- School of Pharmacy, Key Laboratory of Molecular Pharmacology and Drug Evaluation (Yantai University), Ministry of Education, Collaborative Innovation Center of Advanced Drug Delivery System and Biotech Drugs in Universities of Shandong, Yantai University, Yantai, China
Chemodynamic therapy (CDT), a newly developed approach for cancer treatment, can convert hydrogen peroxide (H2O2) into toxic hydroxyl radicals (•OH) by using Fenton/Fenton-like reaction to kill tumor cells. However, due to the complexity of the intracellular environment of tumor cells, the therapeutic efficacy of CDT was severely restricted. Recently, combination therapy strategies have become popular approaches for tumor treatment, and there are numerous studies have demonstrated that the CDT-based combination strategies can significantly improve the anti-tumor efficiency of CDT. In this review, we outline some of the recent progress in cancer chemodynamic therapy from 2020, and discuss the progress in the design of nanosystems for CDT synergistic combination therapies.
Introduction
Currently, malignant tumor has become a major threat to human health, considerable attention has been paid to explore more effective therapeutic strategies for cancer treatment (Cao M. et al., 2020; Ferlay et al., 2021; Sung et al., 2021). With the development of nanoscience and nanobiotechnology, significant progress has been achieved in cancer therapy in the last decades (Wicki et al., 2015; Lin et al., 2022). However, effective treatment for cancer still presents significant challenges, for example, multidrug resistance is one of the difficult issues in current clinical chemotherapy treatment. Therefore, it is particularly necessary to develop new therapeutic agents and approaches for the more satisfactory cancer treatment (Rasool et al., 2022). In recent years, chemodynamic therapy (CDT) has attracted extensive attention in the field of tumor therapy.
CDT is inherently oxidative stress-induced cell killing process, it was first proposed in 2016 (Zhang et al., 2016). Briefly, by using the transition metal ions (Fe2+, Cu+, Mn2+, and so on), intracellular hydrogen peroxide (H2O2) is catalyzed to generate high reactivity hydroxyl radical (•OH), which would induce oxidative stress and further the death of cancer cells (Zhang X. et al., 2020; Chen et al., 2020; Zhang H. et al., 2021) (Figure 1). Generally, in order to improve the specificity and therapeutic efficacy of CDT, those CDT agents are usually designed as nanosystems. In this review, recent advancements of nanosystems for enhancing the CDT application and efficiency are reviewed. The major obstacles for the clinical applications of CDT are introduced, and those CDT enhanced strategies that CDT in combination with other therapies are focused and emphasized, such as combined with photothermal therapy, immunotherapy, sonodynamic therapy, and so on. The present review aims to provide better understanding of how to design effective chemodynamic agents, thus to broaden the application of CDT and maximize the CDT therapeutic effectiveness.
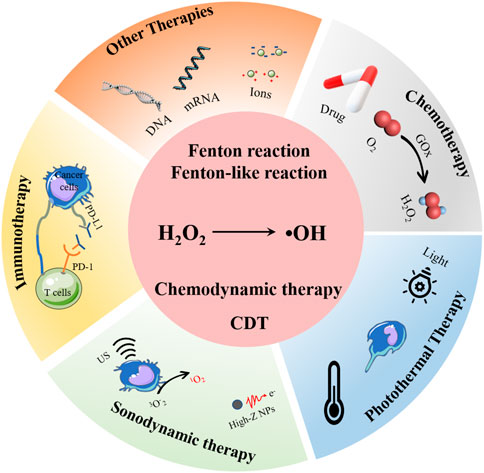
FIGURE 1. CDT and CDT-based combined therapy strategies, including combination with chemotherapy, photothermal therapy, sonodynamic therapy, and immunotherapy.
Chemodynamic therapy
It is well known that the tumor microenvironment (TME) exhibits unique physiological features compare with the normal tissues, including acidic pH, hypoxia, overproduced H2O2, the alteration of specific enzyme activities and elevated level of glutathione (GSH) (Roma-Rodrigues et al., 2019; Zhang Y. et al., 2022). On one side, these features of TME play crucial roles in tumor initiation, progression, metastasis, and even resistance to therapy. On the other side, the unique physiological conditions of TME can also be used as the stimuli to trigger the antitumor drug release at tumor sites to improve antitumor efficacy, which are known as the TME stimuli-responsive drug delivery systems (Wu and Dai, 2017; Li et al., 2021).
Considering the acidic and H2O2 overexpressed conditions in TME, the concept of CDT was proposed as a therapeutic strategy that using Fenton or Fenton-like reaction to generate hydroxyl radical in tumor site. In the first report of CDT, ferrous ions (Fe2+) was released from iron-based nanomaterials, which could catalyze endogenous H2O2 decomposition into •OH under acidic conditions of TME (Fe2+ + H2O2 → Fe3+ + •OH + OH−, known as Fenton reaction). The over produced •OH, one type of cytotoxic reactive oxygen species (ROS), had strong oxidative activity and caused severe oxidative damage to induce tumor apoptosis by phospholipid peroxidation, mitochondrial dysfunctions, and DNA damage (Khan et al., 2012; Srinivas et al., 2019).
Besides Fenton reaction, Fenton-like reactions are also employed for CDT application. Not restricted to iron, other reactive metal components, such as Cu+, Mn2+, Mo6+, are used in Fenton-like reaction to catalyze the generation of •OH from H2O2 (Cao et al., 2019, Cao et al., 2020 S.; Liang et al., 2021, 2022; Lin et al., 2021). It should be noted that Fenton reaction highly relies on the overexpression of H2O2 and acidic condition of TME, which makes CDT strategy much safer to the normal tissues, since the Fenton reaction was suppressed under the neutral and insufficient H2O2 conditions in the healthy cell microenvironment.
Challenges and limitations of CDT
As a type of ROS-mediated cancer treatment modality, there is considerable development of understanding and in CDT during the last years. However, there are still challenges and limitations that hinder its further application in clinical. First, the acidic environment of solid tumor site is insufficient to trigger the efficient Fenton/Fenton-like reaction; second, the endogenous H2O2 level in tumor cells is not enough to generate adequate •OH to induce apoptosis of tumor cells; third, the high concentration of reduced glutathione and redox homeostasis of tumor cells could eliminate the free radical and weaken the CDT effect.
From the perspective of chemical kinetic, hydroxyl radicals could be catalyzed via Fenton/Fenton-like reaction over a wide range of pH conditions (between acidic and neutral pH) (Zhu et al., 2019). However, the efficiency and reactivity of Fenton reaction strongly depend on the pH condition, the reactivity is obviously increased in the acidic pH range (pH 2–4), and strongly reduced (even lost) in alkaline media. Considered the TME is slightly acidic pH condition (pH 6.5–6.8), at one side, the limited reactivity of Fenton/Fenton-like reaction in healthy tissues (pH 7.2–7.4) could avoid the damage on healthy tissues and cells, which makes CDT might selectively kill tumor cells; on the other side, the weakly acidic TME limits the efficiency of Fenton/Fenton-like reactions, which significantly reduce the generation of •OH.
To address the problem of insufficient acidity, attempts have been made to reconstitute the tumor microenvironment via in situ H+ generation strategy. For example, several studies have integrated glucose oxidase (GOx) into CDT agents, since GOx can oxidize glucose to gluconic acid to further increases acidity at TME, thus could enhance Fenton/Fenton-like reaction efficiency (Cheng et al., 2020; Zhang X. et al., 2021). It should be noted that the tumor cells employ various mechanisms to maintain cellular homeostasis during tumorigenesis and metastasis, this property makes the in situ acidization of intracellular strategy couldn’t support a long-term change. Therefore, the design and development of pH-independent CDT nanotherapeutics and/or combinational therapies with CDT are viable approaches to overcome pH limitations of CDT.
Besides the pH condition, Fenton/Fenton-like systems are also H2O2 dependent. Compared to normal cells (extremely low H2O2 level), the tumor cells produce and accumulate a large amount of H2O2 (100 μM–1 mM). However, the initial endogenous H2O2 level in tumor cells was still not enough to generate sufficient •OH to achieve satisfactory antitumor therapeutic effect. In addition, there are endogenous reducing substances that are involved in maintaining cellular redox homeostasis in the tumor cells. These reductive substances, for example reduced glutathione, could mitigate oxidative stress and repair the oxidative damage. Therefore, increasing H2O2 concentration and reducing GSH level in tumor cells are potential approaches to enhance the efficacy of CDT. To overcome the limited-H2O2-level obstacle, tremendous H2O2 self-supplying systems are designed to enhance the efficiency of Fenton/Fenton-like reactions to achieve satisfactory CDT effect. From the reported studies, there are two important design roles for constructing these H2O2 self-supplying systems: 1) delivery of exogenous H2O2 to tumors (Gao et al., 2019; Han et al., 2020; Zhao et al., 2022); 2) promote the ability of endogenous H2O2 production (Liu et al., 2020; Sang et al., 2020; Jiang et al., 2022).
Nanosystems for CDT based combination therapy strategies
With the rapid expansion of chemistry and materials science, nanotechnology shows great potential to overcome the limitations of conventional tumor therapy, and nanosystems (or nanotherapeutics) are regarded as one of the most promising strategies for better tumor treatment (Zhang P. et al., 2022). The recent reports have revealed that the combination therapy strategies with CDT nanosystems can optimize the therapy effects and minimize the toxicity and adverse effects of treatment (He et al., 2021; Yang et al., 2021; Liu Y. et al., 2022; Hao et al., 2022).
CDT-chemotherapy combination therapy
Although there is tremendous progress in cancer therapy during last years, chemotherapy is still main therapeutic modality in clinic treatment of tumor. However, the low targeting activity, multidrug resistance problems, and associated serious adverse-effects (for example myelosuppression) are still the major challenges and limitation in the development of effective chemotherapeutic agents (Holohan et al., 2013; Javarappa et al., 2018). Therefore, the combinational therapy strategy has become the potential cancer treatment. For example, the synergistic anti-tumor strategy of CDT combined with chemotherapy shows excellent prospects for tumor treatment since the chemotherapy can decrease GSH level in tumor cells. Furthermore, specifically activated by the stimuli (such as pH) in TME, CDT-chemotherapy combination therapy could solve the drug-resistance problem and significantly enhance the anti-tumor efficacy (Tan et al., 2020; Xiao et al., 2021; Yao et al., 2021).
Doxorubicin (DOX), a commonly used chemotherapy drug, has been demonstrated that has the ability to regulate the generation of intracellular H2O2 and GSH. Recent studies have revealed that the synergistic enhanced therapy by combining CDT with DOX. Mu reported a nanosystem prepared from epigallocatechin gallate (EGCG) and Fe3+ to simultaneously deliver DOX and iron ions to tumor sites. DOX/Fe3+/EGCG nanoparticles exhibit good stability, and response to the high level of glutathione and acidic conditions in TME to achieve efficient release of DOX and Fe3+ after internalization. After Fe3+ was reduced to Fe2+ by EGCG, Fenton reaction was induced to produce •OH, which further induced tumor death to amplify the therapeutic effect of DOX. In vitro and in vivo studies have also shown the promising tumor treatment results by this CDT-chemotherapy combined system (Mu et al., 2020). Huang’s group constructed a biodegradable multifunctional copper-doped calcium phosphate nanocomposite system to incorporate DOX. Due to the glucose oxidase, this nanocomposite catalyzed glucose to produce gluconic acid and hydrogen peroxide, and Cu2+ was reduced to Cu+ by endogenous GSH. This strategy could consume GSH and enhance H2O2 at the same time, thus to amplify CDT effect. Within tumor cells, Cu2+/Cu+ induced the Fenton-like reaction to increase •OH level, enhancing the chemotherapy results. In addition, the DOX in this system can not only be used for chemotherapy, but also for fluorescence imaging to monitor of tumor targeting and drug release in real-time due to its inherent fluorescence property (Fu L. H. et al., 2021).
CDT-immunotherapy combination therapy
Immunotherapy is an emerging field in tumor treatment. Accumulating evidence indicates that the engagement of CD8+ T lymphocytes and nature killer cells in TME is the critical process for successful immunotherapy (Wang et al., 2019). Recent studies have shown that reactive oxygen species play a vital role in inducing the immunogenic death of tumor cells and facilitating antitumor immune responses. Immunogenic cell death induced by ROS oxidative stress could increase the release of inflammatory cytokines, such as tumor necrosis factor α (TNFα), which could further activate the immune response in vivo to inhibit and eliminate both primary tumor cells and distant sites tumor (or secondary cancer cells) (Fu L. et al., 2021; Huang et al., 2021).
Li and Rong reported a novel nanosystem by loading ultra-small CaO2 and Fe3O4 nanoparticles into dendritic mesoporous silica which coated with a pH-responsive membrane. This nanocomposite realized the synergism of CDT and immunotherapy for effective cancer therapy. After intravenous administration of this nanocomposite, acidic condition of TME triggered CaO2 to generate abundant H2O2, which was catalyzed to produce •OH through Fenton reaction mediated by Fe3O4. By inducing tumor cells death and the release of tumor-associated antigens, the tumor immunogenic microenvironment was altered and immune responsibility was enhanced by increasing the rates of CD8+ Treg cells and M1/M2 macrophage. This study provides a feasible strategy to achieve highly effective cancer treatment through the synergistic effect of CDT-immunotherapy (Li and Rong, 2020).
CDT-photothermal combination therapy
Photothermal therapy (PTT) is a unique cancer therapeutic strategy, it employs photo-absorbing agents to convert the light energy into thermal energy, and the heat generated through photo-thermal reaction is utilized to directly destroy tumor tissues (Doughty et al., 2019; Zhao et al., 2021). However, PTT usually relies on a high-energy light source for long term treatment, and it is difficult to achieve satisfactory therapeutic effect with only PTT one single modal therapy.
By hybridizing Fenton agents, PTT systems can be designed to combine with chemodynamic therapy. In the combination system, the heat generated by photo-thermal reaction can not only effectively kill tumor cells, but also increase the rate of ROS production, which ultimately enhanced the effect of chemodynamic therapy (Zhang M. et al., 2020; Jiang et al., 2020; Shi et al., 2022). Li et al. designed a biomimetic nanosystem for self-enhanced photothermal/chemodynamic synergistic therapy. In this study, a nanoplatform was constructed by loading glucose oxidase (GOD) and Ag2S quantum dots into MnO2 nanosheets and then coated with the 4T1 cell membrane, inducing successfully escape immune clearance. This nanoplatform exhibited good tumor targeting ability and biocompatibility. In addition to the photothermal treatment effect, glucose oxidase (GOD) can oxidize excess glucose to H2O2, and at the same time, the released Mn2+ can catalyze H2O2 to generate abundant •OH through Fenton-like reaction. Therefore, photothermal enhanced chemodynamic therapy can be achieved under near-infrared light irradiation (Li et al., 2022).
CDT-sonodynamic combination therapy
Sonodynamic therapy (SDT) has been developed as a promising noninvasive approach for tumor treatment in recent decades. The antitumor mechanism of SDT is ROS-based process, briefly, a sonosensitizer is activated by ultrasound energy to generate ROS that destroy the tumor cells (Gong and Dai, 2021; Geng et al., 2022; Sun et al., 2022). However, SDT also has some problems, such as low ROS yield, limited delivery efficiency and short/long-term safety concerns of sonosensitizers. There is a two-dimensional nanosonosensitizer/nanocatalyst combined nanosystem reported by Tang’s group. In this nanosystem, integrated Cu2O could promote the in situ generation of H2O2 in the acidic tumor microenvironment, and the generated H2O2 further oxidized Ti3C2 to TiO2, which was the nanosonosensitizer and reacted with water and oxygen into the cells to generate ROS. In addition, ultrasound also enhances the Cu-induced Fenton-like reaction during the sonodynamic process to generate more ROS to achieve sonodynamic/chemodynamic improved synergistic tumor therapy. This study also confirmed that the antitumor mechanism of synergistic chemodynamic and sonodynamic therapies are associated with the upregulation of oxidative phosphorylation and ROS generation (Zhang M. et al., 2022).
In addition to the above-mentioned combination therapy strategies, CDT can be also applied with other therapy to achieve the synergistic effects and enhanced therapeutic effects. Lin et al. constructed a hyaluronic acid-modified bimetallic peroxide CaO2-CuO2@HA nano-system, which was effectively accumulated at the tumor site through the EPR effect, and the modified hyaluronic acid could recognize CD44 on the surface of tumor cells, which achieved active targeted property. Subsequently, the nanocomposites are able to generate a large number of Ca2+, Cu2+, and hydrogen peroxide in the acidic and hyaluronidase-overexpressing tumor microenvironment. Accompanied with glutathione depletion, Cu2+ and H2O2 induced Fenton-like reaction to generate more •OH. In addition, the excess Ca2+ released from the nanosystem leaded to the mitochondrial damage, which further enhanced oxidative stress in tumor cells. In addition, the imbalance of calcium transport channels caused by oxidative stress further promoted the calcification, inducing the necrosis of tumor cells (also named as ion interference therapy). Therefore, during ROS generation process, the Fenton-like reaction generated by Cu2+ is synergistic with the mitochondrial dysfunction induced by Ca2+ (Liu B. et al., 2022).
Conclusion and prospects
Although it has been proven that CDT is an ideal therapeutic approach for tumor therapy, the intrinsic barrier of TME is the main obstacle to hinder the further development and clinical translation of CDT. Fortunately, with the rapid expansion of nanoscience and nanobiotechnology, there are more nanosystems have been designed and studied that have potential to overcome those challenges of CDT. However, there are still some problems need to be solved before further clinical applications:
(1) Biosafety issues. At the cellular level, CDT-based nanosystems can enter cells in a variety of approaches, which may lead to changes or even loss of normal cellular functions, resulting in unnecessary toxic side effects. Especially, the current reported CDT-based nanosystems are mainly inorganic or hybrid nanomaterials, which are liable to elicit in vivo immune response. Thus, the biosafety issue of CDT-based nanosystems has raised intense concerns in the clinical applications.
(2) Complexity of CDT-based nanosystems. In the present studies, CDT-based nanosystems are designed overly complicated but hardly used in clinical practice. On one side, complicated nanosystems usually are associated to biological toxicity since the chemical compositions are too complex to precisely predict the biocompatibility. On the other side, the synthesis of CDT-based nanosystems is only reported in the lab level, but for practical applications, the reproducibility is too low for industrial scale. Therefore, how to design and construct CDT-based nanosystems with simple structure, stable compositions, and efficient responsibility to endogenous and/or exogenous stimuli has attracted much attention.
(3) The synergistic mechanism of CDT-based combined strategies. CDT and CDT-based combined strategies are ROS-mediated process, however, the mechanism for •OH production by Fenton/Fenton-like reactions in vivo need be deeply investigated, especially the CDT-based combined strategies. A successful combined therapy can overcome the shortcomings of monotherapy, however, synergistic therapy may provide new therapeutic mechanism and opportunities for tumor therapy. To further broaden the application of CDT and CDT-based combined therapy, ROS synergistic mechanism in vivo should be deeply investigated to provide more effective therapeutic options.
In the future, research efforts should shift to practical trials of using CDT and CDT-based combined therapy strategies in disease treatment. There is still a long way to go before achieving clinical application, we expect more nanosystems for CDT therapy are designed and more cancer patients will benefit from this treatment.
Author contributions
PZ proposed the project and edited the manuscript; ML drew the scheme; WZ, XX, GL, and MD carriered out reference searching and checking, all the authors carried out reference searching and checking. All authors read and approved the manuscript.
Funding
This work was supported by the National Natural Science Foundation of China (No. 82001961) and the Youth Start-up Fund Project of Yantai University (No. YX19B04).
Conflict of interest
The authors declare that the research was conducted in the absence of any commercial or financial relationships that could be construed as a potential conflict of interest.
Publisher’s note
All claims expressed in this article are solely those of the authors and do not necessarily represent those of their affiliated organizations, or those of the publisher, the editors and the reviewers. Any product that may be evaluated in this article, or claim that may be made by its manufacturer, is not guaranteed or endorsed by the publisher.
References
Cao, S., Fan, J., Sun, W., Li, F., Li, K., Tai, X., et al. (2019). A novel Mn-Cu bimetallic complex for enhanced chemodynamic therapy with simultaneous glutathione depletion. Chem. Commun. 55, 12956–12959. doi:10.1039/c9cc06040e
Cao, M., Li, H., Sun, D., and Chen, W. (2020a). Cancer burden of major cancers in China: A need for sustainable actions. Cancer Commun. 40, 205–210. doi:10.1002/cac2.12025
Cao, S., Li, X., Gao, Y., Li, F., Li, K., Cao, X., et al. (2020b). A simultaneously GSH-depleted bimetallic Cu(ii) complex for enhanced chemodynamic cancer therapy. Dalton Trans. 49, 11851–11858. doi:10.1039/d0dt01742f
Chen, X., Zhang, H., Zhang, M., Zhao, P., Song, R., Gong, T., et al. (2020). Amorphous Fe-based nanoagents for self-enhanced chemodynamic therapy by Re-establishing tumor acidosis. Adv. Funct. Mat. 30, 1908365. doi:10.1002/adfm.201908365
Cheng, K., Ling, C., Gu, D., Gao, Z., Li, Y., An, P., et al. (2020). Multimodal therapies: Glucose oxidase-triggered tumor starvation-induced synergism with enhanced chemodynamic therapy and chemotherapy. New J. Chem. 44, 1524–1536. doi:10.1039/c9nj05469c
Doughty, A. C. V., Hoover, A. R., Layton, E., Murray, C. K., Howard, E. W., and Chen, W. R. (2019). Nanomaterial applications in photothermal therapy for cancer. Mater. (Basel) 12, E779. doi:10.3390/ma12050779
Ferlay, J., Colombet, M., Soerjomataram, I., Parkin, D. M., Piñeros, M., Znaor, A., et al. (2021). Estimating the global cancer incidence and mortality in 2018: GLOBOCAN sources and methods. Int. J. Cancer 149, 1941–1953. doi:10.1002/ijc.31937
Fu, L. H., Wan, Y., Qi, C., He, J., Li, C., Yang, C., et al. (2021a). Nanocatalytic theranostics with glutathione depletion and enhanced reactive oxygen species generation for efficient cancer therapy. Adv. Mat. 33, e2006892. doi:10.1002/adma.202006892
Fu, L., Zhou, X., and He, C. (2021b). Polymeric nanosystems for immunogenic cell death-based cancer immunotherapy. Macromol. Biosci. 21, e2100075. doi:10.1002/mabi.202100075
Gao, S., Jin, Y., Ge, K., Li, Z., Liu, H., Dai, X., et al. (2019). Self-Supply of O2 and H2O2 by a nanocatalytic medicine to enhance combined chemo/chemodynamic therapy. Adv. Sci. 6, 1902137. doi:10.1002/advs.201902137
Geng, B., Xu, S., Li, P., Li, X., Fang, F., Pan, D., et al. (2022). Platinum crosslinked carbon dot@TiO2−x p-n junctions for relapse-free sonodynamic tumor eradication via high-yield ROS and GSH depletion. Small 18, e2103528. doi:10.1002/smll.202103528
Gong, Z., and Dai, Z. (2021). Design and challenges of sonodynamic therapy system for cancer theranostics: From equipment to sensitizers. Adv. Sci. 8, 2002178. doi:10.1002/advs.202002178
Han, Y., Ouyang, J., Li, Y., Wang, F., and Jiang, J. H. (2020). Engineering H2O2 self-supplying nanotheranostic platform for targeted and imaging-guided chemodynamic therapy. ACS Appl. Mat. Interfaces 12, 288–297. doi:10.1021/acsami.9b18676
Hao, Y., Gao, Y., Fan, Y., Zhang, C., Zhan, M., Cao, X., et al. (2022). A tumor microenvironment-responsive poly(amidoamine) dendrimer nanoplatform for hypoxia-responsive chemo/chemodynamic therapy. J. Nanobiotechnology 20, 43. doi:10.1186/s12951-022-01247-6
He, C., Zhang, X., Chen, C., Liu, X., Chen, Y., Yan, R., et al. (2021). A solid lipid coated calcium peroxide nanocarrier enables combined cancer chemo/chemodynamic therapy with O2/H2O2 self-sufficiency. Acta Biomater. 122, 354–364. doi:10.1016/j.actbio.2020.12.036
Holohan, C., Van Schaeybroeck, S., Longley, D. B., and Johnston, P. G. (2013). Cancer drug resistance: An evolving paradigm. Nat. Rev. Cancer 13, 714–726. doi:10.1038/nrc3599
Huang, J., Yang, B., Peng, Y., Huang, J., Wong, S. H. D., Bian, L., et al. (2021). Nanomedicine-boosting tumor immunogenicity for enhanced immunotherapy. Adv. Funct. Mat. 31, 2011171. doi:10.1002/adfm.202011171
Javarappa, K. K., Tsallos, D., and Heckman, C. A. (2018). A multiplexed screening assay to evaluate chemotherapy-induced myelosuppression using healthy peripheral blood and bone marrow. SLAS Discov. 23, 687–696. doi:10.1177/2472555218777968
Jiang, C., He, T., Tang, Q., He, J., Ren, Q., Zhang, D. Y., et al. (2022). Nanozyme catalyzed cascade reaction for enhanced chemodynamic therapy of low-H2O2 tumor. Appl. Mat. Today 26, 101357. doi:10.1016/j.apmt.2021.101357
Jiang, F., Ding, B., Zhao, Y., Liang, S., Cheng, Z., Xing, B., et al. (2020). Biocompatible CuO-decorated carbon nanoplatforms for multiplexed imaging and enhanced antitumor efficacy via combined photothermal therapy/chemodynamic therapy/chemotherapy. Sci. China Mat. 63, 1818–1830. doi:10.1007/s40843-019-1397-0
Khan, M. I., Mohammad, A., Patil, G., Naqvi, S. A. H., Chauhan, L. K. S., and Ahmad, I. (2012). Induction of ROS, mitochondrial damage and autophagy in lung epithelial cancer cells by iron oxide nanoparticles. Biomaterials 33, 1477–1488. doi:10.1016/j.biomaterials.2011.10.080
Li, H., Liu, Y., Huang, B., Zhang, C., Wang, Z., She, W., et al. (2022). Highly efficient GSH-responsive “off–on” NIR-II fluorescent fenton nanocatalyst for multimodal imaging-guided photothermal/chemodynamic synergistic cancer therapy. Anal. Chem. 94, 10470–10478. doi:10.1021/acs.analchem.2c01738
Li, L., Zhang, P., Li, C., Guo, Y., and Sun, K. (2021). In vitro/vivo antitumor study of modified-chitosan/carboxymethyl chitosan “boosted” charge-reversal nanoformulation. Carbohydr. Polym. 269, 118268. doi:10.1016/j.carbpol.2021.118268
Li, Z., and Rong, L. (2020). Cascade reaction-mediated efficient ferroptosis synergizes with immunomodulation for high-performance cancer therapy. Biomater. Sci. 8, 6272–6285. doi:10.1039/d0bm01168a
Liang, L., Duan, Y., Xiong, Y., Zuo, W., Ye, F., and Zhao, S. (2022). Synergistic cocatalytic effect of MoO3 and creatinine on Cu–Fenton reactions for efficient decomposition of H2O2. Mat. Today Chem. 24, 100805. doi:10.1016/j.mtchem.2022.100805
Liang, S., Xiao, X., Bai, L., Liu, B., Yuan, M., Ma, P., et al. (2021). Conferring Ti-based MOFs with defects for enhanced sonodynamic cancer therapy. Adv. Mat. 33, e2100333. doi:10.1002/adma.202100333
Lin, L., Chen, H., Zhao, R., Zhu, M., and Nie, G. (2022). Nanomedicine targets iron metabolism for cancer therapy. Cancer Sci. 113, 828–837. doi:10.1111/cas.15250
Lin, L., Yu, J., Lu, H., Wei, Z., Chao, Z., Wang, Z., et al. (2021). Mn-DNA coordination of nanoparticles for efficient chemodynamic therapy. Chem. Commun. 57, 1734–1737. doi:10.1039/d0cc08191d
Liu, B., Bian, Y., Liang, S., Yuan, M., Dong, S., He, F., et al. (2022a). One-step integration of tumor microenvironment-responsive calcium and copper peroxides nanocomposite for enhanced chemodynamic/ion-interference therapy. ACS Nano 16, 617–630. doi:10.1021/acsnano.1c07893
Liu, C., Cao, Y., Cheng, Y., Wang, D., Xu, T., Su, L., et al. (2020). An open source and reduce expenditure ROS generation strategy for chemodynamic/photodynamic synergistic therapy. Nat. Commun. 11, 1735. doi:10.1038/s41467-020-15591-4
Liu, Y., Chi, S., Cao, Y., and Liu, Z. (2022b). Glutathione-responsive biodegradable core-shell nanoparticles that self-generate H2O2and deliver doxorubicin for chemo-chemodynamic therapy. ACS Appl. Nano Mat. 5, 2592–2602. doi:10.1021/acsanm.1c04277
Mu, M., Wang, Y., Zhao, S., Li, X., Fan, R., Mei, L., et al. (2020). Engineering a pH/glutathione-responsive tea polyphenol nanodevice as an apoptosis/ferroptosis-inducing agent. ACS Appl. Bio Mat. 3, 4128–4138. doi:10.1021/acsabm.0c00225
Rasool, M., Malik, A., Waquar, S., Arooj, M., Zahid, S., Asif, M., et al. (2022). New challenges in the use of nanomedicine in cancer therapy. Bioengineered 13, 759–773. doi:10.1080/21655979.2021.2012907
Roma-Rodrigues, C., Mendes, R., Baptista, P. V., and Fernandes, A. R. (2019). Targeting tumor microenvironment for cancer therapy. Int. J. Mol. Sci. 20, E840. doi:10.3390/ijms20040840
Sang, Y., Cao, F., Li, W., Zhang, L., You, Y., Deng, Q., et al. (2020). Bioinspired construction of a nanozyme-based H2O2 homeostasis disruptor for intensive chemodynamic therapy. J. Am. Chem. Soc. 142, 5177–5183. doi:10.1021/jacs.9b12873
Shi, Z., Tang, J., Lin, C., Chen, T., Zhang, F., Huang, Y., et al. (2022). Construction of iron-mineralized black phosphorene nanosheet to combinate chemodynamic therapy and photothermal therapy. Drug Deliv. 29, 624–636. doi:10.1080/10717544.2022.2039810
Srinivas, U. S., Tan, B. W. Q., Vellayappan, B. A., and Jeyasekharan, A. D. (2019). ROS and the DNA damage response in cancer. Redox Biol. 25, 101084. doi:10.1016/j.redox.2018.101084
Sun, L., Zhou, J. e., Luo, T., Wang, J., Kang, L., Wang, Y., et al. (2022). Nanoengineered neutrophils as a cellular sonosensitizer for visual sonodynamic therapy of malignant tumors. Adv. Mat. 34, e2109969. doi:10.1002/adma.202109969
Sung, H., Ferlay, J., Siegel, R. L., Laversanne, M., Soerjomataram, I., Jemal, A., et al. (2021). Global cancer statistics 2020: GLOBOCAN estimates of incidence and mortality worldwide for 36 cancers in 185 countries. Ca. Cancer J. Clin. 71, 209–249. doi:10.3322/caac.21660
Tan, J., Duan, X., Zhang, F., Ban, X., Mao, J., Cao, M., et al. (2020). Theranostic nanomedicine for synergistic chemodynamic therapy and chemotherapy of orthotopic glioma. Adv. Sci. 7, 2003036. doi:10.1002/advs.202003036
Wang, W., Green, M., Choi, J. E., Gijón, M., Kennedy, P. D., Johnson, J. K., et al. (2019). CD8+ T cells regulate tumour ferroptosis during cancer immunotherapy. Nature 569, 270–274. doi:10.1038/s41586-019-1170-y
Wicki, A., Witzigmann, D., Balasubramanian, V., and Huwyler, J. (2015). Nanomedicine in cancer therapy: Challenges, opportunities, and clinical applications. J. Control. Release 200, 138–157. doi:10.1016/j.jconrel.2014.12.030
Wu, T., and Dai, Y. (2017). Tumor microenvironment and therapeutic response. Cancer Lett. 387, 61–68. doi:10.1016/j.canlet.2016.01.043
Xiao, T., He, M., Xu, F., Fan, Y., Jia, B., Shen, M., et al. (2021). Macrophage membrane-camouflaged responsive polymer nanogels enable magnetic resonance imaging-guided chemotherapy/chemodynamic therapy of orthotopic glioma. ACS Nano 15, 20377–20390. doi:10.1021/acsnano.1c08689
Yang, K., Yu, G., Yang, Z., Yue, L., Zhang, X., Sun, C., et al. (2021). Supramolecular polymerization-induced nanoassemblies for self-augmented cascade chemotherapy and chemodynamic therapy of tumor. Angew. Chem. Int. Ed. Engl. 60, 17570–17578. doi:10.1002/anie.202103721
Yao, L., Tang, Y., Cao, W., Cui, Y., and Qian, G. (2021). Highly efficient encapsulation of doxorubicin hydrochloride in metal-organic frameworks for synergistic chemotherapy and chemodynamic therapy. ACS Biomater. Sci. Eng. 7, 4999–5006. doi:10.1021/acsbiomaterials.1c00874
Zhang, C., Bu, W., Ni, D., Zhang, S., Li, Q., Yao, Z., et al. (2016). Synthesis of iron nanometallic glasses and their application in cancer therapy by a localized fenton reaction. Angew. Chem. Int. Ed. Engl. 55, 2101–2106. doi:10.1002/anie.201510031
Zhang, H., Li, J., Chen, Y., Wu, J., Wang, K., Chen, L., et al. (2021a). Magneto-electrically enhanced intracellular catalysis of FePt-FeC heterostructures for chemodynamic therapy. Adv. Mat. 33, e2100472. doi:10.1002/adma.202100472
Zhang, M., Liu, X., Luo, Q., Wang, Q., Zhao, L., Deng, G., et al. (2020a). Tumor environment responsive degradable CuS@mSiO2@MnO2/DOX for MRI guided synergistic chemo-photothermal therapy and chemodynamic therapy. Chem. Eng. J. 389, 124450. doi:10.1016/j.cej.2020.124450
Zhang, M., Yang, D., Dong, C., Huang, H., Feng, G., Chen, Q., et al. (2022a). Two-dimensional MXene-originated in situ nanosonosensitizer generation for augmented and synergistic sonodynamic tumor nanotherapy. ACS Nano 16, 9938–9952. doi:10.1021/acsnano.2c04630
Zhang, P., Chen, D., Li, L., and Sun, K. (2022b). Charge reversal nano-systems for tumor therapy. J. Nanobiotechnology 20, 31. doi:10.1186/s12951-021-01221-8
Zhang, X., Bu, W., Wang, J., Guo, G., Zhang, H., Shen, H., et al. (2020b). Space-selective chemodynamic therapy of CuFe5O8nanocubes for implant-related infections. ACS Nano 14, 13391–13405. doi:10.1021/acsnano.0c05255
Zhang, X., He, C., Chen, Y., Chen, C., Yan, R., Fan, T., et al. (2021b). Cyclic reactions-mediated self-supply of H2O2 and O2 for cooperative chemodynamic/starvation cancer therapy. Biomaterials 275, 120987. doi:10.1016/j.biomaterials.2021.120987
Zhang, Y., Huo, L., Wei, Z., Tang, Q., and Sui, H. (2022c). Hotspots and Frontiers in inflammatory tumor microenvironment research: A scientometric and visualization analysis. Front. Pharmacol. 13, 862585. doi:10.3389/fphar.2022.862585
Zhao, L., Li, Z., Wei, J., Xiao, Y., She, Y., Su, Q., et al. (2022). Juglone-loaded metal-organic frameworks for H2O2 self-modulating enhancing chemodynamic therapy against prostate cancer. Chem. Eng. J. 430, 133057. doi:10.1016/j.cej.2021.133057
Zhao, L., Zhang, X., Wang, X., Guan, X., Zhang, W., and Ma, J. (2021). Recent advances in selective photothermal therapy of tumor. J. Nanobiotechnology 19, 335. doi:10.1186/s12951-021-01080-3
Keywords: chemodynamic therapy (CDT), nanosystems, combination therapy, Fenton reaction, reactive oxygen species
Citation: Li M, Zhang W, Xu X, Liu G, Dong M, Sun K and Zhang P (2022) Nanosystems for chemodynamic based combination therapy: Strategies and recent advances. Front. Pharmacol. 13:1065438. doi: 10.3389/fphar.2022.1065438
Received: 09 October 2022; Accepted: 14 October 2022;
Published: 28 October 2022.
Edited by:
Defang Li, Binzhou Medical University, ChinaReviewed by:
Xiangpeng Dai, Jilin University, ChinaCopyright © 2022 Li, Zhang, Xu, Liu, Dong, Sun and Zhang. This is an open-access article distributed under the terms of the Creative Commons Attribution License (CC BY). The use, distribution or reproduction in other forums is permitted, provided the original author(s) and the copyright owner(s) are credited and that the original publication in this journal is cited, in accordance with accepted academic practice. No use, distribution or reproduction is permitted which does not comply with these terms.
*Correspondence: Kaoxiang Sun, sunkaoxiang@luye.com; Peng Zhang, peng.zhang@ytu.edu.cn