- 1Department of Gynecology, People’s Hospital of Liaoning Province, Shenyang, China
- 2Liaoning University of Traditional Chinese Medicine, Shenyang, China
Endometriosis (EMs) is a prevalent chronic gynecological condition that depends on estrogen, marked by the presence of active endometrial tissue (glands and stroma) outside the uterus. Although pathologically benign, it exhibits biological behaviors such as invasion and metastasis akin to malignant tumors. Endometriosis-associated ovarian carcinoma (EAOC), arising from malignant transformation of EMs, poses significant clinical challenges. However, the mechanisms underlying EAOC pathogenesis remain incompletely understood, with a lack of reliable biomarkers for early diagnosis and personalized treatment strategies. Considering the significant number of EMs patients and the extended period during which malignant transformation can occur, EAOC deserves significant attention. Current research both domestically and internationally indicates that the pathogenesis of EAOC is complex, involving genetic mutations, immune microenvironment, oxidative stress, epigenetic changes, and related areas. This review summarizes the mechanisms underlying the development of EAOC.
1 Introduction
EMs is a chronic inflammatory disease characterized by the presence of ectopic lesions, most commonly in the ovaries, and presenting with symptoms such as pelvic pain, dysmenorrhea, and infertility (1). It affects 10-18% of women of reproductive age worldwide (2). Despite its benign nature, EMs exhibits biological features akin to malignancy, including histological infiltration and angiogenesis. EAOC, resulting from malignant transformation of EMs, refers to specific types of ovarian cancer (3). Epidemiological studies indicate that EMs increases the risk of developing certain histological subtypes of ovarian cancer, predominantly ovarian clear cell carcinoma (OCCC) and ovarian endometrioid carcinoma (OEC) (4). The lifetime risk of ovarian cancer in EMs patients is modest (~1.9%), yet higher compared to the general female population (~1.4%) (5). Furthermore, clinical diagnosis is complicated by rapid tumor growth that may obscure benign tissues, and challenges in pathological sampling which can lead to underdiagnosis of coexisting benign or atypical EMs lesions in EAOC patients (6). This underscores the difficulty in studying EAOC comprehensively. Early detection of malignant transformation of EMs and identification of early events are crucial for improving prognosis in EAOC. Therefore, understanding the mechanisms and biomarkers associated with EMs malignant transformation is essential. This review discusses current insights into the mechanisms underlying EAOC development, and outlines future directions for basic research and clinical translation, aiming towards stratified management and personalized treatment of ovarian cancer patients.
2 Malignant transformation mechanism
2.1 Genetic mutations
AT-Rich Interaction Domain 1A (ARID1A), a tumor suppressor gene encoding the BAF Family Member 250a(BAF250a) protein, is a critical component of the SWI/SNF chromatin remodeling complex. It is the most commonly mutated gene within the SWI/SNF complex, and its loss-of-function mutations play a crucial role in dysregulating the expression of oncogenic and tumor suppressor genes, particularly in the context of EAOC (7). Genetic sequencing studies have revealed ARID1A mutations in 46% of OCCC patients and 30% of OEC patients. Previous research has shown that ARID1A mutations promote abnormal activation of the PI3K-Akt signaling pathway and interact synergistically with zinc-finger protein 217 (ZNF217), which may contribute to OCCC development (8). The PI3K/Akt signaling pathway is a pivotal pathway involved in tumor cell proliferation, invasion, and metastasis (9). Guan et al. demonstrated that ARID1A, functioning as a tumor suppressor gene, interacts with the p53 protein to inhibit cell proliferation through p53-dependent transcriptional regulation factors. Mutations in either Tumor Protein P53 or ARID1A can disrupt tumor-suppressive transcription, leading to uncontrolled cell proliferation and ultimately promoting EAOC development (10).
Phosphatase And Tensin Homolog (PTEN), located on chromosome 10, is a tumor suppressor gene involved in cell regulation, inhibiting tumor cell proliferation, adhesion, migration, and angiogenesis (11). Mutations in PTEN enhance the anti-apoptotic capability of endometrial cells, disrupt cell cycle regulation mechanisms, and promote retrograde implantation and survival of endometrial tissue (12). Another study indicates that low PTEN expression increases the invasive capacity of endometrial cells, facilitating the growth of ectopic endometrial tissue (13). Research by Dinulescu et al. demonstrates that ovarian surface epithelium-specific oncogenic K-ras or conditional PTEN loss of expression can lead to atypical hyperplasia resembling endometrioid adenocarcinoma structures, suggesting mutations and expression loss may be early drivers of OEC development (14).
Phosphatidylinositol-4,5-Bisphosphate 3-Kinase Catalytic Subunit Alpha (PIK3CA), a member of the PI3K-AKT-mTOR signaling pathway, is closely implicated in OCCC pathogenesis through its mutations (15). Activation of PIK3CA promotes expression of downstream genes in the PI3K-Akt pathway, inhibiting tumor cell apoptosis and facilitating tumor initiation and progression (16). Furthermore, PIK3CA mutations enhance PI3K activity, promoting cell cycle progression, proliferation, and migration (17). Co-mutation of PIK3CA and ARID1A enhances sustained IL-6 production, driving rapid tumor growth (18). A study by Chandler et al. in mice demonstrated that concurrent activation of mutated ARID1A and PIK3CA genes induces OCCC development (19).
The KRAS Proto-Oncogene (KRAS) is a major subtype of RAS mutations, encoding a signaling protein that can be activated by various extracellular stimuli and transduce signals through the downstream RAS/MAPK signaling pathway, promoting malignant biological behaviors such as cell proliferation, invasion, and migration (20, 21). Studies have found that 42.6% of EMs cases exhibit somatic KRAS mutations (22). Somatic mutations in KRAS may represent critical driver events during the malignant transformation of EMs (20). To date, all KRAS mutations detected in EMs have been located within “hotspot” sequences, indicating biological significance of KRAS hotspot mutations in EMs. DNA sequencing results suggest that KRAS mutations may represent a late event in the malignant progression of EMs, associated with OCCC occurrence (23). Overexpression of oncogenic KRAS in ovarian surface epithelium or conditional PTEN loss can lead to precancerous changes in endometrial glandular morphology; their concurrent presence can induce the development of OEC (24) (Figure 1).
The KRAS Proto-Oncogene (KRAS), a major mutant subtype of RAS, encodes a signaling protein activated by various extracellular stimuli, which transmits signals to the downstream RAS/MAPK signaling pathway. The tumor suppressor gene P53 (TP53) mutations impact the expression of its transcription-dependent tumor suppressor genes. Phosphatidylinositol-4,5-Bisphosphate 3-Kinase Catalytic Subunit Alpha (PIK3CA) activation enhances the expression of downstream PI3K-Akt pathway genes, inhibiting apoptosis and promoting tumor development. Additionally, AT-Rich Interaction Domain 1A (ARID1A), a frequently mutated gene in the SWItch/Sucrose Non-Fermentable(SWI/SNF) complex, leads to abnormal activation of the PI3K-Akt signaling pathway. Gene mutations play a critical role in the malignant transformation of endometrial cells by affecting DNA damage repair, cell proliferation, and invasion. (Created with BioRender.com).
2.2 Steroid hormone action
As is widely recognized, EMs is an estrogen-dependent disease, with estrogen and progesterone playing crucial roles in maintaining normal uterine endometrial function. whereas in ectopic endometrial tissue compared to normal endometrium, mRNA levels of the Cytochrome P450 Family 1 Subfamily B Member 1(CYP1B1) gene are significantly elevated (25). CYP1B1 participates in estrogen metabolism within the ectopic endometrial microenvironment, converting androgens such as androstenedione or testosterone into estriol and estradiol (25). High concentrations of estrogens can exert direct toxic effects on DNA of ectopic endometrial cells. Additionally, estrogens play critical roles in cell survival, proliferation, and inflammatory responses (26). Substantial research suggests that dysregulations in estrogen receptor (ER) and progesterone receptor (PR) expression are linked to the malignant progression of endometriosis (27). Exposure to estrogens, such as via hormone replacement therapy (HRT) after menopause, elevates the likelihood of ovarian cancer, notably endometrioid carcinoma (28). Conversely, epidemiological studies show that oral contraceptives can reduce the risk of ovarian cancer (29). Therefore, studying the role of steroid hormones in the malignant transformation of EMs is crucial for developing corresponding therapeutic strategies aimed at preventing its malignant progression.
2.3 Inflammation and oxidative stress
During menstruation, declining levels of estrogen and progesterone prepare the uterus for the shedding and expulsion of endometrial tissue, marking the onset of a new menstrual cycle. This carefully coordinated process engages the innate immune system, where neutrophils, macrophages, and natural killer cells collaborate to aid in the clearance of apoptotic endometrial tissue (30). Retrograde menstruation can lead to the retention of biologically functional ectopic endometrial fragments within the peritoneal cavity, triggering periodic immune reactions (31). Research indicates heightened levels of inflammatory cytokines, growth factors, neutrophils, and prostaglandins in the peritoneal fluid of EMs patients (32). Elevated levels of Prostaglandin E Receptor 2(PGE2), tumor necrosis factor-α (TNF-α), nerve growth factor (NGF), C-C Motif Chemokine Ligand 5(CCL5), interleukins (IL), Interleukin8(IL-8), and Interleukin 1β(IL-1β)within ectopic lesions can activate sensory nerve endings, thereby contributing to chronic pelvic pain (33–35), which may transmit pain sensations to the central nervous system (36). Therefore, understanding cellular inflammatory responses is crucial for elucidating the pathological processes of EMs, offering insights into potential therapeutic strategies for this condition.
The extracellular matrix cells of ectopic endometrium are often in a microenvironment characterized by iron overload, with high levels of free iron in cyst fluid. Iron can catalyze Fenton reactions generating hydroxyl radicals, which damage DNA and other biomolecules, promoting carcinogenesis (37). Fenton reaction accelerates the production of reactive oxygen species (ROS), oxidative stress resulting from imbalance between excessive ROS and antioxidant defense systems within cells (38). Superoxide anions and hydrogen peroxide (H2O2) are principal components of ROS, closely linked to inflammation and extracellular matrix degradation. Oxidative stress plays a crucial role in the pathogenesis of EMs (38), damaging normal cell functions, causing DNA damage, stimulating angiogenesis, and thereby promoting EAOC (39). Excessive oxidative stress activates the NF-κB signaling pathway, promoting the production of inflammatory mediators, exacerbating the pathological processes of EMs through Th1 and Th2 immune responses (40). Interleukin10(IL-10) plays a pivotal role in this process by modulating Matrix Metallopeptidase (MMP) activation in serum and peritoneal fluid, ECM remodeling, and angiogenesis. Increased oxidative stress may upregulate oncogenic genes like Cytochrome C Oxidase-2 (COX-2), inhibiting apoptosis and promoting excessive proliferation of ectopic cells (41).Furthermore, oxidative stress enhances the expression of glycoproteins to stimulate vascular endothelial growth factor (VEGF) production, fostering neovascularization and aggravating the disease process (42). Additionally, ROS-activated ERK1/2 protein kinase alters the proliferation and survival of endometrial cells, exhibiting malignant biological behaviors akin to tumor cells (43). In conclusion, the development of EMs is closely associated with oxidative stress, involving multiple cell signaling pathways and regulation of inflammatory mediators, providing a theoretical basis for future therapeutic strategies (Figure 2).
Changes in the ovarian microenvironment can induce malignant transformation of EMs. Excessive accumulation of estrogen and the imbalance between estrogen receptor-α (ERα) and estrogen receptor-β(ERβ) can cause DNA toxicity in ectopic endometrial cells. Additionally, oxidative stress plays a crucial role in EAOC formation by inducing DNA damage, abnormal cell signaling, and disrupting antioxidant defenses through excessive intracellular reactive oxygen species (ROS). Elevated levels of inflammatory cytokines, such as interleukin (IL) and tumor necrosis factor-α (TNF-α), along with increased Prostaglandin E Receptor 2(PGE2) at ectopic sites, can activate sensory nerve endings at the lesion site, leading to chronic pelvic pain. (Created with BioRender.com).
2.4 Epigenetic changes
Unlike genetic mutations, epigenetics does not alter the DNA sequence of genes but influences gene expression through mechanisms such as DNA methylation, histone modifications, and abnormal expression of microRNAs. Current research indicates that epigenetics plays a crucial role in the onset and progression of ovarian cancer, with its aberrant modifications closely associated with the grading and staging of the disease (44).
2.4.1 DNA methylation
High methylation of gene promoter regions can lead to gene silencing, whereas low methylation promotes gene transcription and protein expression. In studies of EMs malignant transformation, abnormal methylation of CpG islands (CGIs) in many cancer-related genes has been identified, indicating a significant role of epigenetic changes in this process (45). Research has demonstrated high methylation in the promoter region of the E-cadherin gene (46), as well as loss of PTEN homologs on chromosome 10 and p16, which promote the malignant transformation of EMs (47). Additionally, abnormal methylation in the promoter regions of Long Interspersed Elements-1 (LINE-1) and interleukin-1 is also associated with this process (48). Researchers have found that abnormal methylation plays a critical role in the malignant transformation of EMs. Using Methylation CpG Island Amplification Combined with Differential Analysis (MCA-RDA), nine differentially methylated candidate genes associated with the malignant transformation of ovarian EMs were successfully screened, including Ras Association Domain Family Member 2(RASSF2), SPARC (Osteonectin), Cwcv And Kazal Like Domains Proteoglycan 2(SPOCK2), RUNX Family Transcription Factor 3(RUNX3), Glutathione S-Transferase Zeta 1(GSTZ1), Cytochrome P450 Family 2 Subfamily A (CYP2A), Globoside Alpha-1,3-N-Acetylgalactosaminyltransferase 1(GBGT1), NADH: Ubiquinone Oxidoreductase Core Subunit S1(NDUFS1), ADAM Metallopeptidase Domain 22(ADAM22), and Tripartite Motif Containing 36(TRIM36) (49). The DNA methyltransferase (DNMT) family plays a crucial role in mediating DNA methylation. Fang et al. found that estrogen upregulates DNMT1, promoting high methylation of the RUNX3 promoter and playing an important role in the malignant transformation of EMs (50). Thus, abnormal methylation, particularly in promoter regions, is a common epigenetic event in the malignant transformation of EMs, playing a significant role in EMs progression.
2.4.2 Histone modifications
Histones play a crucial role in chromatin structure by influencing the packaging of DNA around nucleosomes and controlling gene expression. Examples of post-translational modifications (PTMs) on histones include acetylation, phosphorylation, methylation, ubiquitination, among others (51). Among these, histone acetylation and methylation are the most extensively studied. Although there is no definitive evidence linking histone modifications to the process of EMs malignant transformation, intriguingly, inhibition of the methyltransferase Enhancer Of Zeste 2 Polycomb Repressive Complex 2 Subunit(EZH2) and the acetyltransferase Histone Deacetylase (HDAC), which regulate histone modifications, can lead to tumor regression in ovarian cancer mouse models with ARID1A mutations (52). Mutations in the ARID1A gene are found in over 50% of OCCCs (53), suggesting a potential role of histone modifications in the malignant transformation of EMs. Studies indicate significant upregulation of histone deacetylases and their encoded proteins in EMs and ovarian cancer (OC) (54, 55). Additionally, histone methyltransferase enhancer of EZH2 can activate methylation of lysine 27 on histone H3 within nucleosomes, promoting cell proliferation and suppressing transcription of tumor suppressor genes in both conditions (56). Thus, these findings imply a potential correlation of histone modifications between EMs and OC, suggesting these modifications may contribute to the progression of epithelial ovarian malignancy.
2.4.3 MicroRNA
miRNA is a class of short, endogenous single-stranded RNA molecules, typically ranging in length from 19 to 25 nucleotides. At the level of the endometrium, miRNA plays a role in regulating the pathophysiological dynamics associated with the menstrual cycle and reproductive disorders such as EMs and recurrent miscarriage (57). These RNA fragments function by inhibiting the synthesis of specific proteins, thereby influencing various biological processes including cell growth, development, differentiation, metabolism, aging, inflammation, and immune responses (58). Despite extensive literature reviews on the importance of miRNA in EMs and its malignant transformation, consensus on research findings remains elusive.
Common miRNA dysregulations in EMs include the miR-200 family, miR-143, miR-145, miR-20a, and miR-199a. Particularly, dysregulation of the miR-200 family is observed in both EMs and its malignant transformation, suggesting its close association with EMs progression (59). Furthermore, studies have focused on exploring miRNA expression profiles in different tissue types of malignant transformation of EMs. It has been found that miR-21, miR-203, and miR-205 are significantly upregulated in OEC, while miR-222 is downregulated (60). A second-generation sequencing study has also reported specific upregulation of miRNAs such as miR-9, 96, 182, 183, 196a, 196b, 205, and 375 in OEC, and miR-30a, 30a*, and 486-5p in OCCC (61). Additionally, research indicates that oxidative stress, as an important factor inducing malignant transformation of EMs, interacts with miRNAs to affect multiple processes in the development of EAOC (62). Thus, identifying specific miRNAs that promote the malignant transformation of EMs and exploring related molecular pathways may offer new strategies for preventing this progression (Figure 3).
Abnormal hypermethylation of tumor suppressor gene promoters, such as E-cadherin and Phosphatase And Tensin Homolog (PTEN), inhibits tumor suppressor gene expression, while hypomethylation of oncogenes like Long Interspersed Elements-1 (LINE-1) promotes oncogene expression and is associated with EAOC development. Inhibition of methyltransferase Repressive Complex 2 Subunit(EZH2)and acetyltransferase Histone Deacetylase(HDAC), which are involved in regulating histone modifications, can lead to tumor regression in AT-Rich Interaction Domain 1A (ARID1A) mutant ovarian cancer mouse models. ARID1A is a commonly mutated gene in EAOC, suggesting that histone modifications may play a role in EAOC. Additionally, microRNAs are crucial post-transcriptional regulators of gene expression; miR-21, miR-203, and miR-205 are significantly upregulated in ovarian epithelial carcinoma (OEC), while miR-222 is downregulated. Conversely, miR-30a, miR-30a*, and miR-486-5p are significantly upregulated in ovarian clear cell carcinoma (OCCC). (Created with BioRender.com).
3 Treatment options
Current treatment approaches for EAOC are primarily informed by research on epithelial ovarian cancer (EOC). The standard initial treatment for EAOC includes adjuvant platinum-based chemotherapy following surgery (63). If first-line chemotherapy fails or disease recurs, salvage chemotherapy is administered. However, the prognosis for advanced and recurrent cases is generally poor, and chemotherapy efficacy significantly declines (64). Therefore, the current treatment strategy focuses on enhancing the efficacy of initial treatments, particularly through improved surgical quality and the integration of chemotherapy drugs, targeted therapies, or immunotherapy.
Despite ongoing advancements, clinical outcomes for OCCC remain poorer compared to those for OEC, largely due to its strong resistance to chemotherapy (65). Researchers have long aimed to improve the treatment outcomes for advanced ovarian cancer through intraperitoneal chemotherapy. Despite the completion of several early clinical trials by the Gynecologic Oncology Group (GOG), including GOG-104,GOG-172, and GOG-252, intraperitoneal chemotherapy has not yet been established as a standard first-line treatment (66–68). This is largely because of issues related to trial design, limited statistical evidence, and the need for more investigation into potential adverse effects. Additionally, global clinical trials, such as JGOG-3016, GOG-262, MITO-7, and ICON-8, have adjusted the cycle and frequency of paclitaxel chemotherapy from every three weeks to weekly, or weekly with carboplatin (69–71). These adjustments have their own advantages and disadvantages, and their impact on treatment efficacy needs further clinical trial validation.
Currently, there is no definitive standard for targeted therapy in EAOC. However, targeted therapies may play a crucial role in improving the prognosis of OCCC, particularly in cases resistant to chemotherapy (72). Furthermore, immunotherapy related to EAOC remains a hot research topic.
PIK3CA is a commonly mutated gene in EAOC, which activates the PI3K/AKT/mTOR pathway (73). Inhibitors targeting this pathway, such as PI3K, AKT, and mTORC1 inhibitors, have been evaluated in clinical trials (74), but no standard treatment drug has emerged yet. However, the potential for targeted therapy remains promising. A study involving exome sequencing of 48 OCCC patients indicated that existing molecular targeted drugs may be effective for some patients, highlighting the value of exome sequencing in EAOC research (75).
Additionally, PARP inhibitors have demonstrated efficacy in the treatment of ovarian cancer (76), particularly in populations with homologous recombination deficiencies due to BRCA1/BRCA2 gene mutations (77). Although only 6-8% of EAOC cases have BRCA1/2 mutations (78), PARP inhibitors might be effective in these mutation cases.
In EAOC, the expression of the angiogenesis factor VEGF is significantly elevated compared to EMs (79). Anti-VEGF antibodies, as angiogenesis inhibitors, play a role in ovarian cancer treatment (80).There is no evidence suggesting that anti-VEGF antibodies are more effective for EAOC compared to other histological types, their potential for combination with other drugs may be further explored in future treatments (81).
(Created with BioRender.com).
4 Discussion
Despite ongoing interest in the study of EAOC, progress remains slow. The diagnostic criteria for endometriosis-associated malignancy have long adhered to those established by Sampson and Scott (82). However, given the strong pathological heterogeneity of EMs, the probability of detecting residual ectopic endometrial cells decreases with disease severity. Notably, the disordered growth of tumor cells following malignancy and the potential oversight of atypical or ectopic endometrial cells can lead to misdiagnosis as primary ovarian cancer, significantly increasing the likelihood of such errors. Consequently, the foundational research on EAOC is heavily constrained by the availability of clinical samples, unlike other cancers. Present challenges include the unclear pathogenesis of EMs, lack of characteristic clinical symptoms and non-invasive diagnostic methods, and difficulties in predicting the risk of endometriosis malignancy. Therefore, further research into the relevant molecular mechanisms is urgently needed to guide clinical treatment. This review provides a comprehensive overview of the mechanisms underlying EAOC and the latest therapeutic strategies. There have been relatively few high-quality reviews on EAOC in recent years, with some focusing solely on specific mechanisms. This article updates the relevant literature and mechanism diagrams, offering guidance for further research into EAOC.
5 Summary
EMs, due to its progressive and invasive growth characteristics, is considered to have potential malignant features. The process of malignant transformation in EMs is complex and may result from various factors acting alone or in combination, including estrogenic effects, immune microenvironment, persistent inflammatory responses, oxidative stress, and genetic mutations (Figure 4). For populations of EMs patients at high risk of malignant transformation, the development of early detection methods and close monitoring is crucial to achieve early detection, diagnosis, and treatment, thereby improving patient prognosis and survival outcomes. In-depth analysis of the pathogenesis and malignant transformation mechanisms of EMs can provide new perspectives and strategies for diagnosis and treatment. Future research could explore advanced technologies like next-generation sequencing and whole transcriptome sequencing for personalized diagnostics, aiming to identify driver mutations and candidate genes linked to EMs’ malignant transformation. Such endeavors may enhance the precision of targeted therapies and immunotherapeutic options for EMs patients, underscoring the need for continued investigation.
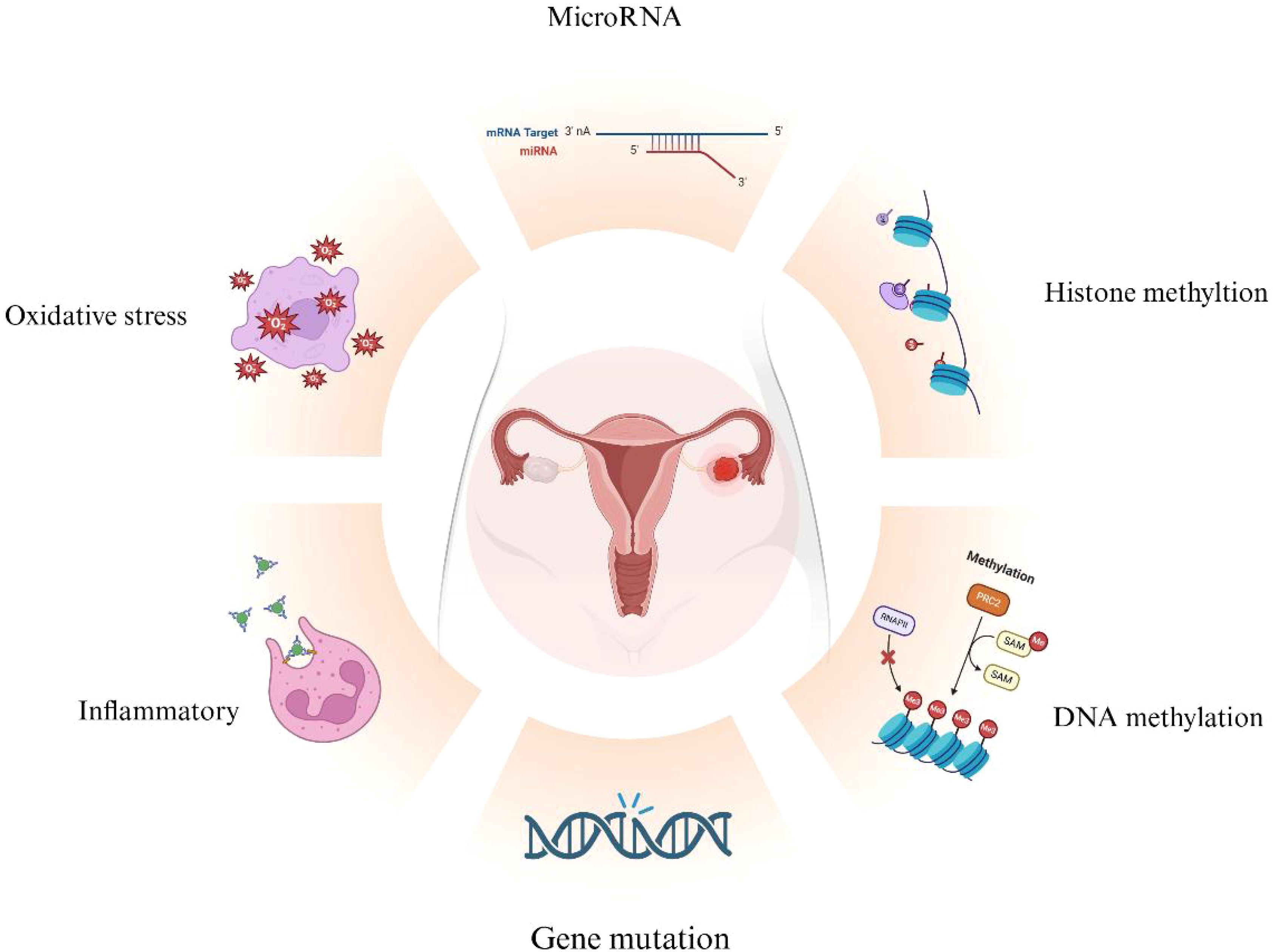
Figure 4. Overview of Mechanisms in EAOC Development. Various mechanisms contribute to the malignant transformation of endometriosis (EMs), including gene mutations, oxidative stress, inflammation, and epigenetic changes related to DNA methylation, histone methylation, and microRNAs. (Created with BioRender.com).
Author contributions
FC: Writing – original draft. MZ: Project administration, Writing – review & editing. WL: Funding acquisition, Writing – review & editing.
Funding
The author(s) declare financial support was received for the research, authorship, and/or publication of this article. This work was supported by the General Funding Program of Liaoning Provincial Joint Fund Project (2023-MSLH-124).
Conflict of interest
The authors declare that the research was conducted in the absence of any commercial or financial relationships that could be construed as a potential conflict of interest.
Publisher’s note
All claims expressed in this article are solely those of the authors and do not necessarily represent those of their affiliated organizations, or those of the publisher, the editors and the reviewers. Any product that may be evaluated in this article, or claim that may be made by its manufacturer, is not guaranteed or endorsed by the publisher.
References
1. Becker CM, Bokor A, Heikinheimo O, Horne A, Jansen F, Kiesel L, et al. ESHRE guideline: endometriosis. Hum Reprod Open. (2022) 2022:hoac009. doi: 10.1093/hropen/hoac009
2. Gambigliani Zoccoli S, La Marca A. Endometriosis affects natural and ART fertility in different ways: let's look at the whole patient and not at the single lesion. Reprod biomedicine Online. (2024) 49:104354. doi: 10.1016/j.rbmo.2024.104354
3. Yachida N, Yoshihara K, Yamaguchi M, Suda K, Tamura R, Enomoto T. How does endometriosis lead to ovarian cancer? The molecular mechanism of endometriosis-associated ovarian cancer development. Cancers. (2021) 13(6):1439. doi: 10.3390/cancers13061439
4. Pejovic T, Thisted S, White M, Nezhat FR. Endometriosis and endometriosis-associated ovarian cancer (EAOC). Adv Exp Med Biol. (2020) 1242:73–87. doi: 10.1007/978-3-030-38474-6_5
5. Pearce CL, Stram DO, Ness RB, Stram DA, Roman LD, Templeman C, et al. Population distribution of lifetime risk of ovarian cancer in the United States. Cancer epidemiology Biomarkers Prev. (2015) 24:671–6. doi: 10.1158/1055-9965.EPI-14-1128
6. del Carmen MG. Evidence for the relationship between endometriosis and epithelial ovarian cancer. Obstetrical gynecological survey. (2015) 70:587–95. doi: 10.1097/OGX.0000000000000218
7. Xu S, Tang C. The role of ARID1A in tumors: tumor initiation or tumor suppression? Front Oncol. (2021) 11:745187. doi: 10.3389/fonc.2021.745187
8. Mullen J, Kato S, Sicklick JK, Kurzrock R. Targeting ARID1A mutations in cancer. Cancer Treat Rev. (2021) 100:102287. doi: 10.1016/j.ctrv.2021.102287
9. Ediriweera MK, Tennekoon KH, Samarakoon SR. Role of the PI3K/AKT/mTOR signaling pathway in ovarian cancer: Biological and therapeutic significance. Semin Cancer Biol. (2019) 59:147–60. doi: 10.1016/j.semcancer.2019.05.012
10. Kobayashi H. Clinicopathological characteristics, molecular features and novel diagnostic strategies for the detection of Malignant transformation of endometriosis (Review). Exp Ther Med. (2023) 25:279. doi: 10.3892/etm.2023.11978
11. Taylor H, Laurence ADJ, Uhlig HH. The role of PTEN in innate and adaptive immunity. Cold Spring Harbor Perspect Med. (2019) 9(12):a036996. doi: 10.1101/cshperspect.a036996
12. Lv J, Zhu Q, Jia X, Yu N, Li Q. In vitro and in vivo effects of tumor suppressor gene PTEN on endometriosis: an experimental study [. Med Sci monitor. (2016) 22:3727–36. doi: 10.12659/MSM.901091
13. Halling GC, Udager AM, Skala SL. Endometrial, ovarian, and peritoneal involvement by endometrioid carcinoma, yolk sac tumor, and endometriosis: molecular evidence for a shared precursor. Int J gynecological Pathol. (2023) 42:247–53. doi: 10.1097/PGP.0000000000000889
14. Cerar J, Dogsa I, Jamnik A, Tomšič M. Physicochemical data on aqueous polymeric systems of methyl cellulose and lambda- and kappa-carrageenan: SAXS, rheological, densitometry, and sound velocity measurements. Data Brief. (2017) 15:427–38. doi: 10.1016/j.dib.2017.09.025
15. Schnack TH, Oliveira DNP, Christiansen AP, Høgdall C, Høgdall E. Prognostic impact of molecular profiles and molecular signatures in clear cell ovarian cancer. Cancer Genet. (2023) 278-279:9–16. doi: 10.1016/j.cancergen.2023.08.001
16. Gray M, Meehan J, Ward C, Langdon SP, Kunkler IH, Murray A, et al. Implantable biosensors and their contribution to the future of precision medicine. Veterinary J (London England: 1997). (2018) 239:21–9. doi: 10.1016/j.tvjl.2018.07.011
17. Caumanns JJ, van Wijngaarden A, Kol A, Meersma GJ, Jalving M, Bernards R, et al. Low-dose triple drug combination targeting the PI3K/AKT/mTOR pathway and the MAPK pathway is an effective approach in ovarian clear cell carcinoma. Cancer Lett. (2019) 461:102–11. doi: 10.1016/j.canlet.2019.07.004
18. Afify SM, Oo AKK, Hassan G, Seno A, Seno M. How can we turn the PI3K/AKT/mTOR pathway down? Insights into inhibition and treatment of cancer. Expert Rev Anticancer Ther. (2021) 21:605–19. doi: 10.1080/14737140.2021.1918001
19. Chandler RL, Raab JR, Vernon M, Magnuson T, Schisler JC. Global gene expression profiling of a mouse model of ovarian clear cell carcinoma caused by ARID1A and PIK3CA mutations implicates a role for inflammatory cytokine signaling. Genomics Data. (2015) 5:329–32. doi: 10.1016/j.gdata.2015.06.027
20. Suda K, Nakaoka H, Yoshihara K, Ishiguro T, Tamura R, Mori Y, et al. Clonal expansion and diversification of cancer-associated mutations in endometriosis and normal endometrium. Cell Rep. (2018) 24:1777–89. doi: 10.1016/j.celrep.2018.07.037
21. Pantsar T. The current understanding of KRAS protein structure and dynamics. Comput Struct Biotechnol J. (2020) 18:189–98. doi: 10.1016/j.csbj.2019.12.004
22. Yachida N, Yoshihara K, Suda K, Nakaoka H, Ueda H, Sugino K, et al. Biological significance of KRAS mutant allele expression in ovarian endometriosis. Cancer Sci. (2021) 112:2020–32. doi: 10.1111/cas.v112.5
23. Al Zubaidi T, Gehrisch OHF, Genois MM, Liu Q, Lu S, Kung J, et al. Targeting the DNA replication stress phenotype of KRAS mutant cancer cells. Sci Rep. (2021) 11:3656. doi: 10.1038/s41598-021-83142-y
24. Son J, Zhang Y, Lin H, Mirallas O, Alvarez Ballesteros P, Nardo M, et al. Clinical and genomic landscape of RAS mutations in gynecologic cancers. Clin Cancer Res. (2024) 30:2986–95. doi: 10.1158/1078-0432.CCR-23-2819
25. Piccinato CA, Neme RM, Torres N, Sanches LR, Cruz Derogis PB, Brudniewski HF, et al. Increased expression of CYP1A1 and CYP1B1 in ovarian/peritoneal endometriotic lesions. Reproduction. (2016) 151:683–92. doi: 10.1530/REP-15-0581
26. Cuffaro F, Russo E, Amedei A. Endometriosis, pain, and related psychological disorders: unveiling the interplay among the microbiome, inflammation, and oxidative stress as a common thread. Int J Mol Sci. (2024) 25(12):6473. doi: 10.3390/ijms25126473
27. Chen P, Li B, Ou-Yang L. Role of estrogen receptors in health and disease. Front Endocrinol. (2022) 13:839005. doi: 10.3389/fendo.2022.839005
28. Pan M, Pan X, Zhou J, Wang J, Qi Q, Wang L. Update on hormone therapy for the management of postmenopausal women. Biosci Trends. (2022) 16:46–57. doi: 10.5582/bst.2021.01418
29. Zeng Z, Fu M, Hu Y, Wei Y, Wei X, Luo M. Regulation and signaling pathways in cancer stem cells: implications for targeted therapy for cancer. Mol Cancer. (2023) 22:172. doi: 10.1186/s12943-023-01877-w
30. Alanazi H, Zhang Y, Fatunbi J, Luu T, Kwak-Kim J. The impact of reproductive hormones on T cell immunity; normal and assisted reproductive cycles. J Reprod Immunol. (2024) 165:104295. doi: 10.1016/j.jri.2024.104295
31. Filip L, Duică F, Prădatu A, Crețoiu D, Suciu N, Crețoiu SM, et al. Endometriosis associated infertility: A critical review and analysis on etiopathogenesis and therapeutic approaches. Medicina (Kaunas Lithuania). (2020) 56(9):460. doi: 10.3390/medicina56090460
32. He Z, Li Z, Lai S, Li H. Electron donor-acceptor complex enabled cyclization/sulfonylation cascade of N-heterocycles with thianthrenium salts. Org Lett. (2024) 26:6652–7. doi: 10.1021/acs.orglett.4c02307
33. Kato A, Romero MF. Regulation of electroneutral NaCl absorption by the small intestine. Annu Rev Physiol. (2011) 73:261–81. doi: 10.1146/annurev-physiol-012110-142244
34. Wu J, Xie H, Yao S, Liang Y. Macrophage and nerve interaction in endometriosis. J Neuroinflamm. (2017) 14:53. doi: 10.1186/s12974-017-0828-3
35. Liang Y, Xie H, Wu J, Liu D, Yao S. Villainous role of estrogen in macrophage-nerve interaction in endometriosis. Reprod Biol endocrinology: RB&E. (2018) 16:122. doi: 10.1186/s12958-018-0441-z
36. Manosalva C, Alarcon P, Quiroga J, Teuber S, Carretta MD, Bustamante H, et al. Bovine tumor necrosis factor-alpha Increases IL-6, IL-8, and PGE2 in bovine fibroblast-like synoviocytes by metabolic reprogramming. Sci Rep. (2023) 13:3257. doi: 10.1038/s41598-023-29851-y
37. Li B, Duan H, Wang S, Li Y. Ferroptosis resistance mechanisms in endometriosis for diagnostic model establishment. Reprod biomedicine Online. (2021) 43:127–38. doi: 10.1016/j.rbmo.2021.04.002
38. Clower L, Fleshman T, Geldenhuys WJ, Santanam N. Targeting oxidative stress involved in endometriosis and its pain. Biomolecules. (2022) 12(8):1055. doi: 10.3390/biom12081055
39. Duan YH, Wang HL, Liu MN, Xu TM, Zhang K. Reflections on the complex mechanisms of endometriosis from the perspective of ferroptosis. Pathology Res Pract. (2024) 259:155353. doi: 10.1016/j.prp.2024.155353
40. Nanda A, K T, Banerjee P, Dutta M, Wangdi T, Sharma P, et al. Cytokines, angiogenesis, and extracellular matrix degradation are augmented by oxidative stress in endometriosis. Ann Lab Med. (2020) 40:390–7. doi: 10.3343/alm.2020.40.5.390
41. Dymanowska-Dyjak I, Frankowska K, Abramiuk M, Polak G. Oxidative imbalance in endometriosis-related infertility-the therapeutic role of antioxidants. Int J Mol Sci. (2024) 25(12):6298. doi: 10.3390/ijms25126298
42. Immediata V, Ronchetti C, Spadaro D, Cirillo F, Levi-Setti PE. Oxidative stress and human ovarian response-from somatic ovarian cells to oocytes damage: A clinical comprehensive narrative review. Antioxidants (Basel Switzerland). (2022) 11(7):1335. doi: 10.3390/antiox11071335
43. Lannigan DA. ERK1/2-RSK2 signaling in regulation of ERα-mediated responses. Endocrinology. (2022) 163(9):bqac106. doi: 10.1210/endocr/bqac106
44. Braunstein C, Sirveaux F, Kalbacher E, Aubry S, Delroeux D, Hubert P, et al. Humeral metastasis as the only recurrence of a 5-year resected gastrointestinal stromal tumor: a case report. J Med Case Rep. (2021) 15:428. doi: 10.1186/s13256-021-02962-8
45. Throwba HP, Unnikrishnan L, Pangath M, Vasudevan K, Jayaraman S, Li M, et al. The epigenetic correlation among ovarian cancer, endometriosis and PCOS: A review. Crit Rev oncology/hematology. (2022) 180:103852. doi: 10.1016/j.critrevonc.2022.103852
46. Li Y, An D, Guan YX, Kang S. Aberrant methylation of the E-cadherin gene promoter region in endometrium and ovarian endometriotic cysts of patients with ovarian endometriosis. Gynecologic obstetric Invest. (2017) 82:78–85. doi: 10.1159/000445293
47. del Mundo MM, Aguilar M, Chen H, Niu S, Sahoo SS, Roy S, et al. [amp]]beta;-catenin, PAX2, and PTEN aberrancy across the spectrum of endometrioid ovarian lesions. International journal of gynecological pathology : official journal of the International Society of Gynecological Pathologists. (2024). doi: 10.1097/PGP.0000000000001046
48. Xia Z, Cochrane DR, Anglesio MS, Wang YK, Nazeran T, Tessier-Cloutier B, et al. LINE-1 retrotransposon-mediated DNA transductions in endometriosis associated ovarian cancers. Gynecol Oncol. (2017) 147:642–7. doi: 10.1016/j.ygyno.2017.09.032
49. Ren F, Wang DB, Li T, Chen YH, Li Y. Identification of differentially methylated genes in the Malignant transformation of ovarian endometriosis. J Ovarian Res. (2014) 7:73. doi: 10.1186/1757-2215-7-73
50. Wang D, Guo C, Li Y, Zhou M, Wang H, Liu J, et al. Oestrogen up-regulates DNMT1 and leads to the hypermethylation of RUNX3 in the Malignant transformation of ovarian endometriosis. Reprod biomedicine Online. (2022) 44:27–37. doi: 10.1016/j.rbmo.2021.06.030
51. Bedrick BS, Courtright L, Zhang J, Snow M, Amendola ILS, Nylander E, et al. A systematic review of epigenetics of endometriosis. F&S Rev. (2024) 5(1):100070. doi: 10.1016/j.xfnr.2024.01.003
52. Bitler BG, Aird KM, Garipov A, Li H, Amatangelo M, Kossenkov AV, et al. Synthetic lethality by targeting EZH2 methyltransferase activity in ARID1A-mutated cancers. Nat Med. (2015) 21:231–8. doi: 10.1038/nm.3799
53. Li S, Meersma GJ, Kupryjanczyk J, de Jong S, Wisman GBA. Genome-wide DNA methylation in relation to ARID1A deficiency in ovarian clear cell carcinoma. J Trans Med. (2024) 22:556. doi: 10.1186/s12967-024-05311-7
54. He J, Chang W, Feng C, Cui M, Xu T. Endometriosis Malignant transformation: epigenetics as a probable mechanism in ovarian tumorigenesis. Int J Genomics. (2018) 2018:1465348. doi: 10.1155/2018/1465348
55. Zubrzycka A, Zubrzycki M, Perdas E, Zubrzycka M. Genetic, epigenetic, and steroidogenic modulation mechanisms in endometriosis. J Clin Med. (2020) 9(5):1309. doi: 10.3390/jcm9051309
56. Brunty S, Mitchell B, Bou-Zgheib N, Santanam N. Endometriosis and ovarian cancer risk, an epigenetic connection. Annals of translational medicine. (2020) 8:1715. doi: 10.21037/atm-20-2449
57. Kori M, Gov E, Arga KY. Molecular signatures of ovarian diseases: Insights from network medicine perspective. Syst Biol Reprod Med. (2016) 62:266–82. doi: 10.1080/19396368.2016.1197982
58. Hull ML, Nisenblat V. Tissue and circulating microRNA influence reproductive function in endometrial disease. Reprod biomedicine Online. (2013) 27:515–29. doi: 10.1016/j.rbmo.2013.07.012
59. Moga MA, Bălan A, Dimienescu OG, Burtea V, Dragomir RM, Anastasiu CV. Circulating miRNAs as biomarkers for endometriosis and endometriosis-related ovarian cancer-an overview. J Clin Med. (2019) 8(5):735. doi: 10.3390/jcm8050735
60. Lohajová Behulová R, Bugalová A, Bugala J, Struhárňanská E, Šafranek M, Juráš I. Circulating exosomal miRNAs as a promising diagnostic biomarker in cancer. Physiol Res. (2023) 72:S193–s207. doi: 10.33549/physiolres
61. Woldemichael BT, Jawaid A, Kremer EA, Gaur N, Krol J, Marchais A, et al. The microRNA cluster miR-183/96/182 contributes to long-term memory in a protein phosphatase 1-dependent manner. Nat Commun. (2016) 7:12594. doi: 10.1038/ncomms12594
62. Marí-Alexandre J, Carcelén AP, Agababyan C, Moreno-Manuel A, García-Oms J, Calabuig-Fariñas S, et al. Interplay between microRNAs and oxidative stress in ovarian conditions with a focus on ovarian cancer and endometriosis. Int J Mol Sci. (2019) 20(21):5322. doi: 10.3390/ijms20215322
63. Veneziani AC, Gonzalez-Ochoa E, Alqaisi H, Madariaga A, Bhat G, Rouzbahman M, et al. Heterogeneity and treatment landscape of ovarian carcinoma. Nat Rev Clin Oncol. (2023) 20:820–42. doi: 10.1038/s41571-023-00819-1
64. Morin L, Grenier LP, Foucault N, Lévesque É, Fabi F, Langlais EL, et al. Comparison of weekly paclitaxel regimens in recurrent platinum-resistant ovarian cancer: A single institution retrospective study. Curr Oncol (Toronto Ont). (2024) 31:4624–31. doi: 10.3390/curroncol31080345
65. Kitamura S, Yamaguchi K, Murakami R, Furutake Y, Higasa K, Abiko K, et al. PDK2 leads to cisplatin resistance through suppression of mitochondrial function in ovarian clear cell carcinoma. Cancer Sci. (2021) 112:4627–40. doi: 10.1111/cas.v112.11
66. Alberts DS, Delforge A. Maximizing the delivery of intraperitoneal therapy while minimizing drug toxicity and maintaining quality of life. Semin Oncol. (2006) 33:S8–17. doi: 10.1053/j.seminoncol.2006.11.003
67. Barlin JN, Dao F, Bou Zgheib N, Ferguson SE, Sabbatini PJ, Hensley ML, et al. Progression-free and overall survival of a modified outpatient regimen of primary intravenous/intraperitoneal paclitaxel and intraperitoneal cisplatin in ovarian, fallopian tube, and primary peritoneal cancer. Gynecol Oncol. (2012) 125:621–4. doi: 10.1016/j.ygyno.2012.03.027
68. van Driel WJ, Koole SN, Sikorska K, Schagen van Leeuwen JH, Schreuder HWR, Hermans RHM, et al. Hyperthermic intraperitoneal chemotherapy in ovarian cancer. New Engl J Med. (2018) 378:230–40. doi: 10.1056/NEJMoa1708618
69. Seagle BL, Shahabi S. Cost-effectiveness analysis of dose-dense versus standard intravenous chemotherapy for ovarian cancer: An economic analysis of results from the Gynecologic Oncology Group protocol 262 randomized controlled trial. Gynecol Oncol. (2017) 145:9–14. doi: 10.1016/j.ygyno.2017.02.014
70. Clamp AR, James EC, McNeish IA, Dean A, Kim JW, O'Donnell DM, et al. Weekly dose-dense chemotherapy in first-line epithelial ovarian, fallopian tube, or primary peritoneal cancer treatment (ICON8): overall survival results from an open-label, randomised, controlled, phase 3 trial [J. Lancet Oncol. (2022) 23:919–30. doi: 10.1016/S1470-2045(22)00283-2
71. Chan JK, Brady MF, Penson RT, Huang H, Birrer MJ, Walker JL, et al. Weekly vs. Every-3-week paclitaxel and carboplatin for ovarian cancer. New Engl J Med. (2016) 374:738–48. doi: 10.1056/NEJMoa1505067
72. Su KM, Wang PH, Yu MH, Chang CM, Chang CC. The recent progress and therapy in endometriosis-associated ovarian cancer. J Chin Med Association: JCMA. (2020) 83:227–32. doi: 10.1097/JCMA.0000000000000262
73. Kuo KT, Mao TL, Jones S, Veras E, Ayhan A, Wang TL, et al. Frequent activating mutations of PIK3CA in ovarian clear cell carcinoma. Am J Pathol. (2009) 174:1597–601. doi: 10.2353/ajpath.2009.081000
74. Mabuchi S, Sugiyama T, Kimura T. Clear cell carcinoma of the ovary: molecular insights and future therapeutic perspectives. J gynecologic Oncol. (2016) 27:e31. doi: 10.3802/jgo.2016.27.e31
75. Shibuya Y, Tokunaga H, Saito S, Shimokawa K, Katsuoka F, Bin L, et al. Identification of somatic genetic alterations in ovarian clear cell carcinoma with next generation sequencing. Genes Chromosomes Cancer. (2018) 57:51–60. doi: 10.1002/gcc.22507
76. Tattersall A, Ryan N, Wiggans AJ, Rogozińska E, Morrison J. Poly(ADP-ribose) polymerase (PARP) inhibitors for the treatment of ovarian cancer. Cochrane Database systematic Rev. (2022) 2:Cd007929. doi: 10.1002/14651858.CD007929.pub4
77. Ledermann JA, Oza AM, Lorusso D, Aghajanian C, Oaknin A, Dean A, et al. Rucaparib for patients with platinum-sensitive, recurrent ovarian carcinoma (ARIEL3): post-progression outcomes and updated safety results from a randomised, placebo-controlled, phase 3 trial. Lancet Oncol. (2020) 21:710–22. doi: 10.1016/S1470-2045(20)30061-9
78. Alsop K, Fereday S, Meldrum C, deFazio A, Emmanuel C, George J, et al. BRCA mutation frequency and patterns of treatment response in BRCA mutation-positive women with ovarian cancer: a report from the Australian Ovarian Cancer Study Group. J Clin Oncol. (2012) 30:2654–63. doi: 10.1200/JCO.2011.39.8545
79. Barreta A, Sarian LO, Ferracini AC, Costa LBE, Mazzola PG, de Angelo Andrade L, et al. Immunohistochemistry expression of targeted therapies biomarkers in ovarian clear cell and endometrioid carcinomas (type I) and endometriosis. Hum Pathol. (2019) 85:72–81. doi: 10.1016/j.humpath.2018.10.028
80. Wei Z, Yuan T, Tarkowská D, Kim J, Nam HG, Novák O, et al. Brassinosteroid biosynthesis is modulated via a transcription factor cascade of COG1, PIF4, and PIF5. Plant Physiol. (2017) 174:1260–73. doi: 10.1104/pp.16.01778
81. Fukumoto T, Fatkhutdinov N, Zundell JA, Tcyganov EN, Nacarelli T, Karakashev S, et al. HDAC6 inhibition synergizes with anti-PD-L1 therapy in ARID1A-inactivated ovarian cancer. Cancer Res. (2019) 79:5482–9. doi: 10.1158/0008-5472.CAN-19-1302
Keywords: endometriosis, endometriosis-associated ovarian carcinoma, malignant transformation, ovarian clear cell carcinoma, ovarian endometrioid carcinoma
Citation: Chen F, Zhu M and Li W (2024) Advances in research on malignant transformation of endometriosis-associated ovarian cancer. Front. Oncol. 14:1475231. doi: 10.3389/fonc.2024.1475231
Received: 03 August 2024; Accepted: 10 September 2024;
Published: 09 October 2024.
Edited by:
Anthony Taylor, University of Leicester, United KingdomReviewed by:
Yu-gang Huang, Hubei University of Medicine, ChinaCopyright © 2024 Chen, Zhu and Li. This is an open-access article distributed under the terms of the Creative Commons Attribution License (CC BY). The use, distribution or reproduction in other forums is permitted, provided the original author(s) and the copyright owner(s) are credited and that the original publication in this journal is cited, in accordance with accepted academic practice. No use, distribution or reproduction is permitted which does not comply with these terms.
*Correspondence: Wenjuan Li, bHdqMTM3MTM4OTgyQGhvdG1haWwuY29t