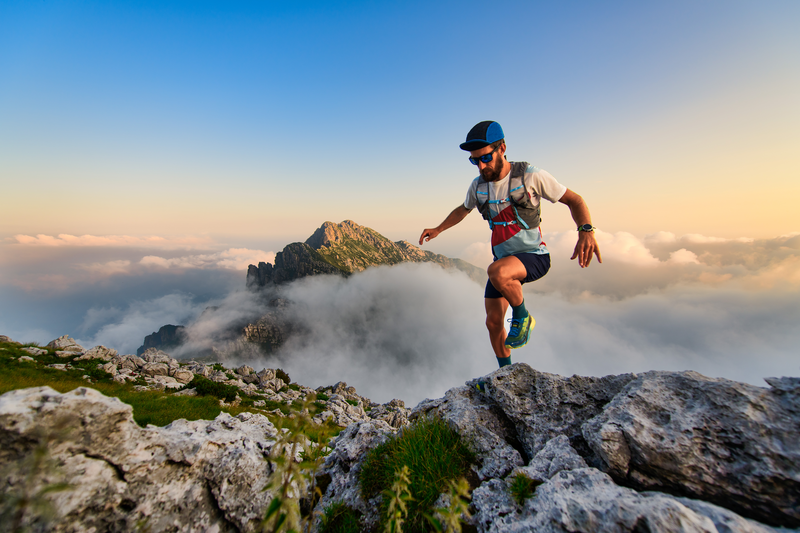
95% of researchers rate our articles as excellent or good
Learn more about the work of our research integrity team to safeguard the quality of each article we publish.
Find out more
REVIEW article
Front. Pharmacol. , 01 April 2021
Sec. Obstetric and Pediatric Pharmacology
Volume 12 - 2021 | https://doi.org/10.3389/fphar.2021.635345
This article is part of the Research Topic Model-Based Evaluation of Antimicrobial Agents in Children View all 16 articles
The response to medications in children differs not only in comparison to adults but also between children of the different age groups and according to the disease. This is true for anti-infectives that are widely prescribed in children with malignancy. In the absence of pharmacokinetic/pharmacodynamic paediatric studies, dosage is frequently based on protocols adapted to adults. After a short presentation of the drugs, we reviewed the population pharmacokinetic studies available for glycopeptides (vancomycin and teicoplanin, n = 5) and antifungals (voriconazole, posaconazole, and amphotericin B, n = 9) currently administered in children with onco-hematological malignancies. For each of them, we reported the main study characteristics including identified covariates affecting pharmacokinetics and proposed paediatric dosage recommendations. This review highlighted the very limited amount of data available, the lack of consensus regarding PK/PD targets used for dosing optimization and regarding dosage recommendations when available. Additional PK studies are urgently needed in this specific patient population. In addition to pharmacokinetics, efficacy may be altered in immunocompromised patients and prospective clinical evaluation of new dosage regimen should be provided as they are missing in most cases.
Child specific challenges of treatment include 1) frequent off-label/unlicensed use (which increases the risk of adverse drug reactions and lack of efficacy), 2) limited pharmacokinetic (PK) and/or pharmacodynamic (PD) age group and disease specific data, 3) considerable variation in drug dosages, and 4) an increased risk of medication errors (Gore et al., 2017; Jong, et al., 2001).
According to pharmacoepidemiologic studies, anti-infective drugs are widely prescribed in children (de Bie et al., 2016) but paediatric pharmacokinetic/pharmacodynamic (PK/PD) studies are limited and dosage frequently based on protocols adapted to adults. However, the response of children to medication differs in comparison to adults and also between different paediatric age groups and between diseases. Data are also missing in many paediatric subpopulations presenting with frequent specific diseases.
In the past 20 years, several initiatives were undertaken in the United States and in Europe to encourage paediatric research and drug development (Choonara, 2007). Although the number of paediatric investigation plans and marketing authorisations increased, studies evaluating dosage regimen and therapeutic strategies in children presenting with frequent specific conditions are sparse and require public-private fundings (Turner et al., 2014; Ruggieri et al., 2015). This is particularly true in paediatric malignancies, representing specific diseases different from adults.
We selected paediatric onco-hematologic malignancies as a key area where anti-infectives are used regularly to manage infections complicating chemotherapy. However, dosage recommandations based on PK/PD targets originate frequently for adult studies. In this context, we reviewed the population PK (PopPK) studies available for currently prescribed glycopeptides (vancomycin and teicoplanin) and antifungals (posaconazole, voriconazole, and amphotericin B) to report on the key variables impacting PK parameters in children and analyze available dosage recommendations.
PK-PD indices represent the quantitative relationship between pharmacokinetic measures the test drug (such as area under the curve AUC) and a microbiologic measure of susceptibility (minimum inhibitory concentration - MIC). The microbiological data from animal or in vitro experiments provide initial insight into PK-PD most likely to be associated with efficacy.
A concentration-dependent pattern of activity may be observed and AUC0-24/MIC ratio and/or the Cmax/MIC ratio are the PK/PD indices that usually predict efficacy in PK-PD models.
A time-dependent pattern of bactericidal activity may be observed and time > MIC and/or AUC0–24/MIC ratio are the indices that usually predict efficacy in PK-PD models.
Glycopeptides are time-dependent/concentration-independent antibiotics with moderate persistent killing, active against susceptible strains of methicillin-resistant (beta-lactam resistant) staphylococci. For azole antifungals, the PK/PD index that best relates to the outcome is the AUC0–24/MIC (European Medicines Agency, 2015; Gómez-López, 2020).
The first line treatment for invasive methicillin-resistant Staphylococcus aureus (MRSA) infections is a glycopeptide antibiotic, either vancomycin (a glycopeptide) or teicoplanin (a lipoglycopeptide). Both are often prescribed to broaden initial empirical antibiotic in case of persistent fever in paediatric and adult patients with HM (Libuit et al., 2014; Lehrnbecher et al., 2017). Teicoplanin is not inferior to vancomycin with regard to efficacy and is associated with a lower adverse event rate than vancomycin (Menichetti et al., 1994; Finch and Eliopoulos, 2005).
Vancomycin is a large, hydrophilic molecule with poor oral absorption. Hence it is given intravenously to treat systemic infections. Vancomycin is 25–50% protein-bound, mainly to albumin and immunoglobulins, and protein binding changes non-linearly with vancomycin concentrations. It is almost exclusively eliminated by the renal route via glomerular filtration and to some extent via active tubular secretion. Elimination half-life is 6–12 h. Factors that affect its clinical activity, include variable tissue distribution, inoculum size, and emerging resistance.
The AUC0–24/MIC ratio is the best predictor of vancomycin efficacy in adults. Various studies have shown that a vancomycin AUC0–24/MIC ratio >400 best predicts treatment outcomes for invasive MRSA infection in adults.
Many vancomycin PK studies and reviews are available in adults, including PopPK studies and report that both vancomycin clearance (CL) and volume of distribution were higher in cancer than non-cancer patients (Yasuhara et al., 1998; Buelga et al., 2005; Jarkowski et al., 2012). PK studies also reported large variability in vancomycin disposition in children (neonates excluded) (Chang, 1995; Krivoy et al., 1998; Wrishko et al., 2000; Marsot et al., 2012; Hadi et al., 2016; Tkachuk et al., 2018).
Similarly to vancomycin, teicoplanin needs to be administered intravenously as bioavailability is extremely low, it is 25–50% protein bound and also exclusively eliminated by the renal route (Marsot et al., 2012).
PopPK studies available in adults receiving the drug for various indications, showed high variability in drug disposition (Yu et al., 1995; Lortholary et al., 1996; Soy et al., 2006). In children, different classical PK studies but with a relatively low number of patients (12,13, and 6, respectively, in the three studies) provided conflicting conclusions on the impact of age on teicoplanin PK parameters (Tarral et al., 1988; Terragna et al., 1988; Reed et al., 1997).
Invasive fungal disease (IFD) is an important cause of morbidity and mortality in immunocompromised children receiving chemotherapy for cancer and those undergoing hematopoietic stem cell transplant (HSCT). Its incidence varies according to chemotherapy regimen and supportive care practices (Lehrnbecher et al., 2009; Groll et al., 2014).
Antifungal chemoprophylaxis is therefore recommended for high risk patients with prolonged neutropenia, prolonged use of steroids or in different subgroups of leukemia, taking into account the local epidemiology, patient comorbidity or specific treatment modalities (Fisher et al., 2018).
There are no major differences between children and adults in the choice of treatment of established infections and triazole antifungal agents are potential choices both for prophylaxis and treatment of probable and proven IFDs.
Voriconazole is a recent triazole antifungal agent with potent activity against a wide range of clinically significant pathogens, including Aspergillus and Candida. Voriconazole may be administered orally and intravenously. Bioavailability has been estimated to be >90% in healthy volunteers, substantially lower in adults and even more in children with malignancies (Karlsson et al., 2009). A potential mechanistic explanation could be that paediatric patients exhibit greater systemic metabolism but also greater first-pass metabolism than that of adults.
Voriconazole is extensively metabolized by polymorphic cytochrome P450 (CYP) isoenzymes CYP2C9, CYP2C19 and CYP3A4. The prevalence of CYP2C19 poor metabolizers is 3–5% among Caucasians and black Africans, 15–20% among Asian populations. Only <2% of the dose is excreted unchanged in the urine. AUC/MIC ratio >20–25 was previously proposed as the PK/PD target to optimize voriconazole dosing (Andes, 2003). Previously published reports in adult patients suggested aiming at a plasma trough drug concentration between 1 and 5.5 mg/L for efficacy and limiting toxicity (Pascual et al., 2008). PopPK studies on voriconazole have been conducted in adults, either healthy volunteers or sick patients (Vehreschild et al., 2012; van Iersel et al., 2018; Liu et al., 2019; Shi et al., 2019) and available studies were recently reviewed (Shi et al., 2019), reporting marked IIV in PK parameters and only limited intraindividual variation.
Posaconazole is licensed for prophylaxis of IFD in 1) patients with prolonged neutropenia and who are at high risk of developing IFD complicating HM, 2) patients at high risk of developing IFD following HSCT and under immunosuppressive therapy for graft-versus-host disease.
Posaconazole showed potent dose-dependent in vivo antifungal activity on prophylaxis and treatment against most fungal infections. PK studies were predominantly performed in healthy volunteers and hematological adult patients and were recently reviewed (Chen et al., 2019). Bioavailability was reported to be around 50% in healthy volunteers with the suspension and delayed-release tablet (Chen et al., 2019), but lower in patients receiving the posaconazole suspension (Dolton et al., 2014). It is bound to plasma proteins for more than 98%. It is predominantly eliminated unchanged in feces or in urine. Elimination by glucuronidation (UGT1A4) is only limited (less than 20%).
Different biomarkers of efficacy are reported in adults, 1) the AUC/MIC showed the strongest correlation with therapeutic success, 2) the posaconazole average plasma concentration (Cavg) ≥ 1.25mg/L at steady-state was fixed as a valid cut-off value for IFD treatment as it was associated with 75% successful response rates in patients with invasive aspergillosis (Chen et al., 2020), while Cavg of 0.7 mg/L is accepted as a target for prophylaxis. Trough concentrations (Cmin) proved to be well correlated with Cavg or AUC 0–24 are biomarkers easier to use for monitoring.
According to the European marketing authorization, safety and efficacy are not established in children aged below 18 years (Table 1). Posaconazole can be administered as an oral suspension (40 mg/ml), a delayed-release tablet (100 mg), and more recently as intravenous formulation (18 mg/ml): only the oral formulations are licensed for paediatric patients. Prophylactic posaconazole was shown superior to fluconazole or itraconazole in reducing IFD and fungal related mortality in patients with graft vs. host disease (Ullmann et al., 2007). In addition, acceptability is high. Dosing information depends on the formulation, patient’s age and indication (prophylaxis or treatment). No PK/PD data exist on tablets and intravenous formulation for all paediatric age groups.
TABLE 1. Indications and European marketing authorization status of glycopeptides and antifungals in the different paediatric age groups.
Amphotericin B is a highly lipophilic drug administered intravenously as it is poorly absorbed orally. Amphotericin B-deoxycholate (D-AmB), a mixed micellar dispersion with deoxycholate, has been the cornerstone for the management of life-threatening fungal infections. This formulation has suboptimal clinical success and frequent nephron-toxic effects at usual recommended doses. It was replaced in the 1990 by the lipid-based formulations (L-AmB) encapsulating amphotericin B into liposomes or binding the drug to lipids (Walsh et al., 1999; Chen et al., 2020). L-AmB is less toxic and has limited infusion-associated reactions, increased therapeutic index and can be administered at higher dosages (Janoff, 1990; Moreau et al., 1992). AmB exhibits concentration-dependent killing of fungal organisms, with a long post-antifungal effect (Andes et al., 2003).
Standard PK studies, comparing the pharmacokinetics and tolerability of amphotericin B administered in a conventional 5% dextrose (glucose) (5% D) solution and in a 20% fat emulsion formulation (Intralipid; 20% IL) were initially conducted in adults. Differences in PK profile between the two formulations resulted in higher distribution volume, decreased Cmax and AUC, and increased CL with the 20% IL form (Ayestarán et al., 1996). A possible reason for the PK differences between the two methods of administration is the larger particle size of AmB in lipid emulsion.
The first PK studies in neutropenic adults showed dose-related, non-linear, saturation-like PK. The Cmax/MIC ratio of 2 may provide sufficient antifungal efficacy. The first population PK in adults (75 patients received 0.5–8.0 mg/kg of body weight of amphotericin B colloidal dispersion for 28 days) showed that plasma CL and volume of distribution increased with escalating doses, but without net change in renal function (Amantea et al., 1995).
We search for PopPK studies of anti-infectives (glycopeptides and antifungals) in onco-hematological malignancies (leukemia or lymphoma or multiple myeloma or malignant disease) affecting paediatric patients (neonates excluded), published up to August 31, 2020, using Pubmed to identify the PopPK studies of glycopeptides/vancomycin/teicoplanin, antifungals/voriconazole/posaconazole/amphotericinB, in children/paediatric patients with hemato-oncology malignancy/acute leukemia/lymphoma. We selected the studies on one of our five anti-infectives of interest and selected additional articles by reviewing the bibliography of the selected publications. Tables were built to summarize the PopPK studies, presenting the study drug, patients’ characteristics (number, age, and weight), underlying disease and indication of treatment, number of samples, software, covariates analyzed, and retained in the PK model, PK/PD target used for simulations and dosage recommendations.
A total of 19 paediatric PopPK studies were identified and 14 included in this review: five for glycopeptides (vancomycin n = 3 and teicoplanin n = 2) and 9 for antifungals (voriconazole n = 4, posaconazole n = 1, amphotericin B n = 4) administered in children with malignancy.
The PopPK studies of glycopeptides in paediatric HM are presented in Table 2.
TABLE 2. Population pharmacokinetic studies of glycopeptides in paediatric onco-hematological malignancies.
During our review process, two vancomycin PopPK studies in sick children were excluded as the underlying disease and indications of treatment were missing, or patients with various diseases were included (Lamarre et al., 2000; Hahn et al., 2015). We identified and analyzed three vancomycin PopPK studies (Zhao et al., 2014; Marsot et al., 2018; Guilhaumou et al., 2016) including two PopPK studies in paediatric HM and 1 being an external validation (Guilhaumou et al., 2016) of one of them (Marsot et al., 2018). Data are presented in Table 2. The number of patients included were 70 (Zhao et al., 2014) and 121 (Marsot et al., 2018), with a wide range of both ages and weights. Concentrations were obtained during therapeutic drug monitoring (TDM). Among all covariates tested, weight (with fixed or estimated allometric coefficients) was always significant, creatinine CL was significant only in the model by Zhao (Zhao et al., 2014), type of disease and coadministration of cyclosporin were significant only in the model by Guilhaumou (Guilhaumou et al., 2016). The primary PK/PD target used for simulations was AUC/MIC ≥400 h in one case and the steady-state concentration of 20–25 mg/L in the other one. After accounting for significant covariates, the mean value of the interindividual variability (IIV) in vancomycin CL was 34.8 and 31.1%, respectively, in the two studies. Both studies showed that higher doses are required in cancer paediatric patients. Dosage adaptation might use either a patient tailored dose or a proposed chart, taking into account the identified variables. In both cases, drug monitoring is still recommended.
We identified and analyzed two teicoplanin PopPK studies in children with cancer (Ramos-Martín et al., 2014; Zhao et al., 2015). Two additional studies population PK studies are available but in children without malignancy treated in intensive care or with renal dysfunction (Lukas et al., 2004; Gao et al., 2020). Results of the analyzed PopPK studies showed that weight and renal function (quantified by creatinine CL) are covariates explaining part of the observed variability. PK/PD targets used for simulations were different between the two studies. A patient tailored dose based on weight and creatinine CL might reduce variability in teicoplanin AUC and trough concentration (Cmin) values compared with the mgkg−1 basis dose (Zhao et al., 2015). Drug monitoring is still recommended.
The PopPK studies of antifungals in paediatric onco-hematological malignancies are presented in Tables 3, 4.
TABLE 3. Population pharmacokinetic studies of the antifungals Voriconazole and Posaconazole in paediatric onco-hematological malignancies.
TABLE 4. Population pharmacokinetic studies of dextrose and lipid Amphotericin B (D and L-AmB) in paediatric onco-hematological malignancies.
In children, voriconazole PK studies demonstrated high IIV with no apparent relationship to dose in immunocompromised children (Walsh et al., 2010; Pieper et al., 2012). Bioavailability is substantially lower in children than in adults with HM (20 and 59.4%, respectively) (Karlsson et al., 2009).
Four PopPK studies in children were analyzed and summarized in Table 3 (Karlsson et al., 2009; Walsh et al., 2004; Muto et al., 2015; Gastine et al., 2017), the larger one being by Karlsson (Karlsson et al., 2009). An additional one was excluded because of missing information (Carlesse et al., 2019). Data were obtained from rich sampling in patients receiving voriconazole in experimental settings in all four cases. Voriconazole was modeled either with linear or non-linear or mixed of both linear and non-linear elimination. The following covariates were significant in 1–4 of the analyzed models: weight, CYP2C19 genotype status, alanine aminotransferase (ALT), alkaline phosphatase (ALKP) and population age groups (adolescent or child). CYP2C19 deficient genotypes and increased levels of ALT were the most important determinants of voriconazole CL, associated with lower CL values. The additional importance of age was evidenced in the recent study by Yan, showing that, in the paediatric population, the patients younger than 3years, might need higher doses to reach the same trough concentrations and exposure than patients over 3years (Yan et al., 2018). Simulations conducted in the four analyzed studies led to the conclusion that the dose required in children was higher than in adults. However, recommended dosages differed from one study to one other for the same age group (Muto et al., 2015; Gastine et al., 2017). Only one Posaconazole PopPK study was conducted in children (Boonsathorn et al., 2019): weight, formulation (suspension or tablet), dose, diarrhea and coadministration of proton pump inhibitors had a significant impact on PK parameters. The estimated values of CL/F and V/F related to the delayed-release tablet formulation and standardized to a 70 kg individual were comparable to those reported in adults. These children showed a higher IIV on CL/F compared to adults (63.0 vs. 24.2 or 37.9%) (van Iersel et al., 2018; Petitcollin et al., 2017) suggesting a potential age-associated maturation of hepatic UGT1A4. Suspension had poor and saturable bioavailability, which decreased with increasing dose. Diarrhea and proton pump inhibitors were also associated with reduced bioavailability of the suspension. Based on the probability of target attainment (PTA) of trough concentration >1mg/L in fungal infection treatment, the authors issued dosage recommendations in children up to 6 years and between 7 and 12 years with an initial dose of 200 and 300 mg suspension four times daily, respectively. When tablets can be used in patients aged seven or over, 200 mg tablets three times daily are required. Dosage have then to be adapted to TDM after the initial phase of treatment.
For amphotericin B, the first PopPK study in children by Nath (Nath et al., 2001) compared D and L-Amb, and then analyzed the two formulations separately (Table 4). This study was conducted with significant number of patients and samples, in a wide range of ages, and showed that weight and formulation (D or L-AmB) had a significant impact on PK parameters. Only one of the four PopPK studies identified resulted in dosage recommendation. Using their previously developed model (Nath et al., 2001), Nath et al. proposed weight-based dosage recommendation for D-AmB (i.e., 1.25–1.5, 1, and 0.75 mg/kg/day for children weighing 10–25, 25–45, and 45–55 kg, respectively) targeting the 0.76–1.05 mg/L range of through level at steady-state (Nath et al., 2007). Lestner and co-authors showed the absence of correlation between absolute dose and exposure (maximum concentration–Cmax, minimum concentration–Cmin, or AUC0–24) but a significant correlation between steady-state exposure (AUC0–24) and change in serum creatinine. In Japanese paediatric patients (Ohata et al., 2015), the predicted parameters Cmax/dose and AUC0-24/dose were similar to those in the non-Japanese paediatric patients (Kohno et al., 2013) and Japanese adult patients at 1.0, 2.5, and 5.0 mg/kg/day given as 1 h infusion.
In this review, we analyzed the population pharmacokinetics of five anti-infectives in paediatric patients with onco-hematological diseases. The studies, based on a nonlinear mixed effects modeling approach, aimed to estimate the typical population PK parameters, their variability between patients, and the variability between occasions and within patients, and to identify the covariates with significant impact on variability in PK.
Most PK studies conducted in children focus on age and organ maturation/function to explain variability in drug disposition. In addition to these key covariates, studies focusing on the potential impact of the disease underlying infection on anti-infective PK are sparse in the paediatric field. Data are missing in many paediatric subpopulations presenting with specific diseases. In paediatric malignancies, most paediatric chemotherapy regimens are intensive with high risk of infection complicating profound neutropenia and requiring anti-infectives at effective and non-toxic doses. We focused our research on the frequently administered glycopeptides (vancomycin and teicoplanin) and antifungals (posaconazole, voriconazole and amphotericin B). Our review shows that data on anti-infectives are limited in children with cancer and that their optimal dosing regimen remains controversial or undefined.
PK and PopPK determine the relation dose/concentration and participate to identify and quantify the impact of covariates on drug disposition. Exposure to anticancer drugs, most of them having a narrow therapeutic range, is central to optimize cure rate of paediatric patients with malignant diseases. However, during treatment, induced immunosuppression is at high risk of infection and anti-infective dosage, if inadequate, may result in infection-related morbidity and increased mortality, making optimization of dosing regimen essential. According to regulatory guidelines, antimicrobial agents are good examples of drugs for which modelling and simulation techniques can be used to develop dosage recommendations in children. PK/PD surrogate markers of efficacy that are used for this purpose include 1) a PK parameter (AUC, Cmax, Time) (Kearns et al., 2003), 2) a PD parameter (MIC) which is function of the germ responsible for the infection, and based either on identification of the germ or more frequently on local germ epidemiology if infection is only suspected.
PK and PopPK of glycopeptides in children with HM diseases are sparse.
IIV in vancomycin disposition in children (neonates excluded) was reported to be primarily linked to patient’s age, type of disease and clinical condition (e.g., renal function, proven infection) (Chang, 1995; Krivoy et al., 1998; Wrishko et al., 2000; Marsot et al., 2012; Hadi et al., 2016; Tkachuk et al., 2018). The impact of malignancy on vancomycin disposition was initially reported by Chang, who showed that vancomycin CL in 33 paediatric patients with malignancy was significantly larger than in 31 patients without cancer while the volumes of distribution were similar (Chang, 1995). The impact of febrile neutropenia was tested in only one study including 109 children with hematological and solid malignancies (Keita et al., 2016), using the model previously developed in adults by Yasuhara (Yasuhara et al., 1998). Multilinear regression analysis of individual patient CL identified age and estimated glomerular filtration rate (eGFR) as covariates affecting drug disposition. Febrile neutropenia did not show any significant impact on CL. Accordingly, in children with malignancy, higher doses than the currently used dosage regimen of 30–40 mg/kg/24 h, are needed to increase the percentage of patients reaching the PK/PD vancomycin AUC/MIC breakpoint of at least 400 h (value determined in adults with Staphylococcus aureus pneumonia (Moise-Broder et al., 2004)) or the steady-state target concentration of 20–25 mg/L, while limiting the risk of emergence of vancomycin-resistant microorganisms (Seixas et al., 2016). Optimal doses have to be adapted to weight, creatinine CL, type of disease and co-administration of cyclosporin if any, but remain to be validated prospectively, both in terms of safety and efficacy.
In the teicoplanin PK studies in paediatric malignancy, children had more variability in drug exposures than the adults. The two PopPK studies on paediatric malignancies confirmed that teicoplanin CL was higher in paediatric cancer patients than in children without cancer, with weight and creatinine CL being significant covariates (Ramos-Martín et al., 2014; Zhao et al., 2015). This is most probably related to high glomerular filtration secondary to hyperhydration which is included in HM protocols. In addition, the complex composition of generic teicoplanin products may have a potential impact on both biological analysis and PD. Additional data showed that current weight-based dosage was associated with a low proportion of patients attaining minimum recommended serum drug concentrations at steady state (Cmin value of 10 mg/L) (Dufort et al., 1996; Sánchez et al., 1999; Strenger et al., 2013). According to these data, teicoplanin individualized dosing regimen needs to be recommended for different renal function groups and TDM remains recommended in HM patients.
For antifungals, data are even more limited.
For voriconazole, high paediatric variability is partially explained by body weight, cytochrome P450 2C19 genotype, liver function, and concomitant medications. However, although the genotyping status helps to explain the variability in voriconazole exposure, the CYP2C19 genotyping status alone does not warrant dose adjustment as the voriconazole exposures varied widely within each genotype and overlap considerably across CYP2C19 genotypes. Voriconazole monitoring remains recommended. Therefore, experts advise TDM, in particular in younger children (Chen et al., 2012).
For posaconazole, and according to data obtained in children with malignancy, weight and formulation (suspension or tablet) have an important impact on bioavailability. However, data are extremely limited, did not explore additional covariates already identified in adults such as pharmacogenetic biomarkers and additional studies are particularly needed to validate posaconazole use in paediatric malignancies. Although used in children, this drug is prescribed off-label, as the marketing authorization stated that “safety and efficacy are not established in children aged below 18years” (Table 1).
Amphotericin B is formulated as amphotericin B-deoxycholate (D-AmB) and lipid emulsions (L-AmB). In children, a classical dose escalation study including 40 immunocompromised paediatric patients (2.5, 5.0, 7.5 or 10 mg per kg L-AMB) concluded that L-AMB could be administered to paediatric patients at dosages similar to those of adults but azotemia may develop, especially in those receiving ≥5.0 mg/kg/day (Seibel et al., 2017). In children, Lestner and co-authors (Lestner et al., 2016) showed the absence of correlation between absolute dose and exposure (Cmax, Cmin, or AUC0–24) but a significant correlation between steady-state exposure (AUC0–24) and change in serum creatinine. Weigt-based dosage recommendation to reach the target through level at steady-state were issued for D-AmB but not for L-AmB (Nath et al., 2001; Nath et al., 2007). When immunocompromised children experience fever that persists in spite of broad-spectrum antibiotic therapy, they receive D-AmB by the standard dose of 1 mg/kg/day that may be insufficient to prevent fungal surinfection or to control clinically detected or undetected fungal infection. Here again, additional PK and efficacy studies are required for a safer use in cancer children.
As illustrated by the present review, PopPK studies on antibiotics and antifungals including in paediatric malignancy are limited for well-known reasons, ethical and technical. The major barriers to paediatric PK studies are the relatively large volumes of blood loss during the study period, difficulty in timing of PK samples due to the critical clinical condition and a relatively low rate of informed parental consent (Baker et al., 2018). For this reason, many drugs are used off-label and enter the paediatric care protocols because clinicians perceive them to have a more useful spectrum of activity and/or better profile of tolerance than the currently used anti-infectives.
Population PK allows to determine PK parameters with a formal PK design with planned (pre-selected) sampling times, with opportunistic samples or a combination of planned and opportunistic samples (Leroux et al., 2015). In our review, it should be noted that, in most PopPK studies, sometimes retrospective and based on TDM, the number of patients was limited (lower than 100), and age range and malignant underlying disease were variable. Only a few studies performed a meta-analysis of data from different studies, as previously done in neonates (Jacqz-Aigrain et al., 2019) allowing to combine sufficient data to reach a larger number of patients, increase study power and identify covariates.
Most studies used a nonlinear mixed effects mathematical method, estimating PopPK parameters (CL and V) and their variability, based on the significant impact of covariates. Covariates in the context of paediatric malignancy include age, weight, organ maturation and function, but also other determinants such as underlying disease groups, comedications, and pharmacogenetics. Allometric scaling is an empirical examination of the relationships between the PK parameters and size (body weight). Allometric power parameters are often fixed at values of 0.75 for CL and 1 for distribution volume on the basis of physiologic consideration of size impact on metabolic processes (Anderson et al., 1997; Anderson and Holford, 2011). As shown here, the allometric coefficients need to be estimated in a limited number of cases (Johnson, 2008). The covariates renal function (reflected by creatinine or creatinine CL), hepatic function (reflected by ALT and ALKP) and pharmacogenetics were frequently tested. In the case of voriconazole, CYP2C19 genetic polymorphism, identified in adults affecting voriconazole disposition was not identified as a significant contributor to variability in children. As illustrated with this example, the role of pharmacogenetic biomarkers in variability may not be significant when the number of patients of deficient metabolizer genotypes is low, when PK overlap exists between the different genotypes and/or when genotype expression did not reach maturation (Lestner et al., 2016).
Once the PK model is developed, internal validation (using the same dataset) and external validation (requiring additional independent patients) are required. In most paediatric studies, validation was internal, predominantly based on goodness of fit plots and bootstrapping. In the studies that we analyzed, external validation was the exception, although it is more stringent.
Simulations of dosing regimens based on the validated model aim to inform optimal dosing in children that achieves target exposure comparable to that of adults. Of note, the adult PK/PD target thresholds do not take into account developmental aspects of immunocompetence; indeed, the immune system gradually changes during infancy to mature and expand during growth and to respond efficiently to acute infections (Anderson and Holford, 2011; Friberg et al., 2012). In addition to reduced immunocompetence due to incomplete immune maturation, the role of therapeutic immunosuppression would require to be explored. As illustrated in this review, different PK/PD targets may be used for dosing optimization of the same drug, with a lack of consensus regarding which target is optimal for this purpose. Efforts should be made to further explore this issue.
Before implementation of the new dosing regimen into the clinics, validation of exposure, safety, and tolerability in a carefully designed clinical trial will be needed. However, for most if not all studies, the clinical validation is not available, although response to anti-infectives is known to depend not only on drug exposure but also on age, associated therapies and type of disease.
In conclusion, many antibiotic and antifungal compounds are not approved for children or their optimal dosage is unknown, although differences in drug disposition may be anticipated in children compared to adults. We showed that PopPK data of the frequently prescribed glycopeptides and antifungals are very limited in children, although they are prescribed in most patients with hematologic malignancy. A few inform variability in disposition, identify significant impact of weight and additional covariates (organ function, disease subgroups) and led to dosage recommendations taking into account the identified variables. This review highlighted the lack of consensus regarding PK/PD targets used for dosing optimization, and regarding dosage recommendations when available. Additional PopPK and PK/PD studies are needed in this specific population of patients. In addition, clinical studies should be performed to prospectively validate the dosing regimens adapted to infection in paediatric patients with malignancy.
EJ-A initiated the review and identified the PopPK studies of interest, SL and EJ-A analysed the different PopPK studies and wrote the first version of the review, FM-H provided her expertise in treatment of paediatric malignancies, all three authors finalized the manuscript and agreed on the submitted version.
The authors declare that the research was conducted in the absence of any commercial or financial relationships that could be construed as a potential conflict of interest.
Amantea, M. A., Bowden, R. A., Forrest, A., Working, P. K., Newman, M. S., and Mamelok, R. D. (1995). Population pharmacokinetics and renal function-sparing effects of amphotericin B colloidal dispersion in patients receiving bone marrow transplants. Antimicrob. Agents Chemother. 39, 2042. doi:10.1128/aac.39.9.2042
Anderson, B. J., and Holford, N. H. (2011). Tips and traps analyzing pediatric PK data. Paediatr. Anaesth. 21, 222–2237. doi:10.1111/j.1460-9592.2011.03536.x
Anderson, B. J., McKee, A. D., and Holford, N. H. (1997). Size, myths and the clinical pharmacokinetics of analgesia in paediatric patients. Clin. Pharmacokinet. 33, 313–327. doi:10.2165/00003088-199733050-00001
Andes, D., Marchillo, K., Stamstad, T., and Conklin, R. (2003). In vivo pharmacokinetics and pharmacodynamics of a new triazole, voriconazole, in a murine candidiasis model. Antimicrob. Agents Chemother. 47, 3165. doi:10.1128/aac.47.4.1193-1199.2003
Andes, D. (2003). Minireview. In vivo pharmacodynamics of antifungal drugs in treatment of candidiasis. Antimicrob. Agents Chemother. 47, 1179–1186. doi:10.1128/aac.47.4.1179-1186.2003
Ayestarán, A., López, R. M., Montoro, J. B., Estíbalez, A., Pou, L., Julià, A., et al. (1996). Pharmacokinetics of conventional formulation versus fat emulsion formulation of amphotericin B in a group of patients with neutropenia. Antimicrob. Agents Chemother. 40, 609–612. doi:10.1128/AAC.40.3.609
Baker, C. I. S., Standing, J. F., Kelly, L. E., Faught, L. H., Needham, A. C., Rieder, M. J., et al. (2018). Pharmacokinetic studies in children: recommendations for practice and research. Arch. Dis. Child. 103, 695–702. doi:10.1136/archdischild-2017-314506
Boonsathorn, S., Cheng, I., Kloprogge, F., Alonso, C., Lee, C., Doncheva, B., et al. (2019). Clinical pharmacokinetics and dose recommendations for posaconazole in infants and children. Clin. Pharmacokinet. 58, 53–61. doi:10.1007/s40262-018-0658-1
Buelga, D. S., del Mar Fernandez de Gatta, M., Herrera, E. V., Dominguez-Gil, A., and García, M. J. (2005). Population pharmacokinetic analysis of vancomycin in patients with haematological malignancies. Antimicrob. Agents Chemother. 49, 4934–4941. doi:10.1128/AAC.49.12.4934-4941.2005
Carlesse, F. A. M. C., de Araujo, O. R., Marques, L. M. A., Silva, D. C. B. D., Senerchia, A. A., and Petrilli, A. S. (2019). A pharmacokinetic model for voriconazole in a highly diversified population of children and adolescents with cancer. Mycoses 62, 399–404. doi:10.1111/myc.12899
Chang, D. (1995). Influence of malignancy on the pharmacokinetics of vancomycin in infants and children. Pediatr. Infect. Dis. J. 14, 667–673. doi:10.1097/00006454-199508000-00004
Chen, J., Chan, C., Colantonio, D., and Seto, W. (2012). Therapeutic drug monitoring of voriconazole in children. Ther. Drug Monit. 34, 77–84. doi:10.1097/FTD.0b013e31823f3516
Chen, L., Brüggemann, R. J. M., Knibbe, C. A. J., and Krekels, E. H. J. (2019). Bioavailability and the variability of posaconazole exposure in healthy volunteers using a population pharmacokinetic analysis. Popul. Approach Group Eur. Available at: http://page-meeting.org/defau lt.asp?abstract=8958 (Accessed October 26, 2020).
Chen, L., Krekels, E. H. J., Verweij, P. E., Buil, J. B., Knibbe, C. A. J., and Brüggemann, R. J. M. (2020). Pharmacokinetics and pharmacodynamics of posaconazole. Drugs 80, 671–695. doi:10.1007/s40265-020-01306-y
Choonara, I. (2007). Regulation of drugs for children in Europe. BMJ 335, 1221–1222. doi:10.1136/bmj.39400.376424.BE
de Bie, S., Kaguelidou, F., Verhamme, K. M., De Ridder, M., Picelli, G., Straus, S. M., et al. (2016). Using prescription patterns in primary care to derive new quality indicators for childhood community antibiotic prescribing pediatr. Pediatr. Infect. Dis. J. 35, 1317–1323. doi:10.1097/INF.0000000000001324
Dolton, M. J., Brüggemann, R. J., Burger, D. M., and McLachlan, A. J. (2014). Understanding variability in posaconazole exposure using an integrated population pharmacokinetic analysis. Antimicrob. Agents Chemother. 58, 6879–6885. doi:10.1128/AAC.03777-14
Dufort, G., Ventura, C., Olivé, T., and Ortega, J. J. (1996). Teicoplanin pharmacokinetics in pediatric patients. Pediatr. Infect. Dis. J. 15, 494–498. doi:10.1097/00006454-199606000-00005
European Medicines Agency (2015). EMA/CHMP/594085/2015. Guideline on the use of pharmacokinetics and 5 pharmacodynamics in the development of antibacterial medicinal products Committee for Human Medicinal Products. Available at: http://file://s1hcifs01/DEMProfiles/18026/Downloads/W0317.pdf (Accessed July 21, 2016).
Finch, R. G., and Eliopoulos, G. M. (2005). Safety and efficacy of glycopeptide antibiotics. J. Antimicrob. Chemother. 55 (Suppl. 2), ii5–13. doi:10.1093/jac/dki004
Fisher, B. T., Robinson, P. D., Lehrnbecher, T., Steinbach, W. J., Zaoutis, T. E., Phillips, B., et al. (2018). Risk factors for invasive fungal disease in pediatric cancer and hematopoietic stem cell transplantation: a systematic review. J. Pediatr. Infect. Dis. Soc. 7, 191–198. doi:10.1093/jpids/pix030
Friberg, L. E., Ravva, P., Karlsson, M. O., and Liu, P. (2012). Integrated population pharmacokinetic analysis of voriconazole in children, adolescents, and adults. Antimicrob. Agents Chemother. 56, 3032–3042. doi:10.1128/AAC.05761-11
Gao, L., Xu, H., Ye, Q., Li, S., Wang, J., Mei, Y., et al. (2020). Population pharmacokinetics and dosage optimization of teicoplaninin children with different renal functions. Front. Pharmacol. 11, 552. doi:10.3389/fphar.2020.00552
Gastine, S., Lehrnbecher, T., Müller, C., Farowski, F., Bader, P., Ullmann-Moskovits, J., et al. (2017). Pharmacokinetic modeling of voriconazole to develop an alternative dosing regimen in children. Antimicrob. Agents Chemother. 62, e01194. doi:10.1128/AAC.01194-17
Gómez-López, A. (2020). Antifungal therapeutic drug monitoring: focus on drugs without a clear recommendation. Clin. Microbiol. Infect. 26 (11), 1481–1487. doi:10.1016/j.cmi.2020.05.037
Gore, R., Chugh, P. K., Tripathi, C. D., Lhamo, Y., and Gautam, S. (2017). Pediatric off-label and unlicensed drug use and its implications. Curr. Clin. Pharmacol. 12, 18–25. doi:10.2174/1574884712666170317161935
Groll, A. H., Castagnola, E., Cesaro, S., Dalle, J. H., Engelhard, D., Hope, W., et al. (2014). Fourth European conference on infections in leukaemia (ECIL-4): guidelines for diagnosis, prevention, and treatment of invasive fungal diseases in paediatric patients with cancer or allogeneic haemopoietic stem-cell transplantation. Lancet Oncol. 15, e327–e340. doi:10.1016/S1470-2045(14)70017-8
Guilhaumou, R., Marsot, A., Dupouey, J., Galambrun, C., Boulamery, A., Coze, C., et al. (2016). Pediatric patients with solid or hematological tumor disease: vancomycin population pharmacokinetics and dosage optimization. Ther. Drug Monit. 38, 559–566. doi:10.1097/FTD.0000000000000318
Hadi, O. A., Al Omar, S., and NazerS Le, L. H. M. J. (2016). Vancomycin pharmacokinetics and predicted dosage requirements in pediatric cancer patients. J. Oncol. Pharm. 22, 448–453. doi:10.1177/1078155215591386
Hahn, A., Frenck, R. W., Zou, Y., and Vinks, A. A. (2015). Validation of a pediatric population pharmacokinetic model for vancomycin. Ther. Drug Monit. 37, 413–416. doi:10.1097/FTD.0000000000000153
Hong, Y., Shaw, P. J., Nath, C. E., Yadav, S. P., Stephen, K. R., Earl, J. W., et al. (2006). Population pharmacokinetics of liposomal amphotericin B in pediatric patients with malignant diseases. Antimicrob. Agents Chemother. 50, 935–942. doi:10.1128/AAC.50.3.935-942.2006
Jacqz-Aigrain, E., Leroux, S., Thomson, A. H., Allegaert, K., Capparelli, E. V., Biran, V., et al. (2019). Population pharmacokinetic meta-analysis of individual data to design the first randomized efficacy trial of vancomycin in neonates and young infants. J. Antimicrob. Chemother. 74, 2128–2138. doi:10.1093/jac/dkz158
Janoff, A. S. (1990). Liposomes and lipid structures as carriers of amphotericin B. Eur. J. Clin. Microbiol. Infect. Dis. 9, 146–215.
Jarkowski, A., Forrest, A., Sweeney, R. P., Tan, W., Segal, B. H., Almyroudis, N., et al. (2012). Characterization of vancomycin pharmacokinetics in the adult acute myeloid leukemia population. J. Oncol. Pharm. Pract. 18, 91–96. doi:10.1177/1078155211402107
Johnson, T. N. (2008). The problems in scaling adult drug doses to children. Arch. Dis. Child. 93, 207–211. doi:10.1136/adc.2006.114835
Jong, G. W., Vulto, A. G., de Hoog, M., Schimmel, K. J., Tibboel, D., and van den Anker, J. N. (2001) A survey of the use of off-label and unlicensed drugs in a Dutch children’s hospital, Pediatrics 108, 1089–1093. doi:10.1542/peds.108.5.1089
Karlsson, M. O., Lutsar, I., and Milligan, P. A. (2009). Population pharmacokinetic analysis of voriconazole plasma concentration data from pediatric studies. Antimicrob. Agents Chemother. 53, 935–944. doi:10.1128/AAC.00751-08
Kearns, G. L., Abdel-Rahman, S. M., Alander, S. W., Blowey, D. L., Leeder, J. S., and Kauffman, R. E. (2003). Developmental pharmacology–drug disposition, action, and therapy in infants and children. N. Engl. J. Med. 349, 1157–1167. doi:10.1056/NEJMra035092
Keita, H., Setsuko, I., Ayumi, K., Ikegaya, K., Suzuki, M., Hirano, K., et al. (2016). Augmented renal clearance in pediatric patients with febrile neutropenia associated with vancomycin clearance. Ther. Drug Monit. 38, 393–397. doi:10.1097/FTD.0000000000000270
Kohno, S., Kobayashi, H., Masaoka, T., Urabe, A., Kanamaru, A., Niki, Y., et al. (2013). Evaluation of pharmacokinetics and safety of liposomal amphotericin B (L-AMB) in patients with deep-seated fungal infection. Jpn. J. Chemother. 61 (4), 369–379.
Krivoy, N., Peleg, S., Postovsky, S., and Ben Arush, M. W. (1998). Pharmacokinetic analysis of vancomycin in steady state in pediatric cancer patients. Pediatr. Hematol. Oncol. 15, 333. doi:10.3109/08880019809014017
Lamarre, P., Lebel, D., and Ducharme, M. P. (2000). A population pharmacokinetic model for vancomycin in pediatric patients and its predictive value in a naive population antimicrobial agents. Antimicrob. Agents Chemother. 44, 278–282. doi:10.1128/aac.44.2.278-282.2000
Lehrnbecher, T., Ethier, M. C., Zaoutis, T., Creutzig, U., Gamis, A., Reinhardt, D., et al. (2009). International variations in infection supportive care practices for paediatric patients with acute myeloid leukaemia. Br. J. Haematol. 147, 125–128. doi:10.1111/j.1365-2141.2009.07844.x
Lehrnbecher, T., Robinson, P., Fisher, B., Alexander, S., Ammann, R. A., Beauchemin, M., et al. (2017). International pediatric fever and neutropenia guideline panel. Guideline for the management of fever and neutropenia in children with cancer and hematopoietic stem-cell transplantation recipients. J. Clin. Oncol. 35, 2082–2094. doi:10.1200/JCO.2016.71.7017
Leroux, S., Turner, M. A., Guellec, C. B., Hill, H., van den Anker, J. N., Kearns, G. L., et al. (2015). TINN (treat infections in NeoNates) and GRiP (global research in paediatrics) consortiums. Pharmacokinetic studies in neonates: the utility of an opportunistic sampling design. Clin. Pharmacokinet. 54, 1273–1285. doi:10.1007/s40262-015-0291-1
Lestner, J. M., Groll, A. H., Aljayyoussi, G., Seibel, N. L., Shad, A., Gonzalez, C., et al. (2016). Population pharmacokinetics of liposomal amphotericin B in immunocompromised children. Antimicrob. Agents Chemother. 60, 7340–7346. doi:10.1128/AAC.01427-16
Libuit, J., Whitman, A., Wolfe, R., and Washington, C. S. (2014). Empiric vancomycin use in febrile neutropenic oncology patients. Open Forum Infect. Dis. 1, ofu006. doi:10.1093/ofid/ofu006
Liu, Y., Qiu, T., Liu, Y., Wang, J., Hu, K., Bao, F., et al. (2019). Model-based voriconazole dose optimization in Chinese adult patients with hematologic malignancies. Clin. Ther. 41, 1151–1163. doi:10.1016/j.clinthera.2019.04.027
Lortholary, O., Tod, M., Rizzo, N., Padoin, C., Biard, O., Casassus, P., et al. (1996). Population pharmacokinetic study of teicoplanin in severely neutropenic patients. Antimicrob. Agents Chemother. 40, 1242–1247. doi:10.1128/AAC.40.5.1242
Lukas, J. C., Karikas, G., Gazouli, M., Kalabalikis, P., Hatzis, T., and Macheras, P. (2004). Pharmacokinetics of teicoplanin in an ICU population of children and infants. Pharm. Res. 21, 2064–2071. doi:10.1023/b:pham.0000048198.56873.d8
Marsot, A., Boulamery, A., Bruguerolle, B., and Simon, N. (2012). Vancomycin: a review of population pharmacokinetic analyses. Clin. Pharmacokinet. 51, 1–13. doi:10.2165/11596390-000000000-00000
Marsot, A., Gallais, F., Galambrun, C., Coze, C., Blin, O., Andre, N., et al. (2018). Vancomycin in pediatric patients with solid or hematological malignant disease: predictive performance of a population pharmacokinetic model and new optimized dosing regimens. Pediatr. Drugs 20, 375–381. doi:10.1007/s40272-018-0295-z
Menichetti, F., Martino, P., Bucaneve, G., Gentile, G., D'Antonio, D., Liso, V., et al. (1994). Effects of teicoplanin and those of vancomycin in initial empirical antibiotic regimen for febrile, neutropenic patients with hematologic malignancies. Antimicrob. Agents Chemother. 38, 2041–2046. doi:10.1128/aac.38.9.2041
Moise-Broder, P. A., Forrest, A., Birmingham, M. C., and Schentag, J. J. (2004). Pharmacodynamics of vancomycin and other antimicrobials in patients with Staphylococcus aureus lower respiratory tract infections. Clin. Pharmacokinet. 43, 925–942. doi:10.2165/00003088-200443130-00005
Moreau, P., Milpied, N., Fayette, N., Ramée, J. F., and Harousseau, J. L. (1992). Reduced renal toxicity and improved clinical tolerance of amphotericin B mixed Intralipid compared with conventional amphotericin B in neutropenic patients. J. Antimicrob. Chemother. 30, 535–541. doi:10.1093/jac/30.4.535
Muto, C., Shoji, S., Tomono, Y., and Liu, P. (2015). Population pharmacokinetic analysis of voriconazole from a pharmacokinetic study with immunocompromised Japanese pediatric subjects. Antimicrob. Agents Chemother. 59, 3216–3223. doi:10.1128/AAC.04993-14
Nath, C. E., McLachlan, A. J., Shaw, P. J., Coakley, J. C., and Earl, J. W. (2007). Amphotericin B dose optimization in children with malignant diseases. Chemotherapy 53, 142–147. doi:10.1159/000100013
Nath, C. E., McLachlan, A. J., Shaw, P. J., Gunning, R., and Earl, J. W. (2001). Population pharmacokinetics of amphotericin B in children with malignant diseases. Br. J. Clin. Pharmacol. 52, 671–680. doi:10.1046/j.0306-5251.2001.01496.x
Ohata, Y., Tomita, Y., Suzuki, K., Maniwa, T., Yano, Y., and Sunakawa, K. (2015). Pharmacokinetic evaluation of liposomal amphotericin B (L-AMB) in patients with invasive fungal infection: population approach in Japanese pediatrics. Drug Metab. Pharmacokinet. 30, 400–409. doi:10.1016/j.dmpk.2015.08.003
Pascual, A., Calandra, T., Bolay, S., Buclin, T., Bille, J., and Marchetti, O. (2008). Voriconazole therapeutic drug monitoring in patients with invasive mycoses improves efficacy and safety outcomes. Clin. Infect. Dis. 46, 201–211. doi:10.1086/524669
Petitcollin, A., Boglione-Kerrien, C., Tron, C., Nimubona, S., Lalanne, S., Lemaitre, F., et al. (2017). Population pharmacokinetics of posaconazole tablets and Monte Carlo simulations to determine whether all patients should receive the same dose. Antimicrob. Agents Chemother. 61, e01166–17. doi:10.1128/AAC.01166-17
Pieper, S., Kolve, H., Gumbinger, H. G., Goletz, G., Würthwein, G., and Groll, A. H. (2012). Monitoring of voriconazole plasma concentrations in immunocompromised paediatric patients. J. Antimicrob. Chemother. 67, 2717–2724. doi:10.1093/jac/dks258
Ramos-Martín, V., Paulus, S., Siner, S., Scott, E., Padmore, K., Newland, P., et al. (2014). Population pharmacokinetics of teicoplanin in children. Antimicrob. Agents Chemother. 58, 6920–6927. doi:10.1128/AAC.03685-14
Reed, M. D., Yamashita, T. S., Myers, C. M., and Blumer, J. L. (1997). The pharmacokinetics of teicoplanin in infants and children. J. Antimicrob. Chemother. 39, 789–796. doi:10.1093/jac/39.6.789
Ruggieri, L., Giannuzzi, V., Baiardi, P., Bonifazi, F., Davies, E. H., Giaquinto, C., et al. (2015). Successful private-public funding of paediatric medicines research: lessons from the EU programme to fund research into off-patent medicines. Eur. J. Pediatr. 174, 481–491. doi:10.1007/s00431-014-2398-z
Sánchez, A., López-Herce, J., Cueto, E., Carrillo, A., and Moral, R. (1999). Teicoplanin pharmacokinetics in critically ill paediatric patients. J. Antimicrob. Chemother. 44, 407–409. doi:10.1093/jac/44.3.407
Seibel, N. L., Shad, A. T., Bekersky, I., Groll, A. H., Gonzalez, C., Wood, L. V., et al. (2017). Safety, tolerability, and pharmacokinetics of liposomal amphotericin B in immunocompromised pediatric patients. Antimicrob. Agents Chemother. 61, e01477–16. doi:10.1128/AAC.01477-16
Seixas, G. T., Araujo, O. R., Silva, D. C., Arduini, R. G., Petrilli, A. S., et al. (2016). Vancomycin therapeutic targets and nephrotoxicity in critically ill children with cancer. J. Pediatr. Hematol. Oncol. 38, e56–e62. doi:10.1097/MPH.0000000000000470
Shi, C., Xiao, Y., Mao, Y., Wu, J., and Lin, N. (2019). Voriconazole: a review of population pharmacokinetic analyses. Clin. Pharmacokinet. 58 (6), 687–703. doi:10.1007/s40262-019-00735-7
Soy, D., López, E., and Ribas, J. (2006). Teicoplanin population pharmacokinetic analysis in hospitalized patients. Ther. Drug Monit. 28, 737–743. doi:10.1097/01.ftd.0000249942.14145.ff
Strenger, V., Hofer, N., Rödl, S., Hönigl, M., Raggam, R., Seidel, M. G., et al. (2013). Age and gender-related differences in teicoplanin levels in paediatric patients. J. Antimicrob. Chemother. 68, 2318–2323. doi:10.1093/jac/dkt176
Tarral, E., Jehl, F., Tarral, A., Simeoni, U., Monteil, H., Willard, D., et al. (1988). Pharmacokinetics of teicoplanin in children. J. Antimicrob. Chemother. 21 (Suppl. A), 47–51. doi:10.1093/jac/21.suppl_a.47
Terragna, A., Ferrea, G., Loy, A., Danese, A., Bernareggi, A., Cavenaghi, L., et al. (1988). Pharmacokinetics of teicoplanin in pediatric patients. Antimicrob. Agents Chemother. 32, 1223–1226. doi:10.1128/aac.32.8.1223
Tkachuk, S., Collins, K., and Ensom, M. H. H. (2018). The relationship between vancomycin trough concentrations and AUC/MIC ratios in pediatric patients: a qualitative systematic review. Paediatr. Drugs 20, 153–164. doi:10.1007/s40272-018-0282-4
Turner, M. A., Catapano, M., Hirschfeld, S., and Giaquinto, C. (2014). Global Research in Paediatrics. Paediatric drug development: the impact of evolving regulations. Adv. Drug Deliv. Rev. 73, 2–13. doi:10.1016/j.addr.2014.02.003
Ullmann, A. J., Lipton, J. H., Vesole, D. H., Chandrasekar, P., Langston, A., Tarantolo, S. R., et al. (2007). Posaconazole or fluconazole for prophylaxis in severe graft-versus-host disease. N. Engl. J. Med. 356, 335–347. doi:10.1056/NEJMoa061098
van Iersel, M. L. P. S., Rossenu, S., de Greef, R., and Waskin, H. (2018). A population pharmacokinetic model for a solid oral tablet formulation of posaconazole. Antimicrob. Agents Chemother. 62, e02465–17. doi:10.1128/AAC.02465-17
Vehreschild, J. J., Müller, C., Farowski, F., Vehreschild, M. J., Cornely, O. A., Fuhr, U., et al. (2012). Factors influencing the pharmacokinetics of prophylactic posaconazole oral suspension in patients with acute myeloid leukemia or myeloblastic syndrome. Eur. J. Clin. Pharmacol. 68, 987–995. doi:10.1007/s00228-012-1212-y
Walsh, T. J., Driscoll, T., Milligan, P. A., Wood, N. D., Schlamm, H., Groll, A. H., et al. (2010). Pharmacokinetics, safety, and tolerability of voriconazole in immunocompromised children. Antimicrob. Agents Chemother. 54, 4116–4123. doi:10.1128/AAC.00896-10
Walsh, T. J., Finberg, R. W., Arndt, C., Hiemenz, J., Schwartz, C., Bodensteiner, D., et al. (1999). Liposomal amphotericin B for empirical therapy in patients with persistent fever and neutropenia. National institute of allergy and infectious diseases mycoses study group. N. Engl. J. Med. 340, 764–771. doi:10.1056/NEJM199903113401004
Walsh, T. J., Karlsson, M. O., Driscoll, T., Arguedas, A. G., Adamson, P., Saez-Llorens, X., et al. (2004). Pharmacokinetics and safety of intravenous voriconazole in children after single- or multiple- dose administration. Antimicrob. Agents Chemother. 48, 2166–2172. doi:10.1128/AAC.48.6.2166-2172.2004
Wrishko, R. E., Levine, M., Khoo, D., Abbott, P., and Hamilton, D. (2000). Vancomycin pharmacokinetics and Bayesian estimation in pediatric patients. Ther. Drug Monit. 22, 522–531. doi:10.1097/00007691-200010000-00004
Yan, S. Q., Seyboth, B., Kobos, R., Eaton, A., Seo, S. K., and Cohen, N. (2018). Voriconazole dosing in children younger than 3 yundergoing cancer chemotherapy or hematopoietic stem cell transplantation. J. Pediatr. Infect. Dis. Soc. 7, 169–171. doi:10.1093/jpids/pix022
Yasuhara, M., Iga, T., Zenda, H., Okumura, K., Oguma, T., Yano, Y., et al. (1998). Population pharmacokinetics of vancomycin in Japanese adult patients. Ther. Drug Monit. 20, 139–148. doi:10.1097/00007691-199804000-00003
Yu, D. K., Nordbrock, E., Hutcheson, S. J., Lewis, E. W., Sullivan, W., Bhargava, V. O., et al. (1995). Population pharmacokinetics of teicoplanin in patients with endocarditis. J. Pharmacokinet. Biopharm. 23, 25–39. doi:10.1007/BF02353784
Zhao, W., Zhang, D., Fakhoury, M., Fahd, M., Duquesne, F., Storme, T., et al. (2014). Population pharmacokinetics and dosing optimization of vancomycin in children with malignant hematological disease. Antimicrob. Agents Chemother. 58, 3191–3199. doi:10.1128/AAC.02564-13
Keywords: paediatrics, malignancy, onco-hematology, glycopeptides, antifungals, population pharmacokinetics, drug dosage
Citation: Leroux S, Mechinaud-Heloury F and Jacqz-Aigrain E (2021) Contribution of Population Pharmacokinetics of Glycopeptides and Antifungals to Dosage Adaptation in Paediatric Onco-hematological Malignancies: A Review. Front. Pharmacol. 12:635345. doi: 10.3389/fphar.2021.635345
Received: 30 November 2020; Accepted: 08 February 2021;
Published: 01 April 2021.
Edited by:
Wei Zhao, Shandong University, ChinaReviewed by:
Clinton F. Stewart, St. Jude Children's Research Hospital, United StatesCopyright © 2021 Leroux, Mechinaud-Heloury and Jacqz-Aigrain This is an open-access article distributed under the terms of the Creative Commons Attribution License (CC BY). The use, distribution or reproduction in other forums is permitted, provided the original author(s) and the copyright owner(s) are credited and that the original publication in this journal is cited, in accordance with accepted academic practice. No use, distribution or reproduction is permitted which does not comply with these terms.
*Correspondence: Evelyne Jacqz-Aigrain, Evelyne.jacqz-aigrain@aphp.fr
†Present address: Professeur de PharmacologieHôpital Saint-Louis, Pharmacologie biologique et Pharmacogénétique Hôpital Robert Debré, Paris, France
Disclaimer: All claims expressed in this article are solely those of the authors and do not necessarily represent those of their affiliated organizations, or those of the publisher, the editors and the reviewers. Any product that may be evaluated in this article or claim that may be made by its manufacturer is not guaranteed or endorsed by the publisher.
Research integrity at Frontiers
Learn more about the work of our research integrity team to safeguard the quality of each article we publish.