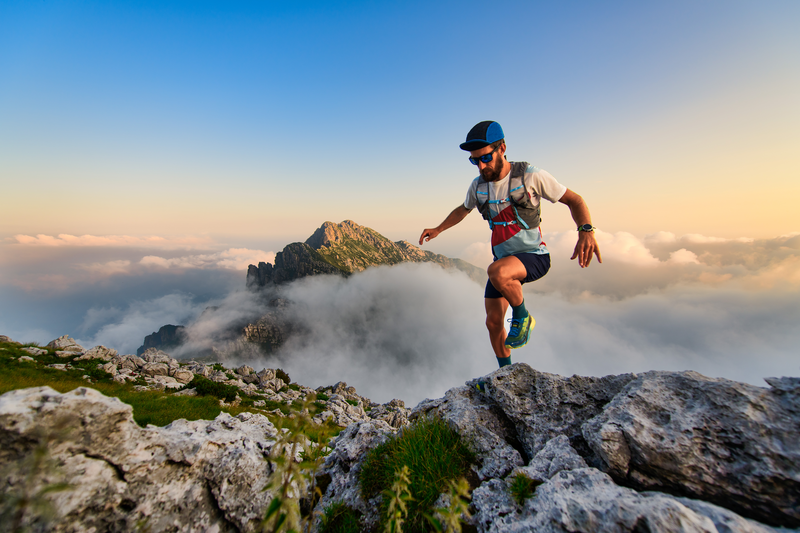
95% of researchers rate our articles as excellent or good
Learn more about the work of our research integrity team to safeguard the quality of each article we publish.
Find out more
REVIEW article
Front. Oral. Health , 22 October 2024
Sec. Oral Infections and Microbes
Volume 5 - 2024 | https://doi.org/10.3389/froh.2024.1477004
This article is part of the Research Topic Oral Diseases and Prevention in Pregnant Women, Infants and Preschool Children View all 5 articles
The oral microbiome is a complex community of microorganisms residing in the oral cavity interacting with each other and with the host in a state of equilibrium. Disruptions in this balance can result in both oral and systemic conditions. Historically, studying the oral microbiome faced limitations due to culture-dependent techniques that could not capture the complexity and diversity of the microbial community. The emergence of advanced genomic technologies and the ease of sample collection from the oral cavity has revolutionized the understanding of the oral microbiome, providing valuable insights into the bacterial community in both health and disease. This review explores the oral microbiome in children, discussing its formation and dynamics in both states of health and disease, its role in various conditions such as dental caries, periodontal disease, oral cancer, cleft lip and palate, and explores its connection to several systemic consequences.
First introduced by Joshua Lederberg, the human microbiome represents an ecosystem of microorganisms that inhabit the human body and interact with each other in a symbiotic way (1). The current microbiome reflects millions of years of coevolution between humans and microorganisms. Over time, both have mutually adapted to each other, and the microbiome now plays an essential role in our health and disease (2). To research host-microbe interactions, traditional microbial ecology methods have relied on culture-dependent methodologies which led to a limited perspective of microbiota (3). These methods rely on cultivating microorganisms in laboratory settings, enabling in-depth analysis of their biochemical and physiological traits, such as metabolic functions and resistance to antibiotics. While these methods have been fundamental in microbiology, they face notable limitations, particularly in detecting anaerobic microbes that cannot easily grow in standard lab environments. Research indicates that traditional culturing techniques can overlook a large portion of the oral microbiome, with estimates suggesting that more than 50% of oral bacteria are “unculturable” using conventional approaches (4). Molecular technologies, such as next-generation sequencing, enabled comprehensive investigation of the microbes in the different body habitat, including those that cannot be cultured in a laboratory. This approach has led to a revolution in understanding microbial communities, allowing researchers to explore the composition, diversity, and interactions of these communities in their natural habitat (3). However, these techniques also have limitations, including higher costs, the need for advanced bioinformatics expertise, and the inability to directly measure microbial viability or activity as culture-based studies do (5).
The oral cavity represents the second most diverse microbial community in the human body after the gut, harbouring over 700 different types of bacteria (6). These bacteria inhabit various locations in the mouth, including the teeth, tongue, periodontal structures, and cheeks; all are interconnected by saliva (7). The human oral microbiome was reported to be influenced by different factors from maternal and environmental sources. The interactions occurring between the microbiome and the human host during early life are accountable for shaping innate and acquired immune functions, as well as physiological development, which in turn have implications for future health outcomes (8).
Most of the literature on the oral microbiome focuses on adults, detailing both its composition in healthy individuals and its alterations in various pathological conditions, in contrast, the studies exploring the microbiome of children is relatively sparse, with a predominant focus on dental caries (9). This review aims to highlight the current state of knowledge regarding the microbiome of children in both health and disease, state gaps in current understanding, and propose future research directions.
A literature search was conducted across several electronic databases, including PubMed/MEDLINE, Scopus, the COCHRANE library and Web of Science. The search was performed using a combination of relevant keywords and MeSH terms such as “oral microbiome”, “oral microbiota”, “oral bacteria”, “children”, “pediatric oral health”, “dental caries”, “periodontal disease”, “Molar-Incisor Hypomineralization”, “oral cancer”, “cleft lip and palate”, “systemic disease”, “diseases”. Only articles published between January 1990 and January 2024 in English have been included.
The search obtained 345,163 results, after the screening of the titles and abstracts, non-topic entries were excluded. References were exported and managed using EndNote 20.
Due to the extensive number of articles included and the diverse methods and outcomes among the identified studies, it was not feasible to present the findings as a systematic review or meta-analysis. As a result, a narrative review was carried out.
A pregnant woman's oral health and oral microbiome can have a direct impact on her pregnancy and her developing baby. Additionally, it is proposed that the immune system of the fetus develops a prenatal tolerance to the maternal microbiome considering it safe during postnatal exposure to and potentially contributing to the successful development of a balanced microbiome (10). Once a healthy microbiome is established, it must constantly adapt to changes in the oral environment, including changes in temperature, pH, forces of brushing and mastication, and the presence of different types of food and oral hygiene products (10).
At birth, infants encounter their mother's microbiome via vertical transmission. Vaginally born babies are exposed to their mother's vaginal and rectal microbes, while caesarean section babies are exposed to their mother's skin and hospital environment microbes (11, 12). This difference in exposure can have a significant impact on the development of the baby's microbiome. At the age of 3 months, vaginally born infants exhibit higher diversity of the microbiome at the taxonomic level (13). A study comparing maternal factors in the acquisition of Streptococcus mutans in infants revealed that infants born with caesarean section acquired S. mutans almost 12 months earlier compared to vaginally born infants (14). Diet is also reported as a factor affecting the microbiome, breast-fed infants possess oral Lactobacillus spp. that are absent in formula-fed infants at 3 months of age both in vivo and in vitro (15, 16). It has been proposed that microorganisms are obtained during a specific period during which colonization of certain bacteria occurs, known as “window of infectivity”. In the case of S. mutans, its colonization occurs between 19 and 31 months of age (17). An additional suggested “window”, occurring between the ages of 6 and 12 years, aligns with the emergence of permanent dentition (18).
In addition to vertical transmission, the oral microbiome can also be shared among individuals living in the same environment, such as siblings, this is termed horizontal transmission which contributes to the diversity of the oral microbiome (19, 20). This transmission may occur due to shared use of toys, foods, or utensils, potentially influenced by hygiene practices and awareness in these environments (21).
Analysis of the composition of the healthy oral microbiome in infants, children, and adolescents based on 16S rRNA gene sequencing techniques using plaque and saliva samples found that Proteobacteria, Fusobacterium, Actynobacteria, Bacteroidetes, Firmicutes, Synergistetes, Tenericutes, Capnocytophaga, Neisseria, Sreptococcus, Kingella, Leptotrichia. Burkholderia, and Strenotrophomas Enterobacteriaceae are dominant genera with a high level of abundance at 12–24 months old. On the other hand, Firmicutes, Proteobacteria, Actinobacillus, Bacteroidetes, Fusobacterium, Streptococcus, Prevotella, Veillonella, Neisseria, Rothya, and Haemophilus are predominant genera in children (9).
In a cohort of 1-year old infants with a healthy oral cavity, a notable difference in the oral microbial composition was identified between children who maintained a caries-free status and those who developed cavitated caries. The relative abundance of Prevotella nanceiensis, Leptotrichia sp. HMT 215, Prevotella melaninogenica, and Campylobacter concisus was found to be significantly higher in the group of children who remained caries-free. This observation suggests a potential association between the prevalence of these specific microbial species and a reduced likelihood of developing cavitated caries in the studied population (22).
A multicenter longitudinal study exploring the establishment of a healthy oral microbiome in caries-free children aged 1–4 years, based on a sample of 119 individuals, the microbial composition in saliva and plaque experienced significant alterations from the age of 1–2.5 years, followed by more subtle microbial changes up to the age of 4 years. The study identified a general increase in microbial diversity as age increased, with limited number of taxa, including various species of the Streptococcus and Gemella genera, were consistently present in all samples across different time points in children. The study concluded that the oral ecosystem of caries-free children is characterized by significant heterogeneity and dynamic changes (23). By adolescence, puberty brings significant hormonal changes that influence the oral microbiome. Increased levels of sex hormones (e.g., estrogen, progesterone) create an environment conducive to the growth of Porphyromonas spp. and Prevotella spp. associated with gingival inflammation and periodontitis (24) (Table 1).
Studies have also compared the oral microbiome between primary and permanent dentition, particularly focusing on the transitional period of mixed dentition. During this stage, significant differences in microbial composition are observed between primary and permanent teeth within the same oral cavity. One study found that permanent molars tend to have a distinct microbial profile compared to primary teeth, with variations in the abundance of specific bacterial genera including Fusobacteria and Bacteroidetes. These differences are influenced by the physicochemical and developmental changes in the oral environment during the eruption of permanent teeth, such as oxygen levels (25). Another study revealed that the transition from primary to permanent dentition is accompanied by increased microbial diversity. As it leads to the colonization of additional microbial species from genera such as Streptococcus, Gemella, Granulicatella, and Veillonella. As well as expanded functional capacities within the oral microbiome. These changes reflect the oral microbiome's adaptation to new niches created by the eruption of permanent teeth (26).
Dental caries is a complex disease caused by the interaction of four main factors, the susceptible host, the microbiome, the substrate, and the element of time (27). It is important to highlight that S. mutans, the extensively researched species linked to caries, comprises merely <1% of the overall bacterial community (28). As a result, identifying the microorganisms causing dental caries has been a significant focus of research for decades, and developments in the field of oral microbiome identification and analysis techniques have resulted in changes in the documented composition and characteristics of oral microbiota (29). Caries can develop in the absence of Streptococcus and Lactobacillus species, in addition, the S. mutans count has been found to be low even when caries is present, suggesting that other species may be responsible for caries development and progression (30). Besides S. mutans, various other species associated with caries were reported in the literature, including but not limited to species such as Veillonella dispar, Prevotella spp., Lactobacillus spp., Leptotrichia spp., Actinomyces spp., Neisseria spp., Porphyromonas spp., Treponema spp., and Streptococcus sobrinus (31–41).
When comparing microbial composition, the unstimulated saliva of children affected by severe early childhood caries with that of caries-free children, saliva of caries-free individuals showed elevated quantities of Capnocytophaga and Leptotrichia while levels of Porphyromonas and Neisseria were lower at genus level (34).
As alterations in the microbiome can contribute to oral disease, certain conditions can, in turn, induce changes in the microbiome, thereby influencing the oral health. MIH is one of those unique conditions in which microbiome is altered and caries progress faster in the affected teeth compared to none-affected teeth (42). Molar-incisor hypomineralization (MIH) is defined as a developmental enamel defect that affects at least one permanent first molar. Affected anterior teeth might also be observed (43). Caries can easily develop in the affected teeth due to their porous nature, and this problem is exacerbated by the fact that children avoid cleaning their sensitive teeth, resulting in greater food and biofilm stagnation (43). The study by Hernández et al. observed more bacterial diversity, higher count of bacterial niches, and predominance of proteolytic bacterial genera such as Catonella, Fusobacterium, Campylobacter, Tannerella, Centipeda, Streptobacillus, and Alloprevotella in teeth affected with MIH, suggesting the presence of a unique microbiome related to MIH (42).
The term “periodontal diseases” encompasses both inherited and acquired disorders affecting the tissues that surround and support the teeth, including the gingiva, cementum, periodontal ligament (PDL), and alveolar bone (44). The evidence suggests that the development of periodontal diseases is associated with an increase in the quantities of Gram-negative bacteria and anaerobes in subgingival plaque (45, 46). Gingivitis is very common in children and adolescents (47, 48) and can progress to more severe forms of periodontal disease, such as periodontitis, involving the loss of connective tissue and bone around the teeth (12, 49–51). A systematic reviews on the relationship between maternal periodontitis and adverse pregnancy outcomes concluded that mothers with periodontal disease face a higher risk of preterm birth, delivering a low-birth-weight baby, and developing preeclampsia (52). It is suggested that periodontal pathogens or their by-products, such as endotoxins, reach the placenta and the fetus (53).
Several studies have investigated the microbiome of periodontal disease in children. The main evidence related to a particular microbial cause of periodontitis in children is derived from research on a distinct clinical periodontal syndrome that impacts adolescents exhibiting a pattern of lesions distributed around molars and incisors, known as localized juvenile periodontitis (LJP). In LJP, A. actinomycetemcomitans, Capnocytophaga sp., Eikenella corrodens, Prevotella intermedia, and motile anaerobic rods, such as Campylobacter rectus are the most detected organisms (54). A. actinomycetemcomitans, Capnocytophaga sp., and P. intermedia have been commonly identified as well in the subgingival microbiota of periodontitis in the primary dentition in children (55–60). A recent study found that schoolchildren with early-onset periodontitis had a higher abundance of certain bacteria, such as Campylobacter species, Prevotella intermedia, and Fusobacterium nucleatum, Porphyromonas gingivalis, Treponema denticola, and Tannerella forsythia (61).
A developing concept in the field of cancer biology suggests that the microbiome serves as a significant environmental factor that influences the process of oncogenesis. There is a growing body of evidence indicating a connection between alterations in the human microbiome and specific types of cancer (62–65). Specific oral taxa, such as Porphyromonas gingivalis and Fusobacterium nucleatum, possess carcinogenic characteristics, including the inhibition of apoptosis, stimulation of cellular proliferation, initiation of chronic inflammation, facilitation of cellular invasion, and the generation of carcinogenic substances (66). Both of the aforementioned taxa have the capacity to release endotoxins, specifically lipopolysaccharides, which, in turn, can trigger the production of cytokines associated with inflammation, which is a primary factor in bacteria-induced inflammation and serves as a contributing factor to the process of carcinogenesis as well (67–69). A novel study investigated the oral microbiome of children diagnosed with solid tumors, analyzing diversity, composition, and gene profiles using saliva samples. Children with tumors exhibited a reduction in oral microbiome diversity compared to the healthy controls, with genera such as Veillonellaceae, Firmicutes unclassified, Coriobacteriia, Atopobiaceae, Negativicutes significantly enriched among them. This study suggests that oral microbiome could function as a non-invasive diagnostic tool for patients with pediatric solid tumors (70).
Each year, a significant number of newly diagnosed cancer cases are reported, with oral cancers exhibiting a particularly high prevalence among them, specifically oral squamous cell carcinoma (OSCC) (71). While OSCC is very rare in children, studies primarily focused on the adult population have shown variations in the microbiome between a healthy oral cavity and OSCC. Although the specific pathogenic bacteria or bacterial spectrum linked to OSCC has not been determined (72), elevated concentrations of Peptostreptococcus spp., Fusobacterium spp., Prevotella melaninogenica, Porphyromonas spp., Veillonella parvula, Haemophilus spp., Rothia spp., and Streptococcus spp. have been identified in samples of OSCC (73–77).
The theory suggesting a connection between oral microbiome and systemic diseases revolves around the potential consequences of bacteremia, which denotes the presence of bacteria in the bloodstream, dental procedures as well as routine practices such as eating, chewing, or brushing can trigger bacteremia (78). Usually the immune system promptly manages and eliminates microorganisms from the systemic circulation, however, in specific cases, particularly in individuals with compromised immune systems, oral organisms may persist without elimination, colonizing certain distant sites and increasing the likelihood of systemic disease development (79). It was also found that certain systemic bacteria can reside in specific oral locations, such as: Hemophilus influenzae, Pseudomonas aeruginosa, and Trophyrema whipplei have been identified in the gingival sulcus (79). Conversely, oral bacteria such as Porphyromonas gingivalis, Treponema denticola, and Campylobacter rectus have also been detected in systemic sites, including atheroma plaques, valvular vegetations, joint cavities, and the pancreas (79). Moreover, it is not just bacteria that can enter the bloodstream; their bacterial byproducts and endotoxins can also be discharged into the systemic circulation. This has the potential to initiate inflammatory reactions in particular locations, thereby heightening the risk of developing systemic diseases (80). On the other hand, systemic diseases can significantly influence the composition and dynamics of the oral microbiota, this is attributed to the high level of inflammation associated with these conditions affects the oral microbiota, leading to significant alterations (81).
Many studies have been conducted to examine the oral microbiome of children with systemic diseases. Francavilla et al. studied the salivary microbiome and metabolome in 13 children with celiac disease (CD) following gluten-free diets (T-CD) and their healthy counterparts (HC). The findings indicate an association between celiac disease and dysbiosis in the oral microbiota, potentially influencing the oral metabolome. Those with T-CD exhibited a less diverse salivary microbiome, with increased abundance of Rothia spp., Porphyromonas endodontalis, Gemella spp., Prevotella nanceiensis, S. sanguinis, and Lachnospiraceae spp. compared to their healthy counterparts. Furthermore, children with T-CD showed a reduced abundance of Actinomyces species, Atopobium species, and Corynebacterium durum (82). This is attributed to the fact that patients with CD typically exhibit lower levels of amylase and secretory IgA and IgM in their saliva (83, 84). Additionally, their saliva tends to have reduced buffering capacity, lower salivary flow rates, and lower concentrations of calcium, as well as decreased calcium/ phosphate ratios (85, 86).
Recent studies have revealed a link between neuropsychiatric disorders (NPD), such as autism spectrum disorder (ASD) and the gut microbiota, which can interact with and impact the brain via the Gut-Brain Axis (GBA). Similarly, there is an emerging concept of oral-microbiota-brain axis (OMBA). Research on the oral microbiome and its relationship with the brain indicates that microbes in the mouth may also play a role in influencing NPD outcomes (87). Oral microbiota can enter the brain through the cardiovascular system, where they are believed to directly contribute to the disruption of essential neurological functions and the deterioration of brain tissue due to the buildup of virulent byproducts (88). P. gingivalis is known to enter the bloodstream and reach the brain, where it establishes colonies and secretes neurotoxic proteases known as gingipains. These gingipains play a role in disrupting the processing of the transmembrane protein Amyloid Precursor Protein (APP), which is crucial for maintaining synaptic stability, as well as for promoting neuronal growth and protection (88).
Hicks et al. studied the oral microbiome alterations in children with ASD, they detected alterations in the salivary microbiome among children in the age range of 2–6 years, categorized into three developmental profiles: autism spectrum disorder (ASD; n = 180), nonautistic developmental delay (DD; n = 60), and typically developing (TD; n = 106) children (89). In the comparison of taxa between children with ASD and TD children, there was an increased abundance of two species in ASD children: Limnohabitans sp. 63ED37-2 and Planctomycetales (89). The findings of the study suggest that disturbances in the gut microbiome observed in ASD may also extend to the oropharynx. Subsequently, the regular evaluation of children's oral microbiome could potentially be developed as a non-invasive and sensitive tool for diagnosing ASD and monitoring its progression (89).
Several studies have confirmed a relationship between diabetes mellitus (DM) and periodontitis in adults, as DM induces changes in connective tissue metabolism, leading to a decreased ability to resolve inflammation and undergo remodeling, which, in turn, exacerbates periodontal damage (90). However, studies in children are still limited. A cohort study conducted in 2021 analyzed the salivary microbiome of 37 children aged 5–15 years diagnosed with type 1 DM, in children with type 1 DM, Streptococcus genus was found to be more prevalent. In addition, 22 taxa at the genus level and 33 taxa at the species level were absent in the control group, whereas the control group showed 6 taxa at the genus level and 9 taxa at the species level that were not present in the diabetes group (91). A cross-sectional study conducted in the same year evaluated the composition and abundance of bacterial microbiota in the oral swabs of children aged 10–18 years diagnosed with type 1 DM (well controlled and poorly controlled), compared with those of healthy children. The group of children with well controlled DM exhibited a notably elevated count of bacteria belonging to the Streptococcus genus compared to healthy children. The presence of Streptococcus mitis was notably higher in the group of children diagnosed with type 1 DM compared to their healthy counterparts, which is considered one of the primary colonizers of the pellicle (92). A recent study assessed the relationship between the composition of oral bacteria in saliva samples, oral hygiene practices, and glycemic control in a group of children with a mean age of 12.6 years diagnosed with type 1 DM. A high abundance of bacteria related to dental caries and periodontal disease, specifically, Actinomyces spp., Aggregatibacter actinomycetemcomitans, Prevotella intermedia, and Lactobacillus spp. were identified in all subjects. S. mutans was detected in approximately half of the samples, particularly among patients exhibiting poor glycemic control. Positive oral hygiene behaviors, such as regular toothbrush replacement and professional dental cleanings, were inversely correlated with the concurrent presence of Tannerella forsythia, Treponema denticola, and Porphyromonas gingivalis bacteria associated with periodontal disease (93). In children with DM, the formation of advanced glycation end products (AGEs), which occurs through a non-enzymatic reaction between sugars and proteins, lipids, or nucleic acids, is accelerated as a result of chronic hyperglycemia (91). AGEs disrupt the normal function of nearly all body organs including the oral cavity by triggering apoptosis, inflammation, protein dysfunction, mitochondrial impairment, and oxidative stress (94). It was also found that the periodontal pathogen Tannerella forsythia produces methylglyoxal (a precursor of AGEs) in gingival tissues, this finding suggests bidirectional relationship between periodontal disease and poor glycemic control (95).
Cleft lip and/or palate (CLP) is one of the conditions that has a significant influence on the composition of the oral microbiome. It is the most prevalent congenital craniofacial defect impacting the structure and function of the oral cavity and causing alterations in facial features (96–99). Children born with CLP may experience significant functional challenges related to routine activities such as sucking, swallowing, chewing, speaking, breathing, and social integration. Consequently, they necessitate extensive and prolonged rehabilitation starting from infancy and extending throughout adulthood (100–102).
A systematic review by Świtała et al. examined the current scientific literature on the oral microbiome in children with CLP, the review encompassed twelve studies investigating the microbiological status of individuals with CLP in comparison to non-cleft individuals (103). The overall findings revealed a higher incidence of caries in children with CLP compared to those without, associated with higher levels of S. mutans and/or Lactobacillus spp. (102, 104–111). Most of the studies suggested that this is attributed to various factors affecting the oral hygiene of those children, including, and not limited to: anxiety related to tooth brushing in the cleft area, lack of motivation, existence of malocclusion and structural irregularities, anomalies in tooth count, shape, and position, and prolonged presence of orthodontic appliances (103). The results of the studies presented in this review are shown in a summary table (Table 2).
When addressing ethical considerations in pediatric oral microbiome research, several factors must be considered: First, informed consent and assent, which is typically obtained from parents or legal guardians, as children are generally not legally capable of providing full consent. However, ethical standards recommend also seeking assent from the child, depending on their age and cognitive development. This approach involves explaining the research in a way that is understandable for the child, ensuring they comprehend the study, and respecting their decision to participate or decline (112, 113). Second, sample collection, which is usually non-invasive (e.g., saliva, swabs) and cause minimal discomfort. Researchers should also ensure the child's understanding and comfort with the procedures. Any invasive methods must be justified by clear benefits outweighing the risks and discomfort (113). Third, privacy and data protection, which is a key concern, particularly when managing genetic or sensitive health data. Adherence to data protection laws, such as the General Data Protection Regulation (GDPR) in Europe and the Health Insurance Portability and Accountability Act (HIPAA) in the U.S., is essential. Researchers must ensure that personal data is anonymized and securely stored to protect children's privacy during and after the research (113).
After years of investigation, researchers have progressively unveiled a novel understanding of the microbiome's involvement in both health and disease. It is now established that there is a bidirectional relationship between the host and its microbiome, as the microbiome can influence nearly every aspect of the host, and disturbances in its balance are linked to a broad spectrum of diseases, certain conditions can prompt alterations in the microbiome, consequently impacting oral health. Advanced research technologies facilitate the close examination of how the microbiome contributes to human health and participates in the development of diseases. However, the predominant focus of microbiome studies has been on the bacterial component, leaving the roles of fungi, viruses, and other microbes uncertain. Moreover, although dysbiosis of the microbiome is frequently observed in disease states, establishing the causative role of the microbiota remains an ongoing challenge. Consequently, numerous questions persist in this field, awaiting further exploration and clarification.
The future application of the oral microbiome for enhancing human health will rely on additional validation of microbial biomarkers specific to diseases. These markers need to be integrated into diagnostic and preventive programs that are not only sensitive and specific but also provide rapid results and are cost-effective for widespread implementation. When combined with human genomics, proteomics, transcriptomics, metabolomics, and utilization of artificial intelligence, the oral microbiome of children has the potential to play a central role in the advancement of precision medicine, facilitating the development of personalized preventive dental programs in the future.
SA: Conceptualization, Project administration, Writing – original draft, Writing – review & editing. AA: Conceptualization, Supervision, Writing – original draft, Writing – review & editing. SB: Conceptualization, Supervision, Writing – original draft, Writing – review & editing. TA: Conceptualization, Supervision, Writing – original draft, Writing – review & editing. NB: Conceptualization, Supervision, Writing – original draft, Writing – review & editing, Project administration.
The author(s) declare that no financial support was received for the research, authorship, and/or publication of this article.
The authors declare that the research was conducted in the absence of any commercial or financial relationships that could be construed as a potential conflict of interest.
All claims expressed in this article are solely those of the authors and do not necessarily represent those of their affiliated organizations, or those of the publisher, the editors and the reviewers. Any product that may be evaluated in this article, or claim that may be made by its manufacturer, is not guaranteed or endorsed by the publisher.
1. Lederberg J, McCray AT. ‘Ome sweet ‘omics—a genealogical treasury of words. Scientist. (2001) 15:8–9.
2. Kilian M. The oral microbiome—friend or foe? Eur J Oral Sci. (2018) 126(Suppl 1):5–12. doi: 10.1111/eos.12527
3. Thirunavukarasou A, Kaur S, Khera HK. Metagenomics for Studying Microbes in Wastewater Treatment Plants, in Microbial Community Studies in Industrial Wastewater Treatment. Boca Raton, Florida, USA: CRC Press (2022). p. 171–83.
4. Wade WG. Has the use of molecular methods for the characterization of the human oral microbiome changed our understanding of the role of bacteria in the pathogenesis of periodontal disease? J Clin Periodontol. (2011) 38(s11):7–16. doi: 10.1111/j.1600-051X.2010.01679.x
5. Forbes JD, Knox NC, Ronholm J, Pagotto F, Reimer A. Metagenomics: the next culture-independent game changer. Front Microbiol. (2017) 8:1–21. doi: 10.3389/fmicb.2017.01069
6. Shaiber A, Willis AD, Delmont TO, Roux S, Chen LX, Schmid AC, et al. Functional and genetic markers of niche partitioning among enigmatic members of the human oral microbiome. Genome Biol. (2020) 21(1):292. doi: 10.1186/s13059-020-02195-w
7. Mark Welch JL, Ramírez-Puebla ST, Borisy GG. Oral microbiome geography: micron-scale habitat and niche. Cell Host Microbe. (2020) 28(2):160–8. doi: 10.1016/j.chom.2020.07.009
8. Xiao J, Fiscella KA, Gill SR. Oral microbiome: possible harbinger for children’s health. Int J Oral Sci. (2020) 12(1):12. doi: 10.1038/s41368-020-0082-x
9. D'Agostino S, Ferrara E, Valentini G, Stoica SA, Dolci M. Exploring oral microbiome in healthy infants and children: a systematic review. Int J Environ Res Public Health. (2022) 19(18):1–13. doi: 10.3390/ijerph191811403
10. Zaura E, Nicu EA, Krom BP, Keijser BJ. Acquiring and maintaining a normal oral microbiome: current perspective. Front Cell Infect Microbiol. (2014) 4:85–93. doi: 10.3389/fcimb.2014.00085
11. Dominguez-Bello MG, Costello EK, Contreras M, Magris M, Hidalgo G, Fierer N, et al. Delivery mode shapes the acquisition and structure of the initial microbiota across multiple body habitats in newborns. Proc Natl Acad Sci U S A. (2010) 107(26):11971–5. doi: 10.1073/pnas.1002601107
12. Kaan AMM, Kahharova D, Zaura E. Acquisition and establishment of the oral microbiota. Periodontol 2000. (2021) 86(1):123–41. doi: 10.1111/prd.12366
13. Lif Holgerson P, Harnevik L, Hernell O, Tanner AC, Johansson I. Mode of birth delivery affects oral Microbiota in infants. J Dent Res. (2011) 90(10):1183–8. doi: 10.1177/0022034511418973
14. Li Y, Caufield PW, Dasanayake AP, Wiener HW, Vermund SH. Mode of delivery and other maternal factors influence the acquisition of Streptococcus mutans in infants. J Dent Res. (2005) 84(9):806–11. doi: 10.1177/154405910508400905
15. Holgerson PL, Vestman NR, Claesson R, Ohman C, Domellof M, Tanner AC, et al. Oral microbial profile discriminates breast-fed from formula-fed infants. J Pediatr Gastroenterol Nutr. (2013) 56(2):127–36. doi: 10.1097/MPG.0b013e31826f2bc6
16. Vestman NR, Timby N, Holgerson PL, Kressirer CA, Claesson R, Domellof M, et al. Characterization and in vitro properties of oral lactobacilli in breastfed infants. BMC Microbiol. (2013) 13:193. doi: 10.1186/1471-2180-13-193
17. Caufield PW, Cutter GR, Dasanayake AP. Initial acquisition of mutans streptococci by infants: evidence for a discrete window of infectivity. J Dent Res. (1993) 72(1):37–45. doi: 10.1177/00220345930720010501
18. Straetemans MM, van Loveren C, de Soet JJ, de Graaff J, ten Cate JM. Colonization with mutans streptococci and lactobacilli and the caries experience of children after the age of five. J Dent Res. (1998) 77(10):1851–5. doi: 10.1177/00220345980770101301
19. Baca P, Castillo AM, Liebana MJ, Castillo F, Martin-Platero A, Liebana J. Horizontal transmission of Streptococcus mutans in schoolchildren. Med Oral Patol Oral Cir Bucal. (2012) 17(3):e495–500. doi: 10.4317/medoral.17592
20. Stahringer SS, Clemente JC, Corley RP, Hewitt J, Knights D, Walters WA, et al. Nurture trumps nature in a longitudinal survey of salivary bacterial communities in twins from early adolescence to early adulthood. Genome Res. (2012) 22(11):2146–52. doi: 10.1101/gr.140608.112
21. Manchanda S, Sardana D, Liu P, Lee GHM, Lo ECM, Yiu CKY. Horizontal transmission of Streptococcus mutans in children and its association with dental caries: a systematic review and meta-analysis. Pediatr Dent. (2021) 43:1E–12E.33662253
22. Raksakmanut R, Thanyasrisung P, Sritangsirikul S, Kitsahawong K, Seminario AL, Pitiphat W, et al. Prediction of future caries in 1-year-old children via the salivary microbiome. J Dent Res. (2023) 102(6):626–35. doi: 10.1177/00220345231152802
23. Kahharova D, Brandt BW, Buijs MJ, Peters M, Jackson R, Eckert G, et al. Maturation of the oral microbiome in caries-free toddlers: a longitudinal study. J Dent Res. (2020) 99(2):159–67. doi: 10.1177/0022034519889015
24. Cornejo Ulloa P, Krom BP, van der Veen MH. Sex steroid hormones as a balancing factor in oral host microbiome interactions. Front Cell Infect Microbiol. (2021) 11:714229. doi: 10.3389/fcimb.2021.714229
25. Shi W, Qin M, Chen F, Xia B. Supragingival microbial profiles of permanent and deciduous teeth in children with mixed dentition. PLoS One. (2016) 11(1):e0146938. doi: 10.1371/journal.pone.0146938
26. Mason MR, Chambers S, Dabdoub SM, Thikkurissy S, Kumar PS. Characterizing oral microbial communities across dentition states and colonization niches. Microbiome. (2018) 6(1):67. doi: 10.1186/s40168-018-0443-2
27. Davenport ES. Caries in the preschool child: aetiology. J Dent. (1990) 18(6):300–3. doi: 10.1016/0300-5712(90)90127-Z
28. Aas JA, Griffen AL, Dardis SR, Lee AM, Olsen I, Dewhirst FE, et al. Bacteria of dental caries in primary and permanent teeth in children and young adults. J Clin Microbiol. (2008) 46(4):1407–17. doi: 10.1128/JCM.01410-07
29. Keller MK, Kressirer CA, Belstrom D, Twetman S, Tanner ACR. Oral microbial profiles of individuals with different levels of sugar intake. J Oral Microbiol. (2017) 9(1):1355207. doi: 10.1080/20002297.2017.1355207
30. van Ruyven FO, Lingstrom P, van Houte J, Kent R. Relationship among mutans streptococci, “low-pH” Bacteria, and lodophilic polysaccharide-producing Bacteria in dental plaque and early enamel caries in humans. J Dent Res. (2000) 79(2):778–84. doi: 10.1177/00220345000790021201
31. Agnello M, Marques J, Cen L, Mittermuller B, Huang A, Chaichanasakul Tran N, et al. Microbiome associated with severe caries in Canadian first nations children. J Dent Res. (2017) 96(12):1378–85. doi: 10.1177/0022034517718819
32. Richards VP, Alvarez AJ, Luce AR, Bedenbaugh M, Mitchell ML, Burne RA, et al. Microbiomes of site-specific dental plaques from children with different caries status. Infect Immun. (2017) 85(8):10–1128. doi: 10.1128/IAI.00106-17
33. Dashper SG, Mitchell HL, Le Cao KA, Carpenter L, Gussy MG, Calache H, et al. Temporal development of the oral microbiome and prediction of early childhood caries. Sci Rep. (2019) 9(1):19732. doi: 10.1038/s41598-019-56233-0
34. Hurley E, Barrett MPJ, Kinirons M, Whelton H, Ryan CA, Stanton C, et al. Comparison of the salivary and dentinal microbiome of children with severe-early childhood caries to the salivary microbiome of caries-free children. BMC Oral Health. (2019) 19(1):13. doi: 10.1186/s12903-018-0693-1
35. Zheng Y, Zhang M, Li J, Li Y, Teng F, Jiang H, et al. Comparative analysis of the microbial profiles in supragingival plaque samples obtained from twins with discordant caries phenotypes and their mothers. Front Cell Infect Microbiol. (2018) 8:361. doi: 10.3389/fcimb.2018.00361
36. Ortiz S, Herrman E, Lyashenko C, Purcell A, Raslan K, Khor B, et al. Sex-specific differences in the salivary microbiome of caries-active children. J Oral Microbiol. (2019) 11(1):1653124. doi: 10.1080/20002297.2019.1653124
37. de Jesus VC, Shikder R, Oryniak D, Mann K, Alamri A, Mittermuller B, et al. Sex-Based diverse plaque microbiota in children with severe caries. J Dent Res. (2020) 99(6):703–12. doi: 10.1177/0022034520908595
38. Gussy M, Mnatzaganian G, Dashper S, Carpenter L, Calache H, Mitchell H, et al. Identifying predictors of early childhood caries among Australian children using sequential modelling: findings from the VicGen birth cohort study. J Dent. (2020) 93:103276. doi: 10.1016/j.jdent.2020.103276
39. Qudeimat MA, Alyahya A, Karched M, Behbehani J, Salako NO. Dental plaque microbiota profiles of children with caries-free and caries-active dentition. J Dent. (2021) 104:103539. doi: 10.1016/j.jdent.2020.103539
40. Dinis M, Agnello M, Cen L, Shokeen B, He X, Shi W, et al. Oral microbiome: Streptococcus mutans/caries concordant-discordant children. Front Microbiol. (2022) 13:1–10. doi: 10.3389/fmicb.2022.782825
41. Xu X, Shan B, Zhang Q, Lu W, Zhao J, Zhang H, et al. Oral microbiome characteristics in children with and without early childhood caries. J Clin Pediatr Dent. (2023) 47(2):58–67. doi: 10.22514/jocpd.2023.012
42. Hernandez M, Planells P, Martinez E, Mira A, Carda-Dieguez M. Microbiology of molar-incisor hypomineralization lesions. A pilot study. J Oral Microbiol. (2020) 12(1):1766166–1766166. doi: 10.1080/20002297.2020.1766166
43. Weerheijm K. Molar incisor hypomineralisation (MIH). Eur J Paediatr Dent. (2003) 4:114–20.14529330
44. Pihlstrom BL, Michalowicz BS, Johnson NW. Periodontal diseases. Lancet. (2005) 366(9499):1809–20. doi: 10.1016/S0140-6736(05)67728-8
45. Ximénez-Fyvie LA, Haffajee AD, Socransky SS. Comparison of the microbiota of supra- and subgingival plaque in health and periodontitis. J Clin Periodontol. (2000) 27(9):648–57. doi: 10.1034/j.1600-051x.2000.027009648.x
46. Ramberg P, Sekino S, Uzel NG, Socransky S, Indhe J. Bacterial colonization during de novo plaque formation. J Clin Periodontol. (2003) 30(11):990–5. doi: 10.1034/j.1600-051X.2003.00419.x
47. Chapple ILC, Mealey BL, Van Dyke TE, Bartold PM, Dommisch H, Eickholz P, et al. Periodontal health and gingival diseases and conditions on an intact and a reduced periodontium: consensus report of workgroup 1 of the 2017 world workshop on the classification of periodontal and peri-implant diseases and conditions. J Periodontol. (2018) 89(Suppl 1):S74–84. doi: 10.1002/JPER.17-0719
48. Lang NP, Bartold PM. Periodontal health. J Periodontol. (2018) 89(Suppl 1):S9–16. doi: 10.1002/JPER.16-0517
49. Caton JG, Armitage G, Berglundh T, Chapple ILC, Jepsen S, Kornman KS, et al. A new classification scheme for periodontal and peri-implant diseases and conditions—introduction and key changes from the 1999 classification. J Clin Periodontol. (2018) 45(Suppl 20):S1–8. doi: 10.1111/jcpe.12935
50. Murakami S, Mealey BL, Mariotti A, Chapple ILC. Dental plaque-induced gingival conditions. J Periodontol. (2018) 89(Suppl 1):S17–27. doi: 10.1002/JPER.17-0095
51. Trombelli L, Farina R, Silva CO, Tatakis DN. Plaque-induced gingivitis: case definition and diagnostic considerations. J Periodontol. (2018) 89(Suppl 1):S46–73. doi: 10.1002/JPER.17-0576
52. Daalderop LA, Wieland BV, Tomsin K, Reyes L, Kramer BW, Vanterpool SF, et al. Periodontal disease and pregnancy outcomes: overview of systematic reviews. JDR Clin Trans Res. (2018) 3(1):10–27. doi: 10.1177/2380084417731097
53. Madianos PN, Bobetsis YA, Offenbacher S. Adverse pregnancy outcomes (APOs) and periodontal disease: pathogenic mechanisms. J Periodontol. (2013) 84(4 Suppl):S170–80. doi: 10.1902/jop.2013.1340015
54. Tonetti M, Mombelli A. Early-onset periodontitis. Ann Periodontol. (2000) 4:39–53. doi: 10.1902/annals.1999.4.1.39
55. Asikainen S, Alaluusua S, Saxén L. Recovery of A. actinomycetemcomitans from teeth, tongue, and saliva. J Periodontol. (1991) 62(3):203–6. doi: 10.1902/jop.1991.62.3.203
56. Dibart S, Chapple IL, Skobe Z, Shusterman S, Nedleman HL. Microbiological findings in prepubertal periodontitis. A case report. J Periodontol. (1998) 69(10):1172–5. doi: 10.1902/jop.1998.69.10.1172
57. Kamma JJ, Lygidakis NA, Nakou M. Subgingival microflora and treatment in prepubertal periodontitis associated with chronic idiopathic neutropenia. J Clin Periodontol. (1998) 25(9):759–65. doi: 10.1111/j.1600-051X.1998.tb02518.x
58. Moore WE, Burmeister JA, Brooks CN, Ranney RR, Hinkelmann KH, Schieken RM, et al. Investigation of the influences of puberty, genetics, and environment on the composition of subgingival periodontal floras. Infect Immun. (1993) 61(7):2891–8. doi: 10.1128/iai.61.7.2891-2898.1993
59. Ram D, Bimstein E. Subgingival bacteria in a case of prepubertal periodontitis, before and one year after extractions of the affected primary teeth. J Clin Pediatr Dent. (1994) 19(1):45–7.7865423
60. Sixou JL, Robert JC, Bonnaure-Mallet M. Loss of deciduous teeth and germs of permanent incisors in a 4-year-old child. An atypic prepubertal periodontitis? A clinical, microbiological, immunological and ultrastructural study. J Clin Periodontol. (1997) 24(11):836–43. doi: 10.1111/j.1600-051X.1997.tb01198.x
61. Piwat S, Basic A, Pahumunto N, Teanpaisan R, Dahlen G. Periodontal diseases in Thai schoolchildren. Clinical and microbiological observations. Odontology. (2023) 112:232–41. doi: 10.1007/s10266-023-00817-w
62. Irfan M, Delgado RZR, Frias-Lopez J. The oral microbiome and cancer. Front Immunol. (2020) 11:591088. doi: 10.3389/fimmu.2020.591088
63. Kostic AD, Gevers D, Pedamallu CS, Michaud M, Duke F, Earl AM, et al. Genomic analysis identifies association of Fusobacterium with colorectal carcinoma. Genome Res. (2012) 22(2):292–8. doi: 10.1101/gr.126573.111
64. Chang AH, Parsonnet J. Role of bacteria in oncogenesis. Clin Microbiol Rev. (2010) 23(4):837–57. doi: 10.1128/CMR.00012-10
65. Whitmore SE, Lamont RJ. Oral bacteria and cancer. PLoS Pathog. (2014) 10(3):e1003933. doi: 10.1371/journal.ppat.1003933
66. Tuominen H, Rautava J. Oral Microbiota and cancer development. Pathobiology. (2021) 88(2):116–26. doi: 10.1159/000510979
67. Chattopadhyay I, Verma M, Panda M. Role of oral microbiome signatures in diagnosis and prognosis of oral cancer. Technol Cancer Res Treat. (2019) 18:1533033819867354. doi: 10.1177/1533033819867354
68. Vogelmann R, Amieva MR. The role of bacterial pathogens in cancer. Curr Opin Microbiol. (2007) 10(1):76–81. doi: 10.1016/j.mib.2006.12.004
69. Zhang G, Ghosh S. Molecular mechanisms of NF-kappaB activation induced by bacterial lipopolysaccharide through toll-like receptors. J Endotoxin Res. (2000) 6(6):453–7. doi: 10.1179/096805100101532414
70. Cui X, Du X, Cui X, Fan R, Pan J, Wang Z. Oral microbiome characteristics in patients with pediatric solid tumor. Front Microbiol. (2024) 14:1–12. doi: 10.3389/fmicb.2023.1286522
71. Tomazelli KB, Modolo F, Rivero ER. Evaluation of AgNORs in oral potentially malignant lesions. J Oncol. (2015) 2015:218280. doi: 10.1155/2015/218280
72. Wang L, Ganly I. The oral microbiome and oral cancer. Clin Lab Med. (2014) 34(4):711–9. doi: 10.1016/j.cll.2014.08.004
73. Chocolatewala N, Chaturvedi P, Desale R. The role of bacteria in oral cancer. Indian J Med Paediatr Oncol. (2010) 31(4):126–31. doi: 10.4103/0971-5851.76195
74. Lee WH, Chen HM, Yang SF, Liang C, Peng CY, Lin FM, et al. Bacterial alterations in salivary microbiota and their association in oral cancer. Sci Rep. (2017) 7(1):16540. doi: 10.1038/s41598-017-16418-x
75. Pushalkar S, Mane SP, Ji X, Li Y, Evans C, Crasta OR, et al. Microbial diversity in saliva of oral squamous cell carcinoma. FEMS Immunol Med Microbiol. (2011) 61(3):269–77. doi: 10.1111/j.1574-695X.2010.00773.x
76. Schmidt BL, Kuczynski J, Bhattacharya A, Huey B, Corby PM, Queiroz EL, et al. Changes in abundance of oral microbiota associated with oral cancer. PLoS One. (2014) 9(6):e98741. doi: 10.1371/journal.pone.0098741
77. Nagy KN, Sonkodi I, Szoke I, Nagy E, Newman HN. The microflora associated with human oral carcinomas. Oral Oncol. (1998) 34(4):304–8. doi: 10.1016/S1368-8375(98)80012-2
78. Poveda-Roda R, Jiménez Y, Carbonell E, Gavaldá C, Margaix-Muñoz MM, Sarrión-Pérez G. Bacteremia originating in the oral cavity. A review. Med Oral Patol Oral Cir Bucal. (2008) 13(6):E355–62.18521055
79. Loesche WJ. Association of the oral flora with important medical diseases. Curr Opin Periodontol. (1997) 4:21–8.9655017
80. Kumar PS. Oral microbiota and systemic disease. Anaerobe. (2013) 24:90–3. doi: 10.1016/j.anaerobe.2013.09.010
81. Pisano M, Giordano F, Sangiovanni G, Capuano N, Acerra A, D’Ambrosio F. The interaction between the oral microbiome and systemic diseases: a narrative review. Microbiol Res (Pavia). (2023) 14(4):1862–78. doi: 10.3390/microbiolres14040127
82. Francavilla R, Ercolini D, Piccolo M, Vannini L, Siragusa S, De Filippis F, et al. Salivary microbiota and metabolome associated with celiac disease. Appl Environ Microbiol. (2014) 80(11):3416–25. doi: 10.1128/AEM.00362-14
83. Lenander-Lumikari M, Ihalin R, Lähteenoja H. Changes in whole saliva in patients with coeliac disease. Arch Oral Biol. (2000) 45(5):347–54. doi: 10.1016/S0003-9969(00)00008-X
84. Samaşca G, Iancu M, Farcău D, Butnariu A, Pop T, Pirvan A, et al. Iga anti-tissue transglutaminase antibodies, first line in the diagnosis of celiac disease. Clin Lab. (2011) 57(9–10):695–701.
85. Mina SS, Azcurra AI, Dorronsoro S, Brunotto MN. Alterations of the oral ecosystem in children with celiac disease. Acta Odontol Latinoam. (2008) 21(2):121–6.19177847
86. Acar S, Yetkıner AA, Ersın N, Oncag O, Aydogdu S, Arıkan C. Oral findings and salivary parameters in children with celiac disease: a preliminary study. Med Princ Pract. (2012) 21(2):129–33. doi: 10.1159/000331794
87. Bowland GB, Weyrich LS. The oral-microbiome-brain axis and neuropsychiatric disorders: an anthropological perspective. Front Psychiatry. (2022) 13:810008. doi: 10.3389/fpsyt.2022.810008
88. Bulgart HR, Neczypor EW, Wold LE, Mackos AR. Microbial involvement in Alzheimer disease development and progression. Mol Neurodegener. (2020) 15(1):42. doi: 10.1186/s13024-020-00378-4
89. Hicks SD, Uhlig R, Afshari P, Williams J, Chroneos M, Tierney-Aves C, et al. Oral microbiome activity in children with autism spectrum disorder. Autism Res. (2018) 11(9):1286–99. doi: 10.1002/aur.1972
90. Xiao X, Liu S, Deng H, Song Y, Zhang L, Song Z. Advances in the oral microbiota and rapid detection of oral infectious diseases. Front Microbiol. (2023) 14:1121737. doi: 10.3389/fmicb.2023.1121737
91. Moskovitz M, Nassar M, Moriel N, Cher A, Faibis S, Ram D, et al. Characterization of the oral microbiome among children with type 1 diabetes compared with healthy children. Front Microbiol. (2021) 12:756808. doi: 10.3389/fmicb.2021.756808
92. Pachoński M, Koczor-Rozmus A, Mocny-Pachońska K, Łanowy P, Mertas A, Jarosz-Chobot P. Oral microbiota in children with type 1 diabetes mellitus. Pediatr Endocrinol Diabetes Metab. (2021) 27(2):100–8. doi: 10.5114/pedm.2021.104343
93. Carelli M, Maguolo A, Zusi C, Olivieri F, Emiliani F, De Grandi G, et al. Oral Microbiota in children and adolescents with type 1 diabetes Mellitus: novel insights into the pathogenesis of dental and periodontal disease. Microorganisms. (2023) 11(3):668. doi: 10.3390/microorganisms11030668
94. Sruthi CR, Raghu KG. Advanced glycation end products and their adverse effects: the role of autophagy. J Biochem Mol Toxicol. (2021) 35(4):e22710. doi: 10.1002/jbt.22710
95. Chopra A, Jayasinghe TN, Eberhard J. Are inflamed periodontal tissues endogenous source of advanced glycation end-products (AGEs) in individuals with and without diabetes Mellitus? A systematic review. Biomolecules. (2022) 12(5):642. doi: 10.3390/biom12050642
96. Szyszka-Sommerfeld L, Matthews-Brzozowska T, Kawala B, Mikulewicz M, Machoy M, Więckiewicz W, et al. The electrical activity of the masticatory muscles in children with cleft lip and palate. Int J Paediatr Dent. (2018) 28(2):257–65. doi: 10.1111/ipd.12349
97. Mossey P. Global strategies to reduce the healthcare burden of craniofacial anomalies. Br Dent J. (2003) 195(10):613. doi: 10.1038/sj.bdj.4810738
98. Mossey PA, Little J, Munger RG, Dixon MJ, Shaw WC. Cleft lip and palate. Lancet. (2009) 374(9703):1773–85. doi: 10.1016/S0140-6736(09)60695-4
99. Rawashdeh MA, Ayesh JA, Darwazeh AM. Oral candidal colonization in cleft patients as a function of age, gender, surgery, type of cleft, and oral health. J Oral Maxillofac Surg. (2011) 69(4):1207–13. doi: 10.1016/j.joms.2010.02.044
100. Szyszka-Sommerfeld L, Woźniak K, Matthews-Brzozowska T, Kawala B, Mikulewicz M. Electromyographic analysis of superior orbicularis oris muscle function in children surgically treated for unilateral complete cleft lip and palate. J Craniomaxillofac Surg. (2017) 45(9):1547–51. doi: 10.1016/j.jcms.2017.06.012
101. Millard T, Richman LC. Different cleft conditions, facial appearance, and speech: relationship to psychological variables. Cleft Palate Craniofac J. (2001) 38(1):68–75. doi: 10.1597/1545-1569_2001_038_0068_dccfaa_2.0.co_2
102. Perdikogianni H, Papaioannou W, Nakou M, Oulis C, Papagiannoulis L. Periodontal and microbiological parameters in children and adolescents with cleft lip and /or palate. Int J Paediatr Dent. (2009) 19(6):455–67. doi: 10.1111/j.1365-263X.2009.01020.x
103. Świtała J, Sycińska-Dziarnowska M, Spagnuolo G, Woźniak K, Mańkowska K, Szyszka-Sommerfeld L. Oral Microbiota in children with cleft lip and palate: a systematic review. J Clin Med. (2023) 12(18):1–17. doi: 10.3390/jcm12185867
104. Grewcock RE, Innes NPT, Mossey PA, Robertson MD. Caries in children with and without orofacial clefting: a systematic review and meta-analysis. Oral Dis. (2022) 28(5):1400–11. doi: 10.1111/odi.14183
105. Cheng LL, Moor SL, Kravchuk O, Meyers IA, Ho CT. Bacteria and salivary profile of adolescents with and without cleft lip and/or palate undergoing orthodontic treatment. Aust Dent J. (2007) 52(4):315–21. doi: 10.1111/j.1834-7819.2007.tb00508.x
106. Ahluwalia M, Brailsford SR, Tarelli E, Gilbert SC, Clark DT, Barnard K, et al. Dental caries, oral hygiene, and oral clearance in children with craniofacial disorders. J Dent Res. (2004) 83(2):175–9. doi: 10.1177/154405910408300218
107. Sundell AL, Ullbro C, Dahlen G, Marcusson A, Twetman S. Salivary microbial profiles in 5-year old children with oral clefts: a comparative study. Eur Arch Paediatr Dent. (2018) 19(1):57–60. doi: 10.1007/s40368-018-0326-z
108. Antoszewska J, Kawala B, Minch L. Selected aspects of the oral environment in cleft palate patients–a problem evidently beyond dentists’ scope. Postepy Hig Med Dosw (Online). (2010) 64:659–64.21228441
109. Parapanisiou V, Gizani S, Makou M, Papagiannoulis L. Oral health status and behaviour of Greek patients with cleft lip and palate. Eur Arch Paediatr Dent. (2009) 10(2):85–9. doi: 10.1007/BF03321606
110. Shashni R, Goyal A, Gauba K, Utreja AK, Ray P, Jena AK. Comparison of risk indicators of dental caries in children with and without cleft lip and palate deformities. Contemp Clin Dent. (2015) 6(1):58–62. doi: 10.4103/0976-237X.149293
111. Sundell AL, Ullbro C, Marcusson A, Twetman S. Comparing caries risk profiles between 5- and 10- year-old children with cleft lip and/or palate and non-cleft controls. BMC Oral Health. (2015) 15:85. doi: 10.1186/s12903-015-0067-x
112. American Academy of Pediatrics (AAP) Policy Statement by Committee on Bioethics. Informed consent, parental permission, and assent in pediatric practice. Committee on bioethics, American academy of pediatrics. Pediatrics. (1995) 95(2):314–7. doi: 10.1542/peds.95.2.314
Keywords: children, caries, dental plaque, gingivitis, periodontitis, oral microbiome, oral cancer
Citation: AlHarbi SG, Almushayt AS, Bamashmous S, Abujamel TS and Bamashmous NO (2024) The oral microbiome of children in health and disease—a literature review. Front. Oral. Health 5:1477004. doi: 10.3389/froh.2024.1477004
Received: 6 August 2024; Accepted: 2 October 2024;
Published: 22 October 2024.
Edited by:
Mariia Faustova, Poltava State Medical University, UkraineReviewed by:
Lifu Song, Chinese Academy of Sciences (CAS), ChinaCopyright: © 2024 AlHarbi, Almushayt, Bamashmous, Abujamel and Bamashmous. This is an open-access article distributed under the terms of the Creative Commons Attribution License (CC BY). The use, distribution or reproduction in other forums is permitted, provided the original author(s) and the copyright owner(s) are credited and that the original publication in this journal is cited, in accordance with accepted academic practice. No use, distribution or reproduction is permitted which does not comply with these terms.
*Correspondence: Nada Othman Bamashmous, bm9iYW1hc2htb3VzQGthdS5lZHUuc2E=
Disclaimer: All claims expressed in this article are solely those of the authors and do not necessarily represent those of their affiliated organizations, or those of the publisher, the editors and the reviewers. Any product that may be evaluated in this article or claim that may be made by its manufacturer is not guaranteed or endorsed by the publisher.
Research integrity at Frontiers
Learn more about the work of our research integrity team to safeguard the quality of each article we publish.