- 1College of Life Science, North China University of Science and Technology, Tangshan, China
- 2Jiangsu Province Engineering Research Center of Molecular Target Therapy and Companion Diagnostics in Oncology, Suzhou Vocational Health College, Suzhou, China
- 3Department of General Surgery, The First Affiliated Hospital of Soochow University, Suzhou, China
This review summarizes the applications and research progress of organoid models in colorectal cancer research. First, the high incidence and mortality rates of colorectal cancer are introduced, emphasizing the importance of organoids as a research model. Second, this review provides a detailed introduction to the concept, biological properties, and applications of organoids, including their strengths in mimicking the structural and functional aspects of organs. This article further analyzes the applications of adult stem cell-derived and pluripotent stem cell-derived organoids in colorectal cancer research and discusses advancements in organoids for basic research, drug research and development, personalized treatment evaluation and prediction, and regenerative medicine. Finally, this review summarizes the prospects for applying organoid technology in colorectal cancer research, emphasizing its significant value in improving patient survival rates. In conclusion, this review systematically explains the applications of organoids in colorectal cancer research, highlighting their tremendous potential and promising prospects in basic research, drug research and development, personalized treatment evaluation and prediction, and regenerative medicine.
Introduction
Colorectal cancer (CRC) (1) is one of the most severe diseases and is a secondary cause of cancer-related death worldwide (2). Fifty-six percent of patients with CRC die from this disease (3), and for those with advanced metastatic colorectal cancer, the survival rate is only 4% to 12% (4). Compared with healthy colorectal cells, colorectal cancer cells undergo significant and frequent somatic mutations, and the genetic diversity of the cancer leads to prominent biological heterogeneity (5). Although the current preclinical study model, which includes cell lines, animal models, and clinical samples, has been used to contribute to the understanding of CRC, it still has certain limitations (6). To address these limitations, the organoid model provides a more advanced scientific tool (7).
The organoid model is an in vitro cultured three-dimensional tissue structure that mimics the microarchitecture of organs in vivo. Models can be derived from primary tissue samples, embryonic stem cells, somatic stem cells, or pluripotent stem cells (8, 9). Organoids contain many organ-specific cell types and exhibit spatial organization arrangements similar to those of their in vivo counterparts. This structural property endows organoids with a significant advantage in mimicking organ function. Organoids are considered advanced in vitro cancer models that can efficiently recapitulate the tumor microenvironment and maintain the heterogeneity of cell populations (10, 11).
In 2009, Sato, T, and colleagues first confirmed that single Lgr5+ intestinal stem cells (ASCs) are capable of self-organizing and differentiating to form crypt–villus structures that encompass all intestinal cell types (12). This discovery effectively replaced traditional cell lines and animal models; although this study has led to a breakthrough in stem cell research, the understanding of stem cells is still limited, and more research is needed to gain a deeper understanding of their differentiation mechanisms and regulatory networks (13, 14). As effective models for colorectal cancer research, organoids exhibit complex three-dimensional structures, cell heterogeneity, self-renewal, and self-organization (15). The transplanted organ plays a protective role by regulating the self-renewal of intestinal stem cells and modulating the immune microenvironment in recipient mice. For example, Fang-Ling Zhang’s research team reported that intestinal organ transplantation, as a therapeutic strategy, attenuates intestinal I/R injury in mice by promoting the self-renewal of intestinal stem cells and modulating the immune microenvironment. L-Malic acid (MA)-mediated polarization of M2 macrophages is dependent on SOCS2, a finding that provides a new understanding for the treatment of intestinal I/R injury (16).
To date, organoid models featuring diverse pathological characteristics, such as genetic diseases (17, 18), host− interactions (18), cancer (19, 20), intestinal hyperplastic polyps (21, 22) and gastrointestinal metaplasia (23), have been successfully developed, which further highlights the effectiveness of organoids in reflecting the biological characteristics of the colorectum and aids in deepening our understanding of the mechanisms underlying colorectal disease development. This also validates the methods used to develop these models, which are fundamentally capable of demonstrating high fidelity and genetic consistency (24).
Types of organoids
The biological properties of organoids derived from adult stem cells
Adult stem cells (ASCs) are a class of cells that possess the capacity for self-renewal and multidirectional differentiation, with the primary function of facilitating tissue repair and regeneration within the body (45). These cells are capable of differentiating into a variety of cell types. These compounds have demonstrated significant therapeutic effects in clinical treatment. It has become the gold standard in the field of stem cell research and therapy because of its minimal ethical controversy, broad acceptance, and successful application in treating patients.
Organoids derived from ASCs can regenerate in vitro, and they are primarily responsible for maintaining and repairing tissue functions (46, 47), which are closely associated with the development of colorectal cancer and have mutations and aberrant differentiation that may lead to tumor formation. According to current scientific research, colorectal organoids are capable of replicating the physiological state or degenerative conditions of the original tissue (48). FGFBP1 is overexpressed in colon, pancreas, breast, and skin cancers, and its expression accelerates skin wound healing in mice with conditional overexpression. Therefore, Fgfbp1 functions as a soluble autocrine/paracrine factor that mediates cross-talk between the epithelial stem/progenitor compartment and the niche, thereby reinforcing Fgfbp1+ cell identity and self-renewal (49). For example, Claudia Capdevila’s research team has recently achieved significant progress in the use of intestinal epithelial organoids. These findings indicate that FGFBP1-labeled pluripotent stem cells are capable of generating Lgr5+ cells, which can maintain the regenerative capacity of the intestinal epithelium when Lgr5+ cells are depleted (5). This research has challenged the traditional understanding of intestinal stem cells and revealed the important role of Fgfbp1+ cells in intestinal epithelial regeneration.
In a previous study, Johanna F Dekkers and colleagues employed biopsied intestinal organoids to investigate a disease resulting from a mutation in CFTR (50). However, organoid models at that time could not fully replicate the intricacies of cancer development, including tissue structure, cellular diversity and in vivo homeostasis. Consequently, the study of tumorigenesis in vitro is challenging, and the use of alternative models would incur significant costs. With the advent of organoids, many studies have been conducted to address this gap. Most recently, L. F. Lorenzo-Martín’s research team developed a topologically and biologically complex mini-colon organoid. It can be guided to cancer by blue light irradiation-activated spatiotemporally controlled tumorigenic transformation and can track neoplastic colon tumors in real time for weeks at single-cell resolution without destroying the organoid. The induced mini-colonic organoids demonstrate rich intratumoral and intertumoral diversity, as well as the physiological features characteristic of key pathologies of colorectal tumors in vivo (51). This study effectively bridges the gap between tumor formation in vitro and provides a novel approach to cancer initiation, colorectal cancer lesions, and mechanisms of development in living organisms in vitro (52).
The initial materials for isolating adult and embryonic stem cells were patient-derived tumor tissues and mouse models. The final stage of the process results in the formation of organoids, which represent the primary end product of this research. Organoids are prominent in several application areas, including but not limited to, basic biological research, drug toxicity assessment, the construction of disease models, the creation of organoid biobanks, and the development of organoid microarray technology, which are also expected to have positive impacts on human health and the advancement of science. Organoid research is being used to mimic human disease states, which will provide an in-depth understanding of disease mechanisms.
The functional properties of organoids derived from pluripotent stem cells
It is widely acknowledged that pluripotent stem cells (Figure 1) are capable of differentiating into cell types derived from the three embryonic germ layers (53, 54). These cells possess significant developmental potential and are acquired through both embryonic stem cells (ESCs) and induced pluripotent stem cells obtained through reprogramming (55) (Figure 1). The reprogramming of somatic cells to pluripotent stem cells can be achieved through two main strategies: the transduction of specific combinations of transcription factors [e.g., SOX2 (56)] and the application of small molecule compounds to induce pluripotency. The potential applications and research value of pluripotent stem cell technology are extensive, spanning the fields of pathophysiology modeling, drug research and development, and regenerative medicine (57).
Researchers have successfully differentiated human colonic organoids from pluripotent stem cells, which closely resemble the human colon in both structure and function. This provides a powerful in vitro model for the study of colorectal diseases (58). For example, Xiaobo Zheng’s research team discovered that the overexpression of BMX and HCK markedly enhanced the proliferation of colorectal epithelial cells. Further studies demonstrated that the upregulation of BMX and HCK activated the JAK-STAT signaling pathway, resulting in the formation of multilayered polypoid structures that mimic the pathologic polyps commonly found in colorectal cancers (59). These findings provide a theoretical foundation for the early prevention and development of new therapies for CRC epithelial cell transformation. In the field of human colorectal organoid research, pluripotent stem cells can be differentiated into cell types with specific functions through the guidance of specific factors (60). For example, the results of Pilar Bustamante-Madrid and colleagues suggested that the BMP and Notch signaling pathways play pivotal roles in directing the differentiation of human colonic stem cells toward enterocyte and goblet cells. Furthermore, the modulation of these processes by calcitriol has also been demonstrated to contribute to the maintenance of stemness traits (61). Organoids contribute to understanding the role of factors and signals in the human colonic epithelium, which is relevant to the study of intestinal pathologies, including colon cancer and inflammatory bowel disease (ulcerative colitis and Crohn’s disease). Organoids can mimic not only the process of human intestinal development but also key cell types and structural features present during intestinal development. For example, Na Qu and colleagues constructed human colonic organoids (HCOs) and human intestinal organoids (HIOs) via human pluripotent stem cells. The removal of BMP signaling or the addition of the inhibitor NOGGIN facilitated the formation of organs resembling the developing small intestine and colon, as well as primary epithelial and mesenchymal cells within the colon (62). These results elucidate the mechanisms of intestinal regional specification and provide a powerful tool for studying intestinal development, disease modeling, and drug screening.
The versatile applications of organoids
Basic research: the applications of organoids in CRC
By 2024, the number of articles related to organoids indexed on PubMed will have reached 26,227, with the number of publications increasing rapidly, making organoid research a burgeoning field of study, particularly in the area of single-cell transcriptomic sequencing (63) integrated with organoid research. In colorectal cancer research, the use of organoids has greatly advanced our understanding of the pathogenic mechanisms underlying this disease and has become a widely used tool in exploratory studies of its onset and progression (64) (Figure 2A). For example, Fengjiao Li and colleagues developed CRC organoids and subsequently extracted RNA for transcriptomic analysis, which revealed a significant correlation between the elevated expression of the ITGB7 and ITGA2B genes and sodium butyrate-induced apoptosis in these organoids. Additionally, sodium butyrate may induce cell cycle arrest and subsequent apoptosis by activating the PI3K−Akt signaling pathway (65). The utilization of organoids is also applicable to disease modeling and simulation. For example, Hao Zheng and colleagues established a colorectal cancer organoid biobank and demonstrated the induction of drug resistance following repeated exposure to low-dose chemotherapeutic agents. On this basis, the team developed a specific monoclonal antibody (LGR4-mAb) that can precisely block the LGR4-Wnt signaling pathway. Further studies demonstrated that LGR4-mAb not only effectively inhibited LGR4-Wnt signaling but also significantly enhanced drug-induced iron-induced apoptosis. The combination of LGR4-mAb with chemotherapeutic agents targeting the Wnt signaling pathway resulted in a notable increase in iron apoptosis in the organoids. This study illustrates the potential benefits of antibodies in CRC therapy. However, further studies are needed to analyze the heterogeneity of organoid responses to chemotherapeutic agents in greater depth (66). The use of organoids enables the discovery and validation of new therapeutic targets by accurately mimicking the microenvironment of human colorectal cancer in vitro. For example, Leon P. Loevenich’s research team discovered that NLE1 is capable of limiting the biosynthesis of ab initio proteins and the tumorigenic potential of advanced colorectal cancer cells (67). This significant outcome not only demonstrates the potential therapeutic efficacy of NLE1 but also provides a more precise targeting strategy to enhance the treatment of metastatic colorectal cancer in comparison with conventional approaches.
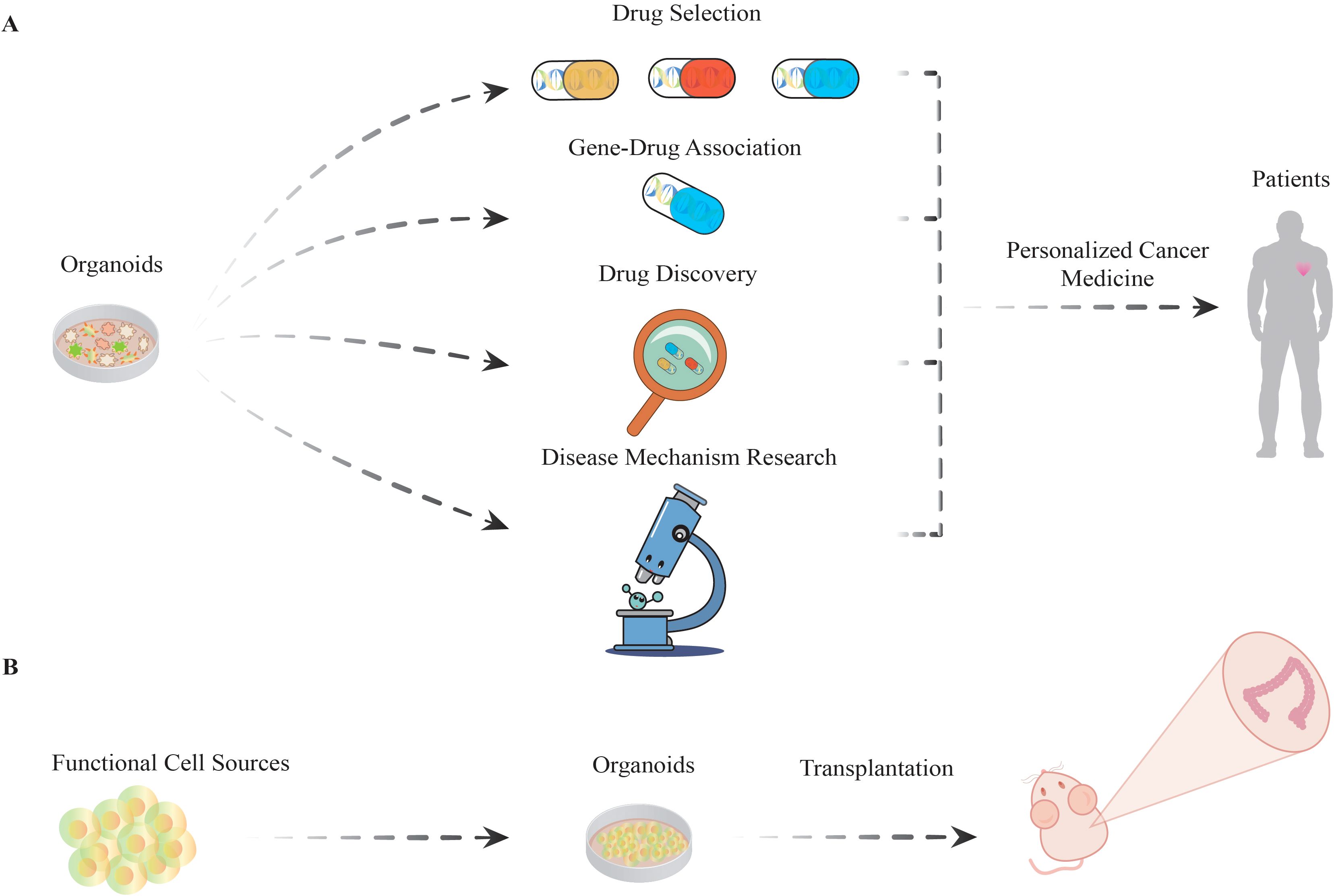
Figure 2. The versatile applications of organoids. (A) Organoids are employed to identify drugs with optimal efficacy among various drug candidates. Subsequently, drug−gene interactions are investigated to elucidate the correlation between specific gene mutations and drug responsiveness. On this basis, research will progress to the drug development phase, with in-depth analyses of disease mechanisms. Ultimately, the integration of cumulative research results will be used to construct personalized treatment protocols aimed at improving therapeutic efficacy and reducing the incidence of adverse effects, thereby increasing patient survival. (B) Organoids have the potential to be utilized in regenerative medicine as functional cell sources. This involves directing stem cells with multidirectional differentiation potential to develop into organoids, which are subsequently transplanted into mouse models to replicate and study functional and differentiation processes in living organisms.
In conclusion, in the investigation of molecular mechanisms and prospective therapeutic targets, the use of gene editing and modification techniques in organoids has been explored. It has been employed in several fields, including tumor mechanism research, genomics, and the construction of tumor animal models. Nevertheless, there are still some limitations to the use of colorectal cancer organoids in basic research. These include the nonspecificity of sample collection, the impact of time-point differences on study accuracy, the diversity of cohorts that may lead to an underestimation of the relevance of treatment response, the problems posed by the limited number of human-derived colorectal cancer organoids and small sample sizes, and the validity of the drug screening methodology, which needs to be further validated (68).
Drug research and development: the application of organoids in CRC
A further crucial application of organoid models is their capacity for high-throughput (69) drug screening in vitro (68). The introduction of specific compounds into organoids to simulate the influence of drugs on cellular proliferation, differentiation, and function allows the assessment of potential adverse effects of treatment (70, 71) (Figure 2A). The standardization and large-scale production of organoid technology still present significant challenges, which may impact its potential for a wide range of clinical applications. Multicenter clinical studies should be conducted to validate the efficacy of organoid drug screening and investigate its integration with immunological and targeted therapies, among other approaches, to achieve a more comprehensive cancer therapeutic effect (9, 72). Concurrently, a drug screening program utilizing organoids has been initiated. These organoids serve as a highly realistic platform for investigating the effects of drugs on tumor cells under conditions that closely approximate human physiological settings (73). For example, Zhongguang Luo’s research team established a biobank comprising 33 patients and 37 patient-derived high-risk colorectal adenoma organoids (HRCA-PDOs). A high-throughput, high-content HRCA drug screen of 139 compounds was subsequently conducted. The drugs metformin, BMS754807, panobinostat, and AT9283 were screened and identified as potentially effective treatments, and all demonstrated generally consistent inhibitory effects on HRCA-PDO (10). A high-throughput screen was recently conducted by Iram Fatima and colleagues utilizing an annotated library of 1,600 FDA-approved drugs. During the screening process, albendazole modulated RNF20 expression and promoted the apoptosis of colorectal cancer cells. This occurs by delaying the G2/M phase and inhibiting the antiapoptotic transcription of the Bcl2 family of proteins (74).
Organoids enable rapid assessment of the efficacy of multiple drugs against tumors and predict patient response to specific treatments. Organoids facilitate the study of tumor drug resistance mechanisms and the development of novel therapeutic drugs. The high cost of culturing and screening organoids requires specialized techniques and equipment, which limits their wide application in clinical practice. Currently, there are potential issues with the reproducibility of organoid screening results. The development of more efficient and economical organoid culture and screening methods is necessary to reduce costs and expand the range of organoid applications. Moreover, larger-scale clinical trials are needed to validate the value of organoid applications in clinical practice.
Predictive models: organoids for evaluating personalized treatment responses in CRC
In the conventional approach to oncology, the majority of patients typically undergo aggressive tumor resection following a cancer diagnosis (75). However, outcomes can vary significantly among individuals (76). As the understanding of the molecular heterogeneity of tumors and the pharmacogenomics of cancer therapies have advanced, the concept of tailored treatment strategies has become increasingly prominent in the field of therapeutic innovation (77, 78) (Figure 2A).
Nevertheless, organoid models provide an invaluable tool for predicting and assessing therapeutic outcomes, enabling researchers to elucidate the diverse sensitivities of cancer subtypes to a spectrum of interventions and to devise patient-specific treatment regimens (79). These promising approaches have the potential to not only increase treatment efficacy and minimize adverse effects but also significantly prolong patient survival. For example, S. N. Ooft and colleagues cultured colorectal organoids from biopsies and exposed them to eight different drugs, namely, vistusertib (an mTOR inhibitor) (80, 81), capivasertib (an AKT inhibitor) (82–84), and selumetinib (a MEK inhibitor) (85, 86). The objective of this study was to assess the antitumor activities of gefitinib (an EGFR inhibitor) (87, 88), palbociclib (a CDK4/6 inhibitor) (89, 90), axitinib (a VEGFR inhibitor) (91, 92), gedatolisib (a PI3K/mTOR inhibitor) (93), and glasdegib (a SMO inhibitor) (94). Nineteen patients exhibited sensitivity to at least one of the drugs, with 16 responding to mTOR inhibitors, 5 to AKT inhibitors, 3 to MEK inhibitors, 5 to EGFR inhibitors, and 2 to PI3K/mTOR inhibitors. Furthermore, no patients demonstrated sensitivity to CDK4/6 inhibitors, VEGFR inhibitors, or SMO inhibitors (95).
As organoid culture technology continues to be optimized, its potential for application as a preclinical predictive model has increased. Nevertheless, the limited drug screening activity and nonuniversal predictive ability of organoids, in addition to lower in vitro growth thresholds, may result in the persistence of tumor growth. It is essential to determine whether in vitro organoid sensitivity can be used to predict in vivo clinical response, as well as to improve culture success and the clinical efficacy of treatments. In light of the accelerated disease progression observed in patients, it is imperative to devote greater attention to the design of drug screening programs (96–98).
Regenerative medicine: novel tissue engineering materials and functional cell sources in CRC
Despite the considerable promise of organoid technology for modeling and regenerating human organs, significant challenges remain before it can be applied in a clinical setting. In particular, the majority of organoid culture systems continue to utilize animal-derived materials, such as matrix gels (99, 100), which limits the extensive range of potential applications within the human body and presents a significant challenge for clinical applications. Currently, the field of biomaterials is actively promoting research and development activities aimed at creating new materials with practical applications. Hyaluronic acid-gelatin hydrogels exhibit distinctive biocompatibilities (101). This represents a significant area of current research interest. For example, Xiaobei Luo’s research team encapsulated CRC PDOs in a three-dimensional hyaluronic acid-gelatin hydrogel and subsequently cocultured them with cancer-associated fibroblasts (CAFs). The hydrogels were found to maintain the key molecular features of the original patient tumors in the CRC PDO, as evidenced by RNA and whole exome sequencing. The initial findings indicated that the hydrogels were not conducive to the cultivation of CAFs. Nevertheless, a subsequent coculture strategy was developed to maintain the viability of both CRC PDOs and CAFs (102). The feasibility of extracting essential biochemical and mechanical characteristics without relying on biochemically undefined and mechanically invariant animal-derived matrices has been demonstrated. These findings may facilitate the development of frozen matrices with tissue-specific properties for the culture of other types of patient-derived tumor-like organs. Organoids are increasingly recognized as promising transplantation media and as a source of functional cells within the field of regenerative medicine for cellular therapies (Figure 2B) (103).
The feasibility of conducting proof-of-concept studies in animal models has been demonstrated by experimental evidence (104). A methodology for the generation of human organoid tissues with codeveloping resident immune cells has been developed, which can be employed to simulate inflammatory diseases and organize the developmental roles of resident immune populations. For example, Jorge O. Mu employed pluripotent stem cell cultures of human colonic organoids (HCOs) to successfully differentiate functional macrophages. The transcriptional properties of HCO macrophages are analogous to those observed in resident macrophages within human fetal gut tissue. Macrophages regulate cytokine secretion, respond to pro- and anti-inflammatory signals, and effectively phagocytose pathogenic bacteria. Following transplantation into mice, HCO macrophages remain stable in colonic organoid tissues and are firmly attached to epithelial cells, with no replacement by host macrophages (105). This approach demonstrated the capacity of HCO to generate pluripotent hematopoietic progenitors and functional tissue-resident macrophages and was also employed to evaluate the potential of the cells.
Organoids represent a significant advancement in regenerative medicine. Its high-throughput screening capability has led to the establishment of an efficient translational research platform for regenerative medicine. Research and application of organoids have the potential to facilitate tissue damage repair and organ regeneration. However, organoids face several limitations, including the inefficiency of transplantation and reliance on cancer models, transplantation site limitations, and cellular heterogeneity. It is important to standardize protocols for organoid transplantation to improve transplantation efficiency and experimental reproducibility; broaden the sources of organoids, including other tissues, organs and human-derived organoids, to meet diverse research needs; and establish a comprehensive system of functional assessments of organoids, including intestinal barriers and immune functions, to ensure their functionality (106).
Challenges and limitations: organoids in colorectal cancer research
At present, organoid models are confronted with many challenges and constraints. On the one hand, employing costly growth factors and animal-derived matrix extracts increases the financial burden associated with the cultivation process. Conversely, the clinical trial process necessitates the utilization of specialized equipment, consumables, and specific technical operations, which results in a markedly elevated cost in comparison to traditional 2D cell culture (Table 1). For example, Matrigel, a widely used product produced by BD Biosciences (107), is more expensive and holds a leading position in industry. Although colorectal cancer organoids can be constructed relatively quickly, scaling them up to a scale suitable for high-throughput drug screening may require an additional 2–4 weeks and resource investment compared with that of cell lines (108).
A further technical challenge for organoids is the development of a standardized and reproducible procedure that encompasses the maintenance, culture, cryopreservation, and processing of organoids. Because of the significant interpatient heterogeneity of organoids, it is important to optimize cell culture media to ensure cell survival and proliferation. In the development of culture protocols, it is essential to consider the impact of human factors, including operational errors and differences between laboratory equipment, which may have a significant impact on the reliability of experimental results. Furthermore, in high-throughput drug screening, a standardized seeding step is essential to ensure that the size of the organoid is relevant to its clinical context (109). Organoids lack the complexity of living organisms, which limits their use in multiple therapeutic assays (110). Additionally, heterogeneity in terms of tumor phenotypes, genotypes, and cellular composition is a crucial parameter (111). For example, Ning Li et al. revealed the advantages of the PDO in probing the functional interactions between colorectal cancer and the tumor microenvironment by mapping the cellular distribution of CRC patient samples and establishing a CRC tumor model in mice. Furthermore, a coculture model of CRC patient-derived organoids was employed (45), suggesting that PDOs can be utilized to explore the functional interactions between CRC and the tumor microenvironment.
The utilization of automated platforms has become increasingly prevalent in comparison with traditional organoid cultures, reducing the requisite time and human resources. However, the application of this technology is accompanied by several challenges, including inconsistent volume measurements due to differences in solution viscosity, sample contamination, and possible damage to the sample caused by improper pipetting, which can lead to the accumulation of data errors and reduced reproducibility of experiments (112). For example, Diana Pinho and colleagues developed a novel low-cost microfluidic device, the organoid chip, for the culture and amplification of colorectal cancer organoids. Compared with the traditional 24-well plate culture method, the organoid chip not only markedly increased the survival rate and proliferation activity of colorectal cancer organoids but also significantly improved the formation efficiency and overall size of the organoids (113).
In conclusion, the CRC organoid model offers a comprehensive understanding of tumor biology, tumor heterogeneity, and therapeutic response through the combination of coculture with multiple cell types and multiomics analyses (97). These findings are expected to increase the identification of novel drug targets and diagnostic biomarkers and provide support for in-depth probing of the mechanism of action of a drug or mechanisms of resistance, thereby facilitating more efficient clinical translation (39).
Future applications prospects
The field of organoid technology has undergone considerable advancements and evolution over the past few decades. This has involved overcoming numerous technical constraints, more rigorously assessing the fidelity of colorectal physiology, and demonstrating the practical utility of this technology. Researchers have sought to increase the fidelity of organoid physiology by optimizing culture formats and conditions (115). For example, organoid microarray technology (116) has been developed for high-throughput drug screening, whereas microfluidics has been employed for coculture (117). Inconsistencies in organoid characteristics have been observed across different culture protocols, emphasizing the urgent need to establish uniform criteria to define the characteristics of a true organoid (118).
The advent of new technologies has led to overcoming some of the earlier technical barriers, including those related to technical complexity and the necessity for experimental standardization (119). The experimental methods have now reached a point of maturity and are beginning to be translated into clinical applications. These developments are anticipated to facilitate a multitude of applications across a range of fields, including angiogenesis and coculture. For example, key domains such as organoid microarray technology, personalized precision medicine, drug screening and evaluation, biospecimen library construction, drug toxicity assessment, gene and cell therapies, regenerative medicine, and organ transplantation will undoubtedly benefit from these technological advancements (Table 2) (119, 120). As organoid technology continues to evolve, its applications in colorectal cancer research are anticipated to not only become more diverse but also significantly enhance our comprehension of the disease and provide invaluable assistance in improving patient survival rates (121).
Author contributions
YW: Data curation, Writing – original draft. YS: Data curation, Writing – original draft. XG: Data curation, Writing – original draft. LG: Data curation, Investigation, Writing – review & editing. JH: Data curation, Investigation, Writing – review & editing. S-BL: Data curation, Investigation, Writing – review & editing.
Funding
The author(s) declare financial support was received for the research, authorship, and/or publication of this article. This study was supported by the Jiangsu Higher Education Institution Innovative Research Team for Science and Technology (2021), the Natural Science Key Foundation of the Jiangsu Higher Education Institutions of China (Grant No. 24KJA310008), Program of Jiangsu Vocational College Engineering Technology Research Center (2023), Key Technology Program of Suzhou People’s Livelihood Technology Projects (Grant No. SKY2021029), Project of State Key Laboratory of Radiation Medicine and Protection, Soochow University (No. GZK12023013), Programs of the Suzhou Vocational Health College (Grant No. SZWZYTD202201, szwzy202304), and Qing‐Lan Project of Jiangsu Province in China (2021, 2022), Project of Jiangsu Province Engineering Research Center of Molecular Target Therapy and Companion Diagnostics in Oncology.
Conflict of interest
The authors declare that the research was conducted in the absence of any commercial or financial relationships that could be construed as a potential conflict of interest.
Publisher’s note
All claims expressed in this article are solely those of the authors and do not necessarily represent those of their affiliated organizations, or those of the publisher, the editors and the reviewers. Any product that may be evaluated in this article, or claim that may be made by its manufacturer, is not guaranteed or endorsed by the publisher.
References
1. Rosendahl Huber A, Pleguezuelos-Manzano C, Puschhof J, Ubels J, Boot C, Saftien A, et al. Improved detection of colibactin-induced mutations by genotoxic E. coli in organoids and colorectal cancer. Cancer Cell. (2024) 42:487–496.e486. doi: 10.1016/j.ccell.2024.02.009
2. Sung H, Ferlay J, Siegel RL, Laversanne M, Soerjomataram I, Jemal A, et al. Global cancer statistics 2020: GLOBOCAN estimates of incidence and mortality worldwide for 36 cancers in 185 countries. CA: A Cancer J Clin. (2021) 71:209–49. doi: 10.3322/caac.21660
3. Siegel RL, Miller KD, Wagle NS, Jemal A. Cancer statistics, 2023. CA: A Cancer J Clin. (2023) 73:17–48. doi: 10.3322/caac.21763
4. Wang S, Zheng R, Li J, Zeng H, Li L, Chen R, et al. Global, regional, and national lifetime risks of developing and dying from gastrointestinal cancers in 185 countries: a population-based systematic analysis of GLOBOCAN. Lancet Gastroenterol Hepatol. (2024) 9:229–37. doi: 10.1016/s2468-1253(23)00366-7
5. Capdevila C, Miller J, Cheng L, Kornberg A, George JJ, Lee H, et al. Time-resolved fate mapping identifies the intestinal upper crypt zone as an origin of Lgr5+ crypt base columnar cells. Cell. (2024) 187:3039–3055.e3014. doi: 10.1016/j.cell.2024.05.001
6. Yan HHN, Chan AS, Lai FP-L, Leung SY. Organoid cultures for cancer modeling. Cell Stem Cell. (2023) 30:917–37. doi: 10.1016/j.stem.2023.05.012
7. Margiotta F, Di Cesare Mannelli L, Morabito A, Ghelardini C, Lucarini E. Investigating epithelial-neuronal signaling contribution in visceral pain through colon organoid-dorsal root ganglion neuron co-cultures. Neural Regeneration Res. (2024) 19:1199–200. doi: 10.4103/1673-5374.386403
8. Ding S, Hsu C, Wang Z, Natesh NR, Millen R, Negrete M, et al. Patient-derived micro-organospheres enable clinical precision oncology. Cell Stem Cell. (2022) 29:905–917.e906. doi: 10.1016/j.stem.2022.04.006
9. Toshimitsu K, Takano A, Fujii M, Togasaki K, Matano M, Takahashi S, et al. Organoid screening reveals epigenetic vulnerabilities in human colorectal cancer. Nat Chem Biol. (2022) 18:605–14. doi: 10.1038/s41589-022-00984-x
10. Luo Z, Wang B, Luo F, Guo Y, Jiang N, Wei J, et al. Establishment of a large-scale patient-derived high-risk colorectal adenoma organoid biobank for high-throughput and high-content drug screening. BMC Med. (2023) 21:336. doi: 10.1186/s12916-023-03034-y
11. Hirokawa Y, Clarke J, Palmieri M, Tan T, Mouradov D, Li S, et al. Low-viscosity matrix suspension culture enables scalable analysis of patient-derived organoids and tumoroids from the large intestine. Commun Biol. (2021) 4:1067. doi: 10.1038/s42003-021-02607-y
12. Sato T, Vries RG, Snippert HJ, van de Wetering M, Barker N, Stange DE, et al. Single Lgr5 stem cells build crypt-villus structures in vitro without a mesenchymal niche. Nature. (2009) 459:262–5. doi: 10.1038/nature07935
13. Yoshida GJ. Applications of patient-derived tumor xenograft models and tumor organoids. J Hematol Oncol. (2020) 13:4. doi: 10.1186/s13045-019-0829-z
14. Wei H, Li W, Zeng L, Ding N, Li K, Yu H, et al. OLFM4 promotes the progression of intestinal metaplasia through activation of the MYH9/GSK3beta/beta-catenin pathway. Mol Cancer. (2024) 23:124. doi: 10.1186/s12943-024-02016-9
15. Tan H, Chen X, Wang C, Song J, Xu J, Zhang Y, et al. Intestinal organoid technology and applications in probiotics. Crit Rev Food Sci Nutr. (2023) 63:1–15. doi: 10.1080/10408398.2023.2288887
16. Zhang F-L, Hu Z, Wang YF, Zhang WJ, Zhou BW, Sun QS, et al. Organoids transplantation attenuates intestinal ischemia/reperfusion injury in mice through L-Malic acid-mediated M2 macrophage polarization. Nat Commun. (2023) 14:6779. doi: 10.1038/s41467-023-42502-0
17. Kinoshita H, Martinez-Ordonez A, Cid-Diaz T, Han Q, Duran A, Muta Y, et al. Epithelial aPKC deficiency leads to stem cell loss preceding metaplasia in colorectal cancer initiation. Dev Cell. (2024) 59:1972–87.e8. doi: 10.1016/j.devcel.2024.05.001
18. Hedayat S, Cascione L, Cunningham D, Schirripa M, Lampis A, Hahne JC, et al. Circulating microRNA analysis in a prospective co-clinical trial identifies MIR652-3p as a response biomarker and driver of regorafenib resistance mechanisms in colorectal cancer. Clin Cancer Res. (2024) 30:2140–59. doi: 10.1158/1078-0432.CCR-23-2748
19. Spiekman IAC, Zeverijn LJ, Geurts BS, Verkerk K, Haj Mohammad SF, van der Noort V, et al. Trastuzumab plus pertuzumab for HER2-amplified advanced colorectal cancer: Results from the drug rediscovery protocol (DRUP). Eur J Cancer. (2024) 202:113988. doi: 10.1016/j.ejca.2024.113988
20. Tan R, Zhang Z, Ding P, Liu Y, Liu H, Lu M, et al. A growth factor-reduced culture system for colorectal cancer organoids. Cancer Lett. (2024) 588:216737. doi: 10.1016/j.canlet.2024.216737
21. Yan HHN, Siu HC, Ho SL, Yue SSK, Gao Y, Tsui WY, et al. Organoid cultures of early-onset colorectal cancers reveal distinct and rare genetic profiles. Gut. (2020) 69:2165–79. doi: 10.1136/gutjnl-2019-320019
22. Maru Y, Kawata A, Taguchi A, Ishii Y, Baba S, Mori M, et al. Establishment and molecular phenotyping of organoids from the squamocolumnar junction region of the uterine cervix. Cancers. (2020) 12:694. doi: 10.3390/cancers12030694
23. Tong Y, Cheng PSW, Or CS, Yue SSK, Siu HC, Ho SL, et al. Escape from cell-cell and cell-matrix adhesion dependence underscores disease progression in gastric cancer organoid models. Gut. (2023) 72:242–55. doi: 10.1136/gutjnl-2022-327121
24. Aiderus A, Barker N, Tergaonkar V. Serrated colorectal cancer: preclinical models and molecular pathways. Trends Cancer. (2024) 10:76–91. doi: 10.1016/j.trecan.2023.09.011
25. Stribbling SM, Beach C, Ryan AJ. Orthotopic and metastatic tumour models in preclinical cancer research. Pharmacol Ther. (2024) 257:108631. doi: 10.1016/j.pharmthera.2024.108631
26. Han X, Cai C, Deng W, Shi Y, Li L, Wang C, et al. Landscape of human organoids: Ideal model in clinics and research. Innovation. (2024) 5:100620. doi: 10.1016/j.xinn.2024.100620
27. Hu C, Yang S, Zhang T, Ge Y, Chen Z, Zhang J, et al. Organoids and organoids-on-a-chip as the new testing strategies for environmental toxicology-applications & advantages. Environ Int. (2024) 184:108415. doi: 10.1016/j.envint.2024.108415
28. McCoy R, Oldroyd S, Yang W, Wang K, Hoven D, Bulmer D, et al. In vitro models for investigating intestinal host–pathogen interactions. Advanced Sci. (2023) 11:e2306727. doi: 10.1002/advs.202306727
29. Rubahamya B, Dong S, Thurber GM. Clinical translation of antibody drug conjugate dosing in solid tumors from preclinical mouse data. Sci Adv. (2024) 10:eadk1894. doi: 10.1126/sciadv.adk1894
30. Stribbling SM, Ryan AJ. The cell-line-derived subcutaneous tumor model in preclinical cancer research. Nat Protoc. (2022) 17:2108–28. doi: 10.1038/s41596-022-00709-3
31. Pothuraju R, Khan I, Jain M, Bouvet M, Malafa M, Roy HK, et al. Colorectal cancer murine models: Initiation to metastasis. Cancer Lett. (2024) 587:216704. doi: 10.1016/j.canlet.2024.216704
32. Kaushal JB, Takkar S, Batra SK, Siddiqui JA. Diverse landscape of genetically engineered mouse models: Genomic and molecular insights into prostate cancer. Cancer Lett. (2024) 593:216954. doi: 10.1016/j.canlet.2024.216954
33. Thomas EM, Wright JA, Blake SJ, Page AJ, Worthley DL, Woods SL. Advancing translational research for colorectal immuno-oncology. Br J Cancer. (2023) 129:1442–50. doi: 10.1038/s41416-023-02392-x
34. Lago C, Gianesello M, Santomaso L, Leva G, Ballabio C, Anderle M, et al. Medulloblastoma and high-grade glioma organoids for drug screening, lineage tracing, co-culture and. Vivo assay Nat Protoc. (2023) 18:2143–80. doi: 10.1038/s41596-023-00839-2
35. Clarke S, Chin SN, Dodds L, George SHL, Badal S. Racial disparities in breast cancer preclinical and clinical models. Breast Cancer Res. (2022) 24:56. doi: 10.1186/s13058-022-01551-x
36. Fallahi P, Ferrari SM, Elia G, Ragusa F, Patrizio A, Paparo SR, et al. Primary cell cultures for the personalized therapy in aggressive thyroid cancer of follicular origin. Semin Cancer Biol. (2022) 79:203–16. doi: 10.1016/j.semcancer.2020.06.013
37. Cong J, Wu J, Fang Y, Wang J, Kong X, Wang L, et al. Application of organoid technology in the human health risk assessment of microplastics: A review of progresses and challenges. Environ Int. (2024) 188:108744. doi: 10.1016/j.envint.2024.108744
38. Jin H, Xue Z, Liu J, Ma B, Yang J, Lei L. Advancing organoid engineering for tissue regeneration and biofunctional reconstruction. Biomaterials Res. (2024) 28:0016. doi: 10.34133/bmr.0016
39. Xiang D, He A, Zhou R, Wang Y, Xiao X, Gong, et al. Building consensus on the application of organoid-based drug sensitivity testing in cancer precision medicine and drug development. Theranostics. (2024) 14:3300–16. doi: 10.7150/thno.96027
40. Kaluthantrige Don F, Huch M. Organoids, where we stand and where we go. Trends Mol Med. (2021) 27:416–8. doi: 10.1016/j.molmed.2021.03.001
41. Pawlowski KD, Duffy JT, Babak MV, Balyasnikova IV. Modeling glioblastoma complexity with organoids for personalized treatments. Trends Mol Med. (2023) 29:282–96. doi: 10.1016/j.molmed.2023.01.002
42. Phan MAT, Madigan MC, Stapleton F, Willcox M, Golebiowski B. Human meibomian gland epithelial cell culture models: Current progress, challenges, and future directions. Ocular Surface. (2022) 23:96–113. doi: 10.1016/j.jtos.2021.11.012
43. Idris M, Alves MM, Hofstra RMW, Mahe MM, Melotte V. Intestinal multicellular organoids to study colorectal cancer. Biochim Biophys Acta (BBA) - Rev Cancer. (1876) 2021):188586. doi: 10.1016/j.bbcan.2021.188586
44. Rubert J, Schweiger PJ, Mattivi F, Tuohy K, Jensen KB, Lunardi A. Intestinal organoids: A tool for modelling diet–microbiome–host interactions. Trends Endocrinol Metab. (2020) 31:848–58. doi: 10.1016/j.tem.2020.02.004
45. Li N, Zhu Q, Tian Y, Ahn KJ, Wang X, Cramer Z, et al. Mapping and modeling human colorectal carcinoma interactions with the tumor microenvironment. Nat Commun. (2023) 14:7915. doi: 10.1038/s41467-023-43746-6
46. Fumagalli A, Oost KC, Kester L, Morgner J, Bornes L, Bruens L, et al. Plasticity of lgr5-negative cancer cells drives metastasis in colorectal cancer. Cell Stem Cell. (2020) 26:569–578.e567. doi: 10.1016/j.stem.2020.02.008
47. Zhao H, Ming T, Tang S, Ren S, Yang H, Liu ML, et al. Wnt signaling in colorectal cancer: pathogenic role and therapeutic target. Mol Cancer. (2022) 21:144. doi: 10.1186/s12943-022-01616-7
48. Pastorino GA, Sheraj I, Huebner K, Ferrero G, Kunze P, Hartmann A, et al. A partial epithelial-mesenchymal transition signature for highly aggressive colorectal cancer cells that survive under nutrient restriction. J Pathol. (2024) 262:347–61. doi: 10.1002/path.6240
49. Tassi E, McDonnell K, Gibby KA, Tilan JU, Kim SE, Kodack DP, et al. Impact of fibroblast growth factor-binding protein–1 expression on angiogenesis and wound healing. Am J Pathol. (2011) 179:2220–32. doi: 10.1016/j.ajpath.2011.07.043
50. Dekkers JF, Wiegerinck CL, de Jonge HR, Bronsveld I, Janssens HM, de Winter-de Groot KM, et al. A functional CFTR assay using primary cystic fibrosis intestinal organoids. Nat Med. (2013) 19:939–45. doi: 10.1038/nm.3201
51. Lorenzo-Martin LF, Hubscher T, Bowler AD, Broguiere N, Langer J, Tillard L, et al. Spatiotemporally resolved colorectal oncogenesis in mini-colons ex vivo. Nature. (2024) 629:450–7. doi: 10.1038/s41586-024-07330-2
52. Levring J, Terry DS, Kilic Z, Fitzgerald G, Blanchard SC, Chen J, et al. CFTR function, pathology and pharmacology at single-molecule resolution. Nature. (2023) 616:606–14. doi: 10.1038/s41586-023-05854-7
53. Kurzawa-Akanbi M, Tzoumas N, Corral-Serrano JC, Guarascio R, Steel DH, Cheetham ME, et al. Pluripotent stem cell-derived models of retinal disease: Elucidating pathogenesis, evaluating novel treatments, and estimating toxicity. Prog Retin Eye Res. (2024) 100:101248. doi: 10.1016/j.preteyeres.2024.101248
54. Agostinho de Sousa J, Wong CW, Dunkel I, Owens T, Voigt P, Hodgson A, et al. Epigenetic dynamics during capacitation of naive human pluripotent stem cells. Sci Adv. (2023) 9:eadg1936. doi: 10.1126/sciadv.adg1936
55. Wang L, Koui Y, Kanegae K, Kido T, Tamura-Nakano M, Yabe S, et al. Establishment of human induced pluripotent stem cell-derived hepatobiliary organoid with bile duct for pharmaceutical research use. Biomaterials. (2024) 310:122621. doi: 10.1016/j.biomaterials.2024.122621
56. Zhu Y, Huang S, Chen S, Chen J, Wang Z, Wang Y, et al. SOX2 promotes chemoresistance, cancer stem cells properties, and epithelial–mesenchymal transition by β-catenin and Beclin1/autophagy signaling in colorectal cancer. Cell Death Dis. (2021) 12:449. doi: 10.1038/s41419-021-03733-5
57. Cerneckis J, Cai H, Shi Y. Induced pluripotent stem cells (iPSCs): molecular mechanisms of induction and applications. Signal Transduct Target Ther. (2024) 9:112. doi: 10.1038/s41392-024-01809-0
58. Wang Q, Guo F, Jin Y, Ma Y. Applications of human organoids in the personalized treatment for digestive diseases. Signal Transduct Target Ther. (2022) 7:336. doi: 10.1038/s41392-022-01194-6
59. Zheng X, Song J, Yu C, Zhou Z, Liu X, Yu J, et al. Single-cell transcriptomic profiling unravels the adenoma-initiation role of protein tyrosine kinases during colorectal tumorigenesis. Signal Transduct Target Ther. (2022) 7:60. doi: 10.1038/s41392-022-00881-8
60. Chen X, Wang C, Jiang Y, Wang Q, Tao Y, Zhang H, et al. Bcl-3 promotes Wnt signaling by maintaining the acetylation of β-catenin at lysine 49 in colorectal cancer. Signal Transduction Targeted Ther. (2020) 5:52. doi: 10.1038/s41392-020-0138-6
61. Bustamante-Madrid P, Barbachano A, Albandea-Rodriguez D, Rodriguez-Cobos J, Rodriguez-Salas N, Prieto I, et al. Vitamin D opposes multilineage cell differentiation induced by Notch inhibition and BMP4 pathway activation in human colon organoids. Cell Death Dis. (2024) 15:301. doi: 10.1038/s41419-024-06680-z
62. Múnera JO, Jeffcoat B, Daoud A, Qu N. Generation, maintenance, and characterization of human pluripotent stem cell-derived intestinal and colonic organoids. J Visualized Experiments. (2021) 173:10.3791/62721. doi: 10.3791/62721
63. Zhang X, Zhu R, Yu D, Wang J, Yan Y, Xu K. Single-cell RNA sequencing to explore cancer-associated fibroblasts heterogeneity: "Single" vision for "heterogeneous" environment. Cell Prolif. (2024) 57:e13592. doi: 10.1111/cpr.13592
64. Xiang K, Wang E, Mantyh J, Rupprecht G, Negrete M, Sanati G, et al. Chromatin remodeling in patient-derived colorectal cancer models. Adv Sci (Weinh). (2024) 11:e2303379. doi: 10.1002/advs.202303379
65. Li F, Wu Y, Yan Y, Wu S, Zhu J, Zhang G, et al. Transcriptomic landscape of sodium butyrate-induced growth inhibition of human colorectal cancer organoids. Mol Omics. (2022) 18:754–64. doi: 10.1039/d2mo00127f
66. Zheng H, Liu J, Cheng Q, Zhang Q, Zhang Y, Jiang L, et al. Targeted activation of ferroptosis in colorectal cancer via LGR4 targeting overcomes acquired drug resistance. Nat Cancer. (2024) 5:572–89. doi: 10.1038/s43018-023-00715-8
67. Loevenich LP, Tschurtschenthaler M, Rokavec M, Silva MG, Jesinghaus M, Kirchner T, et al. SMAD4 loss induces c-MYC–mediated NLE1 upregulation to support protein biosynthesis, colorectal cancer growth, and metastasis. Cancer Res. (2022) 82:4604–23. doi: 10.1158/0008-5472.Can-22-1247
68. Smabers LP, Wensink E, Verissimo CS, Koedoot E, Pitsa KC, Huismans MA, et al. Organoids as a biomarker for personalized treatment in metastatic colorectal cancer: drug screen optimization and correlation with patient response. J Exp Clin Cancer Res. (2024) 43:61. doi: 10.1186/s13046-024-02980-6
69. Jung YH, Choi DH, Park KW, Lee SB, Kim J, Kim H, et al. Drug screening by uniform patient derived colorectal cancer hydro-organoids. Biomaterials. (2021) 276:121004. doi: 10.1016/j.biomaterials.2021.121004
70. Mertens S, Huismans MA, Verissimo CS, Ponsioen B, Overmeer R, Proost N, et al. Drug-repurposing screen on patient-derived organoids identifies therapy-induced vulnerability in KRAS-mutant colon cancer. Cell Rep. (2023) 42:112324. doi: 10.1016/j.celrep.2023.112324
71. Kong J, Lee H, Kim DH, Han SK, Ha D, Shin K, et al. Network-based machine learning in colorectal and bladder organoid models predicts anti-cancer drug efficacy in patients. Nat Commun. (2020) 11:5485. doi: 10.1038/s41467-020-19313-8
72. Tan T, Mouradov D, Lee M, Gard G, Hirokawa Y, Li S, et al. Unified framework for patient-derived, tumor-organoid-based predictive testing of standard-of-care therapies in metastatic colorectal cancer. Cell Rep Med. (2023) 4:101335. doi: 10.1016/j.xcrm.2023.101335
73. Castro F, Leite Pereira C, Helena Macedo M, Almeida A, José Silveira M, Dias S, et al. Advances on colorectal cancer 3D models: The needed translational technology for nanomedicine screening. Advanced Drug Delivery Rev. (2021) 175:113824. doi: 10.1016/j.addr.2021.06.001
74. Fatima I, Ahmad R, Barman S, Gowrikumar SP, Pravoverov K, Primeaux M, et al. Albendazole inhibits colon cancer progression and therapy resistance by targeting ubiquitin ligase RNF20. Br J Cancer. (2024) 130:1046–58. doi: 10.1038/s41416-023-02570-x
75. Swartjes H, Sijtsma FPC, Elferink MAG, van Erning FN, Moons LMG, Verheul HMW, et al. Trends in incidence, treatment, and relative survival of colorectal cancer in the Netherlands between 2000 and 2021. Eur J Cancer. (2024) 205:114104. doi: 10.1016/j.ejca.2024.114104
76. Huang Y, Ge K, Fu G, Chu J, Wei W. Efficacy of primary tumor resection in metastatic colorectal cancer. Med Sci Monitor. (2020) 26:e923501. doi: 10.12659/msm.923501
77. Cartry J, Bedja S, Boilève A, Mathieu JRR, Gontran E, Annereau M, et al. Implementing patient derived organoids in functional precision medicine for patients with advanced colorectal cancer. J Exp Clin Cancer Res. (2023) 42:281. doi: 10.1186/s13046-023-02853-4
78. Chen L, Bedja S, Boilève A, Mathieu JRR, Gontran E, Annereau M, et al. Specific metabolic response of patient-derived organoids to curcumin of colorectal cancer. J Chromatogr B. (1203) 2022):123260. doi: 10.1016/j.jchromb.2022.123260
79. Li Y, Tang P, Cai S, Peng J, Hua G. Organoid based personalized medicine: from bench to bedside. Cell Regeneration. (2020) 9:21. doi: 10.1186/s13619-020-00059-z
80. Lee J, Kim ST, Kim K, Lee H, Kozarewa I, Mortimer PGS, et al. Tumor genomic profiling guides patients with metastatic gastric cancer to targeted treatment: the VIKTORY umbrella trial. Cancer Discovery. (2019) 9:1388–405. doi: 10.1158/2159-8290.Cd-19-0442
81. Zormpas-Petridis K, Poon E, Clarke M, Jerome NP, Boult JKR, Blackledge MD, et al. Noninvasive MRI native T1 mapping detects response to MYCN-targeted therapies in the th-MYCN model of neuroblastoma. Cancer Res. (2020) 80:3424–35. doi: 10.1158/0008-5472.Can-20-0133
82. Turner NC, Oliveira M, Howell SJ, Dalenc F, Cortes J, Gomez Moreno HL, et al. Capivasertib in hormone receptor–positive advanced breast cancer. New Engl J Med. (2023) 388:2058–70. doi: 10.1056/NEJMoa2214131
83. Burstein HJ, DeMichele A, Fallowfield L, Somerfield MR, Henry NL, Dayao Z, et al. Endocrine and targeted therapy for hormone receptor–positive, human epidermal growth factor receptor 2–negative metastatic breast cancer—Capivasertib-fulvestrant: ASCO rapid recommendation update. J Clin Oncol. (2024) 42:1450–3. doi: 10.1200/jco.24.00248
84. Dilawari A, Buturla J, Osgood C, Gao X, Chen W, Ricks TK, et al. US food and drug administration approval summary: capivasertib with fulvestrant for hormone receptor–positive, human epidermal growth factor receptor 2–negative locally advanced or metastatic breast cancer with PIK3CA/AKT1/PTEN alterations. J Clin Oncol. (2024) 42:1450–3. doi: 10.1200/jco.24.00427
85. Gross AM, Wolters PL, Dombi E, Baldwin A, Whitcomb P, Fisher MJ, et al. Selumetinib in children with inoperable plexiform neurofibromas. New Engl J Med. (2020) 382:1430–42. doi: 10.1056/NEJMoa1912735
86. Dombi E, Baldwin A, Marcus LJ, Fisher MJ, Weiss B, Kim AR, et al. Activity of selumetinib in neurofibromatosis type 1–related plexiform neurofibromas. New Engl J Med. (2016) 375:2550–60. doi: 10.1056/NEJMoa1605943
87. Hosomi Y, Morita S, Sugawara S, Kato T, Fukuhara T, Gemma A, et al. Gefitinib alone versus gefitinib plus chemotherapy for non–small-cell lung cancer with mutated epidermal growth factor receptor: NEJ009 study. J Clin Oncol. (2020) 38:115–23. doi: 10.1200/jco.19.01488
88. Noronha V, Patil VM, Joshi A, Menon N, Chougule A, Mahajan A, et al. Gefitinib versus gefitinib plus pemetrexed and carboplatin chemotherapy in EGFR-mutated lung cancer. J Clin Oncol. (2020) 38:124–36. doi: 10.1200/jco.19.01154
89. Finn RS, Martin M, Rugo HS, Jones S, Im SA, Gelmon K, et al. Palbociclib and letrozole in advanced breast cancer. New Engl J Med. (2016) 375:1925–36. doi: 10.1056/NEJMoa1607303
90. Mayer EL, Dueck AC, Martin M, Rubovszky G, Burstein HJ, Bellet-Ezquerra M, et al. Palbociclib with adjuvant endocrine therapy in early breast cancer (PALLAS): interim analysis of a multicentre, open-label, randomised, phase 3 study. Lancet Oncol. (2021) 22:212–22. doi: 10.1016/s1470-2045(20)30642-2
91. Rini BI, Plimack ER, Stus V, Gafanov R, Hawkins R, Nosov D, et al. Pembrolizumab plus Axitinib versus Sunitinib for Advanced Renal-Cell Carcinoma. New Engl J Med. (2019) 380:1116–27. doi: 10.1056/NEJMoa1816714
92. Powles T, Plimack ER, Soulières D, Waddell T, Stus V, Gafanov R, et al. Pembrolizumab plus axitinib versus sunitinib monotherapy as first-line treatment of advanced renal cell carcinoma (KEYNOTE-426): extended follow-up from a randomised, open-label, phase 3 trial. Lancet Oncol. (2020) 21:1563–73. doi: 10.1016/s1470-2045(20)30436-8
93. Layman RM, Han HS, Rugo HS, Stringer-Reasor EM, Specht JM, Dees EC, et al. Gedatolisib in combination with palbociclib and endocrine therapy in women with hormone receptor-positive, HER2-negative advanced breast cancer: results from the dose expansion groups of an open-label, phase 1b study. Lancet Oncol. (2024) 25:474–87. doi: 10.1016/s1470-2045(24)00034-2
94. Cortes JE, Heidel FH, Fiedler W, Smith BD, Robak T, Montesinos P, et al. Survival outcomes and clinical benefit in patients with acute myeloid leukemia treated with glasdegib and low-dose cytarabine according to response to therapy. J Hematol Oncol. (2020) 13:92. doi: 10.1186/s13045-020-00929-8
95. Ooft SN, Weeber F, Schipper L, Dijkstra KK, McLean CM, Kaing S, et al. Prospective experimental treatment of colorectal cancer patients based on organoid drug responses. ESMO Open. (2021) 6:100103. doi: 10.1016/j.esmoop.2021.100103
96. Wang T, Tang Y, Pan W, Yan B, Hao Y, Zeng Y, et al. Patient-derived tumor organoids can predict the progression-free survival of patients with stage IV colorectal cancer after surgery. Dis Colon Rectum. (2023) 66:733–43. doi: 10.1097/dcr.0000000000002511
97. Papaccio F, García-Mico B, Gimeno-Valiente F, Cabeza-Segura M, Gambardella V, Gutiérrez-Bravo MF, et al. Proteotranscriptomic analysis of advanced colorectal cancer patient derived organoids for drug sensitivity prediction. J Exp Clin Cancer Res. (2023) 42:8. doi: 10.1186/s13046-022-02591-z
98. Vlachogiannis G, Hedayat S, Vatsiou A, Jamin Y, Fernández-Mateos J, Khan K, et al. Patient-derived organoids model treatment response of metastatic gastrointestinal cancers. Science. (2018) 359:920–6. doi: 10.1126/science.aao2774
99. Ng S, Tan WJ, Pek MMX, Tan M-H, Kurisawa M. Mechanically and chemically defined hydrogel matrices for patient-derived colorectal tumor organoid culture. Biomaterials. (2019) 219:119400. doi: 10.1016/j.biomaterials.2019.119400
100. Wang J, Sui Z, Huang W, Yu Z, Guo L. Biomimetic hydrogels with mesoscale collagen architecture for patient-derived tumor organoids culture. Bioactive Materials. (2024) 38:384–98. doi: 10.1016/j.bioactmat.2024.04.035
101. Gjorevski N, Lutolf MP. Synthesis and characterization of well-defined hydrogel matrices and their application to intestinal stem cell and organoid culture. Nat Protoc. (2017) 12:2263–74. doi: 10.1038/nprot.2017.095
102. Luo X, Fong ELS, Zhu C, Lin QXX, Xiong M, Li A, et al. Hydrogel-based colorectal cancer organoid co-culture models. Acta Biomaterialia. (2021) 132:461–72. doi: 10.1016/j.actbio.2020.12.037
103. Beetler DJ, Di Florio DN, Law EW, Groen CM, Windebank AJ, Peterson QP, et al. The evolving regulatory landscape in regenerative medicine. Mol Aspects Med. (2023) 91:101138. doi: 10.1016/j.mam.2022.101138
104. Mulero-Russe A, García AJ. Engineered synthetic matrices for human intestinal organoid culture and therapeutic delivery. Advanced Materials. (2023) 36:e2307678. doi: 10.1002/adma.202307678
105. Múnera JO, Kechele DO, Bouffi C, Qu N, Jing R, Maity P, et al. Development of functional resident macrophages in human pluripotent stem cell-derived colonic organoids and human fetal colon. Cell Stem Cell. (2023) 30:1434–1451.e1439. doi: 10.1016/j.stem.2023.10.002
106. Watanabe S, Kobayashi S, Ogasawara N, Okamoto R, Nakamura T, Watanabe M, et al. Transplantation of intestinal organoids into a mouse model of colitis. Nat Protoc. (2022) 17:649–71. doi: 10.1038/s41596-021-00658-3
107. Lee J-Y, Han H-J, Lee S-J, Cho E-H, Lee H-B, Seok J-H, et al. Use of 3D human liver organoids to predict drug-induced phospholipidosis. Int J Mol Sci. (2020) 21:2982. doi: 10.3390/ijms21082982
108. Zha J-M, Ding N, He W-Q, Xu H, Lin Q, Chen Y, et al. Current applications of colorectal cancer organoids: A review. J Gastrointestinal Liver Dis. (2024) 33:269–77. doi: 10.15403/jgld-5388
109. Ramzy GM, Norkin M, Koessler T, Voirol L, Tihy M, Hany D, et al. Platform combining statistical modeling and patient-derived organoids to facilitate personalized treatment of colorectal carcinoma. J Exp Clin Cancer Res. (2023) 42:79. doi: 10.1186/s13046-023-02650-z
110. Fior R, Póvoa V, Mendes RV, Carvalho T, Gomes A, Figueiredo N, et al. Single-cell functional and chemosensitive profiling of combinatorial colorectal therapy in zebrafish xenografts. Proc Natl Acad Sci. (2017) 114:E8234–43. doi: 10.1073/pnas.1618389114
111. Neal JT, Li X, Zhu J, Giangarra V, Grzeskowiak CL, Ju J, et al. Organoid modeling of the tumor immune microenvironment. Cell. (2018) 175:1972–1988.e1916. doi: 10.1016/j.cell.2018.11.021
112. Louey A, Hernández D, Pébay A, Daniszewski M. Automation of organoid cultures: current protocols and applications. SLAS Discovery. (2021) 26:1138–47. doi: 10.1177/24725552211024547
113. Pinho D, Santos D, Vila A, Carvalho S. Establishment of colorectal cancer organoids in microfluidic-based system. Micromachines. (2021) 12:497. doi: 10.3390/mi12050497
114. Berg KCG, Eide PW, Eilertsen IA, Johannessen B, Bruun J, Danielsen SA, et al. Multi-omics of 34 colorectal cancer cell lines - a resource for biomedical studies. Mol Cancer. (2017) 16:116. doi: 10.1186/s12943-017-0691-y
115. Creff J, Malaquin L, Besson A. In vitro models of intestinal epithelium: Toward bioengineered systems. J Tissue Eng. (2021) 12:2041731420985202. doi: 10.1177/2041731420985202
116. Shirure VS, Hughes CCW, George SC. Engineering vascularized organoid-on-a-chip models. Annu Rev Biomed Eng. (2021) 23:141–67. doi: 10.1146/annurev-bioeng-090120-094330
117. Jalili-Firoozinezhad S, Miranda CC, Cabral JMS. Modeling the human body on microfluidic chips. Trends Biotechnol. (2021) 39:838–52. doi: 10.1016/j.tibtech.2021.01.004
118. Gu Y, Zhang W, Wu X, Zhang Y, Xu K, Su J. Organoid assessment technologies. Clin Trans Med. (2023) 13:e1499. doi: 10.1002/ctm2.1499
119. Mauri G, Arena S, Siena S, Bardelli A, Sartore-Bianchi A. The DNA damage response pathway as a land of therapeutic opportunities for colorectal cancer. Ann Oncol. (2020) 31:1135–47. doi: 10.1016/j.annonc.2020.05.027
120. Yao Y, Xu X, Yang L, Zhu J, Wan J, Shen L, et al. Patient-derived organoids predict chemoradiation responses of locally advanced rectal cancer. Cell Stem Cell. (2020) 26:17–26.e16. doi: 10.1016/j.stem.2019.10.010
Keywords: organoids, colorectal cancer, research advances, cancer, applications
Citation: Wu Y, Sha Y, Guo X, Gao L, Huang J and Liu S-B (2025) Organoid models: applications and research advances in colorectal cancer. Front. Oncol. 15:1432506. doi: 10.3389/fonc.2025.1432506
Received: 14 May 2024; Accepted: 20 January 2025;
Published: 07 February 2025.
Edited by:
Ilyas Sahin, Massachusetts General Hospital and Harvard Medical School, United StatesCopyright © 2025 Wu, Sha, Guo, Gao, Huang and Liu. This is an open-access article distributed under the terms of the Creative Commons Attribution License (CC BY). The use, distribution or reproduction in other forums is permitted, provided the original author(s) and the copyright owner(s) are credited and that the original publication in this journal is cited, in accordance with accepted academic practice. No use, distribution or reproduction is permitted which does not comply with these terms.
*Correspondence: Song-Bai Liu, bGl1c29uZ2JhaUAxMjYuY29t; Ling Gao, bWNob3RraWRAc2luYS5jb20=; Jian Huang, amh1YW5nQHN6aGN0LmVkdS5jbg==
†These authors have contributed equally to this work and share first authorship