- 1Princess Srisavangavadhana College of Medicine, Chulabhorn Royal Academy, Bangkok, Thailand
- 2Department of Pharmacology, Faculty of Medicine Siriraj Hospital, Mahidol University, Bangkok, Thailand
Ethnopharmacological studies have become increasingly valuable in the development of botanical products and their bioactive phytochemicals as novel and effective preventive and therapeutic strategies for various diseases including skin photoaging and photodamage-related skin problems including abnormal pigmentation and inflammation. Exploring the roles of phytochemicals in mitigating ultraviolet radiation (UVR)-induced skin damage is thus of importance to offer insights into medicinal and ethnopharmacological potential for development of novel and effective photoprotective agents. UVR plays a role in the skin premature aging (or photoaging) or impaired skin integrity and function through triggering various biological responses of skin cells including apoptosis, oxidative stress, DNA damage and inflammation. In addition, melanin produced by epidermal melanocytes play a protective role against UVR-induced skin damage and therefore hyperpigmentation mediated by UV irradiation could reflect a sign of defensive response of the skin to stress. However, alteration in melanin synthesis may be implicated in skin damage, particularly in individuals with fair skin. Oxidative stress induced by UVR contributes to the process of skin aging and inflammation through the activation of related signaling pathways such as the mitogen-activated protein kinase (MAPK)/activator protein-1 (AP-1), the phosphatidylinositol 3-kinase (PI3K)/protein kinase B (Akt), the nuclear factor kappa B (NF-κB) and the signal transducer and activator of transcription (STAT) in epidermal keratinocytes and dermal fibroblasts. ROS formation induced by UVR also plays a role in regulation of melanogenesis in melanocytes via modulating MAPK, PI3K/Akt and the melanocortin 1 receptor (MC1R)-microphthalmia-associated transcription factor (MITF) signaling cascades. Additionally, nuclear factor erythroid 2-related factor 2 (Nrf2)-regulated antioxidant defenses can affect the major signaling pathways involved in regulation of photoaging, inflammation associated with skin barrier dysfunction and melanogenesis. This review thus highlights the roles of phytochemicals potentially acting as Nrf2 inducers in improving photoaging, inflammation and hyperpigmentation via regulation of cellular homeostasis involved in skin integrity and function. Taken together, understanding the role of phytochemicals targeting Nrf2 in photoprotection could provide an insight into potential development of natural products as a promising strategy to delay skin photoaging and improve skin conditions.
Introduction
The skin is the largest organ in the body, and one of its main functions is to protect the body from environmental stressors including ultraviolet radiation (UVR), which can result in dermatological disorders, such as skin premature aging, abnormal pigmentation and inflammatory reactions. UVR has been generally known to have both beneficial and detrimental effects on human health. While UVR plays a role in natural synthesis of vitamin D, melanin, and various peptides in the skin that have positive biological implications (Slominski et al., 2000; Lin et al., 2016), excessive exposure to UVR can lead to acute and chronic adverse effects on the health of skin and is involved in the pathogenesis of premature aging (or photoaging) and increased risk of photocarcinogenesis. In addition, UVR is accepted as human carcinogen through oxidative mechanisms accountable for increased risk of developing skin cancers including non-melanoma and melanoma skin cancers (Tran et al., 2008). The incidence of skin cancer has dramatically risen in particular among fair-skinned populations, primarily due to lifestyle changes and increased recreational exposure to UVR including outdoor activities and sunbathing for cosmetic purposes (Narayanan et al., 2010). Both male and female independent of age are affected by dermatological concerns and increasingly interested in rejuvenation and skin cancer prevention. While the use of sunscreens is recommended in order to minimize the risk of photoaging and other UV-related skin disorders, sunscreen alone does not provide sufficient protection against deleterious effects of UVR. Oxidative stress plays a crucial role in UVR-induced photodamage via mediating multiple biological responses including apoptosis, DNA damage, mitochondrial dysfunction, inflammation, abnormal pigmentation and upregulation of matrix metalloproteinases (MMPs) (such as MMP-1) in related skin cell types including keratinocytes, fibroblasts and melanocytes (Liebel et al., 2012; Denat et al., 2014; Silva et al., 2017; Lohakul et al., 2021b). Therefore, cellular and molecular regulation of antioxidant defenses to combat oxidative stress and promote redox balance could be a potential therapeutic and preventive strategies for photodamaged skin.
Nuclear factor erythroid 2-related factor 2 (Nrf2) is an important transcription factor controlling antioxidant responses in various tissues including the skin and plays a major role in cytoprotection against chemical and environmental insults including UVR (Ikehata and Yamamoto, 2018). Targeting Nrf2 could improve photoaging, wound repair and dyspigmentation as well as prevent photocarcinogenesis via regulation of cellular homeostasis involved in skin integrity and function (Saw et al., 2011; Gegotek and Skrzydlewska, 2015). Phytochemicals, which are ubiquitously present in plant-based diets and are active ingredients in several botanical drugs, have thus gained remarkable attention as promising candidates for effective photoprotective agents due to their abilities to activate Nrf2 signaling-regulated redox balance and subsequently maintain cellular homeostasis involved in skin integrity and function. Much attention has been focused on the role of dietary polyphenols in the repair of photodamaged skin and prevention of solar-induced skin diseases (Surh and Na, 2008; Saw et al., 2011; Dunaway et al., 2018). In this review, we provide an overview of the promising roles of phytochemicals in mitigating UVR-induced skin damage via regulation of Nrf2-mediated antioxidant response to offer an insight into ethnopharmacological potential for development of novel and effective anti-photodamaging agents.
The Role of UVR-Induced Oxidative Stress in Skin Photodamage
The skin is a primary target of oxidative stress because it is constantly exposed to environment including UVR, which induces reactive oxygen species (ROS) generation in the skin. It has been well accepted that both UVA and UVB rays play a significant role in the premature aging and photodamage of the skin through various mechanisms involving oxidative stress (Lephart, 2016; Gegotek et al., 2017). While UVB has biological impact on the skin primarily by causing direct damage to DNA and inflammation (Halliday and Lyons, 2008), UVA accounts for skin photodamage by generating various types of ROS, such as superoxide anion radical (O2•-), singlet oxygen (1ΔgO2) and hydrogen peroxide (H2O2). ROS can interact with biomolecules and interfere with cell signaling, affecting cell survival and function of the skin cells (Dunaway et al., 2018). Considerable studies have reported that UVA exposure significantly led to ROS accumulation responsible for oxidative damage to biomolecules including DNA (O'Donovan et al., 2005), lipid (Dissemond et al., 2003) and protein in the skin cells including fibroblasts, keratinocytes (Brem et al., 2017) and melanocytes. Oxidative damage mediated by both UVA and UVB is associated with apoptosis and necrosis of the skin cells associated with sunburn reaction and photoaging process (Didier et al., 2001; Suschek et al., 2001; Kawachi et al., 2008; Parrado et al., 2016).
Skin Photoaging
Photoaging is characterized by epidermal thickness, termed hyperkeratosis, due to increased keratinocyte hyperproliferation as well as degradation or degeneration and disorganization of collagen fibers caused by upregulation of MMPs (Quan et al., 2009; Pittayapruek et al., 2016). In addition, dysregulated proliferation of transformed neoplastic keratinocytes or actinic keratosis is the key event in the progression from photoaged skin to squamous cell carcinoma (Berman and Cockerell, 2013). UVR (both UVA and UVB) is well accepted to play a vital role in photoaging via several mechanisms including DNA damage, oxidative stress, apoptosis, senescence, inflammation, immunomodulation (Rijken and Bruijnzeel-Koomen, 2011; Brand et al., 2017) and degradation and/or remodeling of the extracellular matrix (ECM) (Bosch et al., 2015). Generally, the characteristic hallmarks of photoaged skin are alterations in the ECM including accumulation of disorganized elastin fibers and depletion of collagens, the main structural proteins of the dermal connective tissues. Both UVA and UVB radiation can induce hyperkeratosis and several types of MMPs (including MMP-1 or collagenase) in mouse models of photoaging (Chaiprasongsuk et al., 2017; Misawa et al., 2017). Several in vitro and in vivo studies have reported that UV irradiation stimulates expression of MMP-1, MMP-3 and MMP-9, which are the major UV-inducible collagenolytic enzymes, regulated at the transcriptional level (Afaq et al., 2009; Quan et al., 2009; Pittayapruek et al., 2016). MMPs are co-expressed in response to various stimuli including oxidative insults, inflammatory cytokines and growth factors (Greenlee et al., 2007; Lee et al., 2021). MMPs are suggested to be downstream targets within signaling pathways of upstream response genes, which encode several signaling proteins that activate different transcription factors capable of binding the promoters of MMP genes. The key transcription-binding sites involved in the regulation of MMP genes include the activator protein-1 (AP-1) site, the nuclear factor kappa B (NF-κB) site and the signal transducer and activator of transcription (STAT) site (Fanjul-Fernandez et al., 2010). In addition, MMPs can be co-regulated because they share several transcription-binding sites in their promoter sequences. NF-κB and AP-1 are the transcription factors that can bind the promoters of MMP-1, 3 and 9 (Watanabe et al., 2004). The AP-1 transcription complex, a family of dimeric transcription factors composed of members of the Jun and Fos family proteins, is the main transcription factor regulating MMP-1 gene (Angel et al., 2001). In general, the c-Jun and c-Fos genes are activated rapidly and transiently in response to stimuli and are thus considered immediate-early response genes. Binding of heterodimer complexes of c-Jun with c-Fos to the AP-1 site, which is specific DNA sequences (5′-TGAG/CTCA-3′), termed TREs (TPA (tetradecanoylphorbol-12-Acetate)-response elements), is responsible for transactivation of AP-1 that regulates MMP-1, 3 and 9 expressions (Mackay et al., 1992; Watanabe et al., 2004). Both c-Jun and c-Fos are controlled by mitogen-activated protein kinase (MAPK) signaling pathways which are stimulated by extracellular stimuli including growth factors and cytokines as well as environmental stimuli including UVR. Three distinct types of MAPKs, ERK (extracellular signal-regulated kinase), JNK (c-Jun NH2-terminal kinase) and p38 MAPK, differentially affect AP-1 activity in response to various stimuli (Karin, 1995). The ERKs generally are triggered by growth factors and hormones as well as JNK and p38 MAPK are activated by environmental stresses including UVR and pro-inflammatory mediators, such as tumor necrosis factor (Chang and Karin, 2001; Silvers et al., 2003; Whitmarsh, 2007). UVR is suggested to primarily cause the greatest increases in JNK activity. Upon exposure to some stimuli, phosphorylation of c-Fos in the AP-1 complex at two C-terminal sites (Ser362 and Ser374) by MAPKs, in particular ERK, is required for transactivation at the specific AP-1 site (McBride and Nemer, 1998). In addition, c-Jun is activated and stabilized by JNK- and p38-catalyzed phosphorylation at the NH2-terminal sites (Ser63 and Ser73) located within transactivation domain of c-Jun. p38 MAPK indirectly activates AP-1 by phosphorylating other transcription factors such as AP-1 family proteins ATF2 (the activating transcription factor) forming a heterodimer with c-Jun, which then binds to the promoter elements in the c-Jun gene and regulates its transcription, leading to the subsequent upregulation of c-Jun expression and synthesis (Pramanik et al., 2003).
ROS participates in the photoaging process through several mechanisms including DNA damage, apoptosis, upstream modulation of MAPK/AP-1, NF-κB and JAK (janus kinase)-STAT signaling cascades, activation of cytokine and growth factor receptors and immune reaction of melanocytes and keratinocytes. Upregulation of MAPK/AP-1 signaling results in induction of transcription and production of MMPs (such as collagenase-1 (MMP-1), stromelysin-1 (MMP-3), and gelantinase A (MMP-2), that subsequently degrade ECM including collagen and elastin as well as suppress the collagen synthesis in the dermal fibroblasts. Furthermore, keratinocytes play an indirect role in photoaging through secreting paracrine factors, which stimulate the signaling cascades-mediated upregulation of MMPs in dermal fibroblasts. Several studies including ours suggested that ROS formation is involved in the molecular mechanisms of photoaging via activating MAPK/AP-1 signaling pathway, resulting in both upregulation of MMPs and downregulation of procollagen I production (Li et al., 2019). ROS induced by UVR is implicated in MAPKs-dependent activation of AP-1 signaling, leading to upregulation of various MMPs including MMP-1, MMP-3 and MMP-9 in both keratinocytes and fibroblasts (Rittie and Fisher, 2002; Pittayapruek et al., 2016). Our in vitro and in vivo studies demonstrated the role of ROS induced by UVA exposure in upregulation of MMP-1 through activation of MAPK/AP-1 signaling pathway in keratinocytes and mouse skin. In addition, UVB has been shown to trigger MMP-1 and MMP-3 expressions through ROS generation and MAPK/AP-1 activation in irradiated keratinocytes and fibroblasts (Kim et al., 2013; Kim et al., 2015; Lu et al., 2016). In addition, UVB radiation is suggested to induce ROS formation, leading to increased MMP-9 activity and expression in mouse embryonic fibroblasts and HaCaT keratinocytes (Chang et al., 2017; Ma et al., 2018). UVB-induced oxidative stress was observed to activate MAPK signaling in association with increased expression of MMP-9 in UVB-exposed dermal fibroblasts (Gunaseelan et al., 2017). Moreover, ROS was found to be involved in activation of p38 MAPK and induction of MMP-9 expression in UVB-exposed HaCaT keratinocytes (Li et al., 2017b) and mouse dermis (Li et al., 2017b). In addition to collagen and elastin degradation by MMPs, UVB plays a role in a reduction of procollagen type I synthesis through activating AP-1-mediated downregulation of transforming growth factor beta (TGF-β) signaling (Pittayapruek et al., 2016; Gao et al., 2018a).
It should also be taken into account that photoaging of the skin is a complex multifactorial process. Apart from ROS/MAPK/AP-1 signaling cascades, UVR which can activate various cell surface receptors can stimulate downstream signaling pathways that control other different transcription factors regulating expression of many genes involved in photoaging process. Eventually, it is important to inhibit ECM degradation that leads to solar scar, a process taking place with each exposure to UV even at low doses. Since ROS serve as important second messengers, which act upstream of MAPK/AP-1 signaling-mediated induction of MMPs and reduction of procollagen implicated in pathogenesis of photoaging, controlling ROS homeostasis could represent promising pharmacological and molecular approaches to impede photoaging.
Skin Inflammation and Skin Barrier Dysfunction
The skin barrier dysfunction and oxidative stress are suggested to play a role in the development of chronic inflammatory skin conditions (e.g., dermatitis) and alteration of wound healing process (Wikramanayake et al., 2014). The accumulation and alteration of external stimuli exposures result in a compromised barrier function of the skin through cutaneous inflammation and the imbalance of skin homeostasis (Egawa and Kabashima, 2018). Previous studies have demonstrated that UVB irradiation has a negative impact on epidermal morphology and barrier function by increasing stratum corneum (SC) thickness, causing changes in SC lipids and stimulating transepidermal water loss (Biniek et al., 2012). ROS generation induced by UVR plays a role in epidermal barrier dysfunction through oxidative damage to proteins and lipids, leading to alteration of tissue structure (Rinnerthaler et al., 2015). Regulation of Nrf2 activity has also been proposed to offer a potential strategy to improve skin barrier integrity by mitigating UVR-induced damage of keratinocytes and modulating inflammatory responses of the skin. Downregulation of Nrf2 signaling was shown to be involved in UVB-induced upregulation of pro-inflammatory mediators such as tumor necrosis factor alpha (TNF-α), cyclooxygenase-2 (COX-2), interleukin-6 (IL-6), interleukin-1 beta (IL-1β), and interleukin-8 (IL-8) in keratinocyte HaCaT cells (Park et al., 2021). A previous study using a mouse model of UVB-induced photodamage revealed that basal activity of Nrf2 in keratinocytes of normal skin is vital for improvement of skin barrier integrity and for prevention of skin carcinogenesis. UVB-mediated apoptosis of epidermal cells was involved in impaired skin integrity and activation of Nrf2 was observed to protect against UVB-induced apoptosis of basal keratinocytes in a paracrine, glutathione (GSH)/cysteine-dependent manner. Furthermore, enhanced levels of Nrf2-dependent genes in all layers of epidermis in response to UVB exposure were involved in the suppression of apoptosis in vivo (Schafer et al., 2010). The connection between the Nrf2 and antioxidant response element (ARE) system proved the protective pathways of skin inflammation via the regulation of the inflammatory factors (Saha et al., 2020). The NFE2L2 gene encoding for Nrf2 contains ARE-like sequences, providing a positive feedback mechanism to amplify antioxidant and anti-inflammatory signaling such as glutathione S-transferase (GST), NAD(P)H quinone oxidoreductase-1 (NQO-1), heme oxygenase-1 (HO-1) (Nguyen et al., 2009; Luo et al., 2018). Nrf2 has been suggested to play a role in modulating several signaling pathways involved in the inflammatory responses include NF-κB, MAPK, and JAK-STAT. Previous studies have reported the crosstalk between Nrf2 and NF-κB pathway. Nrf2 negatively regulated the NF-κB signaling pathway and proinflammatory cytokine production by inhibiting oxidative stress-induced NF-κB and preventing the IκB-α (NF-κB inhibitor) proteasomal degradation (Ma, 2013; Saha et al., 2020). In addition, several proinflammatory cytokines (e.g., IL-6, TNF-α and IL-1β), growth factors (e.g., epidermal growth factor (EGF), fibroblast growth factor, keratinocyte growth factor (KGF) and vascular endothelial growth factor (VEGF) and MMPs (e.g., MMP-2 and MMP-9) play a role in wound repair consisting of a series of multiple stages including inflammation, proliferation and remodeling (Shao et al., 2019; Suntar et al., 2021). Activation of Nrf2 in response to ROS production in inflamed tissues is thus suggested to play a role in promoting wound healing and regulating repair-related inflammation. Moreover, Nrf2 transcripts several genes encoding skin barrier structural and functional components including the keratins (KRT), the cornified envelope family members, small proline rich proteins, secretory leukocyte protease inhibitor, and the EGF family member epigen (Rojo de la Vega et al., 2017). In addition to Nrf2, STAT3 (in cell proliferation and differentiation), Smad proteins (in collagen production) and Forkhead box protein N1 (FOXN1) (in re-epithelization) are important transcriptional regulators involved in the process of wound repair. Furthermore, without involvement of inflammatory cells, upregulation of Nrf2 activity and its target antioxidant NQO-1 or HO-1 was demonstrated to promote the migration of corneal epithelial cells during wound repair in vitro and in vivo (Hayashi et al., 2013). In response to ROS produced in the early phase of wound repair, upregulation of Nrf2 as a target of KGF in keratinocytes is involved in the healing process in association with modulating proinflammatory cytokine IL-1, IL-6, and TNF-α and TGF-β1 and VEGF in vitro and in vivo (Braun et al., 2002). Nevertheless, the regulatory role of Nrf2 in epidermal homeostasis is complex and needs further clarification as prolonged activation of Nrf2 in keratinocytes could interfere skin homeostasis. Previous in vitro and in vivo studies demonstrated that increased activity of Nrf2 in keratinocytes resulted in epithelial abnormalities, altered epidermal barrier and development of hyperkeratosis (Kypriotou et al., 2012; Schafer et al., 2012).
Skin Hyperpigmentation
While melanin plays a crucial role in protecting the skin against harmful effects of UVR, excessive production of melanin could be detrimental because melanin precursors and intermediate metabolites produced during melanogenesis in response to UVR exert phototoxic properties (Schmitz et al., 1995). Whereas hyperpigmentation mediated by UV irradiation could reflect a sign of defensive response of the skin to stress, alteration in melanin synthesis may be implicated in skin damage, particularly in individuals with fair skin. UVR-dependent elevated melanogenesis has been suggested to be biologically harmful, genotoxic and contributed to development of melanoma skin cancer, especially in lightly pigmented individuals. The incidence of skin cancer has dramatically risen in particular among fair-skinned populations, primarily due to lifestyle changes and increased recreational exposure to UVR including outdoor activities and sunbathing for cosmetic purposes (Narayanan et al., 2010; D'Orazio et al., 2013; Watson et al., 2016). Furthermore, the growth of skin fairness products is dramatic particularly in Asia and Africa, although the use of skin bleaching products is associated with adverse side effects (Shroff et al., 2017). Thus, there is a need to develop effective and safe strategies for improvement of skin dyspigmentation or uneven complexion. Melanogenesis in melanocytes is a complex biosynthetic process involving the tyrosinase-catalyzed oxidation of tyrosine. Two main types of melanin, pheomelanin and eumelanin, are found in human skin and hair. Eumelanin is the brown/black insoluble pigment, characterizing dark phenotypes, and pheomelanin is the red/yellow, sulfur-containing pigment, predominating in red-haired individuals (Slominski et al., 2004). Eumelanin functions as a UV absorbent and subsequently has photoprotective action. Pheomelanin is photolabile and can produce ROS as by-products that lead to further DNA damage and is thus suggested to be carcinogenic following UVR (Brenner and Hearing, 2008).
Tyrosinase, a copper-containing membrane-bound located in melanosomes, catalyzes hydroxylation of L-tyrosine to L-DOPA, which is the first and the rate-limiting step of melanogenesis for both eumelanin and pheomelanin. In addition to tyrosinase, crucial enzymes involved in eumelanin synthesis include tyrosinase related proteins (TRP-1) and dopachrome tautomerase (DCT or TRP-2). Pheomelanin is produced via benzothiazine intermediates deriving from the oxidative polymerization of cysteinyl dopa derivatives generated through the condensation of the cysteine or GSH with the dopaquinone (Lu et al., 2021). Environmental stimuli (e.g., UVR and drugs), endogenous factors (e.g., hormone and mediators) and genetic factors can influence melanogenesis regulated by tyrosinase via various signal pathways, primarily the melanocortin 1 receptor (MC1R)-microphthalmia-associated transcription factor (MITF) signaling. MC1R is a G protein-coupled receptor that controls the quantity and quality of melanin synthesized in melanocytes. Important agonists of MC1-R acting as the main intrinsic regulator of pigmentation are peptide hormones and neuropeptides including stimulating hormone (α-MSH), endothelin-1 (ET-1) and adrenocorticotropic hormone (ACTH), which are cleavage products of proopiomelanocortin (POMC) (Lin and Fisher, 2007). The major signal transduction pathways that mediate the regulation of melanogenesis involve the binding of agonists to MC1R that trigger events inside melanocytes through raising intracellular cyclic 3′-5′-cyclic adenosine monophosphate (cAMP) and activating the adenylate cyclase enzyme, protein kinase A (PKA), leading to phosphorylation of the cAMP responsive binding element (CREB), which promotes the activation of MITF, which is the master transcription factor that regulates expression of several melanogenic genes including tyrosinase, TYRP1 and TYRP2. Moreover, upon activation of MC1R, enhanced levels of cAMP and subsequent activation of PKA were observed to activate the MAPK signaling cascades including p38, leading to activation of MITF (Smalley and Eisen, 2000). However, inhibition of MC1R in normal melanocytes and melanoma cells was observed to trigger PI3K/Akt and MAPK/ERK pathways, leading to inhibition of MITF and subsequent suppression of melanogenesis (Chae et al., 2017; Wu et al., 2018). Mechanisms underlying the role of phytochemicals in regulating pigmentation involve the direct suppression of tyrosinase activity and/or gene expression, direct scavenging of ROS, promotion of Nrf2-regulated antioxidant defense and inhibition of signaling pathways involved in inflammatory responses (Vomund et al., 2017; Boo, 2019). Nrf2 is suggested to play a role in modulating crucial signaling pathways including MAPK, PI3K/Akt and MC1R-MITF signaling cascades involved in regulation of melanin synthesis (Shin et al., 2014; Chaiprasongsuk et al., 2016). Moreover, exposure of the skin to UVR can stimulate keratinocytes to secrete hormones including ACTH, ET-1, α-MSH that bind to MC1R, activating MITF and upregulating melanogenesis-related proteins. Activation of Nrf2 has been observed to suppress the paracrine factors (such as α-MSH) derived from keratinocytes that results in downregulation of signaling pathways (including the cAMP/CREB/MITF pathway) involved in melanogenesis in melanocytes (Hseu et al., 2020; Chen SJ. et al., 2021). Therefore, application of compounds having abilities to activate Nrf2 might represent a promising approach to prevent and treat hyperpigmentation disorders.
Moreover, in response to UVR, melanogenesis acts as a shield against the harmful effect of UVR on the skin and thus approaches promoting melanin production can mitigate UVR-induced melanocyte damage. We previously demonstrated the role of Nrf2 in regulating the release of paracrine factor α-MSH by keratinocytes that influenced UVB-mediated melanocyte responses including DNA damage, oxidative stress, apoptosis and inflammation (Jeayeng et al., 2017). Several natural compounds such as flavonoids and coumarins having abilities to induce melanogenesis and restore melanocyte viability might thus be useful in the prevention and treatment of hypopigmentation disorders such as vitiligo (Niu and Aisa, 2017). Therefore, phytochemicals have been proposed to exert beneficial effects against abnormal melanogenesis via improving hyperpigmentation or hypopigmentation caused by disruption of melanocyte homeostasis and/or loss of functional melanocytes. While this review highlights the studies demonstrating the roles of phytochemicals in improving UVR-induced hyperpigmentation via Nrf2-dependent mechanisms, it should be taken into account that melanocyte biology is complex and the role of phytochemicals in regulating melanogenesis involved in maintaining the skin homeostasis needs further clarification.
The Role of Nrf2-Regulated Antioxidant Defense Against Cutaneous Photodamage
The primary endogenous antioxidant defenses include antioxidant and detoxification enzymes such as catalase, glutamate cysteine ligase (GCL) (composed of a catalytic subunit GCLC and a modifier subunit GCLM), the rate-limiting enzyme in GSH synthesis, glutathione peroxidase (GPx), GST, HO-1, NQO-1 and superoxide dismutase (SOD) regulated by various transcription factors including Nrf2 (Surh, 2003; Hseu et al., 2012). Nrf2 is a master regulator of antioxidant and cytoprotective genes involved in the human skin adaption to the environmental insults including UVR and thus plays a beneficial role in maintenance of skin homeostasis. Activity of Nrf2 is tightly regulated by proteins including Kelch-ECH associated protein 1 (Keap 1) and proteasome degradation system and thus regulation of Nrf2-mediated antioxidant response pathway is complicated. Under homeostatic conditions, two molecules of Keap1 bound to Nrf2 is responsible for the continuous ubiquitylation and degradation of Nrf2. In response to Nrf2 activating stimuli or oxidative stress, Keap1 is oxidized at critical cysteine residues, especially Cys151, leading to dissociation of Keap1-Nrf2 that allows Nrf2 to escape from Keap1-mediated ubiquitination. Nrf2 is then translocated into the nucleus and binds to the ARE promoter, a cis-acting enhancer sequence located in the 5′-flanking regions of genes encoding phase II and antioxidant cytoprotective enzymes including GST, NQO-1 and GCL (Schafer et al., 2010; Liu et al., 2016; Boo, 2020b).
Nrf2 plays a vital role in maintaining redox homeostasis and cellular metabolism in skin cells involved in the skin’s structural integrity and function (Ikehata and Yamamoto, 2018). Oxidative insults, such as UVR and H2O2, and electrophilic chemicals, such as butylated hydroxyanisole and its active de-methylated metabolite tert-butyl hydroquinone (tBHQ); phenolic flavonoids [e.g., green tea polyphenols and epigallocatechin-3-gallate (EGCG)]; and the naturally occurring isothiocyanates including sulforaphane (SFN) and curcumin, can stimulate Nrf2 activity via modification of Keap1 cysteine residues, suggested as the stress sensors for Nrf2 activator (Baird and Yamamoto, 2020). The cysteine modifications result in a conformational change in the associated motif of Keap1–Nrf2 that facilitates the dissociation of Nrf2 from Keap1 and subsequently Nrf2 nuclear translocation (Kong et al., 2001). Generally, various environmental stressors including UVR lead to post-translational activation of Nrf2 through Keap1 inactivation. The upregulation of Nrf2-mediated antioxidant defense system was demonstrated in vitro and in vivo to protect the human skin from harmful effects of UVR. UVA-1-mediated lipid oxidation induces expression of antioxidant response genes, which is dependent on the redox-regulated transcription factor Nrf2 in dermal fibroblasts (Gruber et al., 2010). Exposure of keratinocytes (including primary human epidermal keratinocytes and HaCaT keratinocyte cell lines) to UVA (20 J/cm2) increased Nrf2 activity via enhancing Keap1 expression. UVA exposure led to stimulation of Nrf2 activity and its target proteins (HO-1, NQO-1, GST) in HaCaT keratinocytes and dermal fibroblasts, although Nrf2 activity was minimally affected in UVA-irradiated primary keratinocytes (Rysava et al., 2020). In fact, the regulatory role of Nrf2 in skin cell survival and function affected by UVR is complex because UVR can either upregulate or downregulate Nrf2-mediated antioxidant defense in various skin cell types. Changes in the Nrf2 activity are dynamic and dependent on types of UV ray, UVR’s intensity and time following the exposure (Chaiprasongsuk et al., 2016; Rysava et al., 2020; Rysava et al., 2021). Previous observations indicate that both UVA and UVB downregulate Nrf2 antioxidant signaling pathway in skin keratinocytes, fibroblasts and melanocytes in vitro and in skin tissues in vivo. UVB exposure led to reduced expressions of Nrf2 and its target antioxidant HO-1 proteins in HaCaT keratinocyte cells and mouse skin in vivo (Rodriguez-Luna et al., 2018; Rodriguez-Luna et al., 2019). UVR was observed to downregulate antioxidant and detoxifying enzymes including GST, NQO-1 and γ-GCS (γ-glutamylcysteine synthetase) in the skin cells through modulating activity of Nrf2 (Kannan and Jaiswal, 2006; Lohakul et al., 2021b). The DNA damage or modulation of signaling cascades (including MAPKs) that take place rapidly in response to UVR exposure is suggested to mediate the downregulation of Nrf2 antioxidant response pathway (Lopez-Camarillo et al., 2012). The p38 was suggested to reduce Nrf2 nuclear translocation and its transcriptional activity (Boo, 2020b). In addition, activation of Nrf2 signaling has been suggested to protect against UVR-mediated skin damage via several mechanisms including promotion of antioxidant and cytoprotective defense, DNA repair, anti-inflammatory signaling. Upregulation of Nrf2/HO-1 signaling accompanied with increased activities and protein levels of catalase, GPx and SOD was observed to suppress apoptosis induced by UVR [UVA (3 J/cm2)+UVB (90 mJ/cm2)] via activating the PI3K/Akt signaling pathway in the 3D skin model (Xian et al., 2019). Thus, understanding the role of Nrf2 in the pathogenesis of skin photodamage could give an insight into development of potential compounds having Nrf2 inducing activity for prevention and treatment of skin photodamage.
Phytochemicals Targeting Nrf2 in Skin Photodamage: Development of Botanicals and Phytochemicals as Promising Photoprotective Agents
Several reports have highlighted the potential role of bioactive phytochemicals of plant-based diets and botanical drugs that have been used in ethnomedicine or reported in ethnopharmacological studies. The bioactive phytochemicals are naturally occurring compounds in botanical products including plants and botanical drugs which exert biological activities providing medical and nutritional benefit. These compounds exert antioxidant effects by directly scavenging ROS or by promoting the antioxidant defense system through the Nrf2-dependent pathway (L Suraweera et al., 2020). Phytochemicals include polyphenols and the non-phenolic phytochemicals. Phenolic compounds are classified into different groups on the basis of the number of phenolic rings they contain and of the structural elements binding the rings to one another. They are generally divided into four classes including phenolic acids, flavonoids, stilbenes and lignans (Figure 1). Phenolic acids are further classified as hydroxyl benzoic and hydroxyl cinnamic acid derivatives (Pandey and Rizvi, 2009). The natural sources of polyphenols include fruits (e.g., apple, berries, cherries, grapes, strawberries and pomegranate), vegetables, soybeans, cereals, tea, cocoa, soy and Phlebodium aureum (L.) J.Sm. The common flavonoids include catechins, quercetin, genistein, epicatechin, catechin and anthocyanins (Bosch et al., 2015). The common phenolic acids are hydroxycinnamic acids including caffeic acid and ferulic acid as well as gallic acid (also known as 3,4,5-trihydroxybenzoic acid). Gallic acid is the most abundant phenolic acid found in plant-based diets (Hano and Tungmunnithum, 2020). For non-flavonoid phenolics, the most widely studied stilbene is resveratrol. The commonly studied non-phenolic phytochemicals include carotenoids, caffeine and sulforaphane (SFN) (Bosch et al., 2015).
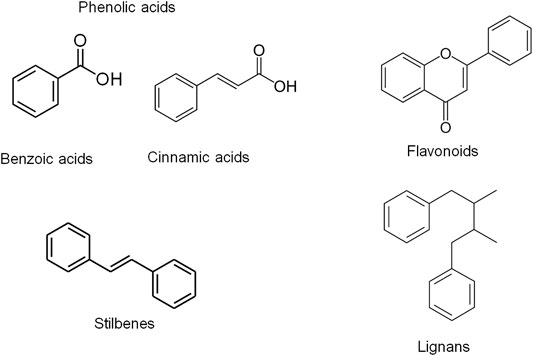
FIGURE 1. Chemical structures of the different classes of phenolic compounds including phenolic acids, flavonoids, stilbenes and lignans. Phenolic compounds are classified into different groups on the basis of the number of phenolic rings they contain and of the structural elements binding the rings to one another. They are generally divided into four classes including phenolic acids, flavonoids, stilbenes and lignans. Phenolic acids are further classified as hydroxyl benzoic and hydroxyl cinnamic acid derivatives (Johnsson, 2004; Nishiumi et al., 2011).
Phytochemicals play a crucial role in photoprotection against UVR-induced skin photodamage via UV-absorbing, antioxidant, melanin-modulating, anti-inflammatory properties Antioxidant phytochemicals have been demonstrated to mitigate skin photodamage in vitro and in vivo via directly scavenging ROS, promoting antioxidant defense capacity, modulating various signaling pathways involved in inflammation, controlling DNA repair, cellular viability and function of the skin (Boo, 2020a; Garg et al., 2020). This review focuses the photoprotective role of phytochemicals in UVR-mediated photoaging, hyperpigmentation and inflammation affecting skin barrier integrity via Nrf2-dependent pathway (Figure 2). The phytochemicals as electrophiles can promote cytoprotective proteins and antioxidant defenses via upregulating Nrf2 signaling. Keap1 and Cul3 comprise a unique ubiquitin E3 ligase responsible for degradation of Nrf2. Keap1 is a homodimeric protein belonging to the BTB (Broad complex, Tramtrack, Bric-á-brac)-Kelch family of proteins, which are named Kelch-like 1 to 42 (KLHL1–42). The BTB domain of Keap1 is necessary for Keap1 homodimerization and for mediating interactions with cul3/Rbx1 E3 ubiquitin ligase system. The BTB domain contains reactive cysteine residue responsible for interaction with electrophiles and thus plays a crucial role in sensing environmental electrophiles. Post-translational modifications of the highly reactive Cys 151 in Keap1 result in dimerization of Keap1, resulting in loss of Nrf2 ubiquitination, which stabilize the Nrf2 proteasomal degradation (Shin et al., 2020) and subsequent accumulation of Nrf2 and activation of the Nrf2-driven cytoprotective gene machinery (Cleasby et al., 2014). Phytochemicals, which are thiol-reactive electrophiles, covalently bind to the cysteine residue(s) in the dimerization domain of Keap1. Then, the activated ligase complex fails to degrade Nrf2, allowing the transcriptional activation of Nrf2 target genes (Yamamoto et al., 2008). Well-known Nrf2 activators including the isothiocyanate SFN, the alkylating agent iodoacetamide, tBHQ and diethylmaleate were demonstrated to modify C151 in Keap1, that mediates proteasomal degradation, leading to Nrf2 stabilization and enhancing its nuclear accumulation (Deshmukh et al., 2017; Dayalan Naidu et al., 2018; Dayalan Naidu and Dinkova-Kostova, 2020; Taguchi and Yamamoto, 2020).
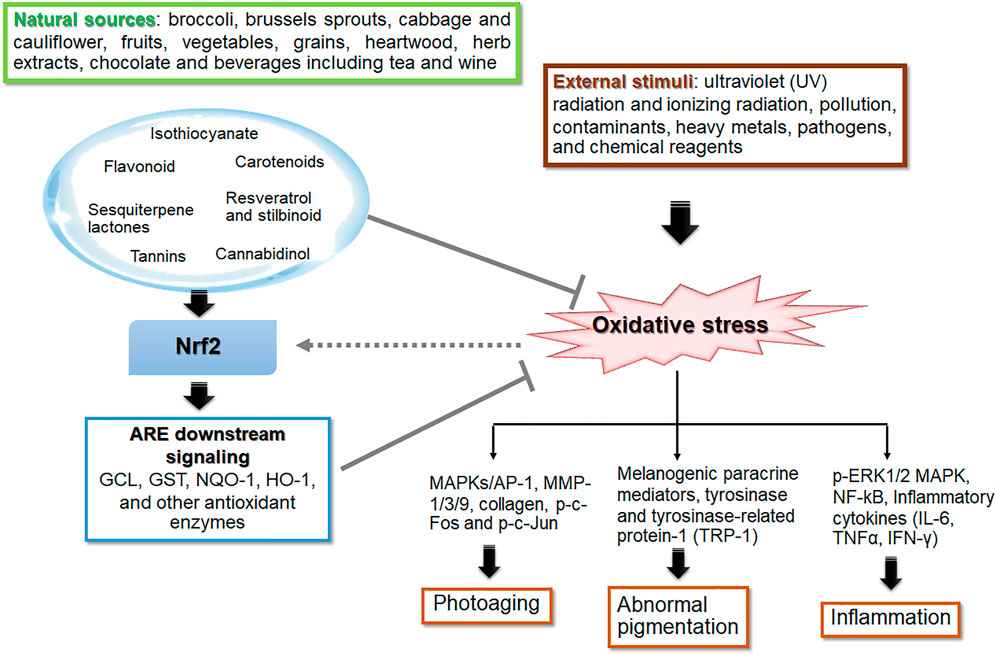
FIGURE 2. The role of phytochemicals in skin photoprotection via regulation of Nrf2 signaling. Phytochemicals are naturally occurring compounds in botanical products which exert biological activities providing medical and nutritional benefit. Oxidative stress, an imbalance in the redox state of the cell, is the result of cellular response to various stimuli including UV and ionizing radiation, pollution, contaminants, heavy metal, pathogens, and chemical reagents. Most of the natural antioxidants are derived from plant materials such as fruits, vegetables, grains, legumes, botanical drugs, spices and plant-based beverages (including tea, coffee, wine and cocoa). The bioactive phytochemicals, e.g., isothiocyanates (in broccoli, brussels sprouts, cabbage and cauliflower), flavonoids, carotenoids, resveratrol and stilbinoid, exhibit a wide range of photoprotective effects, including anti-photoaging, anti-inflammation, and anti-melanogenesis. These protective effects involve activation of nuclear factor erythroid 2-related factor 2 related to the antioxidant response element (Nrf2-ARE) signaling pathway that regulates expression of its downstream target genes including glutamate cysteine ligase (GCL), glutathione S-transferase (GST), NAD(P)H quinone oxidoreductase-1 (NQO-1), heme oxygenase-1 (HO-1) and other antioxidant genes, to cope with various stressors including UVR. The thick arrows and bar-headed lines mean activation and inhibition of the pathway, respectively. The dash arrow means modulation of Nrf2 signaling by ROS levels.
In addition to the role of phytochemicals as indirect antioxidants by upregulating antioxidant genes, phytochemicals can act as direct antioxidants by their ROS scavenging activities (Dinkova-Kostova and Talalay, 2008). Direct antioxidants, such as (–)-epicatechin-3-gallate and carotenoids (i.e., β-carotene and lycopene) can protect skin cells from ROS-induced damage to the skin cells. Their protective effects are short-lived and involve their abilities to neutralize ROS. ROS is involved in regulating the activity of Nrf2 via several mechanisms. Keap1 is considered as a cysteine-based sensor for a variety of endogenous and exogenous stressors including electrophiles and oxidants. ROS is involved in modulate Nrf2 activity via Keap-1-dependent and independent mechanisms. For Keap1-dependent mechanism, ROS (e.g., H2O2) has been demonstrated to promote Nrf2 activity via oxidative modification of Keap1 cysteines, leading to the release of Nrf2 and allowing its nuclear translocation (Espinosa-Diez et al., 2015; Suzuki et al., 2019). For Keap1-independent regulation of Nrf2 activity, MAPKs and glycogen synthase kinase-3 (GSK-3) are suggested to play a role in posttranslational modifications of Nrf2 via phosphorylation accountable for the alterations in its binding to the proteins involved in controlling Nrf2 stability and subsequent transcriptional activity (He et al., 2020). For instance, GSK-3 activation can stimulate Nrf2 nuclear export as well as ubiquitination and degradation, leading to downregulation of the Nrf2/ARE signaling pathway of brain ischemia and reperfusion injury (Chen et al., 2016). In addition, suppression of GSK-3 by activation of upstream phosphoinositide 3-kinase-protein kinase B/Akt (PI3K-PKB/Akt) results in Nrf2 stabilization. Activation of ERK leads to Nrf2 downregulation in diabetic hearts in response to oxidative stress (Tan et al., 2011). Thus, it is possible that ROS which can act as second messengers in protein kinase cascades also have a regulatory role in Nrf2 activity via Keap1-independent manner. Phytochemical polyphenols that act as both pro-oxidants through autoxidation to generate ROS (Babich et al., 2011) and direct antioxidants that can increase and decrease cellar ROS levels can affect Nrf2 activity via both Keap1-dependent and -independent manners.
In fact, skin is the largest body organ that is continuously exposed to environmental stressors including UVR. Oxidative stress induced by UVR plays a role in the stress responses of keratinocytes, melanocytes and fibroblasts responsible for photodamaged skin. Hence, development of phytochemicals that can activate the Nrf2 transcription factor is considered a promising pharmacological strategy to prevent and treat UVR-induced skin damage. The phytochemical derivatives that are effective for these photoprotective strategies include polyphenols, flavonoids, non-flavonoids and non-phenolic derivatives. The phytochemicals having ROS scavenging properties could suppress UVB-induced MMP-1 expression in HaCaT cells and human dermal fibroblasts and promote type I procollagen production in human dermal fibroblasts via downregulation of MAPK/AP-1 signaling cascades in association with upregulation of Nrf2 signaling (Kim et al., 2015). Moreover, botanical extracts including extracts of sunflower (Helianthus annuus L.) (Hwang et al., 2019), cherry blossoms (Li et al., 2018) and Foeniculum vulgare Mill. (Sun et al., 2016), which are rich sources of antioxidant phytochemicals, were observed to exert the protective effect against UVB-induced ROS formation, MMPs (MMP-1 and MMP-3) production and procollagen type I depletion via downregulation of MAPK signaling in association with upregulation of Nrf2 activity in human dermal fibroblasts. The anti-photoaging actions of the sunflower extract were also related to suppression of UVB-induced inflammatory cytokines including IL-6, COX-2, iNOS (inducible nitric oxide synthase), and TNF-α production (Hwang et al., 2019). In addition, the phytochemicals acting as direct or indirect Nrf2 inducers were demonstrated to exert the anti-photoaging effects via downregulation of MMPs including MMP-1 via MAPK/AP-1 signaling pathways in mouse skin (Sun et al., 2016; Li et al., 2018). Thus, indirect or direct targeting of Nrf2-dependent antioxidant response could offer a promising pharmacological strategy for prevention and inhibition of skin photodamage. The crosstalk between Nrf2 and other signaling pathways (e.g., MAPK/AP-1 pathway) involved in the mechanisms underlying the protective effects of phytochemicals on photodamaged skin were shown in Figure 3 (Kim et al., 2015; Sun et al., 2016; Li et al., 2018; Hwang et al., 2019; Garg et al., 2020). Several in vitro, in vivo and clinical studies showing the protective roles of botanicals and phytochemicals against photoaging, inflammation, skin barrier dysfunction and hyperpigmentation via Nrf2-dependent mechanisms are described below and summarized in Table 1.
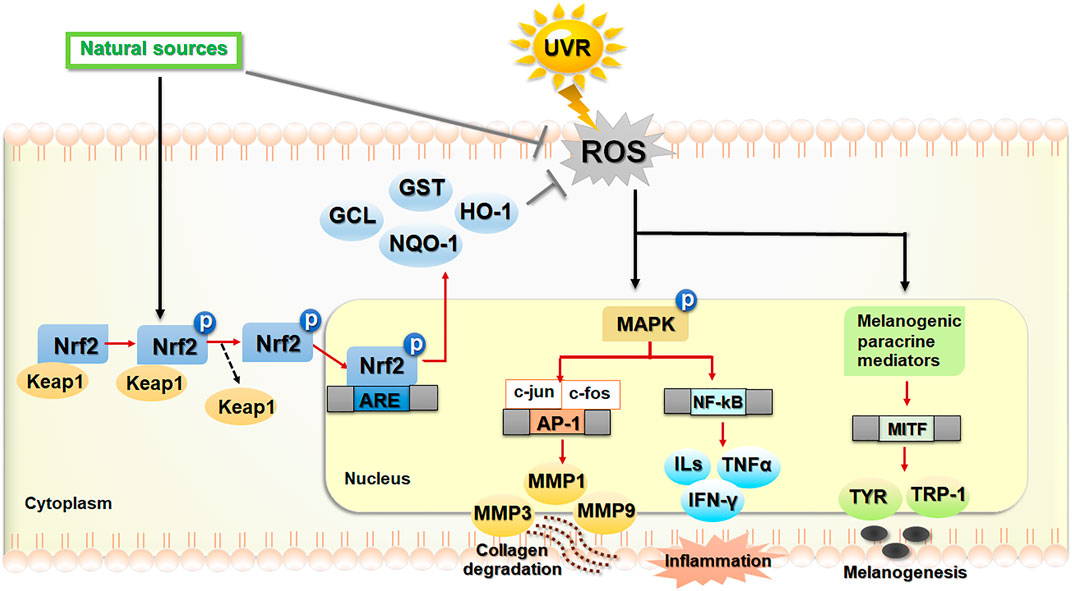
FIGURE 3. The regulatory mechanism of Nrf2-dependent antioxidant and cytoprotective actions against photoaging, inflammation and hyperpigmentation. Phytochemicals play a photoprotective role against skin photodamage directly via scavenging reactive oxygen species (ROS) and indirectly via activation of nuclear factor erythroid 2-related factor 2 (Nrf2) signaling, leading to upregulation of antioxidant and cytoprotective genes. In response to ultraviolet ray (UVR)-induced oxidative stress, ROS, produced in keratinocytes, melanocytes and fibroblasts, can modulate several related signaling pathways involved in photoaging, inflammation and melanogenesis. Exposure of human skin to UVR causes cells to produce ROS, which can modulate the signaling pathways involved in upregulation of matrix metalloproteinases (MMPs) which includes MMP-1/3/9, leading to collagen degradation, inflammation (nuclear factor kappa B also known as NF-κB and its downstream signaling) and the upregulation of melanogenesis-related genes including the microphthalmia-associated transcription factor (MITF), tyrosinase (TYR), and tyrosinase related proteins (TRP-1). Dietary phytochemicals as natural sources of antioxidants play the protective roles against UVR-induced ROS by the inhibition of ROS formation and the activation of Nrf2 signaling. In response to oxidative insults, Nrf2 is activated by the phosphorylation and disassociation of Nrf2 from Kelch-ECH associated protein 1 (Keap1), a repressor protein in the cytoplasm. Then, Nrf2 translocated to the nucleus binds to the ARE in the promoter region of downstream genes encoding antioxidant and phase II detoxifying enzymes including glutamate cysteine ligase (GCL), glutathione S-transferase (GST), NAD(P)H quinone oxidoreductase-1 (NQO-1), heme oxygenase-1 (HO-1). The activation of Nrf2 improves oxidative status of the cells and promotes cytoprotection against skin oxidative damage and inflammation. The black/red arrows and bar-headed lines mean activation and inhibition of the pathway, respectively. The dash arrow means dissociation of the Keap1–Nrf2 complex.
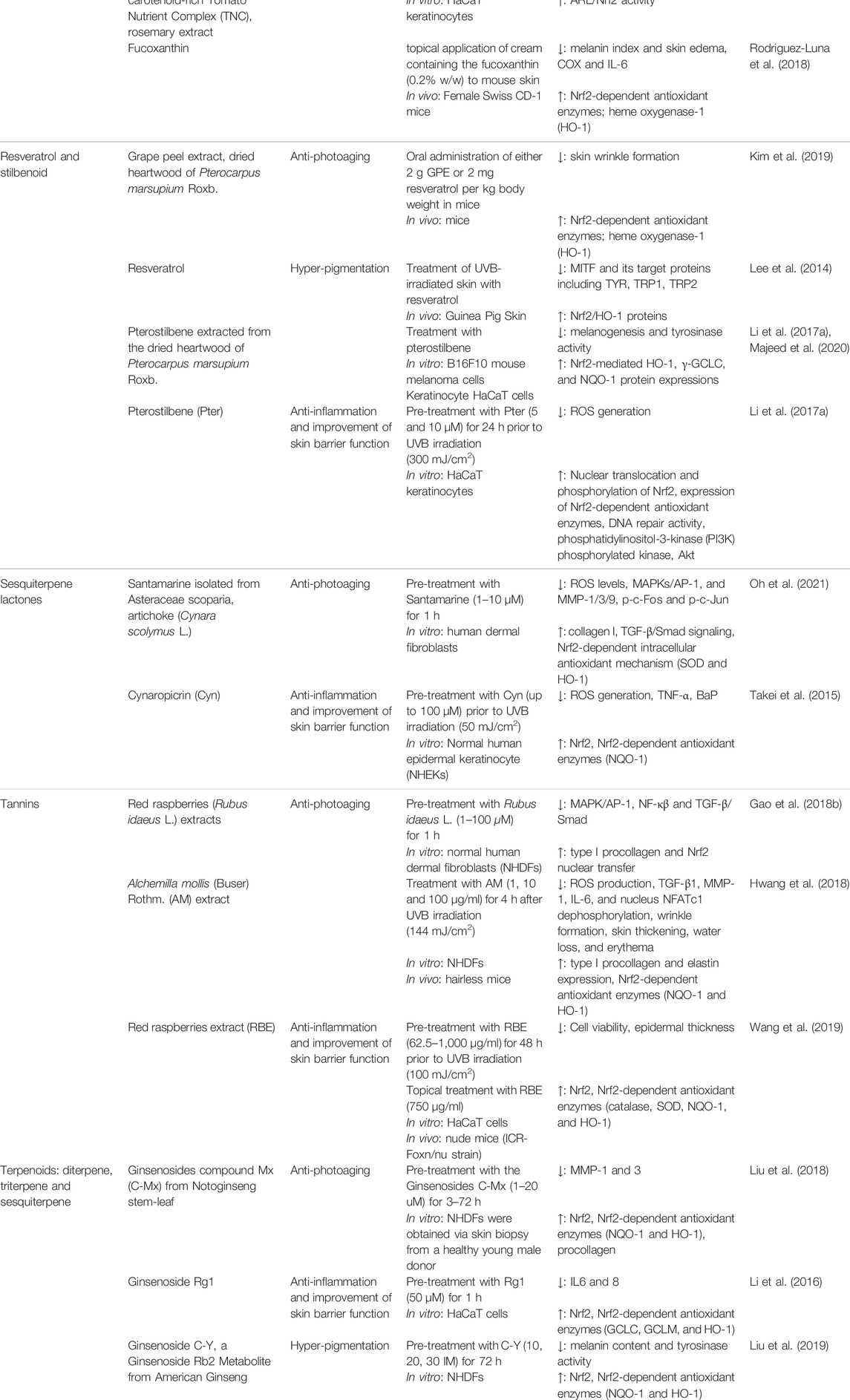
TABLE 1. The protective roles of botanicals and phytochemicals against photoaging, inflammation, skin barrier dysfunction and hyperpigmentation via Nrf2 regulation.
Isothiocyanates
Isothiocynates are sulfur-containing compounds having the general formula R–N=C=S and are commonly found in cruciferous vegetables from the Brassica genus including broccoli, brussels sprouts, cabbage and cauliflower. Members of isothiocyanates widely known as Nrf2 activators including SFN and phenethyl isothiocyanate (PEITC) protected human ex vivo full skin against UVR-induced sunburn cells, apoptosis and the decreased activity of the antioxidant enzyme catalase in correlation to upregulation of Nrf2 activity and its target genes (γ-GCS, HO-1, NQO-1) in HaCaT keratinocytes (Kleszczynski et al., 2013). SFN (0.6 μM/cm2) was observed to exert anti-photoaging effects on mouse skin via inhibition of MAPK/AP-1 signaling in UVA-irradiated mouse skin (Chaiprasongsuk et al., 2017). Treatment of keratinocyte cell line NCTC2544 with SFN (10 µM) combined with the Fernblock® XP (1 and 2 mg/ml), obtained from the tropical fern Phlebodium aureum (L.) J.Sm., substantially suppressed the production of MMP-1, MMP-3 and IL-1 in association with a decrease in ROS production. The SFN (5 and 10 µM) and Fernblock® XP (1 mg/ml) combination also showed inhibitory effects on melanoma cell growth and migration in vitro in association with the ability to inhibit the inflammatory microenvironment and neo-angiogenesis (Serini et al., 2020).
Benzyl isothiocyanate and 6-(Methylsulfinyl)hexyl isothiocyanate derived from Wasabi, have been reported to suppress a transcriptional levels of COX-2, an enzyme synthesizing the pro-inflammatory mediators (Lee et al., 2009; Uto et al., 2012). The disturbance of phosphorylated MAPKs signaling, ERK, p38 kinase, and JNK, was observed in the ITCs treatment, resulting in the downregulation of the transcription of inflammatory genes such as COX-2, iNOS, TNF-α, IL-1β, and IL-6 (Lee et al., 2009; Latronico et al., 2021). The effects of allyl-isothiocyanate and SFN on the Nrf2 nuclear translocation were associated with the downregulation of p65 protein, a subunit of the transcription factor NF-κB (Wagner et al., 2012). A previous study also suggested a connection between activation of Nrf2 and expression of keratin 16, a key intermediate cytoskeletal protein responsible for maintaining the skin barrier integrity in response to injury or inflammation. While genetic deletion of Nrf2 caused an early onset of hyperkeratotic lesions in Krt16 null mice which developed palmoplantar keratoma, topical treatment with SFN prevented the development of the skin lesions in footpad skin in association with restoring redox balance (Kerns et al., 2016).
Isothiocyanates including SFN and 7-methylsulfonylheptyl isothiocyanate (7-MSI), the sulfur-rich phytochemicals found in cruciferous vegetables, have been observed to exert anti-melanogenic effects via downregulation of MAPKs, the main regulatory pathways of melanogenesis (Shirasugi et al., 2010). 7-MSI treatment significantly reduced melanogenesis in B16F1 melanoma cells via activation of ERK signaling, leading to activation of autophagy and downregulation of MITF, tyrosinase and TRP-1 (Kim et al., 2021). Additionally, SFN exerted protective effects on particulate matter-induced melanogenesis via decreasing the release of paracrine factors by keratinocytes (Ko et al., 2020). Our previous study also revealed that the mechanisms underlying the anti-melanogenic effects of SFN involved the activation of Nrf2-mediated antioxidant response (Chaiprasongsuk et al., 2016).
Flavonoids
Flavonoids are most abundant polyphenols found in fruits, vegetables, grains, chocolate and beverages including tea and wine. This group has a common basic structure consisting of two aromatic rings bound together by three carbon atoms forming an oxygenated heterocycle. The flavonoids include flavanols (e.g., catechin, epicatechin), flavonols (e.g., quercetin, kaempferol, rutin), flavanones (e.g., hesperetin), flavones (e.g., apigenin, luteolin, hispidulin), isoflavones (e.g., daidzein, genistein) and anthocyanins (e.g., cyanidin) (Panche et al., 2016).
Rutin (Q-3-O-rutinoside) is a flavonol glycoside abundantly found in plants such as tea, onions, wine, apples and berries. Previous studies reported the protective role of rutin in aging on human dermal fibroblasts (HDFs) via upregulation of collagen type 1 and downregulation of MMP-1 mRNA in HDF. Application of the rutin-containing cream also improved skin elasticity as well as length and area of crow’s feet (Choi et al., 2016). Furthermore, the analysis of proteome profiles revealed that rutin treatment caused an induction of proteins involved in the antioxidant defenses and a reduction of proteins involved in the degradation of Nrf2 in UVB-irradiated dermal fibroblasts (Gegotek et al., 2018). Hesperidin, (hesperetin-7-rutinoside), and its aglycone, hesperetin, mostly found in citrus fruits and botanical drugs, have been demonstrated to provide in vitro and in vivo anti-photoaging effects on the skin via stimulating collagen synthesis in association with the antioxidant properties (Lohakul et al., 2021a). Citrus sinensis peel extract containing hesperidin loaded lipid nanoparticles showed photoprotective effects on UVR-mediated induction of MMP-1 via JNK signaling, reduction of collagen accompanied by decreased SOD protein production as well as stimulated inflammatory markers (COX-2, prostaglandin E2) and lipid oxidation product, malondialdehyde (MDA), in mouse skin (Amer et al., 2021). It was also suggested that the protective effect of a mixture of methylated derivatives of hesperidin on UVB-induced skin damage might involve the abilities to promote Nrf2 nuclear translocation and mRNA levels of its target gene GCLC and HO-1 in keratinocytes (Kuwano et al., 2015). In addition, a clinical trial showed that a 12-week topical application of a serum containing 0.1% hesperetin significantly promoted skin hydration and elasticity via enhancing the synthesis of hyaluronic acid (Sheen et al., 2021). Moreover, hesperetin and polyherbal formula extracts containing hesperetin topically applied to mouse skin before UVA exposure three times per week for 2 weeks (a total dose of 60 J/cm2) significantly attenuated MMP-1 upregulation and collagen depletion concomitant with promoting Nrf2 activity and the level of its target proteins (GST and NQO-1) as well as reducing 8-hydroxy-2′-deoxyguanosine (8-OHdG), a product of oxidatively damaged DNA damage, in irradiated mouse skin (Lohakul et al., 2021a). Grape fruit stem extract from Muscat Bailey A containing catechin, epicatechin and trans-resveratrol showed protective effects on UVB-induced destruction of collagen fiber through reduction of MMP-1 expression in association with a decrease in lipid peroxidation and restoration of GSH levels in mouse skin (Cho et al., 2018).
Furthermore, flavonoids consequently affect immune mechanisms that are essential in the development of the inflammatory processes (Maleki et al., 2019). Treatment of human epidermal keratinocytes (HaCaT cells) with 6-shogaol, an active ingredient of ginger, resulted in suppressing the UVB (180 mJ/cm2)-induced iNOS, COX-2 and TNF-α, which are the key mediators of inflammatory response, through modulating Nrf2 signaling (Wu et al., 2011; Du et al., 2018; Chen et al., 2019). In addition, the flavonoids have been suggested to exert anti-inflammatory actions in association with Nrf2 activation in vitro and in vivo. The mechanisms underlying the anti-inflammatory effects of flavonoids involved inhibition of production of pro-inflammatory cytokines including IL-33, TNF-α, IL-1β, IL-6 and downregulation of NF-κB activity (Staurengo-Ferrari et al., 2018). Gallocatechin-silver nanoparticle was observed to improve wound healing in diabetic rats via inhibiting TLR4/NF-κB inflammatory signaling pathway and modulating Nrf2/HO-1 pathway (Ni et al., 2015). The flavonol Galangin, obtained from Alpinia officinarum Hance and propolis extracts, was able to mitigate imiquimod-induced psoriasis-like skin inflammation in BALB/c mice via inhibiting pro-inflammatory mediators of COX-2, iNOS, NF-κB pathway and pro-inflammatory cytokines IL-17, IL-23, IL-1β in the skin as well as IL-6, TNF-α in both skin and serum. The anti-inflammatory effects of galangin were also associated with its ability to induce Nrf2 activity (Sangaraju et al., 2021). Recent evidence has revealed that the flavone luteolin improved impaired healing and promoted re-epithelization of skin wound in streptozotocin-induced diabetic rats via suppressing expressions of inflammatory proteins including MMP-9, TNF-α, IL-6, IL-1β and downregulating NF-κB in association with activation of Nrf2-dependent upregulation of antioxidant enzymes (Chen LY. et al., 2021). Topical application of a flavonoid fisetin (50 and 200 µM) for 10 weeks to mouse skin after UVB exposure was demonstrated to mitigate skin photodamage by inhibiting MMP-1 and MMP-2 protein expressions and collagen degradation as well as by improving skin barrier dysfunction. The fisetin treatment resulted in restoring skin hydration and barrier function in UVB-irradiated mouse skin through promoting contents of filaggrin, a structural protein in the stratum corneum, and aquaporins, integral epidermal cell membrane proteins, responsible for epidermal hydration and barrier function. The anti-photodamaging effects of fisetin are suggested to involve upregulation of Nrf2 and downregulation of pro-inflammatory mediators (COX-2, IL-6, and NF-κB) (Wu et al., 2017).
We previously observed that caffeic acid, ferulic acid, quercetin and rutin provided anti-melanogenic effects via enhancing Nrf2-mediated antioxidant defense responses in UVA-irradiated B16F10 cells (Chaiprasongsuk et al., 2016). Ellagic acid was shown to suppress α-MSH-induced melanin synthesis and tyrosinase activity by downregulating cAMP-mediated CREB and MITF signaling in B16F10 cells. Ellagic acid also had ability to suppress protein levels of the paracrine factors, POMC and α-MSH, through Nrf2 activation in keratinocyte HaCaT cells (Yang et al., 2021).
Licorice root extracts have traditionally been used for skin problems and are suggested as one the top cosmeceutical ingredients for hyperpigmentation (Searle et al., 2020). The root and rhizome extracts of licorice and several flavonoids identified as its bioactive ingredients have been suggested to provide beneficial effects on the skin through tyrosinase inhibitory activity, ROS scavenging activity, anti-inflammatory activity and Nrf2 inducing activity (Ciganovic et al., 2019). Glycyrrhiza flavonoids and licochalcone A, a major component of the licorice root extracts, showed the inhibitory effects on melanogenesis via activation of ERK and the subsequent downregulation of MITF/tyrosinase pathway in B16F10 cells. Isoliquiritigenin, a flavonoid component from the hydrolysis products of licorice root, was observed to exert anti-melanogenic effects on α-MSH-, ACTH- and UV-induced melanin synthesis and on melanocyte dendricity and melanosome transport through downregulation of melanogenic proteins including tyrosinase, TRP-1, DCT, Rab27a and Cdc42 in melanocytes (Lv et al., 2020).
Tannins
There are three major classes of tannins: condensed tannins (e.g., proanthocyanidins, flavonol-based compounds); hydrolyzable tannins (gallotannins and ellagitannins) and phlorotannins. Gallic acid, a chemical constituent of tannins, and its derivatives are found in almost all organ of a plant including bark, wood, leaf (in particular tea leaves), fruit, root and seed.
Red raspberries (Rubus idaeus L.) extracts containing high levels of anthocyanins and ellagitannins including Sanguiin H-6 and lambertianin C showed the protective effect on UVB-induced photoaging in normal human dermal fibroblasts (NHDFs). Treatment with the red raspberry extracts resulted in a significant reduction of MMPs secretion and production of pro-inflammatory mediator IL-6 possibly via downregulating MAPK, NF-κβ and AP-1 as well as increased procollagen type I production via activating the TGF-β/Smad pathway. The anti-photoaging effects of the tannin-rich botanical drugs involved promotion of Nrf2 activity and its target antioxidants including HO-1 and NQO-1 (Gao et al., 2018b). Alchemilla mollis (Buser) Rothm. ethanolic extract possessing gallic acid showed protective effects on UVB-induced photoaging in NHDFs and in mouse skin in vivo. Treatment with Alchemilla mollis (Buser) Rothm. ethanolic extract led to a substantial reduction in ROS formation as well as MMP-1 and IL-6 promotion through downregulating AP-1 activity in NHDFs exposed to UVB (144 mJ/cm2) irradiation. Furthermore, treatment with Alchemilla mollis (Buser) Rothm. extract and gallic acid protected against UVB-induced a reduction of type I procollagen levels in association with promotion of TGF-β1 in vitro and in vivo. Oral administration of Alchemilla mollis (Buser) Rothm. extract and gallic acid also improved UVB-induced wrinkle formation, skin dryness, epidermal thickening and collagen fiber density in hairless mice. The antioxidant mechanism underlying the anti-photoaging effects of Alchemilla mollis (Buser) Rothm. extract also involved the upregulation of Nrf2/HO-1 pathway (Hwang et al., 2018). Black tea (Fuzhuan-brick tea, rich in gallic acid and tea polyphenols) and gallic acid were also demonstrated to provide anti-photoaging effects via upregulation of Nrf2/HO-1 signaling in association with activation of MAPK signaling (p38 and ERK1/2 phosphorylation) in UVB-exposed keratinocyte HaCaT cells (Zhao et al., 2018). Cocoa phytochemicals including procyanidins and the flavanol catechin and epicatechin have been suggested to have several biological activities including antioxidant and anti-inflammatory activities possibly responsible for their beneficial effects on various age-related diseases including skin aging (Kim et al., 2014). Long term consumption of cocoa beverage for 12 weeks protected against UV-induced skin erythema and improved skin conditions (including erythema, skin roughness and scaling) in women (Heinrich et al., 2006). Procyanidins showed abilities to activate Nrf2 signaling in various in vitro and in vivo models (Truong et al., 2014). Therefore, it is possible that mechanisms underlying the anti-photoaging effects of procyanidins involve activation of Nrf2-regulated antioxidant defenses.
Phlorotannins (PTNs) are tannins found primarily in brown algae and play a role in protecting cells against UVR. PTNs applied topically attenuated radiation-induced inflammatory responses by downregulating NF-κB signaling and its downstream COX-2 and inflammasome activation in a mouse model of radiation dermatitis. PTNs also showed the abilities to promote wound healing process by enhancing aquaporin 3 involved in epidermal hydration and homeostasis. The mechanisms underlying the anti-inflammatory and wound healing-promoting effects of PTNs on irradiated mouse skin also involved upregulation of Nrf2/HO-1 signaling (Yang et al., 2020).
Gallic acid, gallotannin, valonia tannin and extracts of plants (e.g., Ceylon olive leaves and pomegranate peel) containing gallic acid exerted antimelanogenic effects directly by acting as a competitive inhibitor of tyrosinase and indirectly by inhibiting tyrosinase via antioxidant actions that affect melanogenesis pathway in vitro and in vivo (Chen et al., 2009; Panich et al., 2013; Su et al., 2013; Kanlayavattanakul et al., 2020; Huang et al., 2021; Liu et al., 2021). A randomized, double-blind, placebo-controlled clinical trial demonstrated that continuous administration of apple polyphenol rich in procyanidins for 12 weeks improved UV-induced skin pigmentation in heathy women (Shoji et al., 2020). Our previous study demonstrated that gallic acid protected against UVA (8 J/cm2)-induced melanogenesis via modulation of Nrf2 signaling and promotion of antioxidant defenses (including GSH, catalase, GPx and GST) in B16F10 melanoma cells (Onkoksoong et al., 2018).
Resveratrol and its Derivatives
Resveratrol was observed to provide photoprotective effects in UVB-induced photoaging via the antioxidant, anti-inflammatory and antiapoptotic actions in human keratinocyte HaCaT cells and ICR mice in vivo. The mechanism underlying its antiphotoaging actions involves upregulation of Nrf2 signaling and the antioxidant defenses (including HO-1, NQO-1, SOD1, GPx-4) in association with suppression of aging markers (MMP-1 and MMP-9) and proinflammatory mediators (IL-6, TNF-α, VEGF-B) by inhibiting ROS-mediated MAPK and COX-2 signaling cascades (Cui et al., 2022). Oral administration of grape peel extract and resveratrol exerted the anti-photoaging effects on UVB-induced skin wrinkle formation via promotion of Nrf2/HO-1 signaling cascades (Kim et al., 2019). In addition, a formulation containing 0.4% pterostilbene, the resveratrol analog, extracted from the dried heartwood of Pterocarpus marsupium Roxb., showed substantial reduction of aging markers and improvement of wrinkles, skin hydration elasticity in healthy volunteers (Majeed et al., 2020).
Topical treatment with pterostilbene, the resveratrol analog, suppressed an acute UVB radiation-induced skin inflammation and prevented chronic UVB radiation-mediated carcinogenesis in mice. The mechanisms involved in the photoprotective effects of pterostilbene might be attributed its ability to absorb UVB, protect against oxidative damage to DNA, protein and lipid, promote activities of antioxidant enzymes including catalase, SOD and GPx as well as activate Nrf2-dependent antioxidant response (Pastore et al., 2012; Sirerol et al., 2015). Moreover, resveratrol was observed to promote wound healing by restoring cell proliferation and migration, along with increased Nrf2 activity and Mn-SOD expression in the diabetic rat model (Zhou et al., 2021).
Resveratrol acting as a direct antioxidant, Nrf2 activator and tyrosinase inhibitor has been suggested to suppress melanogenesis (Wang et al., 2018; Boo, 2019). Resveratrol treatment led to a substantial reduction of UVB-induced melanogenesis via downregulation of MITF and its target proteins including TYR, TRP1, TRP2 in correspondence to upregulation of Nrf2/HO-1 proteins in melanocytes (Jian et al., 2011). Topical administration of 0.4% resveratryl triacetate and 0.4% resveratryl triglycolate twice daily for up to 8 weeks after the artificial pigmentation was shown to provide whitening efficacy in human subjects (Ryu et al., 2015; Jo et al., 2018). Pterostilbene, a stilbinoid, found in blueberries and grapes was demonstrated to exert anti-melanogenic effects via promotion of autophagy in melanocytes and downregulation of CREB (cAMP response element-binding protein)-MITF-tyrosinase pathway in B16F10 cells treated with HaCaT conditioned medium. The protective effects of pterostilbene on melanogenesis involved suppression of UVA-induced α-MSH expression and upregulation of Nrf2-mediated HO-1, γ-GCLC, and NQO-1 protein expressions in HaCaT cells (Hseu et al., 2021). Additionally, the application of a 0.4% formulation of natural pterostilbene for 4 and 8 weeks showed the skin brightening and anti-aging effects, respectively, in healthy volunteers in an open-label, single-arm, monocentric efficacy study (Majeed et al., 2020).
Carotenoids
Carotenoids, which belong to the tetraterpenes family, are liposoluble pigments responsible for the yellow, orange or red color of fruits, leaves and flowers. The carotenoids are divided into carotenes, xanthophylls and lycopene, and are abundantly present in tomato, carrots, pumpkin, seaweeds and algae (Milani et al., 2017). The main carotenoids including astaxanthin, canthaxanthin, β-carotene, lycopene and lutein have been suggested to exert photoprotective effects against UVR-induced skin photodamage via inhibition of inflammatory responses and photoaging biomarkers as well as promotion of antioxidant defense system in several in vitro, in vivo and clinical studies (Aust et al., 2005; Camera et al., 2009; Cavinato et al., 2017; Cooperstone et al., 2017; Kohandel et al., 2022). Previous reports have suggested that the carotenoids including astaxanthin and lycopene exert pharmacological activities including antioxidant, anti-inflammatory and chemopreventive activities against skin damage via activation of Nrf2 signaling (Wang et al., 2020; Ahn and Kim, 2021; Kohandel et al., 2021).
Dietary carotenoids combined with rosemary extract containing carnosic acid having abilities to activate Nrf2/ARE system exerted an inhibitory effect on UVB (20–60 mJ/cm2)-induced TNF-α and MMP-1 secretion from dermal fibroblasts (Calniquer et al., 2021). The tomato extracts rich in lycopene also protected against H2O2-induced photodamage of fibroblasts via promotion of pro-collagen secretion and suppression of apoptotic cell death and ROS formation (Darawsha et al., 2021).
Lutein, a xanthophyll carotenoid obtained from green leafy vegetables and egg yolk, has been reported to exert the anti-inflammatory effects via modulation of oxidant-sensitive inflammatory signaling pathways including NF-κB and STAT3 pathways and suppression of inflammatory cytokines (such as IL-1β, IL-6, TNF-α, COX-2, iNOS) (Ahn and Kim, 2021). Astaxanthin was demonstrated to exert anti-inflammatory effects by suppressing the expression of pro-inflammatory cytokines, for example, COX-2, LOX-1, NF-κB p65, TNF, and IL-1 (Kishimoto et al., 2010). Additionally, combinations of tomato nutrient complex containing lycopene and carotenoids and rosemary extract containing carnosic acid were observed to protect against UVB-induced oxidative stress, photoaging and inflammatory responses by inhibition of NF-κB activity and IL-6 production, along with activation of the ARE/Nrf2 system using HaCaT and KERTr keratinocyte cell lines (Calniquer et al., 2021). Bixin, an apocarotenoid isolated from the Achiote (Bixa Orellana L.), is one of the most consumed food colorants and topical preparations of seed extracts of the achiote have been in ethno-pharmacological use for treatment of wound healing and the pathologies related to epithelial barrier disruption. The mechanisms underlying the pharmacological actions of bioxin in improving skin barrier function were suggested to involve activation of Nrf2-mediated antioxidant systems including thioredoxin (TRX)/thioredoxin reductase (TXNRD1), regulation of peroxisome proliferator-activated receptors (PPARs), responsible for skin homeostasis and epithelial repair and modulation of Toll-like receptor 4 (TLR4)/NF-κB inflammatory signaling pathway (Rojo de la Vega et al., 2017). The marine carotenoid fucoxanthin (found in brown seaweeds, the microalgae and diatoms) showed anti-inflammatory actions through inhibiting proinflammatory cytokines including TNF-α, IL-6 and IL-8 levels as well as suppressing UVB-mediated oxidative stress in keratinocyte HaCaT cells. Moreover, topical application of cream containing the fucoxanthin to mouse skin protected against UVB-induced skin inflammation and hyperplasia via downregulation of COX-2 and iNOS, along with upregulation of Nrf2 activity and its target protein HO-1 (Rodriguez-Luna et al., 2018).
Terpenoids: Diterpene, Triterpene and Sesquiterpene
Santamarine, a sesquiterpene lactone, isolated from sunflower family provided anti-photoaging effects via suppression of UVA (8 J/cm2)-induced MAPK/AP-1 pathways involved in upregulation of MMPs and via promotion of TGF-β/Smad-mediated collagen production in HDFs. Furthermore, santamarine treatment led to a significant restoration of UVA-mediated downregulation of Nrf2-dependent antioxidant defenses including SOD-1 and HO-1 at the mRNA and protein levels (Oh et al., 2021). Zerumbone (ZER), a natural sesquiterpene, from Zingiber zerumbet (L.) Roscoe ex Sm. rhizomes was demonstrated to protect against UVA irradiation (3 J/cm2)-induced ROS formation, MMP-1 activity and collagen III degradation in HDFs. ZER was suggested to exert the anti-photoaging effects via downregulation of AP-1 activity and promotion of Nrf2/ARE pathway (Hseu et al., 2019). Furthermore, treatment of dermal fibroblasts with rosemary extracts rich in the diterpene carnosic acid having abilities to induce ARE/Nrf2 reporter activity protected against TNF-α-induced MMP-1 secretion (Calniquer et al., 2021). Ginsenosides, triterpene saponins, are major bioactive compounds responsible for pharmacological activities of Panax (ginseng), which has traditionally been used to treat and prevent various conditions associated with aging including skin aging. Previous studies demonstrated that the rare minor ginsenosides (C-Mc and Mx), which may act as potential antiphotoaging compounds, suppressed MMP production via regulating MAPK/AP-1/NF-κB pathway and promoted collagen production via the TGF-β/Smad pathway in association with upregulation of Nrf2 signaling in UVB-irradiated human dermal fibroblasts (Liu et al., 2018; Liu et al., 2022).
Cynaropicrin, a sesquiterpene lactone, is the major bioactive phytochemical in the artichoke (Cynara cardunculus L.) that can activate aryl hydrocarbon receptor (AhR), resulting in nuclear translocation of Nrf2. Activation of AhR/Nrf2/NQO-1 pathway by cynaropicrin was involved in its inhibitory effects on UVB-mediated production of proinflammatory cytokines including IL-1 and TNF-α in keratinocytes (Takei et al., 2015). Hemistepsin A, a sesquiterpene lactone isolated from Saussurea lyrata (Bunge) Franch., has been demonstrated to exert pharmacological actions including anti-inflammatory and antioxidant activities. Treatment of keratinocyte HaCaT cells with hemistepsin A protected against H2O2-induced cytotoxicity, DNA damage and apoptosis-mediated by mitochondrial dysfunction via upregulation of Nrf2/HO-1 signaling pathway (Park et al., 2020). The ginseng leaf extract rich in ginsenosides (including ginsenoside Rg1) applied topically to mouse skin protected against UVB-induced photoaging and skin barrier dysfunction through suppression of MMP-2, MMP-9 and MMP-13 protein expressions (Hong et al., 2017). Moreover, ginsenoside Rg1 showed anti-inflammatory effects against UVB-induced glucocorticoid resistance in keratinocyte HaCaT cells via promotion of Nrf2 activity (Li et al., 2016).
The sesquiterpene lactone eupalinolide A and B from Eupatorium lindleyanum DC. showed inhibitory effects against UVB-induced melanogenesis, skin damage and inflammatory responses in vitro and mouse skin in vivo (Yamashita et al., 2012). Previous evidence revealed that, apart from the anti-photoaging effect, a minor ginsenoside (C-Y) having ability to induce Nrf2 activity was observed to exert whitening effects by inhibiting melanin production, tyrosinase activity in Melan-a and zebrafish embryos (Liu et al., 2019).
Cannabidinol
Cannabidiol (CBD), the second most prevalent active ingredient in cannabis, is the non-psychoactive phytocannabinoid that has antioxidant and anti-inflammatory effects. CBD has been reported to provide photoprotective effects against UVA and UVB-induced damage to skin cells including NHDFs and keratinocyte HaCaT cells (Vacek et al., 2021). Treatment of 2D and 3D cultured fibroblasts with CBD caused a substantial attenuation in the levels of lipid peroxidation-derived aldehydes (4-hydroxynonenal (HNE), MDA and acrolein-protein adducts) in UVA (20 J/cm2)- and UVB (200 mJ/cm2)-irradiated cells (Gegotek et al., 2019). The formation of aldehyde-protein adducts induced by the highly reactive aldehydes could subsequently change the structure and/or function of several proteins including main ECM elastin in hairless mice exposed to UVA (Larroque-Cardoso et al., 2015). In addition, 4-HNE was suggested to play a role in UVA-induced fibroblast senescence in skin photoaging (Swiader et al., 2021). The compounds having abilities to neutralize aldehydes and inhibit the formation of protein adducts could thus have a beneficial role against skin photoaging.
Moreover, the analytical chemistry revealed that CBD compound could interact with the Nrf2/NF-κB transcriptional activity (Jastrzab et al., 2019). The biological effects of CBD have been found to maintain membrane integrity by preventing protein and phospholipid modifications (Atalay et al., 2020) and prevent the inflammatory responses (nuclear receptor coactivator-3 and paralemmin-3) (Atalay et al., 2021).
Conclusion and Future Challenges: Insight Into Ethnopharmacology
Ethnopharmacology is defined as “the interdisciplinary exploration of biologically active agents traditionally employed or observed by man” (Bruhn and Rivier, 2019). Identifying the ingredients and exploring the effects of the ingredients are crucial in the study of traditional medicine. Phytochemicals are bioactive compounds in plant-based products that have been historically used to rejuvenate the skin and alleviate skin disorders. Bioactive compounds of plant origin have thus been considered as invaluable sources of potential preventive or therapeutic agents for dermatological indications due to their pharmacological activities including antioxidant, UV absorption and anti-inflammation. The phytochemicals exert antioxidant effects by directly scavenging ROS or by promoting the antioxidant defense system through activation of Nrf2 signaling. It should also be taken into consideration that while Nrf2 plays a crucial role in maintaining cellular homeostasis under stress and inflammatory conditions, several studies have discussed a detrimental aspect of Nrf2 defined as the “dark side of Nrf2” in the cancer biology as enhanced Nrf2 activity is involved in a pro-carcinogenic effect and therapeutic resistance of cancer cells (Sporn and Liby, 2012). Thus, dietary phytochemicals having the potential to provide either chemopreventive or cancer-promotive properties, depending on the stage of carcinogenesis (L Suraweera et al., 2020). This review discusses the protective role of Nrf2 against UVR-induced skin photodamage and thus application of phytochemicals acting as Nrf2 activators is regarded as a promising strategy to prevent and treat premature aging and photodamage-related skin problems. Targeting Nrf2-dependent antioxidant and cytoprotective response has been suggested to represent a promising pharmacological strategy for development of effective and safe anti-photoaging and photoprotective agents. Furthermore, the therapeutic potential of phytochemicals can be limited by their poor bioavailability and thus development of drug delivery systems (such as nano-engineered formulations) is needed to improve efficacy of promising bioactive compounds as effective photoprotective agents.
Author Contributions
AC wrote the manuscript and created the graphical figure and tables; UP contributed to the design, the conception of the study and wrote the manuscript. All authors have read and approved the final manuscript.
Funding
This work was supported by the Thailand Research Fund (Grant Number: RSA6280101), the “Mahidol University” Grant; and the Chulabhorn Royal Academy. This work was financially supported by Office of the Permanent Secretary, Ministry of Higher Education, Science, Research and Innovation, “Research Grant for New Scholar (RGNS)”, Grant No. RGNS 63-246.
Conflict of Interest
The authors declare that the research was conducted in the absence of any commercial or financial relationships that could be construed as a potential conflict of interest.
Publisher’s Note
All claims expressed in this article are solely those of the authors and do not necessarily represent those of their affiliated organizations, or those of the publisher, the editors and the reviewers. Any product that may be evaluated in this article, or claim that may be made by its manufacturer, is not guaranteed or endorsed by the publisher.
References
Afaq, F., Zaid, M. A., Khan, N., Dreher, M., and Mukhtar, H. (2009). Protective Effect of Pomegranate-Derived Products on UVB-Mediated Damage in Human Reconstituted Skin. Exp. Dermatol. 18 (6), 553–561. doi:10.1111/j.1600-0625.2008.00829.x
Ahn, Y. J., and Kim, H. (2021). Lutein as a Modulator of Oxidative Stress-Mediated Inflammatory Diseases. Antioxidants (Basel) 10 (9). doi:10.3390/antiox10091448
Amer, R. I., Ezzat, S. M., Aborehab, N. M., Ragab, M. F., Mohamed, D., Hashad, A., et al. (2021). Downregulation of MMP1 Expression Mediates the Anti-aging Activity of Citrus Sinensis Peel Extract Nanoformulation in UV Induced Photoaging in Mice. Biomed. Pharmacother. 138, 111537. doi:10.1016/j.biopha.2021.111537
Angel, P., Szabowski, A., and Schorpp-Kistner, M. (2001). Function and Regulation of AP-1 Subunits in Skin Physiology and Pathology. Oncogene 20 (19), 2413–2423. doi:10.1038/sj.onc.1204380
Atalay, S., Dobrzyńska, I., Gęgotek, A., and Skrzydlewska, E. (2020). Cannabidiol Protects Keratinocyte Cell Membranes Following Exposure to UVB and Hydrogen Peroxide. Redox Biol. 36, 101613. doi:10.1016/j.redox.2020.101613
Atalay, S., Gęgotek, A., Wroński, A., Domigues, P., and Skrzydlewska, E. (2021). Therapeutic Application of Cannabidiol on UVA and UVB Irradiated Rat Skin. A Proteomic Study. J. Pharm. Biomed. Anal. 192, 113656. doi:10.1016/j.jpba.2020.113656
Aust, O., Stahl, W., Sies, H., Tronnier, H., and Heinrich, U. (2005). Supplementation with Tomato-Based Products Increases Lycopene, Phytofluene, and Phytoene Levels in Human Serum and Protects against UV-Light-Induced Erythema. Int. J. Vitam Nutr. Res. 75 (1), 54–60. doi:10.1024/0300-9831.75.1.54
Babich, H., Schuck, A. G., Weisburg, J. H., and Zuckerbraun, H. L. (2011). Research Strategies in the Study of the Pro-oxidant Nature of Polyphenol Nutraceuticals. J. Toxicol. 2011, 467305. doi:10.1155/2011/467305
Baird, L., and Yamamoto, M. (2020). The Molecular Mechanisms Regulating the KEAP1-NRF2 Pathway. Mol. Cell Biol 40 (13). doi:10.1128/MCB.00099-20
Berman, B., and Cockerell, C. J. (2013). Pathobiology of Actinic Keratosis: Ultraviolet-dependent Keratinocyte Proliferation. J. Am. Acad. Dermatol. 68 (1 Suppl. 1), S10–S19. doi:10.1016/j.jaad.2012.09.053
Biniek, K., Levi, K., and Dauskardt, R. H. (2012). Solar UV Radiation Reduces the Barrier Function of Human Skin. Proc. Natl. Acad. Sci. U S A. 109 (42), 17111–17116. doi:10.1073/pnas.1206851109
Boo, Y. C. (2020a). Emerging Strategies to Protect the Skin from Ultraviolet Rays Using Plant-Derived Materials. Antioxidants (Basel) 9 (7). doi:10.3390/antiox9070637
Boo, Y. C. (2019). Human Skin Lightening Efficacy of Resveratrol and its Analogs: From In Vitro Studies to Cosmetic Applications. Antioxidants (Basel) 8 (9). doi:10.3390/antiox8090332
Boo, Y. C. (2020b). Natural Nrf2 Modulators for Skin Protection. Antioxidants (Basel) 9 (9). doi:10.3390/antiox9090812
Bosch, R., Philips, N., Suárez-Pérez, J. A., Juarranz, A., Devmurari, A., Chalensouk-Khaosaat, J., et al. (2015). Mechanisms of Photoaging and Cutaneous Photocarcinogenesis, and Photoprotective Strategies with Phytochemicals. Antioxidants (Basel) 4 (2), 248–268. doi:10.3390/antiox4020248
Brand, R. M., Epperly, M. W., Stottlemyer, J. M., Skoda, E. M., Gao, X., Li, S., et al. (2017). A Topical Mitochondria-Targeted Redox-Cycling Nitroxide Mitigates Oxidative Stress-Induced Skin Damage. J. Invest. Dermatol. 137 (3), 576–586. doi:10.1016/j.jid.2016.09.033
Braun, S., Hanselmann, C., Gassmann, M. G., auf dem Keller, U., Born-Berclaz, C., Chan, K., et al. (2002). auf dem Keller, UNrf2 transcription factor, a novel target of keratinocyte growth factor action which regulates gene expression and inflammation in the healing skin wound. Mol. Cell Biol 22 (15), 5492–5505. doi:10.1128/MCB.22.15.5492-5505.2002
Brem, R., Macpherson, P., Guven, M., and Karran, P. (2017). Oxidative Stress Induced by UVA Photoactivation of the Tryptophan UVB Photoproduct 6-Formylindolo[3,2-B]carbazole (FICZ) Inhibits Nucleotide Excision Repair in Human Cells. Sci. Rep. 7 (1), 4310. doi:10.1038/s41598-017-04614-8
Brenner, M., and Hearing, V. J. (2008). The Protective Role of Melanin against UV Damage in Human Skin. Photochem. Photobiol. 84 (3), 539–549. doi:10.1111/j.1751-1097.2007.00226.x
Bruhn, J. G., and Rivier, L. (2019). Ethnopharmacology - A Journal, a Definition and a Society. J. Ethnopharmacol 242, 112005. doi:10.1016/j.jep.2019.112005
Calniquer, G., Khanin, M., Ovadia, H., Linnewiel-Hermoni, K., Stepensky, D., Trachtenberg, A., et al. (2021). Combined Effects of Carotenoids and Polyphenols in Balancing the Response of Skin Cells to UV Irradiation. Molecules 26 (7). doi:10.3390/molecules26071931
Camera, E., Mastrofrancesco, A., Fabbri, C., Daubrawa, F., Picardo, M., Sies, H., et al. (2009). Astaxanthin, Canthaxanthin and Beta-Carotene Differently Affect UVA-Induced Oxidative Damage and Expression of Oxidative Stress-Responsive Enzymes. Exp. Dermatol. 18 (3), 222–231. doi:10.1111/j.1600-0625.2008.00790.x
Cavinato, M., Waltenberger, B., Baraldo, G., Grade, C. V. C., Stuppner, H., and Jansen-Dürr, P. (2017). Plant Extracts and Natural Compounds Used against UVB-Induced Photoaging. Biogerontology 18 (4), 499–516. doi:10.1007/s10522-017-9715-7
Chae, J. K., Subedi, L., Jeong, M., Park, Y. U., Kim, C. Y., Kim, H., et al. (2017). Gomisin N Inhibits Melanogenesis through Regulating the PI3K/Akt and MAPK/ERK Signaling Pathways in Melanocytes. Int. J. Mol. Sci. 18 (2). doi:10.3390/ijms18020471
Chaiprasongsuk, A., Lohakul, J., Soontrapa, K., Sampattavanich, S., Akarasereenont, P., and Panich, U. (2017). Activation of Nrf2 Reduces UVA-Mediated MMP-1 Upregulation via MAPK/AP-1 Signaling Cascades: The Photoprotective Effects of Sulforaphane and Hispidulin. J. Pharmacol. Exp. Ther. 360 (3), 388–398. doi:10.1124/jpet.116.238048
Chaiprasongsuk, A., Onkoksoong, T., Pluemsamran, T., Limsaengurai, S., and Panich, U. (2016). Photoprotection by Dietary Phenolics against Melanogenesis Induced by UVA through Nrf2-dependent Antioxidant Responses. Redox Biol. 8, 79–90. doi:10.1016/j.redox.2015.12.006
Chang, L., and Karin, M. (2001). Mammalian MAP Kinase Signalling Cascades. Nature 410 (6824), 37–40. doi:10.1038/35065000
Chang, T. M., Tsen, J. H., Yen, H., Yang, T. Y., and Huang, H. C. (2017). Extract from Periostracum Cicadae Inhibits Oxidative Stress and Inflammation Induced by Ultraviolet B Irradiation on HaCaT Keratinocytes. Evid. Based Complement. Alternat Med. 2017, 8325049. doi:10.1155/2017/8325049
Chen, F., Tang, Y., Sun, Y., Veeraraghavan, V. P., Mohan, S. K., and Cui, C. (2019). 6-shogaol, a Active Constiuents of Ginger Prevents UVB Radiation Mediated Inflammation and Oxidative Stress through Modulating NrF2 Signaling in Human Epidermal Keratinocytes (HaCaT Cells). J. Photochem. Photobiol. B 197, 111518. doi:10.1016/j.jphotobiol.2019.111518
Chen, L. G., Chang, W. L., Lee, C. J., Lee, L. T., Shih, C. M., and Wang, C. C. (2009). Melanogenesis Inhibition by Gallotannins from Chinese Galls in B16 Mouse Melanoma Cells. Biol. Pharm. Bull. 32 (8), 1447–1452. doi:10.1248/bpb.32.1447
Chen, L. Y., Cheng, H. L., Kuan, Y. H., Liang, T. J., Chao, Y. Y., and Lin, H. C. (2021a). Therapeutic Potential of Luteolin on Impaired Wound Healing in Streptozotocin-Induced Rats. Biomedicines 9 (7). doi:10.3390/biomedicines9070761
Chen, S. J., Hseu, Y. C., Gowrisankar, Y. V., Chung, Y. T., Zhang, Y. Z., Way, T. D., et al. (2021b). The Anti-melanogenic Effects of 3-O-Ethyl Ascorbic Acid via Nrf2-Mediated α-MSH Inhibition in UVA-Irradiated Keratinocytes and Autophagy Induction in Melanocytes. Free Radic. Biol. Med. 173, 151–169. doi:10.1016/j.freeradbiomed.2021.07.030
Chen, X., Liu, Y., Zhu, J., Lei, S., Dong, Y., Li, L., et al. (2016). GSK-3β Downregulates Nrf2 in Cultured Cortical Neurons and in a Rat Model of Cerebral Ischemia-Reperfusion. Sci. Rep. 6, 20196. doi:10.1038/srep20196
Cho, B. O., Che, D. N., Shin, J. Y., Kang, H. J., and Jang, S. I. (2018). Ameliorative Effects of Fruit Stem Extract from Muscat Bailey A against Chronic UV-Induced Skin Damage in BALB/c Mice. Biomed. Pharmacother. 97, 1680–1688. doi:10.1016/j.biopha.2017.12.003
Choi, S. J., Lee, S. N., Kim, K., Joo, da. H., Shin, S., Lee, J., et al. (2016). Biological Effects of Rutin on Skin Aging. Int. J. Mol. Med. 38 (1), 357–363. doi:10.3892/ijmm.2016.2604
Ciganović, P., Jakimiuk, K., Tomczyk, M., and Zovko Končić, M. (2019). Glycerolic Licorice Extracts as Active Cosmeceutical Ingredients: Extraction Optimization, Chemical Characterization, and Biological Activity. Antioxidants 8 (10), 445. doi:10.3390/antiox8100445
Cleasby, A., Yon, J., Day, P. J., Richardson, C., Tickle, I. J., Williams, P. A., et al. (2014). Structure of the BTB Domain of Keap1 and its Interaction with the Triterpenoid Antagonist CDDO. PLoS One 9 (6), e98896. doi:10.1371/journal.pone.0098896
Cooperstone, J. L., Tober, K. L., Riedl, K. M., Teegarden, M. D., Cichon, M. J., Francis, D. M., et al. (2017). Tomatoes Protect against Development of UV-Induced Keratinocyte Carcinoma via Metabolomic Alterations. Sci. Rep. 7 (1), 5106. doi:10.1038/s41598-017-05568-7
Cui, B., Wang, Y., Jin, J., Yang, Z., Guo, R., Li, X., et al. (2022). Resveratrol Treats UVB-Induced Photoaging by Anti-MMP Expression, through Anti-inflammatory, Antioxidant, and Antiapoptotic Properties, and Treats Photoaging by Upregulating VEGF-B Expression. Oxid Med. Cell Longev 2022, 6037303. doi:10.1155/2022/6037303
D'Orazio, J., Jarrett, S., Amaro-Ortiz, A., and Scott, T. (2013). UV Radiation and the Skin. Int. J. Mol. Sci. 14 (6), 12222–12248. doi:10.3390/ijms140612222
Darawsha, A., Trachtenberg, A., Levy, J., and Sharoni, Y. (2021). The Protective Effect of Carotenoids, Polyphenols, and Estradiol on Dermal Fibroblasts under Oxidative Stress. Antioxidants (Basel) 10 (12). doi:10.3390/antiox10122023
Dayalan Naidu, S., and Dinkova-Kostova, A. T. (2020). KEAP1, a Cysteine-Based Sensor and a Drug Target for the Prevention and Treatment of Chronic Disease. Open Biol. 10 (6), 200105. doi:10.1098/rsob.200105
Dayalan Naidu, S., Muramatsu, A., Saito, R., Asami, S., Honda, T., Hosoya, T., et al. (2018). C151 in KEAP1 Is the Main Cysteine Sensor for the Cyanoenone Class of NRF2 Activators, Irrespective of Molecular Size or Shape. Sci. Rep. 8 (1), 8037. doi:10.1038/s41598-018-26269-9
Denat, L., Kadekaro, A. L., Marrot, L., Leachman, S. A., and Abdel-Malek, Z. A. (2014). Melanocytes as Instigators and Victims of Oxidative Stress. J. Invest. Dermatol. 134 (6), 1512–1518. doi:10.1038/jid.2014.65
Deshmukh, P., Unni, S., Krishnappa, G., and Padmanabhan, B. (2017). The Keap1-Nrf2 Pathway: Promising Therapeutic Target to Counteract ROS-Mediated Damage in Cancers and Neurodegenerative Diseases. Biophys. Rev. 9 (1), 41–56. doi:10.1007/s12551-016-0244-4
Didier, C., Kerblat, I., Drouet, C., Favier, A., Béani, J. C., and Richard, M. J. (2001). Induction of Thioredoxin by Ultraviolet-A Radiation Prevents Oxidative-Mediated Cell Death in Human Skin Fibroblasts. Free Radic. Biol. Med. 31 (5), 585–598. doi:10.1016/s0891-5849(01)00617-7
Dinkova-Kostova, A. T., and Talalay, P. (2008). Direct and Indirect Antioxidant Properties of Inducers of Cytoprotective Proteins. Mol. Nutr. Food Res. 52 Suppl 1 (Suppl. 1), S128–S138. doi:10.1002/mnfr.200700195
Dissemond, J., Schneider, L. A., Brenneisen, P., Briviba, K., Wenk, J., Wlaschek, M., et al. (2003). Protective and Determining Factors for the Overall Lipid Peroxidation in Ultraviolet A1-Irradiated Fibroblasts: In Vitro and In Vivo Investigations. Br. J. Dermatol. 149 (2), 341–349. doi:10.1046/j.1365-2133.2003.05457.x
Du, D., Yao, L., Zhang, R., Shi, N., Shen, Y., Yang, X., et al. (2018). Protective Effects of Flavonoids from Coreopsis Tinctoria Nutt. On Experimental Acute Pancreatitis via Nrf-2/ARE-Mediated Antioxidant Pathways. J. Ethnopharmacol 224, 261–272. doi:10.1016/j.jep.2018.06.003
Dunaway, S., Odin, R., Zhou, L., Ji, L., Zhang, Y., and Kadekaro, A. L. (2018). Natural Antioxidants: Multiple Mechanisms to Protect Skin from Solar Radiation. Front. Pharmacol. 9, 392. doi:10.3389/fphar.2018.00392
Egawa, G., and Kabashima, K. (2018). Barrier Dysfunction in the Skin Allergy. Allergol. Int. 67 (1), 3–11. doi:10.1016/j.alit.2017.10.002
Espinosa-Diez, C., Miguel, V., Mennerich, D., Kietzmann, T., Sánchez-Pérez, P., Cadenas, S., et al. (2015). Antioxidant Responses and Cellular Adjustments to Oxidative Stress. Redox Biol. 6, 183–197. doi:10.1016/j.redox.2015.07.008
Fanjul-Fernández, M., Folgueras, A. R., Cabrera, S., and López-Otín, C. (2010). Matrix Metalloproteinases: Evolution, Gene Regulation and Functional Analysis in Mouse Models. Biochim. Biophys. Acta 1803 (1), 3–19. doi:10.1016/j.bbamcr.2009.07.004
Gao, W., Lin, P., Hwang, E., Wang, Y., Yan, Z., Ngo, H. T. T., et al. (2018a). Pterocarpus Santalinus L. Regulated Ultraviolet B Irradiation-Induced Procollagen Reduction and Matrix Metalloproteinases Expression through Activation of TGF-β/Smad and Inhibition of the MAPK/AP-1 Pathway in Normal Human Dermal Fibroblasts. Photochem. Photobiol. 94 (1), 139–149. doi:10.1111/php.12835
Gao, W., Wang, Y.-s., Hwang, E., Lin, P., Bae, J., Seo, S. A., et al. (2018b). Rubus Idaeus L. (Red Raspberry) Blocks UVB-Induced MMP Production and Promotes Type I Procollagen Synthesis via Inhibition of MAPK/AP-1, NF-Κβ and Stimulation of TGF-β/Smad, Nrf2 in normal Human Dermal Fibroblasts. J. Photochem. Photobiol. B: Biol. 185, 241–253. doi:10.1016/j.jphotobiol.2018.06.007
Garg, C., Sharma, H., and Garg, M. (2020). Skin Photo-protection with Phytochemicals against Photo-Oxidative Stress, Photo-Carcinogenesis, Signal Transduction Pathways and Extracellular Matrix Remodeling-An Overview. Ageing Res. Rev. 62, 101127. doi:10.1016/j.arr.2020.101127
Gęgotek, A., Atalay, S., Domingues, P., and Skrzydlewska, E. (2019). The Differences in the Proteome Profile of Cannabidiol-Treated Skin Fibroblasts Following UVA or UVB Irradiation in 2D and 3D Cell Cultures. Cells 8 (9). doi:10.3390/cells8090995
Gęgotek, A., Domingues, P., and Skrzydlewska, E. (2018). Proteins Involved in the Antioxidant and Inflammatory Response in Rutin-Treated Human Skin Fibroblasts Exposed to UVA or UVB Irradiation. J. Dermatol. Sci. 90 (3), 241–252. doi:10.1016/j.jdermsci.2018.02.002
Gęgotek, A., Rybałtowska-Kawałko, P., and Skrzydlewska, E. (2017). Rutin as a Mediator of Lipid Metabolism and Cellular Signaling Pathways Interactions in Fibroblasts Altered by UVA and UVB Radiation. Oxidative Med. Cell Longevity 2017, 1–20. doi:10.1155/2017/4721352
Gęgotek, A., and Skrzydlewska, E. (2015). The Role of Transcription Factor Nrf2 in Skin Cells Metabolism. Arch. Dermatol. Res. 307 (5), 385–396. doi:10.1007/s00403-015-1554-2
Greenlee, K. J., Werb, Z., and Kheradmand, F. (2007). Matrix Metalloproteinases in Lung: Multiple, Multifarious, and Multifaceted. Physiol. Rev. 87 (1), 69–98. doi:10.1152/physrev.00022.2006
Gruber, F., Mayer, H., Lengauer, B., Mlitz, V., Sanders, J. M., Kadl, A., et al. (2010). NF-E2-related Factor 2 Regulates the Stress Response to UVA-1-Oxidized Phospholipids in Skin Cells. FASEB J. 24 (1), 39–48. doi:10.1096/fj.09-133520
Gunaseelan, S., Balupillai, A., Govindasamy, K., Ramasamy, K., Muthusamy, G., Shanmugam, M., et al. (2017). Linalool Prevents Oxidative Stress Activated Protein Kinases in Single UVB-Exposed Human Skin Cells. PLoS One 12 (5), e0176699. doi:10.1371/journal.pone.0176699
Halliday, G. M., and Lyons, J. G. (2008). Inflammatory Doses of UV May Not Be Necessary for Skin Carcinogenesis. Photochem. Photobiol. 84 (2), 272–283. doi:10.1111/j.1751-1097.2007.00247.x
Hano, C., and Tungmunnithum, D. (2020). Plant Polyphenols, More Than Just Simple Natural Antioxidants: Oxidative Stress, Aging and Age-Related Diseases. Medicines (Basel) 7 (5). doi:10.3390/medicines7050026
Hayashi, R., Himori, N., Taguchi, K., Ishikawa, Y., Uesugi, K., Ito, M., et al. (2013). The Role of the Nrf2-Mediated Defense System in Corneal Epithelial Wound Healing. Free Radic. Biol. Med. 61, 333–342. doi:10.1016/j.freeradbiomed.2013.04.008
He, F., Ru, X., and Wen, T. (2020). NRF2, a Transcription Factor for Stress Response and beyond. Int. J. Mol. Sci. 21 (13). doi:10.3390/ijms21134777
Heinrich, U., Neukam, K., Tronnier, H., Sies, H., and Stahl, W. (2006). Long-term Ingestion of High Flavanol cocoa Provides Photoprotection against UV-Induced Erythema and Improves Skin Condition in Women. J. Nutr. 136 (6), 1565–1569. doi:10.1093/jn/136.6.1565
Hong, Y. H., Lee, H. S., Jung, E. Y., Han, S. H., Park, Y., and Suh, H. J. (2017). Photoprotective Effects of Topical Ginseng Leaf Extract Using Ultraflo L against UVB-Induced Skin Damage in Hairless Mice. J. Ginseng Res. 41 (4), 456–462. doi:10.1016/j.jgr.2016.07.007
Hseu, Y. C., Chang, C. T., Gowrisankar, Y. V., Chen, X. Z., Lin, H. C., Yen, H. R., et al. (2019). Zerumbone Exhibits Antiphotoaging and Dermatoprotective Properties in Ultraviolet A-Irradiated Human Skin Fibroblast Cells via the Activation of Nrf2/ARE Defensive Pathway. Oxid Med. Cell Longev 2019, 4098674. doi:10.1155/2019/4098674
Hseu, Y. C., Chen, X. Z., Vudhya Gowrisankar, Y., Yen, H. R., Chuang, J. Y., and Yang, H. L. (2020). The Skin-Whitening Effects of Ectoine via the Suppression of α-MSH-Stimulated Melanogenesis and the Activation of Antioxidant Nrf2 Pathways in UVA-Irradiated Keratinocytes. Antioxidants (Basel) 9 (1). doi:10.3390/antiox9010063
Hseu, Y. C., Chou, C. W., Senthil Kumar, K. J., Fu, K. T., Wang, H. M., Hsu, L. S., et al. (2012). Ellagic Acid Protects Human Keratinocyte (HaCaT) Cells against UVA-Induced Oxidative Stress and Apoptosis through the Upregulation of the HO-1 and Nrf-2 Antioxidant Genes. Food Chem. Toxicol. 50 (5), 1245–1255. doi:10.1016/j.fct.2012.02.020
Hseu, Y. C., Vudhya Gowrisankar, Y., Wang, L. W., Zhang, Y. Z., Chen, X. Z., Huang, P. J., et al. (2021). The In Vitro and In Vivo Depigmenting Activity of Pterostilbene through Induction of Autophagy in Melanocytes and Inhibition of UVA-Irradiated α-MSH in Keratinocytes via Nrf2-Mediated Antioxidant Pathways. Redox Biol. 44, 102007. doi:10.1016/j.redox.2021.102007
Huang, C.-Y., Liu, I.-H., Huang, X.-Z., Chen, H.-J., Chang, S.-T., Chang, M.-L., et al. (2021). Antimelanogenesis Effects of Leaf Extract and Phytochemicals from Ceylon Olive (Elaeocarpus Serratus) in Zebrafish Model. Pharmaceutics 13 (7), 1059. doi:10.3390/pharmaceutics13071059
Hwang, E., Gao, W., Xiao, Y. K., Ngo, H. T. T., and Yi, T. H. (2019). Helianthus Annuus L. Flower Prevents UVB-Induced Photodamage in Human Dermal Fibroblasts by Regulating the MAPK/AP-1, NFAT, and Nrf2 Signaling Pathways. J. Cell Biochem 120 (1), 601–612. doi:10.1002/jcb.27417
Hwang, E., Ngo, H. T. T., Seo, S. A., Park, B., Zhang, M., and Yi, T. H. (2018). Protective Effect of Dietary Alchemilla Mollis on UVB-Irradiated Premature Skin Aging through Regulation of Transcription Factor NFATc1 and Nrf2/ARE Pathways. Phytomedicine 39, 125–136. doi:10.1016/j.phymed.2017.12.025
Ikehata, H., and Yamamoto, M. (2018). Roles of the KEAP1-NRF2 System in Mammalian Skin Exposed to UV Radiation. Toxicol. Appl. Pharmacol. 360, 69–77. doi:10.1016/j.taap.2018.09.038
Jastrząb, A., Gęgotek, A., and Skrzydlewska, E. (2019). Cannabidiol Regulates the Expression of Keratinocyte Proteins Involved in the Inflammation Process through Transcriptional Regulation. Cells 8 (8), 827. doi:10.3390/cells8080827
Jeayeng, S., Wongkajornsilp, A., Slominski, A. T., Jirawatnotai, S., Sampattavanich, S., and Panich, U. (2017). Nrf2 in Keratinocytes Modulates UVB-Induced DNA Damage and Apoptosis in Melanocytes through MAPK Signaling. Free Radic. Biol. Med. 108, 918–928. doi:10.1016/j.freeradbiomed.2017.05.009
Jian, Z., Li, K., Liu, L., Zhang, Y., Zhou, Z., Li, C., et al. (2011). Heme Oxygenase-1 Protects Human Melanocytes from H2O2-Induced Oxidative Stress via the Nrf2-ARE Pathway. J. Invest. Dermatol. 131 (7), 1420–1427. doi:10.1038/jid.2011.56
Jo, D. J., Seok, J. K., Kim, S. Y., Park, W., Baek, J. H., Kim, Y. M., et al. (2018). Human Skin-Depigmenting Effects of Resveratryl Triglycolate, a Hybrid Compound of Resveratrol and Glycolic Acid. Int. J. Cosmet. Sci. 40, 256–262. doi:10.1111/ics.12458
Johnsson, P. (2004). Phenolic Compounds in Flaxseed Chromatographic and Spectroscopic Analyses of Glucosidic Conjugates. Uppsala, Sweden: Swedish University of Agricultural Sciences.
Kanlayavattanakul, M., Chongnativisit, W., Chaikul, P., and Lourith, N. (2020). Phenolic-rich Pomegranate Peel Extract: In Vitro, Cellular, and In Vivo Activities for Skin Hyperpigmentation Treatment. Planta Med. 86 (11), 749–759. doi:10.1055/a-1170-7785
Kannan, S., and Jaiswal, A. K. (2006). Low and High Dose UVB Regulation of Transcription Factor NF-E2-Related Factor 2. Cancer Res. 66 (17), 8421–8429. doi:10.1158/0008-5472.CAN-06-1181
Karin, M. (1995). The Regulation of AP-1 Activity by Mitogen-Activated Protein Kinases. J. Biol. Chem. 270 (28), 16483–16486. doi:10.1074/jbc.270.28.16483
Kawachi, Y., Xu, X., Taguchi, S., Sakurai, H., Nakamura, Y., Ishii, Y., et al. (2008). Attenuation of UVB-Induced Sunburn Reaction and Oxidative DNA Damage with No Alterations in UVB-Induced Skin Carcinogenesis in Nrf2 Gene-Deficient Mice. J. Invest. Dermatol. 128 (7), 1773–1779. doi:10.1038/sj.jid.5701245
Kerns, M. L., Hakim, J. M., Lu, R. G., Guo, Y., Berroth, A., Kaspar, R. L., et al. (2016). Oxidative Stress and Dysfunctional NRF2 Underlie Pachyonychia Congenita Phenotypes. J. Clin. Invest. 126 (6), 2356–2366. doi:10.1172/JCI84870
Kim, A. J., Park, J. E., Cho, Y. H., Lim, D. S., and Lee, J. S. (2021). Effect of 7-Methylsulfinylheptyl Isothiocyanate on the Inhibition of Melanogenesis in B16-F1 Cells. Life (Basel) 11 (2). doi:10.3390/life11020162
Kim, J., Kim, J., Shim, J., Lee, C. Y., Lee, K. W., and Lee, H. J. (2014). Cocoa Phytochemicals: Recent Advances in Molecular Mechanisms on Health. Crit. Rev. Food Sci. Nutr. 54 (11), 1458–1472. doi:10.1080/10408398.2011.641041
Kim, J., Oh, J., Averilla, J. N., Kim, H. J., Kim, J. S., and Kim, J. S. (2019). Grape Peel Extract and Resveratrol Inhibit Wrinkle Formation in Mice Model through Activation of Nrf2/HO-1 Signaling Pathway. J. Food Sci. 84 (6), 1600–1608. doi:10.1111/1750-3841.14643
Kim, J. M., Noh, E. M., Kwon, K. B., Hwang, B. M., Hwang, J. K., You, Y. O., et al. (2013). Dihydroavenanthramide D Prevents UV-Irradiated Generation of Reactive Oxygen Species and Expression of Matrix Metalloproteinase-1 and -3 in Human Dermal Fibroblasts. Exp. Dermatol. 22 (11), 759–761. doi:10.1111/exd.12243
Kim, M., Park, Y. G., Lee, H. J., Lim, S. J., and Nho, C. W. (2015). Youngiasides A and C Isolated from Youngia Denticulatum Inhibit UVB-Induced MMP Expression and Promote Type I Procollagen Production via Repression of MAPK/AP-1/NF-κB and Activation of AMPK/Nrf2 in HaCaT Cells and Human Dermal Fibroblasts. J. Agric. Food Chem. 63 (22), 5428–5438. doi:10.1021/acs.jafc.5b00467
Kishimoto, Y., Tani, M., Uto-Kondo, H., Iizuka, M., Saita, E., Sone, H., et al. (2010). Astaxanthin Suppresses Scavenger Receptor Expression and Matrix Metalloproteinase Activity in Macrophages. Eur. J. Nutr. 49 (2), 119–126. doi:10.1007/s00394-009-0056-4
Kleszczyński, K., Ernst, I. M., Wagner, A. E., Kruse, N., Zillikens, D., Rimbach, G., et al. (2013). Sulforaphane and Phenylethyl Isothiocyanate Protect Human Skin against UVR-Induced Oxidative Stress and Apoptosis: Role of Nrf2-dependent Gene Expression and Antioxidant Enzymes. Pharmacol. Res. 78, 28–40. doi:10.1016/j.phrs.2013.09.009
Ko, H. J., Kim, J. H., Lee, G. S., and Shin, T. (2020). Sulforaphane Controls the Release of Paracrine Factors by Keratinocytes and Thus Mitigates Particulate Matter-Induced Premature Skin Aging by Suppressing Melanogenesis and Maintaining Collagen Homeostasis. Phytomedicine 77, 153276. doi:10.1016/j.phymed.2020.153276
Kohandel, Z., Farkhondeh, T., Aschner, M., Pourbagher-Shahri, A. M., and Samarghandian, S. (2022). Anti-inflammatory Action of Astaxanthin and its Use in the Treatment of Various Diseases. Biomed. Pharmacother. 145, 112179. doi:10.1016/j.biopha.2021.112179
Kohandel, Z., Farkhondeh, T., Aschner, M., and Samarghandian, S. (2021). Nrf2 a Molecular Therapeutic Target for Astaxanthin. Biomed. Pharmacother. 137, 111374. doi:10.1016/j.biopha.2021.111374
Kong, A. N., Owuor, E., Yu, R., Hebbar, V., Chen, C., Hu, R., et al. (2001). Induction of Xenobiotic Enzymes by the MAP Kinase Pathway and the Antioxidant or Electrophile Response Element (ARE/EpRE). Drug Metab. Rev. 33 (3-4), 255–271. doi:10.1081/dmr-120000652
Kuwano, T., Watanabe, M., Kagawa, D., and Murase, T. (2015). Hydrolyzed Methylhesperidin Induces Antioxidant Enzyme Expression via the Nrf2-ARE Pathway in Normal Human Epidermal Keratinocytes. J. Agric. Food Chem. 63 (36), 7937–7944. doi:10.1021/acs.jafc.5b01992
Kypriotou, M., Huber, M., and Hohl, D. (2012). The Human Epidermal Differentiation Complex: Cornified Envelope Precursors, S100 Proteins and the 'fused Genes' Family. Exp. Dermatol. 21 (9), 643–649. doi:10.1111/j.1600-0625.2012.01472.x
L Suraweera, T., Rupasinghe, H. P. V., Dellaire, G., and Xu, Z. (2020). Regulation of Nrf2/ARE Pathway by Dietary Flavonoids: A Friend or Foe for Cancer Management? Antioxidants (Basel) 9 (10). doi:10.3390/antiox9100973
Larroque-Cardoso, P., Camaré, C., Nadal-Wollbold, F., Grazide, M. H., Pucelle, M., Garoby-Salom, S., et al. (2015). Elastin Modification by 4-Hydroxynonenal in Hairless Mice Exposed to UV-A. Role in Photoaging and Actinic Elastosis. J. Invest. Dermatol. 135 (7), 1873–1881. doi:10.1038/jid.2015.84
Latronico, T., Larocca, M., Milella, S., Fasano, A., Rossano, R., and Liuzzi, G. M. (2021). Neuroprotective Potential of Isothiocyanates in an In Vitro Model of Neuroinflammation. Inflammopharmacology 29 (2), 561–571. doi:10.1007/s10787-020-00772-w
Lee, E.-J., Zheng, M., Craft, C. M., and Jeong, S. (2021). Matrix Metalloproteinase-9 (MMP-9) and Tissue Inhibitor of Metalloproteinases 1 (TIMP-1) Are Localized in the Nucleus of Retinal Müller Glial Cells and Modulated by Cytokines and Oxidative Stress. PLoS One 16 (7), e0253915. doi:10.1371/journal.pone.0253915
Lee, T. H., Seo, J. O., Baek, S. H., and Kim, S. Y. (2014). Inhibitory Effects of Resveratrol on Melanin Synthesis in Ultraviolet B-Induced Pigmentation in Guinea Pig Skin. Biomol. Ther. (Seoul) 22 (1), 35–40. doi:10.4062/biomolther.2013.081
Lee, Y. M., Seon, M. R., Cho, H. J., Kim, J. S., and Park, J. H. (2009). Benzyl Isothiocyanate Exhibits Anti-inflammatory Effects in Murine Macrophages and in Mouse Skin. J. Mol. Med. (Berl) 87 (12), 1251–1261. doi:10.1007/s00109-009-0532-6
Lephart, E. D. (2016). Skin Aging and Oxidative Stress: Equol's Anti-aging Effects via Biochemical and Molecular Mechanisms. Ageing Res. Rev. 31, 36–54. doi:10.1016/j.arr.2016.08.001
Li, H., Jiang, N., Liang, B., Liu, Q., Zhang, E., Peng, L., et al. (2017a). Pterostilbene Protects against UVB-Induced Photo-Damage through a Phosphatidylinositol-3-kinase-dependent Nrf2/ARE Pathway in Human Keratinocytes. Redox Rep. 22 (6), 501–507. doi:10.1080/13510002.2017.1329917
Li, H., Li, Z., Peng, L., Jiang, N., Liu, Q., Zhang, E., et al. (2017b). Lycium Barbarum Polysaccharide Protects Human Keratinocytes against UVB-Induced Photo-Damage. Free Radic. Res. 51 (2), 200–210. doi:10.1080/10715762.2017.1294755
Li, J., Liu, D., Wu, J., Zhang, D., Cheng, B., Zhang, Y., et al. (2016). Ginsenoside Rg1 Attenuates Ultraviolet B-Induced Glucocortisides Resistance in Keratinocytes via Nrf2/HDAC2 Signalling. Sci. Rep. 6, 39336. doi:10.1038/srep39336
Li, L., Hwang, E., Ngo, H. T. T., Lin, P., Gao, W., Liu, Y., et al. (2018). Antiphotoaging Effect of Prunus Yeonesis Blossom Extract via Inhibition of MAPK/AP-1 and Regulation of the TGF-βI/Smad and Nrf2/ARE Signaling Pathways. Photochem. Photobiol. 94 (4), 725–732. doi:10.1111/php.12894
Li, L., Ngo, H. T. T., Hwang, E., Wei, X., Liu, Y., Liu, J., et al. (2019). Conditioned Medium from Human Adipose-Derived Mesenchymal Stem Cell Culture Prevents UVB-Induced Skin Aging in Human Keratinocytes and Dermal Fibroblasts. Int. J. Mol. Sci. 21 (1). doi:10.3390/ijms21010049
Liebel, F., Kaur, S., Ruvolo, E., Kollias, N., and Southall, M. D. (2012). Irradiation of Skin with Visible Light Induces Reactive Oxygen Species and Matrix-Degrading Enzymes. J. Invest. Dermatol. 132 (7), 1901–1907. doi:10.1038/jid.2011.476
Lin, J. Y., and Fisher, D. E. (2007). Melanocyte Biology and Skin Pigmentation. Nature 445 (7130), 843–850. doi:10.1038/nature05660
Lin, Z., Marepally, S. R., Kim, T. K., Janjetovic, Z., Oak, A. S., Postlethwaite, A. E., et al. (2016). Design, Synthesis and Biological Activities of Novel Gemini 20S-Hydroxyvitamin D3 Analogs. Anticancer Res. 36 (3), 877–886.
Liu, C., Vojnovic, D., Kochevar, I. E., and Jurkunas, U. V. (2016). UV-A Irradiation Activates Nrf2-Regulated Antioxidant Defense and Induces p53/Caspase3-dependent Apoptosis in Corneal Endothelial Cells. Invest. Ophthalmol. Vis. Sci. 57 (4), 2319–2327. doi:10.1167/iovs.16-19097
Liu, J., Liu, Y., He, X., Teng, B., and McRae, J. M. (2021). Valonea Tannin: Tyrosinase Inhibition Activity, Structural Elucidation and Insights into the Inhibition Mechanism. Molecules 26 (9), 2747. doi:10.3390/molecules26092747
Liu, X. Y., Hwang, E., Park, B., Ngo, H. T. T., Xiao, Y. K., and Yi, T. H. (2018). Ginsenoside C-Mx Isolated from Notoginseng Stem-Leaf Ginsenosides Attenuates Ultraviolet B-Mediated Photoaging in Human Dermal Fibroblasts. Photochem. Photobiol. 94 (5), 1040–1048. doi:10.1111/php.12940
Liu, X. Y., Li, H., Hwang, E., Park, B., Xiao, Y. K., Liu, S., et al. (2022). Chemical Distance Measurement and System Pharmacology Approach Uncover the Novel Protective Effects of Biotransformed Ginsenoside C-Mc against UVB-Irradiated Photoaging. Oxid Med. Cell Longev 2022, 4691576. doi:10.1155/2022/4691576
Liu, X. Y., Xiao, Y. K., Hwang, E., Haeng, J. J., and Yi, T. H. (2019). Antiphotoaging and Antimelanogenesis Properties of Ginsenoside C-Y, a Ginsenoside Rb2 Metabolite from American Ginseng PDD-Ginsenoside. Photochem. Photobiol. 95 (6), 1412–1423. doi:10.1111/php.13116
Lohakul, J., Chaiprasongsuk, A., Jeayeng, S., Saelim, M., Muanjumpon, P., Thanachaiphiwat, S., et al. (2021a). The Protective Effect of Polyherbal Formulation, Harak Formula, on UVA-Induced Photoaging of Human Dermal Fibroblasts and Mouse Skin via Promoting Nrf2-Regulated Antioxidant Defense. Front. Pharmacol. 12, 649820. doi:10.3389/fphar.2021.649820
Lohakul, J., Jeayeng, S., Chaiprasongsuk, A., Torregrossa, R., Wood, M. E., Saelim, M., et al. (2021b). Mitochondria-Targeted Hydrogen Sulfide Delivery Molecules Protect against UVA-Induced Photoaging in Human Dermal Fibroblasts, and in Mouse Skin In Vivo. Antioxid. Redox Signaling 2021. doi:10.1089/ars.2020.8255
López-Camarillo, C., Ocampo, E. A., Casamichana, M. L., Pérez-Plasencia, C., Alvarez-Sánchez, E., and Marchat, L. A. (2012). Protein Kinases and Transcription Factors Activation in Response to UV-Radiation of Skin: Implications for Carcinogenesis. Int. J. Mol. Sci. 13 (1), 142–172. doi:10.3390/ijms13010142
Lü, J., Kundrát, M., and Shen, C. (2016). New Material of the Pterosaur Gladocephaloideus Lü et al., 2012 from the Early Cretaceous of Liaoning Province, China, with Comments on Its Systematic Position. PLoS One 11 (6), e0154888. doi:10.1371/journal.pone.0154888
Lu, Y., F. Tonissen, K., and Di Trapani, G. (2021). Modulating Skin Colour: Role of the Thioredoxin and Glutathione Systems in Regulating Melanogenesis. Biosci. Rep. 41 (5). doi:10.1042/BSR20210427
Luo, J. F., Shen, X. Y., Lio, C. K., Dai, Y., Cheng, C. S., Liu, J. X., et al. (2018). Activation of Nrf2/HO-1 Pathway by Nardochinoid C Inhibits Inflammation and Oxidative Stress in Lipopolysaccharide-Stimulated Macrophages. Front. Pharmacol. 9, 911. doi:10.3389/fphar.2018.00911
Lv, J., Fu, Y., Cao, Y., Jiang, S., Yang, Y., Song, G., et al. (2020). Isoliquiritigenin Inhibits Melanogenesis, Melanocyte Dendricity and Melanosome Transport by Regulating ERK-Mediated MITF Degradation. Exp. Dermatol. 29 (2), 149–157. doi:10.1111/exd.14066
Ma, Q., Liu, Q., Yuan, L., and Zhuang, Y. (2018). Protective Effects of LSGYGP from Fish Skin Gelatin Hydrolysates on UVB-Induced MEFs by Regulation of Oxidative Stress and Matrix Metalloproteinase Activity. Nutrients 10 (4). doi:10.3390/nu10040420
Ma, Q. (2013). Role of Nrf2 in Oxidative Stress and Toxicity. Annu. Rev. Pharmacol. Toxicol. 53, 401–426. doi:10.1146/annurev-pharmtox-011112-140320
Mackay, A. R., Ballin, M., Pelina, M. D., Farina, A. R., Nason, A. M., Hartzler, J. L., et al. (1992). Effect of Phorbol Ester and Cytokines on Matrix Metalloproteinase and Tissue Inhibitor of Metalloproteinase Expression in Tumor and normal Cell Lines. Invasion Metastasis 12 (3-4), 168–184.
Majeed, M., Majeed, S., Jain, R., Mundkur, L., Rajalakshmi, H. R., Lad, P. S., et al. (2020). An Open-Label Single-Arm, Monocentric Study Assessing the Efficacy and Safety of Natural Pterostilbene (Pterocarpus Marsupium) for Skin Brightening and Antiaging Effects. Clin. Cosmet. Investig. Dermatol. 13, 105–116. doi:10.2147/CCID.S238358
Maleki, S. J., Crespo, J. F., and Cabanillas, B. (2019). Anti-inflammatory Effects of Flavonoids. Food Chem. 299, 125124. doi:10.1016/j.foodchem.2019.125124
McBride, K., and Nemer, M. (1998). The C-Terminal Domain of C-Fos Is Required for Activation of an AP-1 Site Specific for Jun-Fos Heterodimers. Mol. Cell Biol 18 (9), 5073–5081. doi:10.1128/MCB.18.9.5073
Milani, A., Basirnejad, M., Shahbazi, S., and Bolhassani, A. (2017). Carotenoids: Biochemistry, Pharmacology and Treatment. Br. J. Pharmacol. 174 (11), 1290–1324. doi:10.1111/bph.13625
Misawa, E., Tanaka, M., Saito, M., Nabeshima, K., Yao, R., Yamauchi, K., et al. (2017). Protective Effects of Aloe Sterols against UVB-Induced Photoaging in Hairless Mice. Photodermatol. Photoimmunol Photomed. 33 (2), 101–111. doi:10.1111/phpp.12286
Narayanan, D. L., Saladi, R. N., and Fox, J. L. (2010). Ultraviolet Radiation and Skin Cancer. Int. J. Dermatol. 49 (9), 978–986. doi:10.1111/j.1365-4632.2010.04474.x
Nguyen, T., Nioi, P., and Pickett, C. B. (2009). The Nrf2-Antioxidant Response Element Signaling Pathway and its Activation by Oxidative Stress. J. Biol. Chem. 284 (20), 13291–13295. doi:10.1074/jbc.R900010200
Ni, H., Jin, W., Zhu, T., Wang, J., Yuan, B., Jiang, J., et al. (2015). Curcumin Modulates TLR4/NF-κB Inflammatory Signaling Pathway Following Traumatic Spinal Cord Injury in Rats. J. Spinal Cord Med. 38 (2), 199–206. doi:10.1179/2045772313Y.0000000179
Nishiumi, S., Miyamoto, S., Kawabata, K., Ohnishi, K., Mukai, R., Murakami, A., et al. (2011). Dietary Flavonoids as Cancer-Preventive and Therapeutic Biofactors. Front. Biosci. (Schol Ed. 3, 1332–1362. doi:10.2741/229
Niu, C., and Aisa, H. A. (2017). Upregulation of Melanogenesis and Tyrosinase Activity: Potential Agents for Vitiligo. Molecules 22 (8). doi:10.3390/molecules22081303
O'Donovan, P., Perrett, C. M., Zhang, X., Montaner, B., Xu, Y. Z., Harwood, C. A., et al. (2005). Azathioprine and UVA Light Generate Mutagenic Oxidative DNA Damage. Science 309 (5742), 1871–1874. doi:10.1126/science.1114233
Oh, J. H., Kim, J., Karadeniz, F., Kim, H. R., Park, S. Y., Seo, Y., et al. (2021). Santamarine Shows Anti-photoaging Properties via Inhibition of MAPK/AP-1 and Stimulation of TGF-β/Smad Signaling in UVA-Irradiated HDFs. Molecules 26 (12). doi:10.3390/molecules26123585
Onkoksoong, T., Jeayeng, S., Poungvarin, N., Limsaengurai, S., Thamsermsang, O., Tripatara, P., et al. (2018). Thai Herbal Antipyretic 22 Formula (APF22) Inhibits UVA-Mediated Melanogenesis through Activation of Nrf2-Regulated Antioxidant Defense. Phytother Res. 32 (8), 1546–1554. doi:10.1002/ptr.6083
Panche, A. N., Diwan, A. D., and Chandra, S. R. (2016). Flavonoids: an Overview. J. Nutr. Sci. 5, e47. doi:10.1017/jns.2016.41
Pandey, K. B., and Rizvi, S. I. (2009). Plant Polyphenols as Dietary Antioxidants in Human Health and Disease. Oxid Med. Cell Longev 2 (5), 270–278. doi:10.4161/oxim.2.5.9498
Panich, U., Pluemsamran, T., Tangsupa-a-nan, V., Wattanarangsan, J., Phadungrakwittaya, R., Akarasereenont, P., et al. (2013). Protective Effect of AVS073, a Polyherbal Formula, against UVA-Induced Melanogenesis through a Redox Mechanism Involving Glutathione-Related Antioxidant Defense. BMC Complement. Altern. Med. 13, 159. doi:10.1186/1472-6882-13-159
Park, C., Lee, H., Noh, J. S., Jin, C. Y., Kim, G. Y., Hyun, J. W., et al. (2020). Hemistepsin A Protects Human Keratinocytes against Hydrogen Peroxide-Induced Oxidative Stress through Activation of the Nrf2/HO-1 Signaling Pathway. Arch. Biochem. Biophys. 691, 108512. doi:10.1016/j.abb.2020.108512
Park, C., Park, J., Kim, W.-J., Kim, W., Cheong, H., and Kim, S.-J. (2021). Malonic Acid Isolated from Pinus Densiflora Inhibits UVB-Induced Oxidative Stress and Inflammation in HaCaT Keratinocytes. Polymers 13 (5), 816. doi:10.3390/polym13050816
Parrado, C., Mascaraque, M., Gilaberte, Y., Juarranz, A., and Gonzalez, S. (2016). Fernblock (Polypodium Leucotomos Extract): Molecular Mechanisms and Pleiotropic Effects in Light-Related Skin Conditions, Photoaging and Skin Cancers, a Review. Int. J. Mol. Sci. 17 (7). doi:10.3390/ijms17071026
Pastore, S., Lulli, D., Pascarella, A., Maurelli, R., Dellambra, E., Potapovich, A., et al. (2012). Resveratrol Enhances Solar UV-Induced Responses in normal Human Epidermal Keratinocytes. Photochem. Photobiol. 88 (6), 1522–1530. doi:10.1111/j.1751-1097.2012.01195.x
Pittayapruek, P., Meephansan, J., Prapapan, O., Komine, M., and Ohtsuki, M. (2016). Role of Matrix Metalloproteinases in Photoaging and Photocarcinogenesis. Int. J. Mol. Sci. 17 (6). doi:10.3390/ijms17060868
Pramanik, R., Qi, X., Borowicz, S., Choubey, D., Schultz, R. M., Han, J., et al. (2003). p38 Isoforms Have Opposite Effects on AP-1-dependent Transcription through Regulation of C-Jun. The Determinant Roles of the Isoforms in the P38 MAPK Signal Specificity. J. Biol. Chem. 278 (7), 4831–4839. doi:10.1074/jbc.M207732200
Quan, T., Qin, Z., Xia, W., Shao, Y., Voorhees, J. J., and Fisher, G. J. (2009). Matrix-degrading Metalloproteinases in Photoaging. J. Investig. Dermatol. Symp. Proc. 14 (1), 20–24. doi:10.1038/jidsymp.2009.8
Rijken, F., and Bruijnzeel-Koomen, C. A. (2011). Photoaged Skin: the Role of Neutrophils, Preventive Measures, and Potential Pharmacological Targets. Clin. Pharmacol. Ther. 89 (1), 120–124. doi:10.1038/clpt.2010.221
Rinnerthaler, M., Bischof, J., Streubel, M. K., Trost, A., and Richter, K. (2015). Oxidative Stress in Aging Human Skin. Biomolecules 5 (2), 545–589. doi:10.3390/biom5020545
Rittié, L., and Fisher, G. J. (2002). UV-light-induced Signal Cascades and Skin Aging. Ageing Res. Rev. 1 (4), 705–720. doi:10.1016/s1568-1637(02)00024-7
Rodríguez-Luna, A., Ávila-Román, J., González-Rodríguez, M. L., Cózar, M. J., Rabasco, A. M., Motilva, V., et al. (2018). Fucoxanthin-Containing Cream Prevents Epidermal Hyperplasia and UVB-Induced Skin Erythema in Mice. Mar. Drugs 16 (10). doi:10.3390/md16100378
Rodríguez-Luna, A., Ávila-Román, J., Oliveira, H., Motilva, V., and Talero, E. (2019). Fucoxanthin and Rosmarinic Acid Combination Has Anti-inflammatory Effects through Regulation of NLRP3 Inflammasome in UVB-Exposed HaCaT Keratinocytes. Mar. Drugs 17 (8). doi:10.3390/md17080451
Rojo de la Vega, M., Krajisnik, A., Zhang, D. D., and Wondrak, G. T. (2017). Targeting NRF2 for Improved Skin Barrier Function and Photoprotection: Focus on the Achiote-Derived Apocarotenoid Bixin. Nutrients 9 (12). doi:10.3390/nu9121371
Ryšavá, A., Čížková, K., Franková, J., Roubalová, L., Ulrichová, J., Vostálová, J., et al. (2020). Effect of UVA Radiation on the Nrf2 Signalling Pathway in Human Skin Cells. J. Photochem. Photobiol. B: Biol. 209, 111948. doi:10.1016/j.jphotobiol.2020.111948
Ryšavá, A., Vostálová, J., and Rajnochová Svobodová, A. (2021). Effect of Ultraviolet Radiation on the Nrf2 Signaling Pathway in Skin Cells. Int. J. Radiat. Biol. 97 (10), 1383–1403. doi:10.1080/09553002.2021.1962566
Ryu, J. H., Seok, J. K., An, S. M., Baek, J. H., Koh, J. S., and Boo, Y. C. (2015). A Study of the Human Skin-Whitening Effects of Resveratryl Triacetate. Arch. Dermatol. Res. 307 (3), 239–247. doi:10.1007/s00403-015-1556-0
Saha, S., Buttari, B., Panieri, E., Profumo, E., and Saso, L. (2020). An Overview of Nrf2 Signaling Pathway and its Role in Inflammation. Molecules 25 (22). doi:10.3390/molecules25225474
Sangaraju, R., Alavala, S., Nalban, N., Jerald, M. K., and Sistla, R. (2021). Galangin Ameliorates Imiquimod-Induced Psoriasis-like Skin Inflammation in BALB/c Mice via Down Regulating NF-κB and Activation of Nrf2 Signaling Pathways. Int. Immunopharmacol 96, 107754. doi:10.1016/j.intimp.2021.107754
Saw, C. L., Huang, M. T., Liu, Y., Khor, T. O., Conney, A. H., and Kong, A. N. (2011). Impact of Nrf2 on UVB-Induced Skin Inflammation/photoprotection and Photoprotective Effect of Sulforaphane. Mol. Carcinog 50 (6), 479–486. doi:10.1002/mc.20725
Schäfer, M., Farwanah, H., Willrodt, A. H., Huebner, A. J., Sandhoff, K., Roop, D., et al. (2012). Nrf2 Links Epidermal Barrier Function with Antioxidant Defense. EMBO Mol. Med. 4 (5), 364–379. doi:10.1002/emmm.201200219
Schäfer, M., Dütsch, S., auf dem Keller, U., Navid, F., Schwarz, A., Johnson, D. A., et al. (2010). auf dem Keller, UNrf2 establishes a glutathione-mediated gradient of UVB cytoprotection in the epidermis. Genes Dev. 24 (10), 1045–1058. doi:10.1101/gad.568810
Schmitz, S., Thomas, P. D., Allen, T. M., Poznansky, M. J., and Jimbow, K. (1995). Dual Role of Melanins and Melanin Precursors as Photoprotective and Phototoxic Agents: Inhibition of Ultraviolet Radiation-Induced Lipid Peroxidation. Photochem. Photobiol. 61 (6), 650–655. doi:10.1111/j.1751-1097.1995.tb09883.x
Searle, T., Al-Niaimi, F., and Ali, F. R. (2020). The Top 10 Cosmeceuticals for Facial Hyperpigmentation. Dermatol. Ther. 33 (6), e14095. doi:10.1111/dth.14095
Serini, S., Guarino, R., Ottes Vasconcelos, R., Celleno, L., and Calviello, G. (2020). The Combination of Sulforaphane and Fernblock® XP Improves Individual Beneficial Effects in Normal and Neoplastic Human Skin Cell Lines. Nutrients 12 (6). doi:10.3390/nu12061608
Shao, Y., Dang, M., Lin, Y., and Xue, F. (2019). Evaluation of Wound Healing Activity of Plumbagin in Diabetic Rats. Life Sci. 231, 116422. doi:10.1016/j.lfs.2019.04.048
Sheen, Y. S., Huang, H. Y., and Liao, Y. H. (2021). The Efficacy and Safety of an Antiaging Topical Serum Containing Hesperetin and Sodium Cyclic Lysophosphatidic Acid: A Single‐center Clinical Trial. J. Cosmet. Dermatol. 20, 3960–3967. doi:10.1111/jocd.14063
Shin, J. M., Kim, M. Y., Sohn, K. C., Jung, S. Y., Lee, H. E., Lim, J. W., et al. (2014). Nrf2 Negatively Regulates Melanogenesis by Modulating PI3K/Akt Signaling. PLoS One 9 (4), e96035. doi:10.1371/journal.pone.0096035
Shin, J. W., Chun, K. S., Kim, D. H., Kim, S. J., Kim, S. H., Cho, N. C., et al. (2020). Curcumin Induces Stabilization of Nrf2 Protein through Keap1 Cysteine Modification. Biochem. Pharmacol. 173, 113820. doi:10.1016/j.bcp.2020.113820
Shirasugi, I., Kamada, M., Matsui, T., Sakakibara, Y., Liu, M. C., and Suiko, M. (2010). Sulforaphane Inhibited Melanin Synthesis by Regulating Tyrosinase Gene Expression in B16 Mouse Melanoma Cells. Biosci. Biotechnol. Biochem. 74 (3), 579–582. doi:10.1271/bbb.90778
Shoji, T., Masumoto, S., Moriichi, N., Ohtake, Y., and Kanda, T. (2020). Administration of Apple Polyphenol Supplements for Skin Conditions in Healthy Women: A Randomized, Double-Blind, Placebo-Controlled Clinical Trial. Nutrients 12 (4). doi:10.3390/nu12041071
Shroff, H., Diedrichs, P. C., and Craddock, N. (2017). Skin Color, Cultural Capital, and Beauty Products: An Investigation of the Use of Skin Fairness Products in Mumbai, India. Front. Public Health 5, 365. doi:10.3389/fpubh.2017.00365
Silva, S. A. M. E., Michniak-Kohn, B., and Leonardi, G. R. (2017). An Overview about Oxidation in Clinical Practice of Skin Aging. Bras Dermatol. 92 (3), 367–374. doi:10.1590/abd1806-4841.20175481
Silvers, A. L., Bachelor, M. A., and Bowden, G. T. (2003). The Role of JNK and P38 MAPK Activities in UVA-Induced Signaling Pathways Leading to AP-1 Activation and C-Fos Expression. Neoplasia 5 (4), 319–329. doi:10.1016/S1476-5586(03)80025-8
Sirerol, J. A., Feddi, F., Mena, S., Rodriguez, M. L., Sirera, P., Aupí, M., et al. (2015). Topical Treatment with Pterostilbene, a Natural Phytoalexin, Effectively Protects Hairless Mice against UVB Radiation-Induced Skin Damage and Carcinogenesis. Free Radic. Biol. Med. 85, 1–11. doi:10.1016/j.freeradbiomed.2015.03.027
Slominski, A., Tobin, D. J., Shibahara, S., and Wortsman, J. (2004). Melanin Pigmentation in Mammalian Skin and its Hormonal Regulation. Physiol. Rev. 84 (4), 1155–1228. doi:10.1152/physrev.00044.2003
Slominski, A., Wortsman, J., Luger, T., Paus, R., and Solomon, S. (2000). Corticotropin Releasing Hormone and Proopiomelanocortin Involvement in the Cutaneous Response to Stress. Physiol. Rev. 80 (3), 979–1020. doi:10.1152/physrev.2000.80.3.979
Smalley, K., and Eisen, T. (2000). The Involvement of P38 Mitogen-Activated Protein Kinase in the Alpha-Melanocyte Stimulating Hormone (Alpha-MSH)-Induced Melanogenic and Anti-proliferative Effects in B16 Murine Melanoma Cells. FEBS Lett. 476 (3), 198–202. doi:10.1016/s0014-5793(00)01726-9
Sporn, M. B., and Liby, K. T. (2012). NRF2 and Cancer: the Good, the Bad and the Importance of Context. Nat. Rev. Cancer 12 (8), 564–571. doi:10.1038/nrc3278
Staurengo-Ferrari, L., Badaro-Garcia, S., Hohmann, M. S. N., Manchope, M. F., Zaninelli, T. H., Casagrande, R., et al. (2018). Contribution of Nrf2 Modulation to the Mechanism of Action of Analgesic and Anti-inflammatory Drugs in Pre-clinical and Clinical Stages. Front. Pharmacol. 9, 1536. doi:10.3389/fphar.2018.01536
Su, T. R., Lin, J. J., Tsai, C. C., Huang, T. K., Yang, Z. Y., Wu, M. O., et al. (2013). Inhibition of Melanogenesis by Gallic Acid: Possible Involvement of the PI3K/Akt, MEK/ERK and Wnt/β-Catenin Signaling Pathways in B16F10 Cells. Int. J. Mol. Sci. 14 (10), 20443–20458. doi:10.3390/ijms141020443
Sun, Z., Park, S. Y., Hwang, E., Park, B., Seo, S. A., Cho, J. G., et al. (2016). Dietary Foeniculum Vulgare Mill Extract Attenuated UVB Irradiation-Induced Skin Photoaging by Activating of Nrf2 and Inhibiting MAPK Pathways. Phytomedicine 23 (12), 1273–1284. doi:10.1016/j.phymed.2016.06.008
Süntar, I., Çetinkaya, S., Panieri, E., Saha, S., Buttari, B., Profumo, E., et al. (2021). Regulatory Role of Nrf2 Signaling Pathway in Wound Healing Process. Molecules 26 (9). doi:10.3390/molecules26092424
Surh, Y. J. (2003). Cancer Chemoprevention with Dietary Phytochemicals. Nat. Rev. Cancer 3 (10), 768–780. doi:10.1038/nrc1189
Surh, Y. J., and Na, H. K. (2008). NF-kappaB and Nrf2 as Prime Molecular Targets for Chemoprevention and Cytoprotection with Anti-inflammatory and Antioxidant Phytochemicals. Genes Nutr. 2 (4), 313–317. doi:10.1007/s12263-007-0063-0
Suschek, C. V., Briviba, K., Bruch-Gerharz, D., Sies, H., Kröncke, K. D., and Kolb-Bachofen, V. (2001). Even after UVA-Exposure Will Nitric Oxide Protect Cells from Reactive Oxygen Intermediate-Mediated Apoptosis and Necrosis. Cell Death Differ 8 (5), 515–527. doi:10.1038/sj.cdd.4400839
Suzuki, T., Muramatsu, A., Saito, R., Iso, T., Shibata, T., Kuwata, K., et al. (2019). Molecular Mechanism of Cellular Oxidative Stress Sensing by Keap1. Cell Rep 28 (3), 746–e4. doi:10.1016/j.celrep.2019.06.047
Swiader, A., Camaré, C., Guerby, P., Salvayre, R., and Negre-Salvayre, A. (2021). 4-Hydroxynonenal Contributes to Fibroblast Senescence in Skin Photoaging Evoked by UV-A Radiation. Antioxidants (Basel) 10 (3). doi:10.3390/antiox10030365
Taguchi, K., and Yamamoto, M. (2020). The KEAP1-NRF2 System as a Molecular Target of Cancer Treatment. Cancers (Basel) 13 (1). doi:10.3390/cancers13010046
Takei, K., Hashimoto-Hachiya, A., Takahara, M., Tsuji, G., Nakahara, T., and Furue, M. (2015). Cynaropicrin Attenuates UVB-Induced Oxidative Stress via the AhR-Nrf2-Nqo1 Pathway. Toxicol. Lett. 234 (2), 74–80. doi:10.1016/j.toxlet.2015.02.007
Tan, Y., Ichikawa, T., Li, J., Si, Q., Yang, H., Chen, X., et al. (2011). Diabetic Downregulation of Nrf2 Activity via ERK Contributes to Oxidative Stress-Induced Insulin Resistance in Cardiac Cells In Vitro and In Vivo. Diabetes 60 (2), 625–633. doi:10.2337/db10-1164
Tran, T. T., Schulman, J., and Fisher, D. E. (2008). UV and Pigmentation: Molecular Mechanisms and Social Controversies. Pigment Cell Melanoma Res 21 (5), 509–516. doi:10.1111/j.1755-148X.2008.00498.x
Truong, V. L., Bak, M. J., Jun, M., Kong, A. N., Ho, C. T., and Jeong, W. S. (2014). Antioxidant Defense and Hepatoprotection by Procyanidins from almond (Prunus Amygdalus) Skins. J. Agric. Food Chem. 62 (34), 8668–8678. doi:10.1021/jf5027247
Uto, T., Hou, D. X., Morinaga, O., and Shoyama, Y. (2012). Molecular Mechanisms Underlying Anti-inflammatory Actions of 6-(Methylsulfinyl)hexyl Isothiocyanate Derived from Wasabi (Wasabia Japonica). Adv. Pharmacol. Sci. 2012, 614046. doi:10.1155/2012/614046
Vacek, J., Vostalova, J., Papouskova, B., Skarupova, D., Kos, M., Kabelac, M., et al. (2021). Antioxidant Function of Phytocannabinoids: Molecular Basis of Their Stability and Cytoprotective Properties under UV-Irradiation. Free Radic. Biol. Med. 164, 258–270. doi:10.1016/j.freeradbiomed.2021.01.012
Vomund, S., Schäfer, A., Parnham, M. J., Brüne, B., and von Knethen, A. (2017). Nrf2, the Master Regulator of Anti-Oxidative Responses. Int. J. Mol. Sci. 18 (12). doi:10.3390/ijms18122772
Wagner, A. E., Boesch-Saadatmandi, C., Dose, J., Schultheiss, G., and Rimbach, G. (2012). Anti-inflammatory Potential of Allyl-Isothiocyanate-Rrole of Nrf2, NF-(κ) B and microRNA-155. J. Cell Mol Med 16 (4), 836–843. doi:10.1111/j.1582-4934.2011.01367.x
Wang, P. W., Cheng, Y. C., Hung, Y. C., Lee, C. H., Fang, J. Y., Li, W. T., et al. (2019). Red Raspberry Extract Protects the Skin against UVB-Induced Damage with Antioxidative and Anti-inflammatory Properties. Oxid Med. Cell Longev 2019, 9529676. doi:10.1155/2019/9529676
Wang, S., Wu, Y. Y., Wang, X., Shen, P., Jia, Q., Yu, S., et al. (2020). Lycopene Prevents Carcinogen-Induced Cutaneous Tumor by Enhancing Activation of the Nrf2 Pathway through P62-Triggered Autophagic Keap1 Degradation. Aging (Albany NY) 12 (9), 8167–8190. doi:10.18632/aging.103132
Wang, Y., Hao, M. M., Sun, Y., Wang, L. F., Wang, H., Zhang, Y. J., et al. (2018). Synergistic Promotion on Tyrosinase Inhibition by Antioxidants. Molecules 23 (1). doi:10.3390/molecules23010106
Watanabe, H., Shimizu, T., Nishihira, J., Abe, R., Nakayama, T., Taniguchi, M., et al. (2004). Ultraviolet A-Induced Production of Matrix Metalloproteinase-1 Is Mediated by Macrophage Migration Inhibitory Factor (MIF) in Human Dermal Fibroblasts. J. Biol. Chem. 279 (3), 1676–1683. doi:10.1074/jbc.M303650200
Watson, M., Holman, D. M., and Maguire-Eisen, M. (2016). Ultraviolet Radiation Exposure and its Impact on Skin Cancer Risk. Semin. Oncol. Nurs. 32 (3), 241–254. doi:10.1016/j.soncn.2016.05.005
Whitmarsh, A. J. (2007). Regulation of Gene Transcription by Mitogen-Activated Protein Kinase Signaling Pathways. Biochim. Biophys. Acta 1773 (8), 1285–1298. doi:10.1016/j.bbamcr.2006.11.011
Wikramanayake, T. C., Stojadinovic, O., and Tomic-Canic, M. (2014). Epidermal Differentiation in Barrier Maintenance and Wound Healing. Adv. Wound Care (New Rochelle) 3 (3), 272–280. doi:10.1089/wound.2013.0503
Wu, M. Y., Hung, S. K., and Fu, S. L. (2011). Immunosuppressive Effects of Fisetin in Ovalbumin-Induced Asthma through Inhibition of NF-κB Activity. J. Agric. Food Chem. 59 (19), 10496–10504. doi:10.1021/jf202756f
Wu, P. Y., Lyu, J. L., Liu, Y. J., Chien, T. Y., Hsu, H. C., Wen, K. C., et al. (2017). Fisetin Regulates Nrf2 Expression and the Inflammation-Related Signaling Pathway to Prevent UVB-Induced Skin Damage in Hairless Mice. Int. J. Mol. Sci. 18 (10). doi:10.3390/ijms18102118
Wu, P. Y., You, Y. J., Liu, Y. J., Hou, C. W., Wu, C. S., Wen, K. C., et al. (2018). Sesamol Inhibited Melanogenesis by Regulating Melanin-Related Signal Transduction in B16F10 Cells. Int. J. Mol. Sci. 19 (4). doi:10.3390/ijms19041108
Wu, W., Peng, G., Yang, F., Zhang, Y., Mu, Z., and Han, X. (2019). Sulforaphane Has a Therapeutic Effect in an Atopic Dermatitis Murine Model and Activates the Nrf2/HO-1 axis. Mol. Med. Rep. 20 (2), 1761–1771. doi:10.3892/mmr.2019.10405
Xian, D., Xiong, X., Xu, J., Xian, L., Lei, Q., Song, J., et al. (2019). Nrf2 Overexpression for the Protective Effect of Skin-Derived Precursors against UV-Induced Damage: Evidence from a Three-Dimensional Skin Model. Oxid Med. Cell Longev 2019, 7021428. doi:10.1155/2019/7021428
Yamamoto, T., Suzuki, T., Kobayashi, A., Wakabayashi, J., Maher, J., Motohashi, H., et al. (2008). Physiological Significance of Reactive Cysteine Residues of Keap1 in Determining Nrf2 Activity. Mol. Cell Biol 28 (8), 2758–2770. doi:10.1128/MCB.01704-07
Yamashita, Y., Ikeda, T., Matsuda, M., Maji, D., Hoshino, T., and Mizushima, T. (2012). Purification and Characterization of HSP-Inducers from Eupatorium Lindleyanum. Biochem. Pharmacol. 83 (7), 909–922. doi:10.1016/j.bcp.2011.12.040
Yang, H. L., Lin, C. P., Vudhya Gowrisankar, Y., Huang, P. J., Chang, W. L., Shrestha, S., et al. (2021). The Anti-melanogenic Effects of Ellagic Acid through Induction of Autophagy in Melanocytes and Suppression of UVA-Activated α-MSH Pathways via Nrf2 Activation in Keratinocytes. Biochem. Pharmacol. 185, 114454. doi:10.1016/j.bcp.2021.114454
Yang, K., Kim, S. Y., Park, J. H., Ahn, W. G., Jung, S. H., Oh, D., et al. (2020). Topical Application of Phlorotannins from Brown Seaweed Mitigates Radiation Dermatitis in a Mouse Model. Mar. Drugs 18 (8). doi:10.3390/md18080377
Zhao, P., Alam, M. B., and Lee, S. H. (2018). Protection of UVB-Induced Photoaging by Fuzhuan-Brick Tea Aqueous Extract via MAPKs/Nrf2-Mediated Down-Regulation of MMP-1. Nutrients 11 (1). doi:10.3390/nu11010060
Keywords: phytochemicals, photoaging, Nrf2, inflammation, skin barrier dysfunction
Citation: Chaiprasongsuk A and Panich U (2022) Role of Phytochemicals in Skin Photoprotection via Regulation of Nrf2. Front. Pharmacol. 13:823881. doi: 10.3389/fphar.2022.823881
Received: 28 November 2021; Accepted: 11 April 2022;
Published: 12 May 2022.
Edited by:
Cheorl-Ho Kim, Sungkyunkwan University, South KoreaReviewed by:
Wei Hsum Yap, Taylor’s University, MalaysiaMaged El-Sayed Mohamed, King Faisal University, Saudi Arabia
Copyright © 2022 Chaiprasongsuk and Panich. This is an open-access article distributed under the terms of the Creative Commons Attribution License (CC BY). The use, distribution or reproduction in other forums is permitted, provided the original author(s) and the copyright owner(s) are credited and that the original publication in this journal is cited, in accordance with accepted academic practice. No use, distribution or reproduction is permitted which does not comply with these terms.
*Correspondence: Uraiwan Panich, uraiwan.pan@mahidol.ac.th