- 1Noncommunicable Diseases Research Center, Bam University of Medical Sciences, Bam, Iran
- 2Student Research Committee, School of Medicine, Bam University of Medical Sciences, Bam, Iran
- 3Phytochemistry Research Center, Shahid Beheshti University of Medical Sciences, Tehran, Iran
- 4Dipartimento di Bioscienze, Università degli Studi di Milano, Milano, Italy
- 5Department of Internal Medicine, University Hospital Centre Zagreb, School of Medicine, University of Zagreb, Zagreb, Croatia
- 6Faculty of Allied Health Sciences, University Institute of Diet and Nutritional Sciences, The University of Lahore, Lahore, Pakistan
- 7Department of Pharmacognosy, Faculty of Pharmacy, Gazi University, Ankara, Turkey
- 8Department of Pharmacognosy, Faculty of Pharmacy, Ain-Shams University, Cairo, Egypt
- 9Department of Pharmaceutical Biology, Faculty of Pharmacy and Biotechnology, German University in Cairo, Cairo, Egypt
- 10Department of Nutrition and Dietetics, Faculty of Pharmacy, University of Concepcion, Concepcion, Chile
- 11Unidad de Desarrollo Tecnológico, Universidad de Concepción UDT, Concepcion, Chile
- 12Department of Toxicology, University of Medicine and Pharmacy of Craiova, Craiova, Romania
- 13Department of Clinical Pharmacy, University of Medicine and Pharmacy of Craiova, Craiova, Romania
- 14Department of Botany, University of Fort Hare, Alice, South Africa
Anthocyanins are natural phenolic pigments with biological activity. They are well-known to have potent antioxidant and antiinflammatory activity, which explains the various biological effects reported for these substances suggesting their antidiabetic and anticancer activities, and their role in cardiovascular and neuroprotective prevention. This review aims to comprehensively analyze different studies performed on this class of compounds, their bioavailability and their therapeutic potential. An in-depth look in preclinical, in vitro and in vivo, and clinical studies indicates the preventive effects of anthocyanins on cardioprotection, neuroprotection, antiobesity as well as their antidiabetes and anticancer effects.
Introduction
Anthocyanins (ACNs) are natural bioactive water-soluble phenolic compounds, which represent one of the principal families of natural pigments (orange, red, violet, and blue colors) (Riaz et al., 2016). More than 700 ACNs were identified in nature, and they are produced by plants to attract insects to flowers for pollination and herbivorous animals to fruits for seed dissemination, as well as for the protection of plant cells against UV radiation damage (Markakis, 2012; Wallace and Giusti, 2013; Warner, 2015). ACNs are widely found in different plant families, for example, Vitaceae, Rosaceae, Ericaceae, Saxifragaceae, Caprifoliaceae, Cruciferae, and Fabaceae (Mazza and Miniati, 2018). The name is derived from the Greek word anthos, which means flower and kyanos (blue) (Sui, 2016). ACNs are a subclass of flavonoids and are distributed in different parts of the plants, especially in flowers and fruits (Gupta, 2006; Pascual-Teresa et al., 2010; Wallace and Giusti, 2013; Riaz et al., 2016).
ACNs are natural bioactive compounds with many pharmacological effects: antioxidant, antiinflammatory, prevention of age-related chronic diseases: cardiovascular disease (CVD), cancers, neurodegenerative, and eye-related diseases. ACNs also have antiviral properties. Recent in vitro studies have shown that they can inhibit the replication of viruses such as herpes simplex, parainfluenza virus, syncytial virus, HIV, rotavirus, and adenovirus (Pour et al., 2019).
The broad spectrum of pharmacological properties supported by preclinical and clinical evidence, associated with a low toxicity make their pharmacotherapeutic use very attractive.
ACNs are used in the food industry to replace synthetic colorants (Mazza and Miniati, 2018).
The current review comprehensively analyzes different studies performed on this class of compounds, their bioavailability, the therapeutic potential, molecular mechanisms of action, as well as their clinical significance in the prevention of chronic noncommunicable diseases.
Modern Technology of Extraction, Stability, and Bioavailability of ACNs
Extraction of ACNs
ACNs are a group of phytochemicals that are evaluated as important compounds in nutrition and medicine. As plant metabolites, they are known to be beneficial to health by acting as antioxidants and having antiinflammatory effects. Due to these beneficial effects on health, flavonoids are used in nutraceutical, pharmaceutical, medicinal and cosmetic production. ACNs molecules are found in a variety of fruits, vegetables, grains, roots, stems, leaves, flowers, and bark. In order to process ACNs as nutritional supplements, pharmaceuticals or as active ingredients in food or cosmetics, ACNs must be released from the plant cell matrix.
Therefore, a strong, efficient, and reliable extraction method is needed.
Ultrasonic extraction is a nonthermal insulation technique that prevents thermal decomposition of heat sensitive compounds. Ultrasound extraction promotes the release of high quality ACNs from plants resulting in higher yields and a faster process. Sonicare is a light, green and efficient technique for the industrial production of food ACNs (Belwal et al., 2019)
Advantages of ultrasound extraction: higher yields, fast extraction process: in a few minutes high quality extracts—easy, nonthermal extraction green solvents (water, ethanol, glycerin, vegetable oils, etc.), easy and safe operation, low operating, and investment costs, robustness and low maintenance.
Enzymatic methods are more efficient because they offer the possibility of being exploited high regioselectivity of these biocatalysts, achieving a selective functionalization of the flavonoid.
Stability of ACNs
Therapeutic use of ACNs is limited by reduced stability and their low solubility, both in organic solvents and in aqueous solutions. However, ACNs can be converted to glycosylated or acylated derivatives by chemical, enzymatic or chemoenzimatics methods.
The transformation of ACNs into bioconjugates by fatty acid acylation offers the possibility to introduce into the molecule another biologically active function and thus positively modify not only physical properties such as solubility, but and biological activity.
The protection and especially the controlled release of various organic molecules is achieved almost exclusively by means of encapsulated compounds. For example, cyclodextrins are part of the most used class receptors in host-guest inclusion chemistry. Advantages of encapsulation in cyclodextrins of active substances biological effects are: improving bioavailability, increasing stability, reducing side effects.
Bioavailability of ACNs
The chemical structure of ACNs is composed of an aglycone basic unit (polyhydroxy and polymethoxy derivatives of 2-phenylbenzopyrylium or flavylium salts) and a glycone, the sugar moiety. The position, nature and number of sugar moieties and their acylation as well as the position and number of hydroxyl groups and their degree of methylation result in various types of ACNs (Jackman et al., 1987; Mazza and Miniati, 2018).
Pelargonidin, delphinidin, cyanidin, peonidin, petunidin, and malvidin (Figure 1) are the most frequently occurring anthocyanidins in plants. Similar to other flavonoids, ACNs are characterized by the same carbon skeleton (C6-C3-C6). Acylated ACNs consist of an additional organic acid unit usually bonded to the sugar at the C-3 position (Markakis, 2012).
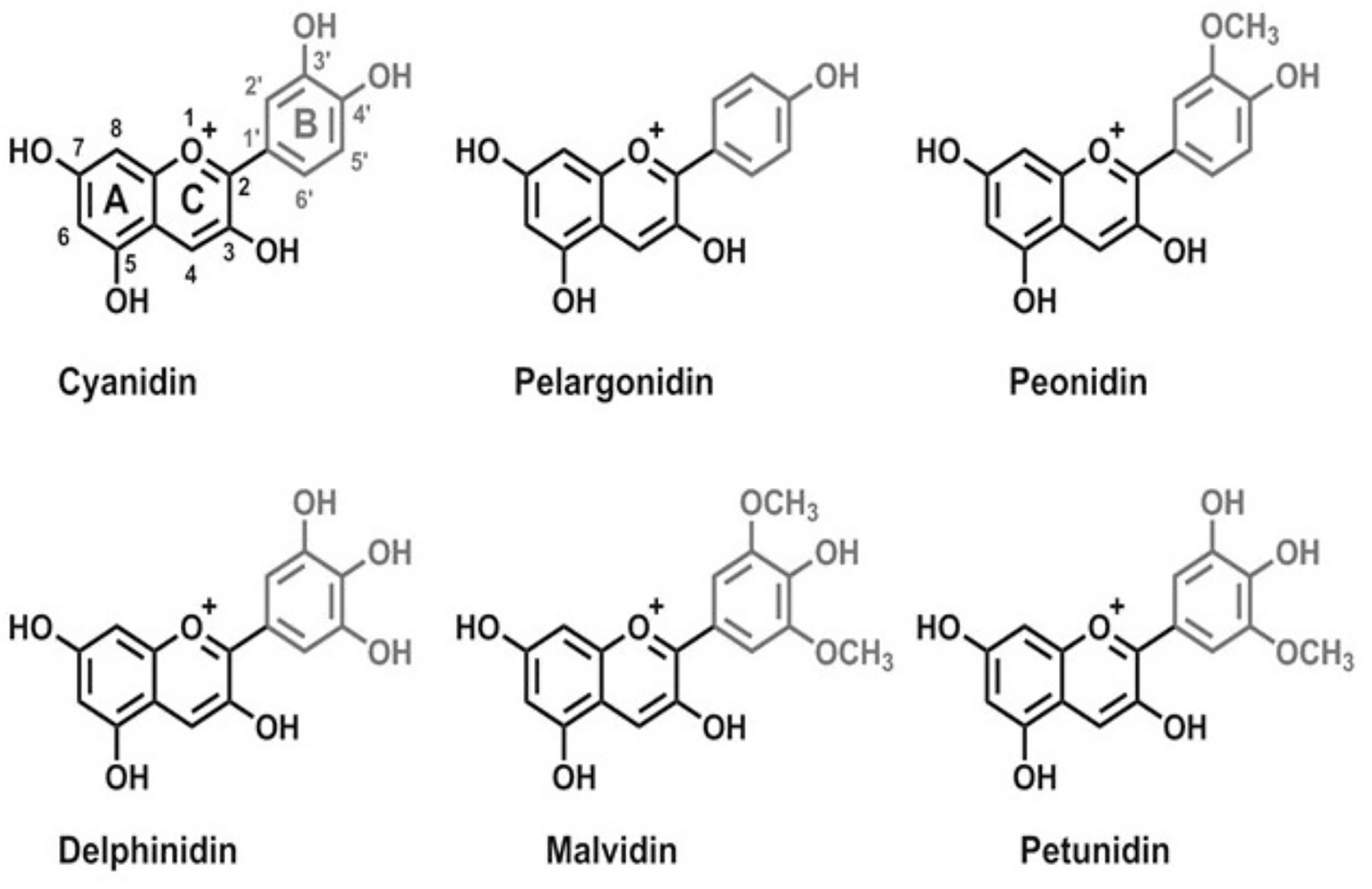
Figure 1 Chemical structures of main anthocyanins: pelargonidin, cyanidin, peonidin, delphinidin, malvidin, and petunidin.
The oral bioavailability of ACNs is poor and it is correlated with their stability, lack of site-specificity in distribution, rapid elimination and clearance from the body, and the dietary source of the compound and food matrix interactions (Milbury et al., 2010).
Gastric administration of ACNs in rats demonstrated that they are initially absorbed in the stomach (about 25%) through a bilitranslocase-mediated mechanism and can be detected in their native form in plasma within 6 min. In contrast, they can be found as methylated and conjugated derivatives in bile within 20 min, suggesting that ACN metabolites are rapidly formed in the liver and eliminated via bile, but not distributed in blood (Passamonti et al., 2003; Talavera et al., 2003).
ACNs are also rapidly absorbed (12%–15%) in the small intestine of rats and found in their natural form and methylated derivatives in plasma within 25 min, but also excreted into bile and urine as intact glycosides or methylated/glucuronidated derivatives (Talavera et al., 2004). Absorption of ACNs in the small intestine may occur by deglycosylation to aglycones followed by passive transport through intestinal epithelium (Kay, 2006) or by an active transport mechanism using intestinal sodium-dependent glucose transporter 1 (SGLT1) or bilintranslocase (Walton et al., 2006a; Passamonti et al., 2009).
Most ACNs in the blood are found in the form of their metabolites which have a lower pharmacological activity than the primary compounds. (Walton et al., 2006a; Passamonti et al., 2009).
The instability, high reactivity and low extraction possibility limit their potential applications of ACNs in food and pharmaceutical industries (Castañeda-Ovando et al., 2009).
Pharmacological Activities of ACNs: An Overview on Molecular Mechanisms
Neuroprotective Effects
Due to its high demand of energy and high lipid content, the central nervous system (CNS), especially the brain, is particularly susceptible to excessive reactive oxygen species (ROS) (Nussbaum et al., 2017) (Figure 2). A high production of oxygen leads to a high production of intracellular ROS during cellular respiration within mitochondria (Salehi et al., 2019c). In addition, exogenous sources of ROS may be environmental pollution, smoking, unhealthy diet, UV-B radiation, drug metabolites and infections (Singh et al., 2019). In the CNS, a specific innate immune system, consisting of resting or activated glial cells, protects the nervous system against pathogens or injuries, but an excessive or prolonged inflammatory response may contribute to neuronal apoptosis and may facilitate the progression of neurodegenerative diseases (Russo and Mcgavern, 2015)
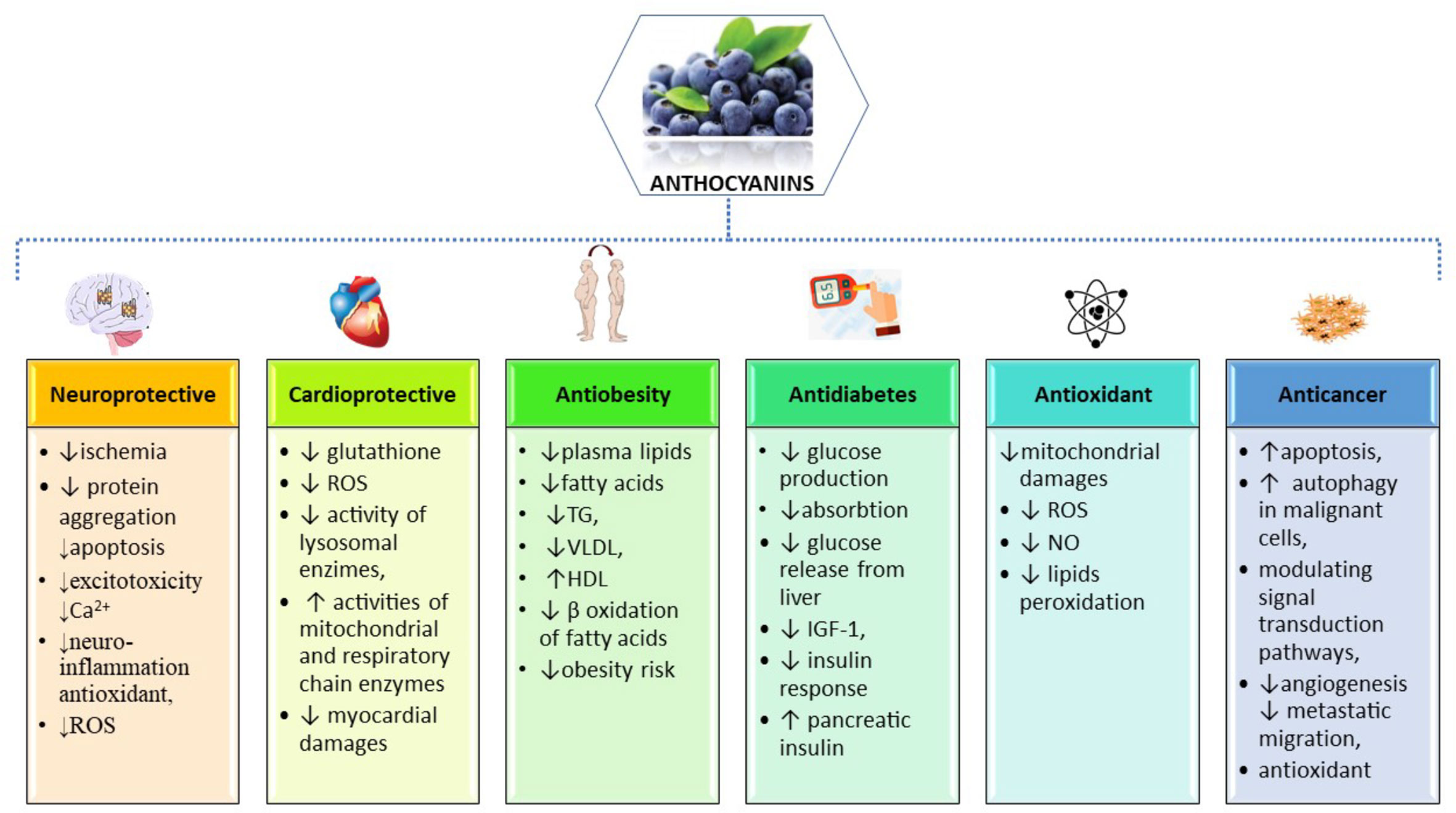
Figure 2 Summarized scheme of the most important pharmacological properties and molecular mechanisms of action of anthocyanins.
Many dietary ACNs contain multiple ACNs compounds with neuroprotective effects. Dietary ACNs from blue corn protected brain from the mitochondrial DNA common deletion (mtDNA CD) induced by a moderate ethanol consumption (Demeilliers et al., 2017). Finally, we showed that the administration of ACN-rich purple corn extract has a protective effect on the development of orofacial allodynia in an in vivo model of inflammatory trigeminal pain, and that it reduces trigeminal macrophage infiltration and microglial activation both in vivo and in vitro (Magni et al., 2018). The neuroprotective effect of purple corn is comparable to the antiinflammatory effects of acetyl salicylic acid, which does not modify microglia activation.
Therefore, a possible application of ACN-rich dietary supplements as co-adjuvant therapy to pharmacological treatment or as a preventive strategy against trigeminal pain, aimed at reducing drugs dosage and adverse effects might be proposed.
Perspectives on Pathophysiology of Neurodegenerative Disorders and Cerebral Ischemia
Neurodegenerative Disorders: A Brief Overview on Pathophysiology
These are a group of chronic diseases characterized by a progressive loss of neurons in brain or spinal cord, leading to a progressive impairment in cognitive and motor functions and ultimately resulting in severe disability (Calina et al., 2020; Sharifi-Rad et al., 2020b). Chronic neurodegenerative diseases include Alzheimer’s disease (AD), Parkinson’s disease (PD), and amyotrophic lateral sclerosis (ALS) (Jellinger, 2009).
Neurodegenerative disorders and cerebral ischemia are associated to three common factors triggering the onset of neuronal apoptosis, (Jellinger, 2009; Li et al., 2019) which is the main mechanism responsible for neuron loss:
i. oxidative stress
ii. excitotoxicity
iii. neuroinflammation
Oxidative stress is due to a significant reduction in the ability of neuronal cells to scavenge excessive ROS, leading to oxidative damage of macromolecules (i.e. DNA oxidation, protein carbonylation, and lipid peroxidation) and to mitochondrial dysfunction, additional generation of ROS and neuronal apoptosis (Mariani et al., 2005; Singh et al., 2019). Increased oxidative stress and mitochondrial dysfunction precede the deposition of neurotoxic amyloid-β (Aβ) protein aggregates, a typical hallmark of AD (Salehi et al., 2020a). They also cause the formation of the Lewy bodies, insoluble inclusions mainly consisting of damaged α-synuclein, associated with neuron loss and dopamine deficiency in the substantia nigra of PD patients (Wang et al., 2014; Puspita et al., 2017).
Excitotoxicity is a common pathogenic factor in neurodegenerative diseases (Dong et al., 2009; Mattson, 2019). Excitotoxicity consists of the overstimulation of glutamate receptors [i.e., N-methyl-D-aspartic acid (NMDA) and α-amino-3-hydroxy-5-methyl-4-isoxazolepropionic acid (AMPA)], causing an excessive influx of calcium ions from the extracellular space (Dong et al., 2009; Mattson, 2019). This calcium overload triggers intracellular signaling cascades, leading to mitochondrial depolarization, increased ROS and nitric oxide (NO) production, degradation of macromolecules and ultimately apoptosis, thus indicating the existence of a link between excitotoxicity and oxidative stress (Dong et al., 2009; Mattson, 2019). Upregulation of NMDA receptors has been associated to Aβ deposition in AD (Parameshwaran et al., 2008), whereas an overstimulation of AMPA receptors with kainic acid in rats specifically promoted loss of motor neurons, which are particularly rich in AMPA receptors, thus suggesting a specific role of excitotoxicity in ALS (Sun et al., 2006).
Neuroinflammation may be also triggered as a response to the aberrant deposition of protein aggregates, such as Aβ in AD, α-synuclein in PD and DNA-binding protein-43 in ALS (TDP-43). (Zhang et al., 2005; Stewart et al., 2010; Van Langenhove et al., 2012).
Cerebral Ischemia: A Brief Overview on Pathophysiology
This is a condition in which the brain does not receive enough blood to meet its metabolic needs. Thus, the resulting lack of oxygen can cause the death of brain tissue and therefore an ischemic stroke (Tsatsakis et al., 2019). Cerebrovascular ischemia causes loss of neurons in localized regions of brain by mechanisms similar to those in neurodegenerative diseases (Chen et al., 2011).
Oxidative stress caused by reperfusion following cerebrovascular ischemia triggers the production of excessive superoxide radicals by mitochondria with increasing ROS and mitochondrial dysfunction. These superoxide radicals combined with NO, produced by ischemia-induced neuronal NO synthase (nNOS), generate reactive nitrogen species (i.e. peroxynitrite) that further damage neuronal cellular proteins by nitrosylation (Eliasson et al., 1999).
Excitotoxicity is also a pathogenic factor in cerebrovascular ischemia. Massive release of presynaptic glutamate caused by cerebrovascular ischemia causes a consequent increase in NMDA post-synaptic receptors (Szydlowska and Tymianski, 2010; Li Y. et al., 2016) and finally loss-of-function mutations in the parkin gene (hereditary PD PARK2 gene) inducing a proliferation of glutamate post-synaptic receptors, thus causing a sensitization to excitotoxicity in the substantia nigra in PD (Helton et al., 2008).
Neuroinflammation in cerebral ischemia: neurons injured by cerebral ischemia release cytokines and chemokines, that activate resting microglial cells to secrete antiinflammatory neuroprotective cytokines, in order to assist in repairing neuronal cells, but also to promote the synthesis of proinflammatory cytokines (e.g,. IL-1β and TNF-α), iNOS and the production of NO, a reactive molecule which destroys invading pathogens (Di Filippo et al., 2010; Buga et al., 2019; Subhramanyam et al., 2019). In case of prolonged production, the inflammatory response may result in neuronal damage and finally in apoptosis (Block and Hong, 2007).
Mechanistic and Molecular Aspects of Neuroprotective Effects of ACNs
Neurodegenerative Diseases
These studies have reported the neuroprotective effect of ACNs in preclinical models of neurodegenerative diseases by multiple mechanisms (Tsuda, 2012; Ullah et al., 2019).
Antioxidant Mechanisms:
i. The neuroprotective effect is accomplished because of rapid absorption of ACNs and their capacity to cross the blood brain barrier (BBB). Since they can reach the brain in their native form, ACNs can exert their antioxidant activity as direct scavengers of ROS (Shih et al., 2011; Casedas et al., 2018).
ii. They can also activate the antioxidant response by promoting the nuclear translocation of nuclear factor erythroid 2–related factor 2 (Nrf2) or by stimulating the activity of antioxidant enzymes, such as SOD, CAT and GPx (Casedas et al., 2018; Pacheco et al., 2018).
In vitro studies have demonstrated that ACNs prevent the intracellular calcium overload, thereby causing excitotoxicity and the progression of neurodegenerative diseases (Ye et al., 2010; Shih et al., 2011; Ullah et al., 2014; Badshah et al., 2015).
AntiNeuroinflammation Mechanisms:
i. Inhibit the nuclear translocation of NF-κB, thereby preventing the activation of proinflammatory molecules, such as COX-2, iNOS, IL-1β, TNF-α (Kim et al., 2017).
ii. Reduce the intracellular signaling pathways of mitogen-activated protein kinases (MAPKs): c-Jun N-terminal kinase (JNK) and P38 mitogen-activated protein kinases (p-38 MAPK), also reducing the activation of proinflammatory cytokines (Amin et al., 2017).
iii. Antiinflammatory effect, by inhibiting the activity of COX-2 enzyme (Mulabagal et al., 2009).
Antiapoptotic Mechanisms:
i. Prevent the release of apoptosis-inducing factor (AIF) from mitochondria and its migration into the nucleus, where it triggers DNA fragmentation by a caspase-independent pathway (Min et al., 2011),
ii. to increase the expression of the proapoptotic factor B cell lymphoma-2 (Bcl-2),
iii. to reduce the expression of the antiapoptotic factor B-cell lymphoma protein associated X (Bax) (Ali Shah et al., 2013; Khan M.s. et al., 2019).
These have proved that ACNs can prevent the onset and progression of neurodegenerative diseases as well as that they can improve learning and memory in aging model mice.
The first evidence of the neuroprotective effect of ACNs was obtained when 19 months-old aged rats (comparable to 60 years old humans) fed with blueberry extracts for 8 weeks showed a significant improvement in motor function in the accelerated rotarod test and effectively reversed age-related deficits in neuronal and cognitive function in a test of learning and memory, such as the Morris water performance (Joseph et al., 1999). More recently, similar results were obtained in galactose-induced aging models or aged rats fed with ACNs, showing a delayed age-related decline in spatial learning and memory (Andres-Lacueva et al., 2005; Rehman et al., 2017; Wei J. et al., 2017).
Concerning Alzheimer diseases (AD), the APP/PS1 transgenic mouse, carrying mutations in amyloid precursor protein (APP) and presenilin-1 (PS1), fed with blueberry extract from 4 months of age showed no deficits in Y-maze performance (at 12 months of age) with no difference in Aβ plaques compared to nontransgenic mice (Joseph et al., 2003). Since then, it was shown that dietary ACNs from mulberry extracts applied to a senescence-accelerated mouse model of AD (SAMP8) reduced Aβ plaques and improved learning and memory ability in avoidance response tests, by activating the Nrf2-dependent antioxidant defense system (Shih et al., 2010).
Using an Aβ-induced model of AD in rats, intragastrically applied ACNs from black soybean were shown to reverse Aβ-induced neuronal apoptosis by suppressing protein expression of intrinsic apoptotic pathway (Bax, cytochrome C, caspase-9 and caspase-3) (Badshah et al., 2015). More recently, dietary supplementation of ACNs from Korean black beans to the APP/PS1 mouse model of AD demonstrated that ACNs reduce oxidative stress induced by Aβ aggregation by activation of the p-PI3K/Akt/GSK3β pathway, which has been found to promote nuclear translocation of Nrf2 and the activation of HO-1 and glutathione cysteine ligase modulatory subunit (GCLM) target genes.
ACNs prevent apoptosis and neurodegeneration by suppressing the activation of caspase-3. As a result, memory-related pre- and post-synaptic protein markers and memory functions in both Morris water maze and the Y-maze tests were improved (Ali et al., 2018).
Concerning Parkinson Disease, an interesting epidemiological study highlighted that a regular intake of ACNs, based on consuming strawberries and blueberries, results in a significantly lower PD risk (Gao et al., 2012). In a MPTP-induced mouse model of PD, oral supplementation of ACNs from a mulberry extract showed a significant reduction in bradykinesia, in loss of dopaminergic neurons in substantia nigra and in depletion of dopamine depletion, related to a reduced expression of the proapoptotic Bax protein (Kim et al., 2010). Studies in rotenone-induced cell models of PD suggest that ACNs attenuate mitochondrial dysfunction by reducing the rotenone-induced damage of mitochondrial complex I of the electron transport system, and reduce neuroinflammation resulting from microglial activation, thus preserving dopaminergic neurons (Strathearn et al., 2014).
Only one study assessed the potential of ACNs in preventing the onset and progression of ALS, characterized by loss of motor neurons in brain, brainstem and spinal cord (Winter et al., 2018). Oral administration of an ACN-rich extract from strawberries in a mouse model of ALS, carrying a G93A mutation in the human SOD1 gene (hSOD1G93A), modestly but significantly delayed the onset of ALS (about 17 days) and extended the survival (about 11 days) when to untreated hSOD1G93A mice, in which ALS onset occurred at about 90 days of age and progress to end-stage of disease at about 120 days age. Supplementation with ACNs significantly preserved grip strength and neuromuscular junctions in gastrocnemius muscle, but did not prevent motor neuron loss in spinal cord. On the other hand, a significant reduction in neuroinflammation (i.e. activated astrocytes) was observed in spinal cord (Winter et al., 2018).
Multiple sclerosis (MS) is a result of a neuronal demyelination process and not neuron loss, but shares some common pathological mechanisms with neurodegenerative diseases(Padureanu et al., 2019). Recent studies have highlighted that ACNs have a protective effect on the onset and progression of MS by reducing oxidative stress, neuroinflammation and the activity of ion pumps. (Carvalho et al., 2015).
ACNs reduced demyelination in a rat model of MS by restoring glutathione level and SOD activity, suggesting that a possible Nrf2-mediated antioxidant mechanism of protection may occur (Carvalho et al., 2015). In the same study, ACNs were found to reduce infiltration of inflammatory cells, the expression of proinflammatory cytokines, such as IL-1β and TNF-α, to increase the expression of antiinflammatory cytokines, like IL-10, and finally to increase the expression of Na+, K+-ATPase and Ca2+-ATPase, thus restoring neuronal functions (Carvalho et al., 2015).
Other neuroprotective effects of ACNs were recently demonstrated in animal models of neurotoxicity or inflammatory pain.
Cerebral Ischemia
Antioxidant defense in cerebral ischemia: ACNs promote activation of Nuclear factor erythroid 2-related factor 2 (Nrf2) and the consequent increase in the expression of the Heme oxygenase-1 (HO-1) and γ-glutamyl cysteine synthase (γ-GCS) genes, contributing to decrease brain levels of superoxide and lipid peroxidation (Min et al., 2011; Di Giacomo et al., 2012; Cui et al., 2018) (Figure 3).
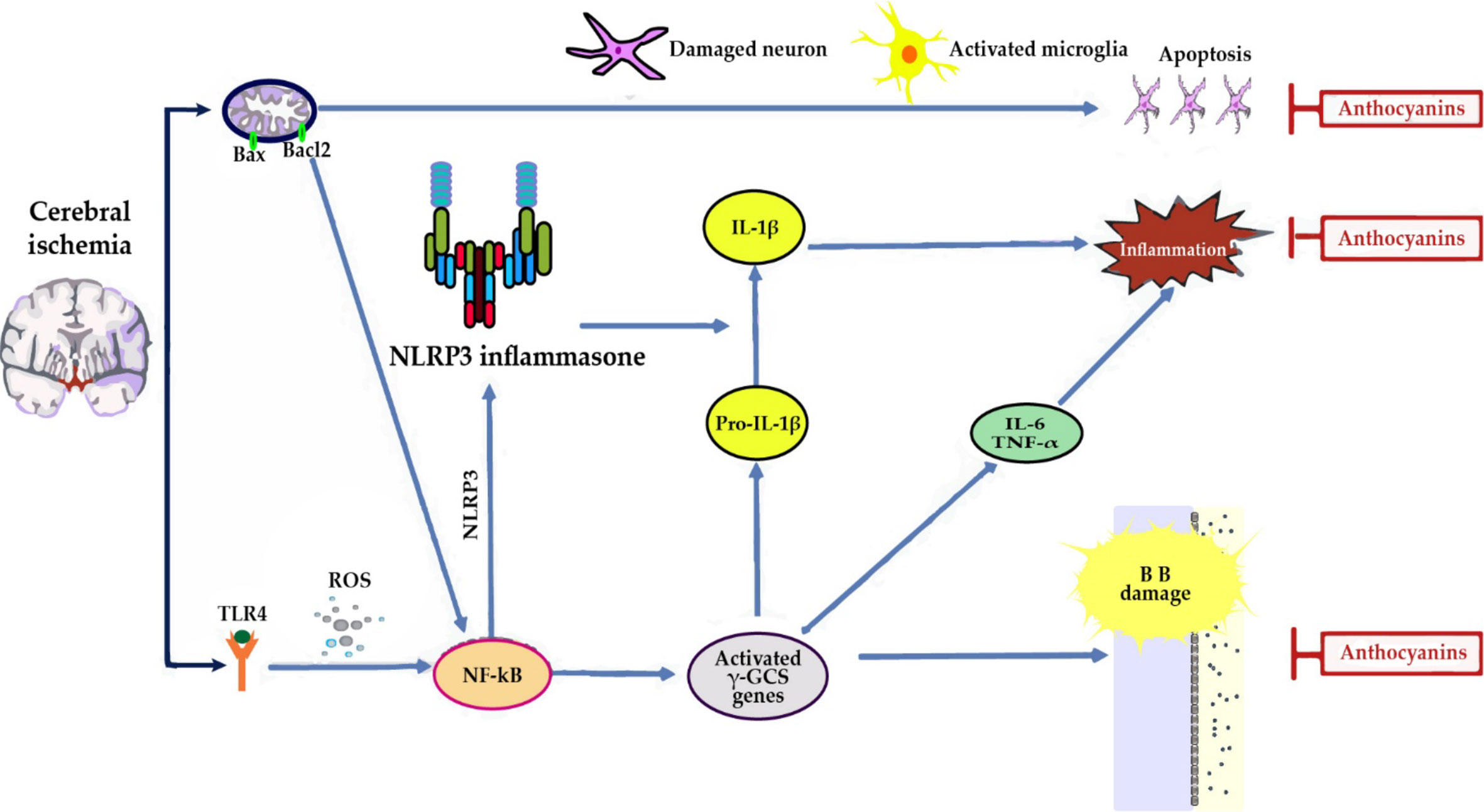
Figure 3 In cerebral ischemia, anthocyanins (ACNs) reduce neuroinflammation by: (i) decreasing the expression of Toll-like receptor 4 (TLR4), an activator of nuclear factor kappa B (NF-κB), and tumor necrosis factor-α (TNF-α) proinflammatory cytokine expression (Cui et al., 2018). (ii) Reducing the expression of inducible nitric oxide synthase (iNOS) and neuronal nitric oxide synthase (nNOS), targets of NF-κB, and as a consequence NO content at cerebral level, thus reflecting a reduction in brain damage (Di Giacomo et al., 2012; Cui et al., 2018). A significant increase of eNOS was observed which may result in vasorelaxation with a consequent attenuation of the ischemic insult and promotion of functional recovery of the ischemic zone (Di Giacomo et al., 2012). (ii) Direct antiapoptotic role, since they partially blocked AIF, but not cytochrome c release from mitochondria, thus indicating that guanine nucleotide exchange factor (C3G) reduced apoptosis by suppressing a caspase-independent pathway (Min et al., 2011).
In addition, Nrf2 activation has been recently found to inhibit nod-like receptor protein 3 (NLRP3) inflammasome by regulating the thioredoxin1/thioredoxin interacting protein complex (TRX1)/TXNIP (Hou et al., 2018). This complex inhibits caspase-1, IL-6 and IL-1β activation, thereby inhibiting apoptosis and inflammatory responses and reducing brain damage (Cui et al., 2018; Padureanu et al., 2019; Mititelu et al., 2020).
These studies have shown the neuroprotective effect of ACNs in rat or mouse models of cerebral ischemia and defined some of the possible mechanisms of protection (Zhang et al., 2019).
Pre-treatment of middle cerebral artery occlusion (MCAo) rat models with orally administered high dose of ACNs (up to 300 mg/kg) significantly reduced cerebral infarct size (Shin et al., 2006; Cui et al., 2018). On the other hand, both pre-treatment (1h) and post-treatment (i.e. during reperfusion) with lower doses of purified C3G (2 mg/kg i.p administered or 10 mg/kg orally administered) also efficiently reduced cerebral infarct size in MCAo rat model (Min et al., 2011) and increased the survival rate at 24 h post transient cerebral ischemia obtained due to bilateral carotid occlusion (Di Giacomo et al., 2012).
CVD Protection
The beneficial effects of dietary ACNs in the prevention of CVDs were shown by several epidemiological studies. Preventive effects of dietary ACNs concerning hypertriglyceridemia, hypercholesterolemia (total cholesterol and LDL-cholesterol) and platelet hyperactivity were reported in rat models of atherosclerosis induced by high-fat or high-fructose diets (Guo et al., 2007; Salgado et al., 2010; Yang et al., 2011) (Figure 2). In addition to their protective effect against atherosclerosis, ACNs also have protective effects at cardiac level against oxidative stress observed in ischemia/reperfusion condition. In rats fed with ACN-rich corn, the cardiac tissue damaged by ischemia was reduced for 39% when compared to rats fed with ACN-free corn (Toufektsian et al., 2008).
Similar results on CVD-related biomarkers were obtained in several human intervention studies on patients taking berries or purified ACNs, showing significantly increased HDL-cholesterol, reduced LDL-cholesterol, triglycerides, blood pressure, flow-mediated dilation and inflammatory markers (García-Conesa et al., 2018; Luís et al., 2018).
Higher ACN intake from berries significantly lowered to 32% the risk of myocardial infarction in young and middle-aged women (Cassidy et al., 2013; Cassidy et al., 2016) and decreased the incidence of coronary heart disease (CHD) and CVD-related mortality (Mink et al., 2007; Mccullough et al., 2012).
Other studies suggested an inverse correlation between high ACN consumption and CVD-related risk biomarkers, such as lower arterial stiffness and blood pressure (Cassidy et al., 2011; Jennings et al., 2012), reduced levels of C-reactive protein (Sesso et al., 2007; Chun et al., 2008) and reduced overall inflammation score (Jennings et al., 2014; Cassidy et al., 2015).
The cardioprotective effect of dietary ACNs may be attributed to: increase in plasma antioxidant capacity and NO levels; reduction of LDL oxidation and platelet aggregation (Erlund et al., 2008; Basu et al., 2010b; Chiva-Blanch et al., 2012; Santhakumar et al., 2015; Zhang et al., 2016; Thompson et al., 2017a; Thompson et al., 2017b).
Cardioprotection was associated with enhanced glutathione levels in pre-ischemic myocardial and marine omega-3 levels in the blood, suggesting that a physiological dose of dietary ACNs (12 mg/kg body weight/day) increase antioxidant effects and omega-3 fatty acids synthesis (Toufektsian et al., 2011).
Consistent with these results, ex vivo cardiac perfusion with low concentrations of bilberry ACNs resulted in a strong cardioprotective activity following ischemia/reperfusion, as shown by the low release of LDH and decrease of incidence and duration of reperfusion arrhythmias. On the contrary, high concentrations of ACNs resulted in a cardiotoxic effect, indicating a concentration-dependent cardioprotection of bilberry ACNs (Ziberna et al., 2010).
The cardioprotective activity is mediated by direct intracellular transport of C3G by the bilirubin-specific plasma membrane transporter bilitranslocase, as demonstrated by the lack of cardioprotective activity of C3G when antibodies against bilitranslocase were used before ischemia reperfusion. This shows that the entry of C3G (or other ACNs) into the endothelium is necessary to interact with intracellular targets and trigger an antioxidant response in cells or isolated organs (Ziberna et al., 2012).
CVDs: Brief Data on Pathophysiology
CVDs including diseases of the heart, blood vessels and the cerebrovascular system, are the killer number one, accounting for 31% of deaths worldwide (Canon, 2013).
Among CVDs, myocardial infarction, ischemic heart disease and stroke are primarily caused by atherosclerotic plaques, progressively growing and causing arterial stenosis or obstruction or eventually plaque rupture and thrombus formation (Sharifi-Rad J. et al., 2020). The process of atherosclerosis is mainly caused by oxidized low-density lipoproteins (oxLDL), typically associated to elevated plasma total- and LDL-cholesterol levels. LDL particles adhere to arterial walls and enter into the intima where they are retained and where resident macrophages and macrophages originating from circulating monocytes which bind to adhesion molecules and also enter the intima phagocytize them. Macrophage activation involves ROS production, which in turn triggers the activation of NF-κB and thereby proinflammatory cytokines and adhesion molecules causing also endothelial dysfunction. As a consequence, endothelial cells express on their surface intercellular adhesion molecule-1 (ICAM-1) and vascular adhesion molecule-1 (VCAM-1), thus attracting monocytes from the bloodstream. Once in the intima, monocytes differentiate into macrophages and massively phagocytize oxLDLs, thus becoming “foam cells”, recruiting other monocytes by releasing the chemokine monocyte chemoattracting protein-1 (MCP-1) and activated macrophages secrete proatherogenic growth-factors and cytokines.
The progression of atherosclerotic plaques involves further lipid accumulation from LDL particles, but also migration of smooth muscle cells from media to intima and their proliferation, collagen deposition, which then form a fibrous cap which covers the lipid core of the plaque. In advanced lesions, macrophage foam cells undergo apoptosis but are not effectively cleared by macrophages (defective efferocytosis), initiating a secondary cellular necrosis process and overtime to the formation of a necrotic lipid core (Moore and Tabas, 2011).
Inflammatory cells at the shoulders of the plaque cause the release of collagenases and elastase from the foam cells which might cause rupturing of the atheroma fibrous cap, platelet aggregation and thrombus formation.
Cardioprotective Mechanisms:
i. Prevention of atherosclerosis using apolipoprotein E (apoE)-deficient mice, a model characterized by hypercholesterolemia and plaques on aorta with morphological features similar to human advanced atherosclerotic lesions,
ii. those directed toward cardioprotection using Langendorff ischemia/reperfusion injury and drug-induced cardiotoxicity and/or myocardial infarction.
In Vivo Studies
Concerning the apo E-deficient mice, supplementation of ACN-rich diet, extracts or ACN metabolites (e.g., protocatechuic acid) showed a significant decrease in the formation of atherosclerotic plaques (Xia et al., 2003; Xia et al., 2006; Mauray et al., 2009; Wang et al., 2010; Jiang et al., 2017; Joo et al., 2018), associated to an improvement of dyslipidemia, such as increased HDL-cholesterol (Xia et al., 2003), reduced triglycerides (Xia et al., 2006; Jiang et al., 2017), reduced total cholesterol and LDL-cholesterol in serum (Xia et al., 2003; Xia et al., 2006; Jiang et al., 2017; Joo et al., 2018) and in plaques on aorta (Xia et al., 2003; Wang et al., 2010).
In apoE-deficient mice, ACN supplementation protects LDL-cholesterol from oxidation, as proven by reduced levels of serum anti-oxLDLs (Xia et al., 2003) and of lipid peroxidation markers, malondialdehyde (MDA) and F2-isoprostane (Wu et al., 2010; Jiang et al., 2017). The reduced formation of oxLDLs may be partly accomplished by a direct scavenging activity of ACNs embedded in cellular membranes or in the cytosol of endothelial cells, where they are transported by a bilitranslocase transporter (Youdim et al., 2000; Youdim et al., 2002; Maestro et al., 2009), but this may more probably result from an increase of gene expression and activity of antioxidant enzymes, such as SOD1, SOD2, glutathione reductase (GR), thioredoxin reductase 1 (TrxR1), paraoxonase 1 (PON1) in the aorta (Wu et al., 2010) and serum SOD1, GPx as well as CAT (Jiang et al., 2017). Lower levels of oxLDLs resulted in lower expression of VCAM-1 and ICAM-1 in aorta (Wang et al., 2010; Wu et al., 2010) and lower inflammatory response, reduced activation of NF-kB and iNOS gene expression and protein levels in the aorta (Xia et al., 2003; Xia et al., 2006; Wang et al., 2010), reduced leukocyte infiltration and circulating proinflammatory cytokines (Xia et al., 2003; Joo et al., 2018).
Daily supplementation of a physiological dose of C3G (10 mg/kg body weight/day) for one week prior to surgery and eight weeks post-surgery in a rat model of myocardial infarction was able to significantly prevent cardiac dilation and improve cardiac function until four weeks after myocardial infarction. Nevertheless it was unable to sustain this cardioprotection since cardiac dysfunction was not significantly improved after 8 weeks (Raj et al., 2017).
Physiological doses of ACNs were also effective against cardiotoxicity induced by the chemotherapeutic drug doxorubicin (Dox) since in mice fed with dietary ACNs from purple corn treated with Dox, medium-term but not long-term survival was improved, and Dox-induced cardiac histopathological alterations were prevented when compared to animals fed with ACN-free diet from yellow corn (Petroni et al., 2017).
Decrease of the cardiac injury induced by cyclophosphamide, another widely used chemotherapeutic drug, was also achieved with a low dose of an ACN-rich extract from blueberry (20 mg/kg body weight/day) and it correlated to the antiinflammatory and antioxidant effects of ACNs (Liu et al., 2015). On the other hand, high concentrations of ACNs (200-250 mg/kg body weight/day) were found to be effective in experimental models of myocardial infarction induced by isoproterenol, which is known to cause an extensive oxidative damage, associated to degradation and subsequent exhaustion of enzymatic antioxidants (SOD and CAT), causing formation of free radicals and severe lipid peroxidation (Jana et al., 2017; Wei H. et al., 2017).
Antiobesity and Antidiabetic Effects
Obesity is the result of the accumulation of adipose tissue, and it causes many metabolic disorders. A healthy lifestyle and a diet rich in ACNs have beneficial antiobesity effects (Parveen et al., 2019; Salehi et al., 2019a).
One mechanism by which dietary ACNs could act as antiobese effect is the increase of energy expenditure. Berries containing ACNs (petunidin 33% and malvidin 57%) were effective to lower HFD induced metabolic damage by increased energy expenditure. In adipose tissue, a reduction in mitochondrial respiration and dissipation of the mitochondrial proton gradient (proton leak) were also reported (Skates et al., 2018). Malvidin decreases the lipopolysaccharide (LPS)-induced NF-κB, activation of poly ADP-ribose polymerase, MAPK, depolarization of mitochondria, and generation of ROS, (Bognar et al., 2013).
Another way to spend the energy is by changing thermogenesis. The upregulation of regulating uncoupling proteins (UCP1 and UCP2), in brown and white adipose tissue respectively, suppress fat accumulation in adipose tissue in case of increased dietary consumption of black soybean seed. (Kanamoto et al., 2011).
The role of dietary ACNs in AMPK modulation is very interesting.
AMP-activated protein kinase (AMPK) is one of the main regulators of energy balance. AMPK can modulate the energetic expenditure and fat accumulation in many ways: i) increasing mitochondrial biogenesis, ii) reducing lipid metabolism and triglyceride synthesis, iii) increasing fatty acid oxidation, iv) reducing hypertriglyceridemia and triglyceride storage in muscles and liver and by regulating the food intake. It seems that dietary ACNs induce AMPK activation by increasing its phosphorylation (Hardie and Ashford, 2014; López, 2018).
ACNs from bilberry extract also increased AMPK activity in skeletal muscle and the liver. In skeletal muscle, AMPK activation stimulated the upregulation of glucose transporter 4 (GLUT4), resulting in an increased glucose uptake and utilization. In the liver, AMPK activation decreased glucose production, improving hyperglycemia. A decrease in liver lipid content and serum lipoproteins was achieved through upregulation of peroxisome proliferator-activated receptor (PPAR)α and acyl-CoA oxidase (Takikawa et al., 2010). ACNs also improved chronic diabetic complications and insulin resistance (Guo and Ling, 2015).
Dietary ACNs can also affect lipid metabolism.
The key molecules of lipid metabolism are fatty acid synthase (FAS) and sterol regulatory element-binding proteins (SREBPs). ACNs from different dietary sources could downregulate mRNA and protein levels of FAS and SREBP1, reducing hyperglycemia and inhibiting hepatic lipogenesis (Tsuda et al., 2003; Hwang et al., 2011; Qin and Anderson, 2012; Park et al., 2015; Wu et al., 2016a).
ACNs from black soybeans were effective in preventing obesity even in normal conditions by hypothalamus modulation. A healthy diet showed a decrease in body weight and food intake when receiving daily intra-gastric administered ACNs (Salehi et al., 2020b). These effects seem to be mediated by neuropeptide Y and c-amino butyric acid receptor (GABAB1R) in the hypothalamus (Badshah et al., 2013). ACN-rich extract from black soybean decreased saturated, monounsaturated and n-6 polyunsaturated fatty acid levels in subcutaneous (but not visceral) fat. Since these long-chain fatty acids play a role in inflammation regulation, their reduction could help in suppressing inflammation in obese subjects (Sato et al., 2015)
Finally, ACNs seem to affect gut microbiota as well. The change in microbiota in obese people might contribute to the development of obese-related metabolic disorders. A recent review examined the role of ACNs in obesity regulation by gut microbiota modulation (Jamar et al., 2017)
Considering the antidiabetic role of ACNs, they provide protection to pancreatic β cells (INS-1) against H2O2-induced necrosis and apoptosis in a time- and concentration-dependent way. These substances in β cells and primary islets also upregulate the HO-1 gene expression and activate ERK1/2 and PI3K/Akt signaling, while ERK1/2 and PI3K inhibitors partially decreased ACN-mediated induction of HO-1 (Zhang et al., 2011).
The supplementation with a daily intake of 4 cups of freeze-dried strawberry beverage during 8 weeks in 27 diabetic subjects caused a reduction in total and LDL-cholesterol levels as well as inhibition of VCAM-1 circulating levels (Basu et al., 2010a).
The simultaneous use of ACNs and apple polyphenols in five postmenopausal women and 20 men showed the initial postprandial glycemic response. Both substances suppressed the early reactions (0–30 min) of plasma glucose and insulin, and reduction of postprandial glycemia. Insulin and incretin excretion were reduced as the secondary results (Castro-Acosta et al., 2017). ACNs consumption during 12 weeks also modulated the lipids and glucose-metabolism, and had antioxidant and antiinflammatory effects l in 37 humans with metabolic syndrome (Kim et al., 2018). In addition, ACNs rich beverages lowered the concentrations of interferon-γ (IFN-γ) and urinary level of 8-isoprostane (Kim et al., 2018).
Wu et al. (2013) have found that ACNs, including cyanidin-3-rutinoside, cyanidin-3-glucoside, and pelargonidin-3-glucoside significantly inhibit the body weight gain, reduction in IR, adipocytes size, decrease lipid accumulation, and reduce leptin secretion. ACNs improved glucose tolerance, enhanced insulin sensitivity and decreased hepatic accumulation of lipids via modulating the AMPK activity and lipid metabolism-associated gene expression (Overall et al., 2017).
Obesity: Brief Data on Pathophysiology
The number of obese people is dramatically increasing worldwide, affecting every year an increasing number of adults, but also children and adolescents (Xie et al., 2018). Obesity is closely related to a decrease in life expectancy and an increase in healthcare expenditures, it is a risk factor for many diseases such as some types of cancer, diabetes mellitus and CVDs (Swinburn et al., 2015).
The localization of the accumulated fat is crucially important: intraabdominal fat is mainly responsible for the development of the metabolic syndrome (MS), which is defined as the combination of impaired glucose tolerance or diabetes mellitus, insulin resistance, high blood pressure, atherogenic dyslipidemia, and obesity (Engin, 2017).
The effects of ACNs on MS have been recently highlighted (Naseri et al., 2018; Xie et al., 2018).
ACNs Antiobesity Mechanisms:
i. Increase in energy expenditure, and regulation of lipid metabolism
ii. reduction of fat absorption
iii. suppression of food intake
iv. gut microbiota modification.
In Vitro Studies
In the treatment of LPS-activated human umbilical vein endothelial cells (HUVECs), pelargonidin inhibited LPS-induced barrier disruption, migration of neutrophils to human endothelial cells, and expression of cell adhesion molecules (CAMs) and adhesion.
Blueberry ACN extract (malvidin, malvidin-3-glucoside, and malvidin-3-galactoside) has effects on high glucose-induced injury in human retinal capillary endothelial cells (HRCECs) by multiple pathways such as enhancement of cell viability, reduction of ROS, suppression of Nox4 expression, increase in enzyme activity of CAT and SOD, inhibition of Akt pathway, reduction of VEGF level, suppression of high glucose-induced intercellular adhesion molecule-1 and NF-κB, (Huang et al., 2018). The administration of 300 µM H2O2 in WI-38 human diploid fibroblasts showed enhanced lipid peroxidation, lowered cell viability, and shortened cells lifespans. In contrast, cyanidin supplementation suppressed oxidative stress via cell viability enhancement and lipid peroxidation inhibition. Cyanidin treatment also enhanced the cells life spans, decreased the NF-κB expression at mRNA and protein level, as well as iNOS, and COX-2 (Choi et al., 2010).
Recent studies showed that pelargonidin inhibited LPS-induced hyperpermeability and leukocytes migration. Furthermore, suppression of activation of NF-κB and production of TNF-α, IL-6, and ERK1/2 by LPS were reported. In addition, pelargonidin resulted in suppressing LPS-induced lethal endotoxemia (Lee et al., 2019).
In Vivo Studies
ACNs inhibit fat accumulation in mice: purple corn extract and purified ACNs from blueberries or strawberries can prevent body fat accumulation and obesity induced by a HFD in C57BL/6J mice (Tsuda et al., 2003; Howard et al., 2008).
Increased AMPK activity has been shown in 3T3-L1 cell line treated with C3G (Guo et al., 2012), in rats treated with black carrots extract (Park et al., 2015) and in obese mice fed with ACNs from purple sweet potato (Hwang et al., 2011). In these studies, AMPK activation was accompanied by a lack of increase in LDL-cholesterol and triglycerides but with an improved serum lipids profile and inhibited accumulation of triglycerides in the liver (Hwang et al., 2011; Park et al., 2015).
Pure ACNs and ACN extract were able to reduce the body and liver weight, the triglycerides accumulation in the liver and the adipocyte size in mice treated with HFD (Jayaprakasam et al., 2006; Zhang et al., 2013; Park et al., 2017).
Blueberry-derived ACNs were effective in reducing body weight and serum glucose and in improving lipid profile in high-fat-fed mice (Wu et al., 2016a), while ACNs derived from adzuki bean decreased lipid accumulation and triglyceride/cholesterol levels in mice fed with high-fat and high-cholesterol diet (Kim et al., 2016).
A very recent study analyzed the effect of ACNs supplementation from Sango sprout juice (SSJ) in obese rats. The results showed that supplementation of SSJ is more effective causing positive effects on liver, ileum and prostate when compared with a switch from a HFD to a regular. Moreover, the SSJ supplementation together with the diet switch is more effective (in respect to a simple diet switch) in opposing the caecal Enterococcus decrease and the Clostridium perfringens increase registered in obese animals. These results demonstrate a potential therapeutic role of ACNs in obese-induced intestinal dysbiosis (Vivarelli et al., 2018).
The adipose tissue secretes various adipocytokines, i.e., leptin, adiponectin, and resistin that causes obesity to be a metabolic disease.
Supplementation of ACNs-rich grape–bilberry juice to experimental rats lowered the concentrations of cholesterol, triglycerides, resistin, and leptin. This supplementation also decreased the saturated fatty acids and increased polyunsaturated fatty acids in plasma (Graf et al., 2013). ACNs decreased the secretion of adipocytokines (adiponectin and leptin) and increased lipoprotein lipase (LPL), PPARγ, UCP2, and adipocyte fatty acid-binding protein (aP2) expression in isolated rat adipocytes (Tsuda et al., 2004). Furthermore, ACNs-rich extracts have an improving effect against D-galactose-induced senescence in a mice model via lowering the uric acid level (Lu et al., 2014). ACNs have also beneficial affects against prostatic hyperplasia (Jang et al., 2010). In sickle cell disease, ACNs stabilize the erythrocyte membrane and suppress the hemoglobin polymerization (Mpiana et al., 2010). Supplementation of ACNs to C57BL/6J mice caused a significant reduction in concentrations of serum cholesterol, insulin resistance (IR), lipid accumulation and leptin secretion. These substances also have the potential to change MAPK and NF-κB stress signaling pathways (Wu et al., 2013).
In another study reported by Seymour et al. (2011), they investigated the administration of blueberry ACNs on white adipose tissue (WAT) and skeletal muscle in Zucker-fatty rats at the concentration of 2% (wt/wt). They have found that ACNs decreased the intraperitoneal fat weight and increased PPAR activity. Likewise, administration of ACNs at concentration of 8% (wt/wt) during 8 weeks decreased the inflammatory markers, enhanced the blood adiponectin levels, decreased the adipose tissue hypertrophy, hepatic steatosis, and insulin resistance in WAT and improved dyslipidemia (Vendrame et al., 2013).
Consistent with this, supplementation of mice under HFD with an ACNs-rich extract from purple corn resulted in lower recruitment and proliferation of macrophages into crown-like structures in the adipose tissue caused by a suppression of NF-kB signaling. Besides attenuating adipose tissue inflammation in vivo, ACNs also showed a long-lasting reprogramming of adipose tissue macrophages and adipocyte profiles toward the antiinflammatory phenotype (Tomay et al., 2019). Alteration in genes expression involved in lipid metabolism protect the induced fatty acid oxidation, and decrease the in vivo biosynthesis of fatty acids and cholesterol (Wu et al., 2013; Song et al., 2016).
Antioxidant and Antiinflammatory Effects
ACNs extracts from different natural sources were able to decrease the oxidative stress. (Figure 2) Dietary ACNs are known to be more effective antioxidants than vitamins E and C (Rice-Evans et al., 1997). ACNs can modulate the antioxidant defense mechanisms, activate antioxidant enzymes and promote glutathione synthesis. They are capable of chelating metal ions, such as iron and copper, thus reducing the production of free radicals by Fenton and other reactions (Jomova and Valko, 2011).
Several studies showed that ACNs activate nuclear factor erythroid 2-related factor 2 (Nrf2) and the antioxidant enzymes, such as superoxide dismutase (SOD), catalase (CAT), glutathione peroxidase (GPx) as well as directly enhance their enzymatic activity (Speciale et al., 2011; Speciale et al., 2013).
ACNs are also able to oppose the harmful action of toxic agents. ACNs from blueberry extract suppress the effects of acrylamide, attenuating ROS overproduction and glutathione depletion in liver. They were also effective in inhibiting cytochrome P450 2E1 (CYP2E1) protein expression in acrylamide-treated mice. CYP2E1 is the first protein involved in acrylamide epoxidation that was shown to cause different toxic effects (Zhao et al., 2015). The inhibition of CYP2E1 protein is also involved in the ACN-mediated protection of ethanol- and ROS-mediated damage. Ethanol, in fact, activates CYP2E1 that causes ROS production and antioxidant defense mechanisms impairment; ACNs in Gynura bicolor (Roxb. ex Willd.) DC. restored the glutathione content and decreased the ROS and glutathione disulfide levels in livers of ethanol-treated mice by reduction of CYP2E1 activity (Yin et al., 2017).
Inflammation: Brief Data on Pathophysiology
Oxidative stress occurs when reactive oxygen and nitrogen species production (ROS and RNS) exceeds the antioxidant mechanisms of cells or tissues, thus resulting in damage of macromolecules (i.e. proteins, lipids and DNA) in chronic diseases and aging process. Acute inflammation is the primary response against injury and pathogens, and it is usually followed by the resolution of inflammation (Salehi et al., 2019b). Chronic inflammation resulting from the failure of resolution is reported to promote the progression of many chronic diseases, such as CVDs, neurodegenerative diseases, diabetes mellitus and cancers (Pham-Huy et al., 2008; Salehi et al., 2018; Sharifi-Rad et al., 2018) (Medzhitov, 2008; Docea et al., 2020).
Antiinflammatory Molecular Mechanisms:
i. ACNs suppress the activation of the nuclear factor kappa B (NF-κB), a transcription factor regulating many genes in inflammatory response, such as inducible NO synthase (iNOS), cycloxygenase-2 (COX-2) and proinflammatory cytokines [tumor necrosis factor-α (TNF-α), interleukin (IL)-1β and IL-6] (Tsuda et al., 2002; Poulose et al., 2012).
ii. ACNs inhibit the mitogen-activated protein kinase (MAPK) signaling cascade involving p38, JNK, and ERK, also inducing suppression of proinflammatory cytokines, iNOS and COX-2 (Hou et al., 2005).
iii. ACNs directly inhibit COX-1 and COX-2 enzymes and as a consequence of this, the production of prostaglandin E2 (PGE2) (Graf et al., 2013; Hassimotto et al., 2013).
iv. ACNs suppress LRR, NACHT and PYD domains-containing protein 3 (NLRP3) inflammasomes by activation of Nrf2 and the thioredoxin-1/thioredoxin-interacting protein (Trx1/TXNIP) inhibitory complex (Cui et al., 2018; Hou et al., 2018). The NLRP3 inflammasome is a multimeric protein complex that initiates an inflammatory form of apoptosis, by triggering the release of proinflammatory cytokines IL-1β, IL-18 and caspase-1 and has been implicated in several diseases (Yang et al., 2019).
In Vivo Studies
The antioxidant activity of ACNs is well known for decades. (Pojer et al., 2013; Khoo et al., 2017). ACN extracts and pure ACNs increase the hepatic and serum levels of SOD and CAT in mice and rats while decreasing free radicals’ generation (Chiang et al., 2006; Roy et al., 2008).
A purple sweet potato color (PSPC) prevents the HFD-induced endoplasmic reticulum-mediated oxidative stress in mice liver. PSPC improved the hepatic redox state of mice treated with HFD by suppressing ROS production and by restoring the glutathione content and the activity of antioxidant enzymes (Zhang et al., 2013).
Similar results were observed in mice under HFD supplemented with ACNs from cherry or mulberry. Significant increases in SOD and GPx activities were detected. It was shown that monoglycoside ACNs might have higher antioxidant effects than di-glycoside or tri-glycoside ACNs (Wu et al., 2016b). Cyanidin 3-glucoside (C3G) was efficient in reducing the oxidative stress induced by lipid peroxidation, neutrophiles infiltration, and hepatic steatosis in diabetic mice. C3G increased glutathione synthesis by the induction of the glutamate-cysteine ligase catalytic subunit mediated by protein kinase A (PKA) and cAMP-response-element binding protein (CREB) (Zhu et al., 2012).
ACNs can improve ROS-caused damage in the brain. ACNs from Korean black bean inhibited ROS production induced by ethanol in the hippocampus of the postnatal rats (Shah et al., 2015). ACNs from black soybean suppressed neuroinflammation and neurodegeneration caused by oxidative stress and ROS increase in the cortex of adult mice (Khan et al., 2016).
ACNs as Natural Compounds With Potential Anticancer Properties
ACNs have attracted interest in the last several decades as potential antitumoral agents (Pojer et al., 2013; Lin et al., 2017).
There are several pathways involved in cancer, and some of them are not yet explained. Uncontrolled cell proliferation, resistance to apoptosis and migration are the main characteristics of tumor cells, which can be due to malfunctioning of Notch, Wnt/β-catenin, NF-κB and MAPK pathways (Dreesen and Brivanlou, 2007). Dietary berry ACNs can modulate the levels of Notch1 and Wnt1 proteins and their downstream mediators. In particular, ACNs mixture showed an enhanced reduction of all the proteins when compared with the single purified ACNs, indicating that some pathways may overlap in the induction of cell growth inhibition (Kausar et al., 2012).
The ACNs-mediated cancer prevention and inhibition mainly includes pathways involved in cell survival, proliferation, apoptosis control and inflammation. PI3K/Akt, NF-κB and COX-2 signaling and activity are the most studied mechanisms.
Although inflammation and oxidative stress play an important role in cancer progression (Kristo et al., 2016), it seems that ACNs, and not anthocyanidins, are responsible for the interaction with different molecules. In contrast, the glucosidic part may reduce the beneficial properties and interactions (Song et al., 2012).
NF-κB is downstream of the PI3K/Akt pathway, which is important for cell survival and proliferation. Still, if activated, it can cause deregulation of cell growth, malignant transformation and, often, therapy resistance (Hennessy et al., 2005). NF-κB has been indicated as the mediator between chronic inflammation and cancer. It can regulate tumor angiogenesis, metastatic process and apoptosis inhibition (Salehi et al., 2019d).
It has been suggested as a possible target in cancer therapy, even if its prolonged inhibition can cause deleterious effects (Xia et al., 2014). Its expression, together with COX-2, another factor involved in inflammation, and PI3K/Akt pathway, are modulated by ACNs, leading to reduced inflammatory response and cancer progression due to reduced proliferation (Song et al., 2012; Peiffer et al., 2014; Medda et al., 2015; Choi et al., 2016; Fragoso et al., 2018).
The main problem with chemotherapy is that some cancers can develop resistance to treatment after several months. Angiogenesis, metastasis progression and cell migration can severely worsen the patient status and compromise the success of chemotherapy (Mishra et al., 2018). Trastuzumab (Herceptin®) is a recombinant humanized monoclonal antibody that is targeted against human HER2 tyrosine kinase receptor, and it has been successfully used to treat patients with HER2-positive breast cancer. However, some trastuzumab-treated patients, who initially responded well, showed disease progression within a year after the end of the treatment (Li X. et al., 2016). C3G can enhance trastuzumab (Liu et al., 2014; Li X. et al., 2016).
Potential Mechanisms of Anticancer Properties:
i. Inhibition of tumor growth
ii. promoting apoptosis and autophagy of malignant cells
iii. modulating signal transduction pathways
iv. inhibition of angiogenesis and metastatic migration
v. antiinflammatory and antioxidant properties
In Vitro Studies
Delphinidin, a substance belonging to ACNs, dose-dependently suppresses cell proliferation and invasion, it induces apoptotic cell death and autophagy in human epidermal growth factor receptor (HER)-2 positive breast cancer MDA-MB-453 and BT474 cells. Moreover, it causes induction of autophagy via inhibiting mTOR signaling pathway and activation of the AMPK signaling pathway in HER-2 positive breast cancer cells (Chen J. et al., 2018).
In a recent study by Zhou et al. (2017), they investigated whether ACNs from black rice could have suppressive effect on HER-2 positive human breast cancer cell metastases in different human cancer cells lines—MCF-7, MCF-10A, and MDA-MB-453 cells. ACNs significantly inhibited the migration and cell invasion, lowered the migration distance of HER-2 positive human breast cancer cells, phosphorylation of cSrc, FAK, and p130C, lowered the levels of mesenchymal markers (fibronectin, vimentin), decreased the interaction between HER-2 and FAK, FAK and cSrc, and inhibited the epithelial-mesenchymal transition (Zhou et al., 2017).
The antitumor effect of ACNs in human hepatoma cells (SMMC-7721) and the murine hepatoma cells (H22) studies were also highlighted. ACNs significantly suppressed the cell growth, blocked the cell cycle in G2/M phase, induced DNA damage and induced apoptosis
C3G is a strong anticancer agent in different human breast cancer cell lines such as Hs‐578T and MDA‐MB‐231 cells. It achieves it by inhibiting the vascular endothelial growth factor (VEGF) expression and secretion, by decreasing the activator of transcription 3 (STAT3) and signal transducer expression at both mRNA and protein level. Additionally, induction of miR-124 expression was also reported after ACNs treatment (Salehi et al., 2019e).
A study conducted by Mazewski et al. (2018) was looking whether ACNs have inhibitory effects in concentrations (IC50) such as 0.9–2.0 mg/ml on human colon cancer cells (HT-29 and HCT-116) proliferation by inducing apoptotic cell death, decreasing the levels of antiapoptotic proteins (cIAP-2, survivin, XIAP), arresting cells in G1 phase as well as having a tyrosine kinase inhibitory potential. They have confirmed all these effects.
In another study, ACNs in a human gastrointestinal model (Colonic Caco-2 cancer cells and nontumorigenic colonic CCD-112CoN cells) cause cytotoxicity and lower cell viability (Kubow et al., 2017). Kuntz et al. (2017) showed that ACNs decreased cells migration, reactive oxygen production, NF-κB as well as matrix metalloproteinase (MMP)-2 and MMP-9 mRNA expression levels in different pancreatic cancer cells (PANC-1 and AsPC-1).
In another study conducted by Giampieri et al. (2018), ACNs have cytotoxic effects on hepatocellular carcinoma cells in a dose/time-dependent manner via enhancing cellular apoptosis and impairing mitochondrial functionality.
Cyanidin 3-rutinoside can inhibit the motility of RKO human colon cancer cells, as demonstrated by a wound-healing assay (Fragoso et al., 2018). Moreover, VEGF-induced angiogenesis was strongly inhibited by black raspberry extract on two organ-specific primary cells [i.e. human intestinal microvascular endothelial cells (HIMEC) and human esophagal microvascular endothelial cells (HEMEC)], isolated from surgically resected human intestine and esophagus (Medda et al., 2015).
In Vivo Studies
Xenograft tumors’ dimension in nude mice is severely reduced by pure C3G, ACNs from black soybean and by berry anthocyanidins mixture (C3G, malvidin, peonidin, petunidin, and delphinidin) (Kausar et al., 2012; Chen et al., 2015; Ha et al., 2015).
In different colorectal cancer models [DMH and TNBS-induced colitis-associated carcinogenesis in rat, azoxymethane (AOM)/dextran sodium sulfate (DSS) mouse model, APCMIN mice] the administration of ACNs sources, like açai pulp (Choi et al., 2016; Fragoso et al., 2018), purple sweet potato extract (Asadi et al., 2017) and bilberry extract (Lippert et al., 2017), resulted in reduced tumor growth, slower tumor development and reduced number of adenoma.
ACN extract of roselle (Hibiscus ACNs) was supplemented in the diet of the rat model of N-methyl-N-nitrosourea (NMU) -induced leukemia, and it significantly reduced the elevated aspartate aminotransferase (AST) and alanine aminotransferase (ALT) levels in serum and blood, and prevented NMU-induced leukemic cell infiltration and subsequent tissue damage (Tsai et al., 2014). Not only ACNs but also their metabolites can prevent tumor growth (Peiffer et al., 2014). In the same study protocatechuic acid, a major metabolite of blackberry ACNs, was able to reduce esophagal carcinogenesis in N-nitroso methyl benzylamine (NMBA)-induced rats carcinogenesis. They also provoked the death of tumor cells, inhibited tumor growth, and improved the survival status of H22 tumor-bearing mice. These effects were associated with an increase of the antioxidant mechanism (SOD, GPx, and glutathione) and a decrease of the lipid peroxidation (MDA). The levels of immune cytokines, including IL-2, IFN-γ, and TNF-α, were also regulated by ACNs (Zhou et al., 2018).
In AOM/DSS-treated C57BL/6J mice, ACNs enhanced the decreased probiotics (Eubacterium rectale, Faecalibacterium prausnitzii, and Lactobacillus) and the enhanced pathogenic bacteria (Desulfovibrio sp. and Enterococcus spp). These substances caused demethylation of the secreted frizzled related protein 2 (SFRP2) gene promoter, resulting in an increased expression of SFRP2, both at the mRNA and protein levels. In addition, they also down-regulated the DNMT31 and DNMT3B, and p-STAT3 expression (Chen L. et al., 2018).
Topical application of C3G can also reduce COX-2 levels and NF-κB activation in the skin of UV-B exposed mice (Pratheeshkumar et al., 2014). The PI3K/Akt pathway can also induce mTOR activation (Hennessy et al., 2005). In a study performed on thyroid cancer cells (SW1736 and HTh-7), mulberry ACNs induced apoptosis by severely enhanced autophagy caused by the suppression of Akt/mTOR signaling (Long et al., 2018). ACNs in vitro induced apoptosis in cancer cells by the increase of cleavage/activation of caspase-3, p53 expression, and Bax/Bcl-2 ratio together with increased NAD+/NADH ratio (Kausar et al., 2012; Ha et al., 2015).
Discussion, Therapeutic Limitations, and Clinical Pitfalls
There is a great interest in general public in the consumption of colorful phytochemicals such as ACNs, carotenoids and flavonoids, which are present in food and dietary supplements as well as in nutraceuticals. Polyphenolic substances, including flavonoids, are one of the most important classes of natural compounds that have a remarkable biological activity (Sharifi-Rad et al., 2020a).
Phenol-derived compounds including polyphenols, flavonoids and anthocyanidins have been recognized among the most promising secondary metabolites of naturally occurring compounds with therapeutic potentials. ACNs could be directly absorbed and found in animal or human plasma, while anthocyanidins have low bioavailability. The increased number of attached sugars in the aglycon might negatively affect the binding ability of ACNs to different targets (Sogo et al., 2014).
The main limitation in clinical therapy emerge from the low bioavailability of ACNs (Czank et al., 2013), their instability at physiological pH (Kay et al., 2009) and their massive conversion into metabolites once absorbed as well as by the intestinal microbiota (Aura et al., 2005; Czank et al., 2013). These aspects suggest that ACNs mainly accomplish their role of direct scavengers in the gut.
In order to improve the bioavailability and clinical usage of ACNs, chemical modifications and new drug design, such as nanotechnology were developed (Braga et al., 2018; Pinzaru et al., 2018).
The protection and especially the controlled release of various organic molecules is achieved almost exclusively by means of encapsulated compounds.
Cyclodextrins are part of the most used class receptors in host-guest inclusion chemistry. Advantages of encapsulation in cyclodextrins of active substances biological effects are: improving bioavailability, increasing stability, reducing side effects. However, they can function as molecular signals, being able to activate the endogenous antioxidant defense mechanisms (Virgili and Marino, 2008).
Nanoencapsulation is an example of a new research possibility that allows enhancing the bioavailability and optimizing the delivery of phytochemicals (Khan H. et al., 2019), including ACNs. Advantages of nanoencapsulation are: preservation of flavor, enhancing thermal and oxidative stability of chemical compounds, overcoming the limitations of high volatility, controlling the release of substances and improving bioavailability. Nanoencapsulation is more efficient and has better encapsulation properties than microencapsulation. All of these characteristics increase the possibilities of applications of phytochemicals in food and beverages. (Wyspianska et al., 2019).
The combination of carriers, e.g., chitosan with protein, improve the capsule efficiency and functionality (Ge et al., 2019). ACNs are hydrophilic natural chemical substances and cannot cross the plasma membrane by passive diffusion. Therefore, they need a hydrophilic carrier (Walton et al., 2006b). Their bioaccessibility potential is also depending upon their mineral contents, particularly potassium. In vitro digestion procedures can be used to evaluate the bioaccessibility (Gomes et al., 2019). Usually are used gastric simulation and small intestinal digestion models, sometimes followed by Caco-2 cells uptake (Vaidyanathan and Walle, 2001).
The preparation parameters of nanocomplexes with chitosan hydrochloride, inulin, and carboxymethyl chitosan as carrier showed maximum ACNs retention rate, preferred particle size and high encapsulation efficiency. For instance, to increase the bioavailability, the ACN source was encapsulated with liposomal micelles. The taste, smell and color of ACNs became more acceptable to consumers with encapsulation of isotonic drinks and extracts of fruits. Besides, the beverages enriched with inulin microcapsules had also better stability during storage (Tarone et al., 2020).
The main clinical pitfall of ACNs therapeutics usage is that certain drugs interact with ACNs. Studies have shown that the cytochrome P450 enzyme, which is involved in drug metabolism, is inhibited by flavonoids. An efflux transporter called P-glycoprotein, which decreases the absorption of certain drugs, is also affected. ACNs also interact with certain nutrients. They can bind to iron, thus decreasing its absorption in the intestine. Some of ACNs also inhibit cellular absorption of vitamin C (Ayabe and Akashi, 2006).
Overall Conclusions and Future Perspectives
ACNs are a diverse group of phytonutrients found in almost all fruits and vegetables. Along with carotenoids, they are responsible for the vivid colors of fruits and vegetables. Anthocyanidins include malvidin, pelargondin, peoidin, and cyanidin. Good sources of anthocyanidins are red, purple and blue fruits, such as pomegranates, plums, red wine and red and black grapes. Anthocyanidins are associated with heart health, antioxidant effects and help prevent obesity and diabetes.
ACNs are also widely used as natural dyes in the food industry. They have a wide range of color tones, ranging from orange to red to purple and blue, depending on the molecular structure and pH value. Interest in ACNs is not only based on their coloring effect, but also due to their health-beneficial properties. Due to the growing environmental and health problems in terms of synthetic dyes, natural dyes are an excellent alternative as an environmentally friendly dye for the food and drug industry.
ACNs are rapidly absorbed and appear in the bloodstream only few minutes after consumption. Future studies need to be planned to enable better understanding of the mechanisms by which food components achieve their effects and their pharmacokinetic characteristics. Antioxidant and antiinflammatory effects of ACNs are proven and it seems that ACNs also have an important role in CVDs, neurodegenerative diseases, diabetes and cancer. The potential of ACNs to affect mammalian metabolism is demonstrated in in vitro and in vivo studies. Dietary ACNs may be a potential regulator of obesity-derived inflammation and associated chronic diseases.
Future clinical studies, using food rich in ACNs and purified ACNs need to be performed, better understand the therapeutic potential of these antioxidant substances.
Author Contributions
Conceptualization JS-R and DC. Validation investigation, resources, data curation, writing—all authors. Review and editing—JS-R, KP, MM, AM, ŽR, and DC. All authors contributed to the article and approved the submitted version.
Funding
This work was supported by CONICYT PIA/APOYO CCTE AFB170007.
Conflict of Interest
The authors declare that the research was conducted in the absence of any commercial or financial relationships that could be construed as a potential conflict of interest.
References
Ali, T., Kim, T., Rehman, S. U., Khan, M. S., Amin, F. U., Khan, M., et al. (2018). Natural Dietary Supplementation of Anthocyanins via PI3K/Akt/Nrf2/HO-1 Pathways Mitigate Oxidative Stress, Neurodegeneration, and Memory Impairment in a Mouse Model of Alzheimer’s Disease. Mol. Neurobiol. 55, 6076–6093. doi: 10.1007/s12035-017-0798-6
Ali Shah, S., Ullah, I., Lee, H. Y., Kim, M. O. (2013). Anthocyanins protect against ethanol-induced neuronal apoptosis via GABAB1 receptors intracellular signaling in prenatal rat hippocampal neurons. Mol. Neurobiol. 48, 257–269. doi: 10.1007/s12035-013-8458-y
Amin, F. U., Shah, S. A., Badshah, H., Khan, M., Kim, M. O. (2017). Anthocyanins encapsulated by PLGA@PEG nanoparticles potentially improved its free radical scavenging capabilities via p38/JNK pathway against Abeta1-42-induced oxidative stress. J. Nanobiotechnol. 15, 12. doi: 10.1186/s12951-016-0227-4
Andres-Lacueva, C., Shukitt-Hale, B., Galli, R. L., Jauregui, O., Lamuela-Raventos, R. M., Joseph, J. A. (2005). Anthocyanins in aged blueberry-fed rats are found centrally and may enhance memory. Nutr. Neurosci. 8, 111–120. doi: 10.1080/10284150500078117
Asadi, K., Ferguson, L. R., Philpott, M., Karunasinghe, N. (2017). Cancer-preventive Properties of an Anthocyanin-enriched Sweet Potato in the APC MIN Mouse Model. J. Cancer Prev. 22, 135–146. doi: 10.15430/JCP.2017.22.3.135
Aura, A. M., Martin-Lopez, P., O’leary, K. A., Williamson, G., Oksman-Caldentey, K. M., Poutanen, K., et al. (2005). In vitro metabolism of anthocyanins by human gut microflora. Eur. J. Nutr. 44, 133–142. doi: 10.1007/s00394-004-0502-2
Ayabe, S.-I., Akashi, T. (2006). Cytochrome P450s in flavonoid metabolism. Phytochem. Rev. 5, 271–282. doi: 10.1007/s11101-006-9007-3
Badshah, H., Ullah, I., Kim, S. E., Kim, T. H., Lee, H. Y., Kim, M. O. (2013). Anthocyanins attenuate body weight gain via modulating neuropeptide Y and GABAB1 receptor in rats hypothalamus. Neuropeptides 47, 347–353. doi: 10.1016/j.npep.2013.06.001
Badshah, H., Kim, T. H., Kim, M. O. (2015). Protective effects of anthocyanins against amyloid beta-induced neurotoxicity in vivo and in vitro. Neurochem. Int. 80, 51–59. doi: 10.1016/j.neuint.2014.10.009
Basu, A., Fu, D. X., Wilkinson, M., Simmons, B., Wu, M., Betts, N. M., et al. (2010a). Strawberries decrease atherosclerotic markers in subjects with metabolic syndrome. Nutr. Res. 30, 462–469. doi: 10.1016/j.nutres.2010.06.016
Basu, A., Rhone, M., Lyons, T. J. (2010b). Berries: emerging impact on cardiovascular health. Nutr. Rev. 68, 168–177. doi: 10.1111/j.1753-4887.2010.00273.x
Belwal, T., Huang, H., Li, L., Duan, Z., Zhang, X., Aalim, H., et al. (2019). Optimization model for ultrasonic-assisted and scale-up extraction of anthocyanins from Pyrus communis ‘Starkrimson’fruit peel. Food Chem. 297, 124993. doi: 10.1016/j.foodchem.2019.124993
Block, M. L., Hong, J. S. (2007). Chronic microglial activation and progressive dopaminergic neurotoxicity. Biochem. Soc. Trans. 35, 1127–1132. doi: 10.1042/BST0351127
Bognar, E., Sarszegi, Z., Szabo, A., Debreceni, B., Kalman, N., Tucsek, Z., et al. (2013). Antioxidant and anti-inflammatory effects in RAW264.7 macrophages of malvidin, a major red wine polyphenol. PloS One 8, e65355. doi: 10.1371/journal.pone.0065355
Braga, A. R. C., Murador, D. C., De Souza Mesquita, L. M., De Rosso, V. V. (2018). Bioavailability of anthocyanins: Gaps in knowledge, challenges and future research. J. Food Composition Anal. 68, 31–40. doi: 10.1016/j.jfca.2017.07.031
Buga, A.-M., Docea, A. O., Albu, C., Malin, R. D., Branisteanu, D. E., Ianosi, G., et al. (2019). Molecular and cellular stratagem of brain metastases associated with melanoma. Oncol. Lett. 17, 4170–4175. doi: 10.3892/ol.2019.9933
Calina, D., Buga, A. M., Mitroi, M., Buha, A., Caruntu, C., Scheau, C., et al. (2020). The Treatment of Cognitive, Behavioural and Motor Impairments from Brain Injury and Neurodegenerative Diseases through Cannabinoid System Modulation—Evidence from In Vivo Studies. J. Clin. Med. 9, 2395. doi: 10.3390/jcm9082395
Carvalho, F. B., Gutierres, J. M., Bohnert, C., Zago, A. M., Abdalla, F. H., Vieira, J. M., et al. (2015). Anthocyanins suppress the secretion of proinflammatory mediators and oxidative stress, and restore ion pump activities in demyelination. J. Nutr. Biochem. 26, 378–390. doi: 10.1016/j.jnutbio.2014.11.006
Casedas, G., Gonzalez-Burgos, E., Smith, C., Lopez, V., Gomez-Serranillos, M. P. (2018). Regulation of redox status in neuronal SH-SY5Y cells by blueberry (Vaccinium myrtillus L.) juice, cranberry (Vaccinium macrocarpon A.) juice and cyanidin. Food Chem. Toxicol. 118, 572–580. doi: 10.1016/j.fct.2018.05.066
Cassidy, A., O’reilly, E. J., Kay, C., Sampson, L., Franz, M., Forman, J. P., et al. (2011). Habitual intake of flavonoid subclasses and incident hypertension in adults. Am. J. Clin. Nutr. 93, 338–347. doi: 10.3945/ajcn.110.006783
Cassidy, A., Mukamal, K. J., Liu, L., Franz, M., Eliassen, A. H., Rimm, E. B. (2013). High anthocyanin intake is associated with a reduced risk of myocardial infarction in young and middle-aged women. Circulation 127, 188–196. doi: 10.1161/CIRCULATIONAHA.112.122408
Cassidy, A., Rogers, G., Peterson, J. J., Dwyer, J. T., Lin, H., Jacques, P. F. (2015). Higher dietary anthocyanin and flavonol intakes are associated with anti-inflammatory effects in a population of US adults. Am. J. Clin. Nutr. 102, 172–181. doi: 10.3945/ajcn.115.108555
Cassidy, A., Bertoia, M., Chiuve, S., Flint, A., Forman, J., Rimm, E. B. (2016). Habitual intake of anthocyanins and flavanones and risk of cardiovascular disease in men. Am. J. Clin. Nutr. 104, 587–594. doi: 10.3945/ajcn.116.133132
Castañeda-Ovando, A., Pacheco-Hernández, M. D. L., Páez-Hernández, M. E., Rodríguez, J. A., Galán-Vidal, C. A. (2009). Chemical studies of anthocyanins: A review. Food Chem. 113, 859–871. doi: 10.1016/j.foodchem.2008.09.001
Castro-Acosta, M. L., Stone, S. G., Mok, J. E., Mhajan, R. K., Fu, C. I., Lenihan-Geels, G. N., et al. (2017). Apple and blackcurrant polyphenol-rich drinks decrease postprandial glucose, insulin and incretin response to a high-carbohydrate meal in healthy men and women. J. Nutr. Biochem. 49, 53–62. doi: 10.1016/j.jnutbio.2017.07.013
Chen, H., Yoshioka, H., Kim, G. S., Jung, J. E., Okami, N., Sakata, H., et al. (2011). Oxidative stress in ischemic brain damage: mechanisms of cell death and potential molecular targets for neuroprotection. Antioxid. Redox Signal 14, 1505–1517. doi: 10.1089/ars.2010.3576
Chen, X.-Y., Zhou, J., Luo, L.-P., Han, B., Li, F., Chen, J.-Y., et al. (2015). Black Rice Anthocyanins Suppress Metastasis of Breast Cancer Cells by Targeting RAS/RAF/MAPK Pathway. BioMed. Res. Int. 2015, 1–11. doi: 10.1155/2015/414250
Chen, J., Zhu, Y., Zhang, W., Peng, X., Zhou, J., Li, F., et al. (2018). Delphinidin induced protective autophagy via mTOR pathway suppression and AMPK pathway activation in HER-2 positive breast cancer cells. BMC Cancer 18, 342. doi: 10.1186/s12885-018-4231-y
Chen, L., Jiang, B., Zhong, C., Guo, J., Zhang, L., Mu, T., et al. (2018). Chemoprevention of colorectal cancer by black raspberry anthocyanins involved the modulation of gut microbiota and SFRP2 demethylation. Carcinogenesis 39, 471–481. doi: 10.1093/carcin/bgy009
Chiang, A. N., Wu, H. L., Yeh, H. I., Chu, C. S., Lin, H. C., Lee, W. C. (2006). Antioxidant effects of black rice extract through the induction of superoxide dismutase and catalase activities. Lipids 41, 797–803. doi: 10.1007/s11745-006-5033-6
Chiva-Blanch, G., Urpi-Sarda, M., Ros, E., Arranz, S., Valderas-Martinez, P., Casas, R., et al. (2012). Dealcoholized red wine decreases systolic and diastolic blood pressure and increases plasma nitric oxide: short communication. Circ. Res. 111, 1065–1068. doi: 10.1161/CIRCRESAHA.112.275636
Choi, M. J., Kim, B. K., Park, K. Y., Yokozawa, T., Song, Y. O., Cho, E. J. (2010). Anti-aging effects of cyanidin under a stress-induced premature senescence cellular system. Biol. Pharm. Bull. 33, 421–426. doi: 10.1248/bpb.33.421
Choi, Y. J., Choi, Y. J., Kim, N., Nam, R. H., Lee, S., Lee, H. S., et al. (2016). Açaí Berries Inhibit Colon Tumorigenesis in Azoxymethane/Dextran Sulfate Sodium-Treated Mice. Gut Liver 11, 243–252. doi: 10.5009/gnl16068
Chun, O. K., Chung, S. J., Claycombe, K. J., Song, W. O. (2008). Serum C-reactive protein concentrations are inversely associated with dietary flavonoid intake in U.S. adults. J. Nutr. 138, 753–760. doi: 10.1093/jn/138.4.753
Cui, H. X., Chen, J. H., Li, J. W., Cheng, F. R., Yuan, K. (2018). Protection of Anthocyanin from Myrica rubra against Cerebral Ischemia-Reperfusion Injury via Modulation of the TLR4/NF-kappaB and NLRP3 Pathways. Molecules 23. doi: 10.3390/molecules23071788
Czank, C., Cassidy, A., Zhang, Q., Morrison, D. J., Preston, T., Kroon, P. A., et al. (2013). Human metabolism and elimination of the anthocyanin, cyanidin-3-glucoside: a (13)C-tracer study. Am. J. Clin. Nutr. 97, 995–1003. doi: 10.3945/ajcn.112.049247
Demeilliers, C., Toufektsian, M. C., Salen, P., Laporte, F., Petroni, K., De Lorgeril, M. (2017). Ethanol drinking, brain mitochondrial DNA, polyunsaturated fatty acids and effects of dietary anthocyanins. Clin. Nutr. Exp. 12, 11–19. doi: 10.1016/j.yclnex.2017.01.003
Di Filippo, M., Chiasserini, D., Tozzi, A., Picconi, B., Calabresi, P. (2010). Mitochondria and the link between neuroinflammation and neurodegeneration. J. Alzheimers Dis. 20 (Suppl 2), S369–S379. doi: 10.3233/JAD-2010-100543
Di Giacomo, C., Acquaviva, R., Santangelo, R., Sorrenti, V., Vanella, L., Li Volti, G., et al. (2012). Effect of Treatment with Cyanidin-3-O-beta-D-Glucoside on Rat Ischemic/Reperfusion Brain Damage. Evid. Based Complement Alternat. Med. 2012, 285750. doi: 10.1155/2012/285750
Docea, A. O., Calina, D., Buga, A. M., Zlatian, O., Paoliello, M., Mogosanu, G. D., et al. (2020). The Effect of Silver Nanoparticles on Antioxidant/Pro-Oxidant Balance in a Murine Model. Int. J. Mol. Sci. 21, 1233. doi: 10.3390/ijms21041233
Dong, X. X., Wang, Y., Qin, Z. H. (2009). Molecular mechanisms of excitotoxicity and their relevance to pathogenesis of neurodegenerative diseases. Acta Pharmacol. Sin. 30, 379–387. doi: 10.1038/aps.2009.24
Dreesen, O., Brivanlou, A. H. (2007). Signaling pathways in cancer and embryonic stem cells. Stem Cell Rev. 3, 7–17. doi: 10.1007/s12015-007-0004-8
Eliasson, M. J., Huang, Z., Ferrante, R. J., Sasamata, M., Molliver, M. E., Snyder, S. H., et al. (1999). Neuronal nitric oxide synthase activation and peroxynitrite formation in ischemic stroke linked to neural damage. J. Neurosci. 19, 5910–5918. doi: 10.1523/JNEUROSCI.19-14-05910.1999
Engin, A. (2017). The Definition and Prevalence of Obesity and Metabolic Syndrome. Adv. Exp. Med. Biol. 960, 1–17. doi: 10.1007/978-3-319-48382-5_1
Erlund, I., Koli, R., Alfthan, G., Marniemi, J., Puukka, P., Mustonen, P., et al. (2008). Favorable effects of berry consumption on platelet function, blood pressure, and HDL cholesterol. Am. J. Clin. Nutr. 87, 323–331. doi: 10.1093/ajcn/87.2.323
Fragoso, M. F., Romualdo, G. R., Vanderveer, L. A., Franco-Barraza, J., Cukierman, E., Clapper, M. L., et al. (2018). Lyophilized açaí pulp (Euterpe oleracea Mart) attenuates colitis-associated colon carcinogenesis while its main anthocyanin has the potential to affect the motility of colon cancer cells. Food Chem. Toxicol. 121, 237–245. doi: 10.1016/j.fct.2018.08.078
Gao, X., Cassidy, A., Schwarzschild, M. A., Rimm, E. B., Ascherio, A. (2012). Habitual intake of dietary flavonoids and risk of Parkinson disease. Neurology 78, 1138–1145. doi: 10.1212/WNL.0b013e31824f7fc4
García-Conesa, M. T., Chambers, K., Combet, E., Pinto, P., Garcia-Aloy, M., Andrés-Lacueva, C., et al. (2018). Meta-Analysis of the Effects of Foods and Derived Products Containing Ellagitannins and Anthocyanins on Cardiometabolic Biomarkers: Analysis of Factors Influencing Variability of the Individual Responses. Int. J. Mol. Sci. 19 (3). doi: 10.3390/ijms19030694
Ge, J., Yue, X., Wang, S., Chi, J., Liang, J., Sun, Y., et al. (2019). Nanocomplexes composed of chitosan derivatives and β-Lactoglobulin as a carrier for anthocyanins: Preparation, stability and bioavailability in vitro. Food Res. Int. 116, 336–345. doi: 10.1016/j.foodres.2018.08.045
Giampieri, F., Gasparrini, M., Forbes-Hernandez, T. Y., Mazzoni, L., Capocasa, F., Sabbadini, S., et al. (2018). Overexpression of the Anthocyanidin Synthase Gene in Strawberry Enhances Antioxidant Capacity and Cytotoxic Effects on Human Hepatic Cancer Cells. J. Agric. Food Chem. 66, 581–592. doi: 10.1021/acs.jafc.7b04177
Gomes, T. M., Toaldo, I. M., Haas, I. C. D. S., Burin, V. M., Caliari, V., Luna, A. S., et al. (2019). Differential contribution of grape peel, pulp, and seed to bioaccessibility of micronutrients and major polyphenolic compounds of red and white grapes through simulated human digestion. J. Funct. Foods 52, 699–708. doi: 10.1016/j.jff.2018.11.051
Graf, D., Seifert, S., Jaudszus, A., Bub, A., Watzl, B. (2013). Anthocyanin-Rich Juice Lowers Serum Cholesterol, Leptin, and Resistin and Improves Plasma Fatty Acid Composition in Fischer Rats. PloS One 8, e66690. doi: 10.1371/journal.pone.0066690
Guo, H., Ling, W. (2015). The update of anthocyanins on obesity and type 2 diabetes: Experimental evidence and clinical perspectives. Rev. Endocrine Metab. Disord. 16, 1–13. doi: 10.1007/s11154-014-9302-z
Guo, H., Ling, W., Wang, Q., Liu, C., Hu, Y., Xia, M., et al. (2007). Effect of anthocyanin-rich extract from black rice (Oryza sativa L. indica) on hyperlipidemia and insulin resistance in fructose-fed rats. Plant Foods Hum. Nutr. 62, 1–6. doi: 10.1007/s11130-006-0031-7
Guo, H., Guo, J., Jiang, X., Li, Z., Ling, W. (2012). Cyanidin-3-O-β-glucoside, a typical anthocyanin, exhibits antilipolytic effects in 3T3-L1 adipocytes during hyperglycemia: Involvement of FoxO1-mediated transcription of adipose triglyceride lipase. Food Chem. Toxicol. 50, 3040–3047. doi: 10.1016/j.fct.2012.06.015
Ha, U. S., Bae, W. J., Kim, S. J., Yoon, B. I., Hong, S. H., Lee, J. Y., et al. (2015). Anthocyanin Induces Apoptosis of DU-145 Cells In Vitro and Inhibits Xenograft Growth of Prostate Cancer. Yonsei Med. J. 56, 16–16. doi: 10.3349/ymj.2015.56.1.16
Hardie, D. G., Ashford, M. L. J. (2014). AMPK: Regulating Energy Balance at the Cellular and Whole Body Levels. Physiology 29, 99–107. doi: 10.1152/physiol.00050.2013
Hassimotto, N. M., Moreira, V., Do Nascimento, N. G., Souto, P. C., Teixeira, C., Lajolo, F. M. (2013). Inhibition of carrageenan-induced acute inflammation in mice by oral administration of anthocyanin mixture from wild mulberry and cyanidin-3-glucoside. BioMed. Res. Int. 2013, 146716. doi: 10.1155/2013/146716
Helton, T. D., Otsuka, T., Lee, M. C., Mu, Y., Ehlers, M. D. (2008). Pruning and loss of excitatory synapses by the parkin ubiquitin ligase. Proc. Natl. Acad. Sci. U.S.A. 105, 19492–19497. doi: 10.1073/pnas.0802280105
Hennessy, B. T., Smith, D. L., Ram, P. T., Lu, Y., Mills, G. B. (2005). Exploiting the PI3K/AKT pathway for cancer drug discovery. Nat. Rev. Drug Discovery 4, 988–1004. doi: 10.1038/nrd1902
Hou, D. X., Yanagita, T., Uto, T., Masuzaki, S., Fujii, M. (2005). Anthocyanidins inhibit cyclooxygenase-2 expression in LPS-evoked macrophages: structure-activity relationship and molecular mechanisms involved. Biochem. Pharmacol. 70, 417–425. doi: 10.1016/j.bcp.2005.05.003
Hou, Y., Wang, Y., He, Q., Li, L., Xie, H., Zhao, Y., et al. (2018). Nrf2 inhibits NLRP3 inflammasome activation through regulating Trx1/TXNIP complex in cerebral ischemia reperfusion injury. Behav. Brain Res. 336, 32–39. doi: 10.1016/j.bbr.2017.06.027
Huang, W., Yan, Z., Li, D. (2018). Antioxidant and Anti-Inflammatory Effects of Blueberry Anthocyanins on High Glucose-Induced Human Retinal Capillary Endothelial Cells. Oxid. Med. Cell Longev. 2018, 1862462. doi: 10.1155/2018/1862462
Hwang, Y. P., Choi, J. H., Han, E. H., Kim, H. G., Wee, J. H., Jung, K. O., et al. (2011). Purple sweet potato anthocyanins attenuate hepatic lipid accumulation through activating adenosine monophosphate-activated protein kinase in human HepG2 cells and obese mice. Nutr. Res. 31, 896–906. doi: 10.1016/j.nutres.2011.09.026
Jackman, R. L., Yada, R. Y., Tung, M. A., Speers, R. A. (1987). Anthocyanins as food colorants—a review. J. Food Biochem. 11, 201–247. doi: 10.1111/j.1745-4514.1987.tb00123.x
Jamar, G., Estadella, D., Pisani, L. P. (2017). Contribution of anthocyanin-rich foods in obesity control through gut microbiota interactions. BioFactors 43, 507–516. doi: 10.1002/biof.1365
Jana, S., Patel, D., Patel, S., Upadhyay, K., Thadani, J., Mandal, R., et al. (2017). Anthocyanin rich extract of Brassica oleracea L. alleviates experimentally induced myocardial infarction. PloS One 12, 1–17. doi: 10.1371/journal.pone.0182137
Jang, H., Ha, U. S., Kim, S. J., Yoon, B. I., Han, D. S., Yuk, S. M., et al. (2010). Anthocyanin extracted from black soybean reduces prostate weight and promotes apoptosis in the prostatic hyperplasia-induced rat model. J. Agric. Food Chem. 58, 12686–12691. doi: 10.1021/jf102688g
Jayaprakasam, B., Olson, L. K., Schutzki, R. E., Tai, M. H., Nair, M. G. (2006). Amelioration of obesity and glucose intolerance in high-fat-fed C57BL/6 mice by anthocyanins and ursolic acid in cornelian cherry (Cornus mas). J. Agric. Food Chem. 54, 243–248. doi: 10.1021/jf0520342
Jellinger, K. A. (2009). Recent advances in our understanding of neurodegeneration. J. Neural Transm. (Vienna) 116, 1111–1162. doi: 10.1007/s00702-009-0240-y
Jennings, A., Welch, A. A., Fairweather-Tait, S. J., Kay, C., Minihane, A. M., Chowienczyk, P., et al. (2012). Higher anthocyanin intake is associated with lower arterial stiffness and central blood pressure in women. Am. J. Clin. Nutr. 96, 781–788. doi: 10.3945/ajcn.112.042036
Jennings, A., Welch, A. A., Spector, T., Macgregor, A., Cassidy, A. (2014). Intakes of anthocyanins and flavones are associated with biomarkers of insulin resistance and inflammation in women. J. Nutr. 144, 202–208. doi: 10.3945/jn.113.184358
Jiang, Y., Dai, M., Nie, W. J., Yang, X. R., Zeng, X. C. (2017). Effects of the ethanol extract of black mulberry (Morus nigra L.) fruit on experimental atherosclerosis in rats. J. Ethnopharmacol. 200, 228–235. doi: 10.1016/j.jep.2017.02.037
Jomova, K., Valko, M. (2011). Advances in metal-induced oxidative stress and human disease. Toxicology 283, 65–87. doi: 10.1016/j.tox.2011.03.001
Joo, H. K., Choi, S., Lee, Y. R., Lee, E. O., Park, M. S., Park, K. B., et al. (2018). Anthocyanin-rich extract from Red Chinese cabbage alleviates vascular inflammation in endothelial cells and apo E –/– mice. Int. J. Mol. Sci. 19. doi: 10.3390/ijms19030816
Joseph, J. A., Shukitt-Hale, B., Denisova, N. A., Bielinski, D., Martin, A., Mcewen, J. J., et al. (1999). Reversals of age-related declines in neuronal signal transduction, cognitive, and motor behavioral deficits with blueberry, spinach, or strawberry dietary supplementation. J. Neurosci. 19, 8114–8121. doi: 10.1523/JNEUROSCI.19-18-08114.1999
Joseph, J. A., Denisova, N. A., Arendash, G., Gordon, M., Diamond, D., Shukitt-Hale, B., et al. (2003). Blueberry supplementation enhances signaling and prevents behavioral deficits in an Alzheimer disease model. Nutr. Neurosci. 6, 153–162. doi: 10.1080/1028415031000111282
Kanamoto, Y., Yamashita, Y., Nanba, F., Yoshida, T., Tsuda, T., Fukuda, I., et al. (2011). A black soybean seed coat extract prevents obesity and glucose intolerance by up-regulating uncoupling proteins and down-regulating inflammatory cytokines in high-fat diet-fed mice. J. Agric. Food Chem. 59, 8985–8993. doi: 10.1021/jf201471p
Kausar, H., Jeyabalan, J., Aqil, F., Chabba, D., Sidana, J., Singh, I. P., et al. (2012). Berry anthocyanidins synergistically suppress growth and invasive potential of human non-small-cell lung cancer cells. Cancer Lett. 325, 54–62. doi: 10.1016/j.canlet.2012.05.029
Kay, C. D., Kroon, P. A., Cassidy, A. (2009). The bioactivity of dietary anthocyanins is likely to be mediated by their degradation products. Mol. Nutr. Food Res. 53 (Suppl 1), S92–101. doi: 10.1002/mnfr.200800461
Kay, C. D. (2006). Aspects of anthocyanin absorption, metabolism and pharmacokinetics in humans. Nutr. Res. Rev. 19, 137–146. doi: 10.1079/NRR2005116
Khan, M. S., Ali, T., Kim, M. W., Jo, M. H., Jo, M. G., Badshah, H., et al. (2016). Anthocyanins protect against LPS-induced oxidative stress-mediated neuroinflammation and neurodegeneration in the adult mouse cortex. Neurochem. Int. 100, 1–10. doi: 10.1016/j.neuint.2016.08.005
Khan, H., Ullah, H., Martorell, M., Valdes, S. E., Belwal, T., Tejada, S., et al. (2019). Flavonoids nanoparticles in cancer: Treatment, prevention and clinical prospects. Semin. Cancer Biol. S1044-579X (19), 30182–30188. doi: 10.1016/j.semcancer.2019.07.023
Khan, M. S., Ali, T., Kim, M. W., Jo, M. H., Chung, J. I., Kim, M. O. (2019). Anthocyanins Improve Hippocampus-Dependent Memory Function and Prevent Neurodegeneration via JNK/Akt/GSK3beta Signaling in LPS-Treated Adult Mice. Mol. Neurobiol. 56, 671–687. doi: 10.1007/s12035-018-1101-1
Khoo, H. E., Azlan, A., Tang, S. T., Lim, S. M. (2017). Anthocyanidins and anthocyanins: colored pigments as food, pharmaceutical ingredients, and the potential health benefits. Food Nutr. Res. 61, 1361779–1361779. doi: 10.1080/16546628.2017.1361779
Kim, H. G., Ju, M. S., Shim, J. S., Kim, M. C., Lee, S. H., Huh, Y., et al. (2010). Mulberry fruit protects dopaminergic neurons in toxin-induced Parkinson’s disease models. Br. J. Nutr. 104, 8–16. doi: 10.1017/S0007114510000218
Kim, S., Hong, J., Jeon, R., Kim, H. S. (2016). Adzuki bean ameliorates hepatic lipogenesis and proinflammatory mediator expression in mice fed a high-cholesterol and high-fat diet to induce nonalcoholic fatty liver disease. Nutr. Res. 36, 90–100. doi: 10.1016/j.nutres.2015.11.002
Kim, M. J., Rehman, S. U., Amin, F. U., Kim, M. O. (2017). Enhanced neuroprotection of anthocyanin-loaded PEG-gold nanoparticles against Abeta1-42-induced neuroinflammation and neurodegeneration via the NF-KB /JNK/GSK3beta signaling pathway. Nanomedicine 13, 2533–2544. doi: 10.1016/j.nano.2017.06.022
Kim, H., Simbo, S. Y., Fang, C., Mcalister, L., Roque, A., Banerjee, N., et al. (2018). Acai (Euterpe oleracea Mart.) beverage consumption improves biomarkers for inflammation but not glucose- or lipid-metabolism in individuals with metabolic syndrome in a randomized, double-blinded, placebo-controlled clinical trial. Food Funct. 9, 3097–3103. doi: 10.1039/C8FO00595H
Kristo, A., Klimis-Zacas, D., Sikalidis, A. (2016). Protective Role of Dietary Berries in Cancer. Antioxidants 5, 37–37. doi: 10.3390/antiox5040037
Kubow, S., Iskandar, M. M., Melgar-Bermudez, E., Sleno, L., Sabally, K., Azadi, B., et al. (2017). Effects of Simulated Human Gastrointestinal Digestion of Two Purple-Fleshed Potato Cultivars on Anthocyanin Composition and Cytotoxicity in Colonic Cancer and Non-Tumorigenic Cells. Nutrients 9. doi: 10.3390/nu9090953
Kuntz, S., Kunz, C., Rudloff, S. (2017). Inhibition of pancreatic cancer cell migration by plasma anthocyanins isolated from healthy volunteers receiving an anthocyanin-rich berry juice. Eur. J. Nutr. 56, 203–214. doi: 10.1007/s00394-015-1070-3
Lee, B. S., Lee, C., Yang, S., Park, E. K., Ku, S. K., Bae, J. S. (2019). Suppressive effects of pelargonidin on lipopolysaccharide-induced inflammatory responses. Chem. Biol. Interact. 302, 67–73. doi: 10.1016/j.cbi.2019.02.007
Li, X., Xu, J., Tang, X., Liu, Y., Yu, X., Wang, Z., et al. (2016). Anthocyanins inhibit trastuzumab-resistant breast cancer in vitro and in vivo. Mol. Med. Rep. 13, 4007–4013. doi: 10.3892/mmr.2016.4990
Li, Y., Sun, H., Chen, Z., Xu, H., Bu, G., Zheng, H. (2016). Implications of GABAergic Neurotransmission in Alzheimer’s Disease. Front. Aging Neurosci. 8:31. doi: 10.3389/fnagi.2016.00031
Li, C., Hu, C., Wang, R., Wang, H., Ma, Q., Chen, S., et al. (2019). Protective effect of sakuranetin in brain cells of dementia model rats. Cell Mol. Biol. (Noisy-le-grand) 65, 54–58. doi: 10.14715/cmb/2019.65.5.9
Lin, B.-W., Gong, C.-C., Song, H.-F., Cui, Y.-Y. (2017). Effects of anthocyanins on the prevention and treatment of cancer. Br. J. Pharmacol. 174, 1226–1243. doi: 10.1111/bph.13627
Lippert, E., Ruemmele, P., Obermeier, F., Goelder, S., Kunst, C., Rogler, G., et al. (2017). Anthocyanins Prevent Colorectal Cancer Development in a Mouse Model. Digestion 95, 275–280. doi: 10.1159/000475524
Liu, W., Xu, J., Liu, Y., Yu, X., Tang, X., Wang, Z., et al. (2014). Anthocyanins potentiate the activity of trastuzumab in human epidermal growth factor receptor 2-positive breast cancer cells in vitro and in vivo. Mol. Med. Rep. 10, 1921–1926. doi: 10.3892/mmr.2014.2414
Liu, Y., Tan, D., Shi, L., Liu, X., Zhang, Y., Tong, C., et al. (2015). Blueberry anthocyanins-enriched extracts attenuate cyclophosphamide-induced cardiac injury. PloS One 10, 1–18. doi: 10.1371/journal.pone.0127813
Long, H.-L., Zhang, F.-F., Wang, H.-L., Yang, W.-S., Hou, H.-T., Yu, J.-K., et al. (2018). Mulberry anthocyanins improves thyroid cancer progression mainly by inducing apoptosis and autophagy cell death. Kaohsiung J. Med. Sci. 34, 255–262. doi: 10.1016/j.kjms.2017.11.004
López, M. (2018). Hypothalamic AMPK and energy balance. Eur. J. Clin. Invest. 48, 1–10. doi: 10.1111/eci.12996
Lu, X., Zhou, Y., Wu, T., Hao, L. (2014). Ameliorative effect of black rice anthocyanin on senescent mice induced by D-galactose. Food Funct. 5, 2892–2897. doi: 10.1039/C4FO00391H
Luís, Â., Domingues, F., Pereira, L. (2018). Association between berries intake and cardiovascular diseases risk factors: A systematic review with meta-analysis and trial sequential analysis of randomized controlled trials. Food Funct. 9, 740–757. doi: 10.1039/C7FO01551H
Maestro, A., Terdoslavich, M., Vanzo, A., Kuku, A., Tramer, F., Nicolin, V., et al. (2009). Expression of bilitranslocase in the vascular endothelium and its function as a flavonoid transporter.
Magni, G., Marinelli, A., Riccio, D., Lecca, D., Tonelli, C., Abbracchio, M. P., et al. (2018). Purple Corn Extract as Anti-allodynic Treatment for Trigeminal Pain: Role of Microglia. Front. Cell Neurosci. 12:378. doi: 10.3389/fncel.2018.00378
`Mariani, E., Polidori, M. C., Cherubini, A., Mecocci, P. (2005). Oxidative stress in brain aging, neurodegenerative and vascular diseases: an overview. J. Chromatogr. B Analyt. Technol. BioMed. Life Sci. 827, 65–75. doi: 10.1016/j.jchromb.2005.04.023
Mattson, M. (2019). ““Excitotoxicity,”,” in Stress: Physiology, Biochemistry, and Pathology (Melbourne, Australia: Academic Press), 125–134.
Mauray, A., Milenkovic, D., Besson, C., Caccia, N., Morand, C., Michel, F., et al. (2009). Atheroprotective Effects of Bilberry Extracts in Apo E-Deficient Mice. J. Agric. Food Chem. 57, 11106–11111. doi: 10.1021/jf9035468
Mazewski, C., Liang, K., Gonzalez De Mejia, E. (2018). Comparison of the effect of chemical composition of anthocyanin-rich plant extracts on colon cancer cell proliferation and their potential mechanism of action using in vitro, in silico, and biochemical assays. Food Chem. 242, 378–388. doi: 10.1016/j.foodchem.2017.09.086
Mazza, G., Miniati, E. (2018). Anthocyanins in Fruits, Vegetables, and Grains: 0 (Boca Raton, Ann Arbor, London, Tokyo: CRC press).
Mccullough, M. L., Peterson, J. J., Patel, R., Jacques, P. F., Shah, R., Dwyer, J. T. (2012). Flavonoid intake and cardiovascular disease mortality in a prospective cohort of US adults. Am. J. Clin. Nutr. 95, 454–464. doi: 10.3945/ajcn.111.016634
Medda, R., Lyros, O., Schmidt, J. L., Jovanovic, N., Nie, L., Link, B. J., et al. (2015). Anti inflammatory and anti angiogenic effect of black raspberry extract on human esophageal and intestinal microvascular endothelial cells. Microvasc. Res. 97, 167–180. doi: 10.1016/j.mvr.2014.10.008
Medzhitov, R. (2008). Origin and physiological roles of inflammation. Nature 454, 428–435. doi: 10.1038/nature07201
Milbury, P. E., Vita, J. A., Blumberg, J. B. (2010). Anthocyanins are bioavailable in humans following an acute dose of cranberry juice. J. Nutr. 140, 1099–1104. doi: 10.3945/jn.109.117168
Min, J., Yu, S. W., Baek, S. H., Nair, K. M., Bae, O. N., Bhatt, A., et al. (2011). Neuroprotective effect of cyanidin-3-O-glucoside anthocyanin in mice with focal cerebral ischemia. Neurosci. Lett. 500, 157–161. doi: 10.1016/j.neulet.2011.05.048
Mink, P. J., Scrafford, C. G., Barraj, L. M., Harnack, L., Hong, C. P., Nettleton, J. A., et al. (2007). Flavonoid intake and cardiovascular disease mortality: a prospective study in postmenopausal women. Am. J. Clin. Nutr. 85, 895–909. doi: 10.1093/ajcn/85.3.895
Mishra, A. P., Salehi, B., Sharifi-Rad, M., Pezzani, R., Kobarfard, F., Sharifi-Rad, J., et al. (2018). Programmed Cell Death, from a Cancer Perspective: An Overview. Mol. Diagnosis Ther. 22, 281–295. doi: 10.1007/s40291-018-0329-9
Mititelu, R. R., Pădureanu, R., Băcănoiu, M., Pădureanu, V., Docea, A. O., Calina, D., et al. (2020). Inflammatory and Oxidative Stress Markers—Mirror Tools in Rheumatoid Arthritis. Biomedicines 8, 125. doi: 10.3390/biomedicines8050125
Moore, K. J., Tabas, I. (2011). Macrophages in the pathogenesis of atherosclerosis. Cell 145, 341–355. doi: 10.1016/j.cell.2011.04.005
Mpiana, P. T., Ngbolua, K. N., Bokota, M. T., Kasonga, T. K., Atibu, E. K., Tshibangu, D. S., et al. (2010). In vitro effects of anthocyanin extracts from Justicia secunda Vahl on the solubility of haemoglobin S and membrane stability of sickle erythrocytes. Blood Transfus 8, 248–254. doi: 10.2450/2009.0120-09
Mulabagal, V., Lang, G. A., Dewitt, D. L., Dalavoy, S. S., Nair, M. G. (2009). Anthocyanin content, lipid peroxidation and cyclooxygenase enzyme inhibitory activities of sweet and sour cherries. J. Agric. Food Chem. 57, 1239–1246. doi: 10.1021/jf8032039
Naseri, R., Farzaei, F., Haratipour, P., Nabavi, S. F., Habtemariam, S., Farzaei, M. H., et al. (2018). Anthocyanins in the Management of Metabolic Syndrome: A Pharmacological and Biopharmaceutical Review. Front. Pharmacol. 9, 1–19. doi: 10.3389/fphar.2018.01310
Nussbaum, L., Hogea, L. M., Călina, D., Andreescu, N., Grădinaru, R., Ştefănescu, R., et al. (2017). Modern treatment approaches in psychoses. Pharmacogenetic, neuroimagistic and clinical implications. Farmacia 65, 75–81.
Overall, J., Bonney, S. A., Wilson, M., Beermann, A., Grace, M. H., Esposito, D., et al. (2017). Metabolic Effects of Berries with Structurally Diverse Anthocyanins. Int. J. Mol. Sci. 18. doi: 10.3390/ijms18020422
Pacheco, S. M., Soares, M. S. P., Gutierres, J. M., Gerzson, M. F. B., Carvalho, F. B., Azambuja, J. H., et al. (2018). Anthocyanins as a potential pharmacological agent to manage memory deficit, oxidative stress and alterations in ion pump activity induced by experimental sporadic dementia of Alzheimer’s type. J. Nutr. Biochem. 56, 193–204. doi: 10.1016/j.jnutbio.2018.02.014
Padureanu, R., Albu, C. V., Mititelu, R. R., Bacanoiu, M. V., Docea, A. O., Calina, D., et al. (2019). Oxidative stress and inflammation interdependence in multiple sclerosis. J. Clin. Med. 8, 1815. doi: 10.3390/jcm8111815
Parameshwaran, K., Dhanasekaran, M., Suppiramaniam, V. (2008). Amyloid beta peptides and glutamatergic synaptic dysregulation. Exp. Neurol. 210, 7–13. doi: 10.1016/j.expneurol.2007.10.008
Park, S., Kang, S., Jeong, D. Y., Jeong, S. Y., Park, J. J., Yun, H. S. (2015). Cyanidin and malvidin in aqueous extracts of black carrots fermented with Aspergillus oryzae prevent the impairment of energy, lipid and glucose metabolism in estrogen-deficient rats by AMPK activation. Genes Nutr. 10. doi: 10.1007/s12263-015-0455-5
Park, C. H., Kim, J. H., Lee, E. B., Hur, W., Kwon, O. J., Park, H. J., et al. (2017). Aronia melanocarpa Extract Ameliorates Hepatic Lipid Metabolism through PPARγ2 Downregulation. PloS One 12. doi: 10.1371/journal.pone.0169685
Parveen, K., Siddiqui, W. A., Arif, J. M., Kuddus, M., Shahid, S. M. A., Kausar, M. A. (2019). Evaluation of vegetables and fish oils for the attenuation of diabetes complications. Cell Mol. Biol. (Noisy-le-grand) 65, 38–45. doi: 10.14715/cmb/2019.65.7.8
Pascual-Teresa, D., Moreno, D. A., García-Viguera, C. (2010). Flavanols and anthocyanins in cardiovascular health: a review of current evidence. Int. J. Mol. Sci. 11, 1679–1703. doi: 10.3390/ijms11041679
Passamonti, S., Vrhovsek, U., Vanzo, A., Mattivi, F. (2003). The stomach as a site for anthocyanins absorption from food. FEBS Lett. 544, 210–213. doi: 10.1016/S0014-5793(03)00504-0
Passamonti, S., Terdoslavich, M., Franca, R., Vanzo, A., Tramer, F., Braidot, E., et al. (2009). Bioavailability of flavonoids: a review of their membrane transport and the function of bilitranslocase in animal and plant organisms. Curr. Drug Metab. 10, 369–394. doi: 10.2174/138920009788498950
Peiffer, D. S., Zimmerman, N. P., Wang, L.-S., Ransom, B., Carmella, S. G., Kuo, C.-T., et al. (2014). Chemoprevention of esophageal cancer with black raspberries, their component anthocyanins, and a major anthocyanin metabolite, protocatechuic acid. Cancer Prev. Res. (Phila) 7, 574–584. doi: 10.1158/1940-6207.CAPR-14-0003
Petroni, K., Trinei, M., Fornari, M., Calvenzani, V., Marinelli, A., Micheli, L. A., et al. (2017). Dietary cyanidin 3-glucoside from purple corn ameliorates doxorubicin-induced cardiotoxicity in mice. Nutr. Metab. Cardiovasc. Dis. 27, 462–469. doi: 10.1016/j.numecd.2017.02.002
Pham-Huy, L. A., He, H., Pham-Huy, C. (2008). Free radicals, antioxidants in disease and health. Int. J. BioMed. Sci. 4, 89–96.
Pinzaru, I., Coricovac, D., Dehelean, C., Moacă, E.-A., Mioc, M., Baderca, F., et al. (2018). Stable PEG-coated silver nanoparticles–A comprehensive toxicological profile. Food Chem. Toxicol. 111, 546–556. doi: 10.1016/j.fct.2017.11.051
Pojer, E., Mattivi, F., Johnson, D., Stockley, C. S. (2013). The case for anthocyanin consumption to promote human health: A review. Compr. Rev. Food Sci. Food Saf. 12, 483–508. doi: 10.1111/1541-4337.12024
Poulose, S. M., Fisher, D. R., Larson, J., Bielinski, D. F., Rimando, A. M., Carey, A. N., et al. (2012). Anthocyanin-rich acai (Euterpe oleracea Mart.) fruit pulp fractions attenuate inflammatory stress signaling in mouse brain BV-2 microglial cells. J. Agric. Food Chem. 60, 1084–1093. doi: 10.1021/jf203989k
Pour, P. M., Fakhri, S., Asgary, S., Farzaei, M. H., Echeverria, J. (2019). The signaling pathways, and therapeutic targets of antiviral agents: focusing on the antiviral approaches and clinical perspectives of anthocyanins in the management of viral diseases. Front. Pharmacol. 10. doi: 10.3389/fphar.2019.01207
Prior, R. L., Wu, X., Gu, L., Hager, T. J., Hager, A., Howard, L. R. (2008). Whole berries versus berry anthocyanins: interactions with dietary fat levels in the C57BL/6J mouse model of obesity. J. Agric. Food Chem. 56 (3), 647–653. doi: 10.1021/jf0719930
Pratheeshkumar, P., Son, Y.-O., Wang, X., Divya, S. P., Joseph, B., Hitron, J. A., et al. (2014). Cyanidin-3-glucoside inhibits UVB-induced oxidative damage and inflammation by regulating MAP kinase and NF-κB signaling pathways in SKH-1 hairless mice skin. Toxicol. Appl. Pharmacol. 280, 127–137. doi: 10.1016/j.taap.2014.06.028
Puspita, L., Chung, S. Y., Shim, J. W. (2017). Oxidative stress and cellular pathologies in Parkinson’s disease. Mol. Brain 10, 53. doi: 10.1186/s13041-017-0340-9
Qin, B., Anderson, R. A. (2012). An extract of chokeberry attenuates weight gain and modulates insulin, adipogenic and inflammatory signalling pathways in epididymal adipose tissue of rats fed a fructose-rich diet. Br. J. Nutr. 108, 581–587. doi: 10.1017/S000711451100599X
Raj, P., Mccallum, J. L., Kirby, C., Grewal, G., Yu, L., Wigle, J. T., et al. (2017). Effects of cyanidin 3-0-glucoside on cardiac structure and function in an animal model of myocardial infarction. Food Funct. 8, 4089–4099. doi: 10.1039/C7FO00709D
Rehman, S. U., Shah, S. A., Ali, T., Chung, J. I., Kim, M. O. (2017). Anthocyanins Reversed D-Galactose-Induced Oxidative Stress and Neuroinflammation Mediated Cognitive Impairment in Adult Rats. Mol. Neurobiol. 54, 255–271. doi: 10.1007/s12035-015-9604-5
Riaz, M., Zia-Ul-Haq, M., Saad, B. (2016). Anthocyanins and human health: biomolecular and therapeutic aspects (New York: Springer), 125–138.
Rice-Evans, C. A., Miller, N. J., Paganga, G. (1997). Antioxidant properties of phenolic compounds. Trends Plant Sci. 2, 152–159. doi: 10.1016/S1360-1385(97)01018-2
Roy, M., Sen, S., Chakraborti, A. S. (2008). Action of pelargonidin on hyperglycemia and oxidative damage in diabetic rats: Implication for glycation-induced hemoglobin modification. Life Sci. 82, 1102–1110. doi: 10.1016/j.lfs.2008.03.011
Russo, M. V., Mcgavern, D. B. (2015). Immune Surveillance of the CNS following Infection and Injury. Trends Immunol. 36, 637–650. doi: 10.1016/j.it.2015.08.002
Salehi, B., Martorell, M., Arbiser, J. L., Sureda, A., Martins, N., Maurya, P. K., et al. (2018). Antioxidants: Positive or Negative Actors? Biomolecules 8. doi: 10.3390/biom8040124
Salehi, B., Capanoglu, E., Adrar, N., Catalkaya, G., Shaheen, S., Jaffer, M., et al. (2019a). Cucurbits plants: A key emphasis to its pharmacological potential. Molecules 24, 1854. doi: 10.3390/molecules24101854
Salehi, B., Lopez-Jornet, P., Pons-Fuster López, E., Calina, D., Sharifi-Rad, M., Ramírez-Alarcón, K., et al. (2019b). Plant-derived bioactives in oral mucosal lesions: A key emphasis to curcumin, lycopene, chamomile, aloe vera, green tea and coffee properties. Biomolecules 9, 106. doi: 10.3390/biom9030106
Salehi, B., Sestito, S., Rapposelli, S., Peron, G., Calina, D., Sharifi-Rad, M., et al. (2019c). Epibatidine: A Promising Natural Alkaloid in Health. Biomolecules 9, 6. doi: 10.3390/biom9010006
Salehi, B., Shivaprasad Shetty, M., V Anil Kumar, N., Živković, J., Calina, D., Oana Docea, A., et al. (2019d). Veronica plants—drifting from farm to traditional healing, food application, and phytopharmacology. Molecules 24, 2454. doi: 10.3390/molecules24132454
Salehi, B., Venditti, A., Sharifi-Rad, M., Kręgiel, D., Sharifi-Rad, J., Durazzo, A., et al. (2019e). The therapeutic potential of Apigenin. Int. J. Mol. Sci. 20. doi: 10.3390/ijms20061305
Salehi, B., Calina, D., Docea, A. O., Koirala, N., Aryal, S., Lombardo, D., et al. (2020a). Curcumin’s Nanomedicine Formulations for Therapeutic Application in Neurological Diseases. J. Clin. Med. 9, 430. doi: 10.3390/jcm9020430
Salehi, B., Rescigno, A., Dettori, T., Calina, D., Docea, A. O., Singh, L., et al. (2020b). Avocado–Soybean Unsaponifiables: A Panoply of Potentialities to Be Exploited. Biomolecules 10, 130. doi: 10.3390/biom10010130
Salgado, J. M., Oliveira, A. G., Mansi, D. N., Donado-Pestana, C. M., Bastos, C. R., Marcondes, F. K. (2010). The role of black rice (Oryza sativa L.) in the control of hypercholesterolemia in rats. J. Med. Food 13, 1355–1362. doi: 10.1089/jmf.2009.0246
Santhakumar, A. B., Kundur, A. R., Fanning, K., Netzel, M., Stanley, R., Singh, I. (2015). Consumption of anthocyanin-rich Queen Garnet plum juice reduces platelet activation related thrombogenesis in healthy volunteers. J. Funct. Foods 12, 11–22. doi: 10.1016/j.jff.2014.10.026
Sato, D., Kusunoki, M., Seino, N., Nishina, A., Feng, Z., Tsutsumi, K., et al. (2015). Black soybean extract reduces fatty acid contents in subcutaneous, but not in visceral adipose triglyceride in high-fat fed rats. Int. J. Food Sci. Nutr. 66, 539–545. doi: 10.3109/09637486.2015.1028907
Sesso, H. D., Gaziano, J. M., Jenkins, D. J., Buring, J. E. (2007). Strawberry intake, lipids, C-reactive protein, and the risk of cardiovascular disease in women. J. Am. Coll. Nutr. 26, 303–310. doi: 10.1080/07315724.2007.10719615
Seymour, E. M., Tanone, I. I., Urcuyo-Llanes, D. E., Lewis, S. K., Kirakosyan, A., Kondoleon, M. G., et al. (2011). Blueberry intake alters skeletal muscle and adipose tissue peroxisome proliferator-activated receptor activity and reduces insulin resistance in obese rats. J. Med. Food 14, 1511–1518. doi: 10.1089/jmf.2010.0292
Shah, S. A., Yoon, G. H., Kim, M. O. (2015). Protection of the Developing Brain with Anthocyanins Against Ethanol-Induced Oxidative Stress and Neurodegeneration. Mol. Neurobiol. 51, 1278–1291. doi: 10.1007/s12035-014-8805-7
Sharifi-Rad, J., Sharifi-Rad, M., Salehi, B., Iriti, M., Roointan, A., Mnayer, D., et al. (2018). In vitro and in vivo assessment of free radical scavenging and antioxidant activities of Veronica persica Poir. Cell. Mol. Biol. 64, 57–64. doi: 10.14715/cmb/2018.64.8.9
Sharifi-Rad, J., Rodrigues, C. F., Sharopov, F., Docea, A. O., Can Karaca, A., Sharifi-Rad, M., et al. (2020). Diet, Lifestyle and Cardiovascular Diseases: Linking Pathophysiology to Cardioprotective Effects of Natural Bioactive Compounds. Int. J. Environ. Res. Public Health 17, 2326. doi: 10.3390/ijerph17072326
Sharifi-Rad, M., Anil Kumar, N., Zucca, P., Varoni, E. M., Dini, L., Panzarini, E., et al. (2020a). Lifestyle, Oxidative Stress and Antioxidants: Back and Forth in the Pathophysiology of Chronic Diseases. Front. Physiol. 11:694. doi: 10.3389/fphys.2020.00694
Sharifi-Rad, M., Lankatillake, C., Dias, D. A., Docea, A. O., Mahomoodally, M. F., Lobine, D., et al. (2020b. Impact of Natural Compounds on Neurodegenerative Disorders: From Preclinical to Pharmacotherapeutics. J. Clin. Med. 9, 1061. doi: 10.3390/jcm9041061
Shih, P. H., Chan, Y. C., Liao, J. W., Wang, M. F., Yen, G. C. (2010). Antioxidant and cognitive promotion effects of anthocyanin-rich mulberry (Morus atropurpurea L.) on senescence-accelerated mice and prevention of Alzheimer’s disease. J. Nutr. Biochem. 21, 598–605. doi: 10.1016/j.jnutbio.2009.03.008
Shih, P. H., Wu, C. H., Yeh, C. T., Yen, G. C. (2011). Protective effects of anthocyanins against amyloid beta-peptide-induced damage in neuro-2A cells. J. Agric. Food Chem. 59, 1683–1689. doi: 10.1021/jf103822h
Shin, W. H., Park, S. J., Kim, E. J. (2006). Protective effect of anthocyanins in middle cerebral artery occlusion and reperfusion model of cerebral ischemia in rats. Life Sci. 79, 130–137. doi: 10.1016/j.lfs.2005.12.033
Singh, A., Kukreti, R., Saso, L., Kukreti, S. (2019). Oxidative Stress: A Key Modulator in Neurodegenerative Diseases. Molecules 24. doi: 10.3390/molecules24081583
Skates, E., Overall, J., Dezego, K., Wilson, M., Esposito, D., Lila, M. A., et al. (2018). Berries containing anthocyanins with enhanced methylation profiles are more effective at ameliorating high fat diet-induced metabolic damage. Food Chem. Toxicol. 111, 445–453. doi: 10.1016/j.fct.2017.11.032
Sogo, T., Kumamoto, T., Ishida, H., Hisanaga, A., Sakao, K., Terahara, N., et al. (2014). Comparison of the Inhibitory Effects of Delphinidin and Its Glycosides on Cell Transformation. Planta Med. 81, 26–31. doi: 10.1055/s-0034-1383311
Song, N. R., Yang, H., Park, J., Kwon, J. Y., Kang, N. J., Heo, Y. S., et al. (2012). Cyanidin suppresses neoplastic cell transformation by directly targeting phosphatidylinositol 3-kinase. Food Chem. 133, 658–664. doi: 10.1016/j.foodchem.2012.01.045
Song, H., Lai, J., Tang, Q., Zheng, X. (2016). Mulberry ethanol extract attenuates hepatic steatosis and insulin resistance in high-fat diet-fed mice. Nutr. Res. 36, 710–718. doi: 10.1016/j.nutres.2016.01.011
Speciale, A., Chirafisi, J., Saija, A., Cimino, F. (2011). Nutritional antioxidants and adaptive cell responses: an update. Curr. Mol. Med. 11, 770–789. doi: 10.2174/156652411798062395
Speciale, A., Anwar, S., Canali, R., Chirafisi, J., Saija, A., Virgili, F., et al. (2013). Cyanidin-3-O-glucoside counters the response to TNF-alpha of endothelial cells by activating Nrf2 pathway. Mol. Nutr. Food Res. 57, 1979–1987. doi: 10.1002/mnfr.201300102
Stewart, C. R., Stuart, L. M., Wilkinson, K., Van Gils, J. M., Deng, J., Halle, A., et al. (2010). CD36 ligands promote sterile inflammation through assembly of a Toll-like receptor 4 and 6 heterodimer. Nat. Immunol. 11, 155–161. doi: 10.1038/ni.1836
Strathearn, K. E., Yousef, G. G., Grace, M. H., Roy, S. L., Tambe, M. A., Ferruzzi, M. G., et al. (2014). Neuroprotective effects of anthocyanin- and proanthocyanidin-rich extracts in cellular models of Parkinsons disease. Brain Res. 1555, 60–77. doi: 10.1016/j.brainres.2014.01.047
Subhramanyam, C. S., Wang, C., Hu, Q., Dheen, S. T. (2019). Microglia-mediated neuroinflammation in neurodegenerative diseases. Semin. Cell Dev. Biol. 94, 112–120. doi: 10.1016/j.semcdb.2019.05.004
Sun, H., Kawahara, Y., Ito, K., Kanazawa, I., Kwak, S. (2006). Slow and selective death of spinal motor neurons in vivo by intrathecal infusion of kainic acid: implications for AMPA receptor-mediated excitotoxicity in ALS. J. Neurochem. 98, 782–791. doi: 10.1111/j.1471-4159.2006.03903.x
Swinburn, B., Dietz, W., Kleinert, S. (2015). A Lancet Commission on obesity. Lancet 386, 1716–1717. doi: 10.1016/S0140-6736(15)00722-9
Szydlowska, K., Tymianski, M. (2010). Calcium, ischemia and excitotoxicity. Cell Calcium 47, 122–129. doi: 10.1016/j.ceca.2010.01.003
Takikawa, M., Inoue, S., Horio, F., Tsuda, T. (2010). Dietary Anthocyanin-Rich Bilberry Extract Ameliorates Hyperglycemia and Insulin Sensitivity via Activation of AMP-Activated Protein Kinase in Diabetic Mice. J. Nutr. 140, 527–533. doi: 10.3945/jn.109.118216
Talavera, S., Felgines, C., Texier, O., Besson, C., Lamaison, J. L., Remesy, C. (2003). Anthocyanins are efficiently absorbed from the stomach in anesthetized rats. J. Nutr. 133, 4178–4182. doi: 10.1093/jn/133.12.4178
Talavera, S., Felgines, C., Texier, O., Besson, C., Manach, C., Lamaison, J. L., et al. (2004). Anthocyanins are efficiently absorbed from the small intestine in rats. J. Nutr. 134, 2275–2279. doi: 10.1093/jn/134.9.2275
Tarone, A. G., Cazarin, C. B. B., Marostica Junior, M. R. (2020). Anthocyanins: New techniques and challenges in microencapsulation. Food Res. Int. 133, 109092. doi: 10.1016/j.foodres.2020.109092
Thompson, K., Hosking, H., Pederick, W., Singh, I., Santhakumar, A. B. (2017a). The effect of anthocyanin supplementation in modulating platelet function in sedentary population: a randomised, double-blind, placebo-controlled, cross-over trial. Br. J. Nutr. 118, 368–374. doi: 10.1017/S0007114517002124
Thompson, K., Pederick, W., Singh, I., Santhakumar, A. B. (2017b). Anthocyanin supplementation in alleviating thrombogenesis in overweight and obese population: A randomized, double-blind, placebo-controlled study. J. Funct. Foods 32, 131–138. doi: 10.1016/j.jff.2017.02.031
Tomay, F., Marinelli, A., Leoni, V., Caccia, C., Matros, A., Mock, H.-P., et al. (2019). Purple corn extract induces long-lasting reprogramming and M2 phenotypic switch of adipose tissue macrophages in obese mice. J. Trans. Med. 17, 237. doi: 10.1186/s12967-019-1972-6
Toufektsian, M. C., De Lorgeril, M., Nagy, N., Salen, P., Donati, M. B., Giordano, L., et al. (2008). Chronic dietary intake of plant-derived anthocyanins protects the rat heart against ischemia-reperfusion injury. J. Nutr. 138, 747–752. doi: 10.1093/jn/138.4.747
Toufektsian, M. C., Salen, P., Laporte, F., Tonelli, C., De Lorgeril, M. (2011). Dietary flavonoids increase plasma very long-chain (n-3) fatty acids in rats. J. Nutr. 141, 37–41. doi: 10.3945/jn.110.127225
Tsai, T.-C., Huang, H.-P., Chang, Y.-C., Wang, C.-J. (2014). An Anthocyanin-Rich Extract from Hibiscus sabdariffa Linnaeus Inhibits N -Nitrosomethylurea-Induced Leukemia in Rats. J. Agric. Food Chem. 62, 1572–1580. doi: 10.1021/jf405235j
Tsatsakis, A., Docea, A. O., Calina, D., Tsarouhas, K., Zamfira, L.-M., Mitrut, R., et al. (2019). A Mechanistic and Pathophysiological Approach for Stroke Associated with Drugs of Abuse. J. Clin. Med. 8, 1295. doi: 10.3390/jcm8091295
Tsuda, T., Horio, F., Osawa, T. (2002). Cyanidin 3-O-beta-D-glucoside suppresses nitric oxide production during a zymosan treatment in rats. J. Nutr. Sci. Vitaminol. (Tokyo) 48, 305–310. doi: 10.3177/jnsv.48.305
Tsuda, T., Horio, F., Uchida, K., Aoki, H., Osawa, T. (2003). Dietary cyanidin 3-O-beta-D-glucoside-rich purple corn color prevents obesity and ameliorates hyperglycemia in mice. J. Nutr. 133, 2125–2130. doi: 10.1093/jn/133.7.2125
Tsuda, T., Ueno, Y., Aoki, H., Koda, T., Horio, F., Takahashi, N., et al. (2004). Anthocyanin enhances adipocytokine secretion and adipocyte-specific gene expression in isolated rat adipocytes. Biochem. Biophys. Res. Commun. 316, 149–157. doi: 10.1016/j.bbrc.2004.02.031
Tsuda, T. (2012). Dietary anthocyanin-rich plants: biochemical basis and recent progress in health benefits studies. Mol. Nutr. Food Res. 56, 159–170. doi: 10.1002/mnfr.201100526
Ullah, I., Park, H. Y., Kim, M. O. (2014). Anthocyanins protect against kainic acid-induced excitotoxicity and apoptosis via ROS-activated AMPK pathway in hippocampal neurons. CNS Neurosci. Ther. 20, 327–338. doi: 10.1111/cns.12218
Ullah, R., Khan, M., Shah, S. A., Saeed, K., Kim, M. O. (2019). Natural Antioxidant Anthocyanins-A Hidden Therapeutic Candidate in Metabolic Disorders with Major Focus in Neurodegeneration. Nutrients 11. doi: 10.3390/nu11061195
Vaidyanathan, J. B., Walle, T. (2001). Transport and metabolism of the tea flavonoid (-)-epicatechin by the human intestinal cell line Caco-2. Pharm. Res. 18, 1420–1425. doi: 10.1023/A:1012200805593
Van Langenhove, T., Van Der Zee, J., Van Broeckhoven, C. (2012). The molecular basis of the frontotemporal lobar degeneration-amyotrophic lateral sclerosis spectrum. Ann. Med. 44, 817–828. doi: 10.3109/07853890.2012.665471
Vendrame, S., Daugherty, A., Kristo, A. S., Riso, P., Klimis-Zacas, D. (2013). Wild blueberry (Vaccinium angustifolium) consumption improves inflammatory status in the obese Zucker rat model of the metabolic syndrome. J. Nutr. Biochem. 24, 1508–1512. doi: 10.1016/j.jnutbio.2012.12.010
Virgili, F., Marino, M. (2008). Regulation of cellular signals from nutritional molecules: a specific role for phytochemicals, beyond antioxidant activity. Free Radic. Biol. Med. 45, 1205–1216. doi: 10.1016/j.freeradbiomed.2008.08.001
Vivarelli, F., Canistro, D., Babot Marquillas, C., Cirillo, S., De Nicola, G. R., Iori, R., et al. (2018). The combined effect of Sango sprout juice and caloric restriction on metabolic disorders and gut microbiota composition in an obesity model. Int. J. Food Sci. Nutr. 69, 192–204. doi: 10.1080/09637486.2017.1350940
Wallace, T. C., Giusti, M. M. (2013). Anthocyanins in health and disease (Boca Raton: CRC Press). doi: 10.1201/b15554
Walton, M. C., Mcghie, T. K., Reynolds, G. W., Hendriks, W. H. (2006a). The flavonol quercetin-3-glucoside inhibits cyanidin-3-glucoside absorption in vitro. J. Agric. Food Chem. 54, 4913–4920. doi: 10.1021/jf0607922
Walton, M. C., Mcghie, T. K., Reynolds, G. W., Hendriks, W. H. (2006b). The Flavonol Quercetin-3-Glucoside Inhibits Cyanidin-3-Glucoside Absorption in Vitro. J. Agric. Food Chem. 54, 4913–4920. doi: 10.1021/jf0607922
Wang, D., Wei, X., Yan, X., Jin, T., Ling, W. (2010). Protocatechuic acid, a metabolite of anthocyanins, inhibits monocyte adhesion and reduces atherosclerosis in apolipoprotein E-deficient mice. J. Agric. Food Chem. 58, 12722–12728. doi: 10.1021/jf103427j
Wang, X., Wang, W., Li, L., Perry, G., Lee, H. G., Zhu, X. (2014). Oxidative stress and mitochondrial dysfunction in Alzheimer’s disease. Biochim. Biophys. Acta 1842, 1240–1247. doi: 10.1016/j.bbadis.2013.10.015
Warner, L. M. (2015). Handbook of Anthocyanins: Food Sources, Chemical Applications and Health Benefits (Incorporated: Nova Science Publishers).
Wei, H., Li, H., Wan, S.-P., Zeng, Q.-T., Cheng, L.-X., Jiang, L.-L., et al. (2017). Cardioprotective Effects of Malvidin Against Isoproterenol-Induced Myocardial Infarction in Rats: A Mechanistic Study. Med. Sci. Monitor 23, 2007–2016. doi: 10.12659/MSM.902196
Wei, J., Zhang, G., Zhang, X., Xu, D., Gao, J., Fan, J., et al. (2017). Anthocyanins from Black Chokeberry (Aroniamelanocarpa Elliot) Delayed Aging-Related Degenerative Changes of Brain. J. Agric. Food Chem. 65, 5973–5984. doi: 10.1021/acs.jafc.7b02136
Winter, A. N., Ross, E. K., Wilkins, H. M., Stankiewicz, T. R., Wallace, T., Miller, K., et al. (2018). An anthocyanin-enriched extract from strawberries delays disease onset and extends survival in the hSOD1(G93A) mouse model of amyotrophic lateral sclerosis. Nutr. Neurosci. 21, 414–426. doi: 10.1080/1028415X.2017.1297023
Wu, X., Kang, J., Xie, C., Burris, R., Ferguson, M. E., Badger, T. M., et al. (2010). Dietary Blueberries Attenuate Atherosclerosis in Apolipoprotein E-Deficient Mice by Upregulating Antioxidant Enzyme Expression. J. Nutr. 140, 1628–1632. doi: 10.3945/jn.110.123927
Wu, T., Tang, Q., Gao, Z., Yu, Z., Song, H., Zheng, X., et al. (2013). Blueberry and mulberry juice prevent obesity development in C57BL/6 mice. PloS One 8, e77585. doi: 10.1371/journal.pone.0077585
Wu, T., Jiang, Z., Yin, J., Long, H., Zheng, X. (2016a). Anti-obesity effects of artificial planting blueberry (Vaccinium ashei) anthocyanin in high-fat diet-treated mice. Int. J. Food Sci. Nutr. 67, 257–264. doi: 10.3109/09637486.2016.1146235
Wu, T., Yin, J., Zhang, G., Long, H., Zheng, X. (2016b). Mulberry and cherry anthocyanin consumption prevents oxidative stress and inflammation in diet-induced obese mice. Mol. Nutr. Food Res. 60, 687–694. doi: 10.1002/mnfr.201500734
Wyspianska, D., Kucharska, A. Z., Sokol-Letowska, A., Kolniak-Ostek, J. (2019). Effect of microencapsulation on concentration of isoflavones during simulated in vitro digestion of isotonic drink. Food Sci. Nutr. 7, 805–816. doi: 10.1002/fsn3.929
Xia, M., Ling, W. H., Ma, J., Kitts, D. D., Zawistowski, J. (2003). Supplementation of diets with the black rice pigment fraction attenuates atherosclerotic plaque formation in apolipoprotein E deficient mice. J. Nutr. 133, 744–751. doi: 10.1093/jn/133.3.744
Xia, X., Ling, W., Ma, J., Xia, M., Hou, M., Wang, Q., et al. (2006). An anthocyanin-rich extract from black rice enhances atherosclerotic plaque stabilization in apolipoprotein E-deficient mice. J. Nutr. 136, 2220–2225. doi: 10.1093/jn/136.8.2220
Xia, Y., Shen, S., Verma, I. M. (2014). NF- B, an Active Player in Human Cancers. Cancer Immunol. Res. 2, 823–830. doi: 10.1158/2326-6066.CIR-14-0112
Xie, L., Su, H., Sun, C., Zheng, X., Chen, W. (2018). Recent advances in understanding the anti-obesity activity of anthocyanins and their biosynthesis in microorganisms. Trends Food Sci. Technol. 72, 13–24. doi: 10.1016/j.tifs.2017.12.002
Yang, Y., Andrews, M. C., Hu, Y., Wang, D., Qin, Y., Zhu, Y., et al. (2011). Anthocyanin extract from black rice significantly ameliorates platelet hyperactivity and hypertriglyceridemia in dyslipidemic rats induced by high fat diets. J. Agric. Food Chem. 59, 6759–6764. doi: 10.1021/jf201079h
Yang, Y., Wang, H., Kouadir, M., Song, H., Shi, F. (2019). Recent advances in the mechanisms of NLRP3 inflammasome activation and its inhibitors. Cell Death Dis. 10, 128. doi: 10.1038/s41419-019-1413-8
Ye, J., Meng, X., Yan, C., Wang, C. (2010). Effect of purple sweet potato anthocyanins on beta-amyloid-mediated PC-12 cells death by inhibition of oxidative stress. Neurochem. Res. 35, 357–365. doi: 10.1007/s11064-009-0063-0
Yin, M. C., Wang, Z. H., Liu, W. H., Mong, M. C. (2017). Aqueous Extract of Gynura Bicolor Attenuated Hepatic Steatosis, Glycative, Oxidative, and Inflammatory Injury Induced by Chronic Ethanol Consumption in Mice. J. Food Sci. 82, 2746–2751. doi: 10.1111/1750-3841.13930
Youdim, K. A., Martin, A., Joseph, J. A. (2000). Incorporation of the elderberry anthocyanins by endothelial cells increases protection against oxidative stress. Free Radical Biol. Med. 29, 51–60. doi: 10.1016/S0891-5849(00)00329-4
Youdim, K. A., Mcdonald, J., Kalt, W., Joseph, J. A. (2002). Potential role of dietary flavonoids in reducing microvascular endothelium vulnerability to oxidative and inflammatory insults. J. Nutr. Biochem. 13, 282–288. doi: 10.1016/S0955-2863(01)00221-2
Zhang, W., Wang, T., Pei, Z., Miller, D. S., Wu, X., Block, M. L., et al. (2005). Aggregated alpha-synuclein activates microglia: a process leading to disease progression in Parkinson’s disease. FASEB J. 19, 533–542. doi: 10.1096/fj.04-2751com
Zhang, B., Kang, M., Xie, Q., Xu, B., Sun, C., Chen, K., et al. (2011). Anthocyanins from Chinese bayberry extract protect beta cells from oxidative stress-mediated injury via HO-1 upregulation. J. Agric. Food Chem. 59, 537–545. doi: 10.1021/jf1035405
Zhang, Z. F., Lu, J., Zheng, Y. L., Wu, D. M., Hu, B., Shan, Q., et al. (2013). Purple sweet potato color attenuates hepatic insulin resistance via blocking oxidative stress and endoplasmic reticulum stress in high-fat-diet-treated mice. J. Nutr. Biochem. 24, 1008–1018. doi: 10.1016/j.jnutbio.2012.07.009
Zhang, X., Zhu, Y., Song, F., Yao, Y., Ya, F., Li, D., et al. (2016). Effects of purified anthocyanin supplementation on platelet chemokines in hypocholesterolemic individuals: a randomized controlled trial. Nutr. Metab. (Lond) 13, 86. doi: 10.1186/s12986-016-0146-2
Zhang, J., Wu, J., Liu, F., Tong, L., Chen, Z., Chen, J., et al. (2019). Neuroprotective effects of anthocyanins and its major component cyanidin-3-O-glucoside (C3G) in the central nervous system: An outlined review. Eur. J. Pharmacol. 858, 172500. doi: 10.1016/j.ejphar.2019.172500
Zhao, M., Wang, P., Zhu, Y., Liu, X., Hu, X., Chen, F. (2015). Blueberry anthocyanins extract inhibits acrylamide-induced diverse toxicity in mice by preventing oxidative stress and cytochrome P450 2E1 activation. J. Funct. Foods 14, 95–101. doi: 10.1016/j.jff.2015.01.035
Zhou, J., Zhu, Y.-F., Chen, X.-Y., Han, B., Li, F., Chen, J.-Y., et al. (2017). Black rice-derived anthocyanins inhibit HER-2-positive breast cancer epithelial-mesenchymal transition-mediated metastasis in vitro by suppressing FAK signaling. Int. J. Mol. Med. 40, 1649–1656. doi: 10.3892/ijmm.2017.3183
Zhou, L., Wang, H., Yi, J., Yang, B., Li, M., He, D., et al. (2018). Anti-tumor properties of anthocyanins from Lonicera caerulea ‘Beilei’ fruit on human hepatocellular carcinoma: In vitro and in vivo study. BioMed. Pharmacother. 104, 520–529. doi: 10.1016/j.biopha.2018.05.057
Zhu, W., Jia, Q., Wang, Y., Zhang, Y., Xia, M. (2012). The anthocyanin cyanidin-3-O-β-glucoside, a flavonoid, increases hepatic glutathione synthesis and protects hepatocytes against reactive oxygen species during hyperglycemia: Involvement of a cAMP-PKA-dependent signaling pathway. Free Radical Biol. Med. 52, 314–327. doi: 10.1016/j.freeradbiomed.2011.10.483
Ziberna, L., Lunder, M., Moze, S., Vanzo, A., Tramer, F., Passamonti, S., et al. (2010). Acute cardioprotective and cardiotoxic effects of bilberry anthocyanins in ischemia-reperfusion injury: beyond concentration-dependent antioxidant activity. Cardiovasc. Toxicol. 10, 283–294. doi: 10.1007/s12012-010-9091-x
Keywords: anthocyanins, biodisponibility, neuroprotective, cardioprotective, antiobesity, antidiabetic, antioxidant, anticancer
Citation: Salehi B, Sharifi-Rad J, Cappellini F, Reiner Ž, Zorzan D, Imran M, Sener B, Kilic M, El-Shazly M, Fahmy NM, Al-Sayed E, Martorell M, Tonelli C, Petroni K, Docea AO, Calina D and Maroyi A (2020) The Therapeutic Potential of Anthocyanins: Current Approaches Based on Their Molecular Mechanism of Action. Front. Pharmacol. 11:1300. doi: 10.3389/fphar.2020.01300
Received: 29 May 2020; Accepted: 05 August 2020;
Published: 26 August 2020.
Edited by:
Yue Liu, Xiyuan Hospital, ChinaReviewed by:
Gao Zhu Ye, China Academy of Chinese Medical Sciences, ChinaYouhua Xu, Macau University of Science and Technology, Macau
Copyright © 2020 Salehi, Sharifi-Rad, Cappellini, Reiner, Zorzan, Imran, Sener, Kilic, El-Shazly, Fahmy, Al-Sayed, Martorell, Tonelli, Petroni, Docea, Calina and Maroyi. This is an open-access article distributed under the terms of the Creative Commons Attribution License (CC BY). The use, distribution or reproduction in other forums is permitted, provided the original author(s) and the copyright owner(s) are credited and that the original publication in this journal is cited, in accordance with accepted academic practice. No use, distribution or reproduction is permitted which does not comply with these terms.
*Correspondence: Javad Sharifi-Rad, javad.sharifirad@gmail.com; Miquel Martorell, mmartorell@udec.cl; Katia Petroni, katia.petroni@unimi.it; Daniela Calina, calinadaniela@gmail.com; Alfred Maroyi, amaroyi@ufh.ac.za
†These authors have contributed equally to this work and share first authorship