pharmacol. 171, 69–82. doi:10.1111/bph.12424
Hua, B., Liu, Q., Gao, S., Li, W., and Li, H. (2022). Protective Role of Activating PPARγ in Advanced Glycation End Products-Induced Impairment of Coronary Artery Vasodilation via Inhibiting P38 Phosphorylation and Reactive Oxygen Species Production. Biomed. Pharmacother. 147, 112641. doi:10.1016/j.biopha.2022.112641
Jackson-Weaver, O., Osmond, J. M., Riddle, M. A., Naik, J. S., Gonzalez Bosc, L. V., Walker, B. R., et al. (2013). Hydrogen Sulfide Dilates Rat Mesenteric Arteries by Activating Endothelial Large-Conductance Ca²⁺-Activated K⁺ Channels and Smooth Muscle Ca²⁺ Sparks. Am. J. Physiol. Heart. Circ. Physiol. 304, H1446–H1454. doi:10.1152/ajpheart.00506.2012
Jackson-Weaver, O., Paredes, D. A., Gonzalez Bosc, L. V., Walker, B. R., and Kanagy, N. L. (2011). Intermittent Hypoxia in Rats Increases Myogenic Tone through Loss of Hydrogen Sulfide Activation of Large-Conductance Ca2+-Activated Potassium Channels. Circ. Res. 108, 1439–1447. doi:10.1161/CIRCRESAHA.110.228999
Köhn, C., Schleifenbaum, J., Szijártó, I. A., Markó, L., Dubrovska, G., Huang, Y., et al. (2012). Differential Effects of Cystathionine-γ-lyase-dependent Vasodilatory H2S in Periadventitial Vasoregulation of Rat and Mouse Aortas. PLoS. One. 7, e41951. doi:10.1371/journal.pone.0041951
Kulkarni-Chitnis, M., Njie-Mbye, Y. F., Mitchell, L., Robinson, J., Whiteman, M., Wood, M. E., et al. (2015). Inhibitory Action of Novel Hydrogen Sulfide Donors on Bovine Isolated Posterior Ciliary Arteries. Exp. Eye. Res. 134, 73–79. doi:10.1016/j.exer.2015.04.001
Kutz, J. L., Greaney, J. L., Santhanam, L., and Alexander, L. M. (2015). Evidence for a Functional Vasodilatatory Role for Hydrogen Sulphide in the Human Cutaneous Microvasculature. J. Physiol. 593, 2121–2129. doi:10.1113/JP270054
Lee, U. S., and Cui, J. (2010). BK Channel Activation: Structural and Functional Insights. Trends. Neurosci. 33, 415–423. doi:10.1016/j.tins.2010.06.004
Li, N., Wu, J. X., Ding, D., Cheng, J., Gao, N., and Chen, L. (2017). Structure of a Pancreatic ATP-Sensitive Potassium Channel. Cell 168, 101–e10. doi:10.1016/j.cell.2016.12.028
Li, Y., Feng, Y., Liu, L., Li, X., Li, X. Y., Sun, X., et al. (2021). The Baroreflex Afferent Pathway Plays a Critical Role in H2S-Mediated Autonomic Control of Blood Pressure Regulation under Physiological and Hypertensive Conditions. Acta. Pharmacol. Sin. 42, 898–908. doi:10.1038/s41401-020-00549-5
Liang, G. H., Adebiyi, A., Leo, M. D., McNally, E. M., Leffler, C. W., and Jaggar, J. H. (2011). Hydrogen Sulfide Dilates Cerebral Arterioles by Activating Smooth Muscle Cell Plasma Membrane KATP Channels. Am. J. Physiol. Heart. Circ. Physiol. 300, H2088–H2095. doi:10.1152/ajpheart.01290.2010
Liao, Y. X., Wang, X. H., Bai, Y., Lin, F., Li, M. X., Mi, W. J., et al. (2021). Relationship between Endogenous Hydrogen Sulfide and Pulmonary Vascular Indexes on High-Resolution Computed Tomography in Patients with Chronic Obstructive Pulmonary Disease. Int. J. Chron. Obstruct. Pulmon. Dis. 16, 2279–2289. doi:10.2147/COPD.S314349
Liu, L., Liu, H., Sun, D., Qiao, W., Qi, Y., Sun, H., et al. (2012). Effects of H₂S on Myogenic Responses in Rat Cerebral Arterioles. Circ. J. 76, 1012–1019. doi:10.1253/circj.cj-11-0890
Liu, Y., Cole, V., Lawandy, I., Ehsan, A., Sellke, F. W., and Feng, J. (2018). Decreased Coronary Arteriolar Response to KCa Channel Opener after Cardioplegic Arrest in Diabetic Patients. Mol. Cell. Biochem. 445, 187–194. doi:10.1007/s11010-017-3264-x
Lorigo, M., Oliveira, N., and Cairrao, E. (2020). Clinical Importance of the Human Umbilical Artery Potassium Channels. Cells 9, 1956. doi:10.3390/cells9091956
Marinko, M., Hou, H. T., Stojanovic, I., Milojevic, P., Nenezic, D., Kanjuh, V., et al. (2021). Mechanisms Underlying the Vasorelaxant Effect of Hydrogen Sulfide on Human Saphenous Vein. Fundam. Clin. Pharmacol. 35, 906–918. doi:10.1111/fcp.12658
Mohammed, R., Provitera, L., Cavallaro, G., Lattuada, D., Ercoli, G., Mosca, F., et al. (2017). Vasomotor Effects of Hydrogen Sulfide in Human Umbilical Vessels. J. Physiol. Pharmacol. 68, 737–747.
Mustafa, A. K., Sikka, G., Gazi, S. K., Steppan, J., Jung, S. M., Bhunia, A. K., et al. (2011). Hydrogen Sulfide as Endothelium-Derived Hyperpolarizing Factor Sulfhydrates Potassium Channels. Circ. Res. 109, 1259–1268. doi:10.1161/CIRCRESAHA.111.240242
Naik, J. S., Osmond, J. M., Walker, B. R., and Kanagy, N. L. (2016). Hydrogen Sulfide-Induced Vasodilation Mediated by Endothelial TRPV4 Channels. Am. J. Physiol. Heart. Circ. Physiol. 311, H1437–H1444. doi:10.1152/ajpheart.00465.2016
Nieves-Cintrón, M., Syed, A. U., Nystoriak, M. A., and Navedo, M. F. (2018). Regulation of Voltage-Gated Potassium Channels in Vascular Smooth Muscle during Hypertension and Metabolic Disorders. Microcirculation 25. doi:10.1111/micc.12423
Olas, B. (2015). Hydrogen Sulfide in Signaling Pathways. Clin. Chim. Acta. 439, 212–218. doi:10.1016/j.cca.2014.10.037
Orlov, S. N., Gusakova, S. V., Smaglii, L. V., Koltsova, S. V., and Sidorenko, S. V. (2017). Vasoconstriction Triggered by Hydrogen Sulfide: Evidence for Na+, K+, 2Cl-cotransport and L-type Ca2+ Channel-Mediated Pathway. Biochem. Biophys. Rep. 12, 220–227. doi:10.1016/j.bbrep.2017.09.010
Parfenova, H., Liu, J., Hoover, D. T., and Fedinec, A. L. (2020). Vasodilator Effects of Sulforaphane in Cerebral Circulation: A Critical Role of Endogenously Produced Hydrogen Sulfide and Arteriolar Smooth Muscle KATP and BK Channels in the Brain. J. Cereb. Blood. Flow. Metab. 40, 1987–1996. doi:10.1177/0271678X19878284
Qian, L., Liu, X., and Wang, R. (2014). Role of BK(Ca) Channels in Diabetic Vascular Complications. Chin. Med. J. Engl. 127, 1775–1781. doi:10.3760/cma.j.issn.0366-6999.20132503
Rhee, S. W., and Rusch, N. J. (2018). Molecular Determinants of Beta-Adrenergic Signaling to Voltage-Gated K+ Channels in the Cerebral Circulation. Microcirculation 25, doi:10.1111/micc.12425
Roubenne, L., Marthan, R., Le Grand, B., and Guibert, C. (2021). Hydrogen Sulfide Metabolism and Pulmonary Hypertension. Cells 10, 1477. doi:10.3390/cells10061477
Salkoff, L., Butler, A., Ferreira, G., Santi, C., and Wei, A. (2006). High-conductance Potassium Channels of the SLO Family. Nat. Rev. Neurosci. 7, 921–931. doi:10.1038/nrn1992.8
Schleifenbaum, J., Köhn, C., Voblova, N., Dubrovska, G., Zavarirskaya, O., Gloe, T., et al. (2010). Systemic Peripheral Artery Relaxation by KCNQ Channel Openers and Hydrogen Sulfide. J. Hypertens. 28, 1875–1882. doi:10.1097/HJH.0b013e32833c20d5
Souza-Paula, E., Polonio, L. C. C., Zochio, G. P., da Silva, K. P., Kushima, H., and Dias-Junior, C. A. (2020). Anticontractile Effect of Perivascular Adipose Tissue but Not of Endothelium Is Enhanced by Hydrogen Sulfide Stimulation in Hypertensive Pregnant Rat Aortae. J. Cardiovasc. Pharmacol. 76, 715–729. doi:10.1097/FJC.0000000000000917
Streeter, E., Hart, J., and Badoer, E. (2012). An Investigation of the Mechanisms of Hydrogen Sulfide-Induced Vasorelaxation in Rat Middle Cerebral Arteries. Naunyn. Schmiedeb. Arch. Pharmacol. 385, 991–1002. doi:10.1007/s00210-012-0779-2
Sun, L., Sun, S., Li, Y., Pan, W., Xie, Y., Wang, S., et al. (2014). Potential Biomarkers Predicting Risk of Pulmonary Hypertension in Congenital Heart Disease: the Role of Homocysteine and Hydrogen Sulfide. Chin. Med. J. Engl. 127, 893–899. doi:10.3760/cma.j.issn.0366-6999.20132694
Sun, N. L., Xi, Y., Yang, S. N., Ma, Z., and Tang, C. S. (2007). Plasma Hydrogen Sulfide and Homocysteine Levels in Hypertensive Patients with Different Blood Pressure Levels and Complications. Zhonghua Xin Xue Guan Bing Za Zhi 35, 1145–1148. doi:10.3760/j.issn:0253-3758.2007.12.016
Sun, Y., Huang, Y., Zhang, R., Chen, Q., Chen, J., Zong, Y., et al. (2015). Hydrogen Sulfide Upregulates KATP Channel Expression in Vascular Smooth Muscle Cells of Spontaneously Hypertensive Rats. J. Mol. Med. Berl. 93, 439–455. doi:10.1007/s00109-014-1227-1
Szabo, C. (2012). Roles of Hydrogen Sulfide in the Pathogenesis of Diabetes Mellitus and its Complications. Antioxid. Redox. Signal. 17, 68–80. doi:10.1089/ars.2011.4451
Tain, Y. L., Hsu, C. N., and Lu, P. C. (2018). Early Short-Term Treatment with Exogenous Hydrogen Sulfide Postpones the Transition from Prehypertension to Hypertension in Spontaneously Hypertensive Rat. Clin. Exp. Hypertens. 40, 58–64. doi:10.1080/10641963.2017.1313847
Tang, G., Wu, L., Liang, W., and Wang, R. (2005). Direct Stimulation of K(ATP) Channels by Exogenous and Endogenous Hydrogen Sulfide in Vascular Smooth Muscle Cells. Mol. Pharmacol. 68, 1757–1764. doi:10.1124/mol.105.017467
Tang, G., Yang, G., Jiang, B., Ju, Y., Wu, L., and Wang, R. (2013). H₂S Is an Endothelium-Derived Hyperpolarizing Factor. Antioxid. Redox. Signal. 19, 1634–1646. doi:10.1089/ars.2012.4805
Vellecco, V., Martelli, A., Bibli, I. S., Vallifuoco, M., Manzo, O. L., Panza, E., et al. (2020). Anomalous Kv7 Channel Activity in Human Malignant Hyperthermia Syndrome Unmasks a Key Role for H2S and Persulfidation in Skeletal Muscle. Br. J. Pharmacol. 177, 810–823. doi:10.1111/bph.14700
Wang, C., Han, J., Xiao, L., Jin, C. E., Li, D. J., and Yang, Z. (2014). Role of Hydrogen Sulfide in Portal Hypertension and Esophagogastric Junction Vascular Disease. World J. Gastroenterol. 20, 1079–1087. doi:10.3748/wjg.v20.i4.1079
Wang, R., Szabo, C., Ichinose, F., Ahmed, A., Whiteman, M., and Papapetropoulos, A. (2015). The Role of H2S Bioavailability in Endothelial Dysfunction. Trends. Pharmacol. Sci. 36, 568–578. doi:10.1016/j.tips.2015.05.007
Wen, J. Y., Zhang, J., Chen, S., Chen, Y., Zhang, Y., Ma, Z. Y., et al. (2021). Endothelium-derived Hydrogen Sulfide Acts as a Hyperpolarizing Factor and Exerts Neuroprotective Effects via Activation of Large-Conductance Ca2+ -activated K+ Channels. Br. J. Pharmacol. 178, 4155–4175. doi:10.1111/bph.15607
White, B. J., Smith, P. A., and Dunn, W. R. (2013). Hydrogen Sulphide-Mediated Vasodilatation Involves the Release of Neurotransmitters from Sensory Nerves in Pressurized Mesenteric Small Arteries Isolated from Rats. Br. J. Pharmacol. 168, 785–793. doi:10.1111/j.1476-5381.2012.02187.x
Wu, D., Hu, Q., Ma, F., and Zhu, Y. Z. (2016). Vasorelaxant Effect of a New Hydrogen Sulfide-Nitric Oxide Conjugated Donor in Isolated Rat Aortic Rings through cGMP Pathway. Oxid. Med. Cell. Longev. 2016, 7075682. doi:10.1155/2016/7075682
Wu, R. S., and Marx, S. O. (2010). The BK Potassium Channel in the Vascular Smooth Muscle and Kidney: α- and β-subunits. Kidney. Int. 78, 963–974. doi:10.1038/ki.2010.325
Keywords: hydrogen sulfide, vasodilation, ATP-sensitive potassium channels, Ca2+-activated potassium channels, voltage-dependent potassium channels
Citation: Liu X-Y, Qian L-L and Wang R-X (2022) Hydrogen Sulfide-Induced Vasodilation: The Involvement of Vascular Potassium Channels. Front. Pharmacol. 13:911704. doi: 10.3389/fphar.2022.911704
Received: 03 April 2022; Accepted: 02 May 2022;
Published: 01 June 2022.
Edited by:
Jinwei Zhang, University of Exeter, United KingdomReviewed by:
Hai-Jian Sun, National University of Singapore, SingaporeCopyright © 2022 Liu, Qian and Wang. This is an open-access article distributed under the terms of the Creative Commons Attribution License (CC BY). The use, distribution or reproduction in other forums is permitted, provided the original author(s) and the copyright owner(s) are credited and that the original publication in this journal is cited, in accordance with accepted academic practice. No use, distribution or reproduction is permitted which does not comply with these terms.
*Correspondence: Ling-Ling Qian, UUxMOTAwQHNpbmEuY29t; Ru-Xing Wang, cnV4aW5nd0BhbGl5dW4uY29t
Disclaimer: All claims expressed in this article are solely those of the authors and do not necessarily represent those of their affiliated organizations, or those of the publisher, the editors and the reviewers. Any product that may be evaluated in this article or claim that may be made by its manufacturer is not guaranteed or endorsed by the publisher.
Research integrity at Frontiers
Learn more about the work of our research integrity team to safeguard the quality of each article we publish.