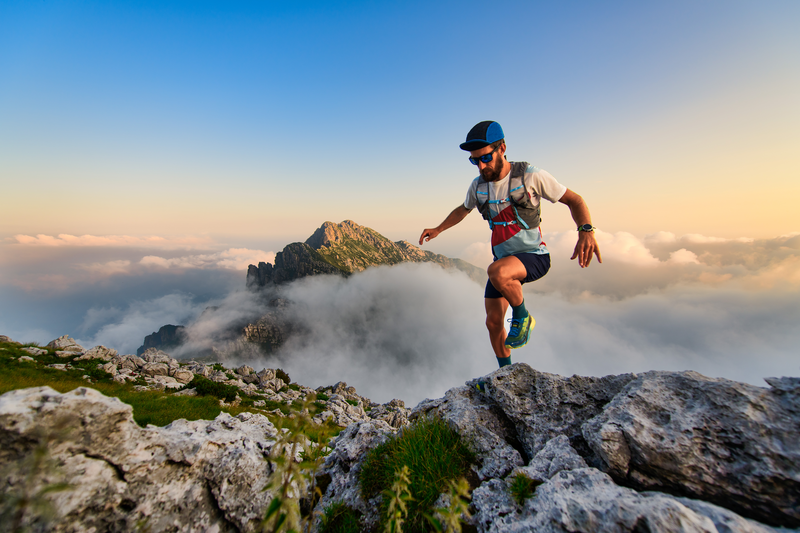
94% of researchers rate our articles as excellent or good
Learn more about the work of our research integrity team to safeguard the quality of each article we publish.
Find out more
ORIGINAL RESEARCH article
Front. Pediatr. , 14 November 2024
Sec. Pediatric Endocrinology
Volume 12 - 2024 | https://doi.org/10.3389/fped.2024.1438195
Background: Previous observational research has demonstrated a possible association between the gut microbiota (GM) and central precocious puberty (CPP). Nevertheless, whether there is a causal relationship between the GM and CPP is uncertain due to the possibility of confounding factors influencing the result.
Methods: We collected summary data from genome-wide association studies of the GM (MiBioGen, n = 18,340) and CPP (FinnGen Consortium, 185 case groups and 395,289 controls). Most of the participants were of European origin. Mendelian randomization analysis was utilized to investigate the causal relationship between the GM and CPP using the inverse-variance weighted average technique, the weighted median, and Mendelian randomization Egger. The reliability of the results was evaluated using the leave-one-out test and sensitivity analyses, including heterogeneity and horizontal pleiotropy testing.
Results: According to the inverse-variance weighted average technique, there was a substantial correlation between CPP and the composition of the GM. Specifically, the relative abundance of the genus Bacteroides (OR 0.222, 95% CI 0.06–0.822, P = 0.024) and Alistipes (OR 0.197, 95% CI 0.056–0.697, P = 0.012), and others, showed significant associations. Furthermore, associations with the phylum Euryarchaeota, the orders Gastranaerophilales, and Rhodospirillales, the families Bacteroidaceae, and Desulfovibrionaceae were also observed. Sensitivity analyses and the leave-one-out test generated positive results for the genus Alistipes, implying that this genus is reliable and reduces the risk of CPP.
Conclusions: The composition of the GM may have a causal effect on CPP. The present finding that Alistipes may be protective against CPP is expected to offer novel insights into the management of CPP.
The term “central precocious puberty” (CPP) refers to the early onset of secondary sexual characteristics in girls and boys before the ages of 8 and 9, respectively, due to the hypothalamic-pituitary-gonadal axis (HPGA) activation (1). The global incidence of CPP has significantly increased in the last two decades (2). One in 5,000–10,000 children are affected by CPP, which is 5–10 times more common in girls than in boys, according to an epidemiologic study (3). The etiology of CPP is gradually becoming elucidated, but a definitive mechanism of development of the condition in approximately 90% of patients remains elusive, and it is therefore designated as idiopathic central precocious puberty (1, 4, 5). The early onset of puberty not only affects growth and developmental processes, but also increases the risk of developing various diseases, including depression, cardiovascular disease, obesity, and cancer (6–11). Consequently, the prevention and treatment of CPP are important for good physical and mental health.
The gut microbiota (GM), an intricate microbial ecosystem (12), is closely associated with many host diseases (13). Recent research has demonstrated that the GM produces metabolites, neurotransmitters, and neuroactive compounds that can act on the central nervous system to regulate sex hormone secretion via the gut-brain axis (14, 15). In particular, Wang et al. (16) showed that the intestinal microbiota and its products reverse precocious puberty in rats by inhibiting the secretion of gonadotropin-releasing hormone (GnRH) and the HPGA. This provides a new perspective regarding the pathogenesis of CPP.
According to the randomization principle, Mendelian randomization (MR) employs single nucleotide polymorphisms (SNPs) as instrumental variables (IVs) to eliminate confounding and potential reverse causation and enable an accurate evaluation of the underlying causality between exposure factors and disease risk (17–20). Utilizing data from the genome-wide association study (GWAS), we conducted a two-sample MR analysis in this study to assess the potential causal relationship between GM and CPP, and thereby aid the management of CPP.
Genetic variants linked to the exposure variables obtained from the GWAS summary statistics were utilized as IVs in our study, which also used GM taxa as exposure factors and CPP as the outcome factor (Figure 1). The primary analytical means of MR analysis that we employed was the inverse-variance weighted (IVW) method. Additionally, sensitivity and leave-one-out analyses were conducted to verify the reliability of the results. The MR analysis was performed having established that the following conditions were satisfied: (i) the IV and exposure had a strong correlation; (ii) the IV was unrelated to any confounding factors; and (iii) the IV only influenced the outcome through the target exposure.
The GWAS data for the GM came from the MiBioGen study, which assembled the 16S rRNA gene sequencing profiles of 18,340 individuals (13,266 from European populations) and included 211 units (comprising families, phyla, orders, genera, and classes) (21). These data were accessed from the study website (www.mibiogen.org). The GWAS data for CPP were sourced from the FinnGen Consortium R10 release (https://r10.finngen.fi/), which included 185 case groups and 395,289 controls overall (22). All of the study's participants were from Europe.
SNPs in the genes representing the exposures that were extracted from original GWAS meta-analyses or public databases were used as the instrumental variables (IVs) for the exposure factors. A threshold for genome-wide significance (P < 1 × 10−5) was established in order to achieve a thorough comprehension of the exposure to genetic variation. Concurrently, to ensure the independence of the IVs and mitigate linkage disequilibrium, we stipulated an r2 < 0.01, and a clumping window size of 500 kb (23). Finally, we discarded SNPs with an F statistic < 10 to ensure only close associations between exposure factors and IV would be obtained. Equation 1 is the formula used to calculate the F statistic, where R2 is the percentage of exposure variance explained by a particular SNP and N is the sample size (24). R2 was determined using the Equation 2.
From the original GWAS meta-analyses, SNPs connected with relevant diseases were taken out and used as IVs for the outcome variables. SNPs that exhibited a close association with the relevant exposure diseases and were also present in the outcome variables were then screened.
The harmonization of selected IVs with outcome-associated SNPs was used to eliminate palindromic sequences and allele-incompatible SNPs. Furthermore, to accept the assumption of exclusivity for this MR study, we required an outcome-related SNP significance level of P > 1 × 10 −5. Anomalous outliers were removed using the MR_radial. Li et al. (25) demonstrated that CPP is associated with obesity, the consumption of beverages and sweets, and sleep quality. Therefore, to remove the influence of these confounding factors, we conducted a systematic search of the PhenoScanner database (http://www.phenoscanner.medschl.cam.ac.uk/) to remove the associated SNPs.
We used the weighted median (WM) and MR–Egger as auxiliary methods in addition to the IVW method as the primary strategy for the MR analysis. When using the IVW approach, it is assumed that each IV is valid and does not exhibit pleiotropy. However, this approach does not take into account the presence of an intercept, which resists bias and has the highest statistical efficacy (26). The MR–Egger method considers the intercept and is susceptible to the effect of anomalous IVs, but can generate unbiased estimates, even using invalid IVs (27). It has been established that the WM can be calculated using a considerable proportion of invalid IVs (approximately 50%), and generates a higher causal efficacy and lower error than the MR–Egger (28). Finally, a stable causal relationship between exposures and outcomes was only considered if P < 0.05 for the IVW.
Sensitivity analyses, such as heterogeneity and horizontal pleiotropy tests, were performed to evaluate the robustness of the primary findings. The heterogeneity of the SNPs was evaluated using Cochran's Q-test, and the horizontal pleiotropy was evaluated using the MR–Egger intercept. When P > 0.05, the lack of heterogeneity or pleiotropy was acknowledged. Additionally, each SNP's impact on causation was assessed using the leave-one-out test (29).
We used the “TwoSampleMR” package in the R software environment (version 4.3.2) to perform these analyses.
We obtained 2,641 eligible SNPs and 196 gut microbial taxa using the described screening criteria for IVs, performing allelic consistency testing, and removing confounders. The F-statistic values for these SNPs were all >10, indicating a stable statistical effect among the selected IVs (Supplementary File S1, Table S1).
We performed MR analysis using three methods (WM, IVW, and MR–Egger), with the results of the IVW method serving as the primary index. The detailed findings are shown in Supplementary File S1, Table S2. Based on the IVW analysis, 10 gut bacterial taxa had P-values below the threshold of <0.05 and were therefore included in the subsequent analysis (Table 1). We used MR_radial to remove the outliers and after repeated causal analyses found that one phylum (IVW: OR 0.536, 95% CI 0.31–0.926, P = 0.025 for Euryarchaeota), two orders (IVW: OR 0.446, 95% CI 0.202–0.987, P = 0.046 for Gastranaerophilales; OR 2.079, 95% CI 1.003–4.309, P = 0.049 for Rhodospirillales), families (IVW: OR 0.222, 95% CI 0.06–0.822, P = 0.024 for Bacteroidaceae; OR 0.250, 95% CI 0.07–0.900, P = 0.034 for Desulfovibrionaceae), and genus (IVW: OR 0.222, 95% CI 0.06–0.822, P = 0.024 for Bacteroides; OR 0.197, 95% CI 0.056–0.697, P = 0.012 for Alistipes) were significantly associated with CPP (Table 1).
Finally, in conjunction with the IVW results, we performed leave-one-out testing, and only obtained a positive result for the genus Alistipes (OR 0.197, 95% CI 0.056–0.697, P = 0.012), implying that this finding was stable and that it is protective against CPP (Figure 2). It should be noted that, because MR–Egger does not necessitate a forced regression to the origin and exhibits limited statistical efficacy, it is acceptable to present findings that are in the direction opposite to that obtained using the IVW and WM methods. After having performed leave-one-out testing, an additional six gut bacterial taxa were excluded, owing to their influence on single SNPs, which resulted in unstable results. Additional information is provided in Supplementary Figure 1. Notably, we did not detect weak instrumental bias (F > 10), horizontal pleiotropy (MR–Egger intercept P > 0.05), or heterogeneity (Cochran's Q-test P > 0.05) (Table 2).
Figure 2. (A) Scatter plots illustrating the causal effect of genus Alistipes on central precocious puberty; (B) Leave-one-out analysis for genus Alistipes on central precocious puberty; (C) MR effect size for genus Alistipes on central precocious puberty.
In this research, we novelly conducted a two-sample MR study to determine the specific causal effects of 196 gut microbial taxa on accelerating or inhibiting the development of CPP. The causal evaluations were examined and verified through multiple analyses, including IVW, WM, MR-Egger, sensitivity, and leave-one-out tests. IVW estimates revealed that the phylum Euryarchaeota, the order Candidatus Gastranaerophilales, the family Bacteroidaceae, the family Desulfovibrionaceae, the genus Bacteroides, and the genus Alistipes could potentially reduce the risk of CPP while the order Rhodospirillales might enhance the risk of CPP. Eventually, after sensitivity analyses and the leave-one-out test, the genus Alistipes was suggested to stably prevent the occurrence of CPP causally. Our findings elucidate whether the human GM can participate in the pathogenesis of CPP and which gut microbial taxon can alter the risk of CPP.
Driven by the early activation of HPGA, the initiation of CPP is highly related to disorders in the endocrine system, especially abnormal sexual maturation and secretion of sex hormones (30). Meanwhile, GM has been demonstrated to play an important role in the disruption of the hormone system and the progression of a series of systemic diseases (31). Since the composition of GM is significantly different in different puberty stages, the effect of GM during the physiological pubertal development may further indicate the potential connection between GM and pathological puberty (32). With the proposal of the sex hormone-gut microbiome axis, increasing studies have focused on the role of GM on pubertal disorders, especially precocious puberty, and the underlying mechanisms (33). A study involving 91 CPP patients investigated the alteration of the composition of GM in CPP through bioinformatics and suggested that the genus Streptococcus could act as a marker for CPP (34). Another observational research identified GM dysbiosis within 25 CPP patients and altered gut microbial taxa in CPP were similar to those in obesity, while obesity had already been recognized as the main cause for CPP (35, 36). In addition, most enriched gut genera in CPP were defined as short-chain fatty acids (SCFAs)-producing bacteria. At the same time, SCFAs can elevate the expression of metabolic peptides from adipocytes and are essential for obesity-induced precocious puberty (16). However, limited populations as well as potential reverse causality and confounders in observational studies hinder the exploration of the causal effects of GM on the risk of CPP. Although existing research has indicated that GM might be correlated to CPP, whether the links in phenotype were solely clinically manifested, achieved through obesity, or causal remains unknown. Therefore, we carried out MR research based on the large-scale GWAS data that can successfully avoid reverse causalities and confounding factors to investigate the causal associations directly between GM and CPP. As a result, we identified the causal impacts of GM on the risk of CPP, which not only was consistent in previous observational studies but also provided genetic evidence for their strong causal links. In terms of mechanisms underlying the causalities, according to a narrative review, GM can produce several metabolically active substances, together with GM, both have been shown to influence sex hormone secretion through nutritional status, hormone regulation, and metabolic pathways (37). On the one hand, GM can directly alter the level of estrogens and androgens in the host organism (38). On the other hand, as typical GM metabolites, SCFAs (31) (mainly consisting of acetate, propionate, and butyrate), neurotransmitters (14) (e.g., serotonin and dopamine), and neuroactive compounds (34) (such as nitric oxide), are important mediators in the gut-brain axis and the transduction of sex hormone signaling, probably influencing the progression of CPP.
Within positive IVW estimates, Bacteroides was found to be reduced in individuals with obesity by several animal and human studies (39–41). The genus Bacteroides principally produces propionate, which is anti-lipogenic (42) and anti-inflammatory (43), enhancing satiety (44), and ameliorating insulin resistance (45). The negative links between the abundance of Bacteroides with obesity might support our results that the family Bacteroidaceae and the genus Bacteroides could inhibit the occurrence of CPP. Previous studies also showed that propionate and butyrate activated the HPGA by increasing leptin gene expression (46). As for the family Desulfovibrionaceae, an observational study showed that the abundance of Desulfovibrio in the feces of obese mice was positively correlated with the circulating concentration of GnRH (15), which might promote intestinal inflammation and sexual development (47, 48). Our MR research further identified the impact of the family Desulfovibrionaceae in causally decreasing the risk of CPP. Additionally, as a CPP promotion taxa suggested by our MR research, the order Rhodospirillales is recognized as a proinflammatory factor, and its metabolites such as lipopolysaccharide could participate in inflammation and immune responses in hosts (49). Furthermore, the order Rhodospirillales is also associated with amino acids, nitrogen, vitamins, and cofactor metabolisms, which might affect the production of sex hormones and the development of CPP (50).
To be noted, the genus Alistipes was the exclusive positive taxa causally related to the risk of CPP after being verified via the leave-one-out test. Alistipes is a recently discovered genus of anaerobic bacteria in the healthy human gut (51) that produces SCFAs (52). Regarding the abundance of Alistipes in patients with CPP, a previous observational study showed that this genus was significantly more abundant in patients with CPP groups than in healthy controls or individuals with over-weight (14). However, another study performed in humans showed no significant difference in the abundance of Alistipes between patients and healthy controls (31). The outcomes of these studies were not consistent, which may be attributable to discrepancies in ethnicity, species, host metabolic status, and/or measurement methods. Our MR research extensively minimized bias and elucidated the protective role of the genus Alistipes for CPP, providing a potential treatment biomarker for CPP. An animal study demonstrated that the addition of acetate, propionate, butyrate, or a combination of these to a high-fat diet reduces GnRH release and reverses precocious puberty (16). Other scholars proposed that Alistipes transplantation could be used to alleviate high-fat diet-induced obesity and its associated complications (53), which also supported our causal estimates. In terms of the underlying mechanism, acetate produced by Alistipes has been reported to regulate lipid metabolism (54) and appetite (55). Butyrate can protect the intestinal mucosa (56) and reduce appetite (57) and the concentrations of inflammatory mediators (58), such as nitric oxide, which acts as a neurotransmitter and promotes the production of sex hormones (59). Furthermore, butyrate is beneficial for mental health (60) and psychological issues have been identified as a significant regulator of sex hormone production (61). However, further research is still required to elucidate the effects of differing abundances of gut microbial taxa and the mechanism of the effects of their metabolites in patients with CPP.
In the present study, we used genetic variables to determine whether there is a causal relationship between exposure factors and the disease of interest, thereby minimizing the effects of confounding factors. Nevertheless, it is important to acknowledge the limitations of the study. The participants in the study were predominantly of European origin, and therefore the findings may not be applicable to individuals of other ethnic origins. Furthermore, the abundances and effects of gut microbial taxa are influenced by several factors, including age, sex, and dietary habits. However, we did not perform subgroup analyses in the present study.
In the present study, we have shown that Alistipes may be protective against CPP. This finding provides novel information to aid the management of CPP. Nevertheless, further large-scale animal and human studies are required to elucidate the mechanism whereby the composition of the GM affects CPP and to develop related strategies for the prevention and treatment of CPP.
The original contributions presented in the study are included in the article/Supplementary Material, further inquiries can be directed to the corresponding author.
MC: Writing – original draft, Conceptualization, Data curation, Formal Analysis, Investigation, Methodology, Project administration, Resources, Software, Validation, Visualization. XH: Conceptualization, Data curation, Methodology, Visualization, Writing – original draft. WH: Conceptualization, Software, Writing – original draft. CD: Writing – review & editing, Supervision.
The author(s) declare that no financial support was received for the research, authorship, and/or publication of this article.
The authors appreciate the MiBioGen consortium for providing the gut microbiota GWAS summary statistics, as well as the researchers and participants of the FinnGen study.
The authors declare that the research was conducted in the absence of any commercial or financial relationships that could be construed as a potential conflict of interest.
All claims expressed in this article are solely those of the authors and do not necessarily represent those of their affiliated organizations, or those of the publisher, the editors and the reviewers. Any product that may be evaluated in this article, or claim that may be made by its manufacturer, is not guaranteed or endorsed by the publisher.
The Supplementary Material for this article can be found online at: https://www.frontiersin.org/articles/10.3389/fped.2024.1438195/full#supplementary-material
Supplementary Figure 1 | Leave-one-out sensitivity analysis for phylum Euryarchaeota, order Gastranaerophilales, order Rhodospirillales, family Bacteroidaceae, family Desulfovibrionaceae, and genus Bacteroides on central precocious puberty.
GM, gut microbiota; CPP, central precocious puberty; HPGA, hypothalamic-pituitary-gonadal axis; GnRH, gonadotropin-releasing hormone; MR, Mendelian randomization; SNP, single nucleotide polymorphism; IV, instrumental variable; GWAS, genome-wide association study; IVW, inverse-variance weighted; WM, weighted median; SCFAs, short-chain fatty acids.
1. Latronico AC, Brito VN, Carel JC. Causes, diagnosis, and treatment of central precocious puberty. Lancet Diabetes Endocrinol. (2016) 4(3):265–74. doi: 10.1016/S2213-8587(15)00380-0
2. Bräuner EV, Busch AS, Eckert-Lind C, Koch T, Hickey M, Juul A. Trends in the incidence of central precocious puberty and normal variant puberty among children in Denmark, 1998 to 2017. JAMA Network Open. (2020) 3(10):e2015665. doi: 10.1001/jamanetworkopen.2020.15665
3. Cesario SK, Hughes LA. Precocious puberty: a comprehensive review of literature. J Obstet Gynecol Neonatal Nurs. (2007) 36(3):263–74. doi: 10.1111/j.1552-6909.2007.00145.x
4. Cisternino M, Arrigo T, Pasquino AM, Tinelli C, Antoniazzi F, Beduschi L, et al. Etiology and age incidence of precocious puberty in girls: a multicentric study. J Pediatr Endocrinol& Metab. (2000) 13(Suppl 1):695–701. doi: 10.1515/jpem.2000.13.s1.695
5. Partsch CJ, Heger S, Sippell WG. Management and outcome of central precocious puberty. Clin Endocrinol (Oxf). (2002) 56(2):129–48. doi: 10.1046/j.0300-0664.2001.01490.x
6. Day FR, Elks CE, Murray A, Ong KK, Perry JR. Puberty timing associated with diabetes, cardiovascular disease and also diverse health outcomes in men and women: the UK Biobank study. Sci Rep. (2015) 5:11208. doi: 10.1038/srep11208
7. Prentice P, Viner RM. Pubertal timing and adult obesity and cardiometabolic risk in women and men: a systematic review and meta-analysis. Int J Obes (Lond). (2013) 37(8):1036–43. doi: 10.1038/ijo.2012.177
8. Ong KK, Bann D, Wills AK, Ward K, Adams JE, Hardy R, et al. Timing of voice breaking in males associated with growth and weight gain across the life course. J Clin Endocrinol Metab. (2012) 97(8):2844–52. doi: 10.1210/jc.2011-3445
9. Hamlat EJ, Stange JP, Abramson LY, Alloy LB. Early pubertal timing as a vulnerability to depression symptoms: differential effects of race and sex. J Abnorm Child Psychol. (2014) 42(4):527–38. doi: 10.1007/s10802-013-9798-9
10. Day FR, Thompson DJ, Helgason H, Chasman DI, Finucane H, Sulem P, et al. Genomic analyses identify hundreds of variants associated with age at menarche and support a role for puberty timing in cancer risk. Nat Genet. (2017) 49(6):834–41. doi: 10.1038/ng.3841
11. Bonilla C, Lewis SJ, Martin RM, Donovan JL, Hamdy FC, Neal DE, et al. Pubertal development and prostate cancer risk: Mendelian randomization study in a population-based cohort. BMC Med. (2016) 14:66. doi: 10.1186/s12916-016-0602-x
12. Vemuri R, Gundamaraju R, Shastri MD, Shukla SD, Kalpurath K, Ball M, et al. Gut microbial changes, interactions, and their implications on human lifecycle: an ageing perspective. BioMed Res Int. (2018) 2018:4178607. doi: 10.1155/2018/4178607
13. Illiano P, Brambilla R, Parolini C. The mutual interplay of gut microbiota, diet and human disease. FEBS J. (2020) 287(5):833–55. doi: 10.1111/febs.15217
14. Li Y, Shen L, Huang C, Li X, Chen J, Li SC, et al. Altered nitric oxide induced by gut microbiota reveals the connection between central precocious puberty and obesity. Clin Transl Med. (2021) 11(2):e299. doi: 10.1002/ctm2.299
15. Bo T, Liu M, Tang L, Lv J, Wen J, Wang D. Effects of high-fat diet during childhood on precocious puberty and Gut Microbiota in mice. Front Microbiol. (2022) 13:930747. doi: 10.3389/fmicb.2022.930747
16. Wang L, Xu H, Tan B, Yi Q, Liu H, Deng H, et al. Gut microbiota and its derived SCFAs regulate the HPGA to reverse obesity-induced precocious puberty in female rats. Front Endocrinol (Lausanne). (2022) 13:1051797. doi: 10.3389/fendo.2022.1051797
17. Burgess S, Small DS, Thompson SG. A review of instrumental variable estimators for Mendelian randomization. Stat Methods Med Res. (2017) 26(5):2333–55. doi: 10.1177/0962280215597579
18. Sekula P, Del Greco MF, Pattaro C, Köttgen A. Mendelian Randomization as an approach to assess causality using observational data. J Am Soc Nephrol. (2016) 27(11):3253–65. doi: 10.1681/ASN.2016010098
19. Davies NM, Holmes MV, Davey Smith G. Reading Mendelian randomisation studies: a guide, glossary, and checklist for clinicians. BMJ. (2018) 362:k601. doi: 10.1136/bmj.k601
20. Skrivankova VW, Richmond RC, Woolf BAR, Yarmolinsky J, Davies NM, Swanson SA, et al. Strengthening the reporting of observational studies in epidemiology using Mendelian randomization: the STROBE-MR statement. JAMA. (2021) 326(16):1614–21. doi: 10.1001/jama.2021.18236
21. Kurilshikov A, Medina-Gomez C, Bacigalupe R, Radjabzadeh D, Wang J, Demirkan A, et al. Large-scale association analyses identify host factors influencing human gut microbiome composition. Nat Genet. (2021) 53(2):156–65. doi: 10.1038/s41588-020-00763-1
22. Kurki MI, Karjalainen J, Palta P, Sipilä TP, Kristiansson K, Donner KM, et al. Finngen provides genetic insights from a well-phenotyped isolated population. Nature. (2023) 613(7944):508–18. doi: 10.1038/s41586-022-05473-8
23. Auton A, Brooks LD, Durbin RM, Garrison EP, Kang HM, Korbel JO, et al. A global reference for human genetic variation. Nature. (2015) 526(7571):68–74. doi: 10.1038/nature15393
24. Burgess S, Thompson SG. Avoiding bias from weak instruments in Mendelian randomization studies. Int J Epidemiol. (2011) 40(3):755–64. doi: 10.1093/ije/dyr036
25. Shi L, Jiang Z, Zhang L. Childhood obesity and central precocious puberty. Front Endocrinol (Lausanne). (2022) 13:1056871. doi: 10.3389/fendo.2022.1056871
26. Holmes MV, Ala-Korpela M, Smith GD. Mendelian Randomization in cardiometabolic disease: challenges in evaluating causality. Nat Rev Cardiol. (2017) 14(10):577–90. doi: 10.1038/nrcardio.2017.78
27. Bowden J, Del Greco MF, Minelli C, Davey Smith G, Sheehan NA, Thompson JR. Assessing the suitability of summary data for two-sample Mendelian randomization analyses using MR-egger regression: the role of the I2 statistic. Int J Epidemiol. (2016) 45(6):1961–74. doi: 10.1093/ije/dyw220
28. Bowden J, Davey Smith G, Haycock PC, Burgess S. Consistent estimation in Mendelian randomization with some invalid instruments using a weighted median estimator. Genet Epidemiol. (2016) 40(4):304–14. doi: 10.1002/gepi.21965
29. Xiang K, Wang P, Xu Z, Hu YQ, He YS, Chen Y, et al. Causal effects of gut microbiome on systemic lupus erythematosus: a two-sample Mendelian randomization study. Front Immunol. (2021) 12:667097. doi: 10.3389/fimmu.2021.667097
30. Zevin EL, Eugster EA. Central precocious puberty: a review of diagnosis, treatment, and outcomes. Lancet Child Adolesc Health. (2023) 7(12):886–96. doi: 10.1016/S2352-4642(23)00237-7
31. Dong G, Zhang J, Yang Z, Feng X, Li J, Li D, et al. The association of gut Microbiota with idiopathic central precocious puberty in girls. Front Endocrinol (Lausanne). (2019) 10:941. doi: 10.3389/fendo.2019.00941
32. Yuan X, Chen R, Zhang Y, Lin X, Yang X. Gut microbiota: effect of pubertal status. BMC Microbiol. (2020) 20(1):334. doi: 10.1186/s12866-020-02021-0
33. Calcaterra V, Rossi V, Massini G, Regalbuto C, Hruby C, Panelli S, et al. Precocious puberty and microbiota: the role of the sex hormone-gut microbiome axis. Front Endocrinol (Lausanne). (2022) 13:1000919. doi: 10.3389/fendo.2022.1000919
34. Huang X, Chen J, Zou H, Huang P, Luo H, Li H, et al. Gut microbiome combined with metabolomics reveals biomarkers and pathways in central precocious puberty. J Transl Med. (2023) 21(1):316. doi: 10.1186/s12967-023-04169-5
35. Burt Solorzano CM, McCartney CR. Obesity and the pubertal transition in girls and boys. Reproduction. (2010) 140(3):399–410. doi: 10.1530/REP-10-0119
36. Reinehr T, Roth CL. Is there a causal relationship between obesity and puberty? Lancet Child Adolesc Health. (2019) 3(1):44–54. doi: 10.1016/S2352-4642(18)30306-7
37. Yue M, Zhang L. Exploring the mechanistic interplay between gut Microbiota and precocious puberty: a narrative review. Microorganisms. (2024) 12(2):323. doi: 10.3390/microorganisms12020323
38. Colldén H, Landin A, Wallenius V, Elebring E, Fändriks L, Nilsson ME, et al. The gut microbiota is a major regulator of androgen metabolism in intestinal contents. Am J Physiol Endocrinol Metab. (2019) 317(6):E1182–e92. doi: 10.1152/ajpendo.00338.2019
39. Thingholm LB, Rühlemann MC, Koch M, Fuqua B, Laucke G, Boehm R, et al. Obese individuals with and without type 2 diabetes show different gut microbial functional capacity and composition. Cell Host Microbe. (2019) 26(2):252–64.e10. doi: 10.1016/j.chom.2019.07.004
40. Liu R, Hong J, Xu X, Feng Q, Zhang D, Gu Y, et al. Gut microbiome and serum metabolome alterations in obesity and after weight-loss intervention. Nat Med. (2017) 23(7):859–68. doi: 10.1038/nm.4358
41. Turnbaugh PJ, Bäckhed F, Fulton L, Gordon JI. Diet-induced obesity is linked to marked but reversible alterations in the mouse distal gut microbiome. Cell Host Microbe. (2008) 3(4):213–23. doi: 10.1016/j.chom.2008.02.015
42. Hosseini E, Grootaert C, Verstraete W, Van de Wiele T. Propionate as a health-promoting microbial metabolite in the human gut. Nutr Rev. (2011) 69(5):245–58. doi: 10.1111/j.1753-4887.2011.00388.x
43. Vinolo MA, Rodrigues HG, Nachbar RT, Curi R. Regulation of inflammation by short chain fatty acids. Nutrients. (2011) 3(10):858–76. doi: 10.3390/nu3100858
44. Arora T, Sharma R, Frost G. Propionate. Anti-obesity and satiety enhancing factor? Appetite. (2011) 56(2):511–5. doi: 10.1016/j.appet.2011.01.016
45. Perry RJ, Peng L, Barry NA, Cline GW, Zhang D, Cardone RL, et al. Acetate mediates a microbiome-brain-β-cell axis to promote metabolic syndrome. Nature. (2016) 534(7606):213–7. doi: 10.1038/nature18309
46. Lin HV, Frassetto A, Kowalik EJ Jr, Nawrocki AR, Lu MM, Kosinski JR, et al. Butyrate and propionate protect against diet-induced obesity and regulate gut hormones via free fatty acid receptor 3-independent mechanisms. PloS One. (2012) 7(4):e35240. doi: 10.1371/journal.pone.0035240
47. Petersen C, Bell R, Klag KA, Lee SH, Soto R, Ghazaryan A, et al. T cell-mediated regulation of the microbiota protects against obesity. Science (New York, NY). (2019) 365(6451):eaat9351. doi: 10.1126/science.aat9351
48. Zhang XY, Chen J, Yi K, Peng L, Xie J, Gou X, et al. Phlorizin ameliorates obesity-associated endotoxemia and insulin resistance in high-fat diet-fed mice by targeting the gut microbiota and intestinal barrier integrity. Gut Microbes. (2020) 12(1):1–18. doi: 10.1080/19490976.2020.1842990
49. Liu K, Zou J, Yuan R, Fan H, Hu H, Cheng Y, et al. Exploring the effect of the gut microbiome on the risk of age-related macular degeneration from the perspective of causality. Invest Ophthalmol Visual Sci. (2023) 64(7):22. doi: 10.1167/iovs.64.7.22
50. McMullen JG, Bueno E, Blow F, Douglas AE. Genome-Inferred correspondence between phylogeny and metabolic traits in the wild Drosophila Gut Microbiome. Genome Biol Evol. (2021) 13(8):evab127. doi: 10.1093/gbe/evab127
51. Parker BJ, Wearsch PA, Veloo ACM, Rodriguez-Palacios A. The genus Alistipes: gut Bacteria with emerging implications to inflammation, cancer, and mental health. Front Immunol. (2020) 11:906. doi: 10.3389/fimmu.2020.00906
52. Shkoporov AN, Chaplin AV, Khokhlova EV, Shcherbakova VA, Motuzova OV, Bozhenko VK, et al. Alistipes inops sp. nov. and Coprobacter secundus sp. nov., isolated from human faeces. Int J Syst Evol Microbiol. (2015) 65(12):4580–8. doi: 10.1099/ijsem.0.000617
53. Jing N, Liu X, Jin M, Yang X, Hu X, Li C, et al. Fubrick tea attenuates high-fat diet induced fat deposition and metabolic disorder by regulating gut microbiota and caffeine metabolism. Food Funct. (2020) 11(8):6971–86. doi: 10.1039/D0FO01282C
54. Yin J, Li Y, Han H, Chen S, Gao J, Liu G, et al. Melatonin reprogramming of gut microbiota improves lipid dysmetabolism in high-fat diet-fed mice. J Pineal Res. (2018) 65(4):e12524. doi: 10.1111/jpi.12524
55. Frost G, Sleeth ML, Sahuri-Arisoylu M, Lizarbe B, Cerdan S, Brody L, et al. The short-chain fatty acid acetate reduces appetite via a central homeostatic mechanism. Nat Commun. (2014) 5:3611. doi: 10.1038/ncomms4611
56. Miquel S, Martín R, Rossi O, Bermúdez-Humarán LG, Chatel JM, Sokol H, et al. Faecalibacterium prausnitzii and human intestinal health. Curr Opin Microbiol. (2013) 16(3):255–61. doi: 10.1016/j.mib.2013.06.003
57. Li Z, Yi CX, Katiraei S, Kooijman S, Zhou E, Chung CK, et al. Butyrate reduces appetite and activates brown adipose tissue via the gut-brain neural circuit. Gut. (2018) 67(7):1269–79. doi: 10.1136/gutjnl-2017-314050
58. Chang PV, Hao L, Offermanns S, Medzhitov R. The microbial metabolite butyrate regulates intestinal macrophage function via histone deacetylase inhibition. Proc Natl Acad Sci U S A. (2014) 111(6):2247–52. doi: 10.1073/pnas.1322269111
59. Ceccatelli S, Hulting AL, Zhang X, Gustafsson L, Villar M, Hökfelt T. Nitric oxide synthase in the rat anterior pituitary gland and the role of nitric oxide in regulation of luteinizing hormone secretion. Proc Natl Acad Sci U S A. (1993) 90(23):11292–6. doi: 10.1073/pnas.90.23.11292
60. Dinan TG, Cryan JF. Melancholic microbes: a link between gut microbiota and depression? Neurogastroenterol Motil. (2013) 25(9):713–9. doi: 10.1111/nmo.12198
Keywords: gut microbiota, central precocious puberty, Mendelian randomization, causal relationship, genetics
Citation: Chen M, Huang X, Huang W and Ding C (2024) Causal relationship between the composition of the Gut Microbiota and central precocious puberty: a two-sample Mendelian randomization study. Front. Pediatr. 12:1438195. doi: 10.3389/fped.2024.1438195
Received: 25 May 2024; Accepted: 28 October 2024;
Published: 14 November 2024.
Edited by:
Mariella Valenzise, University of Messina, ItalyReviewed by:
Domenico Corica, University of Messina, ItalyCopyright: © 2024 Chen, Huang, Huang and Ding. This is an open-access article distributed under the terms of the Creative Commons Attribution License (CC BY). The use, distribution or reproduction in other forums is permitted, provided the original author(s) and the copyright owner(s) are credited and that the original publication in this journal is cited, in accordance with accepted academic practice. No use, distribution or reproduction is permitted which does not comply with these terms.
*Correspondence: Chuangang Ding, ZGwyMjBla0AxNjMuY29t
Disclaimer: All claims expressed in this article are solely those of the authors and do not necessarily represent those of their affiliated organizations, or those of the publisher, the editors and the reviewers. Any product that may be evaluated in this article or claim that may be made by its manufacturer is not guaranteed or endorsed by the publisher.
Research integrity at Frontiers
Learn more about the work of our research integrity team to safeguard the quality of each article we publish.