- 1The Center for Global Health and Diseases, Pathology Department, Case Western Reserve University, Cleveland, OH, United States
- 2Department of Genetics and Genome Sciences, Case Western Reserve University School of Medicine, Cleveland, OH, United States
- 3Department of Population and Quantitative Health Sciences, Case Western Reserve University School of Medicine, Cleveland, OH, United States
- 4Master of Public Health Program, Case Western Reserve University, Cleveland, OH, United States
Clinical trial and individual patient treatment outcomes have produced accumulating evidence that effective primaquine (PQ) treatment of Plasmodium vivax and P. ovale liver stage hypnozoites is associated with genetic variation in the human cytochrome P450 gene, CYP2D6. Successful PQ treatment of individual and population-wide infections by the Plasmodium species that generate these dormant liver stage forms is likely to be necessary to reach elimination of malaria caused by these parasites globally. Optimizing safe and effective PQ treatment will require coordination of efforts between the malaria and pharmacogenomics research communities.
Introduction—malaria, primaquine and human genetics
In 2021, 241 million clinical cases and approximately 627,000 deaths were attributed to malaria (World Health Organization, 2020). Plasmodium vivax and P. falciparum are the most prevalent human malaria parasites, with an estimated 2.5 billion people at risk (Gething et al., 2011; Gething et al., 2012). While P. falciparum is currently recognized as the most lethal malaria parasite, P. vivax is the most geographically dispersed species. Furthermore, a now well-established body of literature describes severe, life-threatening clinical illness caused by P. vivax involving severe anemia (Tjitra et al., 2008), respiratory distress (Genton et al., 2008; Fernandez-Becerra et al., 2009), liver dysfunction (Barcus et al., 2007; Kochar et al., 2009), and renal failure (Siqueira et al., 2010). P. vivax-attributable mortality has been reported and confirmed globally (Beg et al., 2002; Kochar et al., 2005; Genton et al., 2008; Tjitra et al., 2008; Alexandre et al., 2010). In addition to the contribution that P. vivax contributes to significant morbidity and mortality, infections come with special complexity because the parasite can form a dormant hypnozoite stage in the liver that does not cause illness, but can re-emerge to cause a relapse infection from weeks to more than 1 year later, without mosquito transmission (Krotoski et al., 1982; Krotoski, 1985, 1989). Therefore, eliminating the hypnozoite(s) from infected people, termed radical cure, is essential for controlling P. vivax malaria. Achieving this requires a specific treatment as almost all approved antimalarials lack activity against hypnozoites (Thriemer et al., 2017). If P. vivax infection is treated without additional targeting of the liver-stage hypnozoite, the parasite is free to cross a broad geographic range, to persist across seasonal changes where mosquito vectors are absent, and to cause repeated clinical attacks, resulting in substantial cumulative morbidity. Thus, the chronic nature of P. vivax infections, mediated by hypnozoites, means that this parasite’s burden is harder to estimate (Anstey et al., 2012; Battle et al., 2016). Radical cure of P. vivax at the population level, therefore requires eliminating the hypnozoite reservoir to reach worldwide malaria elimination.
Currently, two drugs can kill hypnozoites and achieve radical cure, primaquine (PQ) and tafenoquine (TQ). Both drugs are 8-aminoquinoline (8AQ) derivatives (Wells et al., 2010; White, 2019). Although TQ has been approved by two national regulatory agencies (Australia in 2018; United States in 2019), PQ remains the only WHO-recommended drug to achieve radical cure (WHO approved in 1952 (Hill et al., 2006; World Health Organization, 2015). While effective against the liver stage hypnozoite, these 8AQ drugs are known to induce acute hemolytic anemia (AHA) in individuals who inherit the X-linked glucose-6-phosphate dehydrogenase enzyme deficiency (G6PDd); G6PDd is the most common human enzyme deficiency (Howes et al., 2012; Luzzatto et al., 2016), and it is in particularly high frequency in malaria endemic regions as it confers protection from severe malaria (Bienzle et al., 1979; Ruwende et al., 1995; Sirugo et al., 2014). As PQ is taken daily over 2 weeks (5 h half-life), treatment (0.25–0.5 mg base/kg body weight) can be stopped if any signs of AHA are observed; PQ can also be administered once each week over 8-week (0.75 mg base/kg body weigh) for G6PDd individuals (males or females with less than 30% G6PD enzyme activity (World Health Organization, 2016). Because of a much longer half-life (15 days), TQ is administered via a single dose, observation of AHA cannot be mitigated, and thus the elevated potential for harm to G6PDd individuals supports restricted use protocols and limited approvals (White, 2019). Notably, across the globe, G6PDd presents a barrier to universal implementation of mass drug administration against vivax malaria.
In the past 10 years, complexities of PQ pharmacogenetics have emerged as the therapeutic efficacy of PQ appears to be strongly correlated with activity of the highly polymorphic metabolic enzyme, cytochrome P450 2D6 (CYP2D6) (Bennett et al., 2013; Ingram et al., 2014; Silvino et al., 2016; Baird et al., 2018b; Brasil et al., 2018), an enzyme implicated in metabolism of >20% of marketed drugs (Gaedigk et al., 2018a). PQ metabolism involves three predominant pathways: 1) glucuronide/glucose/carbamate/acetate conjugation; 2) hydroxylation at multiple positions on the quinoline ring; and 3) oxidative deamination at the terminal amine of the aminoalkyl side chain (Avula et al., 2018). Despite increasing knowledge of PQ metabolism and how it is affected by CYP2D6, the active anti-malarial compound has still not been defined with certainty, although data now indicates activity is mediated through hydroxylated metabolites whose formation is CYP2D6 dependent (Camarda et al., 2019). In contrast, while pharmacogenetic studies focused on TQ have suggested no association with CYP2D6, only limited insight into the metabolism of this drug exists based on a single study (St Jean et al., 2016).
Building a global population genetic picture of CYP2D6 variation and how it relates to safety and effectiveness of 8AQ treatment is critical due to the potentially large hypnozoite reservoir in malaria, but also because these drugs can also play a role in limiting P. falciparum transmission through gametocytocidal activity (Single low dose PQ (0.25 mg/kg) blocks P. falciparum gametocyte transmission without adverse reactions in G6PDd individuals (Bancone et al., 2016)). Here we focus on current knowledge of population genetic variation of CYP2D6 and PQ metabolism in hopes of expanding the population that can use this drug effectively (Baird et al., 2018a) to reduce the hypnozoite reservoir. This is consistent with recent clinical trials to test optimal dosing strategies against relapse infections (Brito-Sousa et al., 2022; Chamma-Siqueira et al., 2022). An adjoining article by Stewart et al. focuses on implications of CYP2D6 and G6PD genetic variation on potential treatment approaches and limitations for P. vivax as well as P. ovale (that also produces hypnozoites) liver stage parasites (Stewart et al., 2021).
CYP2D6 genetic variation and metabolic Activity Scores
CYP2D6 is the most polymorphic of the CYP genes (Zhou et al., 2017). Currently, there are 145 known major “star” (*) alleles and multiple sub-alleles that include at least 128 unique SNPs (upstream non-coding and coding region), 7 insertions (one to multiple nucleotides or duplicated nucleotides), 7 deletions (1 to multiple nucleotides), 5 gene deletions, gene duplication, conversion or hybridization variations that can together generate more than 1,220 unique genotypes (Gaedigk et al., 2018b; Luo et al., 2021) (ongoing updates can be followed at https://www.pharmvar.org/gene/CYP2D6). Many of the mutations are quite rare (e.g. occurring in single individuals or isolated populations (Luo et al., 2021) and a significant number of resulting * alleles occur at frequencies less than 0.25%, and hence do not substantially affect drug metabolism at the population-level. That said, we have shown, in a study of CYP2D6 in a Malagasy population, that the unexpected appearance of some alleles associated with diverse populations, including new alleles, new allele frequency patterns, genotype combinations and genotype proportions may reveal population level patterns of special relevance to malaria treatment (Mehlotra et al., 2021; Chan et al., 2022).
Gaedigk et al. (2008) and PharmVar collaborators have further classified CYP2D6 function through metabolism of dextro-methorphan (DM) to dextrorphan (DX). They correlated * allele genotypes with DM/DX ratio to define an Activity Score (AS) metric. Although the AS likely represents a good approximation of the generation of active anti-malarial compounds, the challenge of associating CPY2D6 sequence variation with phenotypic effects is well appreciated (Gaedigk et al., 2018a; Pey, 2020). One example illustrating the complexity of the relationship between CYP2D6 genotype and AS was observed in a study of 270 Trinidadians by Montané et al. and summarized in Figure 1 (Montane Jaime et al., 2013) Herein, the most regular association between genotype and AS was among individuals who were homozygous for the same, or heterozygous for different nonfunctional alleles, *4 and *5, as they clearly were poor metabolizers (i.e., AS = 0). For other allelic combinations the average DM metabolism is observed to increase with genotypes categorized with increasing AS (Montane Jaime et al., 2013). However, the variance of metabolism can be very large within functional * allelic categories, as individuals with the same CYP2D6 genotype can differ in their metabolism of (DM) by up to 4 orders of magnitude (e.g. *1/*1; *1/*4) (Montane Jaime et al., 2013). Gaedigk et al. have discussed several factors that contribute to this AS variability, including SNPs influencing CYP2D6 gene and protein expression. They further call attention to missing or incomplete genetic information—where low or no-function alleles can be incorrectly assigned as *1 or *2 alleles, if specific SNP positions are not included in the genotyping strategy used (Gaedigk et al., 2018a). Of additional relevance, the AS of low activity CYP2D6*10 has recently downgraded to 0.25 (Caudle et al., 2020; Crews et al., 2021). These sources of genetic variation within CYP2D6 and genetic polymorphisms across the human genome create the potential to modify the phenotypic AS for probe drugs as well as drugs to treat malaria and other wide-ranging health conditions (implications are focused on PQ discussed in sections below).
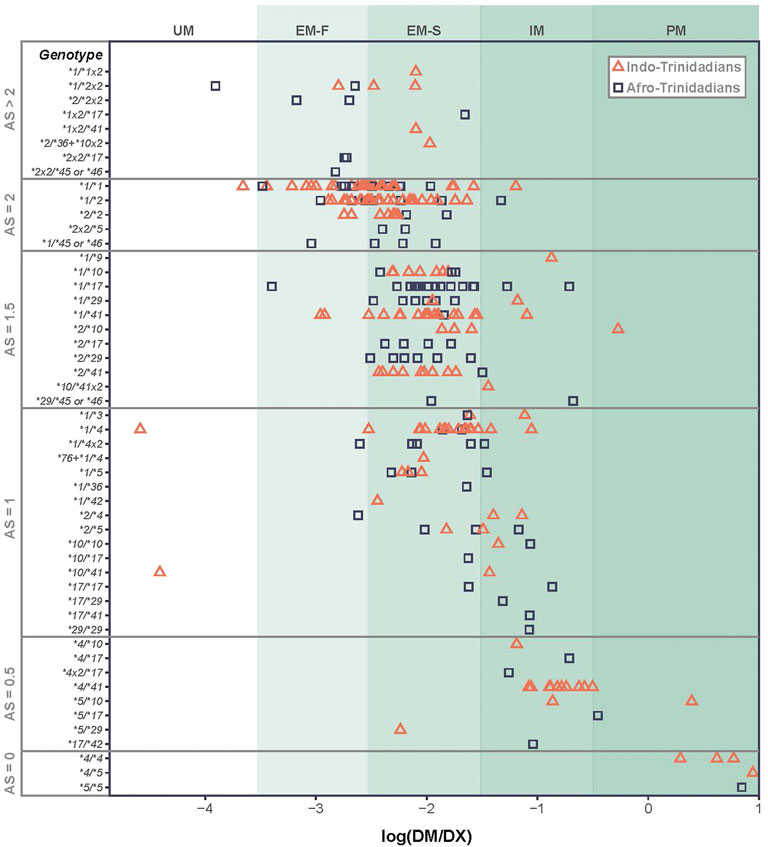
FIGURE 1. Variation in metabolism of dextro-methorphan (DM) to dextrorphan (DX) as a function of CYP2D6 * diplotypes. Diplotypes of the same AS and/or * designation can vary in metabolic rate by orders of magnitude, giving rise to ambiguity of our understanding of likelihood of radical cure based on these criteria.
Pharmacogenomics and CYP2D6 phenotype
Pharmacogenomics studies are certain to improve as large-scale sequence data are associated with phenotypic variation. Developing optimal study designs will require considerable discussion and beginning this process has been a motivation for this perspective. Cohort characteristics (size, gender, age-groups, and ethnicities) and sequencing strategies are key elements to consider. Ensuring sufficient power for detecting statistically significant associations between CYP2D6 genotype, genetic modifiers and AS are necessary to improve specific drug effectiveness on the population-level.
Recent efforts have begun to address the patterns of genetic variation in CYP2D6, although given the level of polymorphism in this gene they are so far limited in their scopes, sizes and generalizability (Del Tredici et al., 2018; Puaprasert et al., 2018; Nguyen et al., 2019; Chan et al., 2022). Cramer (Cramer, 2019) has provided information of how a comprehensive genomic approach can enhance our understanding of CYP2D6 pharmacogenomics. Key to future studies will be the need to determine if a limited number of SNPs can be converted into more tractable methods for assessing CYP2D6 genetic predictions of AS and whether these metrics relate only to a specific population. It will be important to determine if the same * allele in different populations is associated with AS the same functional variation, and whether genotype-phenotype association differences are based on unknown SNPs within CYP2D6 or result from unique distributions of modifiers in other genes distributed throughout the human genome.
With these topics in mind, 1,000 Genomes data, have been used in two studies to illustrate population characteristics of CYP2D6 variation in association with geographic distribution of P. vivax malaria (Puaprasert et al., 2018; Cramer, 2019). Puaprasert showed that simply increasing geographic distance within Asia from their sampled Karen population on the Thai-Myanmar border correlated with increasing genetic differentiation at this locus, indicating that the transferability of genetic information among diverse populations will likely be limited (Puaprasert et al., 2018). Cramer has used this global resource to illustrate first how null and low activity CYP2D6 alleles and genotypes are dispersed throughout the world and further characterize a Malagasy population studied by Mehlotra (Mehlotra et al., 2021) to illustrate how genetic admixture between Asian and African superpopulations may have affected CYP2D6 * allele distributions in this understudied population (Mehlotra et al., 2021). They further used the pattern of CYP2D6 dispersal to hypothesize how PQ may be metabolized and hence used to treat malaria in different populations. Resulting predictions of activity indicate that the use of PQ following current protocols may be more effective in some malaria-endemic regions than in others as a result of high frequencies of poor metabolizer alleles in East Asia. Stewart et al. have called further attention to similar population-specific concerns in Central America as summarized previously (Stewart et al., 2021).
A recent clinical study of 57 Javanese male soldiers who had P. vivax malaria and received the same PQ regimen has provided insight into the complexities of CY2D6 genetic polymorphism, associated AS and P. vivax relapse. Among 21 individuals who relapsed, AS score was <1.5 for 18 and ≥1.5 for 3 individuals whereas of the 36 who did not relapse 14 had an AS <1.5 and 22 had an AS of ≥1.5. The results indicate that CYP2D6 genotypes associated with but did not completely predict PQ efficacy against P. vivax relapse. The AS did have utility, but the study emphasized the need to assess how additional variation at CYP2D6 and elsewhere in the genome, can contribute to PQ metabolism and treatment efficacy. That the role of genotypes on radical cure is less than straightforward was further shown in a study conducted by the Australian Defence Force who returned from Papua New Guinea and East Timor (Chen et al., 2019). In this study, AS was not associated with radical cure, except in the case of non-functional alleles that associated with no cure.
Takeaways from these studies indicate that we need to better understand the allelic variation within single * alleles that are used to delineate AS variation and how patterns of these unknown genetic variants affect gene function. As a number of different sequencing and PCR-based strategies have been used to generate the data in these and other studies, limitations will be encountered in comparing genotype and phenotype associations both within and between studies. We also need to know how genetic variations within CYP2D6 are distributed among human populations, as well as variation in the genes that interact with CYP2D6, to optimize PQ treatment and radical cure. Finally, it will be important to ask if and to what extent variation in CPY2D6 genotype alters risks associated with G6PDd. Specifically, would AS <1 require more than 8 weeks of PQ treatment?
External confounding factors
Beyond the intricacies of gene-specific and genome-wide variation, there are examples that other drugs, foods or herbal treatments can interact with PQ and influence biological phenotypes. Examples include other antimalarial drugs (e.g. chloroquine) (Pukrittayakamee et al., 2014; Fasinu et al., 2016), or medicinal herbal plants commonly found in the tropics and sub-tropics (e.g. Hyptis suaveolens (bush mint) (Thomford et al., 2018). It was observed that chloroquine (still used to treat vivax malaria) administration resulted in increased PQ plasma concentrations (Pukrittayakamee et al., 2014), and inhibited formation of several PQ metabolites predicted to be active against hypnozoites (Avula et al., 2018). Similarly, H. suaveolens can inhibit CYP2D6 in a reversible and time dependent manner; therefore administration of PQ for radical cure needs to be done in full knowledge of these and likely other interacting compounds (Thomford et al., 2018).
We must also be mindful of the potential that PQ efficacy may be influenced by the parasite’s genetic constitution. Traditional approaches for verifying the effectiveness of antimalarial drugs includes testing genetic signatures of pre- and post-treatment infections using highly polymorphic parasite genes (e.g. circumsporozoite protein or merozoite surface protein (Imwong et al., 2007)). However, approaches based on only one or two genes limit the ability to distinguish multiple strains, and a broader genomics strategy has been proposed for detecting evidence of relapse (Popovici et al., 2019). Regardless of the molecular marker strategy to decipher strains in complex infections, assessing recrudescence, reinfection or relapse will continue to pose a significant challenge to studies monitoring drug effectiveness (Ferreira et al., 2021). For CYP2D6 studies this means that it will be important to determine if a relapse has resulted from lower-than-expected metabolism of PQ by the infected person, parasite resistance to PQ, and/or reinfection.
Parting thoughts
The primary themes corresponding to genetic polymorphism associated with safety and effectiveness of PQ treatment are summarized in Figure 2 and frames the path forward for radical cure of the hypnozoite reservoir. Treatment safety is the main concern that has held back the use of PQ for radical cure of hypnozoite infection globally. Emerging point-of-care technologies that demonstrate capacity to perform quantitative assessment of G6PD enzyme activity, have shown promise in repeatability and reproducibility assessments (Ley et al., 2022). If greater precision can be achieved in determining G6PD enzyme activity at the point of PQ treatment, it may become possible to administer this drug safely to a many more G6PDd individuals (those with 5–30% enzyme activity; Figure 2, part A2). Better tools for determining G6PD activity would certainly increase confidence in treating those with 30–80% enzyme activity (Figure 2, part B). Regardless of G6PD enzyme activity, the remaining concern is whether sufficient concentrations of the pharmacologically active PQ metabolites will be generated to kill hypnozoites.
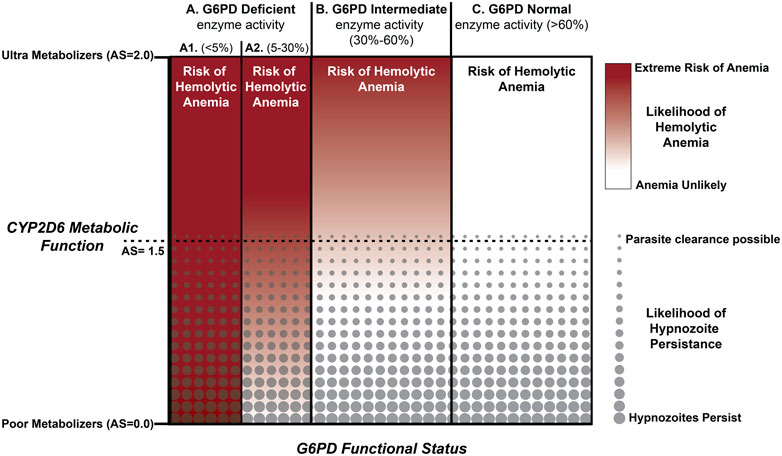
FIGURE 2. Integration of CPY2D6 Metabolic Function and G6PD Enzyme Activity. Those with a CYP2D6 AS < 1.5 are predicted to have an elevated risk of relapse. PQ failure with AS>1.5 appears to be less common and for those with AS > 2.0 radical cure success improves substantially following current standard of care. Integrated across the familiar G6PD enzyme activity benchmarks, potential exists for optimizing treatment strategies for all but those individuals with <5% G6PD enzyme activity.
Finally, while current knowledge limits our ability to make inferences about appropriate dosing, at either the population or individual levels, it is important to continue to improve knowledge of the genotype to phenotype map so that we can more accurately predict PQ metabolism to provide more effective and safer treatment in line with current Clinical Pharmacogenetics Implementation Consortium (CPIC) guidelines (Crews et al., 2021). Simply, we need to consider how genetic and genomics technologies can drive the future of anti-malarial treatment as the motivation and ability to genotype is shifting rapidly. This is increasingly evident where malaria is endemic as reports now encourage expanding diversity in genomic studies (Sirugo et al., 2021; Krainc and Fuentes, 2022) and subsequent improved implementation (Wonkam, 2021; Chimusa et al., 2022). Many such practices are already in place in cancer studies around the world. As a result of these efforts, capacity to perform complex genotyping has advanced in fields of knowledge, attitudes and practice (KAP) (Muzoriana et al., 2017; Rahma et al., 2021; Koufaki et al., 2022), pharmacogenomics (Marsh et al., 2006), diabetes and hematology (Kountouris et al., 2021) globally. Additionally, studies focused on understanding patterns of genetic variation are advancing and will expand capacity to resolve their health implications in diverse populations (Taliun et al., 2021; DiCorpo et al., 2022). Given what we are observing across human genomics investigations, efforts are well underway to extend the breakthroughs available through genomics technologies as a global health mission and must be included as part of malaria elimination strategies.
Author contributions
JO wrote the first draft and designed the figures. SW and PZ designed the research and secured the funding for the overall study. All authors approved the final version.
Funding
This study was supported by grants from the National Institutes of Health, United States (1R01AI148469 to SW and PZ). JO was supported through grants from the National Institutes of Health, United States by T32HL007567 and National Heart, Lung, and Blood Institute, BioData Catalyst program (award 1OT3HL142479-01, 1OT3HL142478-01, 1OT3HL142481-01, 1OT3HL142480-01, 1OT3HL147154-01).
Acknowledgments
We thank James McCarthy and Alexandra Stewart for critical review of our manuscript.
Conflict of interest
The authors declare that the research was conducted in the absence of any commercial or financial relationships that could be construed as a potential conflict of interest.
Publisher’s note
All claims expressed in this article are solely those of the authors and do not necessarily represent those of their affiliated organizations, or those of the publisher, the editors and the reviewers. Any product that may be evaluated in this article, or claim that may be made by its manufacturer, is not guaranteed or endorsed by the publisher.
References
Alexandre, M. A., Ferreira, C. O., Siqueira, A. M., Magalhaes, B. L., Mourao, M. P., Lacerda, M. V., et al. (2010). Severe Plasmodium vivax malaria, Brazilian amazon. Emerg. Infect. Dis. 16 (10), 1611–1614. doi:10.3201/eid1610.100685
Anstey, N. M., Douglas, N. M., Poespoprodjo, J. R., and Price, R. N. (2012). Plasmodium vivax: Clinical spectrum, risk factors and pathogenesis. Adv. Parasitol. 80, 151–201. doi:10.1016/B978-0-12-397900-1.00003-7
Avula, B., Tekwani, B. L., Chaurasiya, N. D., Fasinu, P., Dhammika Nanayakkara, N. P., Bhandara Herath, H. M. T., et al. (2018). Metabolism of primaquine in normal human volunteers: Investigation of phase I and phase II metabolites from plasma and urine using ultra-high performance liquid chromatography-quadrupole time-of-flight mass spectrometry. Malar. J. 17 (1), 294. doi:10.1186/s12936-018-2433-z
Baird, J. K., Battle, K. E., and Howes, R. E. (2018a). Primaquine ineligibility in anti-relapse therapy of Plasmodium vivax malaria: The problem of G6PD deficiency and cytochrome P-450 2D6 polymorphisms. Malar. J. 17 (1), 42. doi:10.1186/s12936-018-2190-z
Baird, J. K., Louisa, M., Noviyanti, R., Ekawati, L., Elyazar, I., Subekti, S., et al. (2018b). Association of impaired cytochrome P450 2D6 activity genotype and phenotype with therapeutic efficacy of primaquine treatment for latent Plasmodium vivax malaria. JAMA Netw. Open 1 (4), e181449. doi:10.1001/jamanetworkopen.2018.1449
Bancone, G., Chowwiwat, N., Somsakchaicharoen, R., Poodpanya, L., Moo, P. K., Gornsawun, G., et al. (2016). Single low dose primaquine (0.25 mg/kg) does not cause clinically significant haemolysis in G6PD deficient subjects. PLoS One 11 (3), e0151898. doi:10.1371/journal.pone.0151898
Barcus, M. J., Basri, H., Picarima, H., Manyakori, C., Sekartuti, E. I., Bangs, M. J., et al. (2007). Demographic risk factors for severe and fatal vivax and falciparum malaria among hospital admissions in northeastern Indonesian Papua. Am. J. Trop. Med. Hyg. 77 (5), 984–991. doi:10.4269/ajtmh.2007.77.984
Battle, K. E., Bisanzio, D., Gibson, H. S., Bhatt, S., Cameron, E., Weiss, D. J., et al. (2016). Treatment-seeking rates in malaria endemic countries. Malar. J. 15 (1), 20. doi:10.1186/s12936-015-1048-x
Beg, M. A., Khan, R., Baig, S. M., Gulzar, Z., Hussain, R., and Smego, J. (2002). Cerebral involvement in benign tertian malaria. Am. J. Trop. Med. Hyg. 67 (3), 230–232. doi:10.4269/ajtmh.2002.67.230
Bennett, J. W., Pybus, B. S., Yadava, A., Tosh, D., Sousa, J. C., McCarthy, W. F., et al. (2013). Primaquine failure and cytochrome P-450 2D6 in Plasmodium vivax malaria. N. Engl. J. Med. 369 (14), 1381–1382. doi:10.1056/NEJMc1301936
Bienzle, U., Guggenmoos-Holzmann, I., and Luzzatto, L. (1979). Malaria and erythrocyte glucose-6-phosphate dehydrogenase variants in West Africa. Am. J. Trop. Med. Hyg. 28 (4), 619–621. doi:10.4269/ajtmh.1979.28.619
Brasil, L. W., Rodrigues-Soares, F., Santoro, A. B., Almeida, A. C. G., Kuhn, A., Ramasawmy, R., et al. (2018). CYP2D6 activity and the risk of recurrence of Plasmodium vivax malaria in the Brazilian amazon: A prospective cohort study. Malar. J. 17 (1), 57. doi:10.1186/s12936-017-2139-7
Brito-Sousa, J. D., Phanor, J., Balieiro, P., Silva-Neto, A. V., Cordeiro, J. S. M., Vitor-Silva, S., et al. (2022). Effect of weekly versus daily primaquine on Plasmodium vivax malaria recurrences: A real-life cohort study. Rev. Soc. Bras. Med. Trop. 55, e07382021. doi:10.1590/0037-8682-0738-2021
Camarda, G., Jirawatcharadech, P., Priestley, R. S., Saif, A., March, S., Wong, M. H. L., et al. (2019). Antimalarial activity of primaquine operates via a two-step biochemical relay. Nat. Commun. 10 (1), 3226. doi:10.1038/s41467-019-11239-0
Caudle, K. E., Sangkuhl, K., Whirl-Carrillo, M., Swen, J. J., Haidar, C. E., Klein, T. E., et al. (2020). Standardizing CYP2D6 genotype to phenotype translation: Consensus recommendations from the clinical pharmacogenetics implementation Consortium and Dutch pharmacogenetics working group. Clin. Transl. Sci. 13 (1), 116–124. doi:10.1111/cts.12692
Chamma-Siqueira, N. N., Negreiros, S. C., Ballard, S. B., Farias, S., Silva, S. P., Chenet, S. M., et al. (2022). Higher-dose primaquine to prevent relapse of Plasmodium vivax malaria. N. Engl. J. Med. 386 (13), 1244–1253. doi:10.1056/NEJMoa2104226
Chan, E. R., Mehlotra, R. K., Pirani, K. A., Ratsimbasoa, A. C., Williams, S. M., Gaedigk, A., et al. (2022). CYP2D6 gene resequencing in the Malagasy, a population at the crossroads between Asia and africa: A pilot study. Pharmacogenomics 23 (5), 315–325. doi:10.2217/pgs-2021-0146
Chen, N., Dowd, S., Gatton, M. L., Auliff, A., Edstein, M. D., and Cheng, Q. (2019). Cytochrome P450 2D6 profiles and their relationship with outcomes of primaquine anti-relapse therapy in Australian Defence Force personnel deployed to Papua New Guinea and East Timor. Malar. J. 18 (1), 140. doi:10.1186/s12936-019-2774-2
Chimusa, E. R., Alosaimi, S., and Bope, C. D. (2022). Dissecting generalizability and actionability of disease-associated genes from 20 worldwide ethnolinguistic cultural groups. Front. Genet. 13, 835713. doi:10.3389/fgene.2022.835713
Cramer, E. Y. (2019). Assessing the global prevalence of CYP2D6 haplotype variation with implications for primaquine distribution and Plasmodium vivax control. Masters of public health. Cleveland, OH: Case Western Reserve University
Crews, K. R., Monte, A. A., Huddart, R., Caudle, K. E., Kharasch, E. D., Gaedigk, A., et al. (2021). Clinical pharmacogenetics implementation Consortium guideline for CYP2D6, OPRM1, and COMT genotypes and select opioid therapy. Clin. Pharmacol. Ther. 110, 888–896. doi:10.1002/cpt.2149
Del Tredici, A. L., Malhotra, A., Dedek, M., Espin, F., Roach, D., Zhu, G. D., et al. (2018). Frequency of CYP2D6 alleles including structural variants in the United States. Front. Pharmacol. 9, 305. doi:10.3389/fphar.2018.00305
DiCorpo, D., Gaynor, S. M., Russell, E. M., Westerman, K. E., Raffield, L. M., Majarian, T. D., et al. (2022). Whole genome sequence association analysis of fasting glucose and fasting insulin levels in diverse cohorts from the NHLBI TOPMed program. Commun. Biol. 5 (1), 756. doi:10.1038/s42003-022-03702-4
Fasinu, P. S., Tekwani, B. L., Avula, B., Chaurasiya, N. D., Nanayakkara, N. P., Wang, Y. H., et al. (2016). Pathway-specific inhibition of primaquine metabolism by chloroquine/quinine. Malar. J. 15, 466. doi:10.1186/s12936-016-1509-x
Fernandez-Becerra, C., Pinazo, M. J., Gonzalez, A., Alonso, P. L., del Portillo, H. A., and Gascon, J. (2009). Increased expression levels of the pvcrt-o and pvmdr1 genes in a patient with severe Plasmodium vivax malaria. Malar. J. 8, 55. doi:10.1186/1475-2875-8-55
Ferreira, M. U., Nobrega de Sousa, T., Rangel, G. W., Johansen, I. C., Corder, R. M., Ladeia-Andrade, S., et al. (2021). Monitoring Plasmodium vivax resistance to antimalarials: Persisting challenges and future directions. Int. J. Parasitol. Drugs Drug Resist. 15, 9–24. doi:10.1016/j.ijpddr.2020.12.001
Gaedigk, A., Dinh, J. C., Jeong, H., Prasad, B., and Leeder, J. S. (2018a). Ten years' experience with the CYP2D6 activity score: A perspective on future investigations to improve clinical predictions for precision therapeutics. J. Pers. Med. 8 (2), E15. doi:10.3390/jpm8020015
Gaedigk, A., Ingelman-Sundberg, M., Miller, N. A., Leeder, J. S., Whirl-Carrillo, M., Klein, T. E., et al. (2018b). The pharmacogene variation (PharmVar) Consortium: Incorporation of the human cytochrome P450 (CYP) allele nomenclature database. Clin. Pharmacol. Ther. 103 (3), 399–401. doi:10.1002/cpt.910
Gaedigk, A., Simon, S. D., Pearce, R. E., Bradford, L. D., Kennedy, M. J., and Leeder, J. S. (2008). The CYP2D6 activity score: Translating genotype information into a qualitative measure of phenotype. Clin. Pharmacol. Ther. 83 (2), 234–242. doi:10.1038/sj.clpt.6100406
Genton, B., D'Acremont, V., Rare, L., Baea, K., Reeder, J. C., Alpers, M. P., et al. (2008). Plasmodium vivax and mixed infections are associated with severe malaria in children: A prospective cohort study from Papua New Guinea. PLoS Med. 5 (6), e127. doi:10.1371/journal.pmed.0050127
Gething, P. W., Elyazar, I. R., Moyes, C. L., Smith, D. L., Battle, K. E., Guerra, C. A., et al. (2012). A long neglected world malaria map: Plasmodium vivax endemicity in 2010. PLoS Negl. Trop. Dis. 6 (9), e1814. doi:10.1371/journal.pntd.0001814
Gething, P. W., Patil, A. P., Smith, D. L., Guerra, C. A., Elyazar, I. R., Johnston, G. L., et al. (2011). A new world malaria map: Plasmodium falciparum endemicity in 2010. Malar. J. 10, 378. doi:10.1186/1475-2875-10-378
Hill, D. R., Baird, J. K., Parise, M. E., Lewis, L. S., Ryan, E. T., and Magill, A. J. (2006). Primaquine: Report from CDC expert meeting on malaria chemoprophylaxis I. Am. J. Trop. Med. Hyg. 75 (3), 402–415. doi:10.4269/ajtmh.2006.75.402
Howes, R. E., Piel, F. B., Patil, A. P., Nyangiri, O. A., Gething, P. W., Dewi, M., et al. (2012). G6PD deficiency prevalence and estimates of affected populations in malaria endemic countries: A geostatistical model-based map. PLoS Med. 9 (11), e1001339. doi:10.1371/journal.pmed.1001339
Imwong, M., Snounou, G., Pukrittayakamee, S., Tanomsing, N., Kim, J. R., Nandy, A., et al. (2007). Relapses of Plasmodium vivax infection usually result from activation of heterologous hypnozoites. J. Infect. Dis. 195 (7), 927–933. doi:10.1086/512241
Ingram, R. J., Crenna-Darusallam, C., Soebianto, S., Noviyanti, R., and Baird, J. K. (2014). The clinical and public health problem of relapse despite primaquine therapy: Case review of repeated relapses of Plasmodium vivax acquired in Papua New Guinea. Malar. J. 13 (1), 488. doi:10.1186/1475-2875-13-488
Kochar, D. K., Das, A., Kochar, S. K., Saxena, V., Sirohi, P., Garg, S., et al. (2009). Severe Plasmodium vivax malaria: A report on serial cases from bikaner in northwestern India. Am. J. Trop. Med. Hyg. 80 (2), 194–198. doi:10.4269/ajtmh.2009.80.194
Kochar, D. K., Saxena, V., Singh, N., Kochar, S. K., Kumar, S. V., and Das, A. (2005). Plasmodium vivax malaria. Emerg. Infect. Dis. 11 (1), 132–134. doi:10.3201/eid1101.040519
Koufaki, M. I., Siamoglou, S., Patrinos, G. P., and Vasileiou, K. (2022). Examining key factors impact on health science students' intentions to adopt genetic and pharmacogenomics testing: A comparative path analysis in two different healthcare settings. Hum. Genomics 16 (1), 9. doi:10.1186/s40246-022-00382-3
Kountouris, P., Stephanou, C., Archer, N., Bonifazi, F., Giannuzzi, V., Kuo, K. H. M., et al. (2021). The International Hemoglobinopathy Research Network (INHERENT): An international initiative to study the role of genetic modifiers in hemoglobinopathies. Am. J. Hematol. 96 (11), E416–E420. doi:10.1002/ajh.26323
Krainc, T., and Fuentes, A. (2022). Genetic ancestry in precision medicine is reshaping the race debate. Proc. Natl. Acad. Sci. U. S. A. 119 (12), e2203033119. doi:10.1073/pnas.2203033119
Krotoski, W. A., Collins, W. E., Bray, R. S., Garnham, P. C., Cogswell, F. B., Gwadz, R. W., et al. (1982). Demonstration of hypnozoites in sporozoite-transmitted Plasmodium vivax infection. Am. J. Trop. Med. Hyg. 31 (6), 1291–1293. doi:10.4269/ajtmh.1982.31.1291
Krotoski, W. A. (1985). Discovery of the hypnozoite and a new theory of malarial relapse. Trans. R. Soc. Trop. Med. Hyg. 79 (1), 1–11. doi:10.1016/0035-9203(85)90221-4
Ley, B., Winasti Satyagraha, A., Kibria, M. G., Armstrong, J., Bancone, G., Bei, A. K., et al. (2022). Repeatability and reproducibility of a handheld quantitative G6PD diagnostic. PLoS Negl. Trop. Dis. 16 (2), e0010174. doi:10.1371/journal.pntd.0010174
Luo, S., Jiang, R., Grzymski, J. J., Lee, W., Lu, J. T., and Washington, N. L. (2021). Comprehensive allele genotyping in critical pharmacogenes reduces residual clinical risk in diverse populations. Clin. Pharmacol. Ther. 110, 759–767. doi:10.1002/cpt.2279
Luzzatto, L., Nannelli, C., and Notaro, R. (2016). Glucose-6-Phosphate dehydrogenase deficiency. Hematol. Oncol. Clin. North Am. 30 (2), 373–393. doi:10.1016/j.hoc.2015.11.006
Marsh, S., Van Booven, D. J., and McLeod, H. L. (2006). Global pharmacogenetics: Giving the genome to the masses. Pharmacogenomics 7 (4), 625–631. doi:10.2217/14622416.7.4.625
Mehlotra, R. K., Gaedigk, A., Howes, R. E., Rakotomanga, T. A., Ratsimbasoa, A. C., and Zimmerman, P. A. (2021). CYP2D6 genetic variation and its implication for vivax malaria treatment in Madagascar. Front. Pharmacol. 12, 654054. doi:10.3389/fphar.2021.654054
Montane Jaime, L. K., Lalla, A., Steimer, W., and Gaedigk, A. (2013). Characterization of the CYP2D6 gene locus and metabolic activity in indo- and afro-Trinidadians: Discovery of novel allelic variants. Pharmacogenomics 14 (3), 261–276. doi:10.2217/pgs.12.207
Muzoriana, N., Gavi, S., Nembaware, V., Dhoro, M., and Matimba, A. (2017). Knowledge, attitude, and perceptions of pharmacists and pharmacy students towards pharmacogenomics in Zimbabwe. Pharm. (Basel) 5 (3), E36. doi:10.3390/pharmacy5030036
Nguyen, H. H., Ma, T. T. H., Vu, N. P., Bach, Q. T. N., Vu, T. H., Nguyen, T. D., et al. (2019). Single nucleotide and structural variants of CYP2D6 gene in Kinh Vietnamese population. Med. Baltim. 98 (22), e15891. doi:10.1097/MD.0000000000015891
Pey, A. L. (2020). Towards accurate genotype-phenotype correlations in the CYP2D6 gene. J. Pers. Med. 10 (4), E158. doi:10.3390/jpm10040158
Popovici, J., Pierce-Friedrich, L., Kim, S., Bin, S., Run, V., Lek, D., et al. (2019). Recrudescence, reinfection, or relapse? A more rigorous framework to assess chloroquine efficacy for Plasmodium vivax malaria. J. Infect. Dis. 219 (2), 315–322. doi:10.1093/infdis/jiy484
Puaprasert, K., Chu, C., Saralamba, N., Day, N. P. J., Nosten, F., White, N. J., et al. (2018). Real time PCR detection of common CYP2D6 genetic variants and its application in a Karen population study. Malar. J. 17 (1), 427. doi:10.1186/s12936-018-2579-8
Pukrittayakamee, S., Tarning, J., Jittamala, P., Charunwatthana, P., Lawpoolsri, S., Lee, S. J., et al. (2014). Pharmacokinetic interactions between primaquine and chloroquine. Antimicrob. Agents Chemother. 58 (6), 3354–3359. doi:10.1128/AAC.02794-13
Rahma, A. T., Elbarazi, I., Ali, B. R., Patrinos, G. P., Ahmed, L. A., Elsheik, M., et al. (2021). Development of the pharmacogenomics and genomics literacy framework for pharmacists. Hum. Genomics 15 (1), 62. doi:10.1186/s40246-021-00361-0
Ruwende, C., Khoo, S. C., Snow, R. W., Yates, S. N., Kwiatkowski, D., Gupta, S., et al. (1995). Natural selection of hemi- and heterozygotes for G6PD deficiency in Africa by resistance to severe malaria. Nature 376 (6537), 246–249. doi:10.1038/376246a0
Silvino, A. C., Costa, G. L., Araujo, F. C., Ascher, D. B., Pires, D. E., Fontes, C. J., et al. (2016). Variation in human cytochrome P-450 drug-metabolism genes: A gateway to the understanding of Plasmodium vivax relapses. PLoS One 11 (7), e0160172. doi:10.1371/journal.pone.0160172
Siqueira, A. M., Alexandre, M. A., Mourao, M. P., Santos, V. S., Nagahashi-Marie, S. K., Alecrim, M. G., et al. (2010). Severe rhabdomyolysis caused by Plasmodium vivax malaria in the Brazilian Amazon. Am. J. Trop. Med. Hyg. 83 (2), 271–273. doi:10.4269/ajtmh.2010.10-0027
Sirugo, G., Predazzi, I. M., Bartlett, J., Tacconelli, A., Walther, M., and Williams, S. M. (2014). G6PD A- deficiency and severe malaria in the Gambia: Heterozygote advantage and possible homozygote disadvantage. Am. J. Trop. Med. Hyg. 90 (5), 856–859. doi:10.4269/ajtmh.13-0622
Sirugo, G., Tishkoff, S. A., and Williams, S. M. (2021). The quagmire of race, genetic ancestry, and health disparities. J. Clin. Invest. 131 (11), 150255. doi:10.1172/JCI150255
St Jean, P. L., Xue, Z., Carter, N., Koh, G. C., Duparc, S., Taylor, M., et al. (2016). Tafenoquine treatment of Plasmodium vivax malaria: Suggestive evidence that CYP2D6 reduced metabolism is not associated with relapse in the phase 2b DETECTIVE trial. Malar. J. 15, 97. doi:10.1186/s12936-016-1145-5
Stewart, A. G. A., Zimmerman, P. A., and McCarthy, J. S. (2021). Genetic variation of G6PD and CYP2D6: Clinical implications on the use of primaquine for elimination of Plasmodium vivax. Front. Pharmacol. 12, 784909. doi:10.3389/fphar.2021.784909
Taliun, D., Harris, D. N., Kessler, M. D., Carlson, J., Szpiech, Z. A., Torres, R., et al. (2021). Sequencing of 53, 831 diverse genomes from the NHLBI TOPMed Program. Nature 590 (7845), 290–299. doi:10.1038/s41586-021-03205-y
Thomford, N. E., Dzobo, K., Adu, F., Chirikure, S., Wonkam, A., and Dandara, C. (2018). Bush mint (Hyptis suaveolens) and spreading hogweed (Boerhavia diffusa) medicinal plant extracts differentially affect activities of CYP1A2, CYP2D6 and CYP3A4 enzymes. J. Ethnopharmacol. 211, 58–69. doi:10.1016/j.jep.2017.09.023
Thriemer, K., Ley, B., Bobogare, A., Dysoley, L., Alam, M. S., Pasaribu, A. P., et al. (2017). Challenges for achieving safe and effective radical cure of Plasmodium vivax: A round table discussion of the APMEN vivax working group. Malar. J. 16 (1), 141. doi:10.1186/s12936-017-1784-1
Tjitra, E., Anstey, N. M., Sugiarto, P., Warikar, N., Kenangalem, E., Karyana, M., et al. (2008). Multidrug-resistant Plasmodium vivax associated with severe and fatal malaria: A prospective study in Papua, Indonesia. PLoS Med. 5 (6), e128. doi:10.1371/journal.pmed.0050128
Wells, T. N., Burrows, J. N., and Baird, J. K. (2010). Targeting the hypnozoite reservoir of Plasmodium vivax: The hidden obstacle to malaria elimination. Trends Parasitol. 26 (3), 145–151. doi:10.1016/j.pt.2009.12.005
White, N. J. (2019). Tafenoquine - a radical improvement? N. Engl. J. Med. 380 (3), 285–286. doi:10.1056/NEJMe1816383
Wonkam, A. (2021). Sequence three million genomes across Africa. Nature 590 (7845), 209–211. doi:10.1038/d41586-021-00313-7
World Health Organization (2015). Guidelines for the treatment of malaria. Geneva: World Health Organization.
World Health Organization (2016). Testing for G6PD deficiency for safe use of primaquine in radical cure of P. vivax and P. ovale malaria. Geneva: Global Malaria Programme WHO.
Keywords: malaria, hypnozoite, CYP2D6, activity score, primaquine
Citation: Olvany JM, Williams SM and Zimmerman PA (2022) Global perspectives on CYP2D6 associations with primaquine metabolism and Plasmodium vivax radical cure. Front. Pharmacol. 13:752314. doi: 10.3389/fphar.2022.752314
Received: 02 August 2021; Accepted: 27 October 2022;
Published: 15 November 2022.
Edited by:
Germana Bancone, Centre for Tropical Medicine and Global Health, University of Oxford, United KingdomReviewed by:
G. Dennis Shanks, Australian Defence Force Malaria and Infectious Diseases Institute (ADFMIDI), AustraliaMichele Spring, Armed Forces Research Institute of Medical Science, Thailand
Copyright © 2022 Olvany, Williams and Zimmerman. This is an open-access article distributed under the terms of the Creative Commons Attribution License (CC BY). The use, distribution or reproduction in other forums is permitted, provided the original author(s) and the copyright owner(s) are credited and that the original publication in this journal is cited, in accordance with accepted academic practice. No use, distribution or reproduction is permitted which does not comply with these terms.
*Correspondence: Scott M. Williams, smw154@case.edu; Peter A. Zimmerman, paz@case.edu