- Department of Translational Biomedicine and Neuroscience, University of Bari Medical School, Bari, Italy
Tryptases represent the most abundant constituent of human mast cells, involved in extracellular matrix degradation, contributing to wound healing and metastasis. Moreover, most recently, it has been demonstrated that tryptase is angiogenic both in vitro and in vivo. Tryptase-positive mast cell number increases parallelly with increased microvascular density in both solid and hematological tumors. The objective and the scope of this review article are to emphasize the important role of tryptase as one of the principal effectors of tumor angiogenesis mediated by mast cells. In this context, tryptase inhibitors may be considered a novel therapeutic approach in cancer treatment.
Introduction
Angiogenesis, forming new blood vessels from pre-existing ones, occurs in various physiological and pathological conditions, such as embryonic development, wound healing, the menstrual cycle, and chronic inflammation and tumors. Tumor angiogenesis is linked to a switch in the equilibrium between positive and negative regulators and mainly depends on the release by neoplastic cells of growth factors specific to endothelial cells and it can stimulate the growth of host’s blood vessels (1, 79). Moreover, different classes of proteases, including matrix metalloproteinases (MMPs) (2), serine proteases (3), aminopeptidases (4), transmembrane proteases (TPs) (5), type II transmembrane serine proteases (TTSPs) (6), kallikrein-related peptidases (KLKs) (7), are involved in tumor angiogenesis (8).
Immune cells are also able to synthesize and secrete pro-angiogenic factors that promote tumor angiogenesis. Among these cells, mast cells exert both anti-tumorigenic and pro-tumorigenic roles. These cells produce several angiogenic factors, including fibroblast growth factor-2 (FGF-2), vascular endothelial growth factors (VEGF), and interleukin-8 (IL-8), as well as proteases, promoting tumor neovascularization. By contrast, mast cells inhibit tumor growth releasing cytokines and growth factors, including tumor necrosis factor-alpha (TNF-α), transforming growth factor beta (TGF-β), interferon-alpha (IFN-α), and bioactive monoamines (9).
An increased number of mast cells have been demonstrated in angiogenesis associated with vascular tumors, like hemangioma and hemangioblastoma, as well as several hematological and solid tumors, including lymphomas, multiple myeloma myelodysplastic syndrome, B-cell chronic lymphocytic leukemia, breast cancer, gastric and colorectal cancer, uterine cervix cancer, melanoma, and pulmonary adenocarcinoma, in which mast cell accumulation correlate with increased neovascularization, tumor aggressiveness, and poor prognosis (10). Conversely, mast cells have been demonstrated to play a protective role in the early stages of intestinal tumorigenesis (11). In this context, similarly to neutrophils (N1 and N2 subpopulations), and macrophages (M1 and M2 subpopulations), also mast cells are polarized toward anti-tumorigenic (MC1) or pro-tumorigenic (MC2) cell types (12).
Mast cells produce different biological mediators, including histamine, proteoglycans, proteases, cytokines, lipid mediators, and growth factors. Most proteins synthesized in mast cells are proteases, stored fully active in a complex with heparin (13). Mast cells are a reservoir of neutral proteases, packed in large amounts in the secretory granules, including tryptases, chymases, cathepsin C and G, and carboxypeptidase A3 (14). The secretion of these mediators is a consequence of mast cell degranulation and occurs as a response to physical factors, toxins, venoms, proteins, tissue proteases, and immune mechanisms, dependent or not dependent on IgE. Human tryptase is considered specific to mast cells (15), even if basophils contain and release tryptase (16).
Genetic analysis of tryptases in different species suggests that these proteases proliferated and changed rapidly during mammalian evolution, arising from ancestral membrane-anchored peptidases, which are present in a variety of vertebrate genomes such as reptiles, amphibians, and fish (17).
Tryptases, a group of 130 kD serine peptidases representing the most abundant constituent of human mast cells, are involved in extracellular matrix degradation, contributing to wound healing and metastasis. In humans, there are five isoforms of mast cell tryptase, α-, β-, γ-, δ-, and ε-tryptase. (18). Alpha-tryptases are classified in α-I and α-II tryptases, while β-tryptases are classified in β-I, β-II, and β-III tryptases (19). Alpha- and β-tryptases are the most abundant and clinically relevant, with approximately 90% sequence homology between them. Tryptases cleave fibronectin and type VI collagen, pro-enzyme forms of MMPs, and urokinase plasminogen activator (uPA), different bronchial and intestinal neuropeptides, such as calcitonin gene-related peptide (CGRP) and vasointestinal peptide (VIP), and IgE molecules, downregulating the allergic response.
βII-tryptase is stored in the secretory granules of mast cells. In contrast, a-pro-tryptase is secreted constitutively from mast cells as an inactive proenzyme.
The activation of βII-pro-tryptase involves two proteolytic steps. The most common mutations of the tryptase gene lead to loss of membrane anchoring, defective zymogen activation, or loss of catalytic function, thereby giving rise to changes in specificity (20). Extra copy numbers of tryptase α1 (TPSAB1) reflecting hereditary α-tryptasemia (HαT), a common genetic trait with increased copy numbers of the α-tryptase encoding gene, that correlates with mast cell activation-related events. In patients with mastocytosis, the presence of a HαT was associated with high serum tryptase independent of the clonal mast cell burden (21).
Tryptase plays a crucial role in mast cell ontogeny (22). The first source of mast cells is the extraembryonic yolk sac, on embryonic day 7. Mast cell progenitors circulate and enter peripheral tissues where they complete their differentiation, and embryonic mast cell populations are gradually replaced by definitive stem cell-derived progenitor cells. Transcriptome analysis of mast cells derived from human umbilical cord blood and peripheral blood, revealed a series of mast cell-specific genes, including tryptase α1 and β1, L-histidine decarboxylase, cathepsin G, and carboxypeptidase A (23).
In humans, mast cells containing tryptase only are designated as MCsT or “immune cells associated” mast cells predominantly located in the respiratory and intestinal mucosa, where they co-localize with T cells. Instead, mast cells that contain both tryptase and chymase referred to as MCsTC, are predominantly found in the skin, submucosa of the stomach and intestine, breast parenchyma, myocardium, lymph nodes, conjunctiva, and synovium (24, 78).
Secretion of tryptase from mast cells triggers the release of more tryptase from neighboring mast cells (25). Tryptase stimulates the proliferation of airway muscle cells (26), fibroblast migration and proliferation (27), and induces the synthesis and release of collagen from fibroblasts in vitro (28). In breast cancer, mast cell tryptase promotes myofibroblast differentiation in the tumor stroma (29). Moreover, tryptase stimulates vascular permeability and chemotaxis of neutrophils and eosinophils.
Tryptase levels in biological fluids reflect the number of mast cells (30). In healthy individuals, serum baseline tryptase levels are very stable over time, ranging between 1 and 15 ng/ml, while altered levels indicate the risk of severe allergic manifestations (31). The predominant indication for tryptase measurement is to document systemic mast cell activation conditions during anaphylaxis or episodes of mast cell activation syndromes. Serum tryptase has been described as a circulating predictive surrogate marker in colorectal cancer and in breast cancer before and after surgical resection when tryptase levels significantly decrease (32, 33).
This review article’s objective and scope are to emphasize the important role of tryptase as one of the principal effectors of tumor angiogenesis mediated by mast cells. In this context, tryptase inhibitors may be considered a novel therapeutic approach in cancer treatment.
Tryptase and tumor angiogenesis
Paul Ehrlich discussed the possible connection of mast cells with tumor growth and progression early in 1878 (34). Indeed, most tumors contain inflammatory cell infiltrates, which often include mast cells. Thus, the importance of a potential functional link between chronic inflammation and cancer has long been recognized and the question of the possible contribution of mast cells to tumor development has progressively emerged. It was Rudolf Virchow in 1863, who critically recognized the presence of inflammatory cells infiltrating neoplastic tissues and first established a causative connection between the “lymphoreticular infiltrate” at sites of chronic inflammation and cancer (35).
There is evidence that different cell types of the innate immune system, including mast cells and macrophages play a critical role in enhancing tumor angiogenesis, either directly through the release of angiogenic cytokines and proteolytic enzymes, or indirectly through paracrine signals.
It is still not completely known and clarified the role of tryptase as another pro-angiogenic factor released by mast cells. Tryptase-positive mast cells are among the first immune cells recruited to tumor sites in response to the chemotactic stimuli and are increased in solid tumors, at the boundary between malignant and healthy tissues (29, 36). The main chemoattractant factor produced by tumor cells is stem cell factor (SCF), the ligand for the tyrosine kinase receptor kit (CD 117) expressed by mast cells and considered the most important factor involved in the regulation of mast cell number in physiological conditions (37, 38).
Blair et al. (39) for the first time investigated in vitro the angiogenic potential of tryptase demonstrating that tryptase added to microvascular endothelial cells cultured on Matrigel causes an increase in capillary growth in a dose-dependent fashion, and specific tryptase inhibitors suppressed this effect.
Tryptase stimulates endothelial cell release of IL-1, IL-6, IL-8, SCF, TNF-α, and other inflammatory mediators (40). Moreover, tryptase promotes chemotaxis of neutrophils and macrophages, which induces the new formation of capillaries, and activates MMP-9, which favors the release of angiogenic factors stored in the extracellular matrix (41).
We have demonstrated an angiogenic activity of human recombinant tryptase with a grade of purity of 95% in vivo in the chick embryo chorioallantoic membrane (CAM) assay (Figure 1), comparable to the angiogenic response induced by a well-known angiogenic cytokine, namely VEGF (42). Tryptase contributes to atherosclerotic plaque angiogenesis and hemorrhage by regulating VEGF, PA inhibitor (PAI), and tissue PA (tPA) expression (43).
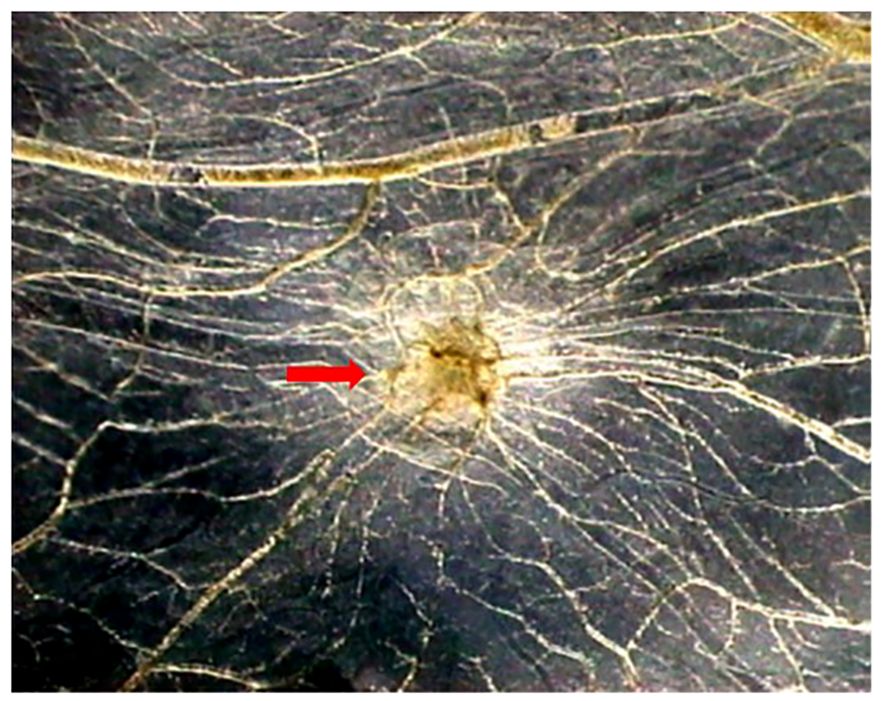
Figure 1. Tryptase is angiogenic in vivo in the CAM assay. Macroscopic picture of a CAM at day 12 of incubation treated with tryptase. Note the presence of numerous blood vessels converging toward the implant (arrow) (Modified from 42).
Tryptase is documented to play a role in tumor angiogenesis (Table 1). Elevation of different angiogenic factors, including VEGF, FGF-2, and platelet-derived growth factor (PDGF) in response to tryptase plays a key role in tumor angiogenesis (10). Tryptase acts on the proteinase-activated receptor-2 (PAR-2), stimulating tumor angiogenesis (44), and induces PAR-2. Tryptase induces PAR-2 proliferative effects on a human colon carcinoma cell line (45). PAR-2 leads to the release of IL-6 and granulocyte-macrophage colony-stimulating factor (GM-CSF), acting as angiogenic factors (46).
Tryptase-positive mast cell number increases parallelly with increased microvascular density in solid tumors, including malignant melanoma (47, 48), endometrial carcinoma (49, 53, 54), breast cancer (50, 56, 57), uterine leiomyomas (51), gastric cancer (52, 55, 58), colorectal cancer (61, 62), pancreatic ductal adenocarcinoma (59, 60, 63). In hematological tumors tryptase-positive mast cell count correlates with angiogenesis in multiple myeloma (64), in B cell non-Hodgkin’s lymphomas (65), in myelodysplastic syndrome (66) and B cell chronic lymphocytic leukemia (67, 68).
Therapeutic implications
Reducing mast cell number is a therapeutic approach in mastocytosis, characterized by mast cell accumulation in the skin (Figure 2) and other tissues, and other diseases characterized by an increase in mast cell number. Different pharmacological agents such as omalizumab, imatinib, disodium cromoglycate, H1 receptors antagonists, steroids, and non-steroidal anti-inflammatory drugs have been developed to modulate the functions of mast cells. The interplay between mast cells and tumor angiogenesis suggests considering the therapeutic use of inhibitors, which specifically target the angiogenic activity of tryptase. Cromolyn, an inhibitor of mast cell degranulation, reduces the expansion and survival of pancreatic cancer and endothelial cells (69). The combination of cromolyn with anti-angiogenic therapy increases the therapeutic efficacy (70). Tryptase inhibitors such as gabexate mesilate and nafamostat mesilate, two inhibitors of trypsin-like serine proteases (71–73) might be used as anti-angiogenic-agents through tryptase inhibition in combination with chemotherapy in the treatment of cancer (Table 2). Anti-angiogenic activity of gabexate mesilate in colon and pancreatic cancer may be due to a selective inhibition of mast cell tryptase (73–75). Nafamostat mesilate inhibits the tryptase-induced proliferation of tumor cells (45). Nafamostat exerts anti-angiogenic activity in pancreatic cancer through blockade of nuclear factor kappa-B (NF-kB) activation, which is mediated by tryptase through PAR-2 (76). Pancreatic cancer cell lines injected into nude mice with tryptase induced the formation of tumors larger than those developed in non-treated mice and nafamostat suppressed the tumorigenic effect of tryptase (77). Finally, different anti-cancer agents including sorafenib, sunitinib, pazopanib, axitinib, and masitinib, are all targeted against c-KitR, whose activation leads to the release of tryptase by mast cells.
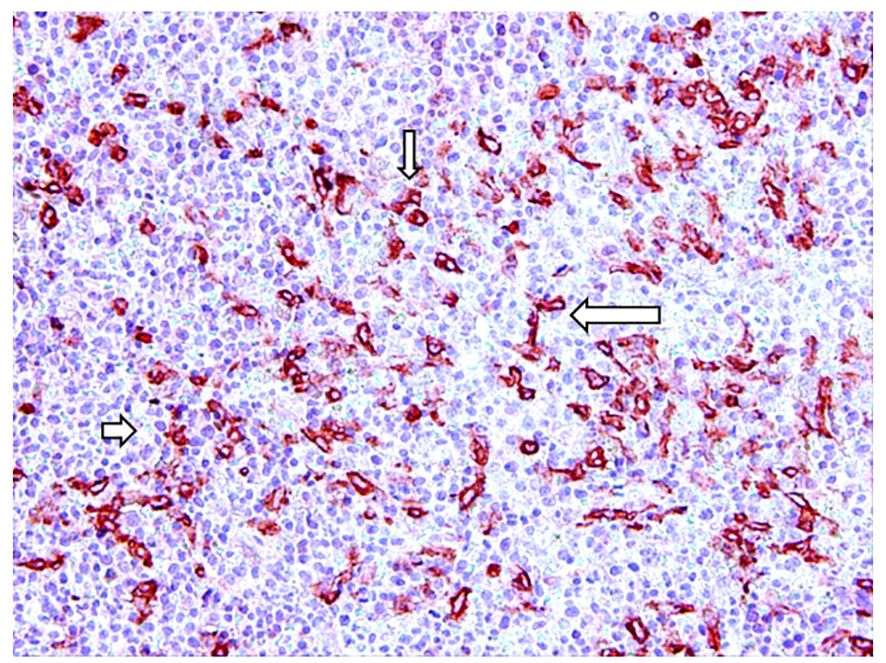
Figure 2. Tryptase-positive mast cells (arrows) in red in a bioptic specimen of human skin mastocytosis.
Concluding remarks
It is well established that mast cell accumulation accompanies most malignancies. However, the knowledge of how mast cells functionally impact tumors is still under investigation. Mast cells modulate the biological activity of immune and non-immune components of the tumor microenvironment through the release of a plethora of mediators, including tryptase. In this context, despite the critical role of tryptase in tumor growth and angiogenesis, the development of effective inhibitors has been a complex challenge, mainly due to the intricate mechanisms governing its activation and regulation. Although numerous tryptase inhibitors have been previously reported, it is important to note that these are largely in investigational stages and have not yet received FDA approval. It is conceivable that tryptase inhibitors might be combined with other novel anticancer approaches, such as anti-PD-1/PDL-1 therapy. The blockade of the PD-1/PD-L1 interaction has been suggested as a useful and novel therapeutic approach in the treatment of tumors in which mast cells are involved.
Author contributions
DR: Writing – original draft, Writing – review & editing.
Funding
The author(s) declare financial support was received for the research, authorship, and/or publication of this article. This work has been supported by the Association against non- Hodgkin lymphomas “Il Sorriso di Antonio”, Corato, Italy, and Associazione Italiana Contro le Leucemie, Linfomi e Mielomi (AIL), Bari, Italy.
Conflict of interest
The authors declares that the research was conducted in the absence of any commercial or financial relationships that could be construed as a potential conflict of interest.
Publisher’s note
All claims expressed in this article are solely those of the authors and do not necessarily represent those of their affiliated organizations, or those of the publisher, the editors and the reviewers. Any product that may be evaluated in this article, or claim that may be made by its manufacturer, is not guaranteed or endorsed by the publisher.
References
1. Folkman J. Angiogenesis in cancer, vascular, rheumatoid and other disease. Nat Med. (1995) 1:27–31. doi: 10.1038/nm0195-27
2. Rundhaug JE. Matrix metalloproteinases and angiogenesis. J Cell Mol Med. (2005) 9:267–85. doi: 10.1111/j.1582-4934.2005.tb00355.x
3. Noël A, Foidart JM. Role of serine proteases and their inhibitors in tumor angiogenesis. In: Foidart J-M, Muschel RJ, editors. Proteases and Their Inhibitors in Cancer Metastasis. Dordrecht: Kluwer Academic Publishers (2002). p. 23–38.
4. Sato Y. Aminopeptidases and angiogenesis. Endothelium. (2003) 10:287–90. doi: 10.1080/10623320390272334
5. Bauvois B. Transmembrane proteases in cell growth and invasion: new contributors to angiogenesis? Oncogene. (2004) 23:317–39. doi: 10.1038/sj.onc.1207124
6. Webb SL, Sanders AJ, Mason MD, Jiang WG. Type II transmembrane serine protease (TTSP) deregulation in cancer. Front Biosci. (2011) 16:539–52. doi: 10.2741/3704
7. Stefanini ACB, Rodrigues da Cunha B, Henrique T, Tajara EH. Involvement of kallikrein-related peptidases in normal and pathologic processes. Dis Markers. (2015) 2015:9465572. doi: 10.1155/2015/946572
8. Radisky ES. Extracellular proteolysis in cancer: Proteases, substrates, and mechanisms in tumor progression and metastasis. J Biol Chem. (2024) 300:107347. doi: 10.1016/j.jbc.2024.107347
9. Ribatti D, Crivellato E. The controversial role of mast cells in tumor growth. Int Rev Cell Mol Biol. (2009) 275:89–131. doi: 10.1016/S1937-6448(09)75004-X
10. Ribatti D, Crivellato E. Mast cells, angiogenesis, and tumour growth. Biochim Biophys Acta. (2012) 1822:2–8. doi: 10.1016/j.bbadis.2010.11.010
11. Sinnamon MJ, Carter KJ, Sims LP, Lafleur B, Fingleton B, Matrisian LM. A protective role of mast cells in intestinal tumorigenesis. Carcinogenesis. (2008) 29:880–66. doi: 10.1093/carcin/bgn040
12. Varicchi G, de Paulis A, Marone G, Galli SJ. Future need in mast cell biology. Int J Mol Sci. (2019) 20:4397. doi: 10.3390/ijms20184397
13. Moon TC, Befus AD, Kuka M. Mast cell mediators: their differential release and the secretory pathway involved. Front Immunol. (2014) 5:569. doi: 10.3389/fimmu.2014.00569
14. Triverdi NN, Caughey GH. Mast cell peptidase. Chameleons of innate immunity and host defense. Am J Respir Cell Mol Biol. (2010) 42:257–67. doi: 10.1165/rcmb.2009-0324RT
15. Schwartz LB, Irani AMA, Roller K, Castells MC, Schechter NM. Quantitation of histamine, tryptase and chymase in dispersed human T and TC mast cells. J Immunol. (1987) 138:2611–5. doi: 10.4049/jimmunol.138.8.2611
16. Castells M, Irani AM, Schwartz LB. Evaluation of human peripheral blood leukocytes for mast cell tryptase. J Immunol. (1987) 138:2184–9. doi: 10.4049/jimmunol.138.7.2184
17. Triverdi NN, Tong Q, Raman K, Bhagwandin VJ, Caughey GH. Mast cell α and β tryptases changed rapidly during primate speciation and evolved from γ-like transmembrane peptidases in ancestral vertebrates. J Immunol. (2007) 179:6072–9. doi: 10.4049/jimmunol.179.9.6072
18. Vitte J. Human mast cell tryptase in biology and medicine. Mol Immunol. (2015) 63:18–24. doi: 10.1016/j.molimm.2014.04.001
19. Peng Q, McEuen AR, Benyon RC, Walls AF. The heterogeneity of mast cell tryptase from human lung and skin. Eur J Biochem. (2003) 270:270–83. doi: 10.1046/j.1432-1033.2003.03385.x
20. Guida M, Riedy M, Lee D, Hall J. Characterization of two highly polymorphic human tryptase loci and comparison with a newly discovered monkey tryptase ortholog. Pharmacogenetics. (2000) 10:389–96. doi: 10.1097/00008571-200007000-00002
21. Greiner G, Sprinzl B, Górska A, Ratzinger F, Gurbisz M, Witzeneder N, et al. Hereditary α tryptasemia is a valid genetic biomarker for severe mediator-related symptoms in mastocytosis. Blood. (2021) 137:238–47. doi: 10.1182/blood.2020006157
22. Gentek R, Ghigo C, Hoeffel G, Bulle MJ, Msallam R, Gautier G, et al. Hemogenic endothelial fate mapping reveals dual developmental origin of mast cells. Immunity. (2018) 48:1160–71. doi: 10.1016/j.immuni.2018.04.025
23. Saito H, Nakajima T, Matsumoto K. Human mast cell transcriptome project. Int Arch Allergy Immunol. (2001) 125:1–8. doi: 10.1159/000053790
24. Welle M. Development, significance, and heterogeneity of mast cells with particular regard to mast cell specific proteases chymase and tryptase. J Leukoc Biol. (1997) 61:233–45. doi: 10.1002/jlb.61.3.233
25. He S, Gaça MD, Walls AF. A role for tryptase in the activation of human mast cells: modulation of histamine release by tryptase and inhibitors of tryptase. J Pharmacol Exp Ther. (1998) 286:289–97.
26. Thabrew H. Mast cell tryptase is a growth factor for human airway smooth muscle. J Allergy Clin Immunol. (1996) 97:969. doi: 10.1016/S0091-6749(96)80566-5
27. Ruoss SJ, Hartmann T, Caughey GH. Mast cell tryptase is a mitogen for cultured fibroblasts. J Clin Invest. (1991) 88:493–9. doi: 10.1172/JCI115330
28. Cairns JA, Walls AF. Mast cell tryptase stimulates the synthesis of type I collagen in human lung fibroblasts. J Clin Invest. (1997) 88:493–9. doi: 10.1172/JCI119290
29. Mangia A, Malfettone A, Rossi R, Paradiso A, Ranieri G, Simone G, et al. Tissue remodelling in breast cancer: human mast cell tryptase as an initiator of myofibroblast differentiation. Histopathology. (2011) 58:1096–106. doi: 10.1111/j.1365-2559.2011.03842.x
30. Schwartz LB. Clinical utility of tryptase levels in systemic mastocytosis and associate hematologic disorders. Leuk Res. (2001) 25:553–62. doi: 10.1016/S0145-2126(01)00020-0
31. Schwartz LB, Bradford TR, Rouse C, Irani AM, Rasp G, van der Zwan JK, et al. Development of a new, more sensitive immunoassay for human tryptase: use in systemic anaphylaxis. J Clin Immunol. (1994) 14:190–201. doi: 10.1007/BF01533368
32. Marech M, Ammendola R, Sacco GS, Capriuolo R, Patruno R, Rubini M, et al. Serum tryptase, mast cells positive to tryptase and microvascular density evaluation in early breast cancer patients: possible translational significance. BMC Cancer. (2014) 14:534–4. doi: 10.1186/1471-2407-14-534
33. Ammendola M, Sacco R, Sammarco G, Donato G, Montemurro S, Ruggieri E, et al. Correlation between serum tryptase, mast cells positive to tryptase and microvascular density in colorectal cancer patients: possible biological-clinical significance. PloS One. (2014) 9:e99512. doi: 10.1371/journal.pone.0099512
34. Crivellato E, Beltrami CA, Mallardi F, Ribatti D. Paul Ehrlich’s doctoral thesis: a milestone in the study of mast cells. Br J Haematol. (2003) 123:19–21. doi: 10.1046/j.1365-2141.2003.04573.x
35. Balkwill F, Mantovani A. Inflammation and cancer: back to Virchow? Lancet. (2001) 357:539–45. doi: 10.1016/S0140-6736(00)04046-0
36. Halova I, Draberova L, Draber P. Mast cell chemotaxis- chemoattractants and signaling pathways. Front Immunol. (2012) 3:119. doi: 10.3389/fimmu.2012.00119
37. Zhang W, Stoica G, Tasca SI, Kelly KA, Meininger CJ. Modulation of tumor angiogenesis by stem cell factor. Cancer Res. (2000) 60:6757–62.
38. Ribatti D. The development of human mast cells. An historical reappraisal. Exp Cell Res. (2016) 342:210–5. doi: 10.1016/j.yexcr.2016.03.013
39. Blair RJ, Meng H, Marchese MJ, Ren S, Schwartz LB, Tonnesen MG, et al. Human mast cells stimulate vascular tube formation. Tryptase is a novel, potent angiogenic factor. J Clin Invest. (1997) 99:2691–700. doi: 10.1172/JCI119458
40. Compton SJ, Cairns JA, Holgate ST, Walls AF. Human mast cell tryptase stimulates the release of an IL-8-dependent neutrophil chemotactic activity from human umbilical vein endothelial cells (HUVEC). Clin Exp Immunol. (2000) 121:31–6. doi: 10.1046/j.1365-2249.2000.01271.x
41. Johnson JL, Jackson CL, Angelini GD, George SJ. Activation of matrix-degrading metalloproteinases by mast cell proteases in atherosclerotic plaques. ArterThromb Vasc Biol. (1998) 18:1707–15. doi: 10.1161/01.ATV.18.11.1707
42. Ribatti D, Ranieri G, Nico B, Benagiano V, Crivellato E. Tryptase and chymase are angiogeni in vivo in the chorioallantoic membrane assay. Int J Dev Biol. (2011) 55:99–102. doi: 10.1387/ijdb.103138dr
43. Zhi X, Xu C, Zhang H, Tian D, Li X, Ning Y, et al. Tryptase promotes atherosclerotic plaque haemorrhage in ApoE-/- mice. PloS One. (2013) 8:e60960. doi: 10.1371/journal.pone.0060960
44. Ammendola M, Leporini C, Marech I, Gadaleta CD, Scognamillo G, Sacco R, et al. Targeting mast cells tryptase in tumor microenvironment: A potential antiangiogenetic strategy. BioMed Res Int. (2014) 2014:154702. doi: 10.1155/2014/154702
45. Yoshii M, Jikuhara A, Mori S, Iwagaki H, Takahashi HK, Nishibori M, et al. Mast cell tryptase stimulates DLD-1 carcinoma through prostaglandin- and MAP kinase-dependent manners. J Pharmacol Sci. (2005) 98:450–8. doi: 10.1254/jphs.FPJ05002X
46. Liu Y, Mueller BM. Protease-activated receptor-2 regulates vascular endothelial growth factor expression in MDAMB-231 cells via MAPK pathways. Biochem Biophys Res Commun. (2006) 344:1263–70. doi: 10.1016/j.bbrc.2006.04.005
47. Ribatti D, Ennas MG, Vacca A, Ferreli F, Nico B, Orru S, et al. Tumor vascularity and tryptase-positive mast cells correlate with a poor prognosis in melanoma. DD Eur J Clin Invest. (2003) 33:420–5. doi: 10.1046/j.1365-2362.2003.01152.x
48. Ribatti D, Vacca A, Ria R, Marzullo A, Nico B, Filotico R, et al. Neovascularisation, expression of fibroblast growth factor-2, and mast cells with tryptase activity increase simultaneously with pathological progression in human Malignant melanoma. Eur J Cancer. (2003) 39:666–74. doi: 10.1016/S0959-8049(02)00150-8
49. Ribatti D, Finato N, Crivellato E, Marzullo A, Mangieri D, Nico B, et al. Neovascularization and mast cells with tryptase activity increase simultaneously with pathologic progression in human endometrial cancer. Am J Obstet Gynecol. (2005) 193:1961 1965. doi: 10.1016/j.ajog.2005.04.055
50. Ribatti D, Finato N, Crivellato E, Guidolin D, Longo V, Mangieri D, et al. Angiogenesis and mast cells in human breast cancer sentinel lymph nodes with and without micrometastases. Histopathology. (2007) 51:837–42. doi: 10.1111/j.1365-2559.2007.02869.x
51. Ribatti D, Belloni AS, Nico B, Salà G, Longo V, Mangieri D, et al. Tryptase- and leptin-positive mast cells correlate with vascular density in uterine leiomyomas. Am J Obstet Gynecol. (2007) 196:1–7. doi: 10.1016/j.ajog.2006.12.039
52. Ribatti D, Guidolin D, Marzullo A, Nico B, Annese T, Benagiano V, et al. Mast cells and angiogenesis in gastric carcinoma. Int J Exp Pathol. (2010) 91:350–6. doi: 10.1111/j.1365-2613.2010.00714.x
53. Benitez-Bribiesca L, Wong A, Utrera D, Castellanos E. The role of mast cell tryptase in neoangiogenesis of premalignant and Malignant lesions of the uterine cervix. J Histochem Cytochem. (2001) 49:1061–2. doi: 10.1177/002215540104900816
54. Guidolin D, Marinaccio C, Tortorella C, Annese T, Ruggieri S, Finato N, et al. Non-random spatial relationships between mast cells and microvessels in human endometrial carcinoma. Clin Exp Med. (2017) 17:71–7. doi: 10.1007/s10238-016-0407-4
55. Guidolin D, Ruggieri S, Annese T, Tortorella C, Marzullo A, Ribatti D. Spatial distribution of mast cells around vessels and glands in human gastric carcinoma. Clin Exp Med. (2017) 17:531–9. doi: 10.1007/s10238-017-0452-7
56. Ranieri G, Ammendola M, Patruno R, Celano G, Zito FA, Montemurro S, et al. Tryptase-positive mast cells correlate with angiogenesis in early breast cancer patients. Int J Oncol. (2009) 35:115–20. doi: 10.3892/ijo_00000319
57. Cimpean AM, Tamma R, Ruggieri S, Nico B, Toma A, Ribatti D. Mast cells in breast cancer angiogenesis. Crit Rev Oncol Hematol. (2017) 115:23–6. doi: 10.1016/j.critrevonc.2017.04.009
58. Ammendola M, Sacco R, Sammarco G, Donato G, Zuccalà V, Romano R, et al. Mast cells positive to tryptase and c-kit receptor expressing cells correlates with angiogenesis in gastric cancer patients surgically treated. Gastroenterol Res Pract. (2013) 2013:703163. doi: 10.1155/2013/703163
59. Ammendola M, Gadaleta CD, Frampton AE, Piardi T, Memeo R, Zuccala V, et al. The density of mast cells c-Kit(+) and tryptase(+) correlates with each other and with angiogenesis in pancreatic cancer patients. Oncotarget. (2017) 8:70463–71. doi: 10.18632/oncotarget.19716
60. Ammendola M, Currò G, Laface C, Zuccalà V, Memeo R, Luposella F, et al. Mast cells positive for c-kit receptor and tryptase correlate with angiogenesis in cancerous and adjacent normal pancreatic tissue. Cells. (2021) 10:444. doi: 10.3390/cells10020444
61. Gulubova M, Vlaykova T. Prognostic significance of mast cell number and microvascular density for the survival of patients with primary colorectal cancer. J Gastroenterol Hepatol. (2009) 24:1265–75. doi: 10.1111/j.1440-1746.2007.05009.x
62. Acikalin MF, Oner U, Topcu I, Yasar B, Kiper H, Colak E. Tumour angiogenesis and mast cell density in the prognostic assessment of colorectal carcinomas. Dig Liver Dis. (2005) 37:162–9. doi: 10.1016/j.dld.2004.09.028
63. Esposito M, Menicagli N, Funel F, Bergmann U, Boggi F, Mosca G, et al. Inflammatory cells contribute to the generation of an angiogenic phenotype in pancreatic ductal adenocarcinoma. J Clin Pathol. (2004) 57:630–6. doi: 10.1136/jcp.2003.014498
64. Ribatti D, Vacca A, Nico B, Quondamatteo F, Ria R, Minischetti M, et al. Bone marrow angiogenesis and mast cell density increase simultaneously with progression of human multiple myeloma. Br J Cancer. (1999) 79:451–5. doi: 10.1038/sj.bjc.6690070
65. Ribatti D, Vacca A, Marzullo A, Nico B, Ria R, Roncali L, et al. Angiogenesis and mast cell density with tryptase activity increase simultaneously with pathological progression in B-cell non-Hodgkin's lymphomas. Int J Cancer. (2000) 85:171–5. doi: 10.1002/(SICI)1097-0215(20000115)85:2%3C171::AID-IJC4%3E3.0.CO;2-W
66. Ribatti D, Polimeno G, Vacca A, Marzullo A, Crivellato E, Nico B, et al. Correlation of bone marrow angiogenesis and mast cells with tryptase activity in myelodysplastic syndromes. Leukemia. (2002) 16:1680–4. doi: 10.1038/sj.leu.2402586
67. Ribatti D, Molica S, Vacca A, Nico B, Crivellato E, Roccaro AM, et al. Tryptase positive mast cells correlate positively with bone marrow angiogenesis in B-cell chronic lymphocytic leukemia. Leukemia. (2003) 17:1428–30. doi: 10.1038/sj.leu.2402970
68. Molica S, Vacca A, Crivellato E, Cuneo A, Ribatti D. Tryptase-positive mast cells predict clinical outcome of patients with early B-cell chronic lymphocytic leukemia. Eur J Haematol. (2003) 71:137–9. doi: 10.1034/j.1600-0609.2003.00110.x
69. Soucek L, Lawlor ER, Soto D, Shchors K, Swigart LB, Evan GI. Mast cells are required for angiogenesis and macroscopic expansion of Myc-induced pancreatic islet tumors. Nat Med. (2007) 13:1211–8. doi: 10.1038/nm1649
70. Cimpean AM, Raica M. The hidden side of disodium cromolyn: from mast cell stabilizer to an angiogenic factor and antitumor agent. Arch Immunol Ther Exp. (2016) 64:515–22. doi: 10.1007/s00005-016-0408-8
71. Erba F, Fiorucci L, Pascarella S, Menegatti E, Ascenzi P, Ascoli F. Selective inhibition of human mast cell tryptase by gabexate mesylate, an antiproteinase drug. Biochem Pharmacol. (2001) 61:271–6. doi: 10.1016/S0006-2952(00)00550-5
72. Mori S, Itoh Y, Shinohata R, Sendo T, Oishi R, Nishibori M. Nafamostat mesilate is an extremely potent inhibitor of human tryptase. J Pharmacol Sci. (2003) 92:420–3. doi: 10.1254/jphs.92.420
73. Uchima Y, Sawada T, Hirakawa K. Action of antiproteases on pancreatic cancer cells. J Pancreas. (2007) 8:479–87.
74. Yoon WH, Jung YJ, Kim TD, Li G, Park BJ, Kim YY, et al. Gabexate mesilate inhibits colon cancer growth, invasion, and metastasis by reducing matrix metalloproteinases and angiogenesis. Clin Cancer Res. (2004) 10:4517–26. doi: 10.1158/1078-0432.CCR-04-0084
75. Brandi G, Tavolari S, de Rosa F, Di Girolamo S, Agostini V, Barbera MA, et al. Antitumoral efficacy of the protease inhibitor gabexate mesilate in colon cancer cells harbouring KRAS, BRAF and PIK3CA mutations. PloS One. (2012) 7:e41347. doi: 10.1371/journal.pone.0041347
76. Zhang S, Zeng X, Yang H, Hu G, He S. Mast cell tryptase induces microglia activation via protease-activated receptor 2 signaling. Cell Physiol Biochem. (2012) 29:931–40. doi: 10.1159/000171029
77. Guo X, Zhai L, Xue R, Shi J, Zeng Q, Gao C. Mast cell tryptase contributes to pancreatic cancer growth through promoting angiogenesis via activation of angiopoietin-1. Int J Mol Sci. (2016) 17:834. doi: 10.3390/ijms17060834
Keywords: angiogenesis, mast cells, tryptase, tumor growth, proteases
Citation: Ribatti D (2024) Tryptase and tumor angiogenesis. Front. Oncol. 14:1500482. doi: 10.3389/fonc.2024.1500482
Received: 24 September 2024; Accepted: 26 November 2024;
Published: 19 December 2024.
Edited by:
Anca Maria Cimpean, Victor Babes University of Medicine and Pharmacy, RomaniaReviewed by:
Kshama Gupta, Mayo Clinic, United StatesCopyright © 2024 Ribatti. This is an open-access article distributed under the terms of the Creative Commons Attribution License (CC BY). The use, distribution or reproduction in other forums is permitted, provided the original author(s) and the copyright owner(s) are credited and that the original publication in this journal is cited, in accordance with accepted academic practice. No use, distribution or reproduction is permitted which does not comply with these terms.
*Correspondence: Domenico Ribatti, ZG9tZW5pY28ucmliYXR0aUB1bmliYS5pdA==