- 1Programa de Pós-graduação em Produtos Naturais e Sintéticos Bioativos, Universidade Federal da Paraíba, João Pessoa, Brazil
- 2Curso de Farmácia, Universidade Federal da Paraíba, João Pessoa, Brazil
- 3Departamento de Química, Universidade Federal Rural de Pernambuco, Recife, Brazil
- 4Departamento de Zoologia, Universidade Federal de Pernambuco, Recife, Brazil
- 5Departmento de Ciências Farmacêuticas, Universidade Federal da Paraíba, João Pessoa, Brazil
- 6Departamento de Fisiologia e Patologia, Universidade Federal da Paraíba, João Pessoa, Brazil
Oceanapia magna Santos-Neto, Nascimento, Cavalcanti and Pinheiro sponges are distributed across tropical worldwide seas. Some studies of marine products have shown interesting activities in smooth muscle models. Hence, we assessed the effect of the ethanolic extract of Oceanapia magna. (OC-EtOH) on acute toxicity and gastrointestinal motility (in vitro and in vivo) in rodent models. On guinea pig ileum, OC-EtOH induced a concentration dependent contraction on basal tonus, which was not inhibited by atropine, but in the presence of pyrilamine or verapamil, the effect was antagonized. Contrastingly, on KCl- or histamine-induced contractions, OC-EtOH presented a transient contraction followed by a concentration-dependent relaxation. Moreover, OC-EtOH presented a relaxant profile on cumulative curves to CaCl2 and tonic contraction induced by S-(-)-BayK8644, through Cav blockade. The acute toxicity assay showed that OC-EtOH (2,000 mg/kg, p.o.) did not present any sign of toxicity in female mice. Additionally, OC-EtOH presented antidiarrheal effect in mice, increased the intestinal normal transit and reduced the castor oil-induced intestinal transit. Thus, OC-EtOH presented a dual effect on guinea pig ileum promoting contraction through activation of H1 and CaV, and relaxation through CaV blockade, besides the effect on upper gastrointestinal transit in mice, showing a potential medicinal use of this sponge in intestinal diseases such as diarrhea.
Introduction
Natural products are considered a natural library of combinatorial chemistry that can provide substances with chemical and pharmacological diversity (Wang et al., 2011). The vast range of products available in the nature can be considered an important source of substances with potential therapeutic interest (Butler et al., 2014). Among the natural products diversity, several researchers are interested in studying natural marine products due to the varied production of compounds that may be used to treat various diseases (Movahhedin et al., 2014).
Marine natural products are sources of compounds that have demonstrated a plethora of biological activities, in both pre-clinical and clinical research, such as antifungal (D’auria et al., 2007), anticoagulant (Jung et al., 2007), antibacterial (Horie et al., 2008), anti-inflammatory (Chao et al., 2008), immunomodulatory (Yamada et al., 2007; Courtois et al., 2008), antiviral (Artan et al., 2008) and anticancer (Malyarenko et al., 2018; Rath et al., 2018). Additionally, spasmolytic activity has been described for the brown alga Dictyota pulchella (Queiroz et al., 2011), the green alga Hydrodictyon reticulatum (Gutierrez et al., 2012), and caulerpina, an alkaloid isolated from algae of Caulerpa genus (Cavalcante-Silva et al., 2013; Cavalcante-Silva et al., 2016).
Oceanapia genus (Oceanapiidae) presents about 100 sponges species distributed across the world’s tropical seas (Van Soest et al., 2015). Studies on Oceanapia genus are scarce in literature and only few biological activities have been described. Oceanapia species produce different classes of metabolites, including alkaloids, sphingolipids, steroids, acetylene, thiocyanate, among others (Ibrahim et al., 2013) that showed antifungal effect (Nicholas et al., 1999) and anticancer activity (Kijjoa et al., 2007).
Oceanapia magna Santos-Neto, Nascimento, Cavalcanti and Pinheiro is a recently described endemic species in Brazil. The word magna is an adjective derived from Latin and refers to the large size of this specimens (Santos-Neto et al., 2018).
There is considerable interest in investigating drugs obtained directly from natural products or their derivatives that act on the smooth muscle, once this type of muscle is present in the walls of various body organs, including blood vessels, stomach, bladder, airways and intestine. The regulation/deregulation of smooth muscle contractility plays a role in many pathophysiological processes such as hypertension, asthma, erectile dysfunction, renal and uterine cramping, diarrhea, and constipation (Webb, 2003).
Diarrhea is a process characterized by the increase of defecation frequency (more than 3 times in 24 h period), which may be watery or semi-liquid (World Health Organization, 2016). This disorder causes thousands of deaths per year, mainly in children under 5 years of age, which implies in a high cost for healthcare systems (Ndikubwimana and Ngendahimana, 2020). On the other hand, constipation is a condition that the individual presents less than three defecation episodes per week or evacuates hard, dry, and small stools, in a painful and difficult process to eliminate feces (Bielefeldt et al., 2016). This condition represents a significant economic burden. In the United States, the medical costs associated with constipation were estimated at United States $235 million in 2001, and about 95.3% of this costs were applied in the outpatient care (Nag et al., 2020). Therefore, there is an unexplored field that stimulates researchers to study natural products as tools to obtain new treatments and/or preventions to gastrointestinal diseases (Guo et al., 2014). Moreover, as described before, there is evidence that marine natural products present action on intestinal smooth muscle, what could represent an important contribution for human health, since marine products are known as a rich secondary metabolites source. Based on this, we decided to investigate a possible intestinal smooth muscle tone and/or antidiarrheal activities of the ethanolic extract from Oceanapia magna (OC-EtOH).
Materials and Methods
Product-Test
The ethanolic extract obtained from Oceanapia magna sponge (OC-EtOH) was used in this study. The sponge was collected by Petrobras (Petróleo of Brazil S/A), in May 2011, in Potiguar Basin/Rio Grande do Norte (04° 44.8945′ S/036° 25.4571′ W), with a 108 m depth, as part of Project: Inter-institutional Network Benthic Algae and INCT in Tropical Marine Environments - AmbTropic (CNPq No. 610013/2011-4) and identified by Professor PhD. Ulisses dos Santos Pinheiro, Federal University of Pernambuco, with the number of fall UFPEPOR 1551. After collected, the material was washed in running water and, then, separated. After, the sponge specimens were lyophilized and weighed. Subsequently, about 2 kg of lyophilized material was triturated and exhaustively extracted with ethanol and vacuum filtered with filter paper into a celite pad on Buchner funnel. The extraction solution was dried using a rotaevaporator to yield 10 g of material, providing a yield of 0.5%.
HPLC Analysis
The Oceanapia extract was extracted in a solid phase extraction cartridge (SPE C-18), which was previously activated using 10 ml of methanol and 10 ml of ultrapure water. Approximately 128.0 mg of the extract was solubilized with 50 µL of MeOH and H2O (1:1) and added to the cartridge, washed with ultrapure water (10 ml). The retained material was eluted with methanol (10 ml) and the solvent was evaporated to obtain 13.9 mg of the methanolic fraction. This fraction was solubilized in MeOH (3.5 mg/ml) for analysis by HPLC-ELSD and quantification of β-sitosterol and stigmasterol.
Quantification of β-sitosterol and stigmasterol was performed using equipment from Shimadzu Prominence LC-20AT with an Evaporative Light Scattering detector–ELSD (Alltech Associates, United States), automatic injector SIL-20AC, oven CTO-20a and degasser DGU-20A5. The chromatographic separation was performed with a column Luna C-18 (150 mm × 4.6 mm × 5 µm, Phenomenex). Methanol (HPLC grade) was used as mobile phase with flow of 1.0 ml/min, column temperature 40 °C, drift tube temperature of 70°C and a nitrogen flow at 2 ml/min. For filtering samples, 0.45 μm nylon filters were used (Whatman).
Animals
For the experimental protocols, 168 Swiss mice (Mus musculus) weighing 31.8 ± 0.4 g and 63 guinea pigs (Cavia porcellus) weighing 370.5 ± 6.1 g, of both sexes, were used. All animals were housed at “Professor Thomas George” Bioterium of the Instituto de Pesquisa em Fármacos e Medicamentos (IPeFarM)/UFPB. The animals were kept in a 12-h light-dark cycle, with monitored temperature (21 ± 1°C), and unrestricted access to food and water. During in vivo experiments, researchers knew about the distribution of animals in the experimental groups. All the experimental procedures were formerly authorized by the Ethics Committee on Animal Use (CEUA)/UFPB, certificate no. 146/2015.
Solutions and Drugs
Magnesium sulfate (MgSO4), potassium chloride (KCl), calcium chloride (CaCl2), glucose, sodium bicarbonate (NaHCO3), sodium chloride (NaCl), and sodium dihydrogen phosphate (NaH2PO4) were purchased from Vetec Química Fina Ltda. (Brazil). Atropine (99%), (S-(−)-Bay K8644), chloride verapamil, pyrilamine, carbamylcholine hydrochloride (CCh), Cremophor®, and histamine were obtained from Sigma-Aldrich (Brazil). Carboxymethylcellulose was obtained from Formula (Brazil). Castor oil was obtained from Farmax (Brazil). Loperamide (99%) was obtained from Janssen Cilag Farmacêutica Ltd. (Brazil) and activated charcoal was obtained from Proquímicos (Brazil). All substances were diluted in distilled water, and the extract was solubilized in Cremophor® (3%), whose concentration never exceeded 0.01% (v/v). At this concentration, this chemical is devoid of contractile or relaxant effects on the studied organ, according to previous obtained data (data not shown).
Measurement of Contractile Response
For isotonic contractions recording, segments of guinea pig ileum were suspended longitudinally by cotton yarn in organ baths (5 ml) and connected to an isotonic lever coupled to a smoked-drum kymograph (DTF, Brazil). Isometric transducers (TIM 05) coupled to an amplifier (AECAD04F) and connected to digital acquisition system AQCAD 2.1.6 from AVS Projetos (São Paulo, SP, Brazil) were used to record isometric contractions. To verify if the spasmolytic effect of OC-EtOH was reversible, the organ preparation was washed with the physiological solution and, after 30 min, a new contraction was induced in order to observe if organ responsiveness was altered (data not shown). In the cumulative concentration-response curves a new concentration of standard drugs or extract were only added when a plateau of the previous concentration was obtained.
Pharmacological Experiments
Effect of OC-EtOH on Basal Tonus of Guinea Pig Ileum
The animals were fasted for 18 h before the euthanasia and subjected to decapitation using a guillotine. The abdomen was opened and an ileum segment of approximately 15 cm in length was removed and placed in a Petri dish containing a modified Krebs nutrient solution at 37°C, at a pH of 7.4, and gassed with carbogen (95% O2 and 5% CO2). Segments of this organ measuring approximately 2 to 3 cm were suspended in organ baths (5 ml). During the stabilization period (30 min) the organs were washed every 15 min by modified Krebs solution (mM): NaCl (117.0), KCl (4.7), MgSO4 (1.3), NaH2PO4 (1.2), CaCl2 (2.5), NaHCO3 (25.0) and glucose (11.0) at 37 °C and bubbled with a carbogen mixture in a resting tension of 1 g. After stabilization, an isotonic contraction with 40 mM KCl was induced to verify organ functionality. The preparations were washed just before adding OC-EtOH. Then, OC-EtOH was added and its effect on basal intestinal muscle tonus observed. A cumulative concentration-response curve to OC-EtOH was induced. The results were evaluated by contractile amplitude percentage response of OC-EtOH. The values of extract concentrations able to produce 50% of maximal effect (EC50) were calculated by non-linear regression.
Effect of OC-EtOH on KCl, Carbachol or Histamine-Induced Tonic Contractions
To evaluate the relaxing effect of the extract against different contracting agents, guinea pig ileum was obtained as described above. After stabilization period, 40 mM KCl, 10–6 M histamine or 10–5 M CCh was added to promote an isometric contraction in different preparations. To obtain the contraction plateau, the preparation continued in the presence of the contractile substances (approximately 10 min) then the organ was washed. After 30 min elapsed, a new contraction was performed with the same contractile agents as before to add OC-EtOH cumulatively (0.1–2,187 μg/ml). Relaxation was expressed as the reverse percentage of initial contraction elicited by the contractile agents. EC50 was obtained graphically from the concentration-response curves.
Evaluation of Muscarinic and Histaminergic Receptors and CaV Participation in Spasmogenic Effect of OC-EtOH
After the stabilization period, isotonic contractions were induced with 40 mM KCl to check the organ’s functionality and, after 15 min, two cumulative concentration-response curves of amplitudes similar to histamine, CCh (positive controls) or OC-EtOH were induced. Then, in the absence of the contractile agents or the extract, atropine (muscarinic antagonist, 10–9 and 3 × 10–8 M), pyrilamine (H1 receptor antagonist, 10−9–3 × 10–7 M) or verapamil (CaV blocker, 10−7–10–6 M) was incubated for 15 min, in independent experiments (Ghayur and Gilani, 2005). After this period, a new cumulative concentration-response curve for CCh, histamine or OC-EtOH was obtained in the presence of atropine, pyrilamine or verapamil, in order to evaluate the participation of the muscarinic and histaminergic receptors, as well as th CaV.
The results were evaluated by comparing the percentage of contractile response in presence and absence of antagonists and blocker. The inhibitory effect was evaluated based on the analysis of EC50 values and Emax of OC-EtOH, which were calculated from the concentration-response curves obtained as described above.
Effect of OC-EtOH on KCl-Induced Tonic Contraction in Presence of Pyrilamine
Guinea pig ileum was obtained as described. After the stabilization, the preparation was washed and, after 15 min, pyrilamine was pre-incubated for 30 min to observe if the spasmogenic component of extract relaxation was removed. Then, an isometric contraction was induced with 40 mM KCl and, during sustained tonic phase (8–10 min), OC-EtOH was added cumulatively (0.1–729 μg/ml). The relaxation produced by OC-EtOH was expressed as percentage contraction reverse produced by contractile agent. The EC50 values were calculated by nonlinear regression.
Effect of OC-EtOH on CaCl2-Induced Contractions in Despolarizing Medium Nominally Without Ca2+
After the stabilization period, a nominally Ca2+-free depolarization solution (with 70 mM KCl in equimolar exchange for NaCl) was used to swap the modified Krebs solution, for a period of 45 min, to obtain an isotonic contraction. Then, CaCl2 was added cumulatively to obtain a concentration-response curve, this step was performed in duplicate. Subsequently, OC-EtOH was pre-incubated at different concentrations and a new contraction curve was performed in the presence of CaCl2 (Van Rossum, 1963) to assess the involvement of CaV in the relaxing effect of the extract. The maximal contraction obtained with the first CaCl2 concentration-response curve was considered to be 100% (control), and all contractions were assessed in reference to it.
Effect of OC-EtOH on S-(-)-Bay K8644-Induced Tonic Contractions
To determine the CaV subtype involved in the extract relaxation activity, guinea pig ileum was obtained as described above. After stabilization period, pyrilamine was added for 30 min, to remove the spasmogenic component of the extract, then, 15 mM KCl was added for 10 min to promote partial depolarization of the ileum (Conte-Camerino et al., 1987). Subsequently, S-(-)-Bay K8644 3 × 10–7 M, a selective CaV1 agonist, induced a isometric contraction. During tonic phase of contraction, verapamil (positive control) or OC-EtOH (0.1–729 μg/ml) were added cumulatively. The relaxation was expressed as percentage of initial contraction reversal produced by S-(-)-Bay K8644 and EC50 value was calculated by nonlinear regression.
Behavioral Pharmacological Screening and Evaluation of Acute Toxicity of OC-EtOH in Mice
For evaluation of acute toxicity, we followed the methodology described by Organization for Economic Co-operation and Development (OECD) no. 423/2001 (OECD, 2001). Initially, three female mice were used, which, after fasting for 4 h, were treated with OC-EtOH (single dose of 2,000 mg/kg, p.o.) or saline +0.9% Cremophor® (control, p.o.). Animals were also evaluated for 14 days for survival analysis purposes, in order to estimate the extract dose that kills 50% of animals tested (LD50).
After administration of OC-EtOH, a series of behavioral parameters were observed during first 4 h, such as hyperactivity, aggression, tremor, convulsion, piloerection, sedation, ataxia, catatonia, analgesia, loss of corneal and headset reflex, dyspnoea, ambulation, scaling, abdominal contractions, bleeding, self-mutilation and nausea-like behaviour, such as pica (adapted from Almeida et al., 1999; Batra and Schrott 2011). The ponderal evolution was also measured before treatment, 7 and 14 days after treatment. This protocol was performed twice, in different experiments.
Effect of OC-EtOH on Upper Gastrointestinal Transit in Mice
After 12 h of food restriction, 0.9% NaCl plus Cremophor® (10 ml/kg, negative control), atropine (2 mg/kg, positive control) or OC-EtOH (31.25; 62.5; 125, 250, 500 and 1,000 mg/kg) were administrated orally in male and female mice separated into three groups (n = 6, each). After 30 min, 5% activated charcoal solubilized in carboxymethylcellulose 0.5% (0.01 ml/g) was administered orally. After 30 min of administration of the marker (activated charcoal), the animals were euthanized by decapitation using guillotine, and an incision was made in the animal’s abdomen to remove the small intestine and measure the distance traveled by the marker. The same methods were used as previously, but 30 min before the administration of the activated charcoal, the castor oil (0.01 ml/g) was added orally (Hsu, 1982; Than et al., 1989). The results were expressed as a percentage of the distance covered by the marker in relation to the total length of the small intestine. The inhibitory effect that was exerted by OC-EtOH was evaluated based on an ED50 analysis.
Effect of OC-EtOH on Castor Oil-Induced Diarrhea in Mice
Animals were treated orally with 0.9% NaCl plus Cremophor® (10 ml/kg, negative control), loperamide (10 mg/kg, positive control) or OC-EtOH (62.5; 125, 250, 500 and 1,000 mg/kg) in different groups (n = 6, each). Then, to induce diarrhea, castor oil (0.01 ml/g) was administered by gavage after 30 min of the initial treatments. These same animals were placed in boxes lined with white paper, separately, and observed for 4 h uninterrupted. The number of stools were assessed, as well as their consistency, and classified as solid or liquid to determine the total number of stools and the total quantity of liquid stool episode (Awouters et al., 1978). The inhibitory effect of OC-EtOH was evaluated based on the ED50 value, which is the dose of a drug that produces 50% of its maximal effect.
Statistical Analysis
The results were expressed as a percentage of the mean ± standard error of the mean (SEM) and statistically analyzed by the one-way ANOVA followed by Bonferroni’s post-test for multiple comparisons. The null hypothesis was discarded when p < 0.05. The EC50 and ED50 values were calculated by nonlinear regression. The data were analyzed using GraphPad Prism® software version 5.01 (GraphPad Software Inc., San Diego, CA, United States).
Results
HPLC Analysis
In the chromatogram regarding the quantification of β-sitosterol and stigmasterol steroids, it was possible by high performance liquid chromatography–evaporative light-scattering detector (HPLC-ELSD). The chromatogram shows that the concentration of stigmasterol is greater than β-sitosterol and can be considered as chemical marker for this specie. Curiously, these compounds are isolated from plant species always together, however in this work it was possible to analyze and quantify the two separate constituents using C-18 column coupled to HPLC-ELSD (Figure 1; Table 1).
The calibration curve was based on peak area and using external standard in concentrations ranging from 0.0156 to 0.125 mg/ml, for both β-sitosterol (Sigma) and stigmasterol (Merck), both in triplicate. The limit of detection (LOD) and the limit of quantification (LOQ) for β-sitosterol were 0.135 and 0.408 μg, respectively, and for stigmasterol were 0.131 and 0.396 µg, respectively.
Effect of OC-EtOH on Basal Tonus of Guinea Pig Ileum
OC-EtOH (0.1–2187 μg/ml, n = 5), cumulatively added on basal tonus, contracted guinea pig ileum in a concentration-dependent manner (EC50 = 48.6 ± 2.7 μg/ml) with a similar amplitude to previous contraction induced by KCl 40 mM (Figure 2A). Maximum contractile effect (Emax) of the OC-EtOH was obtained with 729 μg/ml (Figure 2).
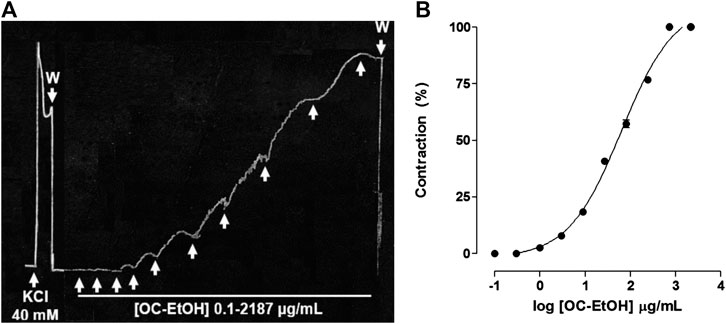
FIGURE 2. Representative record of OC-EtOH (0.1–2,187 μg/ml, n = 5) cumulative contractile effect on basal tonus of guinea pig ileum (A). Cumulative concentration-response curves to OC-EtOH (● on basal tonus of guinea pig ileum (n = 5) (B). The upward arrows represent the concentration of 0.1; 0.3; 1; 3; 9; 27; 81; 243; 729 and 2,187 μg/ml of OC-EtOH. W, wash.
Evaluation of Muscarinic Receptors Participation in Spasmogenic Effect of OC-EtOH
In presence of atropine (10–8 and 3 × 10–8 M), a non-selective muscarinic antagonist (positive control), OC-EtOH-induced cumulative contraction curve (EC50 = 48.6 ± 2.7 μg/ml, n = 5) was not shifted (EC50 = 43.3 ± 2.6 and 41.4 ± 3.8 μg/ml, respectively, n = 5) and Emax was not modified (Figure 3A).
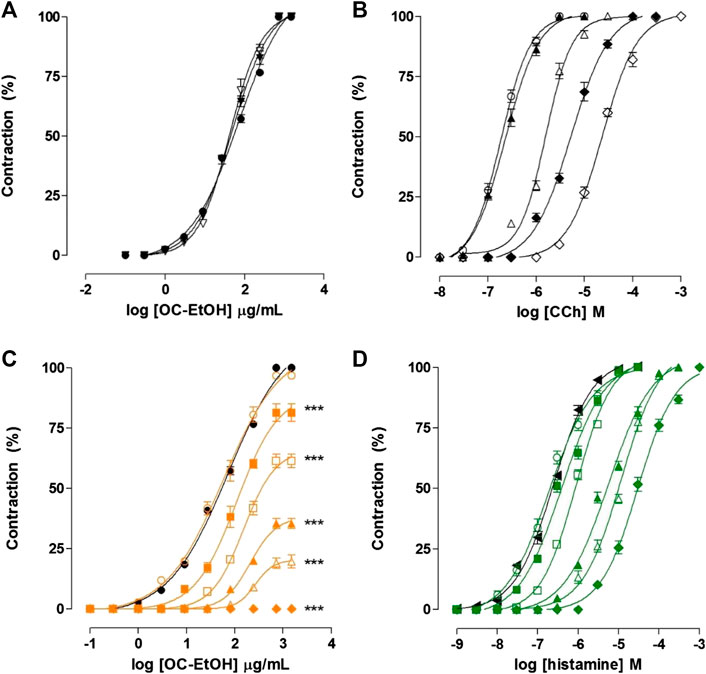
FIGURE 3. Cumulative concentration–response curves to OC-EtOH (A,C), CCh (B) and histamine (D) on guinea pig ileum in both absence (○, ●, ◀, ●) and presence of atropine 10–9 (▲), 3 × 10–9 (△), 10–8 (◆, ▼) and 3 × 10–8 M (◇, ▽) or pyrilamine 10–9 (green unfiled circle, orange unfiled circle), 3 × 10–9 (green filed square, orange filed square), 10–8 (green unfiled square, orange unfiled square), 3 × 10–8 (green filed triangle, orange filed triangle), 10–7 (green unfiled triangle, orange unfiled triangle) and 3 × 10–7 M (green filed diamond, orange filed diamond) on guinea pig ileum. Symbols and vertical bars represent the mean and S.E.M., respectively (n = 5). CCh = Carbachol. One-way ANOVA followed by Bonferroni’s post-test: ***p < 0.001 (control vs. pyrilamine).
Differently, in presence of atropine (positive control), CCh-induced cumulative contraction curve (10−8–3 × 10–5 M, n = 5) was shifted to the right in a parallel manner, without Emax reduction. EC50 values for CCh were 1.9 ± 0.1 × 10–7 M in the absence and 2.4 ± 0.2 × 10–7; 1.5 ± 0.07 × 10–6; 5.3 ± 0.4 × 10–6 and 2.3 ± 0.04 × 10–5 M in the presence of 10–9; 3 × 10–9; 10–8 and 3 × 10–8 M of atropine, respectively (Figure 3B).
Evaluation of Histamine Receptors Participation in Spasmogenic Effect of OC-EtOH
In presence of pyrilamine, a H1 histamine receptors antagonist (positive control), the OC-EtOH-induced cumulative contraction curve (10–9–3 × 10–7 M) was inhibited and shifted to the right in a non-parallel manner and presented Emax reduction of 100% (control) to 96.7 ± 1.1; 81.4 ± 3.6; 61.3 ± 2.8; 35.5 ± 2.1; 19.7 ± 2.7 and 0%, respectively (n = 5). The EC50 values of OC-EtOH were altered from 48.6 ± 2.7 μg/ml (control) to 41.3 ± 3.2; 88.6 ± 10; 131.2 ± 11 and 255.2 ± 25.7 μg/ml using the following pyrilamine concentrations 10–9, 3 × 10–9, 10–8, 3 × 10–8, 10–7 and 3 × 10–7 M, respectively (Figure 3C).
In the same way, in pyrilamine presence (positive control), histamine-induced cumulative contraction curve (10−9–10–3 M) was shifted to the right in a parallel manner without Emax reduction (n = 5). The EC50 values to histamine was 2.3 ± 0.2 × 10–7 M in absence and 2.5 ± 0.3 × 10–7; 3.9 ± 0.3 × 10–7; 8.3 ± 0.3 × 10–7; 5.0 ± 0.2 × 10–6; 9.5 ± 0.2 × 10–6 and 3.1 ± 0.3 × 10–5 M in presence of 10–9; 3 × 10–9; 10–8; 3 × 10–8; 10–7 and 3 × 10–7 M of pyrilamine, respectively (Figure 3D).
Evaluation of CaV Participation in OC-EtOH Spasmogenic Effect
OC-EtOH-induced cumulative contraction curve (EC50 = 48.6 ± 2.7 μg/ml, n = 5) was inhibited in the presence of 10–7, 3 × 10–7 and 10–6 M of verapamil, a CaV blocker, with abolition of OC-EtOH contractile effect (Figure 4).
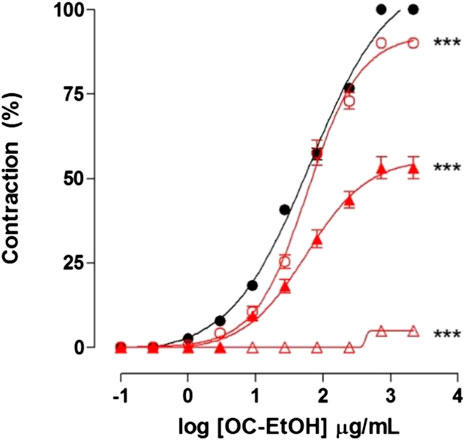
FIGURE 4. Cumulative concentration–response curves to OC-EtOH in absence (●) and presence of verapamil 10–7 (◯), 3 × 10–7 (▲) and 10–6 (△) on guinea pig ileum. Symbols and vertical bars represent the mean and S.E.M., respectively (n = 5). One-way ANOVA followed by Bonferroni's post-test: ***p < 0.001 (control vs. verapamil).
According to EC50 values of OC-EtOH, no differences between control curve (EC50 = 48.6 ± 2.7 μg/ml, n = 5) and those obtained in presence of 10–7 and 3 × 10–7 M of verapamil (EC50 = 55.9 ± 5.3 and 51.8 ± 3.7 μg/ml, respectively, n = 5) were observed. It was not possible to calculate the extract EC50 when the organ was incubated with 10–6 M of verapamil. Emax values were altered in the presence of 10–7; 3 × 10–7 and 10–6 M of verapamil from 100% (control) to 90.0 ± 1.2; 53.2 ± 3.2 and 4.9 ± 1.3%, respectively (Figure 4).
Effect of OC-EtOH on KCl-, Histamine- or Carbachol-Induced Tonic Contractions
OC-EtOH (0.1–2187 μg/ml, n = 5) induced a transient contractile effect (spasmogenic) followed by a relaxation (spasmolytic) in a concentration-dependent manner when guinea pig ileum was pre-contracted both with KCl 40 mM or histamine 10–6 M (Figure 5). Transient contraction induced by OC-EtOH at 27, 81, 243 and 729 μg/ml were 0.0; 24.2 ± 7.1; 83.1 ± 6.9 and 99.8 ± 0.1%, respectively, compared to tonic component induced by KCl and 12.4 ± 2.2; 59.8 ± 10.7; 86.5 ± 7.9 and 88.1 ± 5.8%, respectively, compared to histamine (Figure 6). OC-EtOH spasmogenic potency was around twice more potent when the contraction was induced by histamine (EC50 = 73.5 ± 6.9 μg/ml) than by KCl (EC50 = 176.6 ± 32.6 μg/ml) (Figure 6).
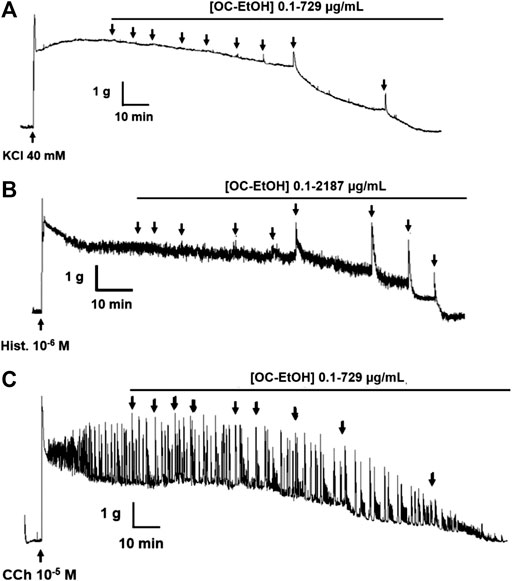
FIGURE 5. Representative records of OC-EtOH relaxant effect on guinea pig ileum pre-contracted by KCl 40 mM (A), histamine 10–6(B) or CCh 10–5 M (C) (n = 5).
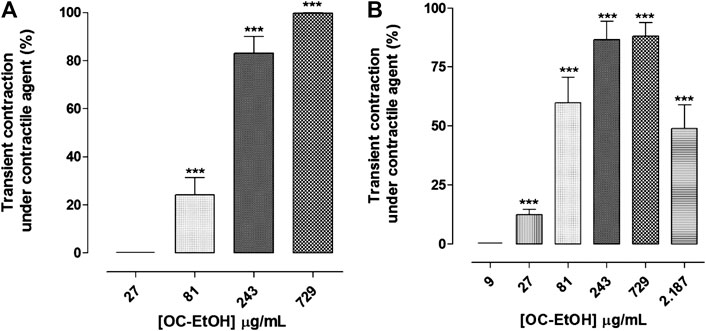
FIGURE 6. OC-EtOH contractile effect under KCl-(A) and histamine-(B) induced tonic contractions on guinea pig ileum. Vertical bars represent the means and S.E.M., respectively. One-way ANOVA followed by Bonferroni’s post-test, significant differences are indicated by ***p < 0.001 (n = 5).
Differently, on tonic contraction induced by CCh 10–5 M, OC-EtOH (0.1–729 μg/ml, n = 5) showed only a relaxant effect profile (Figure 5). About OC-EtOH spasmolytic potency, it was equipotent to relax guinea pig ileum pre-contracted either by KCl (EC50 = 103.9 ± 8.6 μg/ml), histamine (EC50 = 90.1 ± 9.2 μg/ml) or CCh (EC50 = 97.1 ± 17.4 μg/ml). The OC-EtOH spasmolytic Emax (100%) was obtained at 729 μg/ml when guinea pig ileum was contracted by KCl or CCh, and at 2187 μg/ml when the organ was contracted by histamine (Figure 7).
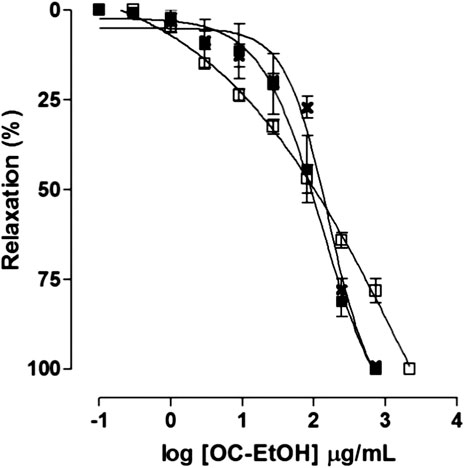
FIGURE 7. Effect of OC-EtOH on guinea pig ileum pre-contracted with KCl 40 mM (✖), histamine 10–6 M (□) or CCh 10–5 M (■) (n = 5). Symbols and vertical bars represent the mean and S.E.M., respectively.
Effect of OC-EtOH on KCl-Induced Tonic Contraction in the Presence of Pyrilamine
In the presence of pyrilamine (3 × 10–7 M), the transient contractile effect of OC-EtOH on KCl-induced tonic contraction was abolished (Figure 8). Interestingly, in presence of pyrilamine, OC-EtOH spasmolytic effect was potentiated (Figure 9).
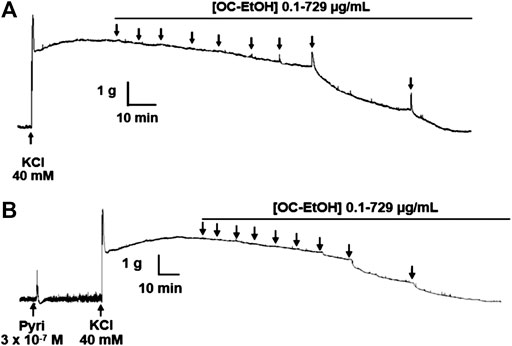
FIGURE 8. Representative records of OC-EtOH relaxant effect on guinea pig ileum pre-contracted with KCl 40 mM in absence (A) and presence (B) of pyrilamine (n = 5). Pyri, pyrilamine.
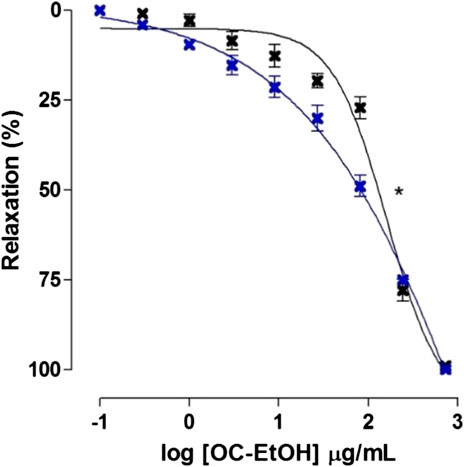
FIGURE 9. Relaxant effect of OC-EtOH on guinea pig ileum pre-contracted with KCl 40 mM in absence (black cross) and presence (blue cross) of pyrilamine. Symbols and vertical bars represent the mean and S.E.M., respectively (n = 5). One-way ANOVA followed by Bonferroni’s post-test: *p < 0.05 (KCl vs. pyrilamine + KCl).
According to EC50 values, OC-EtOH was about 2-fold more potent in relaxing KCl-induced tonic contraction in the presence of pyrilamine 3 × 10–7 M (EC50 = 62.9 ± 9.9 μg/ml, n = 5) than in its absence (EC50 = 103.9 ± 8.6 μg/ml, n = 5).
Effect of OC-EtOH on CaCl2-Induced Contractions in Depolarizing Medium Nominally Without Ca2+
OC-EtOH (27, 81, 243 and 729 μg/ml, n = 5) inhibited the contractions induced by cumulative increase in extracellular concentration of CaCl2 in depolarizing medium nominally without Ca2+ (n = 5). CaCl2 cumulative concentration-response curves were shifted to the right in a non-parallel manner and with Emax reduction from 100% (control) to 98.5 ± 0.9; 69.3 ± 4.8; 45.0 ± 4.4 and 1.8 ± 0.5%, respectively. EC50 values of the CaCl2 were altered from 2.1 ± 0.1 × 10–3 M (control) to 2.5 ± 0.3; 3.9 ± 0.7 and 6.8 ± 1.4 × 10–3 M, respectively. The EC50 could not be determined when the organ was incubated with 729 μg/ml of the extract t (Figure 10A).
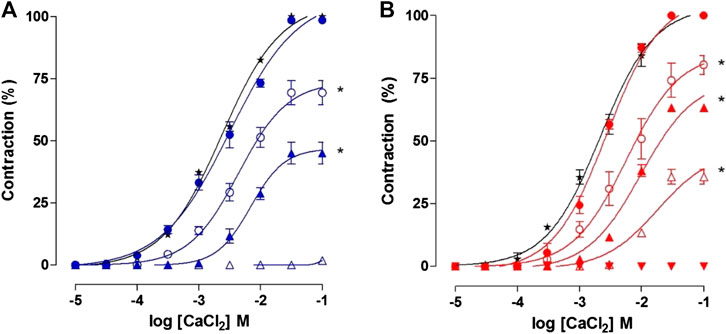
FIGURE 10. Cumulative concentration–response curves to CaCl2 in depolarizing medium nominally without Ca2+ in absence (★) and presence of 27 (blue filed circle), 81 (blue unfiled circle), 243 (blue filed triangle) and 729 μg/ml (blue unfiled triangle) of OC-EtOH (A) and in presence of 3 × 10–8 (red filed circle), 10–7 (red unfiled circle), 3 × 10–7 (red unfiled triangle10–6 (red filed triangle) and 3 × 10–6 M (red inverted filed triangle) of verapamil (B) on guinea pig ileum. Symbols and vertical bars represent the mean and S.E.M., respectively (n = 5). One-way ANOVA followed by Bonferroni's post-test: *p < 0.05 (control vs. verapamil or OC-EtOH).
Similarly, verapamil, a CaV blocker (positive control; 3 × 10–8–3 × 10–6 M) inhibited CaCl2 cumulative concentration-response curves (10−5–10–1 M). The curves were rightwards shifted, in a non-parallel manner, presenting Emax reduction from 100% (control) to 80.2 ± 3.7; 63.2 ± 0.9; 35.6 ± 2.9 and 0%, respectively. EC50 values of the CaCl2 were altered from 2.1 ± 0.3 × 10–3 M (control) to 2.4 ± 0.2; 3.5 ± 0.4; 7.4 ± 0.03 × 10–3 and 1.2 ± 0.01 × 10–2 M; respectively (Figure 10B).
Effect of OC-EtOH on S-(-)-Bay K8644-Induced Tonic Contractions
OC-EtOH relaxed guinea pig ileum pre-contracted with S-(-)-Bay K8644 3 × 10–7 M, a CaV agonist, in pyrilamine presence (EC50 = 166.9 ± 17.8 μg/ml, n = 5) and the relaxant potency of the extract was reduced by 2.6 times when compared to pre-contracted ileum with KCl 40 mM (EC50 = 62.9 ± 9.9 μg/ml n = 5) (Figure 11A).
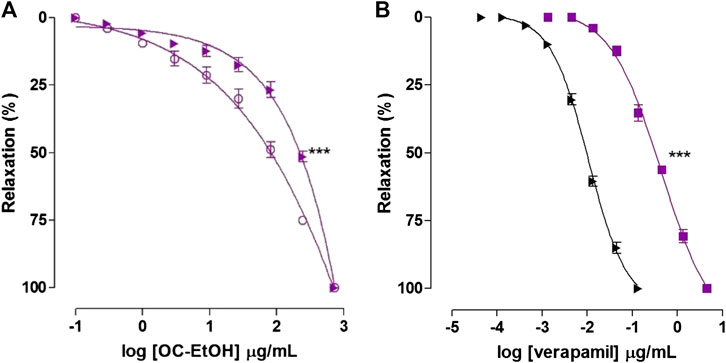
FIGURE 11. Relaxant effect of OC-EtOH (A) on guinea pig ileum pre-contracted with KCl 40 mM (violet unfiled circle) and S-(-)-Bay K8644 3 × 10–7 M (violet right-pointing triangle) in pyrilamine presence and relaxant effect of verapamil (B) on guinea pig ileum pre-contracted with KCl 40 mM (black right-pointing triangle) and S-(-)-Bay K8644 3 × 10–7 M (violet filed square) in pyrilamine presence. Symbols and vertical bars represent the mean and S.E.M., respectively (n = 5). One-way ANOVA followed by Bonferroni's post-test: ***p < 0.001 (S-(-)-Bay K8644 vs. KCl).
As positive control, verapamil relaxed guinea pig ileum pre-contracted with KCl 40 mM (EC50 = 0.0094 ± 0.0006 μg/ml) and S-(-)-Bay K8644 3 × 10–7 M (EC50 = 0.30 ± 0.02 μg/ml, n = 5), in medium partially depolarized with KCl 15 mM (Figure 11B).
Behavioral Pharmacological Screening and Evaluation of Acute Toxicity of OC-EtOH in Mice
After OC-EtOH oral administration (2,000 mg/kg), no behavioral changes were observed in female mice (n = 6) in the evaluated experimental conditions during 4 h of observation. Weight assessment were performed before the treatment, 7 and 14 days after the treatment and no changes in animals’ weight were observed in both negative control animals (33.5 ± 0.3, 33.7 ± 0.8 and 34.8 ± 0.6 g) and in the animals treated with OC-EtOH (29.0 ± 0.5, 30.0 ± 0.7 and 30.7 ± 0.6 g)., respectively. Interestingly, there were no death events during 14 days of observation.
Effect of OC-EtOH on Upper Gastrointestinal Transit in Mice
OC-EtOH (250, 500 and 1,000 mg/kg, p.o.) increased dose dependently the intestinal transit traveled by the activated charcoal (marker) (72.9 ± 1.2%) to 82.6 ± 1.8 and 90.5 ± 1.5%, respectively, showing an ED50 of 477.6 ± 14.8 mg/ml (n = 6). The distance traveled by the marker in the intestine of control animals (72.9 ± 1.2% of intestine total length) was decreased to 42.2 ± 1.4% when the animals were treated with atropine (2 mg/kg, p.o.) (Figure 12A).
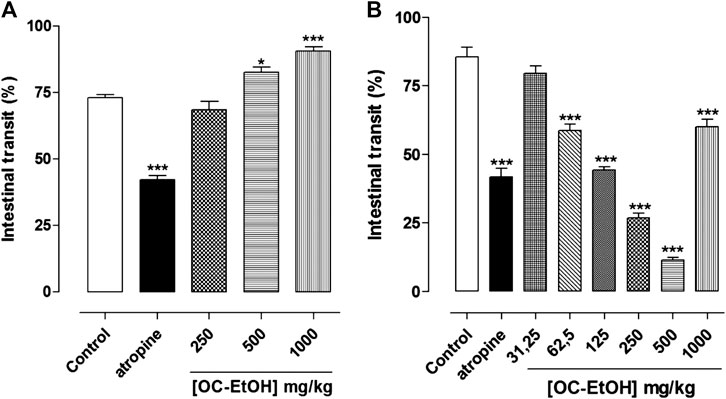
FIGURE 12. Effect of OC-EtOH on normal (A) and castor-oil-induced (B) upper gastrointestinal transit in mice (n = 6). Columns and vertical bars represent the mean and S.E.M., respectively. One-way ANOVA followed by Bonferroni’s post-test: *p < 0.05 and ***p < 0.001 (saline vs. atropine/OC-EtOH).
In contrast, OC-EtOH (62.5; 125, 250, 500 and 1,000 mg/kg) inhibited the castor oil-induced intestinal transit, in a dose-dependent manner, reducing the distance in 41.3 ± 2.3; 55.6 ± 1.1; 73.2 ± 1.8; 88.5 ± 0.9 and 40.0 ± 2.8% (n = 6), respectively, in comparison to the negative control (0.9% saline + Cremophor®). It was also observed that atropine (2 mg/kg) inhibited 58.2 ± 3.1% of the distance traveled by the marker in the castor oil-induced intestinal transit as compared to the negative control (Figure 12B). OC-EtOH showed ED50 of 93.30 ± 7.2 mg/kg and the Emax (88.5 ± 0.9%) was observed at 500 mg/kg (n = 6).
Effect of OC-EtOH on Castor Oil-Induced Diarrhea in Mice
OC-EtOH (125, 250, 500 and 1,000 mg/kg, p.o., n = 6) inhibited castor oil-induced diarrhea equipotently and in a dose-dependent manner in terms of defecation frequency (15.4 ± 2.2; 29.8 ± 3.6; 59.8 ± 1.6 and 40.0 ± 5.3%, respectively) and number of liquid stools (30.0 ± 3.6; 66.7 ± 3.3; 85.0 ± 2.2 and 68.3 ± 5.4%) when compared to the negative control. It was also observed that loperamide (positive control, 10 mg/kg) inhibited 100% of castor oil-induced diarrhea (Figure 13). OC-EtOH showed ED50 and Emax 2-3-fold more potent in inhibiting liquid stool (ED50 = 189.1 ± 13.6 mg/ml, Emax = 15.0 ± 2.2%) compared to defecation frequency (ED50 = 387.7 ± 21.6 mg/ml, Emax = 40.2 ± 1.6%).
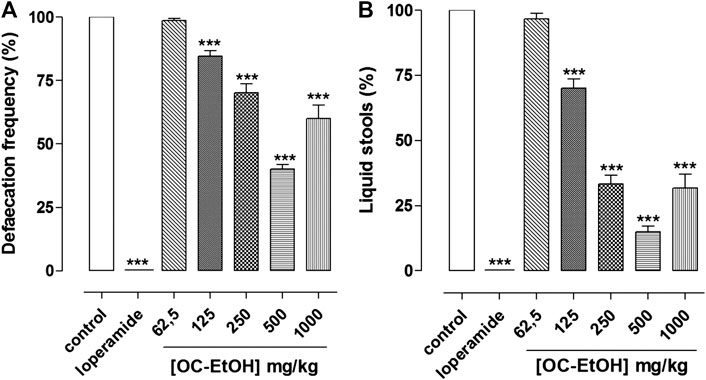
FIGURE 13. Antidiarrheal effect of OC-EtOH on castor-oil-induced diarrhea in mice (n = 6). Percentage of total stools number (A) and percentage of liquid stools (B). Columns and vertical bars represent mean and S.E.M., respectively. One-way ANOVA followed by Bonferroni’s post-test: ***p < 0.001 (saline vs. loperamide/OC-EtOH).
Discussion
In this work, the effects of ethanolic extract obtained from Oceanapia magna. sponge (OC-EtOH) were investigated on rodents’ intestinal smooth muscle. It was demonstrated, for the first time, that OC-EtOH has dual effect on gastrointestinal tract, presenting spasmogenic action in guinea pig ileum, through histamine receptors (H1 subtype) activation, and spasmolytic effect by blocking the influx of Ca2+ through voltage-gated calcium channels (CaV1). Therefore, Oceanapia magna. sponge demonstrated to be a potential source for medicinal metabolites that could be used for intestinal diseases treatment, such as constipation and/or diarrhea.
Initially, the effect of OC-EtOH was assessed on guinea pig ileum basal tonus in order to evaluate its possible activity on intestinal smooth muscle. Thus, the extract promoted intestinal contraction in a concentration-dependent manner (Figure 2), showing the spasmogenic effect of OC-EtOH.
In view of these results, an investigation of the mechanism that leads the tested product to promote a contraction was carried out. One of the possible spasmogenic mechanisms could involve the activation of M3 and/or H1 receptors. These receptors are characterized as receptors coupled to Gq/11 protein-PLCβ effector system, resulting in an increase of intracellular Ca2+ concentration, leading to muscle contraction (Ehlert et al., 1999; Berridge et al., 2003). To evaluate the muscarinic receptors participation in spasmogenic effect, we used the standard drug atropine, a nonselective muscarinic receptors antagonist (Bashir et al., 2006). According to the results, it was observed that atropine, in different concentrations, did not inhibit OC-EtOH-induced cumulative contraction curves. Thus, muscarinic receptors activation by OC-EtOH was discarded in its contractile effect (Figure 3).
H1 histamine receptors are distributed in a variety of tissues, including airways, genitourinary, cardiovascular and gastrointestinal smooth muscle (Hill, 1990). To evaluate the hypothesis that OC-EtOH could act on H1 receptors to produce its contractile effect, pyrilamine (H1 receptor antagonist) was used as standard drug (Ghayur and Gilani, 2005). It was found that OC-EtOH-induced cumulative contraction curves in pyrilamine presence were shifted to right with reduction on both potency and efficacy. According to appearance of curves, it can be said that in OC-EtOH there are components that appear to act via H1 receptors, however there are other components that act in other ways (Figure 3).
An increase of [Ca2+]c in the smooth muscle is the main cause for contraction production (Hill-Eubanks et al., 2011). CaV are an essential element in initialization and/or maintenance of smooth muscle contraction. So, we decided to evaluate if CaV could be involved in the OC-EtOH spasmogenic mechanism of action. Verapamil, a CaV blocker, was used as a standard drug (Staneva-Stoytcheva and Venkova, 1992). OC-EtOH was added in a cumulative manner in the presence of different concentrations of verapamil and the curves were shifted to the right, with a reduction in both potency and efficacy of OC-EtOH (Figure 4). It can be suggested that the secondary metabolites found in OC-EtOH are activating CaV to promote its spasmogenic effect on guinea pig ileum.
Guinea pig ileum has a biphasic contraction (phasic and tonic) and, particularly, this organ depends on extracellular Ca2+ and the influence of this ion is higher on the tonic response (Triggle et al., 1979; Honda et al., 1996). Therefore, we decided to also evaluate the effect of OC-EtOH on tonic component of the contraction induced by different contractile agents such as KCl, histamine and CCh on guinea pig ileum.
The OC-EtOH presented a dual profile on tonic contraction induced by KCl and histamine: in the first moment, the extract induced a transient contraction followed by a slow relaxation, both in a concentration-dependent manner (Figure 5). No significant difference between the values for the spasmolytic extract potency was observed (Figure 7) and this indicates that OC-EtOH has secondary metabolites that act in a common pathway to modulate the intestinal smooth muscle motility. OC-EtOH showed a higher spasmogenic potency when compared to its spasmolytic effectiveness. These findings suggest, for the first time, that OC-EtOH, a marine natural product, presents dual effect (spasmogenic and spasmolytic) on guinea pig intestinal smooth muscle.
As showed until now, OC-EtOH possibly presents secondary metabolites with dual effect on intestinal smooth muscle. So, it was decided to proceed with the investigation of the mechanisms underlying the OC-EtOH spasmolytic activity.
To verify if the OC-EtOH spasmogenic component was interfering in the relaxant action, the experimental protocols were carried out in the presence of pyrilamine to avoid the OC-EtOH spasmogenic activity. The OC-EtOH-induced contraction was abolished in the presence of the H1-antagonist, and the OC-EtOH relaxation effect on tonic contraction induced by KCl was about 2-fold more potent when compared to relaxation in antagonist absence (Figures 8,9). Therefore, this evidence suggests that the transient contractile effect promoted by OC-EtOH is due to a positive modulation of H1 receptors on intestinal smooth muscle.
Smooth muscle is described as a biphasic organ, presenting two phases of contraction. In the first one, the contraction is rapid and transient. The second phase is characterized by a tonic contraction that is maintained for a while (Horie et al., 2005). Both phases are dependent on extracellular Ca2+, as the whole contraction is inhibited by blocking CaV, which means that this is one of the main pathways involved in this entry (Rembold, 1996). Since OC-EtOH inhibited the tonic contractions induced by three distinct contractile agents in a concentration-dependent manner, presenting no difference among the pharmacological potencies (Figure 7), we theorized that OC-EtOH might be acting on CaV, by blocking Ca2+ influx once both coupling mechanisms, mixed (histamine and CCh) and electromechanical (KCl), lead to tonic contractions almost exclusively by Ca2+ influx through CaV (Bolton, 1979; Rembold, 1996; Bolton, 2006).
Verapamil was used as positive control since it is a standard drug that blocks CaV. In presence of OC-EtOH, cumulative concentration-response curves to CaCl2 were shifted to right, in a non-parallel manner, and reduced Emax (Figure 10). The results obtained were similar to the positive control. These findings support the hypothesis that OC-EtOH could inhibit Ca2+ influx through CaV to produce its spasmolytic effect.
To reinforce this hypothesis, OC-EtOH was assessed in relaxing the guinea pig ileum pre-contracted by S-(-)-Bay K8644 (Conte-Camerino et al., 1987), a CaV1 agonist (Ferrante et al., 1989). The experimental protocol was carried out in pyrilamine presence, in order to remove the spasmogenic effect of the extract, and verapamil was used as positive control. OC-EtOH relaxed the organ pre-contracted with S-(-)-Bay K8644 in a concentration-dependent manner and it was 2.6 times less potent when the organ was pre-contracted with KCl (Figure 11). So, surely, OC-EtOH blocked the Ca2+ channels to induce its spasmolytic effect on guinea pig ileum, and CaV1 is the channel subtype involved, but other targets are not discarded in the participation of OC-EtOH dual effect on intestinal smooth muscle.
Following the in vitro investigation, female mice received acute treatment of OC-EtOH 2,000 mg/kg (n = 6, p.o.) and animals were observed for 4 h. No behavioral changes were observed, discarding toxic effect on central and autonomic nervous systems. There was no death of animals during 14 days of observation and no interference in ponderal evolution of animals. This assay was divided into two groups with three animals each. So, in the dosing regimen used (2,000 mg/kg), OC-EtOH showed no toxic effect, and, according to 423/2001 Organization for Economic Cooperation and Development (OEDC) guideline, this result indicates a low acute toxicity and an estimate of LD50 greater than or equal to 5,000 mg/kg. This result shows that OC-EtOH can be considered safe for the following in vivo experimental protocols.
Since OC-EtOH presented spasmogenic and spasmolytic activity in intestinal smooth muscle and diarrhea and constipation can be caused, among other factors, due to changes in intestinal motility (Field and Semrad, 1993), we decided to investigate if OC-EtOH alters the intestinal motility in mice.
Interestingly, OC-EtOH did not inhibit normal upper gastrointestinal transit in mice, but an increase of the intestinal distance traveled by the marker was observed when animals were treated with doses of 500 and 1,000 mg/kg when compared to control group. Because at the dose of 500 mg/kg the observed effect was not 100%, the 1,000 mg/kg dose was used to make sure that the previous dose would be the maximum or not. The fact that the dose of 1,000 mg/ml gave a higher value can be explained by the desensitization of the organ or system, showing that the dose of 500 mg/kg is the one that produces the maximum effect. Given these results and corroborating data found in vitro assays, it may be suggested the OC-EtOH promotes a laxative effect under physiological conditions. Different results were observed in castor-oil-induced intestinal transit, since, in this biological context, OC-EtOH inhibited dose-dependently intestinal motility in mice (Figure 12). This fact may be directly related to an antidiarrheal effect and this might occur by intestinal motility inhibition, in pathological conditions, what could represent a relevant therapeutic effect. This effect can help to reduce the number of evacuations, increase water absorption due to slow passage of intestinal contents, and increase stool viscosity (Gurgel et al., 2007).
It is reported that castor oil inhibits Na+/K+-ATPase, reducing the absorption of normal liquid (Izzo et al., 1999), in addition to promoting the activation of adenylyl cyclase, stimulating the production of cAMP (Gaginella and Bass, 1978). Ricinoleic acid is also responsible for producing irritating and inflammation of the intestinal mucosa, leading to the release of several inflammatory mediators, such as prostaglandins, nitric oxide (NO), platelet activation factor (PAF) and tachykinins (Awouters et al., 1978). In addition, the ricinoleic acid is an agonist of prostaglandin E2 (PGE2) receptors, activating EP3 and EP4, and the activation of the former in intestinal smooth muscle is responsible for promoting its diarrheal effect. OC-EtOH also inhibited castor oil-induced diarrhea in a concentration-dependent manner by inhibiting both defecation frequency and liquid feces. The extract was twice more potent and effective in inhibiting watery stools compared with total defecation frequency. This fact is also quite interesting, as it may suggest that OC-EtOH would be interfering in one of the aforementioned ricinoleic acid-activated pathways, acting on liquid stools formation without preventing normal feces formation, which could cause constipation (Figure 13).
In conclusion, OC-EtOH showed to be a safe marine product since it was assessed by an animal model of toxicity. For the first time, a biological activity was assigned for Oceanapia magna and it was observed that OC-EtOH presented dual effect (spasmogenic due to histamine receptors activation, and spasmolytic by Ca2+ influx through CaV blockade). Further studies are needed to verify other possible targets for the mechanism of action of OC-EtOH. Its potential benefits by acting in a more balanced way than compounds with a single target and, consequently, would show a satisfactory pharmacological combination effect and/or reduced side effects. Thus, Oceanapia magna sponge arises as an oceanic natural product with potential medical use to intestinal diseases, such as diarrhea.
Conflicts of Interest
The authors declare that the research was conducted in the absence of any commercial or financial relationships that could be construed as a potential conflict of interest.
Data Availability Statement
The raw data supporting the conclusions of this article will be made available by the authors, without undue reservation.
Ethics Statement
The animal study was reviewed and approved by Ethics Committee on Animal Use (CEUA)/UFPB, certificate no. 146/2015.
Author Contributions
JP, IF, FO, SF, and GM were involved in experimental work and preparation of the manuscript. BVS, UP, and TS contributed to obtaining and standardizing the OC-EtOH. BAS and FC elaborated the experimental design and all aspects of this project.
Funding
The authors thank to Coordenação de Aperfeiçoamento de Pessoal de Nível Superior (CAPES) and to Conselho Nacional de Desenvolvimento Científico e Tecnológico (CNPq) for financial support and UFPB and PPgPNSB for experimental support.
References
Almeida, R. N., Facão, A. C. G. M., Diniz, R. S. T., Quintans-Júnior, L. J., Polari, R. M., Barbosa-Filho, J. M., et al. (1999). Metodologia para avaliação de plantas com atividade no SNC e alguns dados experimentais. Rev. Bras. Farmacogn. 80, 72–76.
Artan, M., Li, Y., Karadeniz, F., Lee, S. H., Kim, M. M., and Kim, S. K. (2008). Anti-HIV-1 activity of phloroglucinol derivative, 6,6'-bieckol, from Ecklonia cava. Bioorg. Med. Chem. 16, 7921–7926. doi:10.1016/j.bmc.2008.07.078
Awouters, F., Neimegeers, C. J. E., Lenaert, F. M., and Jassen, L. (1978). Delay of castor oil diarrhoea in rats: a new way to evaluate inhibitors of prostaglandin biosynthesis. J. Pharm. Pharmacol. 30, 41–45. doi:10.1111/j.2042-7158.1978.tb13150.x
Bashir, S., Janbaz, K. H., Jabeen, Q., and Gilani, A. H. (2006). Studies on spasmogenic and spasmolytic activities of Calendula officinalis flowers. Phytother. Res. 20, 906–910doi:10.1002/ptr.1980
Batra, V. R., and Schrott, L. M. (2011). Acute oxycodone induces the pro-emetic Pica response in rats. J. Pharmacol. Exp. Therapeut. 339, 738–745. doi:10.1124/jpet.111.183343
Berridge, M. J., Bootman, M. D., and Roderick, H. L. (2003). Calcium signalling: dynamics, homeostasis and remodelling. Nat. Rev. Mol. Cell Biol. 4, 517–529. doi:10.1038/nrm1155
Bielefeldt, K., Levinthal, D. J., and Nusrat, S. (2016). Effective constipation treatment changes more than bowel frequency: a systematic review and meta-analysis. J. Neurogastroenterol. Motil. 22 (1), 31–45. doi:10.5056/jnm15171
Bolton, T. B. (2006). Calcium events in smooth muscles and their interstitial cells; physiological roles of sparks. J. Physiol. (Lond.). 570, 5–11. doi: doi:10.1113/jphysiol.2005.095604
Bolton, T. B. (1979). Mechanisms of action of transmitters and other substances on smooth muscle. Physiol. Rev. 59, 606–718. doi:10.1152/physrev.1979.59.3.606
Butler, M. S., Robertson, A. A., and Cooper, M. A. (2014). Natural product and natural product derived drugs in clinical trials. Nat. Prod. Rep. 31, 1612–1661. doi:10.1039/C4NP00064A
Cavalcante-Silva, L. H., Correia, A. C., Sousa, J. C., Barbosa-Filho, J. M., Santos, B. V., De Miranda, G. E., et al. (2016). Involvement of β adrenergic receptors in spasmolytic effect of caulerpine on Guinea pig ileum. Nat. Prod. Res. 30, 1–6. doi:10.1080/14786419.2015.1120728
Cavalcante-Silva, L. H., Correia, A. C. C., Barbosa-Filho, J. M., Silva, B. A., Santos, B. V. O., Lira, D. P., et al. (2013). Spasmolytic effect of caulerpine involves blockade of Ca²⁺ influx on Guinea pig ileum. Mar. Drugs. 11, 1553–1564. doi:10.3390/md11051553
Chao, C. H., Wen, Z. H., Wu, Y. C., Yeh, H. C., and Sheu, J. H. (2008). Cytotoxic and anti-inflammatory cembranoids from the soft coral Lobophytum crassum. J. Nat. Prod. 71, 1819–1824. doi:10.1021/np8004584
Conte-Camerino, D., Lograno, M. D., De Lucia, A., Persichella, M., and Franconi, F. (1987). The effects of the calcium channel agonist, Bay K-8644, on Guinea-pig ileum and rat uterine horn. J. Pharm. Pharmacol. 39, 954–957. doi:10.1111/j.2042-7158.1987.tb03139.x
Courtois, A., Simon-Colin, C., Boisset, C., Berthou, C., Deslandes, E., Guézennec, J., et al. (2008). Floridoside extracted from the red alga Mastocarpus stellatus is a potent activator of the classical complement pathway. Mar. Drugs. 6, 407–417. doi:10.3390/md20080019
D'auria, M. V., Sepe, V., D'orsi, R., Bellotta, F., Debitus, C., and Zampella, A. (2007). Isolation and structural elucidation of callipeltins J-M: antifungal peptides from the marine sponge Latrunculia sp. Tetrahedron. 63, 131–140. doi:10.1016/j.tet.2006.10.032
Ehlert, F. J., Sawyer, G. W., and Esqueda, E. E. (1999). Contractile role of M2 and M3 muscarinic receptors in gastrointestinal smooth muscle. Life Sci. 64, 387–394. doi:10.1016/S0024-3205(98)00584-0
Ferrante, J., Luchowski, E., Rutledge, A., and Triggle, D. J. (1989). Binding of A 1,4-dihydropyridine calcium channel activator, (-) S Bay K 8644, to cardiac preparations. Biochem. Biophys. Res. Commun. 158, 149–154. doi:10.1016/S0006-291X(89)80190-1
Field, M., and Semrad, C. E. (1993). Toxigenic diarrheas, congenital diarrheas, and cystic fibrosis: disorders of intestinal ion transport. Annu. Rev. Physiol. 55, 631–655. doi:10.1146/annurev.ph.55.030193.003215
Gaginella, T. S., and Bass, P. (1978). Laxatives: an update on mechanism of action. Life Sci. 23, 1001–1009. doi:10.1016/0024-3205(78)90659-8
Ghayur, M. N., and Gilani, A. H. (2005). Pharmacological basis for the medicinal use of ginger in gastrointestinal disorders. Dig. Dis. Sci. 50, 1889–1897. doi:10.1007/s10620-005-2957-2
Guo, H., Zhang, J., Gao, W., Qu, Z., and Liu, C. (2014). Anti-diarrhoeal activity of methanol extract of Santalum album L. in mice and gastrointestinal effect on the contraction of isolated jejunum in rats. J. Ethnopharmacol. 154, 704–710. doi:10.1016/j.jep.2014.04.043
Gurgel, L. A., Silva, R. M., Santos, F. A., Martins, D. T., Mattos, P. O., and Rao, V. S. (2007). Studies on the antidiarrhoeal effect of dragon’s blood from Croton urucurana. Phytother Res. 15, 319–322. doi:10.1002/ptr.728
Gutierrez, R. M. P., Victoria, T. C., and Flores, J. M. M. (2012). Sesquiterpene lactones: antispasmodic principles of the freshwater algae Hydrodictyon reticulatum. Med. Chem. Res. 21, 1023–1029. doi:10.1007/s00044-011-9601-9
Hill, S. J. (1990). Distribution, properties, and functional characteristics of three classes of histamine receptor. Pharmacol. Rev. 42, 45–83.
Hill-Eubanks, D. C., Werner, M. E., Heppner, T. J., and Nelson, M. T. (2011). Calcium signaling in smooth muscle. Cold Spring Harb. Perspect. Biol. 3, a004549. doi:10.1101/cshperspect.a004549
Honda, K., Takano, Y., and Kamiya, H. (1996). Involvement of protein kinase C in muscarinic agonist-induced contractions of Guinea pig ileal longitudinal muscle. Gen. Pharmacol. 27, 957–961. doi:10.1016/0306-3623(95)02124-8
Horie, S., Tsurumaki, Y., Someya, A., Hirabayashi, T., Saito, T., Okuma, Y., Nomura, Y., and Murayama, T. (2005). Involvement of cyclooxygenase-dependent pathway in contraction of isolated ileum by urotensin II. Peptides. 26, 323–329. doi:10.1016/j.peptides.2004.09.009
Horie, S., Tsutsumi, S., Takada, Y., and Kimura, J. (2008). Antibacterial quinone metabolite from the brown alga. Sargassum Sagamianum. Bull. Chem. Soc. Jpn. 81, 1125–1130. doi:10.1246/bcsj.81.1125
Hsu, W. H. (1982). Xylidine-induced delay of small intestinal transit in mice. Eur. J. Pharmacol. 83, 55–60. doi:10.1016/0014-2999(82)90285-0
Ibrahim, S. R., Mohamed, G. A., Elkhayat, E. S., Fouad, M. A., and Proksch, P. (2013). Sagitol C, a new cytotoxic pyridoacridine alkaloid from the sponge Oceanapia sp. Bull. Fac. Pharm. 51, 229–232. doi:10.1016/j.bfopcu.2013.05.004
Izzo, A. A., Mascolo, N., Capassco, R., Germano, M. P., De Pasuele, R., and Caspassco, F. (1999). Inhibitory effect of cannabinoid agonists on gastric emptying in the rat. Naunyn-Schmiedeberg’s Arch. Pharmacol. 360, 221–223. doi:10.1007/s002109900054
Jung, W. K., Jo, H. Y., Qian, Z. J., Jeong, Y. J., Park, S. G., Choi, I. W., et al. (2007). A novel anticoagulant protein with high affinity to blood coagulation factor Va from Tegillarca granosa. J. Biochem. Mol. Biol. 40, 832–838. doi:10.5483/BMBRep.2007.40.5.832
Kijjoa, A., Wattanadilok, R., Herz, W., Campos, N., Nascimento, M. S. J., and Pinto, M. (2007). Anticancer activity evaluation of kuanoniamines A and C isolated from the marine sponge Oceanapia sagittaria, collected from the gulf of Thailand. Mar. Drugs. 5, 6–22. doi:10.3390/md502006
Malyarenko, T. V., Malyarenko, O. S., Kicha, A. A., Ivanchina, N. V., Kalinovsky, A. I., Dmitrenok, P. S., et al. (2018). In Vitro anticancer and proapoptotic activities of steroidal glycosides from the starfish anthenea aspera. Mar. Drugs. 16, 420. doi:10.3390/md16110420
Movahhedin, N., Barar, J., Fathi-Azad, F., barzegari, A., and Nazemiyeh, H. (2014). Phytochemistry and biologic activities of Caulerpa Peltata native to Oman sea. Iran. J. Pharm. Res. (IJPR). 13, 515–521. doi:10.22037/IJPR.2014.1489
Nag, A., Martin, S. A., Mladsi, D., Olayinka-Amao, O., Purser, M., and Vekaria, R. M. (2020). The humanistic and economic burden of chronic idiopathic constipation in the USA: a systematic literature review. Clin. Exp. Gastroenterol. 13, 255–265. doi:10.2147/CEG.S239205
Ndikubwimana, J. B., and Ngendahimana, F. (2020). Risk factors associated with under-five diarrhea and their effect on under-five mortality in Rwanda: secondary data analysis of 2014-2015 Rwanda demographic and health survey.Available at: https://ssrn.com/abstract=3601648 or http://dx.doi.org/10.2139/ssrn.3601648 (Accessed May 26, 2020).
Nicholas, G. M., Hong, T. W., Molinski, T. F., Lerch, M. L., Cancilla, M. T., and Lebrilla, C. B. (1999). Oceanapiside, an antifungal bis-alpha,omega-amino alcohol glycoside from the marine sponge Oceanapia phillipensis. J. Nat. Prod. 62, 1678–1681. doi:10.1021/np990190v
OECD (2001). Acute oral Toxicity–Acute Toxic Class Method. OECD guideline for testing of chemicals. North America, Europe and the Pacific: OECD, 423.
Queiroz, T. M., Machado, N. T., Furtado, F. F., Oliveira-Filho, A. A., Alustau, M. C., Figueiredo, C. S., et al. (2011). Vasorelaxation, induced by Dictyota pulchella (Dictyotaceae), a brown alga, is mediated via inhibition of calcium influx in rats. Mar. Drugs. 9, 2075–2088. doi:10.3390/md9102075
Rath, B., Hochmair, M., Plangger, A., and Hamilton, G. (2018). Anticancer activity of fascaplysin against lung cancer cell and small cell lung cancer circulating tumor cell lines. Mar. Drugs. 16, 383. doi:10.3390/md16100383
Rembold, C. M. (1996). “Electromechanical and pharmacomechanical coupling,” in Biochemistry of smooth contraction. San Diego, CA: Academic Press.
Santos-Neto, C., Nascimento, E., Cavalcanti, T., and Pinheiro, U. (2018). Taxonomy of Oceanapia norman, 1869 (Demospongiae: Haplosclerida: phloeodictyidae) from the Brazilian coast. Zootaxa. 4455, 363–376. doi:10.11646/zootaxa.4455.2.6
Staneva-Stoytcheva, D., and Venkova, K. (1992). Effects of the calcium antagonists diltiazem, verapamil and nitrendipine on the contractile responses of Guinea-pig isolated ileum to electrical stimulation or carbachol. J. Pharm. Pharmacol. 44, 321–325. doi:10.1111/j.2042-7158.1992.tb03614.x
Than, A., Kulkarni, H. J., Hmone, W., and Tha, S. J. (1989). Antidiarrheal efficacy of some Burmese indigenous drug formulations in experimental diarrhea test models. J. Crude Drug Res. 27, 195–200. doi:10.3109/13880208909116903
Triggle, C. R., Swamy, V. C., and Triggle, D. J. (1979). Calcium antagonists and contractile responses in rat vas deferens and guinea pig ileal smooth muscle. Can. J. Physiol. Pharmacol. 57, 804–818. doi:10.1139/y79-124
Van Rossum, J. M. (1963). Cumulative dose-response curves. II. Technique for the making of dose-response curves in isolated organs and the evaluation of drug parameters. Arch. Int. Pharmacodyn. Ther. 143, 299–330.
Van Soest, R., Boury-Esnault, N., Hooper, J., Rützler, K., De Voogd, N., Alvarez DE Glasby, B., et al. (2015). World Porifera database. The world register of marine species (WoRMS). Available at: http://www.marinespecies.org/porifera (Accessed May 16, 2015).
Wang, B., deng, J., Gao, Y., Zhu, L., He, R., and Xu, Y. (2011). The screening toolbox of bioactive substances from natural products: a review. Fitoterapia. 82, 1141–1151. doi:10.1016/j.fitote.2011.08.007
Webb, R. C. (2003). Smooth muscle contraction and relaxation. Adv. Physiol. Educ. 27, 201–206. doi:10.1152/advan.00025.2003
World Health Organization (2016). Diarrhoeal disease. Available at: http://www.who.int/mediacentre/factsheets/fs330/en/ (Accessed January 13, 2016).
Keywords: marine sponge, Oceanapia magna, spasmolytic, spasmogenic, intestinal transit
Citation: Pereira JC, Figueiredo IAD, de Oliveira FRMB, Ferreira SRD, Aires Moreno GT, da Silva TMS, Pinheiro UdS, Santos BVdO, da Silva BA and Cavalcante FdA (2020) Oceanapia magna Sponge Presents Dual Effect on the Gastrointestinal Motility of Rodents: In Vitro and In Vivo Assays. Front. Pharmacol. 11:572574. doi: 10.3389/fphar.2020.572574
Received: 14 June 2020; Accepted: 23 November 2020;
Published: 23 December 2020.
Edited by:
Gianluca Ianiro, Catholic University of the Sacred Heart, ItalyReviewed by:
Raquel Abalo, Rey Juan Carlos University, SpainMaria Grazia Zizzo, University of Palermo, Italy
Copyright © 2020 Pereira, Figueiredo, de Oliveira, Ferreira, Aires Moreno, da Silva, Pinheiro, Santos, da Silva and Cavalcante. This is an open-access article distributed under the terms of the Creative Commons Attribution License (CC BY). The use, distribution or reproduction in other forums is permitted, provided the original author(s) and the copyright owner(s) are credited and that the original publication in this journal is cited, in accordance with accepted academic practice. No use, distribution or reproduction is permitted which does not comply with these terms.
*Correspondence: Fabiana de Andrade Cavalcante, ZmFiaWFuYWNhdmFsY2FudGVAbHRmLnVmcGIuYnI=