- 1Department of Internal Medicine, Siziwangqi People’s Hospital, Wulancabu, China
- 2Translational Pharmaceutical Laboratory, Jining NO.1 People’s Hospital, Jining, China
- 3Department of Gastrointestinal Surgery, Jining NO.1 People’s Hospital, Jining, China
- 4Department of Oncology, Jining No.1 People’s Hospital, Jining, China
Short-chain fatty acids (SCFAs), which are saturated fatty acids consisting of six or fewer carbon atoms, have been found to be closely associated with the biological behavior of malignant tumors. This manuscript provides a comprehensive review on the role of SCFAs in regulating cell cycle, apoptosis, tumor angiogenesis, epithelial-mesenchymal transition, protein regulatory pathways, and histone regulation in promoting the development of malignant tumors. Furthermore, we discuss the potential therapeutic strategies targeting SCFAs for treating malignant tumors. This review offers a theoretical foundation for investigating the mechanisms by which SCFAs impact malignant tumors and provides insights into developing novel treatment targets.
1 Introduction
Short-chain fatty acids (SCFAs) are saturated fatty acids consisting of six or fewer carbon atoms. Due to their low molecular weight, they readily volatilize at room temperature and are also referred to as volatile fatty acids (VFAs) (1). The majority of SCFAs in the human body are metabolic byproducts resulting from the fermentation of dietary fiber by anaerobic bacteria or yeast in the colon. The types and quantities of SCFAs primarily rely on the composition of gut microbiota, digestion time, host microbial metabolism flux, and fiber content in the host’s diet. Acetate, propionate, butyrate, and isovalerate (lactate) are among the most prevalent SCFAs. Acetate and propionate are predominantly produced by Bacteroides while butyrate is synthesized by Firmicutes (2). SCFAs play a crucial role in maintaining energy supply, regulating motility, and safeguarding mucosal barrier integrity within the intestine (3).
In recent years, the role of SCFAs in tumor pathogenesis has garnered significant attention, with a particular focus on Valproic Acid (VPA), Butyric Acid, Acetate Salt, and Propionate, as shown in Table 1. These SCFAs have been extensively studied for their anti-tumorigenic properties and underlying mechanisms. However, despite the compelling evidence presented by these compounds, research exploring the effects of other SCFAs, such as Caproic Acid (4) and Succinate (5), on tumor growth and progression remains relatively scarce, particularly in terms of elucidating their specific mechanisms of action. Therefore, this review has unveiled the role of mainly SCFAs in malignant tumor biology, as in Figure 1.
2 The role of SCFAs in cell cycle and apoptosis of cancer
The hallmark of tumor cells is aberrant proliferation, and the cell cycle is tightly regulated through the modulation of proteins (Cyclin), Cyclin-dependent kinases (CDKs), and Cyclin-dependent kinase inhibitors (CKIs) to govern cellular growth and division. Cyclin assumes a pivotal role throughout the entirety of the cell cycle process, ensuring precise regulation at distinct stages of cell cycle progression while mediating checkpoint functions (6). These three regulatory factors primarily achieve their functions through Rb pathway and p53 pathway (7, 8). Research has demonstrated that SCFAs can induce cell cycle arrest and inhibit cell proliferation in tumor cells by modulating factors associated with the cell cycle. Valproic acid (VPA) induces the expression of p21 and topoisomerase II (α/β), where p21 acts as a conventional cell cycle inhibitor, restraining the activity of cycDl-CDK4 and cycE-CDK2, thereby leading to G1 phase arrest (Figure 2) (9). Studies conducted by Bacon C L (10) have revealed that exposure to VPA significantly upregulates Cyclin D3 expression during mid-G1 phase and translocates it into the nucleus in glioma cells. Cyclin D plays a pivotal role as a core component driving cellular division throughout the cell cycle, with Cyclin D1 typically expressed during early G1 phase and Cyclin D3 expressed during late G1 phase (Figure 2) (11), suggesting that elevated levels of Cyclin D3 expression and ectopic activation are crucial determinants for VPA-induced G1 phase arrest. Through the Warburg effect, differentiated tumor cells primarily rely on glycolysis to meet their energy demands. It has been discovered that Cyclin D3 can phosphorylate and deactivate PFK1 (phosphofructokinase 1) and PKM2 (pyruvate kinase m2), consequently inhibiting glycolysis during G1 phase while weakening energy supply to tumor cells (12, 13). The molecular mechanisms underlying short-chain fatty acid-induced cell cycle arrest may vary depending on the specific type of cells, as VPA treatment has also been observed to induce G2/M phase arrest in lung cancer cells (13), whereas liver cancer cells treated with VPA experience both G2/M phase arrest and G0/G1 phases arrest (Figure 2) (14). Similarly, butyrate strongly arrested the cell cycle at G2 phase and promoted apoptosis, leading to tumor cell death (15, 16).
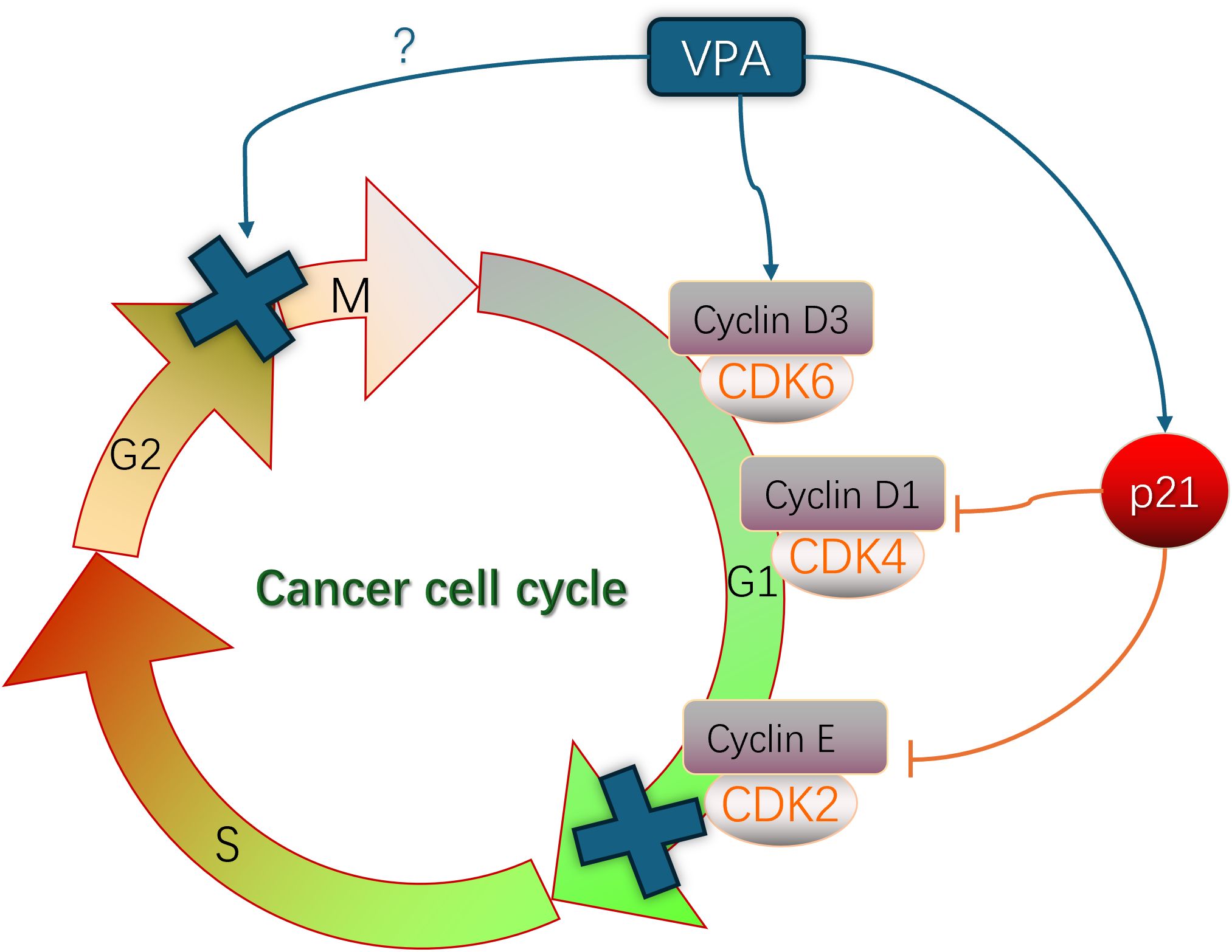
Figure 2. The role of VPA in the regulation to cancer cell cycle involved in the mechanism of cancer.
Cellular apoptosis is considered to be a crucial mechanism for preventing tumorigenesis; however, one hallmark feature of tumor cells is their ability to suppress apoptosis (17). In tumor cells, the equilibrium between pro-apoptotic and anti-apoptotic proteins such as the Bcl-2 protein family and IAPs becomes disrupted, resulting in attenuated caspase activity. The study revealed that SCFAs can modulate apoptosis-related proteins and impact the cellular apoptosis process. There are two primary pathways for initiating cell apoptosis: intrinsic and extrinsic pathways, both of which activate the Caspase family of proteases. Caspase has the ability to hydrolyze over 400 types of proteins, thereby accelerating cell death (18). Butyrate triggers the endogenous apoptotic pathway by regulating Bcl-2 activity, a mitochondrial-mediated apoptotic regulatory factor, upregulating BH3-only transcriptional activators, releasing pro-apoptotic factors BAX and BAK to form oligomers, increasing mitochondrial outer membrane permeability, releasing cytochrome C and Smac/DIABLO apoptotic factors from mitochondria. These apoptotic factors facilitate activation of Caspase 9 followed by activation of Caspases 3 and 7 leading to cellular apoptosis (19). In most cases, all apoptotic signals converge on the final executor Caspase-3; thus detecting the content or activity level of cleaved Caspase-3 reflects the progression of cellular apoptosis (20). Kim K et al. (21) discovered that propionate treatment significantly decreased Survivin expression levels while increasing p21 protein expression levels in H1299 and H1703 cells; early-stage and late-stage apoptotic cells were more abundant in SP-treated groups compared to control groups; caspase 3/7 activity was also notably increased.
3 The role of SCFAs in angiogenesis of cancer
Tumor growth and metastasis rely on angiogenesis, with VEGF playing a crucial role in promoting vascular formation and tumor cell proliferation. Targeting pro-angiogenic genes is an effective therapeutic strategy for inhibiting tumor progression (22). The research findings indicate that short-chain fatty acids (SCFAs) possess potential as anti-angiogenic drugs due to their impact on the expression of vascular endothelial growth factor (VEGF). Butyrate salts can downregulate VEGF expression by inhibiting the binding affinity of Sp1, a transcription factor, to the promoter region of neuropilin-1 (NRP-1), thereby reducing its capacity for expression. NRP-1 acts as a co-receptor for VEGF and enhances the interaction between VEGF165 and EGFR-2, promoting chemotaxis and mitogenesis in endothelial cells induced by VEGF165, thus facilitating VEGF-mediated angiogenesis (23, 24). Valproic acid (VPA) exhibits time-dependent inhibition of HIF-1α, VEGF, and tumor necrosis factor (TNF) expression in cervical cancer cells through suppression of PI3K/Akt and ERK1/2 signaling pathways. Additionally, it suppresses endothelial cell migration and regulates tumor neovascularization (25).
4 The role of SCFAs in epithelial-to-mesenchymal transition of cancer
Epithelial-to-mesenchymal transition (EMT) is a biological process wherein epithelial cells undergo a specific program to acquire mesenchymal characteristics, playing a crucial role in conferring migration and invasion abilities upon malignant tumor cells derived from epithelium. EMT leads to the loss of certain epithelial cell traits, resulting in decreased expression levels of key epithelial genes such as E-cadherin, ZO-1, and occludin. Consequently, there is reduced contact with surrounding and stromal cells, diminished intercellular interactions, and acquisition of mesenchymal cell features. Concurrently, the expression levels of mesenchymal genes like N-cadherin, vimentin, and fibronectin increase during this process. This ultimately enhances cell migration and motility while promoting increased invasive capacity and detachment capability. These effects can be mediated through the classical Smad pathway (26, 27). VPA exhibits the ability to downregulate SMAD4 protein levels—a pivotal factor in TGF-β-induced EMT—and effectively inhibits metastatic potential in prostate cancer (28) as well as renal cancer cells (29).
5 The role of SCFAs in protein pathways of cancer
The G-protein coupled receptors (GPCRs), also known as seven-transmembrane receptors, constitute the largest family of cell surface receptors in eukaryotes and participate in numerous cellular signaling pathways. Several members of the GPCR family have been implicated in tumor initiation and progression, including SMO protein - a pivotal component of the Hedgehog signaling pathway that, when aberrantly activated, can contribute to various cancers such as basal cell carcinoma and rhabdomyosarcoma (30). The chemokine receptor CXCR4 is frequently overexpressed in tumors and is believed to play a crucial role in angiogenesis, tumor cell migration, invasion, and metastasis (31). Short-chain fatty acids (SCFAs) serve as natural ligands for GPCRs. Upon binding, they further activate signal cascades mediated by phospholipase C, mitogen-activated protein kinases (MAPKs), phospholipase A2, and nuclear factor κB (32). In colon cancer cells, SCFAs can inhibit cell proliferation through NF-КB, MAPKs ERK1/2 PI3K, and Wnt signaling pathways while inducing apoptosis and cell cycle arrest (33, 34). GPCRs expressed in breast cancer cell lines can elevate intracellular calcium concentration upon binding with SCFAs thereby activating the p38 MAPK pathway to suppress tumor cell proliferation (35). Moreover, GPCRs like GPR109A selectively bind to butyric acid which mediates its anticancer activity thus reducing invasive capabilities of breast cancer cells (36).
6 The role of SCFAs in histones regulation mechanism of cancer
Proteins are small, alkaline proteins found in the chromatin of eukaryotic cells that, together with DNA, form nucleosomes. Modifications such as methylation, acetylation, phosphorylation, and ubiquitination of histones can alter chromatin structure and play a pivotal role in epigenetic regulation (37, 38). Acetylation is one of the most prevalent modifications of histones and its extent is finely regulated by the metabolic state of organisms. Histone acetylation facilitates the dissociation of DNA from histone octamers, thereby loosening the structure of nucleosomes. This enables specific binding of various transcription factors and coactivators to DNA binding sites, activating gene transcription. Transcriptionally active regions exhibit a high density of acetylated core histones while inactive regions have a low density. Conversely, histone deacetylases (HDACs) exert opposing effects by compacting DNA and inhibiting transcription processes (39). Histone acetylation and deacetylation modifications serve as major regulators for gene expression control. Alterations in nucleosome structure are crucial for precise gene expression in eukaryotic cells. Targeting HDACs holds significant potential for anti-tumor activity; HDAC inhibitors induce chromatin remodeling through increased levels of histone acetylation, rectifying epigenetic errors, promoting anti-tumor activity, and enhancing expression levels of tumor suppressor genes to inhibit cancer cell proliferation (40).
The HDACIs encompass a variety of compounds with diverse structures, including short-chain fatty acids like butyrate salts, butyrate esters, and valproic acid. Treatment with VPA in glioblastoma cell lines resulted in enhanced acetylation of histone H4 (41). Similarly, treatment with butyrate in colon cancer cells led to increased acetylation of histone H3, indicating the inhibition of HDACs within these cells (42). HDACIs exert their effects by regulating the extent of DNA wrapping around histones. Histone deacetylases remove acetyl groups from histones, resulting in tightly wrapped DNA that is less accessible to transcription factors. Consequently, this leads to the suppression of protein expression associated with cell cycle arrest and apoptosis in damaged cells as well as a decrease in the expression of tumor suppressor genes and other anti-cancer genes, thereby promoting cancer development (40). Butyrate salts can impede CRC cell migration and invasion by blocking the activation of HDAC3, which subsequently reduces phosphorylation levels of Akt1 and erk1/2 leading to inhibited cell motility (43).
7 The role of SCFAs in treatment of cancer
Supplementation with exogenous bacteria that produce short-chain fatty acids has been demonstrated to augment the efficacy and sensitivity of chemotherapy, radiotherapy, or immunotherapy in the treatment of malignant tumors, while concurrently mitigating treatment-related toxicity. Notably, butyrate salts have been observed to deplete vancomycin-sensitive bacteria in lung cancer and potentiate the anti-tumor activity of radiation therapy (44). VPA synergistically enhances the cytotoxicity of temozolomide in high-grade glioma cell lines. The combination of valproic acid and arsenic trioxide induces G2/M phase arrest and promotes apoptosis cell death, effectively inhibiting lung cancer cell growth by modulating the cell cycle. In vivo studies have substantiated a synergistic anti-tumor effect (45).
SCFAs exhibit a dose-dependent inhibition on colony formation and proliferation of colorectal cancer cells, regulate the composition of colonic microbiota in colon cancer, and enhance the proportion of SCFA-producing bacteria. Faecalibaculum rodentium and its human homolog biformholdemanella are two microbial strains discovered to possess anti-colon tumor properties (46). They demonstrate the ability to produce SCFAs in both mouse and human experiments, control protein acetylation and tumor cell proliferation by suppressing calcium-regulated phosphatase secretion while activating NFATc3, as well as inhibit the growth of tumor cell lines or patient tumor samples in vitro (47). Therefore, inducing an increase in SCFA content within the intestinal tract may potentially serve as an adjuvant therapy for colorectal cancer.
Butyrate can attenuate oxidative stress on the gastric mucosa, upregulate the expression of GPR109A, decrease the levels of pro-inflammatory factors such as TNF-α and IL-1β, and play a crucial role in gastric mucosal repair. Studies have demonstrated that oral supplementation of butyric acid bacteria post-gastrectomy can enhance intestinal SCFA content through fermentation of various carbohydrates, leading to reduced expression of inflammatory cells and factors, improved immune function, decreased postoperative complications, and enhanced recovery in patients following gastric cancer surgery (48). Moreover, there is evidence supporting that acetate salts possess dose-dependent apoptotic effects on gastric cancer cells and mesothelioma cells with heightened sensitivity towards human tumor cells. Local application of acetate combined with chemotherapy may represent a viable treatment approach and novel therapeutic strategy for drug-resistant mesothelioma (49). Tri-butyrylglycerol (a classical derivative of short-chain fatty acids) inhibits the activity of gastric cancer cells in a dose-dependent manner. Appropriate supplementation may exert preventive effects against gastric cancer (50). Kim et al. (51) discovered through plasma level analysis that propionate levels were significantly elevated in patients with gastric cancer, suggesting its potential as a novel biomarker for evaluating disease progression. Furthermore, it has been found that acetate salts also possess the ability to downregulate estrogen receptors in breast cancer and exhibit certain clinical efficacy in treating ER-positive endocrine-resistant breast cancer patients (52).
It has been confirmed that SCFAs may play an important regulatory role in the immune system in a complex manner. Previous studies have found a negative correlation between pre-treatment serum concentrations of butyrate and propionate and overall survival and progression-free survival in patients with metastatic melanoma receiving CTLA-4 monoclonal antibody therapy. High levels of butyrate in the blood inhibit the accumulation of memory T cells and ICOS+ CD4+ T cells induced by CTLA-4 monoclonal antibody, as well as reduce the efficacy of CTLA-4 monoclonal antibody in three different tumor mouse models. Butyrate also inhibits the upregulation of CD80/CD86 on dendritic cells and ICOS on T cells induced by CTLA-4 monoclonal antibody, while increasing the proportion of Tregs (53). However, further research has revealed that pectin, a major soluble fiber extracted from plant cell walls, can alter butyrate levels in humanized tumor-bearing mice with gut microbiota derived from colorectal cancer patients. It suppresses tumor growth in humanized mouse models resistant to anti-PD-1 monoclonal antibodies due to their gut microbiota composition, suggesting its potential ability to reverse resistance to anti-PD-1 monoclonal antibodies in colon cancer patients (53). Combining acetate salts with PD-1 therapy significantly delays the growth of hepatocellular carcinoma compared to administering acetate salts alone (54). Therapeutic supplementation of short-chain fatty acids (SCFAs) or a high-fiber diet, which enhances endogenous SCFA production, inhibits osteoclast activity and prevents pathological fractures (55). In vitro studies by Luu et al. (56) demonstrated that SCFAs modulate the activity of reprogrammed CD8+ CTLs and CAR-T cells by inhibiting HDAC, leading to increased production of effector molecules such as CD25, IFN-γ, and TNF-α. This augmentation strengthens mTOR’s role as a central cellular metabolic sensor in CD8+ T cells. mTOR influences cytokine expression in T cells and is involved in immune suppression, DNA transcription regulation, cell growth, and apoptosis. Consequently, it enhances the anti-tumor activity of T cells and significantly amplifies the anti-tumor efficacy of antigen-specific CTLs targeting ROR1 in melanoma and pancreatic cancer models using genetically modified mice. These findings have positive implications for improving the therapeutic efficacy of tumor immunotherapy and hold promise for optimizing CAR-T cell therapy as well as other tumor therapies through modulation of bacterial species within the gut microbiome.
8 Conclusions
SCFAs, primary metabolic byproducts of dietary fiber fermentation mediated by anaerobic bacteria and yeasts within the intestinal milieu, exhibit profound biological functions in human physiology. This comprehensive review meticulously examines the intricate mechanisms of SCFAs in malignancy, encompassing their intricate interplay with cell cycle regulation, apoptosis, tumor angiogenesis, EMT, and histone modulation, while also elucidating the pivotal roles of SCFAs-associated metabolic pathways in cancer progression. In summary, SCFAs occupy a central position in the intricate web of malignancy initiation, progression, and treatment. Future endeavors aimed at elucidating the intricate metabolic mechanisms of SCFAs and their therapeutic potential in cancer will undoubtedly yield invaluable insights, paving the way for the development of innovative anti-tumor strategies. By harnessing the power of gut microbiota modulation and SCFAs production, we may uncover novel avenues for the prevention and treatment of malignancies, thereby revolutionizing the landscape of cancer medicine.
Author contributions
MH: Writing – original draft, Writing – review & editing. Q-QY: Writing – original draft. LY: Writing – review & editing. HZ: Writing – review & editing. PJ: Conceptualization, Writing – review & editing. LQ: Supervision, Writing – review & editing. QZ: Conceptualization, Investigation, Supervision, Validation, Writing – review & editing.
Funding
The author(s) declare that no financial support was received for the research, authorship, and/or publication of this article.
Conflict of interest
The authors declare that this research was conducted in the absence of commercial or financial relationships that could be construed as a potential conflict of interest.
Publisher’s note
All claims expressed in this article are solely those of the authors and do not necessarily represent those of their affiliated organizations, or those of the publisher, the editors and the reviewers. Any product that may be evaluated in this article, or claim that may be made by its manufacturer, is not guaranteed or endorsed by the publisher.
References
1. Fei Q, Chang HN, Shang L, Choi JD, Kim N, Kang J. The effect of volatile fatty acids as a sole carbon source on lipid accumulation by Cryptococcus albidus for biodiesel production. Bioresour Technol. (2011) 102:2695–701. doi: 10.1016/j.biortech.2010.10.141
2. Levy M, Thaiss CA, Elinav E. Metabolites: messengers between the microbiota and the immune system. Genes Dev. (2016) 30:1589–97. doi: 10.1101/gad.284091.116
3. Parada Venegas D, de la Fuente MK, Landskron G, González MJ, Quera R, Dijkstra G, et al. Short chain fatty acids (SCFAs)-mediated gut epithelial and immune regulation and its relevance for inflammatory bowel diseases. Front Immunol. (2019) 10:277. doi: 10.3389/fimmu.2019.00277
4. Narayanan A, Baskaran SA, Amalaradjou MA, Venkitanarayanan K. Anticarcinogenic properties of medium chain fatty acids on human colorectal, skin and breast cancer cells in vitro. Int J Mol Sci. (2015) 16:5014–27. doi: 10.3390/ijms16035014
5. Wu JY, Huang TW, Hsieh YT, Wang YF, Yen CC, Lee GL, et al. Cancer-derived succinate promotes macrophage polarization and cancer metastasis via succinate receptor. Mol Cell. (2020) 77:213–227.e5. doi: 10.1016/j.molcel.2019.10.023
6. Santamaria D, Ortega S. Cyclins and CDKS in development and cancer: lessons from genetically modified mice. Front Biosci. (2006) 11:1164–88. doi: 10.2741/1871
7. Dyson NJ. RB1: a prototype tumor suppressor and an enigma. Genes Dev. (2016) 30:1492–502. doi: 10.1101/gad.282145.116
8. Engeland K. Cell cycle arrest through indirect transcriptional repression by p53: I have a DREAM. Cell Death Differ. (2018) 25:114–32. doi: 10.1038/cdd.2017.172
9. Gilardini Montani MS, Granato M, Santoni C, Del Porto P, Merendino N, D’Orazi G, et al. Histone deacetylase inhibitors VPA and TSA induce apoptosis and autophagy in pancreatic cancer cells. Cell Oncol (Dordr). (2017) 40:167–80. doi: 10.1007/s13402-017-0314-z
10. Bacon CL, Gallagher HC, Haughey JC, Regan CM. Antiproliferative action of valproate is associated with aberrant expression and nuclear translocation of cyclin D3 during the C6 glioma G1 phase. J Neurochem. (2002) 83:12–9. doi: 10.1046/j.1471-4159.2002.01081.x
11. Kato JY, Sherr CJ. Inhibition of granulocyte differentiation by G1 cyclins D2 and D3 but not D1. Proc Natl Acad Sci USA. (1993) 90:11513–7. doi: 10.1073/pnas.90.24.11513
12. Yalcin A, Clem BF, Simmons A, Lane A, Nelson K, Clem AL, et al. Nuclear targeting of 6-phosphofructo-2-kinase (PFKFB3) increases proliferation via cyclin-dependent kinases. J Biol Chem. (2009) 284:24223–32. doi: 10.1074/jbc.M109.016816
14. An HM, Xue YF, Shen YL, Du Q, Hu B. Sodium valproate induces cell senescence in human hepatocarcinoma cells. Molecules. (2013) 18:14935–47. doi: 10.3390/molecules181214935
15. Bultman SJ. The microbiome and its potential as a cancer preventive intervention. Semin Oncol. (2016) 43:97–106. doi: 10.1053/j.seminoncol.2015.09.001
16. Zeng H, Hamlin SK, Safratowich BD, Cheng WH, Johnson LK. Superior inhibitory efficacy of butyrate over propionate and acetate against human colon cancer cell proliferation via cell cycle arrest and apoptosis: linking dietary fiber to cancer prevention. Nutr Res. (2020) 83:63–72. doi: 10.1016/j.nutres.2020.08.009
17. Wong RS. Apoptosis in cancer: from pathogenesis to treatment. J Exp Clin Cancer Res. (2011) 30:87. doi: 10.1186/1756-9966-30-87
18. Schafer ZT, Kornbluth S. The apoptosome: physiological, developmental, and pathological modes of regulation. Dev Cell. (2006) 10:549–61. doi: 10.1016/j.devcel.2006.04.008
19. Zhang K, Ji X, Song Z, Wu F, Qu Y, Jin X, et al. Butyrate inhibits gastric cancer cells by inducing mitochondriamediated apoptosis. Comb Chem High Throughput Screen. (2023) 26:630–8. doi: 10.2174/1386207325666220720114642
20. Liang Y, Yan C, Schor NF. Apoptosis in the absence of caspase 3. Oncogene. (2001) 20:6570–8. doi: 10.1038/sj.onc.1204815
21. Kim K, Kwon O, Ryu TY, Jung CR, Kim J, Min JK, et al. Propionate of a microbiota metabolite induces cell apoptosis and cell cycle arrest in lung cancer. Mol Med Rep. (2019) 20:1569–74. doi: 10.3892/mmr.2019.10431
22. Cao Y, Arbiser J, D’Amato RJ, D’Amore PA, Ingber DE, Kerbel R, et al. Forty-year journey of angiogenesis translational research. Sci Transl Med. (2011) 3:114rv3. doi: 10.1126/scitranslmed.3003149
23. Yu DC, Waby JS, Chirakkal H, Staton CA, Corfe BM. Butyrate suppresses expression of neuropilin I in colorectal cell lines through inhibition of Sp1 transactivation. Mol Cancer. (2010) 9:276. doi: 10.1186/1476-4598-9-276
24. Sawa H, Murakami H, Ohshima Y, Murakami M, Yamazaki I, Tamura Y, et al. Histone deacetylase inhibitors such as sodium butyrate and trichostatin A inhibit vascular endothelial growth factor (VEGF) secretion from human glioblastoma cells. Brain Tumor Pathol. (2002) 19:77–81. doi: 10.1007/BF02478931
25. Zhao Y, You W, Zheng J, Chi Y, Tang W, Du R. Valproic acid inhibits the angiogenic potential of cervical cancer cells via HIF-1α/VEGF signals. Clin Transl Oncol. (2016) 18:1123–30. doi: 10.1007/s12094-016-1494-0
26. Yang J, Antin P, Berx G, Blanpain C, Brabletz T, Bronner M, et al. Guidelines and definitions for research on epithelial-mesenchymal transition. Nat Rev Mol Cell Biol. (2020) 21:341–52. doi: 10.1038/s41580-020-0237-9
27. Lamouille S, Xu J, Derynck R. Molecular mechanisms of epithelial-mesenchymal transition. Nat Rev Mol Cell Biol. (2014) 15:178–96. doi: 10.1038/nrm3758
28. Lan X, Lu G, Yuan C, Mao S, Jiang W, Chen Y, et al. Valproic acid (VPA) inhibits the epithelial-mesenchymal transition in prostate carcinoma via the dual suppression of SMAD4. J Cancer Res Clin Oncol. (2016) 142:177–85. doi: 10.1007/s00432-015-2020-4
29. Mao S, Lu G, Lan X, Yuan C, Jiang W, Chen Y, et al. Valproic acid inhibits epithelial−mesenchymal transition in renal cell carcinoma by decreasing SMAD4 expression. Mol Med Rep. (2017) 16:6190–9. doi: 10.3892/mmr.2017.7394
30. Taipale J, Chen JK, Cooper MK, Wang B, Mann RK, Milenkovic L, et al. Effects of oncogenic mutations in Smoothened and Patched can be reversed by cyclopamine. Nature. (2000) 406:1005–9. doi: 10.1038/35023008
31. Phillips RJ, Mestas J, Gharaee-Kermani M, Burdick MD, Sica A, Belperio JA, et al. Epidermal growth factor and hypoxia-induced expression of CXC chemokine receptor 4 on non-small cell lung cancer cells is regulated by the phosphatidylinositol 3-kinase/PTEN/AKT/mammalian target of rapamycin signaling pathway and activation of hypoxia inducible factor-1alpha. J Biol Chem. (2005) 280:22473–81. doi: 10.1074/jbc.M500963200
32. Tremaroli V, Bäckhed F. Functional interactions between the gut microbiota and host metabolism. Nature. (2012) 489:242–9. doi: 10.1038/nature11552
33. Singh N, Gurav A, Sivaprakasam S, Brady E, Padia R, Shi H, et al. Activation of Gpr109a, receptor for niacin and the commensal metabolite butyrate, suppresses colonic inflammation and carcinogenesis. Immunity. (2014) 40:128–39. doi: 10.1016/j.immuni.2013.12.007
34. Salem HA, Wadie W. Effect of niacin on inflammation and angiogenesis in a murine model of ulcerative colitis. Sci Rep. (2017) 7:7139. doi: 10.1038/s41598-017-07280-y
35. Yonezawa T, Kobayashi Y, Obara Y. Short-chain fatty acids induce acute phosphorylation of the p38 mitogen-activated protein kinase/heat shock protein 27 pathway via GPR43 in the MCF-7 human breast cancer cell line. Cell Signal. (2007) 19:185–93. doi: 10.1016/j.cellsig.2006.06.004
36. Elangovan S, Pathania R, Ramachandran S, Ananth S, Padia RN, Lan L, et al. The niacin/butyrate receptor GPR109A suppresses mammary tumorigenesis by inhibiting cell survival. Cancer Res. (2014) 74:1166–78. doi: 10.1158/0008-5472.CAN-13-1451
37. Lachner M, O’Carroll D, Rea S, Mechtler K, Jenuwein T. Methylation of histone H3 lysine 9 creates a binding site for HP1 proteins. Nature. (2001) 410:116–20. doi: 10.1038/35065132
38. Zegerman P, Canas B, Pappin D, Kouzarides T. Histone H3 lysine 4 methylation disrupts binding of nucleosome remodeling and deacetylase (NuRD) repressor complex. J Biol Chem. (2002) 277:11621–4. doi: 10.1074/jbc.C200045200
39. Seto E, Yoshida M. Erasers of histone acetylation: the histone deacetylase enzymes. Cold Spring Harb Perspect Biol. (2014) 6:a018713. doi: 10.1101/cshperspect.a018713
40. Ramaiah MJ, Tangutur AD, Manyam RR. Epigenetic modulation and understanding of HDAC inhibitors in cancer therapy. Life Sci. (2021) 277:119504. doi: 10.1016/j.lfs.2021.119504
41. Das CM, Aguilera D, Vasquez H, Prasad P, Zhang M, Wolff JE, et al. Valproic acid induces p21 and topoisomerase-II (alpha/beta) expression and synergistically enhances etoposide cytotoxicity in human glioblastoma cell lines. J Neurooncol. (2007) 85:159–70. doi: 10.1007/s11060-007-9402-7
42. Hatayama H, Iwashita J, Kuwajima A, Abe T. The short chain fatty acid, butyrate, stimulates MUC2 mucin production in the human colon cancer cell line, LS174T. Biochem Biophys Res Commun. (2007) 356:599–603. doi: 10.1016/j.bbrc.2007.03.025
43. Li Q, Ding C, Meng T, Lu W, Liu W, Hao H, et al. Butyrate suppresses motility of colorectal cancer cells via deactivating Akt/ERK signaling in histone deacetylase dependent manner. J Pharmacol Sci. (2017) 135:148–55. doi: 10.1016/j.jphs.2017.11.004
44. Uribe-Herranz M, Rafail S, Beghi S, Gil-de-Gómez L, Verginadis I, Bittinger K, et al. Gut microbiota modulate dendritic cell antigen presentation and radiotherapy-induced antitumor immune response. J Clin Invest. (2020) 130:466–79. doi: 10.1172/JCI124332
45. Xiao X, Cao Y, Chen H. Profiling and characterization of microRNAs responding to sodium butyrate treatment in A549 cells. J Cell Biochem. (2018) 119:3563–73. doi: 10.1002/jcb.v119.4
46. Gomes S, Baltazar F, Silva E, Preto A. Microbiota-derived short-chain fatty acids: new road in colorectal cancer therapy. Pharmaceutics. (2022) 14:2359. doi: 10.3390/pharmaceutics14112359
47. Zagato E, Pozzi C, Bertocchi A, Schioppa T, Saccheri F, Guglietta S, et al. Endogenous murine microbiota member Faecalibaculum rodentium and its human homologue protect from intestinal tumour growth. Nat Microbiol. (2020) 5:511–24. doi: 10.1038/s41564-019-0649-5
48. Zhou Y, Ji X, Chen J, Fu Y, Huang J, Guo R, et al. Short-chain fatty acid butyrate: A novel shield against chronic gastric ulcer. Exp Ther Med. (2021) 21:329. doi: 10.3892/etm.2021.9760
49. Okabe S, Okamoto T, Zhao CM, Chen D, Matsui H. Acetic acid induces cell death: an in vitro study using normal rat gastric mucosal cell line and rat and human gastric cancer and mesothelioma cell lines. J Gastroenterol Hepatol. (2014) 29 Suppl 4:65–9. doi: 10.1111/jgh.2014.29.issue-s4
50. Yan J, Xu YH. Tributyrin inhibits human gastric cancer SGC-7901 cell growth by inducing apoptosis and DNA synthesis arrest. World J Gastroenterol. (2003) 9:660–4. doi: 10.3748/wjg.v9.i4.660
51. Kim YL, Lee W, Chung SH, Yu BM, Lee YC, Hong J. Metabolic alterations of short-chain fatty acids and TCA cycle intermediates in human plasma from patients with gastric cancer. Life Sci. (2022) 309:121010. doi: 10.1016/j.lfs.2022.121010
52. Schoeller A, Karki K, Jayaraman A, Chapkin RS, Safe S. Short chain fatty acids exhibit selective estrogen receptor downregulator (SERD) activity in breast cancer. Am J Cancer Res. (2022) 12:3422–36.
53. Coutzac C, Jouniaux JM, Paci A, Schmidt J, Mallardo D, Seck A, et al. Systemic short chain fatty acids limit antitumor effect of CTLA-4 blockade in hosts with cancer. Nat Commun. (2020) 11:2168. doi: 10.1038/s41467-020-16079-x
54. Hu C, Xu B, Wang X, Wan WH, Lu J, Kong D, et al. Gut microbiota-derived short-chain fatty acids regulate group 3 innate lymphoid cells in HCC. Hepatology. (2023) 77:48–64. doi: 10.1002/hep.32449
55. Lucas S, Omata Y, Hofmann J, Böttcher M, Iljazovic A, Sarter K, et al. Short-chain fatty acids regulate systemic bone mass and protect from pathological bone loss. Nat Commun. (2018) 9:55. doi: 10.1038/s41467-017-02490-4
Keywords: short-chain fatty acid, metabolism, cancer, pathogenesis, diagnosis, treatment
Citation: Hou M, Yu Q-Q, Yang L, Zhao H, Jiang P, Qin L and Zhang Q (2024) The role of short-chain fatty acid metabolism in the pathogenesis, diagnosis and treatment of cancer. Front. Oncol. 14:1451045. doi: 10.3389/fonc.2024.1451045
Received: 19 June 2024; Accepted: 19 September 2024;
Published: 07 October 2024.
Edited by:
Qingfei Zheng, Purdue University, United StatesReviewed by:
Santhakumar Manicassamy, Augusta University, United StatesJinghua Wu, Purdue University, United States
Copyright © 2024 Hou, Yu, Yang, Zhao, Jiang, Qin and Zhang. This is an open-access article distributed under the terms of the Creative Commons Attribution License (CC BY). The use, distribution or reproduction in other forums is permitted, provided the original author(s) and the copyright owner(s) are credited and that the original publication in this journal is cited, in accordance with accepted academic practice. No use, distribution or reproduction is permitted which does not comply with these terms.
*Correspondence: Qiujie Zhang, emhhbmdxaXVqaWU4NkAxNjMuY29t; Lei Qin, cWlubGVpMDA3QDEyNi5jb20=; Pei Jiang, amlhbmdwZWljc3VAc2luYS5jb20=
†These authors have contributed equally to this work