- Department of Pediatrics, Sheng Jing Hospital of China Medical University, Shenyang, China
Telomere wear and dysfunction may lead to aging-related diseases. Moreover, increasing evidence show that the occurrence, development, and prognosis of some pediatric diseases are also related to telomere dysfunction. In this review, we systematically analyzed the relationship between telomere biology and some pediatric congenital and growth-related diseases and proposed new theoretical basis and therapeutic targets for the treatment of these diseases.
1. Introduction
Telomeres are part of the genome at the end of a linear chromosome. The telomeric DNA of vertebrates is composed of TTAGGG repeats, which bind to a group of proteins that regulate their biological functions and protect them from being recognized as DNA damage. In DNA replication, the wear of the telomere length (TL) accompanies each division; hence, the telomere length gradually shortens with age. Further evidence shows that the TL wear caused by childhood stress and other factors is a risk factor for diseases in adulthood and even in later life (1). Lin et al. (2) proved that the early telomere wear in infants has a certain impact on their long-term growth. Consistent with this, TL abrasion is closely related to the development of the nervous system within 2 years after birth (3, 4). Increasing evidence show that telomere attrition is related to children's growth and pediatric diseases. Thus, we focus on the latest progress in understanding the molecular properties of telomere biology in some pediatric diseases.
2. Telomere dysfunction in preterm infants
Approximately, 15 million children are born preterm (at 37 weeks gestational age) yearly worldwide. Although the mortality rate of premature infants is decreasing with the continuous improvement of rescue technology in the neonatal intensive care unit, more than one million young people subsequently die of premature birth-related diseases each year. The degree of premature delivery is also closely related to the morbidity and mortality of surviving infants. Therefore, more research has been conducted on telomere dysfunction in premature infants (Figure 1 and Table 1); however, to date, the regulatory mechanism of premature infants is still unclear (8, 51–53). However, in detecting the TL of premature infants at birth, studies showed that the TL of premature infants decreased with the increase in gestational age, which was more obvious in premature infants less than 32 weeks of age (54, 55). Casavant et al. (56) demonstrated in a recent study that the mean absolute length of telomeres in the peripheral blood of premature infants is greater than that of adults. The TL of males gradually becomes shorter than that of females with age. They also studied the effects of pain stimuli exposure, feeding methods, and nervous system development on the TL of premature infants. The results show that these factors have no significant effect on the absolute length of telomeres. In contrast to this, some studies showed that exposure to adverse factors (such as pain and stress) during childhood is positively related with telomere shortening, and these factors can also affect their long-term mental health (57–59).
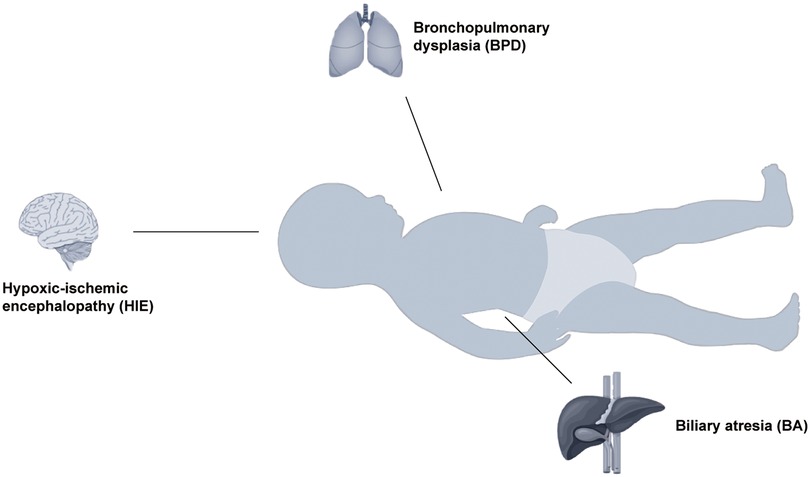
Figure 1. Evidence that telomere dysfunction causes some diseases in infants. For newborns, bronchopulmonary dysplasia is mainly associated with the respiratory system, hypoxic-ischemic encephalopathy with the nervous system, and biliary atresia with the digestive system.
2.1. Congenital biliary atresia (BA)
Congenital BA is the blockage of the intrahepatic and extrahepatic bile conduits, which can prompt cholestatic cirrhosis, and ultimately liver failure. It is one of the indications for liver transplantation in children and one of the most common digestive diseases in neonatal surgery. Patients with BA with hindered bile stream have determined jaundice, earth-like stool, hepatomegaly, and/or splenomegaly. If timely and reasonable intervention is not given in the early stage of the disease, most children may die at 2 years of age, with the cause of death mostly chronic liver disease (severe liver fibrosis, biliary cirrhosis, liver failure, etc.) (60). Hartley et al. (61) reported that the relative length of short telomeres in the peripheral blood of children was associated with the incidence of BA (adjusted for age and sex using logistic regression analysis). Their data also showed that the TL of patients with BA negatively correlated with the degree of liver cirrhosis. TL can also be used as one of the important indicators to evaluate the progress of BA in children (such as liver fibrosis) and is an important reference value for the timing of liver transplantation (5–7).
2.2. Bronchopulmonary dysplasia (BPD)
BPD is a chronic lung infection that causes constant respiratory misery. It is one of the most well-known serious diseases that influence preterm infants, particularly extremely preterm infants (Babies born before 28 weeks of pregnancy). In the long run, prolonged mechanical ventilation or oxygen inhalation may lead to stagnation of lung development, decline of vital capacity, emphysema, pulmonary hypertension, and even neurological and cognitive dysfunction. Nordlund et al. (62) suggested that BPD may damage the lung diffusion capacity of an infant. Airway hyperresponsiveness is more serious in premature infants than in full-term children with asthma at the same age. In a large-scale survey of young people with early pregnancy, Hadchouel et al. (8) showed that a shorter salivary TL was related with hindered lung capability. Previous studies also suggested that TL remained to be associated with forced expiratory flow at 25%–75% of the vital capacity in preterm adolescents, after adjusting for potential confounders by multiple linear regression. Henckel et al. (9) suggested that in children with early BPD, chitinase-3-like protein 1 and relative TL can be used as biomarkers for long-term lung development outcomes.
2.3. Hypoxic-ischemic encephalopathy (HIE)
Neonatal HIE is a severe disease caused by hypoxia in perinatal babies. Its normal cause is the fetal pain in the womb caused by different sources, such as the umbilical cord on the neck and abnormal amniotic fluid. It is very common in children who have experienced serious and life-threatening diseases (hypoxia, neonatal asphyxia) during or after childbirth, and occasionally other diseases that endanger the brain. This ordinarily happens in full-term babies, yet it can likewise happen in untimely newborn children. It has been reported that its incidence rate in live births was 1.5 ‰ (63). A total of 25% of neonates with HIE have severe neurological complications sooner or later (64).Telomerase reverse transcriptase (TERT) encodes rate-limiting catalytic subunits and key regulators of telomerase activity(65). Some studies reported that TERT is a protective molecule against hypoxic-ischemic brain damage. This theory has been verified in HIE models of neonatal rats (10, 11). Li et al. (66) believed that TERT can promote cell proliferation, increase the migration and separation of sensory cells, and then reduce the apoptosis of neurons after hypoxia-ischemia. This theory has been fully confirmed in in vitro experiments. Moreover, the overexpression of TERT can activate myelination in the brain of newborn rodents. In addition, TERT overexpression reduced learning disabilities, memory ability, and neural function after hypoxic-ischemic brain injury. They also conducted in-depth research on the neuroprotective mechanism of TERT and found that the sonic hedgehog/glioma-associated oncogene 1 signaling pathway may play an important and positive role.
3. Telomere dysfunction in nutritional and endocrine diseases in children
Telomere wear may be closely related to some endocrine diseases (such as diabetes) and may increase the early mortality of patients with these diseases. In some economically underdeveloped areas, children are more likely to experience various environmental stress factors, leading to malnutrition, stunting, and even fatality. Increasing evidence shows that telomere dysfunction is related to the nutritional status of and metabolic diseases in children.
3.1. Obesity in children
The incidence rate of childhood obesity is rising rapidly, as well as its related comorbidities, including obstructive sleep apnea syndrome, psychological problems, cardiovascular diseases, type 2 diabetes, and cancer. They seriously threaten the short- and long-term health of children. The telomerase plays a crucial role in human embryonic development. Telomerase action can result in a uniform TL in cells of different tissues, as well as differentiated cells (67–69). The shortening rates of telomeres are high during the first three years of life (approximately 250 bp/year) and reach a stable phase at 4 years of age. The loss rate of telomeres is stable or may decrease (approximately 50 bp/year) from the age of 4 years to youth and old age (approximately 50 bp/year) (70–74). In children with obesity, the wear rate of telomeres is increased (12, 13, 15). Different scholars hold different views on whether obesity is related to TL. Some research showed that there was a clear correlation between childhood obesity and shorter telomeres (14, 75, 76). In contrast, others reported that there was no clear correlation between obesity and TL (16, 17). Liu et al. (18) reported that in preschool children with obesity, leukocyte TL shortening negatively correlated with the body mass index. They also reported that the TL of peripheral blood lymphocytes negatively correlated with arachidonic acid (AA)/ docosahexaenoic acid (DHA) ratio but positively correlated with saturated fatty acid and DHA levels, respectively. In any case, no affiliation was found between erythrocyte DHA levels and the methylation of the TERT promoter. One study likewise focused on the effect of the sex distinctions of children with obesity on TL. Other relevant studies also indicated that only the shortening of TL in males was found to be associated with the incidence of obesity; however, this association was not obvious in females (14).
3.2. Diabetes in children
Diabetes in children is an endocrine and metabolic illness caused by the lack of insulin emission. This mainly results in carbohydrate, protein, and fat metabolism disorders, giving rise to fasting, postprandial hyperglycemia, and urine sugar. Type 1 diabetes (T1D) is common in young people and often occurs during childhood. Its main disease feature is the absolute lack of insulin, and it is a chronic immune system disease (77, 78). Previous studies showed that the TL of children with T1D was significantly shorter than that of healthy children. In-depth follow-up studies reported that shorter telomeres were associated with all-cause mortality (19, 20). Tesovnik et al. (21) reported that the average TL of young people with T1D was adversely connected with the body mass index standard deviation score, and that serum vitamin D levels were not associated with TL in these individuals. In another study by Tesovnik et al. (22), they reported that a shorter telomere indicates an earlier and longer onset and duration of T1D; however, TL negatively correlated with glycated hemoglobin A1c. In the study of Törn et al. (79), after a comprehensive sequencing test of the gene level of thousands of children with T1D, results showed that the age of onset negatively correlated with TL, and that children with HLA-DR4/4 or HLA-DR4/X phenotypes had longer telomeres compared with children with HLA-DR3/3 or HLA-DR/9 genes.
4. Telomere dysfunction in hereditary diseases of children
4.1. Trisomy 21 syndrome
Trisomy 21 syndrome, also known as Down syndrome (DS), is caused by chromosomal abnormalities (an additional chromosome 21). Moreover, 60% of Trisomy 21 pregnancies were miscarried in the early fetal period. Children with DS have clear mental retardation, exceptional faces, developmental obstacles, and multiple malformations. A comparative study of three different Indian trisomy 21 data sets by Bhattacharya et al. (23) indicated that the TL of children with trisomy 21 syndrome decreased by 58 bps/year. In contrast, the TL of normal children decreased by 38 bps/year. The telomere attenuation rate of children with trisomy 21 was evidently higher. However, Nakamura et al. (80) tested the peripheral lymphocytes (diploid, 10; trisomy 18, 10; trisomy 21, 11) of 31 live newborns. They observed that there was no measurable difference in TL among trisomy 18, trisomy 21, and diploid infants. In contrast, some studies showed that normal children have longer TL than children with trisomy 21 (81, 82). Thus, the above views on the divergence between TL and trisomy 21 must be further studied.
4.2. Dyskeratosis congenita (DC)
Dyskeratosis congenita (DC), a multisystem inherited condition, is characterized by skin symptoms and can lead to bone marrow regeneration disorder or tumor. The inheritance of DC can be X-linked latent, autosomal predominant, or autosomal passive. Practically, patients with X-linked latent DC were male. Hoyeraal–Hreidarsson disease, Revesz syndrome, or Coats plus disease is usually the first symptom of DC (83–86). Some sporadic, non-classical cases are usually related to DC-related reproductive biological gene mutations, which mainly manifested as aplastic anemia, familial myelodysplastic syndromes/acute myeloid leukemia (AML) carriers, etc (87–90).. Mutations in the genes encoding TERT, telomerase RNA component (TERC), and dyskerin pseudo uridine synthase 1 (DKC1) genes are all related to the pathogenesis of DC (91–93). Mitchell et al. (24) reported that the level of TERC RNA in the DKC1-mutant cells of patients with DC was reduced. In the past years, there has been increasing evidence that gene mutations that maintain telomere gene length may increase the incidence of DC by 70%. At present, 13 known genes have this effect (25–28). They include the genes encoding telomerase-associated components [TERC, TERT, DKC1, nucleolar protein 10, H/ACA ribonucleoprotein complex subunit 2, telomerase Cajal body protein 1, nuclear assembly factor 1, and poly(A)-specific ribonuclease], shelterin proteins (TERF1-interacting nuclear factor 2, tripeptidyl-peptidase 1), and other regulators of TL and replication (regulator of telomere elongation helicase 1, CTC1, STN1).
4.3. Duchenne muscular dystrophy (DMD)
DMD is an X-chromosome recessive disease, which mainly occurs in males. Approximately one in each 3,500 newborn infants have this disease. Patients usually experience progressive degeneration of skeletal muscles, which gradually leads to muscle atrophy and eventually loss of motor function. These manifestations generally occur during the early-school age. It is mainly characterized by the gradual replacement of necrotic skeletal muscle cells by fibrocytes and adipocytes. Gradually, patients lose their exercise ability before and after school age, leading to death during adulthood when respiratory muscles are involved in serious cases. This disease is caused by the lack of proteins connecting the cytoskeleton and extracellular matrix, resulting in serious destruction of the integrity of the skeletal muscle cell membrane. The main genetic factors of this disease have been studied; however, its main pathogenesis must be further investigated (94). Sacco et al. (29) reported that the TL of dystrophin-deficient (mdx) mouse muscle cells lacking telomerase RNA components was shortened, leading to extremely severe malnutrition, which gradually worsened with age. The severity of muscle atrophy parallels the decline of the regeneration ability of muscle stem cells. Vita et al. (30) showed that in an mdx mouse model, the TL of the diaphragm was significantly shortened, and exercise factors could accelerate the telomere wear rate. Chang et al. (31) showed that telomere shortening is progressive, contraction-dependent, and mechanically sensitive in cardiomyocytes of DMD mice.
5. Telomere dysfunction in pediatric cancers
The incidence of cancer in children ranges from 0.05 to 3 per 10,000, accounting for 1% of all types of cancer (95). Childhood cancer is mostly related to congenital and genetic factors, but not strongly related to environmental factors and poor lifestyle, which is different from adult cancer. The role of telomere dysfunction in the occurrence and development of childhood cancer has gained more attention in recent years.
5.1. Neuroblastoma (NB)
NB is the most common extracranial tumor in children and may originate from early nerve cells of the sympathetic nerve system. Currently, only a few human tumors are known to spontaneously degenerate from undifferentiated malignant tumors to completely benign tumors, including NB. Lindner et al. (32) reported that the increased expression of TERT was detected in most NBs. Telomerase activation occurs in parallel with telomere shortening in NB (33, 96). Ackermann et al. (97) reported that repeated genome rearrangement in the proximal 5 p15.33 chromosome region may be the reason for the overexpression of TERT in NB cells. In the biological behavior of NBs, telomerase activation and the maintenance of TL are very important and necessary links that provide relatively accurate molecular mechanisms that determine the clinical phenotype of the tumor (33).
5.2. Leukemia
Leukemia is a malignant proliferative disease of the human hematopoietic system, which seriously threaten children's lives. Among the types of childhood leukemia, acute lymphoblastic leukemia (ALL) and AML are the two most common, accounting for approximately 3% of childhood malignant tumors (98, 99). A study by Engelhardt et al. (34) used data from the telomerase activity of peripheral blood monocytes. Compared with healthy children, the telomerase activity of children with ALL was 10–20 times higher. Similarly, Hu et al. (35) reported that the methylation of the cyclin dependent kinase inhibitor 2B gene was closely related to the telomerase activity in childhood cancer, and that telomerase activation is one of the important monitoring indicators for childhood cancer. It is interesting to note that, unlike previous perceptions, the mutation of the gene with reduced TERT activity is an important risk factor for the disease in children with AML (36). The telomerase activity of bone marrow cells positively correlated with prognosis in adult patients with AML, but negatively correlated with prognosis in children with AML (37).
5.3. Brain tumors
Intracranial tumors are the most common tumors during childhood, which makes it a serious threat to the life and health of children. Its overall incidence in the population ranks second (only next to the incidence of leukemia). People of all ages may have this disease; however, its peak of occurrence is during 5–8 years of age. In recent years, some studies have shown the close relationship between the maintenance mechanism of TL and occurrence of intracranial tumors in children (100–102). In pediatric glioma, the level of differentiation is related to telomerase activity. Some studies suggested that according to the standards of the World Health organization, telomerase activity was significantly reduced in low-grade gliomas in children (38, 39). In contrast, telomerase activity is significantly enhanced in most children with high-grade glioma (40, 41). Telomerase activity was increased in primary medulloblastoma but did not change in secondary medulloblastoma (101, 103).
6. Pediatric diseases in other body systems
Telomere dysfunction is rarely studied in the pediatric diseases of other body systems, such as the cardiovascular and urinary systems. Nevertheless, we included them in this review (Figure 2 and Table 1).
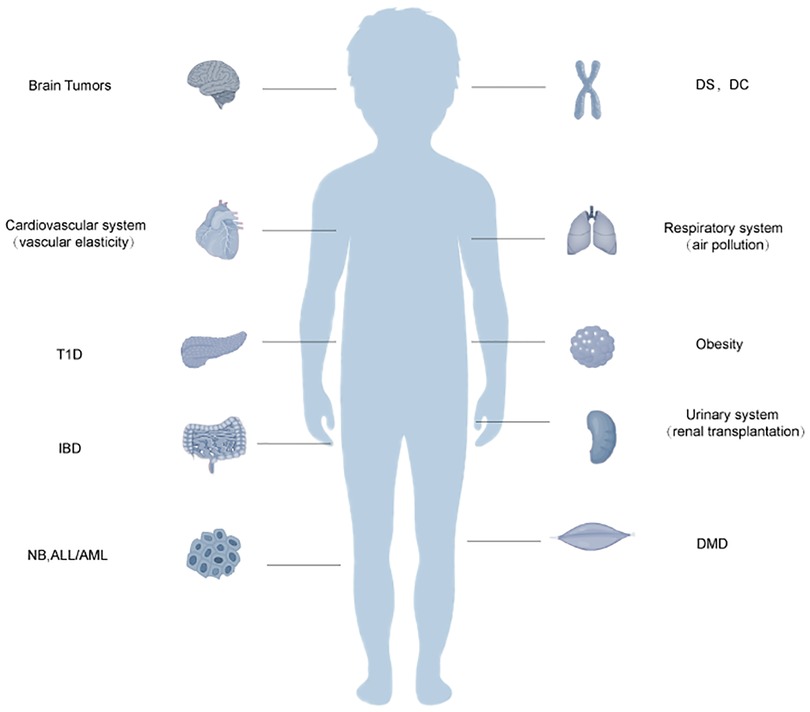
Figure 2. Schematic diagram of the relationship between telomere dysfunction and certain systemic disorders in older children. Acute lymphoblastic leukemia, ALL, acute myeloid leukemia; AML, dyskeratosis congenita; DC, Duchenne muscular dystrophy; DMD, Down syndrome; DS, inflammatory bowel disease; IBD, neuroblastoma; NB, type 1 diabetes, T1D. Telomere dysfunction may also be related to the body systems in children. It may be related to vascular elasticity in the cardiovascular system. In the respiratory system, it can enhance the sensitivity of the respiratory tract to air pollution. In the urinary system, it may be related to the success rate of renal transplantation.
Carotid intima-media thickness (IMT) is a risk factor for cardiovascular events in adult patients. Skilton et al. (42) confirmed that a shorter TL in youth was related to carotid IMT at 8 years of age but not to early life risk factors (birth weight, gestational age, skewed weight gain at the beginning, asthma, and atopy). In contrast, Nguyen et al. (43) reported that in middle-aged adults, TL was related with vascular versatility but not thickness. The influence of the telomere on respiratory diseases in children mainly focuses on its effect on asthma. Barbe-Tuana et al. (44) suggested that telomere shortening is positively related to severe therapy-resistant asthma in children, and that telomere attrition was associated with increased plasma eotaxin-1 expression in children. Lee et al. (45) confirmed that in children with asthma, exposure to air pollution is related to a shorter TL and that telomere wear may be reduced by steroid intervention. Similarly, Isaevska et al. (104) reported that for pregnant mothers, air pollution factors were related to shorter telomeres at birth, which can increase the long-term incidence of asthma and other diseases in children. In the study of Chakravarti et al. (46) found that telomere dysfunction leads to activation of ATM/YAP1 pathway, which is related to the pathogenesis and severity of inflammatory bowel disease (IBD).However, some scholars also believe that no evidence has been found that TL is associated with IBD in children (47, 48).
For patients undergoing renal transplantation, TL can be used as one of the indicators of long-term renal function; moreover, the incidence of renal transplantation or long-term complications is positively correlated with shorter telomeres (49). In contrast to adult patients, in children undergoing renal transplantation, plasma TRF2 correlated with the creatinine level and epidermal growth factor. Notably, although there is no significant difference in TL between children receiving renal transplantation and healthy children, the telomerase activity of children undergoing renal transplantation was significantly increased (50).
7. Prospect of treatment
It can be inferred that most telomere dysfunction-related diseases are caused by TL wear; hence, considering how to enhance telomerase activity and reduce telomere wear may potentially be the main targets of treatment. There are currently two main views. One is to promote the increase in the endogenous expression of TERT. As an endogenous promoter of TERT, androgen has been widely used in the treatment of aplastic anemia (105). As a specific PAPD5 inhibitor, BCH001 can restore telomerase activity in patients with DC and reduce the TL wear of pluripotent stem cells (106). In a mouse model, TA-65 has been shown to delay telomere wear by increasing telomerase activity (107). Recently, as a therapeutic method, TERT was overexpressed in an adeno-associated virus vector and transfected into animal models. Significant achievements have been made in the treatment of some adult aging-related diseases such as Alzheimer's disease, aplastic anemia, and idiopathic pulmonary fibrosis (96, 108–110). However, these kinds of treatment have not been investigated in terms of the risk of tumor occurrence and pediatric application.
Telomere overexpression is common in most patients with pediatric cancers; hence, inhibiting telomerase activity may be a potential therapeutic target. The 1,3,4-Oxadiazole scaffold is a five-member heterocyclic ring, which can be used as a telomerase inhibitor in tumor therapy (111, 112). As a telomerase inhibitor, imetelstat also plays a significant role in the treatment of adult AML (113). It can competitively inhibit the binding of telomerase and the human telomerase RNA component sequence, thereby inhibiting telomerase activity. For myelofibrosis, myelodysplastic syndrome, and other hematological malignancies, the application of imetelstat has also achieved gratifying results (114). As discussed above, telomerase expression in adults and children with AML is different; thus, this treatment is not applicable to children.
Antisense oligodeoxynucleotides (ASO) are a new class of drugs, which have gained more attention. It was confirmed in a mouse model that telomere ASO can reduce the DNA abrasion caused by telomere abrasion (115, 116).
Most of the above treatments for telomeres are based on the treatment of diseases related to adult telomere dysfunction (Table 2 and Figure 3). Research on the treatment of telomere dysfunction-associated pediatric diseases is lacking. Therefore, both the change in telomerase activity and maintenance of TL may be potential targets for pediatric diseases.
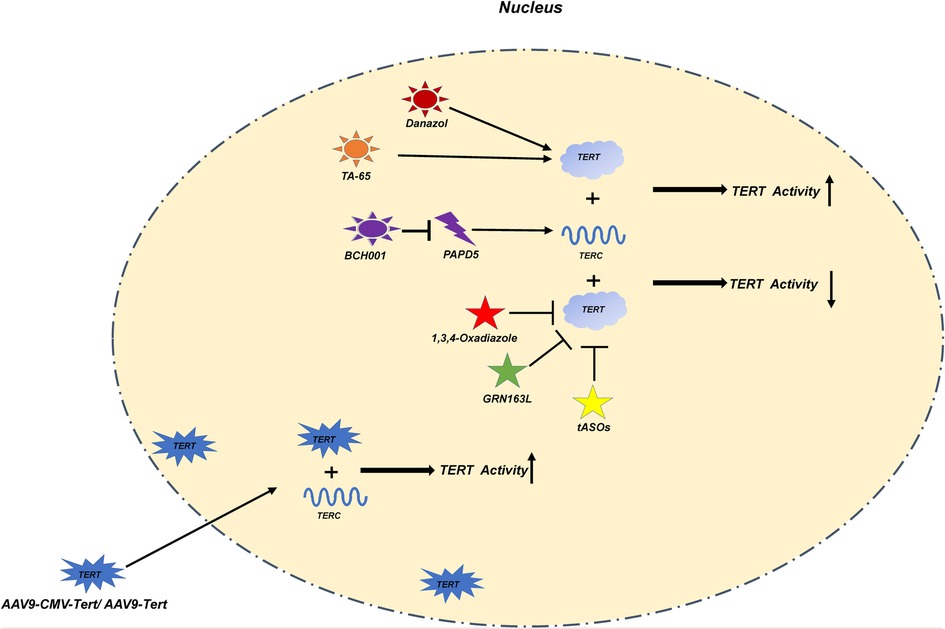
Figure 3. In this figure, we show various treatment methods and their mechanisms in detail. Danazol, TA-65 directly increase the activity of TERT; BCH001 reduces the consumption of TERC by inhibiting PAPD5 to improve the activity of TERT; The exogenous AAV9-CMV-Tert/AAV9-Tert enhances its activity by increasing the relative number of TERT in the nucleus; 1,3,4-Oxadiazole, GRN163L; ASOs inhibit the activity of TERT.
8. Conclusions
Telomere dysfunction is often associated with some adult cancers and aging related diseases. Since increasing evidence show that telomere dysfunction is related to the occurrence, development, and prognosis of some pediatric diseases, we systematically analyzed the relationship between telomere biology and some pediatric congenital and growth-related diseases in this article. Similar to that of adults, shorter TL is closely related to the pathological process of some children's diseases. To the preterm infants, shorter TL is mainly related to BPD and BA; For older children, studies on telomere dysfunction mainly focus on the following kinds of diseases: In endocrine system diseases, the influence of shorter TL on obese children of different genders is controversial. It is clear that the shorter TL is closely related to children's T1D in terms of disease occurrence and all-cause mortality of diseases; For Hereditary diseases, children with DS have longer telomeres, at the same time, they have higher telomere decay rate. For children with DC and DMD, telomere dysfunction is mainly related to the abnormality of telomere RNA structure TERC; Similar to the adult cancer, the overexpression and activation of TERT were also detected in most pediatric cancer patients such as NB, Brain tumor, et al. but there are exceptions, such as AML. There are also sporadic reports that telomere dysfunction is related to other body systems, such as cardiovascular system, respiratory system, digestive system and urinary system. Most of them are related to shorter TL. An interesting hypothesis is as follows. TERT acts as an RNA-dependent reverse transcriptase in the nucleus under stable conditions. When stress events occur, ROS production in mitochondria increases. At this time, TERT will translocated to mitochondria and combine with mitochondrial circular DNA (Mit DNA) to inhibit ROS production. The relative number of TERT in the nucleus decreases, resulting in telomere attrition. (Figure 4) Since telomere length is hereditary, it will be interesting to evaluate the correlation between the telomere length of newborns with diseases and the telomere length of their parents. This is of great significance for the long-term complications and treatment of newborns.
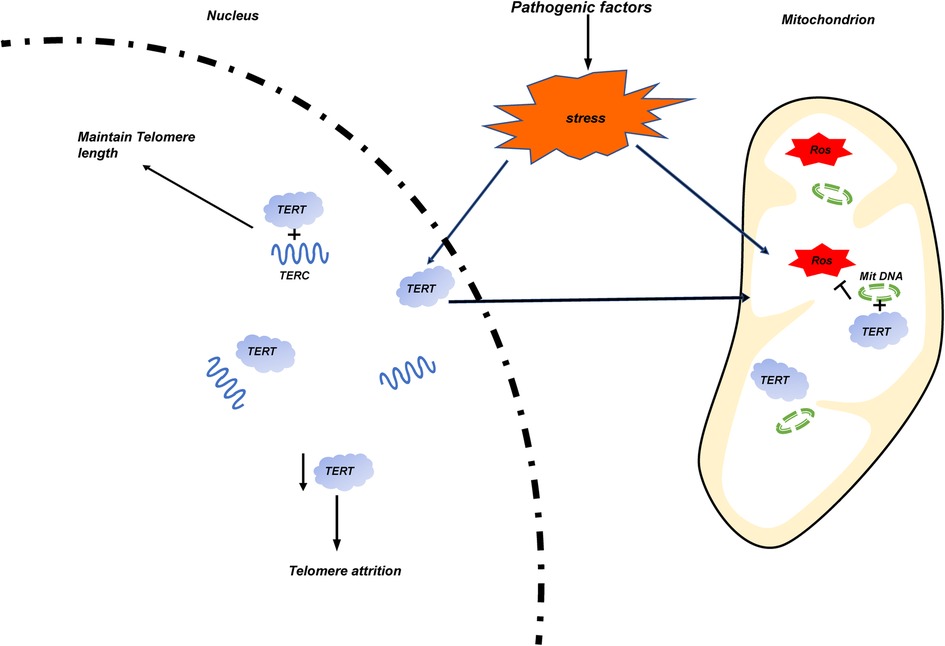
Figure 4. The occurrence of stress events is caused by pathogenic factors of various diseases. Mitochondrial damage increases the release of ROS, and TERT transfers from the nucleus to the mitochondria and binds with its circular DNA (Mit DNA) to inhibit the production of ROS. The reduction of the number of TERT in the nucleus leads to the wear of telomere length.
The length of telomeres at birth has a very important impact on the length of telomeres in children and adults. In the study of Martens DS et al. (117), the telomere length of newborns has a high predictive value to the telomere length of their later years. In the study of Martens DS et al. (118), it was found that the blood pressure of early school-age children was related to the length of telomere at birth. However, telomere length in childhood is also affected by other factors: Exposure to environmental pollution in early life is closely related to telomere wear (104, 119). Socioeconomic factor, and health and lifestyle factors also related to telomere wear (120). Sleep duration may also have a significant impact on TL in the first few years of life (121).
Most of the current treatments considering telomeres are based on the treatment of diseases related to adult telomere dysfunction. These therapeutic targets mainly focus on the intervention of TERT activity. Danazol, and BCH001 TA-65 used for endogenous TERT expression. AAV9-CMV-Tert/ AAV9-Tert used for exogenous import TERT. 1,3,4-Oxadiazole, GRN163L used for TERT inhibitor. Antisense strand RNA interference can also be used to inhibit TERT activity. The above treatment suggestions are based on the summary of the treatment of adult diseases and the validation of animal models. If they are applied to children's diseases, a large number of experiments are needed to verify their safety.
But telomerase activity and telomere length maintenance may be potential therapeutic targets for pediatric diseases.
Author contributions
JF conceived the review and revised the manuscript. BZ performed the search, collect the data, and took charge of writing the original manuscript. All authors contributed to the article and approved the submitted version.
Funding
This study was supported by grants from the National Natural Science Foundation of China (No. 82071688). Joint plan of Liaoning Provincial key R&D Guidance Plan Projects of Liaoning Province (2020JH1/10300001).
Acknowledgments
We wish to thank JF for guidance and support during completion of this review.
Conflict of interest
The authors declare that the research was conducted in the absence of any commercial or financial relationships that could be construed as a potential conflict of interest.
Publisher's note
All claims expressed in this article are solely those of the authors and do not necessarily represent those of their affiliated organizations, or those of the publisher, the editors and the reviewers. Any product that may be evaluated in this article, or claim that may be made by its manufacturer, is not guaranteed or endorsed by the publisher.
References
1. Price LH, Kao HT, Burgers DE, Carpenter LL, Tyrka AR. Telomeres and early-life stress: an overview. Biol Psychiatry. (2013) 73(1):15–23. doi: 10.1016/j.biopsych.2012.06.025
2. Lin A, Mertens AN, Arnold BF, Tan S, Lin J, Stewart CP, et al. Telomere length is associated with growth in children in rural Bangladesh. Elife. (2021) 10. doi: 10.7554/eLife.60389. [Epub ahead of print]
3. Elwood N. Telomere biology of human hematopoietic stem cells. Cancer Control. (2004) 11(2):77–85. doi: 10.1177/107327480401100214
4. Bosquet Enlow M, Kane-Grade F, De Vivo I, Petty CR, Nelson CA. Patterns of change in telomere length over the first three years of life in healthy children. Psychoneuroendocrinology. (2020) 115:104602. doi: 10.1016/j.psyneuen.2020.104602
5. Plentz RR, Caselitz M, Bleck JS, Gebel M, Flemming P, Kubicka S, et al. Hepatocellular telomere shortening correlates with chromosomal instability and the development of human hepatoma. Hepatology. (2004) 40(1):80–6. doi: 10.1002/hep.20271
6. Wiemann SU, Satyanarayana A, Tsahuridu M, Tillmann HL, Zender L, Klempnauer J, et al. Hepatocyte telomere shortening and senescence are general markers of human liver cirrhosis. FASEB J. (2002) 16(9):935–42. doi: 10.1096/fj.01-0977com
7. Sanada Y, Aida J, Kawano Y, Nakamura K, Shimomura N, Ishikawa N, et al. Hepatocellular telomere length in biliary atresia measured by Q-fish. World J Surg. (2012) 36(4):908–16. doi: 10.1007/s00268-012-1453-z
8. Hadchouel A, Marchand-Martin L, Franco-Montoya ML, Peaudecerf L, Ancel PY, Delacourt C, et al. Salivary telomere length and lung function in adolescents born very preterm: a prospective multicenter study. PLoS One. (2015) 10(9):e0136123. doi: 10.1371/journal.pone.0136123
9. Henckel E, James A, Konradsen JR, Nordlund B, Kjellberg M, Berggren-Broström E, et al. A novel association between ykl-40, a marker of structural lung disease, and short telomere length in 10-year-old children with bronchopulmonary dysplasia. Children (Basel). (2021) 8(2). doi: 10.3390/children8020080. [Epub ahead of print]33498968
10. Li J, Qu Y, Chen D, Zhang L, Zhao F, Luo L, et al. The neuroprotective role and mechanisms of tert in neurons with oxygen-glucose deprivation. Neuroscience. (2013) 252:346–58. doi: 10.1016/j.neuroscience.2013.08.015
11. Li J, Tang B, Qu Y, Mu D. Telomerase reverse transcriptase: a novel neuroprotective mechanism involved in neonatal hypoxic-ischemic brain injury. Int J Dev Neurosci. (2011) 29(8):867–72. doi: 10.1016/j.ijdevneu.2011.07.010
12. Benetos A, Kark JD, Susser E, Kimura M, Sinnreich R, Chen W, et al. Tracking and fixed ranking of leukocyte telomere length across the adult life course. Aging Cell. (2013) 12(4):615–21. doi: 10.1111/acel.12086
13. Aviv A, Chen W, Gardner JP, Kimura M, Brimacombe M, Cao X, et al. Leukocyte telomere dynamics: longitudinal findings among young adults in the bogalusa heart study. Am J Epidemiol. (2009) 169(3):323–9. doi: 10.1093/aje/kwn338
14. Al-Attas OS, Al-Daghri N, Bamakhramah A, Shaun Sabico S, McTernan P, Huang TT. Telomere length in relation to insulin resistance, inflammation and obesity among arab youth. Acta Paediatr. (2010) 99(6):896–9. doi: 10.1111/j.1651-2227.2010.01720.x
15. Nordfjäll K, Eliasson M, Stegmayr B, Melander O, Nilsson P, Roos G. Telomere length is associated with obesity parameters but with a gender difference. Obesity (Silver Spring). (2008) 16(12):2682–9. doi: 10.1038/oby.2008.413
16. Kjaer TW, Faurholt-Jepsen D, Mehta KM, Christensen VB, Epel E, Lin J, et al. Shorter preschool, leukocyte telomere length is associated with obesity at age 9 in latino children. Clin Obes. (2018) 8(2):88–94. doi: 10.1111/cob.12233
17. Ooi DSQ, Dorajoo R, Gurung RL, Dehghan R, Lim YY, Ho CWL, et al. Association of leukocyte telomere length with obesity-related traits in Asian children with early-onset obesity. Pediatr Obes. (2021) 16(8):e12771. doi: 10.1111/ijpo.12771
18. Liu X, Shi Q, Fan X, Qi K. Association of telomere length and telomerase methylation with N-3 fatty acids in preschool children with obesity. BMC Pediatr. (2021) 21(1):24. doi: 10.1186/s12887-020-02487-x
19. Jeanclos E, Krolewski A, Skurnick J, Kimura M, Aviv H, Warram JH, et al. Shortened telomere length in white blood cells of patients with iddm. Diabetes. (1998) 47(3):482–6. doi: 10.2337/diabetes.47.3.482
20. Astrup AS, Tarnow L, Jorsal A, Lajer M, Nzietchueng R, Benetos A, et al. Telomere length predicts all-cause mortality in patients with type 1 diabetes. Diabetologia. (2010) 53(1):45–8. doi: 10.1007/s00125-009-1542-1
21. Tesovnik T, Kovac J, Hovnik T, Kotnik P, Battelino T, Trebusak Podkrajsek K. Association of average telomere length with body-mass index and vitamin D Status in juvenile population with type 1 diabetes. Zdr Varst. (2015) 54(2):74–8. doi: 10.1515/sjph-2015-0011
22. Tesovnik T, Kovac J, Hovnik T, Dovc K, Bratina N, Battelino T, et al. Association of glycemic control and cell stress with telomere attrition in type 1 diabetes. JAMA Pediatr. (2018) 172(9):879–81. doi: 10.1001/jamapediatrics.2018.1175
23. Bhattacharya M, Bhaumik P, Ghosh P, Majumder P, Kumar Dey S. Telomere length inheritance and shortening in trisomy 21. Fetal Pediatr Pathol. (2020) 39(5):390–400. doi: 10.1080/15513815.2019.1661049
24. Mitchell JR, Wood E, Collins K. A telomerase component is defective in the human disease dyskeratosis congenita. Nature. (1999) 402(6761):551–5. doi: 10.1038/990141
25. Stanley SE, Gable DL, Wagner CL, Carlile TM, Hanumanthu VS, Podlevsky JD, et al. Loss-of-function mutations in the rna biogenesis factor Naf1 predispose to pulmonary fibrosis-emphysema. Sci Transl Med. (2016) 8(351):351ra107. doi: 10.1126/scitranslmed.aaf7837
26. Townsley DM, Dumitriu B, Young NS. Bone marrow failure and the telomeropathies. Blood. (2014) 124(18):2775–83. doi: 10.1182/blood-2014-05-526285
27. Bertuch AA. The molecular genetics of the telomere biology disorders. RNA Biol. (2016) 13(8):696–706. doi: 10.1080/15476286.2015.1094596
28. Simon AJ, Lev A, Zhang Y, Weiss B, Rylova A, Eyal E, et al. Mutations in Stn1 cause coats plus syndrome and are associated with genomic and telomere defects. J Exp Med. (2016) 213(8):1429–40. doi: 10.1084/jem.20151618
29. Sacco A, Mourkioti F, Tran R, Choi J, Llewellyn M, Kraft P, et al. Short telomeres and stem cell exhaustion model duchenne muscular dystrophy in mdx/mtr mice. Cell. (2010) 143(7):1059–71. doi: 10.1016/j.cell.2010.11.039
30. Vita GL, Aguennouz M, Sframeli M, Sanarica F, Mantuano P, Oteri R, et al. Effect of exercise on telomere length and telomere proteins expression in mdx mice. Mol Cell Biochem. (2020) 470(1–2):189–97. doi: 10.1007/s11010-020-03761-3
31. Chang ACY, Pardon G, Chang ACH, Wu H, Ong SG, Eguchi A, et al. Increased tissue stiffness triggers Contractile dysfunction and telomere shortening in dystrophic cardiomyocytes. Stem Cell Rep. (2021) 16(9):2169–81. doi: 10.1016/j.stemcr.2021.04.018
32. Lindner S, Bachmann HS, Odersky A, Schaefers S, Klein-Hitpass L, Hero B, et al. Absence of telomerase reverse transcriptase promoter mutations in neuroblastoma. Biomed Rep. (2015) 3(4):443–6. doi: 10.3892/br.2015.463
33. Peifer M, Hertwig F, Roels F, Dreidax D, Gartlgruber M, Menon R, et al. Telomerase activation by genomic rearrangements in high-risk neuroblastoma. Nature. (2015) 526(7575):700–4. doi: 10.1038/nature14980
34. Engelhardt M, Ozkaynak MF, Drullinsky P, Sandoval C, Tugal O, Jayabose S, et al. Telomerase activity and telomere length in pediatric patients with malignancies undergoing chemotherapy. Leukemia. (1998) 12(1):13–24. doi: 10.1038/sj.leu.2400889
35. Hu Q, Chen X, Liu S, Wen R, Yuan X, Xu D, et al. Methylation of Cdkn2b Cpg islands is associated with upregulated telomerase activity in children with acute lymphoblastic leukemia. Oncol Lett. (2017) 13(4):2115–20. doi: 10.3892/ol.2017.5710
36. Calado RT, Regal JA, Hills M, Yewdell WT, Dalmazzo LF, Zago MA, et al. Constitutional hypomorphic telomerase mutations in patients with acute myeloid leukemia. Proc Natl Acad Sci USA. (2009) 106(4):1187–92. doi: 10.1073/pnas.0807057106
37. Verstovsek S, Manshouri T, Smith FO, Giles FJ, Cortes J, Estey E, et al. Telomerase activity is prognostic in pediatric patients with acute myeloid leukemia: comparison with adult acute myeloid leukemia. Cancer. (2003) 97(9):2212–7. doi: 10.1002/cncr.11313
38. Tabori U, Vukovic B, Zielenska M, Hawkins C, Braude I, Rutka J, et al. The role of telomere maintenance in the spontaneous growth arrest of pediatric low-grade gliomas. Neoplasia. (2006) 8(2):136–42. doi: 10.1593/neo.05715
39. Schmandt SM, Packer RJ, Vezina LG, Jane J. Spontaneous regression of low-grade astrocytomas in childhood. Pediatr Neurosurg. (2000) 32(3):132–6. doi: 10.1159/000028917
40. Koelsche C, Sahm F, Capper D, Reuss D, Sturm D, Jones DT, et al. Distribution of tert promoter mutations in pediatric and adult tumors of the nervous system. Acta Neuropathol. (2013) 126(6):907–15. doi: 10.1007/s00401-013-1195-5
41. Sturm D, Bender S, Jones DT, Lichter P, Grill J, Becher O, et al. Paediatric and adult glioblastoma: multiform (epi)Genomic culprits emerge. Nat Rev Cancer. (2014) 14(2):92–107. doi: 10.1038/nrc3655
42. Skilton MR, Nakhla S, Ayer JG, Harmer JA, Toelle BG, Leeder SR, et al. Telomere length in early childhood: early life risk factors and association with carotid intima-media thickness in later childhood. Eur J Prev Cardiol. (2016) 23(10):1086–92. doi: 10.1177/2047487315607075
43. Nguyen MT, Vryer R, Ranganathan S, Lycett K, Grobler A, Dwyer T, et al. Telomere length and vascular phenotypes in a population-based cohort of children and midlife adults. J Am Heart Assoc. (2019) 8(11):e012707. doi: 10.1161/JAHA.119.012707
44. Barbe-Tuana FM, Grun LK, Pierdona V, Parisi MM, Friedrich F, Guma F, et al. Shorter telomeres in children with severe asthma, an indicative of accelerated aging. Aging (Albany NY). (2021) 13(2):1686–91. doi: 10.18632/aging.202527
45. Lee EY, Oh SS, White MJ, Eng CS, Elhawary JR, Borrell LN, et al. Ambient air pollution, asthma drug response, and telomere length in African American youth. J Allergy Clin Immunol. (2019) 144(3):839–45 e10. doi: 10.1016/j.jaci.2019.06.009
46. Chakravarti D, Hu B, Mao X, Rashid A, Li J, Li J, et al. Telomere dysfunction activates Yap1 to drive tissue inflammation. Nat Commun. (2020) 11(1):4766. doi: 10.1038/s41467-020-18420-w
47. Truta B, Wohler E, Sobreira N, Datta LW, Brant SR. Role of telomere shortening in anticipation of inflammatory bowel disease. World J Gastrointest Pharmacol Ther. (2020) 11(4):69–78. doi: 10.4292/wjgpt.v11.i4.69
48. Salk JJ, Bansal A, Lai LA, Crispin DA, Ussakli CH, Horwitz MS, et al. Clonal expansions and short telomeres are associated with neoplasia in early-onset, but not late-onset, ulcerative colitis. Inflamm Bowel Dis. (2013) 19(12):2593–602. doi: 10.1097/MIB.0b013e3182a87640
49. Domanski L, Kloda K, Kwiatkowska E, Borowiecka E, Safranow K, Drozd A, et al. Effect of delayed graft function, acute rejection and chronic allograft dysfunction on kidney allograft telomere length in patients after transplantation: a prospective cohort study. BMC Nephrol. (2015) 16:23. doi: 10.1186/s12882-015-0014-8
50. Enden K, Tainio J, Hou M, Suominen A, Pakarinen M, Huang T, et al. Telomere length regulators are activated in young men after pediatric kidney transplantation compared to healthy controls and survivors of childhood cancer-a cross-sectional study. Pediatr Transplant. (2019) 23(7):e13550. doi: 10.1111/petr.13550
51. Salihu HM, Pradhan A, King L, Paothong A, Nwoga C, Marty PJ, et al. Impact of intrauterine tobacco exposure on fetal telomere length. Am J Obstet Gynecol. (2015) 212(2):205.e1–e8.doi: 10.1016/j.ajog.2014.08.026
52. Entringer S, Epel ES, Lin J, Blackburn EH, Buss C, Shahbaba B, et al. Maternal folate concentration in early pregnancy and newborn telomere length. Ann Nutr Metab. (2015) 66(4):202–8. doi: 10.1159/000381925
53. Marchetto NM, Glynn RA, Ferry ML, Ostojic M, Wolff SM, Yao R, et al. Prenatal stress and newborn telomere length. Am J Obstet Gynecol. (2016) 215(1):94.e1–e8. doi: 10.1016/j.ajog.2016.01.177
54. Menon R, Yu J, Basanta-Henry P, Brou L, Berga SL, Fortunato SJ, et al. Short fetal leukocyte telomere length and preterm prelabor rupture of the membranes. PLoS One. (2012) 7(2):e31136. doi: 10.1371/journal.pone.0031136
55. Friedrich U, Schwab M, Griese EU, Fritz P, Klotz U. Telomeres in neonates: new insights in fetal hematopoiesis. Pediatr Res. (2001) 49(2):252–6. doi: 10.1203/00006450-200102000-00020
56. Casavant SG, Li H, Reese B, Chen MH, Cong XS. Pilot study of absolute telomere lengths in preterm infants. Nurs Res. (2021) 70(6):481–6. doi: 10.1097/NNR.0000000000000535
57. Kananen L, Surakka I, Pirkola S, Suvisaari J, Lönnqvist J, Peltonen L, et al. Childhood adversities are associated with shorter telomere length at adult age both in individuals with an anxiety disorder and controls. PLoS One. (2010) 5(5):e10826. doi: 10.1371/journal.pone.0010826
58. O’Donovan A, Pantell MS, Puterman E, Dhabhar FS, Blackburn EH, Yaffe K, et al. Cumulative inflammatory load is associated with short leukocyte telomere length in the health, aging and body composition study. PLoS One. (2011) 6(5):e19687. doi: 10.1371/journal.pone.0019687
59. Provenzi L, Giorda R, Fumagalli M, Pozzoli U, Morandi F, Scotto di Minico G, et al. Pain exposure associates with telomere length erosion in very preterm infants. Psychoneuroendocrinology. (2018) 89:113–9. doi: 10.1016/j.psyneuen.2018.01.009
60. Udomsinprasert W, Poovorawan Y, Chongsrisawat V, Vejchapipat P, Zhan D, Honsawek S. Telomere length in peripheral blood leukocytes is associated with severity of biliary atresia. PLoS One. (2015) 10(7):e0134689. doi: 10.1371/journal.pone.0134689
61. Hartley JL, Davenport M, Kelly DA. Biliary atresia. Lancet. (2009) 374(9702):1704–13. doi: 10.1016/S0140-6736(09)60946-6
62. Nordlund B, James A, Ebersjö C, Hedlin G, Broström EB. Differences and similarities between bronchopulmonary dysplasia and asthma in schoolchildren. Pediatr Pulmonol. (2017) 52(9):1179–86. doi: 10.1002/ppul.23741
63. Kurinczuk JJ, White-Koning M, Badawi N. Epidemiology of neonatal encephalopathy and hypoxic-ischaemic encephalopathy. Early Hum Dev. (2010) 86(6):329–38. doi: 10.1016/j.earlhumdev.2010.05.010
64. Magai DN, Karyotaki E, Mutua AM, Chongwo E, Nasambu C, Ssewanyana D, et al. Long-term outcomes of survivors of neonatal insults: a systematic review and meta-analysis. PLoS One. (2020) 15(4):e0231947. doi: 10.1371/journal.pone.0231947
65. Wyatt HD, West SC, Beattie TL. Intertpreting telomerase structure and function. Nucleic Acids Res. (2010) 38(17):5609–22. doi: 10.1093/nar/gkq370
66. Li J, Liu HT, Zhao J, Chen HJ. Telomerase reverse transcriptase (tert) promotes neurogenesis after hypoxic-ischemic brain damage in neonatal rats. Neurol Res. (2022) 44(9):819–29. doi: 10.1080/01616412.2022.2056339
67. Kimura M, Gazitt Y, Cao X, Zhao X, Lansdorp PM, Aviv A. Synchrony of telomere length among hematopoietic cells. Exp Hematol. (2010) 38(10):854–9. doi: 10.1016/j.exphem.2010.06.010
68. Youngren K, Jeanclos E, Aviv H, Kimura M, Stock J, Hanna M, et al. Synchrony in telomere length of the human Fetus. Hum Genet. (1998) 102(6):640–3. doi: 10.1007/s004390050755
69. Wright DL, Jones EL, Mayer JF, Oehninger S, Gibbons WE, Lanzendorf SE. Characterization of telomerase activity in the human oocyte and preimplantation embryo. Mol Hum Reprod. (2001) 7(10):947–55. doi: 10.1093/molehr/7.10.947
70. Frenck RW, Blackburn EH, Shannon KM. The rate of telomere sequence loss in human leukocytes varies with age. Proc Natl Acad Sci USA. (1998) 95(10):5607–10. doi: 10.1073/pnas.95.10.5607
71. Zeichner SL, Palumbo P, Feng Y, Xiao X, Gee D, Sleasman J, et al. Rapid telomere shortening in children. Blood. (1999) 93(9):2824–30. doi: 10.1182/blood.V93.9.2824
72. Rufer N, Brümmendorf TH, Kolvraa S, Bischoff C, Christensen K, Wadsworth L, et al. Telomere fluorescence measurements in granulocytes and T lymphocyte subsets point to a high turnover of hematopoietic stem cells and memory T cells in early childhood. J Exp Med. (1999) 190(2):157–67. doi: 10.1084/jem.190.2.157
73. Takubo K, Nakamura K, Izumiyama N, Furugori E, Sawabe M, Arai T, et al. Telomere shortening with aging in human liver. J Gerontol A Biol Sci Med Sci. (2000) 55(11):B533–6. doi: 10.1093/gerona/55.11.b533
74. Lansdorp PM. Telomeres, stem cells, and hematology. Blood. (2008) 111(4):1759–66. doi: 10.1182/blood-2007-09-084913
75. Buxton JL, Walters RG, Visvikis-Siest S, Meyre D, Froguel P, Blakemore AI. Childhood obesity is associated with shorter leukocyte telomere length. J Clin Endocrinol Metab. (2011) 96(5):1500–5. doi: 10.1210/jc.2010-2924
76. Clemente DBP, Maitre L, Bustamante M, Chatzi L, Roumeliotaki T, Fossati S, et al. Obesity is associated with shorter telomeres in 8 year-old children. Sci Rep. (2019) 9(1):18739. doi: 10.1038/s41598-019-55283-8
77. Chakhtoura M, Azar ST. The role of vitamin D deficiency in the incidence, progression, and complications of type 1 diabetes Mellitus. Int J Endocrinol. (2013) 2013:148673. doi: 10.1155/2013/148673
78. Association AD. Diagnosis and classification of diabetes mellitus. Diabetes Care. (2012) 35(Suppl 1):S64–S71. doi: 10.2337/dc12-s064
79. Törn C, Liu X, Onengut-Gumuscu S, Counts KM, Moreno JL, Remedios CL, et al. Telomere length is not a main factor for the development of islet autoimmunity and type 1 diabetes in the teddy study. Sci Rep. (2022) 12(1):4516. doi: 10.1038/s41598-022-08058-7
80. Nakamura K, Ishikawa N, Izumiyama N, Aida J, Kuroiwa M, Hiraishi N, et al. Telomere lengths at birth in trisomies 18 and 21 measured by Q-fish. Gene. (2014) 533(1):199–207. doi: 10.1016/j.gene.2013.09.086
81. Sukenik-Halevy R, Biron-Shental T, Sharony R, Fejgin MD, Amiel A. Telomeres in trisomy 21 amniocytes. Cytogenet Genome Res. (2011) 135(1):12–8. doi: 10.1159/000329714
82. Wenger SL, Hansroth J, Shackelford AL. Decreased telomere length in metaphase and interphase cells from newborns with trisomy 21. Gene. (2014) 542(1):87. doi: 10.1016/j.gene.2014.03.019
83. Keller RB, Gagne KE, Usmani GN, Asdourian GK, Williams DA, Hofmann I, et al. Ctc1 mutations in a patient with dyskeratosis congenita. Pediatr Blood Cancer. (2012) 59(2):311–4. doi: 10.1002/pbc.24193
84. Knight SW, Heiss NS, Vulliamy TJ, Aalfs CM, McMahon C, Richmond P, et al. Unexplained aplastic anaemia, immunodeficiency, and cerebellar hypoplasia (hoyeraal-hreidarsson syndrome) due to mutations in the dyskeratosis congenita gene, Dkc1. Br J Haematol. (1999) 107(2):335–9. doi: 10.1046/j.1365-2141.1999.01690.x
85. Glousker G, Touzot F, Revy P, Tzfati Y, Savage SA. Unraveling the pathogenesis of hoyeraal-hreidarsson syndrome, a complex telomere biology disorder. Br J Haematol. (2015) 170(4):457–71. doi: 10.1111/bjh.13442
86. Savage SA, Giri N, Baerlocher GM, Orr N, Lansdorp PM, Alter BP. Tinf2, a component of the shelterin telomere protection complex, is mutated in dyskeratosis congenita. Am J Hum Genet. (2008) 82(2):501–9. doi: 10.1016/j.ajhg.2007.10.004
87. Du HY, Pumbo E, Ivanovich J, An P, Maziarz RT, Reiss UM, et al. Terc and tert gene mutations in patients with bone marrow failure and the significance of telomere length measurements. Blood. (2009) 113(2):309–16. doi: 10.1182/blood-2008-07-166421
88. Kirwan M, Vulliamy T, Marrone A, Walne AJ, Beswick R, Hillmen P, et al. Defining the pathogenic role of telomerase mutations in myelodysplastic syndrome and acute myeloid leukemia. Hum Mutat. (2009) 30(11):1567–73. doi: 10.1002/humu.21115
89. Yamaguchi H, Baerlocher GM, Lansdorp PM, Chanock SJ, Nunez O, Sloand E, et al. Mutations of the human telomerase rna gene (Terc) in aplastic anemia and myelodysplastic syndrome. Blood. (2003) 102(3):916–8. doi: 10.1182/blood-2003-01-0335
90. Yamaguchi H, Calado RT, Ly H, Kajigaya S, Baerlocher GM, Chanock SJ, et al. Mutations in tert, the gene for telomerase reverse transcriptase, in aplastic Anemia. N Engl J Med. (2005) 352(14):1413–24. doi: 10.1056/NEJMoa042980
91. Heiss NS, Knight SW, Vulliamy TJ, Klauck SM, Wiemann S, Mason PJ, et al. X-linked dyskeratosis congenita is caused by mutations in a highly conserved gene with putative nucleolar functions. Nat Genet. (1998) 19(1):32–8. doi: 10.1038/ng0598-32
92. Knight SW, Vulliamy TJ, Heiss NS, Matthijs G, Devriendt K, Connor JM, et al. 1.4 Mb candidate gene region for X linked dyskeratosis congenita defined by combined haplotype and X chromosome inactivation analysis. J Med Genet. (1998) 35(12):993–6. doi: 10.1136/jmg.35.12.993
93. Mitchell JR, Cheng J, Collins K. A box H/aca small nucleolar rna-like domain at the human telomerase Rna 3′ end. Mol Cell Biol. (1999) 19(1):567–76. doi: 10.1128/MCB.19.1.567
94. Dubowitz V. Commentary from the editor. Neuromuscul Disord. (2007) 17(1):1–5. doi: 10.1016/j.nmd.2006.12.005
95. Steliarova-Foucher E, Colombet M, Ries LAG, Moreno F, Dolya A, Bray F, et al. International incidence of childhood cancer, 2001–10: a population-based registry study. Lancet Oncol. (2017) 18(6):719–31. doi: 10.1016/S1470-2045(17)30186-9
96. Martínez P, Blasco MA. Telomeric and extra-telomeric roles for telomerase and the telomere-binding proteins. Nat Rev Cancer. (2011) 11(3):161–76. doi: 10.1038/nrc3025
97. Ackermann S, Cartolano M, Hero B, Welte A, Kahlert Y, Roderwieser A, et al. A mechanistic classification of clinical phenotypes in neuroblastoma. Science. (2018) 362(6419):1165–70. doi: 10.1126/science.aat6768
98. Ferlay J, Shin HR, Bray F, Forman D, Mathers C, Parkin DM. Estimates of worldwide burden of cancer in 2008: globocan 2008. Int J Cancer. (2010) 127(12):2893–917. doi: 10.1002/ijc.25516
99. Belson M, Kingsley B, Holmes A. Risk factors for acute leukemia in children: a review. Environ Health Perspect. (2007) 115(1):138–45. doi: 10.1289/ehp.9023
100. Idilli AI, Cusanelli E, Pagani F, Berardinelli F, Bernabé M, Cayuela ML, et al. Expression of. Front Cell Dev Biol. (2020) 8:65. doi: 10.3389/fcell.2020.00065
101. Minasi S, Baldi C, Pietsch T, Donofrio V, Pollo B, Antonelli M, et al. Telomere elongation via alternative lengthening of telomeres (Alt) and telomerase activation in primary metastatic medulloblastoma of childhood. J Neurooncol. (2019) 142(3):435–44. doi: 10.1007/s11060-019-03127-w
102. Marinoff AE, Ma C, Guo D, Snuderl M, Wright KD, Manley PE, et al. Rethinking childhood ependymoma: a retrospective, multi-center analysis reveals poor long-term overall survival. J Neurooncol. (2017) 135(1):201–11. doi: 10.1007/s11060-017-2568-8
103. Mangerel J, Price A, Castelo-Branco P, Brzezinski J, Buczkowicz P, Rakopoulos P, et al. Alternative lengthening of telomeres is enriched in, and impacts survival of Tp53 mutant pediatric malignant brain tumors. Acta Neuropathol. (2014) 128(6):853–62. doi: 10.1007/s00401-014-1348-1
104. Isaevska E, Moccia C, Asta F, Cibella F, Gagliardi L, Ronfani L, et al. Exposure to ambient air pollution in the first 1000 days of life and alterations in the DNA methylome and telomere length in children: a systematic review. Environ Res. (2021) 193:110504. doi: 10.1016/j.envres.2020.110504
105. Townsley DM, Dumitriu B, Liu D, Biancotto A, Weinstein B, Chen C, et al. Danazol treatment for telomere diseases. N Engl J Med. (2016) 374(20):1922–31. doi: 10.1056/NEJMoa1515319
106. Nagpal N, Wang J, Zeng J, Lo E, Moon DH, Luk K, et al. Small-molecule Papd5 inhibitors restore telomerase activity in patient stem cells. Cell Stem Cell. (2020) 26(6):896–909 e8. doi: 10.1016/j.stem.2020.03.016
107. Bernardes de Jesus B, Schneeberger K, Vera E, Tejera A, Harley CB, Blasco MA. The telomerase activator ta-65 elongates short telomeres and increases health span of adult/old mice without increasing cancer incidence. Aging Cell. (2011) 10(4):604–21. doi: 10.1111/j.1474-9726.2011.00700.x
108. Whittemore K, Derevyanko A, Martinez P, Serrano R, Pumarola M, Bosch F, et al. Telomerase gene therapy ameliorates the effects of neurodegeneration associated to short telomeres in mice. Aging (Albany NY). (2019) 11(10):2916–48. doi: 10.18632/aging.101982
109. Martinez P, Blasco MA. Telomere-driven diseases and telomere-targeting therapies. J Cell Biol. (2017) 216(4):875–87. doi: 10.1083/jcb.201610111
110. Bernardes de Jesus B, Vera E, Schneeberger K, Tejera AM, Ayuso E, Bosch F, et al. Telomerase gene therapy in adult and old mice delays aging and increases longevity without increasing cancer. EMBO Mol Med. (2012) 4(8):691–704. doi: 10.1002/emmm.201200245
111. Bajaj S, Roy PP, Singh J. 1,3,4-oxadiazoles as telomerase inhibitor: potential anticancer agents. Anticancer Agents Med Chem. (2018) 17(14):1869–83. doi: 10.2174/1871521409666170425092906
112. Tutone M, Pecoraro B, Almerico AM. Investigation on quantitative structure-activity relationships of 1,3,4-oxadiazole derivatives as potential telomerase inhibitors. Curr Drug Discov Technol. (2020) 17(1):79–86. doi: 10.2174/1570163815666180724113208
113. Saygin C, Carraway HE. Current and emerging strategies for management of myelodysplastic syndromes. Blood Rev. (2021) 48:100791. doi: 10.1016/j.blre.2020.100791
114. Carloni LE, Wechselberger R, De Vijlder T. Characterization of in vitro G-quadruplex formation of imetelstat telomerase inhibitor. Nucleic Acid Ther. (2021) 31(5):341–50. doi: 10.1089/nat.2020.0918
115. Rossiello F, Aguado J, Sepe S, Iannelli F, Nguyen Q, Pitchiaya S, et al. DNA damage response inhibition at dysfunctional telomeres by modulation of telomeric DNA damage response rnas. Nat Commun. (2017) 8:13980. doi: 10.1038/ncomms13980
116. Aguado J, Sola-Carvajal A, Cancila V, Revechon G, Ong PF, Jones-Weinert CW, et al. Inhibition of DNA damage response at telomeres improves the detrimental phenotypes of Hutchinson-Gilford progeria syndrome. Nat Commun. (2019) 10(1):4990. doi: 10.1038/s41467-019-13018-3
117. Martens DS, Van Der Stukken C, Derom C, Thiery E, Bijnens EM, Nawrot TS. Newborn telomere length predicts later life telomere length: tracking telomere length from birth to child- and adulthood. EBioMedicine. (2021) 63:103164. doi: 10.1016/j.ebiom.2020.103164
118. Martens DS, Sleurs H, Dockx Y, Rasking L, Plusquin M, Nawrot TS. Association of newborn telomere length with blood pressure in childhood. JAMA Netw Open. (2022) 5(8):e2225521. doi: 10.1001/jamanetworkopen.2022.25521
119. Vriens A, Nawrot TS, Janssen BG, Baeyens W, Bruckers L, Covaci A, et al. Exposure to environmental pollutants and their association with biomarkers of aging: a multipollutant approach. Environ Sci Technol. (2019) 53(10):5966–76. doi: 10.1021/acs.est.8b07141
120. Woo JMP, Parks CG, Hyde EE, Auer PL, Simanek AM, Konkel RH, et al. Early life trauma and adult leucocyte telomere length. Psychoneuroendocrinology. (2022) 144:105876. doi: 10.1016/j.psyneuen.2022.105876
Keywords: telomere, telomerase (TERT), pediatric diseases, infants, aging
Citation: Zheng B and Fu J (2023) Telomere dysfunction in some pediatric congenital and growth-related diseases. Front. Pediatr. 11:1133102. doi: 10.3389/fped.2023.1133102
Received: 28 December 2022; Accepted: 20 March 2023;
Published: 3 April 2023.
Edited by:
Tim S. Nawrot, University of Hasselt, BelgiumReviewed by:
Hala El-Bassyouni, National Research Centre, EgyptDeepavali Chakravarti, University of Texas MD Anderson Cancer Center, United States
© 2023 Zheng and Fu. This is an open-access article distributed under the terms of the Creative Commons Attribution License (CC BY). The use, distribution or reproduction in other forums is permitted, provided the original author(s) and the copyright owner(s) are credited and that the original publication in this journal is cited, in accordance with accepted academic practice. No use, distribution or reproduction is permitted which does not comply with these terms.
*Correspondence: Jianhua Fu ZnVqaF9zakAxMjYuY29t
Specialty Section: This article was submitted to Children and Health, a section of the journal Frontiers in Pediatrics