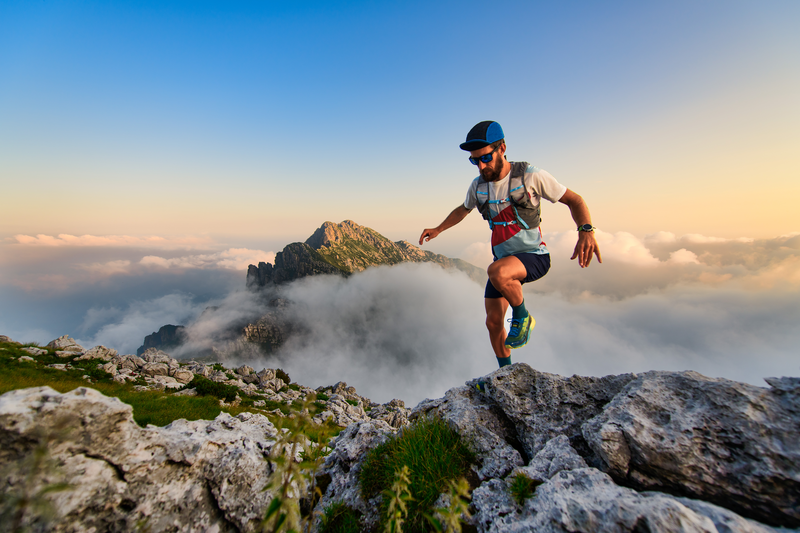
95% of researchers rate our articles as excellent or good
Learn more about the work of our research integrity team to safeguard the quality of each article we publish.
Find out more
REVIEW article
Front. Oncol. , 21 March 2025
Sec. Thoracic Oncology
Volume 15 - 2025 | https://doi.org/10.3389/fonc.2025.1555151
This article is part of the Research Topic Personalized Medicine in Early-Stage Lung Cancer: The Role and Future of Robotic-Assisted Techniques View all articles
In the care of lung cancer patients, early diagnosis followed by timely therapeutic procedures can have a significant impact on overall survival and patient anxiety. While robotic-assisted lung resection is now a widely accepted surgical approach, robotic-assisted bronchoscopy is a more recent diagnostic procedure that improves reach, stability, and precision in the field of bronchoscopic lung nodule biopsy. The ability to combine lung cancer diagnostics with curative-intent surgical resection into a single-setting anesthesia procedure has the potential to decrease costs, improve patient experiences, and most importantly, reduce delays in cancer care. In addition, with the expected adoption of sublobar resection for stage I lung cancer ≤2cm, combining robotic-assisted bronchoscopy with robotic surgery offers a single-setting pathway to take advantage of the precision biopsy and localization technique offered by robotic-assisted bronchoscopy and the precision operation offered by robotic surgery. We herein describe our approach to this single-setting procedure. While limited studies suggest that the combined approach results in shorter overall operative time and cost, we need future work to better characterize the overall operative time, complication rates, long-term oncologic outcomes, and cost analysis.
With advances in technology and initiation of lung cancer screening programs, the frequency of localized lung cancer amenable to curative intent has increased. Though only accounting for a small portion of total lung cancer diagnoses, the 5-year survival for only localized lung cancer is 65% (1). A vital part of lung cancer management is minimizing the time from diagnosis to therapeutic treatment. Delays in treatment, which are defined as resection 8 weeks or more after diagnosis, are associated with higher rates of upstaging, increased 30-day mortality, and decreased median survival (2). Delays in treatment are also accompanied with higher overall healthcare resource utilization and cost. Initiation of diagnostic workup does not always immediately yield a tissue diagnosis either. 46% of lung cancer patients will require two or more biopsies. In such cases, wedge resection for surgical biopsy can be required. Despite being a more invasive option, these can be in turn associated with 10-20% benign resection rate (3). Therefore, the ability to combine precise diagnostic procedures and therapeutic surgical resection, hence reducing delays and resultant rates of upstaging at diagnosis, may allow for less resource utilization and eventual cost. Higher stages have been shown by retrospective analysis to be associated with significantly higher total healthcare costs (4).
In the management of lung nodule/cancer, robot-assisted bronchoscopy (RAB) is an emerging technology to biopsy lung lesions. Combining RAB with robot-assisted thoracoscopic surgery (RATS) offers an approach that takes advantage of the two technologies (5). With the changing landscape of early-stage lung cancer treatment, combining RAB with RATS may offer several advantages with the primary benefit being single setting lung cancer diagnostics, mediastinal staging and definitive treatment while expediting overall lung cancer care. We herein review our approach to single setting RAB/RATS and various factors in this combined setting, such as cost and outcomes.
The American College of Chest Physicians and National Comprehensive Cancer Network guidelines both recommend obtaining a diagnosis of the primary lesion, resultant staging, and tissue for molecular testing by using the least invasive modality. Ideally, this would be a single procedure (6, 7). An initial bronchoscopic approach allows for biopsies of both the primary lesion of interest as well as mediastinal nodes for staging. The set of bronchoscopic procedural tools and technologies that aid in airway navigation, confirmation of target proximity, and tissue sampling are collectively known as guided bronchoscopy (8). The main components of guided bronchoscopy comprise image-mapped airway navigation, videoscopic real-time airway visualization, intraprocedural confirmatory imaging, and specimen acquisition devices. Studies have defined various metrics of guided bronchoscopy (9–16), defining diagnostic yield and safety, and comparing guided bronchoscopy methods with percutaneous sampling, consistently finding it to be safer relative to transthoracic sampling (17, 18). One published downside of the bronchoscopic approach is the diagnostic yield, which has been inconsistent in randomized controlled trials, with yields from 44% to 74%, compared with rates above 90% for percutaneous sampling (10–18). The novel technology of RAB allows us to overcome this main limitation, and there are different available platforms (19, 20). The Ion Robotic-Assisted Endoluminal Platform (Intuitive Surgical, Inc.) is based on novel shape-sensing RAB (ssRAB) technology (Figure 1) (21, 22). The Monarch RAB platform (Auris Health, Inc.) is based on electromagnetic navigation (EMN RAB) technology (23). RAB is designed to allow navigation into the lung periphery via endobronchial approach. The robotic control allows for increased catheter stability and guidance to maximize accuracy and precision for biopsy while still providing direct and/or virtual visualization of peripheral airways with simultaneous computer mapping of the catheter’s path and target (Figure 2). These advantages of RAB have been demonstrated in cadaveric models and subsequently supported by several clinical studies demonstrating a navigational success rate between 96.2% to 100% (24–31). Diagnostic yield for malignancy has been shown to be 69.1% using EMN RAB and as high as 88% using ssRAB, improved from comparative non-robotic bronchoscopic approaches for peripheral lesions (24–29, 31). The RAB technology is also well positioned to allow for integration with other existing valuable resources for the diagnosis, staging, and treatment of lung lesions such as endobronchial ultrasound (EBUS), radial EBUS, fluoroscopy, cone beam computed tomography and the da Vinci Surgical System.
Figure 2. Robotic assisted bronchoscopy is designed to allow endobronchial navigation into the lung periphery while allowing direct visualization of peripheral airways and maintaining catheter stability and shape to maximize precision during sampling.
Another advantage of RAB is its ability to definitively mark small peripheral lung lesions for eventual resection. Especially with improved efforts in lung cancer screening, we will encounter more small peripheral lesions, often ground glass in nature. Identifying these lesions intra-operatively can frequently pose a challenge, and the ability to localize these lesions pre-operatively can be useful, especially when this can be done at the same time as the surgical resection. Current methods for marking lung nodules include coils, hook-wire, or radiotracer placement percutaneously placed under CT guidance by interventional radiologists. Although these transthoracic methods have been found to be effective in delivering their markers, the higher rate of pneumothorax, bleeding, hematoma and dislodgement of tracer are noted limitations when compared to bronchoscopic approach (32, 33). Furthermore, these techniques and interventions require coordination and scheduling across multiple specialists and frequently result in significant delay to surgical resection (32–36). Bronchoscopic lung nodule marking has been found to be effective and safe in comparison with other methods (37, 38). Available techniques for peripheral lung nodule marking using RAB include but are not limited to dye marking (using methylene blue, indocyanine green or iopamidol) or fiducial marker placement.
Based on the systematic review and expert panel convened by the College of American Pathologists (CAP), rapid on-site evaluation (ROSE) is recommended, if available in EBUS- transbronchial needle aspiration (TBNA) and strongly recommended if clinically feasible in transthoracic needle procedures (39). Oki et al. performed a prospective randomized clinical trial on patients undergoing EBUS- TBNA and showed a statistically significant reduction in the need for additional procedures with ROSE (11% of patients in ROSE vs 57% in non-ROSE group; p<0.001) (40). ROSE has also shown to slightly improve diagnostic yield (absolute percentage increase of 2.9% – 8%) (41–43). In addition, studies suggest ROSE can ensure the adequacy of material obtained for ancillary studies and minimize molecular testing failures (44, 45). However, the lack of trained personnel, time commitment and other resources associated with ROSE may act as barriers against its use and thus may not be available in all hospital settings.
Another instance in which RAB/RATS combination may be beneficial is in the treatment of ≤2cm lesions, which we expect to see more of with improved lung cancer screening. With the recent findings from the JCOG 0802 and CALGB 140503 studies, sublobar resection for stage I lung cancer ≤2cm has become an acceptable surgery (46, 47). Hence, we may see an increase in the number of sublobar cases being performed for ≤2cm lesions, further emphasizing the importance of precision biopsy as well as the need for reliable localization technique to aid with the surgery. Segmentectomy can be a more technically demanding operation compared to lobectomy as it requires familiarity of the segmental anatomy. Features of the robotic platform may mitigate the technical demands of more distal dissection that is required in performing segmentectomy. In conjunction with the improved diagnostic tool in RAB, we may be able to obtain accurate diagnosis even in these small lesions, which subsequently could increase the number of segmentectomies being performed.
Preoperative evaluation is performed in a standard fashion, including CT and positron emission tomography (PET). We prefer a thin slice (1.0mm slices) chest CT imaging for pre-procedural planning and staging purposes. Pathway to the nodule is planned based on thin slice chest CT and can be done before the actual procedure. The patient is consented for both the RAB and RATS (in case if malignancy is confirmed on examination intraoperatively). Both procedures will be completed under a single anesthesia event (5).
Patient selection for the single anesthetic pathway is essential, and one must consider optimizing operating room block time, minimizing occult N2 nodal disease rate, and limiting benign resections. Although no published criteria exist for the single anesthetic pathway, the following are criteria our program follows:
● Nodule suspicious for NSCLC and ≤3cm.
● No chest wall or surrounding structure invasion which would make the lesion ≥T3.
● Clinical stage 1-2 NSCLC.
● No mediastinal adenopathy by CT nor PET criteria.
● Adequate PFTs with good performance status for the proposed resection.
● Patients who travel from far distance to receive treatment.
● Agreeable and trusting patient.
As the single anesthesia RAB/RATS approach requires multi-disciplinary approach, pre-procedure planning is of paramount importance. We have a workflow in which CT scans are performed per robotic navigational bronchoscopy protocols with the appropriate slice thickness and amount of overlap. Essential to this approach is a pathology team, as the cytologist not only has to make intraoperative judgements on tissue adequacy but also provide a diagnosis of malignancy or benign. Discussion with the anesthesia team involves single lumen intubation for RAB portion and double lumen for RATS. Communication between the interventional pulmonologist and thoracic surgeon can have various permutations. The pulmonologist may first perform the RAB biopsy and EBUS, then the surgeon performs the RATS in the same room if the set-up allows. Some have done RAB biopsy and EBUS in a bronchoscopy or endoscopy suite and then move the patient to the operating room for resection, while most will perform all stages of the single anesthetic event in the operating room.
We describe our intraoperative RAB/RATS workflow below (Figure 3).
Figure 3. Protocolized flowchart of a single-setting robotic-assisted bronchoscopy and thoracic surgery (created with BioRender.com). RAB, robot-assisted bronchoscopy; EBUS, endobronchial ultrasound; ROSE, rapid on-site evaluation; ICG, indocyanine green.
Patient is placed under general anesthesia with muscle paralysis in the supine position. Patient is then intubated with a single lumen endotracheal tube (ETT), at least 8.0 in size. We perform a standard flexible bronchoscopy to ensure the tip of ETT is at least 2-3 cm from the carina and to clear any secretions.
The robot is docked to an endotracheal tube adapter. The virtual bronchoscopy is registered to the patient’s airway in real time by marking the main carina as well as each subsegment of the upper and lower lobes. After registration, navigation begins using real-time bronchoscopic view of the patient’s airways complemented with a virtual bronchoscopy image, which navigates the user towards the lesion. When the target lesion is within 1-3cm from the tip of the catheter, the vision probe is removed (when using Ion Endoluminal System), and a radial endobronchial ultrasound (R-EBUS) is utilized to identify and confirm lesion. If biopsy requires traversing a cartilaginous bronchial wall, a needle is first used to puncture the wall to create a path for the R-EBUS probe. Once confirmed, biopsies are taken under image guidance with tools such flexible needles for fine needle aspiration (FNA) samples and forceps for frozen section specimens. Adjunct imaging to help with the biopsy include 2D fluoroscopy, 3D fluoroscopy, or cone-beam CT scan. Cytology slides from FNA samples can be read with ROSE. Forcep specimens can be processed by frozen section for intraoperative interpretation or by touch-prep and cytologic interpretation.
Once a diagnosis of malignancy is confirmed, the proceduralist has the option to mark the nodule. Our program uses a mixture of 0.5mL of methylene blue and 0.5mL of ICG. The 1.0mL total volume includes priming of the needle, and it is not necessary to clear the needle with air. This prevents spraying of the dye and maximizes the concentration of the dye at the site of the lesion. Utilization of dye marking may help the surgeon when performing segmentectomies to confirm appropriate anatomic location as well as ensuring adequate margin (48). Thereafter, the optical probe is then inserted again through the catheter to confirm adequate hemostasis. The robotic bronchoscope is then withdrawn and undocked from the ETT.
Mediastinal staging, if applicable, is then performed, usually with EBUS. ROSE or intraoperative cytology interpretation then confirms the absence or presence of metastatic malignant disease. If the nodule biopsy confirms malignancy and mediastinal staging examination is negative for N2 metastatic disease, the patient in the same setting undergoes RATS for surgical treatment of their lung cancer.
Once the RAB portion is done, the single lumen ETT is exchanged for a double lumen ETT, and the patient is positioned in the lateral decubitus position in preparation for the surgical resection with the da Vinci Surgical. The operative lung is isolated, and the robotic ports are placed. Intraoperatively, the firefly function can be used to locate the lesion, which has been marked.
The combination of both the diagnostic biopsy and resection into a single event offers multiple benefits for patients and surgeons. The ability to place dye to mark the lesion can certainly make visualization of the lesion easier in the subsequent resection. This is particularly important with RATS where the surgeon does not have the tactile feedback of lesions that would be available in a traditional video-assisted thoracoscopic surgery (VATS) or thoracotomy that can help with target lesion localization and instead has to rely solely on visual cues. Furthermore, for ground-glass predominant lesions, tumor palpation can still be difficult, and preoperative localization may help. The dye or fiducial marker from the preceding bronchoscopy can help the surgeon ensure the specimen contains both the target lesion and adequate margins. Similarly, the lesion marking can aid with intra-operative decision making regarding the extent of the resection.
Beyond the technical advantages of the single-setting procedures, the decrease in time interval between biopsy proven diagnosis and resection also has tangible benefits for patients. Increases in time between initial biopsy and subsequent resection have been associated with higher rates of pathologic upstaging and decreased cancer-specific and overall survival (2, 49, 50). Obtaining tissue diagnosis and subsequent definitive resection immediately eliminates any delay in treatment. For patients who live remotely with extensive travel to referral centers with thoracic surgeons or who have other barriers to accessing care, a single episode of care with both procedures certainly can ease the burden on these patients. A single anesthesia event can also be safer for patients with other comorbidities that carry risk with repeated anesthetic induction. Eliminating the time between biopsy and surgery also lessens the natural anxiety associated with carrying a new cancer diagnosis and an impending surgery.
As the combined RAB/RATS in one setting is a new approach, there is paucity of literature on various clinical and financial outcomes. Study by Palleiko et al. compared their experience of 36 combined RAB/RATS cohort to 35 who underwent standard workup and provides insights into some of the important clinical as well as financial outcomes (51). In their experience, the combined approach added 73 minutes to lobectomies although when looking at total operating room time, the combined approach was 54 minutes shorter (459 minutes for combined vs 513 minutes for standard). Median hospital stay was similar (3 days) as well as postoperative complication rates between the two groups. In addition, the combined approach resulted in lower direct and indirect costs compared to the traditional workup when comparing the lobectomy cohort. Wong et al. reported their series of 15 combined RAB/RATS approach. While they do not compare their cohort to any control, their study provides insight into time in the OR – median of 284 minutes for RAB plus RATS and 355 minutes when EBUS was added (52). For long term oncologic outcomes, we will have to await for future studies to determine if the combined approach is different from standard.
Lung cancer patients depend on timely diagnosis and treatment. Beyond poorer oncologic outcomes, heavy emotional tolls, increased utilization of health care resources, and increased overall cost of care to patients and the system are all deleterious outcomes from delays in diagnosis and treatment (53). Timeliness is a vital part of delivering quality oncologic care, and it has been named as one of the six dimensions of health care quality by The Institute of Medicine’s Committee on Quality Health Care in America (54). Reflecting this for the treatment of lung cancer specifically, the RAND Corporation (following guidelines by the British Thoracic Society) targeted timeliness for the care of lung cancer (55). These recommendations advocate that lung cancer diagnoses should be established within 2 months after initial abnormal imaging and treatment offered within 6 weeks after diagnosis (55). Subsequently, updated guidelines were proposed in the United Kingdom’s National Optimal Lung Cancer Pathway which set maximum waiting times of 14 days for diagnosis and 28 days for treatment (56). Jacobsen et al. published a comprehensive systematic review on timeliness of lung cancer care and reported a median wait time from PCP referral to specialist consultation of 1 to 17 days, range median delay from diagnosis to treatment of 6 to 45 days, and estimated 15-63% of patients not receiving treatment within 31 days of diagnosis (Figure 3) (57). Authors cite within the constituent studies various barriers to care such as numerous procedures, necessary repetitive procedures, poor appointment availability for specialist visits or procedures, and delays due to physicians not initially suspecting cancer (57). These may reflect broader issues across the field with fragmentation of care, sub-specialization, and poor coordination between providers.
Multidisciplinary evaluation and subsequent treatment offer a potential solution that combines both to timely diagnosis and optimal treatment (57, 58). This multidisciplinary approach is reflected by single setting anesthesia events which offer robotic lung nodule diagnosis with immediate RATS. Similarly to how multidisciplinary clinics can eliminate gaps between evaluations by various specialists, a single setting event can eliminate gaps between tissue diagnosis and necessary resection. The use of robotic assisted procedures has increased dramatically over the last decade despite associated costs. Data are continually emerging that robotic assisted procedures may offer improved lung cancer diagnostic capability and surgical outcomes relative to traditional guided bronchoscopy and VATS (24, 29, 30, 59–63). Close coordination of these technologies offers an integration of the diagnosis, staging, and resection of lung cancer. Multiple ongoing studies support the decreasing time from diagnosis to treatment (64, 65). The following are proposed advantages for the single anesthetic pathway to lung cancer care:
● Shortened timeline from nodule identification to surgery.
● Minimizing anxiety interval between diagnosis and resection.
● Potential reduction in risks associated with multiple anesthetic events.
● Enhanced target lesion identification and visualization in RATS to help with sublobar resections.
● Decreased impact on family resources.
● Earlier return to usual lifestyle.
● Possible decreased cost in lung cancer care without compromising oncologic standards.
Clinicians should target diagnostic and treatment modalities for that maximize accuracy and timeliness while minimizing cost and risk. A multidisciplinary approach in diagnosing and treating malignancy can help provide this optimal pathway. Single setting event robotic-assisted lung nodule diagnosis and resection offers a valuable new tool and strategy in the pursuit of improving the multidisciplinary treatment of lung cancer.
PP: Conceptualization, Writing – original draft, Writing – review & editing. AP: Writing – original draft, Writing – review & editing. BZ: Writing – original draft, Writing – review & editing. KS: Conceptualization, Supervision, Validation, Writing – original draft, Writing – review & editing.
The author(s) declare that no financial support was received for the research and/or publication of this article.
The authors declare that the research was conducted in the absence of any commercial or financial relationships that could be construed as a potential conflict of interest.
The author(s) declare that no Generative AI was used in the creation of this manuscript.
All claims expressed in this article are solely those of the authors and do not necessarily represent those of their affiliated organizations, or those of the publisher, the editors and the reviewers. Any product that may be evaluated in this article, or claim that may be made by its manufacturer, is not guaranteed or endorsed by the publisher.
1. American Cancer Society. Lung cancer survival rates | 5-Year survival rates for lung cancer (2024). Available online at: https://www.cancer.org/cancer/types/lung-cancer/detection-diagnosis-staging/survival-rates.html (Accessed Dec 12, 2024).
2. Samson P, Patel A, Garrett T, Crabtree T, Kreisel D, Krupnick AS, et al. Effects of delayed surgical resection on short-term and long-term outcomes in clinical stage I non-small cell lung cancer. Ann Thorac Surg. (2015) 99:1906–1912; discussion 1913. doi: 10.1016/j.athoracsur.2015.02.022
3. Oudkerk M, Devaraj A, Vliegenthart R, Henzler T, Prosch H, Heussel CP, et al. European position statement on lung cancer screening. Lancet Oncol. (2017) 18:e754–66. doi: 10.1016/S1470-2045(17)30861-6
4. Gildea TR, DaCosta Byfield S, Hogarth DK, Wilson DS, Quinn CC. A retrospective analysis of delays in the diagnosis of lung cancer and associated costs. Clinicoecon Outcomes Res. (2017) 9:261–9. doi: 10.2147/CEOR.S132259
5. Patel PP, Duong DK, Mahajan AK, Imai TA. Single setting robotic lung nodule diagnosis and resection. Thorac Surg Clin. (2023) 33:233–44. doi: 10.1016/j.thorsurg.2023.04.009
6. Gould MK, Donington J, Lynch WR, Mazzone PJ, Midthun DE, Naidich DP, et al. Evaluation of individuals with pulmonary nodules: when is it lung cancer? Diagnosis and management of lung cancer, 3rd ed: American College of Chest Physicians evidence-based clinical practice guidelines. Chest. (2013) 143:e93S–e120S. doi: 10.1378/chest.12-2351
7. Ettinger DS, Wood DE, Aisner DL, Akerley W, Bauman JR, Bharat A, et al. NCCN guidelines insights: non-small cell lung cancer, version 2.2021. J Natl Compr Canc Netw. (2021) 19:254–66.
8. Panchabhai TS, Mehta AC. Historical perspectives of bronchoscopy. Connecting the dots. Ann Am Thorac Soc. (2015) 12:631–41. doi: 10.1513/AnnalsATS.201502-089PS
9. Oki M, Saka H, Ando M, Asano F, Kurimoto N, Morita K, et al. Ultrathin bronchoscopy with multimodal devices for peripheral pulmonary lesions. A randomized trial. Am J Respir Crit Care Med. (2015) 192:468–76. doi: 10.1164/rccm.201502-0205OC
10. Folch EE, Pritchett MA, Nead MA, Bowling MR, Murgu SD, Krimsky WS, et al. Electromagnetic navigation bronchoscopy for peripheral pulmonary lesions: one-year results of the prospective, multicenter NAVIGATE study. J Thorac Oncol. (2019) 14:445–58. doi: 10.1016/j.jtho.2018.11.013
11. Gildea TR, Mazzone PJ, Karnak D, Meziane M, Mehta AC. Electromagnetic navigation diagnostic bronchoscopy: a prospective study. Am J Respir Crit Care Med. (2006) 174:982–9. doi: 10.1164/rccm.200603-344OC
12. Ost DE, Ernst A, Lei X, Kovitz KL, Benzaquen S, Diaz-Mendoza J, et al. Diagnostic yield and complications of bronchoscopy for peripheral lung lesions. Results of the AQuIRE registry. Am J Respir Crit Care Med. (2016) 193:68–77. doi: 10.1164/rccm.201507-1332OC
13. Silvestri GA, Bevill BT, Huang J, Brooks M, Choi Y, Kennedy G, et al. An evaluation of diagnostic yield from bronchoscopy: the impact of clinical/radiographic factors, procedure type, and degree of suspicion for cancer. Chest. (2020) 157:1656–64. doi: 10.1016/j.chest.2019.12.024
14. Tanner NT, Yarmus L, Chen A, Wang Memoli J, Mehta HJ, Pastis NJ, et al. Standard bronchoscopy with fluoroscopy vs thin bronchoscopy and radial endobronchial ultrasound for biopsy of pulmonary lesions: A multicenter, prospective, randomized trial. Chest. (2018) 154:1035–43. doi: 10.1016/j.chest.2018.08.1026
15. Asano F, Shinagawa N, Ishida T, Shindoh J, Anzai M, Tsuzuku A, et al. Virtual bronchoscopic navigation combined with ultrathin bronchoscopy. A randomized clinical trial. Am J Respir Crit Care Med. (2013) 188:327–33. doi: 10.1164/rccm.201211-2104OC
16. Eberhardt R, Anantham D, Ernst A, Feller-Kopman D, Herth F. Multimodality bronchoscopic diagnosis of peripheral lung lesions: a randomized controlled trial. Am J Respir Crit Care Med. (2007) 176:36–41. doi: 10.1164/rccm.200612-1866OC
17. Bhatt KM, Tandon YK, Graham R, Lau CT, Lempel JK, Azok JT, et al. Electromagnetic navigational bronchoscopy versus CT-guided percutaneous sampling of peripheral indeterminate pulmonary nodules: A cohort study. Radiology. (2018) 286:1052–61. doi: 10.1148/radiol.2017170893
18. DiBardino DM, Yarmus LB, Semaan RW. Transthoracic needle biopsy of the lung. J Thorac Dis. (2015) 7:304.
19. 510(k) Premarket Notification- Ion Endoluminal System (2024). Available online at: https://www.accessdata.fda.gov/scripts/cdrh/cfdocs/cfpmn/pmn.cfm?ID=K192367 (Accessed Dec 12, 2024).
20. 510(k) Premarket Notification- Monarch Endoscopy Platform (Monarch Platform) (2024). Available online at: https://www.accessdata.fda.gov/scripts/cdrh/cfdocs/cfpmn/pmn.cfm?ID=k173760 (Accessed Dec 12, 2024).
21. Galloway KC, Chen Y, Templeton E, Rife B, Godage IS, Barth EJ. Fiber optic shape sensing for soft robotics. Soft Robot. (2019) 6:671–84. doi: 10.1089/soro.2018.0131
22. Poeggel S, Tosi D, Duraibabu D, Leen G, McGrath D, Lewis E. Optical fibre pressure sensors in medical applications. Sensors (Basel). (2015) 15:17115–48. doi: 10.3390/s150717115
23. Rojas-Solano JR, Ugalde-Gamboa L, Machuzak M. Robotic bronchoscopy for diagnosis of suspected lung cancer: A feasibility study. J Bronchology Interv Pulmonol. (2018) 25:168–75. doi: 10.1097/LBR.0000000000000499
24. Chen AC, Pastis NJ, Mahajan AK, Khandhar SJ, Simoff MJ, Machuzak MS, et al. Robotic bronchoscopy for peripheral pulmonary lesions: A multicenter pilot and feasibility study (BENEFIT). Chest. (2021) 159:845–52. doi: 10.1016/j.chest.2020.08.2047
25. Chen AC, Gillespie CT. Robotic endoscopic airway challenge: REACH assessment. Ann Thorac Surg. (2018) 106:293–7. doi: 10.1016/j.athoracsur.2018.01.051
26. Yarmus L, Akulian J, Wahidi M, Chen A, Steltz JP, Solomon SL, et al. A prospective randomized comparative study of three guided bronchoscopic approaches for investigating pulmonary nodules: the PRECISION-1 study. Chest. (2020) 157:694–701. doi: 10.1016/j.chest.2019.10.016
27. Kapp CM, Akulian JA, Yu DH, Chen A, Cárdenas-García J, Molena D, et al. Cognitive load in electromagnetic navigational and robotic bronchoscopy for pulmonary nodules. ATS Sch. (2020) 2:97–107. doi: 10.34197/ats-scholar.2020-0033OC
28. Fielding DIK, Bashirzadeh F, Son JH, Todman M, Chin A, Tan L, et al. First human use of a new robotic-assisted fiber optic sensing navigation system for small peripheral pulmonary nodules. Respiration. (2019) 98:142–50. doi: 10.1159/000498951
29. Chaddha U, Kovacs SP, Manley C, Hogarth DK, Cumbo-Nacheli G, Bhavani SV, et al. Robot-assisted bronchoscopy for pulmonary lesion diagnosis: results from the initial multicenter experience. BMC Pulm Med. (2019) 19:243. doi: 10.1186/s12890-019-1010-8
30. Kalchiem-Dekel O, Connolly JG, Lin I-, Husta BC, Adusumilli PS, Beattie JA, et al. Shape-sensing robotic-assisted bronchoscopy in the diagnosis of pulmonary parenchymal lesions. Chest. (2022) 161:572–82. doi: 10.1016/j.chest.2021.07.2169
31. Benn BS, Romero AO, Lum M, Krishna G. Robotic-assisted navigation bronchoscopy as a paradigm shift in peripheral lung access. Lung. (2021) 199:177–86. doi: 10.1007/s00408-021-00421-1
32. Park CH, Han K, Hur J, Lee SM, Lee JW, Hwang SH, et al. Comparative effectiveness and safety of preoperative lung localization for pulmonary nodules: A systematic review and meta-analysis. Chest. (2017) 151:316–28. doi: 10.1016/j.chest.2016.09.017
33. Lizza N, Eucher P, Haxhe JP, De Wispelaere JF, Johnson PM, Delaunois L. Thoracoscopic resection of pulmonary nodules after computed tomographic-guided coil labeling. Ann Thorac Surg. (2001) 71:986–8. doi: 10.1016/S0003-4975(00)02505-4
34. Chen S, Zhou J, Zhang J, Hu H, Luo X, Zhang Y, et al. Video-assisted thoracoscopic solitary pulmonary nodule resection after CT-guided hookwire localization: 43 cases report and literature review. Surg Endosc. (2011) 25:1723–9. doi: 10.1007/s00464-010-1502-3
35. Doo KW, Yong HS, Kim HK, Kim S, Kang E, Choi YH. Needlescopic resection of small and superficial pulmonary nodule after computed tomographic fluoroscopy-guided dual localization with radiotracer and hookwire. Ann Surg Oncol. (2015) 22:331–7. doi: 10.1245/s10434-014-3884-2
36. Lin M, Tseng Y, Lee Y, Hsieh M, Ko W, Chen J, et al. Computed tomography-guided patent blue vital dye localization of pulmonary nodules in uniportal thoracoscopy. J Thorac Cardiovasc Surg. (2016) 152:535–544.e2. doi: 10.1016/j.jtcvs.2016.04.052
37. Yang Y, Li Z, Huang W, Zhuang J, Lin D, Zhong W, et al. Electromagnetic navigation bronchoscopic localization versus percutaneous CT-guided localization for thoracoscopic resection of small pulmonary nodules. Thorac Cancer. (2021) 12:468–74. doi: 10.1111/1759-7714.13775
38. Yanagiya M, Kawahara T, Ueda K, Yoshida D, Yamaguchi H, Sato M. A meta-analysis of preoperative bronchoscopic marking for pulmonary nodules. Eur J Cardiothorac Surg. (2020) 58:40–50. doi: 10.1093/ejcts/ezaa050
39. Roy-Chowdhuri S, Dacic S, Ghofrani M, Illei PB, Layfield LJ, Lee C, et al. Collection and handling of thoracic small biopsy and cytology specimens for ancillary studies: guideline from the college of American pathologists in collaboration with the American college of chest physicians, association for molecular pathology, American society of cytopathology, American thoracic society, pulmonary pathology society, papanicolaou society of cytopathology, society of interventional radiology, and society of thoracic radiology. Arch Pathol Lab Med. (2020). doi: 10.5858/arpa.2020-0119-CP
40. Oki M, Saka H, Kitagawa C, Kogure Y, Murata N, Adachi T, et al. Rapid on-site cytologic evaluation during endobronchial ultrasound-guided transbronchial needle aspiration for diagnosing lung cancer: a randomized study. Respiration. (2013) 85:486–92. doi: 10.1159/000346987
41. Guo H, Liu S, Guo J, Li B, Li W, Lu Z, et al. Rapid on-site evaluation during endobronchial ultrasound-guided transbronchial needle aspiration for the diagnosis of hilar and mediastinal lymphadenopathy in patients with lung cancer. Cancer Lett. (2016) 371:182–6. doi: 10.1016/j.canlet.2015.11.038
42. Madan K, Mohan A, Ayub II, Jain D, Hadda V, Khilnani GC, et al. Initial experience with endobronchial ultrasound-guided transbronchial needle aspiration (EBUS-TBNA) from a tuberculosis endemic population. J Bronchology Interv Pulmonol. (2014) 21:208–14. doi: 10.1097/LBR.0000000000000080
43. Griffin AC, Schwartz LE, Baloch ZW. Utility of on-site evaluation of endobronchial ultrasound-guided transbronchial needle aspiration specimens. Cytojournal. (2011) 8:20. doi: 10.4103/1742-6413.90081
44. Trisolini R, Cancellieri A, Tinelli C, de Biase D, Valentini I, Casadei G, et al. Randomized trial of endobronchial ultrasound-guided transbronchial needle aspiration with and without rapid on-site evaluation for lung cancer genotyping. Chest. (2015) 148:1430–7. doi: 10.1378/chest.15-0583
45. Hopkins E, Moffat D, Parkinson I, Robinson P, Jersmann H, Dougherty B, et al. Cell block samples from endobronchial ultrasound transbronchial needle aspiration provide sufficient material for ancillary testing in lung cancer-a quaternary referral centre experience. J Thorac Dis. (2016) 8:2544–50. doi: 10.21037/jtd.2016.08.74
46. Altorki N, Wang X, Kozono D, Watt C, Landrenau R, Wigle D, et al. Lobar or sublobar resection for peripheral stage IA non-small-cell lung cancer. N Engl J Med. (2023) 388:489–98. doi: 10.1056/NEJMoa2212083
47. Saji H, Okada M, Tsuboi M, Nakajima R, Suzuki K, Aokage K, et al. Segmentectomy versus lobectomy in small-sized peripheral non-small-cell lung cancer (JCOG0802/WJOG4607L): a multicentre, open-label, phase 3, randomised, controlled, non-inferiority trial. Lancet. (2022) 399:1607–17. doi: 10.1016/S0140-6736(21)02333-3
48. Geraci TC, Ferrari-Light D, Kent A, Michaud G, Zervos M, Pass HI, et al. Technique, outcomes with navigational bronchoscopy using indocyanine green for robotic segmentectomy. Ann Thorac Surg. (2019) 108:363–9. doi: 10.1016/j.athoracsur.2019.03.032
49. Zhang L, Hsieh M, Rennert L, Neroda P, Wu X, Hicks C, et al. Diagnosis-to-surgery interval and survival for different histologies of stage I–IIA lung cancer. Transl Lung Cancer Res. (2021) 10:3043–58. doi: 10.21037/tlcr-21-168
50. Tang A, Ahmad U, Raja S, Bribriesco AC, Sudarshan M, Rappaport J, et al. How much delay matters? How time to treatment impacts overall survival in early stage lung cancer. Ann Surg. (2023) 277:e941–7. doi: 10.1097/SLA.0000000000005307
51. Pallieko BA, Maxfield MW, Sood RN, Bai S, Fischer A, Uy K, et al. Robotic one anesthetic diagnosis and treatment: A novel program for lung cancer care. Ann Thorac Surg Short Rep. (2024).
52. Wong AW, Wang EY, Fong A, Pyarali F, Ideta TR, Tokeshi B, et al. Streamlining care with single anesthetic robotic lung cancer diagnosis and resection. Curr Challenges Thorac Surg. (2024) 6. doi: 10.21037/ccts-23-14
53. Kern KA. Medicolegal analysis of the delayed diagnosis of cancer in 338 cases in the United States. Arch Surg. (1994) 129:397–404. doi: 10.1001/archsurg.1994.01420280073009
54. Institute of Medicine (US) Committee on Quality of Health Care in America. Crossing the Quality Chasm: A New Health System for the 21st Century. Washington (DC: National Academies Press (US (2001).
55. Asch SM, Kerr EA, Hamilton EG, Reifel JL, McGlynn EA. Quality of Care for Oncologic Conditions and HIV: A Review of the Literature and Quality Indicators. Santa Monica, CA, USA: RAND (2000).
56. Field JK, deKoning H, Oudkerk M, Anwar S, Mulshine J, Pastorino U, et al. Implementation of lung cancer screening in Europe: challenges and potential solutions: summary of a multidisciplinary roundtable discussion. ESMO Open. (2019) 4:e000577. doi: 10.1136/esmoopen-2019-000577
57. Jacobsen MM, Silverstein SC, Quinn M, Waterston LB, Thomas CA, Benneyan JC, et al. Timeliness of access to lung cancer diagnosis and treatment: A scoping literature review. Lung Cancer. (2017) 112:156–64. doi: 10.1016/j.lungcan.2017.08.011
58. Stone CJL, Vaid HM, Selvam R, Ashworth A, Robinson A, Digby GC. Multidisciplinary clinics in lung cancer care: A systematic review. Clin Lung Cancer. (2018) 19:323–30.e3. doi: 10.1016/j.cllc.2018.02.001
59. Kneuertz PJ, Singer E, D’Souza DM, Abdel-Rasoul M, Moffatt-Bruce SD, Merritt RE. Hospital cost and clinical effectiveness of robotic-assisted versus video-assisted thoracoscopic and open lobectomy: A propensity score-weighted comparison. J Thorac Cardiovasc Surg. (2019) 157:2018–26.e2. doi: 10.1016/j.jtcvs.2018.12.101
60. Deen SA, Wilson JL, Wilshire CL, Vallières E, Farivar AS, Aye RW, et al. Defining the cost of care for lobectomy and segmentectomy: a comparison of open, video-assisted thoracoscopic, and robotic approaches. Ann Thorac Surg. (2014) 97:1000–7. doi: 10.1016/j.athoracsur.2013.11.021
61. Merritt RE, D’Souza DM, Abdel-Rasoul M, Kneuertz PJ. Analysis of trends in perioperative outcomes in over 1000 robotic-assisted anatomic lung resections. J Robot Surg. (2023) 17:435–45. doi: 10.1007/s11701-022-01436-3
62. Arnold BN, Thomas DC, Narayan R, Blasberg JD, Detterbeck FC, Boffa DJ, et al. Robotic-assisted lobectomies in the national cancer database. J Am Coll Surg. (2018) 226:1052–62.e15. doi: 10.1016/j.jamcollsurg.2018.03.023
63. Ross P, Skabla P, Sutter JB, Whealon ST, Ibrahim K, Meyer TJ. Optimizing the resection pathway: can minimally invasive diagnosis and surgery (Midas) guide patient selection? Chest. (2022) 162:A1615. doi: 10.1016/j.chest.2022.08.1354
64. Ross P, Skabla P, Sutter J, Meyer T. Fast track nodule pathway: same day robotic diagnosis and resection. Chest. (2021) 160:A82. doi: 10.1016/j.chest.2021.07.112
Keywords: bronchoscopy, lung cancer, lobectomy, lung nodule, resection, robotic
Citation: Patel PP, Patel A, Zollinger B and Suzuki K (2025) Robotic-assisted lung nodule diagnosis and resection. Front. Oncol. 15:1555151. doi: 10.3389/fonc.2025.1555151
Received: 03 January 2025; Accepted: 28 February 2025;
Published: 21 March 2025.
Edited by:
Elliot Servais, Lahey Hospital and Medical Center, United StatesReviewed by:
Hansheng Wu, First Affiliated Hospital of Shantou University Medical College, ChinaCopyright © 2025 Patel, Patel, Zollinger and Suzuki. This is an open-access article distributed under the terms of the Creative Commons Attribution License (CC BY). The use, distribution or reproduction in other forums is permitted, provided the original author(s) and the copyright owner(s) are credited and that the original publication in this journal is cited, in accordance with accepted academic practice. No use, distribution or reproduction is permitted which does not comply with these terms.
*Correspondence: Kei Suzuki, a2VpLnN1enVraUBpbm92YS5vcmc=
Disclaimer: All claims expressed in this article are solely those of the authors and do not necessarily represent those of their affiliated organizations, or those of the publisher, the editors and the reviewers. Any product that may be evaluated in this article or claim that may be made by its manufacturer is not guaranteed or endorsed by the publisher.
Research integrity at Frontiers
Learn more about the work of our research integrity team to safeguard the quality of each article we publish.