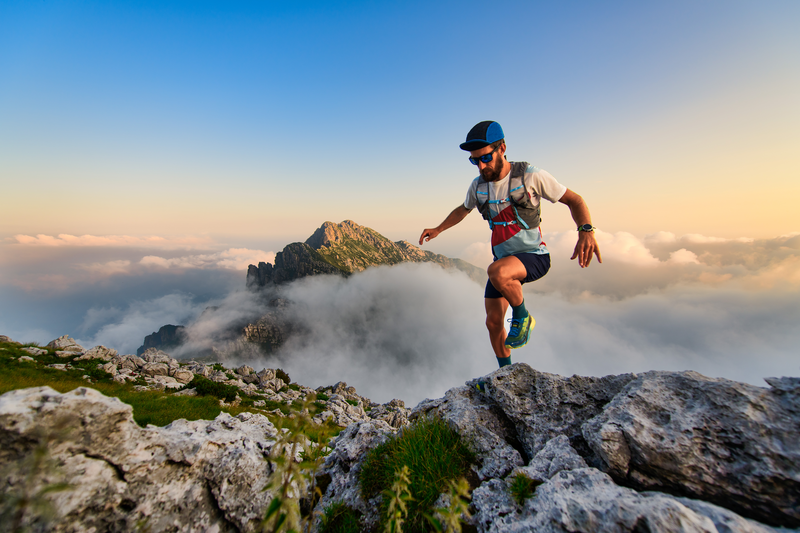
94% of researchers rate our articles as excellent or good
Learn more about the work of our research integrity team to safeguard the quality of each article we publish.
Find out more
ORIGINAL RESEARCH article
Front. Pharmacol. , 23 February 2022
Sec. Pharmacology of Anti-Cancer Drugs
Volume 12 - 2021 | https://doi.org/10.3389/fphar.2021.821518
This article is part of the Research Topic Organelle Targeting: Focus on Drug Discovery and Theranostics View all 15 articles
A novel formal (4 + 1) annulation between N-(o-chloromethyl)aryl amides and 3-chlorooxindoles through in situ generated aza-ortho-QMs with 3-chlorooxindoles is reported for the synthesis of a series of 2,3′-spirobi (indolin)-2′-ones in high yields. Under structured illumination microscopy, compound 3a is found to change the mitochondrial morphology and induce mitophagy pathway, which might then trigger mitophagy in cancer cells.
The high prevalence and fatal incidence of cancer in the population worldwide has fueled an intensified search for new therapeutic treatment options. Chemotherapy is one of the most common strategies. The major challenging factors in developing cancer chemotherapeutics is to increase selectivity and to reduce side effects toward normal cells and tissues. (Wheeler et al., 2013) Since the efficacy and toxicity of a drug is closely associated with its subcellular distribution, interest in subcellular organelle-targeting theranostics is substantially increasing. (Kang, 2018).
Among organelles, mitochondria which is a regulatory center for cellular energy metabolism, substance synthesis and death, function as dynamic networks that often come in varied morphologies and subcellular distribution to fulfill their multiple tasks and thus have received substantial attention. (Li et al., 2020; Chen H. et al., 2021; Zou et al., 2021) Amount of researches disclosed that many human diseases have been closely related with functional mitochondria, such as neurodegenerative disorders, cardiovascular disorders, metabolic disorders, and cancers. (Cho et al., 2020) Recent studies demonstrated dramatic alterations in mitochondrial form during the early stages of cell apoptosis that is a fragmentation of the network and the remodeling of the cristae, indicating mitochondria are closely associated with apoptotic pathways. (Karbowski and Youle, 2003) Moreover, accumulating evidence indicates that the occurrence, development and metastasis of tumors has been linked to mitochondrial dysfunction and malfunctions, whose morphology is sensitive to their effects, featuring mitochondria a striking target in the design of anti-cancer drugs. (Mo et al., 2012; Hao et al., 2019) So far, some interesting and innovative examples have been reported, such as the increased anti-tumor effect of photodynamic therapy through the regulation of mitochondrial form by paclitaxel. (Zhao et al., 2017) However, these therapies are not yet in the preclinical phase. Therefore, the search for new natural or synthetic compounds that can target mitochondria as anticancer treatment is imperative.
The 3,3′-pyrrolidinyl-spirooxindole skeleton is a privileged class of heterocyclic motifs, which form the core of a large family of bioactive oxindole alkaloids and medicinally important compounds. (Kumar et al., 2008; Girgis, 2009; Zhao et al., 2013a; Zhao et al., 2013b; Arumugam et al., 2021; Liu et al., 2021) For instance, coerulescine, the simplest prototype member, was isolated from Horsfieldia superba, extracts of which have found use in indigenous medicine. (Neil et al., 1997) Spirooxindole derivative DS-3032b exhibits MDM2 inhibitory activity employed in the treatment of patients with advanced solid tumors and lymphomas (Figure 1). (Gounder et al., 2016) Their notable biological activities prompted the development of numerous strategies toward the syntheses of 3,3′-pyrrolidinyl-spirooxindole moiety. (Cao and Zhou, 2015; He et al., 2020; Liu X. et al., 2020; Nakamura et al., 2020; Reddy et al., 2020; Bortolami et al., 2021; Nasri et al., 2021; Saranya et al., 2021) Nevertheless, the construction of the structurally similar spirobi (indolin) frameworks (Skeleton B, Figure 1 bottom left) has been less studied, and until now, only two synthetic methods have been reported for the synthesis of 2,3′-spirobi (indolin)-2′-ones. (Gui et al., 2019; Wang et al., 2019) In 2019, Shi and co-workers pioneered the (4 + 1) annulation of 3-isothiocyanato oxindoles and aza-o-quinone methides, affording the corresponding condensed products in two steps. Meanwhile, Zhong’s group reported an ioide salts catalyzed functionalization of carbonyl compounds with sulfonamides.
Although these were elegant and creative strategies, it is still highly desirable to develop a concise protocol to construct the 2,3′-spirobi (indolin)-2′-ones framework from readily available starting materials, especially under mild conditions. Based on our research expertise in the field of domino-cycloaddition, (Enders et al., 2015; Zhao et al., 2016a; Zhao et al., 2016b; Zhi et al., 2016; Zhi et al., 2018) we envisioned that the assembly of 2,3′-spirobi (indolin)-2′-ones 3 could be realized through a formal (4 + 1) reaction between in situ generated aza-ortho-QM 2′ from N-(o-chloromethyl) aryl amide 2 and 3-chloroindolin-2-one 1 in the presence of an appropriate base (Scheme 1). We hope this annulation reaction could provide a general and straightforward method to access 2,3′-spirobi (indolin)-2′-ones 3 that will serve as the basis for evaluation of bioavailabilty especially their effect on mitochondria which is understudied.
SCHEME 2. Gram-Scale synthesis (A) of N-tosylated spirobi (indolin) 3a and X-ray Structure (B) of 3n.
To test the feasibility of our hypothesis, we chose 3-chloroindolin-2-one 1a and N-[2-(chloromethyl) phenyl]-4-methylbenzenesulfonamide 2a as the model substrates to optimize the reaction conditions (Table 1). First, an initial experiment was conducted in ethyl ester at room temperature in the presence of Cs2CO3. To our delight, the expected product 3a was obtained in a yield of 15% (Table 1, entry 1). To improve the reaction yield, the commonly used organic base Et3N was tested while there was no compound 3a obtained. We found that the use of the suitable base is very crucial for the success of this reaction and thus an extensive screening of base was performed (Table 1, entries 3–6). Fortunately, inorganic bases K2CO3 and NH4HCO3 delivered the desired product 3a in 80 and 82% yield respectively. Striving for higher efficiency, kinds of solvents and different temperature were screened and the best result was obtained by raising the reaction temperature to 40°C and using MTBE as the solvent, leading to the desired product 3a in a yield of 88% (Table 1, entry 17).
TABLE 1. Reaction condition optimization studies.a
Having identified the optimal reaction conditions, the substrate scope of the new protocol was explored and the results are shown in Table 2. Initially, we examined the generality of 3-chloro isatin component. A variety of isatins 1 underwent the formal (4 + 1) aunulation reaction to furnish 3b-3g in 70–90% yield. Notably, substrates bearing electron-donating (R = Me, OCF3) or electron-withdrawing groups (R = Cl, Br) at the C5 position of the phenyl ring of 1 underwent this annulation process to furnish the corresponding products in good to excellent efficiencies (3b-3e). Moreover, the C7 position substituted compounds were suitable substrates, and the target products 3f-3g were synthesized with good results under the optimal condition. However, if fluoro group was introduced at the C7 position of isatin 1, the yield of the reaction under the optimal condition was very low. Next, the substrate scope of this reaction was examined further by varying the reaction partner 2. We found that all the substrates 2h-2n reacted efficiently with 1a, furnishing the desired products (Table 2, 3h–3n) in 70–92% yield. The substitution groups on the tosyl benzene ring were well tolerated and delivered the desired compounds with high efficiency (Table 2, 3h–3j). Especially, substrates bearing electron-with-drawing groups (R1 = Cl, Br) on the phenyl ring of 2 readily ccould be easily processed to give the products in good to excellent yields (3j–3n).
In order to test the robustness and general utility of this 1,4-addition reaction, a gram-scale reaction was carried out under the optimal conditions and the expected product 3a could be isolated in 80% yield without erosion of the efficiency of this process (Scheme 1A). In addition, as shown in Scheme 1B, the relative configuration of compound 3n was determined unambiguously by X-ray crystallography.
After the series of 2,3′-spirobi (indolin)-2′-ones were synthesized, 3a was selected to evaluate its damage effect on mitochondria. To verify the cytotoxicity of 3a, we chose a colorimetric measurement tool commonly used in laboratories, CCK-8, (Lou et al., 2010) which relies on WST-8 that can be reduced by mitochondrial dehydrogenase (such as succinate dehydrogenase, SDH) to produce a highly water-soluble orange-yellow formazan product for counting the number of live cells (Figure 2A). (Liu L. Y. et al., 2020) We found that the cells did not respond to the detection threshold for the CCK-8 assay after treatment with 3a at the concentration ranging from 10 to 50 μM. As it is generally accepted that the activity of SDH was applied as an indicator to evaluate the tricarboxylic acid cycle for reflecting cell activity rather than mitochondria behavior, (Farshbaf and Kiani-Esfahani, 2018) colorimetric tools based on a large number of cells are inaccurately for clarifying the regulation of drugs on a single mitochondria.
FIGURE 2. LHJ-090 (3a) damage mitochondria and stimulate the process of mitophagy. (A) The cell viability (%) obtained with cck8 assay. Percentage of viable HeLa cells after treated with different concentrations of 3a (0 10, 20, 30 and 50 μM) for 24 h (B,C) SIM imaging of mitochondria in HeLa cells were treated with 3a (10 μM) for 0, 12, and 24 h and then stained with the mitochondrial tracker probe (mito-tracker-green, MTG) (λex = 488 nm) for 0.5 h. (D) Quantitative analyze of mitochondrial morphology in HeLa cells after treated with 3a for 0, 12, and 24 h. Data was appeared as Mean ± SEM (n = 5). *p < 0.05, all compared with untreated cells. (E) The cell viability (%) obtained with CCK-8 assay at high concentration 3a stimulation, more than 150 μM shows toxicity to cells. (F,G) SIM colocalization images of MTG-stained mitochondria and LTR-stained lysosome with (G) or without (F) 3a treatment, the white solid square indicates fluorescence intensity. (H) The PCC values for MTG and LTR in HeLa cells from (F) and (G). (I) A schematic diagram of the role of 3a in mitochondrial damage.
To more accurately reflect the damage of 3a to the mitochondria, we applied recently developed structured illumination microscopy (SIM), a new tool for investing the effect of drugs at the single mitochondria level in living cells. (Wei et al., 2022) SIM based on a known spatially structured pattern of light to excite a sample whose fringe position and direction can be changed multiple times and to record the emission fluorescence signal at each position, thereby providing up to 100 nm spatial resolution. (Chen et al., 2018) Therefore, this tool can help us accurately and quantitatively study the behavior of 3a at the nanoscale in living cells system.
Next, we checked the 3a at the concentration of 10 μM in HeLa cells, and then observed it under SIM. We used a commercial mitochondrial probe (Mito-Tracker Green, MTG) to label mitochondria in HeLa cells after 3a stimulation. (Chen et al., 2020a; Zhang et al., 2021) Compared to the SIM images captured at 0 h (Figure 2B), we observed that mitochondrial morphology has changed from fibrous-like to round-like after the 3a treatment for 12 and 24 h (Figure 2C), showing the mitochondria were destroyed. To evaluate the mitochondrial morphology, the length-to-width ratio (L/W), was introduced as previously reported. (Shao et al., 2020) This system propose four standards to measure the morphology of mitochondria, namely round or nearly round (1.0 ≤ L/W < 1.5), intermediate (1.5 ≤ L/W < 2.0), tubular (2.0 ≤ L/W < 5.0), and hyperfused (L/W ≥ 5.0). We then quantify the distribution of individual mitochondria in HeLa cells, and found that the distribution of mitochondrial morphology was changed with the 3a treatment for 12 and 24 h (Figure 2D), indicating that 3a at 10 μM could damage mitochondrial morphology’s distribution.
Finally, we increased the concentration of 3a to check the detection threshold of CCK-8, and found that it could not be responded until 150 μM (Figure 2E), which shows that SIM is more accurate in exploring the sensitivity of drugs to subcellular behavior.
Mitophagy is a process by which cells remove and degrade damaged mitochondria, and its typical feature is the overlap of lysosomes and mitochondria. (Chen et al., 2020b; Chen Q. et al., 2021) After clarifying that 3a can damage mitochondria, we further studied whether drug-induced mitochondrial damage is involved in the mitophagy pathway.
We then use MTG and commercial lysosomal probe (Lyso-Tracker Red, LTR) to simultaneously label drug-treated HeLa cells. (Wang et al., 2020; Zhang C. et al., 2021) Results revealed that the mitochondria was damaged to be granular after 24 h of the drug treatment as the green mitochondria stained by MTG and the red lysosome stained by LTR overlapped into yellow (Figure 2F). Compared with that in untreated cells, the overlap of mitochondria and lysosome in cells treated with 3a for 24 h was increased significantly (Figure 2G). Together, these results suggested that 3a induced the change of mitochondrial morphology, and then triggered the mitophagy pathway.
Taken together, we reported a novel (4 + 1) annulation reaction between 3-chlorooxindoles and N-(o-chloromethyl) aryl amides through in situ generated aza-ortho-QM with 3-chlorooxindoles for the efficient synthesis of various 2,3′-spirobi (indolin)-2′-ones in good to excellent yield under mild conditions. By using the highly accurate tool structured illumination microscopy, we found that compound 3a could damage the distribution of mitochondrial form and induce mitophagy pathway, which finally might promote the mitophagy in cancer cells. Further efforts are in progress to evaluate the antiproliferative activity of these spiropyrrolidine analogs against tumor cell lines.
The chemical reagents are commercially available and were used without further purification. Reactions were monitored by Thin Layer Chromatography (TLC) (Silica gel HF254 or GF254 from Qingdao Haiyang Chemical Co., Ltd., Qingdao, China), and the spots were visualized with ultraviolet irradiation (254 nm). Compounds were purified by solvent beating or silica gel column chromatography (200–300 mesh). 1H NMR and 13C NMR spectra were recorded on a Bruker AVANCE AV III 600 spectrometer using CDCl3 or d-DMSO as solvent. Data for 1H NMR are reported as follows: chemical shift (ppm), multiplicity (s = singlet, d = doublet, t = triplet, q = quartet, dd = doublet of doublet, td = triplet of doublet, m = multiplet, br = broad), integration, and coupling constant (Hz). Data for 13C NMR are reported in terms of chemical shift and multiplicity where appropriate. High resolution mass spectra (HRMS) were obtained from Thermo Scientific Q Exactive Plus. The melting points were determined by Büchi 510 apparatus without corrected.
To an oven-dried flask were added 1 (0.4 mmol, 66.8 mg, 1.0 equiv), 2 (0.44 mmol, 130 mg, 1.1 equiv) and NH4HCO3 (1.2 mmol, 94.9 mg, 3.0 equiv) followed by the addition of MTBE (4.0 ml). The reaction mixture was allowed to stir at 40°C for 17 h and then directly poured into water. The solution was extracted with dichloromethane (3 × 15 ml). The organic phases were combined, washed with brine and dried over Na2SO4. Then the solvent was evaporated to give a crude product which was purified by silica gel chromatography (hexane/ethyl acetate = 10/1 to 4/1) to provide the desired products 3a-3n. The scale-up synthesis of 3a was the same as the above steps.
(S)-1-Tosyl-2,3′-spirobi (indolin)-2′-one (3a)
According to general procedure, the crude product was purified by silica gel chromatography (hexane/ethyl acetate = 10/1 to 4/1) to provide 3a as a white solid (132.6 mg, 88% yield). mp: 267–269°C.1H NMR (400 MHz, DMSO-d6) δ 10.76 (s, 1H), 7.70 (d, J = 8.4 Hz, 2H), 7.33 (d, J = 8.4 Hz, 2H), 7.28–7.17 (m, 4H), 7.04–7.00 (m, 1H), 6.94 (d, J = 8.4 Hz, 1H), 6.83 (d, J = 7.6 Hz, 1H), 6.76 (d, J = 7.6 Hz, 1H), 3.54 (d, J = 16.0 Hz, 1H), 3.23 (d, J = 16.4 Hz, 1H), 2.36 (s, 3H) ppm; 13C NMR (150 MHz, CDCl3) δ 177.5, 144.1, 141.6, 139.5, 136.4, 130.9, 129.8, 129.5 (2C), 128.1, 128.0 (2C), 127.2, 125.2, 123.0, 123.0, 122.9, 112.5, 110.6, 71.7, 42.2, 21.6 ppm. HRMS (ESI): m/z (M + H)+ calcd for C22H19N2O3S+ 391.1116; found 391.1112.
(S)-5′-Chloro-1-Tosyl-2,3′-Spirobi (indolin)-2′-One (3b)
According to general procedure, the crude product was purified by silica gel chromatography (hexane/ethyl acetate = 10/1 to 4/1) to provide 3b as a white solid (140 mg, 82% yield). mp: 253–255°C. 1H NMR (400 MHz, DMSO-d6) δ 10.91 (s, 1H), 7.59 (d, J = 8.0 Hz, 2H), 7.36–7.23 (m, 6H), 7.05 (t, J = 7.2 Hz, 1H), 6.95 (d, J = 8.4 Hz, 1H), 6.50 (s, 1H), 3.51 (d, J = 16.4 Hz, 1H), 3.30 (d, J = 16.4 Hz, 1H), 2.36 (s, 3H) ppm; 13C NMR (100 MHz, DMSO-d6) δ 176.8, 145.0, 141.5, 140.6, 136.3, 131.7, 130.1 (2C), 130.0, 128.6, 127.8, 127.6, 126.3, 126.0, 123.6 (2C), 123.3, 112.5, 112.4, 71.4, 41.7, 21.5 ppm; HRMS (ESI): m/z (M + H)+ calcd for C22H18ClN2O3S+ 425.0727; found 425.0718.
(S)-1-Tosyl-5'-(trifluoromethoxy)-2,3′-spirobi (indolin)-2′-one (3c)
According to general procedure, the crude product was purified by silica gel chromatography (hexane/ethyl acetate = 10/1 to 4/1) to provide 3c as a white solid (142 mg, 75% yield). mp: 98–102°C. 1H NMR (600 MHz, DMSO-d6) δ 10.96 (s, 1H), 7.69 (d, J = 8.4 Hz, 2H), 7.34 (d, J = 8.4 Hz, 2H), 7.30 (d, J = 8.4 Hz, 1H), 7.27 (d, J = 7.8 Hz, 1H), 7.24–7.20 (m, 2H), 7.05–7.02 (m, 2H), 6.74 (s, 1H), 3.54 (d, J = 16.2 Hz, 1H), 3.33 (d, J = 15.6 Hz, 1H), 2.36 (s, 3H) ppm; 13C NMR (150 MHz, DMSO-d6) δ 177.1, 145.0, 143.6, 143.5 141.5, 140.9, 136.4, 132.1, 130.2 (2C),128.5, 127.7 (2C), 125.9, 123.6, 116.8, 112.4, 111.8, 71.7, 41.8, 21.4 ppm; HRMS (ESI): m/z (M + H)+ calcd for C23H18F3N2O4S+ 475.0939; found 475.0935.
(S)-5′-Methyl-1-Tosyl-2,3′-Spirobi (indolin)-2′-One (3d)
According to general procedure, the crude product was purified by silica gel chromatography (hexane/ethyl acetate = 10/1 to 4/1) to provide 3d as a white solid (132 mg, 82% yield). mp: 282–284°C. 1H NMR (400 MHz, DMSO-d6) δ 10.64 (s, 1H), 7.55 (d, J = 8.0 Hz, 2H), 7.34–7.25 (m, 5H), 7.07–7.02 (m, 2H), 6.83 (d, J = 8.0 Hz, 1H), 6.34 (s, 1H), 3.51 (d, J = 16.4 Hz, 1H), 3.20 (d, J = 18.8 Hz, 1H), 2.36 (s, 3H), 2.01 (s, 3H) ppm; 13C NMR (150 MHz, CDCl3) δ 177.5, 143.9, 141.8, 137.1, 136.6, 132.5,130.4, 130.1, 129.3 (2C), 128.1, 127.9 (2C), 127.3, 125.2, 123.7, 123.0, 112.6, 110.3, 71.6, 42.2, 21.5, 20.8 ppm; HRMS (ESI): m/z (M + H)+ calcd for C23H21N2O3S+ 405.1273; found 405.1261.
(S)-5′-Bromo-1-Tosyl-2,3′-Spirobi (indolin)-2′-One (3e)
According to general procedure, the crude product was purified by silica gel chromatography (hexane/ethyl acetate = 10/1 to 4/1) to provide 3e as a white solid (169 mg, 90% yield). mp: 269–273°C.1H NMR (400 MHz, DMSO-d6) δ 10.91 (s, 1H), 7.56 (d, J = 8.0 Hz, 2H), 7.44 (d, J = 8.0 Hz, 1H), 7.37 (d, J = 8.0 Hz, 1H), 7.31–7.25 (m, 4H), 7.06 (t, J = 7.6 Hz, 1H), 6.91 (d, J = 8.0 Hz, 1H), 6.57 (s, 1H), 3.51 (d, J = 16.0 Hz, 1H), 3.30 (d, J = 16.4 Hz, 1H), 2.37 (s, 3H) ppm; 13C NMR (100 MHz, DMSO-d6) δ 176.6, 145.0, 141.5, 141.0, 136.3, 132.9, 132.0, 130.2 (2C), 128.6, 127.8, 127.5 (2C), 126.0, 125.9, 123.7, 114.0, 112.9, 112.5, 71.3, 41.7, 21.6 ppm; HRMS (ESI): m/z (M + H)+ calcd for C22H18BrN2O3S+ 469.0222; found 469.0196.
(S)-7′-Chloro-1-Tosyl-2,3′-Spirobi (indolin)-2′-One (3f)
According to general procedure, the crude product was purified by silica gel chromatography (hexane/ethyl acetate = 10/1 to 4/1) to provide 3f as a yellow solid (119 mg, 70% yield). mp: 250–255°C.1H NMR (600 MHz, DMSO-d6) δ 11.24 (s, 1H), 7.74 (d, J = 8.4 Hz, 2H), 7.37 (d, J = 7.8 Hz, 3H), 7.26 (d, J = 7.2 Hz, 1H), 7.22 (t, J = 7.8 Hz, 1H), 7.16 (d, J = 8.4 Hz, 1H), 7.03 (t, J = 7.2 Hz, 1H), 6.88 (t, J = 7.8 Hz, 1H), 6.78 (d, J = 7.8 Hz, 1H), 3.55 (d, J = 16.2 Hz, 1H), 3.30 (d, J = 16.2 Hz, 1H), 2.37 (s, 3H) ppm; 13C NMR (150 MHz, DMSO-d6) δ 176.7, 144.5, 140.9, 138.8, 135.8, 132.3, 129.8 (2C),128.0, 127.5 (2C),127.3, 125.5, 123.5, 123.1, 121.1, 114.5, 111.8, 71.8, 41.6, 21.0 ppm; HRMS (ESI): m/z (M + H)+ calcd for C22H18ClN2O3S+ 425.0727; found 425.0725.
(S)-7′-bromo-1-tosyl-2,3′-spirobi (indolin)-2′-one (3g).
According to general procedure, the crude product was purified by silica gel chromatography (hexane/ethyl acetate = 10/1 to 4/1) to provide 3g as a yellow solid (135 mg, 72% yield). mp: 270–274°C.1H NMR (400 MHz, CDCl3) δ 7.88 (d, J = 8.4 Hz, 2H), 7.74 (s, 1H), 7.40 (d, J = 8.0 Hz, 1H), 7.25 (d, J = 9.6 Hz, 2H), 7.21–7.15 (m, 3H), 7.01–6.93 (m, 2H), 6.80 (t, J = 7.6 Hz, 1H), 3.73 (d, J = 15.6 Hz, 1H), 3.21 (d, J = 15.6 Hz, 1H), 2.39 (s, 3H) ppm; 13C NMR (150 MHz, CDCl3) δ 176.2, 144.4, 141.4, 138.7, 136. 2, 132.5, 132.4, 129.6 (2C), 128.2, 128.1 (2C), 126.8, 125.2, 124.4, 123.1, 121.5, 112.4, 103.6, 72.9, 42.3, 21.6 ppm; HRMS (ESI): m/z (M + H)+ calcd for C22H18BrN2O3S+ 469.0222; found 469.0222.
(S)-1-[(4-methoxyphenyl)sulfonyl]-2,3′-spirobi (indolin)-2′-one (3h)
According to general procedure, the crude product was purified by silica gel chromatography (hexane/ethyl acetate = 10/1 to 4/1) to provide 3h as a white solid (113.7 mg, 70% yield). mp: 245–248°C.1H NMR (600 MHz, CDCl3) δ 7.87 (d, J = 9.0 Hz, 2H), 7.71 (s, 1H), 7.25–7.23 (m, 2H), 7.19–7.15 (m, 2H), 6.99 (t, J = 7.2 Hz, 1H), 6.95 (d, J = 7.2 Hz, 1H), 6.92 (d, J = 7.8 Hz, 1H), 6.89- 6.86 (m, 3H), 3.83 (s, 3H), 3.74 (d, J = 15.6 Hz, 1H), 3.22 (d, J = 16.2 Hz, 1H) ppm; 13C NMR (150 MHz, CDCl3) δ 177.4, 163.3, 141.7, 139.4, 131.0, 130.9, 130.3 (2C), 129.7, 128.1, 127.2, 125.2, 123.1, 122.9, 122.9, 114.1 (2C), 112.4, 110.5, 71.7, 55.6, 42.2 ppm; HRMS (ESI): m/z (M + H)+ calcd for C22H19N2O4S+ 407.1065; found 407.1062.
(S)-1-(phenylsulfonyl)-2,3′-spirobi (indolin)-2′-one (3i)
According to general procedure, the crude product was purified by silica gel chromatography (hexane/ethyl acetate = 10/1 to 4/1) to provide 3i as a red solid (132.5 mg, 88% yield). mp: 138–140°C.1H NMR (400 MHz, DMSO-d6) δ 10.77 (s, 1H), 7.81 (d, J = 7.6 Hz, 2H), 7.67 (t, J = 7.2 Hz, 1H), 7.53 (t, J = 7.6 Hz, 2H), 7.29–7.23 (m, 4H), 7.06–7.02 (m, 1H), 6.95 (d, J = 8.0 Hz, 1H), 6.80 (t, J = 7.2 Hz, 1H), 6.73 (t, J = 7.2 Hz, 1H), 3.55 (d, J = 16.2.4 Hz, 1H), 3.25 (d, J = 16.4 Hz, 1H) ppm; 13C NMR (100 MHz, DMSO-d6) δ 177.1, 141.6, 141.5, 139.34, 134.2, 130.8, 130.2, 129.7 (2C), 128.4, 128.0, 127.8 (2C), 125.9, 123.5, 123.0, 122.5, 112.3, 110.8, 71.8, 42.1 ppm; HRMS (ESI): m/z (M + H)+ calcd for C21H17N2O3S+ 377.0960; found 377.0955.
(S) -1-[(4-fluorophenyl)sulfonyl]-2,3′-spirobi (indolin)-2′-one (3j)
According to general procedure, the crude product was purified by silica gel chromatography (hexane/ethyl acetate = 10/1 to 4/1) to provide 3j as a red solid (141 mg, 90% yield). mp: 207–210°C. 1H NMR (400 MHz, DMSO-d6) δ 10.80 (s, 1H), 7.88–7.85 (m, 2H), 7.38 (t, J = 13.2 Hz, 2H) 7.29–7.25 (m, 4H), 7.07–7.03 (m, 1H), 6.95 (d, J = 7.6 Hz, 1H), 6.81 (t, J = 7.6 Hz, 1H), 6.73 (d, J = 7.2 Hz, 1H) 3.55 (d, J = 16.4 Hz, 1H), 3.25 (d, J = 16.4 Hz, 1H) ppm; 13C NMR (125 MHz, CDCl3) δ 177.7, 141.4, 139.7, 136.4, 130.8, 130.8, 130.5, 130.0, 128.2, 127.3, 125.4, 123.3, 123.0, 122.8, 116.2, 116.1, 112.5, 110.9, 71.8, 42.2, 29.7 ppm; HRMS (ESI): m/z (M + H)+ calcd for C21H16FN2O3S+ 395.0866; found 395.0863.
(S)-4-Chloro-1-tosyl-2,3′-spirobi (indolin)-2′-one (3k)
According to general procedure, the crude product was purified by silica gel chromatography (hexane/ethyl acetate = 10/1 to 4/1) to provide 3k as a red solid (132.6 mg, 78% yield). mp: 222–226°C.1H NMR (600 MHz, CDCl3) δ 8.07 (s, 1H), 7.76 (d, J = 7.6 Hz, 2H), 7.26 (t, J = 9.6 Hz, 1H), 7.22–7.18 (m, 3H), 7.14 (t, J = 8.4 Hz, 1H), 6.98 (d, J = 7.8 Hz, 1H), 6.94 (t, J = 7.8 Hz, 2H),6.88 (t, J = 7.8 Hz, 1H) 3.72 (d, J = 16.8 Hz, 1H), 3.30 (d, J = 16.2 Hz, 1H), 2.38 (s, 3H) ppm; 13C NMR (150 MHz, CDCl3) δ 177.1, 144.5, 142.9, 139.6, 136.2, 131.0, 130.6, 123.0, 129.7, 129.6 (2C),128.0 (2C),125.7, 123.1, 123.2, 123.0, 110.7, 110.6, 71.4, 41.5, 21.6 ppm; HRMS (ESI): m/z (M + H)+ calcd for C22H18ClN2O3S+ 425.0727; found 425.0718.
(S)-6-Bromo-1-tosyl-2,3′-spirobi (indolin)-2′-one (3l)
According to general procedure, the crude product was purified by silica gel chromatography (hexane/ethyl acetate = 10/1 to 4/1) to provide 3l as a white solid (137 mg, 73% yield). mp: 89–93°C.1H NMR (600 MHz, CDCl3) δ 7.76 (d, J = 8.4 Hz, 2H), 7.72 (s, 1H), 7.44 (s, 1H), 7.28-7.27 (m, 1H), 7.23 (d, J = 8.4 Hz, 2H), 7.13–7.12 (m, 1H), 7.01 (d, J = 7.8 Hz, 1H), 6.93–6.90 (M, 2H), 6.87 (d, J = 8.4 Hz, 1H), 3.65 (d, J = 16.2 Hz, 1H), 3.15 (d, J = 15.6 Hz, 1H), 2.39 (s, 3H) ppm; 13C NMR (150 MHz, CDCl3) δ 176.8, 144.5, 143.0, 139.4, 136.0, 130.4, 130.0, 129.7 (2C), 128.0 (2C),126.3, 126.2, 125.9, 123.2, 123.0, 121.7, 115.7, 110.6, 72.1, 41.7, 21.6 ppm; HRMS (ESI): m/z (M + H)+ calcd for C22H18BrN2O3S+ 469.0222; found 469.0108.
(S)-6-Chloro-1-tosyl-2,3′-spirobi (indolin)-2′-one (3m)
According to general procedure A, the crude product was purified by silica gel chromatography (hexane/ethyl acetate = 10/1 to 4/1) to provide 3m as a white solid (144.5 mg, 85% yield). mp: 174–177°C. 1H NMR (400 MHz, DMSO-d6) δ 10.79 (s, 1H), 7.69 (d, J = 8.0 Hz, 2H), 7.37 (d, J = 8.0 Hz, 2H), 7.28 (d, J = 7.6 Hz, 2H), 7.10 (d, J = 10.4 Hz, 2H), 6.94 (d, J = 8.0 Hz, 1H), 6.86–6.82 (m, 2H), 3.51 (d, J = 16.4 Hz, 1H), 3.25 (d, J = 16.4 Hz, 1H), 2.38 (s, 3H) ppm; 13C NMR (100 MHz, DMSO-d6) δ 176.7, 145.2, 143.0, 141.6, 136.1, 132.6, 130.4, 130.3 (2C), 127.9 (2C), 127.3, 127.2 123.2, 122.6, 112.0, 110.9, 72.6, 67.5, 41.4, 25.6, 21.5 ppm; HRMS (ESI): m/z (M + H)+ calcd for C22H18ClN2O3S+ 425.0727; found 425.0725.
(S)-5-Fluoro-1-tosyl-2,3′-spirobi (indolin)-2′-one (3n)
According to general procedure, the crude product was purified by silica gel chromatography (hexane/ethyl acetate = 10/1 to 4/1) to provide 3n as a white solid (150 mg, 92% yield). mp: 195–198°C.1H NMR (400 MHz, DMSO-d6) δ 10.77 (s, 1H), 7.67 (d, J = 8.0 Hz, 2H), 7.33 (d, J = 8.4 Hz, 2H), 7.28 (t, J = 7.6 Hz, 1H), 7.19–7.16 (m, 2H), 7.05 (d, J = 9.2 Hz, 1H), 6.94 (d, J = 8.0 Hz, 1H), 6.85-6.77 (m, 2H), 3.53 (d, J = 15.2 Hz, 1H), 3.24 (d, J = 16.4 Hz, 1H), 2.37 (s, 3H) ppm; 13C NMR (100 MHz, DMSO-d6) δ 176.8, 144.9, 141.5, 138.0, 136.3, 130.6, 130.1 (2C), 127.9 (2C), 123.1, 122.5, 114.8, 114.5, 113.6, 113.3, 113.0, 112.9, 110.8, 41.8, 25.6, 21.5 ppm; HRMS (ESI): m/z (M + H)+ calcd for C22H18FN2O3S+ 409.1022; found 409.1010.
HeLa cells were cultured in Dulbecco’s modified Eagle’s medium (#11965118, DMEM, Thermo Fisher Scientific) supplemented with 10% Certified fetal bovine serum (#C04001-500, FBS, VivaCell, Shanghai, China) penicillin (100 units/ml), and streptomycin (100 μg/ml; #15140163, 10,000 units/ml, Thermo Fisher Scientific) in a 5% CO2 humidified incubator at 37°C.
HeLa cells were incubated with MTG and LTR at 37°C for 30 min in fresh DMEM and then washed three times with fresh DMEM. Super-resolution images were acquired on a commercial OMX-3D-SIM Microscope. Images were obtained at 512 × 512 using Z-stacks with a step size of 0.125 μm. The laser model was set to fast 272 MHz, the gain was set to 1, the output powers at the fiber end: 65 mW. All fluorescence images were analyzed, and their backgrounds were subtracted with Image J software.
Statistical analysis was performed with Prism 8 (GraphPad). Normality and lognormality test to check the normal distribution. In the case of normal distribution, the statistical comparison of results was test with a Student’s t test. In the case of non-normal distribution, the statistical comparison of results was test with a Mann-Whitney test, with levels of significance set at n. s. (no significant difference), *p < 0.05, **p < 0.01, ***p < 0.001, and ****p < 0.0001. Data are presented as mean ± SEM. Analyzed cells were obtained from three replicates. Statistical significances and sample sizes in all graphs are indicated in the corresponding figure legends.
The original contributions presented in the study are included in the article/Supplementary Material, further inquiries can be directed to the corresponding authors.
All authors listed have made a substantial, direct, and intellectual contribution to the work and approved it for publication.
This work was supported by Academic Promotion Programme of Shandong First Medical University (No. 2019LJ003) and grants from National Natural Science Foundation of China (Grant No. 81903473).
The authors declare that the research was conducted in the absence of any commercial or financial relationships that could be construed as a potential conflict of interest.
All claims expressed in this article are solely those of the authors and do not necessarily represent those of their affiliated organizations, or those of the publisher, the editors and the reviewers. Any product that may be evaluated in this article, or claim that may be made by its manufacturer, is not guaranteed or endorsed by the publisher.
The Supplementary Material for this article can be found online at: https://www.frontiersin.org/articles/10.3389/fphar.2021.821518/full#supplementary-material
Arumugam, N., Almansour, A. I., Kumar, R. S., Siva Krishna, V., Sriram, D., and Dege, N. (2021). Stereoselective Synthesis and Discovery of Novel Spirooxindolopyrrolidine Engrafted Indandione Heterocyclic Hybrids as Antimycobacterial Agents. Bioorg. Chem. 110, 104798. doi:10.1016/j.bioorg.2021.104798
Bortolami, M., Leonelli, F., Feroci, M., and Vetica, F. (2021). Step Economy in the Stereoselective Synthesis of Functionalized Oxindoles via Organocatalytic Domino/One-Pot Reactions. Coc 25, 1321–1344. doi:10.2174/1385272825666210518124845
Cao, Z.-Y., and Zhou, J. (2015). Catalytic Asymmetric Synthesis of Polysubstituted Spirocyclopropyl Oxindoles: Organocatalysis versus Transition Metal Catalysis. Org. Chem. Front. 2, 849–858. doi:10.1039/c5qo00092k
Chen, Q., Fang, H., Shao, X., Tian, Z., Geng, S., Zhang, Y., et al. (2020a). A Dual-Labeling Probe to Track Functional Mitochondria-Lysosome Interactions in Live Cells. Nat. Commun. 11 (1), 6290–6300. doi:10.1038/s41467-020-20067-6
Chen, Q., Shao, X., Hao, M., Fang, H., Guan, R., Tian, Z., et al. (2020b). Quantitative Analysis of Interactive Behavior of Mitochondria and Lysosomes Using Structured Illumination Microscopy. Biomaterials 250, 120059. doi:10.1016/j.biomaterials.2020.120059
Chen, H., Wang, H., Wei, Y., Hu, M., Dong, B., Fang, H., et al. (2021). Super-resolution Imaging Reveals the Subcellular Distribution of Dextran at the Nanoscale in Living Cells. Chin. Chem. Lett. doi:10.1016/j.cclet.2021.10.025
Chen, Q., Hao, M., Wang, L., Li, L., Chen, Y., Shao, X., et al. (2021). Prefused Lysosomes Cluster on Autophagosomes Regulated by VAMP8. Cell Death Dis 12 (10), 939. doi:10.1038/s41419-021-04243-0
Chen, Q., Jin, C., Shao, X., Guan, R., Tian, Z., Wang, C., et al. (2018). Super-Resolution Tracking of Mitochondrial Dynamics with an Iridium(III) Luminophore. Small 14 (41), 1802166. doi:10.1002/smll.201802166
Cho, H., Cho, Y. Y., Shim, M. S., Lee, J. Y., Lee, H. S., and Kang, H. C. (2020). Mitochondria-targeted Drug Delivery in Cancers. Biochim. Biophys. Acta Mol. Basis. Dis. 1866 (8), 165808. doi:10.1016/j.bbadis.2020.165808
Enders, D., Zhi, Y., Zhao, K., and Shu, T. (2015). Synthesis of Benzotriazepine Derivatives via [4+3] Cycloaddition of Aza-O-Quinone Methide Intermediates and Azomethine Imines. Synthesis 48 (02), 238–244. doi:10.1055/s-0035-1560809
Farshbaf, M. J., and Kiani-Esfahani, A. (2018). Succinate Dehydrogenase: Prospect for Neurodegenerative Diseases. Mitochondrion 42, 77–83. doi:10.1016/j.mito.2017.12.002
Girgis, A. S. (2009). Regioselective Synthesis of dispiro[1H-indene-2,3'-pyrrolidine-2',3''-[3H]indole]-1,2''(1''H)-diones of Potential Anti-tumor Properties. Eur. J. Med. Chem. 44 (1), 91–100. doi:10.1016/j.ejmech.2008.03.013
Gounder, M. M., Bauer, T. M., Schwartz, G. K., Masters, T., Carvajal, R. D., Song, S., et al. (2016). A Phase 1 Study of the MDM2 Inhibitor DS-3032b in Patients (Pts) with Advanced Solid Tumors and Lymphomas. J. Clin. Oncol. 34 (15), 2581. doi:10.1200/jco.2016.34.15_suppl.2581
Gui, H. Z., Wu, X. Y., Wei, Y., and Shi, M. (2019). A Formal Condensation and [4+1] Annulation Reaction of 3‐Isothiocyanato Oxindoles with Aza‐ O ‐Quinone Methides. Adv. Synth. Catal. 361 (23), 5466–5471. doi:10.1002/adsc.201901124
Hao, L., Li, Z. W., Zhang, D. Y., He, L., Liu, W., Yang, J., et al. (2019). Monitoring Mitochondrial Viscosity with Anticancer Phosphorescent Ir(iii) Complexes via Two-Photon Lifetime Imaging. Chem. Sci. 10 (5), 1285–1293. doi:10.1039/c8sc04242j
He, Y., Liu, Y., Liu, Y., Kou, X. X., Li, Q. Z., Li, J. H., et al. (2020). Diastereodivergent Formal 1,3‐Dipolar Cycloaddition of 5‐alkenyl Thiazolones to Access Stereochemically Diverse Pyrrolidinyl Spirooxindoles. Adv. Synth. Catal. 362 (10), 2052–2058. doi:10.1002/adsc.201901541
Kang, H. C. (2018). Mitochondria-targeting Theranostics. Biomater. Res. 22, 34. doi:10.1186/s40824-018-0145-7
Karbowski, M., and Youle, R. J. (2003). Dynamics of Mitochondrial Morphology in Healthy Cells and during Apoptosis. Cell Death Differ 10 (8), 870–880. doi:10.1038/sj.cdd.4401260
Kumar, R. R., Perumal, S., Senthilkumar, P., Yogeeswari, P., and Sriram, D. (2008). Discovery of Antimycobacterial spiro-piperidin-4-ones: an Atom Economic, Stereoselective Synthesis, and Biological Intervention. J. Med. Chem. 51 (18), 5731–5735. doi:10.1021/jm800545k
Li, X., Zheng, J., Liu, W., Qiao, Q., Chen, J., Zhou, W., et al. (2020). Long-term Super-resolution Imaging of Mitochondrial Dynamics. Chin. Chem. Lett. 31 (11), 2937–2940. doi:10.1016/j.cclet.2020.05.043
Liu, S. J., Zhao, Q., Peng, C., Mao, Q., Wu, F., Zhang, F. H., et al. (2021). Design, Synthesis, and Biological Evaluation of Nitroisoxazole-Containing spiro[pyrrolidin-oxindole] Derivatives as Novel Glutathione Peroxidase 4/mouse Double Minute 2 Dual Inhibitors that Inhibit Breast Adenocarcinoma Cell Proliferation. Eur. J. Med. Chem. 217, 113359. doi:10.1016/j.ejmech.2021.113359
Liu, X., Lu, D., Wu, J. H., Tan, J. P., Jiang, C., Gao, G., et al. (2020). Stereoselective Synthesis of CF 3 ‐Containing Spirooxindoles via 1,3‐Dipolar Cycloaddition by Dipeptide‐Based Phosphonium Salt Catalysis. Adv. Synth. Catal. 362 (7), 1490–1495. doi:10.1002/adsc.202000001
Liu, L. Y., Fang, H., Chen, Q., Chan, M. H., Ng, M., and Wang, K. N. (2020). Multiple-Color Platinum Complex with Super-large Stokes Shift for Super-resolution Imaging of Autolysosome Escape. Angew. Chem. Int. Ed. 59 (43), 19229–19236. doi:10.1002/anie.202007878
Lou, J., Chu, G., Zhou, G., Jiang, J., Huang, F., Xu, J., et al. (2010). Comparison between Two Kinds of Cigarette Smoke Condensates (CSCs) of the Cytogenotoxicity and Protein Expression in a Human B-Cell Lymphoblastoid Cell Line Using CCK-8 Assay, Comet Assay and Protein Microarray. Mutat. Res. 697 (1-2), 55–59. doi:10.1016/j.mrgentox.2010.01.014
Mo, R., Sun, Q., Xue, J., Li, N., Li, W., Zhang, C., et al. (2012). Multistage pH-Responsive Liposomes for Mitochondrial-Targeted Anticancer Drug Delivery. Adv. Mater. 24 (27), 3659–3665. doi:10.1002/adma.201201498
Nakamura, A., Kuwano, S., Sun, J., Araseki, K., Ogino, E., and Arai, T. (2020). Chiral Dinuclear Benzyliminobinaphthoxy‐Palladium Catalyst for Asymmetric Mannich Reaction of Aldimines and Isatin‐Derived Ketimines with Alkylmalononitriles. Adv. Synth. Catal. 362 (15), 3105–3109. doi:10.1002/adsc.202000447
Nasri, S., Bayat, M., and Mirzaei, F. (2021). Recent Strategies in the Synthesis of Spiroindole and Spirooxindole Scaffolds. Top. Curr. Chem. (Cham) 379 (4), 25. doi:10.1007/s41061-021-00337-7
Neil, A., Peter, A., Steven, M., and John, A. (1997). Oxindoles from Phalaris Coerulescens. Photochem 48 (3), 437–439.
Reddy, A. C. S., Reddy, P. M., and Anbarasan, P. (2020). Diastereoselective Palladium Catalyzed Carbenylative Amination of Ortho ‐Vinylanilines with 3‐Diazoindolin‐2‐ones. Adv. Synth. Catal. 362 (4), 801–806. doi:10.1002/adsc.201901286
Saranya, P. V., Neetha, M., Aneeja, T., and Anilkumar, G. (2021). Transition Metal-Catalyzed Synthesis of Spirooxindoles. RSC Adv. 11 (13), 7146–7179. doi:10.1039/d1ra00139f
Shao, X., Chen, Q., Hu, L., Tian, Z., Liu, L., Liu, F., et al. (2020). Super-resolution Quantification of Nanoscale Damage to Mitochondria in Live Cells. Nano Res. 13, 2149–2155. doi:10.1007/s12274-020-2822-9
Wang, D., Lu, X., Sun, S., Yu, H., Su, H., Wu, Y., et al. (2019). Unified and Benign Synthesis of Spirooxindoles via Bifunctional and Recyclable Iodide-Salt-Catalyzed Oxidative Coupling in Water. Eur. J. Org. Chem. 2019 (35), 6028–6033. doi:10.1002/ejoc.201900751
Wang, Y., Duan, H., Shi, H., Zhang, S., Xu, Y., Zhu, W., et al. (2020). A Highly Sensitive Fluorescent Probe for Tracking Intracellular Zinc Ions and Direct Imaging of Prostatic Tissue in Mice. Chin. Chem. Lett. 31 (11), 2933–2936. doi:10.1016/j.cclet.2020.05.028
Wei, Y., Kong, L., Chen, H., Liu, Y., Xu, Y., Wng, H., et al. (2022). Super-resolution Image-Based Tracking of Drug Distribution in Mitochondria of a Label-free Naturally Derived Drug Molecules. Chem. Eng. J. 429, 132134. doi:10.1016/j.cej.2021.132134
Wheeler, H. E., Maitland, M. L., Dolan, M. E., Cox, N. J., and Ratain, M. J. (2013). Cancer Pharmacogenomics: Strategies and Challenges. Nat. Rev. Genet. 14 (1), 23–34. doi:10.1038/nrg3352
Zhang, X., Ren, T., Yang, F., and Yuan, L. (2021). Rational Design of Far Red to Near-Infrared Rhodamine Analogues with Huge Stokes Shifts for Single-Laser Excitation Multicolor Imaging. Chin. Chem. Lett. doi:10.1016/j.cclet.2021.06.038
Zhang, C., Shao, H., Zhang, J., Guo, X., Liu, Y., Song, Z., et al. (2021). Long-term Live-Cell Lipid Droplet-Targeted Biosensor Development for Nanoscopic Tracking of Lipid Droplet-Mitochondria Contact Sites. Theranostics 11 (16), 7767–7778. doi:10.7150/thno.59848
Zhao, H., Yin, R., Wang, Y., Lee, Y. H., Luo, T., Zhang, J., et al. (2017). Modulating Mitochondrial Morphology Enhances Antitumor Effect of 5-ALA-Mediated Photodynamic Therapy Both In Vitro and In Vivo. J. Photochem. Photobiol. B 176, 81–91. doi:10.1016/j.jphotobiol.2017.09.017
Zhao, K., Zhi, Y., Li, X., Puttreddy, R., Rissanen, K., and Enders, D. (2016a). Asymmetric Synthesis of 3,3'-Pyrrolidinyl-Dispirooxindoles via a One-Pot Organocatalytic Mannich/deprotection/aza-Michael Sequence. Chem. Commun. (Camb) 52 (11), 2249–2252. doi:10.1039/c5cc10057g
Zhao, K., Zhi, Y., Shu, T., Valkonen, A., Rissanen, K., and Enders, D. (2016b). Organocatalytic Domino Oxa-Michael/1,6-Addition Reactions: Asymmetric Synthesis of Chromans Bearing Oxindole Scaffolds. Angew. Chem. Int. Ed. Engl. 55 (39), 12104–12108. doi:10.1002/anie.201606947
Zhao, Y., Liu, L., Sun, W., Lu, J., McEachern, D., Li, X., et al. (2013a). Diastereomeric Spirooxindoles as Highly Potent and Efficacious MDM2 Inhibitors. J. Am. Chem. Soc. 135 (19), 7223–7234. doi:10.1021/ja3125417
Zhao, Y., Yu, S., Sun, W., Liu, L., Lu, J., McEachern, D., et al. (2013b). A Potent Small-Molecule Inhibitor of the MDM2-P53 Interaction (MI-888) Achieved Complete and Durable Tumor Regression in Mice. J. Med. Chem. 56 (13), 5553–5561. doi:10.1021/jm8400570810.1021/jm4005708
Zhi, Y., Zhao, K., Liu, Q., Wang, A., and Enders, D. (2016). Asymmetric Synthesis of Functionalized Trifluoromethyl-Substituted Pyrrolidines via an Organocatalytic Domino Michael/Mannich [3+2] Cycloaddition. Chem. Commun. (Camb) 52 (97), 14011–14014. doi:10.1039/c6cc08352h
Zhi, Y., Zhao, K., von Essen, C., Rissanen, K., and Enders, D. (2018). Synthesis of Trans-disubstituted-2,3-dihydrobenzofurans by a Formal [4 + 1] Annulation between Para-Quinone Methides and Sulfonium Salts. Org. Chem. Front. 5 (8), 1348–1351. doi:10.1039/c8qo00008e
Zou, W., Chen, Q., Slone, J., Yang, L., Lou, X., Diao, J., et al. (2021). Nanoscopic Quantification of Sub-mitochondrial Morphology, Mitophagy and Mitochondrial Dynamics in Living Cells Derived from Patients with Mitochondrial Diseases. J. Nanobiotechnol 19 (1), 136–146. doi:10.1186/s12951-021-00882-9
Keywords: spirooxindole, aza-ortho-quinone methides, mitochondria, morphology, annulation
Citation: Li H, Yu Z, Sun H, Liu B, Wang X, Shao Z, Wang M, Xie W, Yao X, Yao Q and Zhi Y (2022) Efficient Synthesis of 2,3′-Spirobi (Indolin)-2′-Ones and Preliminary Evaluation of Their Damage to Mitochondria in HeLa Cells. Front. Pharmacol. 12:821518. doi: 10.3389/fphar.2021.821518
Received: 24 November 2021; Accepted: 31 December 2021;
Published: 23 February 2022.
Edited by:
Peixue Ling, Shandong University (Qingdao), ChinaReviewed by:
Yinan Zhang, Nanjing University of Chinese Medicine, ChinaCopyright © 2022 Li, Yu, Sun, Liu, Wang, Shao, Wang, Xie, Yao, Yao and Zhi. This is an open-access article distributed under the terms of the Creative Commons Attribution License (CC BY). The use, distribution or reproduction in other forums is permitted, provided the original author(s) and the copyright owner(s) are credited and that the original publication in this journal is cited, in accordance with accepted academic practice. No use, distribution or reproduction is permitted which does not comply with these terms.
*Correspondence: Ying Zhi, emhpeWluZ0BzZGZtdS5lZHUuY24=; Qingqiang Yao, cXF5YW9Ac2RmbXUuZWR1LmNu
†These authors have contributed equally to this work and share first authorship
Disclaimer: All claims expressed in this article are solely those of the authors and do not necessarily represent those of their affiliated organizations, or those of the publisher, the editors and the reviewers. Any product that may be evaluated in this article or claim that may be made by its manufacturer is not guaranteed or endorsed by the publisher.
Research integrity at Frontiers
Learn more about the work of our research integrity team to safeguard the quality of each article we publish.