- Department of Otolaryngology—Head and Neck Surgery, Keck School of Medicine of USC, University of Southern California, Los Angeles, CA, United States
Head and neck squamous cell carcinoma (HNSCC) is the sixth most common cancer globally. Notably, human papillomavirus (HPV)-positive oropharyngeal squamous cell carcinoma (OPSCC) is on the rise, accounting for 70% of all OPSCC cases. Persistent high-risk HPV infection is linked to various cancers, but HPV infection alone is not sufficient to cause cancer. Advances in next-generation sequencing have improved our understanding of changes in the human microbiome of cancerous environments. Yet, there remains a dearth of knowledge on the impact of HPV-microbiome crosstalk in HPV-positive OPSCC. In this review, we examine what is known about the oropharyngeal microbiome and the compositional shifts in this microbiome in HPV-positive OPSCC. We also review potential mechanisms of crosstalk between HPV and specific microorganisms. Additional research is needed to understand these interactions and their roles on cancer development and progression.
Highlights
Head and neck squamous cell carcinoma (HNSCC) is the sixth most common cancer globally. Notably, human papillomavirus (HPV)-positive oropharyngeal squamous cell carcinoma (OPSCC) is on the rise, accounting for 70% of all OPSCC cases. Persistent high-risk HPV infection is linked to various cancers, but HPV infection alone is not sufficient to cause cancer. Additional factors such as chronic inflammation, immune deficiency, and host microbiome alterations may contribute to cancer development. Recent advances in next-generation sequencing have improved our understanding of the role of human microbiome in cancer. This review focuses on the unique features of the oropharyngeal microbiome and its interaction with HPV in carcinogenesis. It highlights the distinct differences between the oral and oropharyngeal microbiomes and the compositional shifts in the microbiome of HPV-positive OPSCC. The review proposes potential mechanisms of HPV-microbiome crosstalk contributing to carcinogenesis, emphasizing the need for further research to understand these interactions and their impact on cancer development and progression.
Introduction
While the overall incidence of head and neck squamous cell carcinoma (HNSCC) is declining globally, human papillomavirus (HPV)-positive oropharyngeal squamous cell carcinoma (OPSCC) has been increasing, with HPV accounting for 70% of all OPSCC (1–3). HPV is among the most prevalent viral infections worldwide, with nearly all sexually active people being infected with HPV in their lifetime, and half of these being high-risk HPV. Despite this, most people will clear the infection, and only a small minority will have persistent infection (4). It is well-established that persistent high-risk HPV infection is responsible for the development of cervical, anogenital, and oropharyngeal cancers. However, HPV alone is not sufficient to cause cancer (4). Factors such as chronic inflammation, host microbiome alterations, and immune deficiency may be related to pathogenesis, but the mechanisms remain unclear (5).
Recent advances in next generation sequencing have revolutionized our ability to characterize the human microbiome (6). Microbiome studies of different normal and cancer samples have led to improved understanding of associations between microbes and carcinogenesis with potential for risk stratification and prognostication in cancer (7). The oral microbiome harbors over 700 microorganisms (8–10), and disruptions to this microbiome are known to contribute to inflammatory environments with carcinogenic potential (11–14). Firmicutes, Proteobacteria, Bacteroidetes, Fusobacteria, and Actinobacteria have been identified as the most abundant phyla in HNSCC, accounting for > 90% of the oral bacterial community (15, 16). The association between oral dysbiosis and oral squamous cell carcinoma (OSCC) has been well established (15–20). However, the oropharyngeal microbiome and its association with HPV-positive OPSCC are just being uncovered (5). Furthermore, as HPV infection alone is insufficient for cancer development, it is crucial to explore the possibility of HPV-microbial crosstalk and its role in carcinogenesis.
It is important to distinguish the microbiomes of oral cavity and oropharyngeal cancers. The oral cavity microbiome is heavily influenced by environmental factors and subsequent dysbiosis leads to HPV-negative OSCC (19, 21, 22). On the other hand, being primarily HPV driven, OPSCC is an entirely different disease which may be less influenced by environmental factors (23). This review will focus on the unique features of the oropharyngeal microbiome and its interplay with HPV infection in oropharyngeal carcinogenesis. Many reviews discuss the associations between the oral microbiome and OSCC, which will not be discussed. Given the lack of studies on HPV-microbial crosstalk in the context of OPSCC, we also aim to propose potential mechanisms of crosstalk between HPV and specific microbes and highlight areas requiring future study.
Literature search strategy
We conducted a review of the literature to identify articles addressing the oropharyngeal microbiome, the oropharyngeal microbiome in HPV-positive OPSCC, and HPV-oropharyngeal microbial crosstalk in OPSCC carcinogenesis. This work is not a systematic review; therefore, we did not use PRISMA guidelines. Each query of PubMed’s online repository used the keywords “oropharyngeal microbiome” or “oropharynx [MeSH] microbiota [MeSH].” These keywords were paired with combinations of “human papillomavirus,” “HPV,” “human papillomavirus [MeSH],” and “oropharyngeal neoplasms [MeSH].” Articles were sorted based on relevance and evaluated for content. Exclusion criteria included non-English studies, studies with only pediatric participants, and studies that utilized non-specific oropharyngeal collection methods.
Microbiome of normal oropharynx and HPV-positive OPSCC
The normal oropharyngeal microbiome
While there is overlap between the oral and oropharyngeal microbiome, the unique function and histology of the oropharynx lends to differences in how each region interacts with its microbiome. Most studies recognize six major phyla present in the oropharynx, including Firmicutes (44%), Bacteroidetes (20%), Fusobacteria (15%), Proteobacteria (14%), Actinobacteria (6%), and Spirochaetes (0.5%) as comprising over 99% of the microbiome (Table 1) (37). The most common genera of bacteria include Streptococcus (24%), Prevotella (11%), Fusobacterium (10%), Veillonella (8%), Neisseria (5%), and Actinomyces (4%). The relative abundance of anaerobic bacteria in the oropharynx is slightly higher than the oral cavity, likely due to the anaerobic niche of the tonsillar crypts (38).
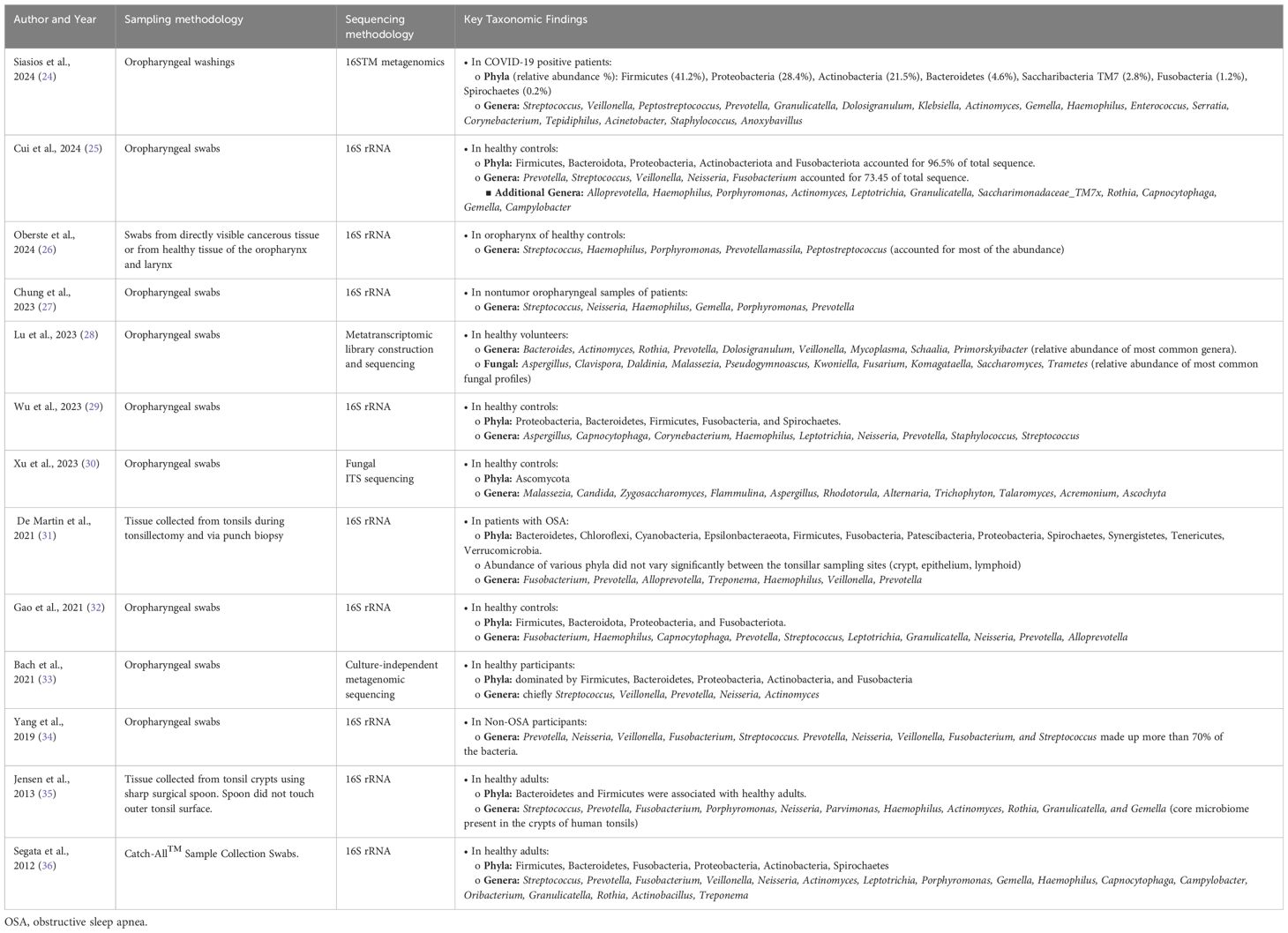
Table 1. Summary of the key taxonomic findings in the normal oropharyngeal microbiome based on sampling methods specific to the oropharynx.
The oropharyngeal cancer microbiome
Few studies have compared the microbiomes of normal oropharynx and oropharyngeal cancers (Table 2). Differences in sample collection ranging from saliva samples or oral washes, which is non-specific to the oropharynx, to oropharyngeal swabs and tissues, which is more specific to the oropharynx, has led to discordance in the literature (38, 44–50). As the normal flora of the oropharynx is dominated by a small number of phyla and genera of bacteria, identifying significant differences between normal and cancer requires careful evaluation using precise methods. Some studies report significant differences in bacteria that account for less than 0.1% of the overall abundance of the whole oropharyngeal microbiome, putting in question the real biological relevance of these differences (38, 44, 49). In this review, we focus on the shifts in relative abundance in the more abundant bacteria of the oropharynx as well as those with evidence of pathogenic roles in association with HPV (Figure 1).
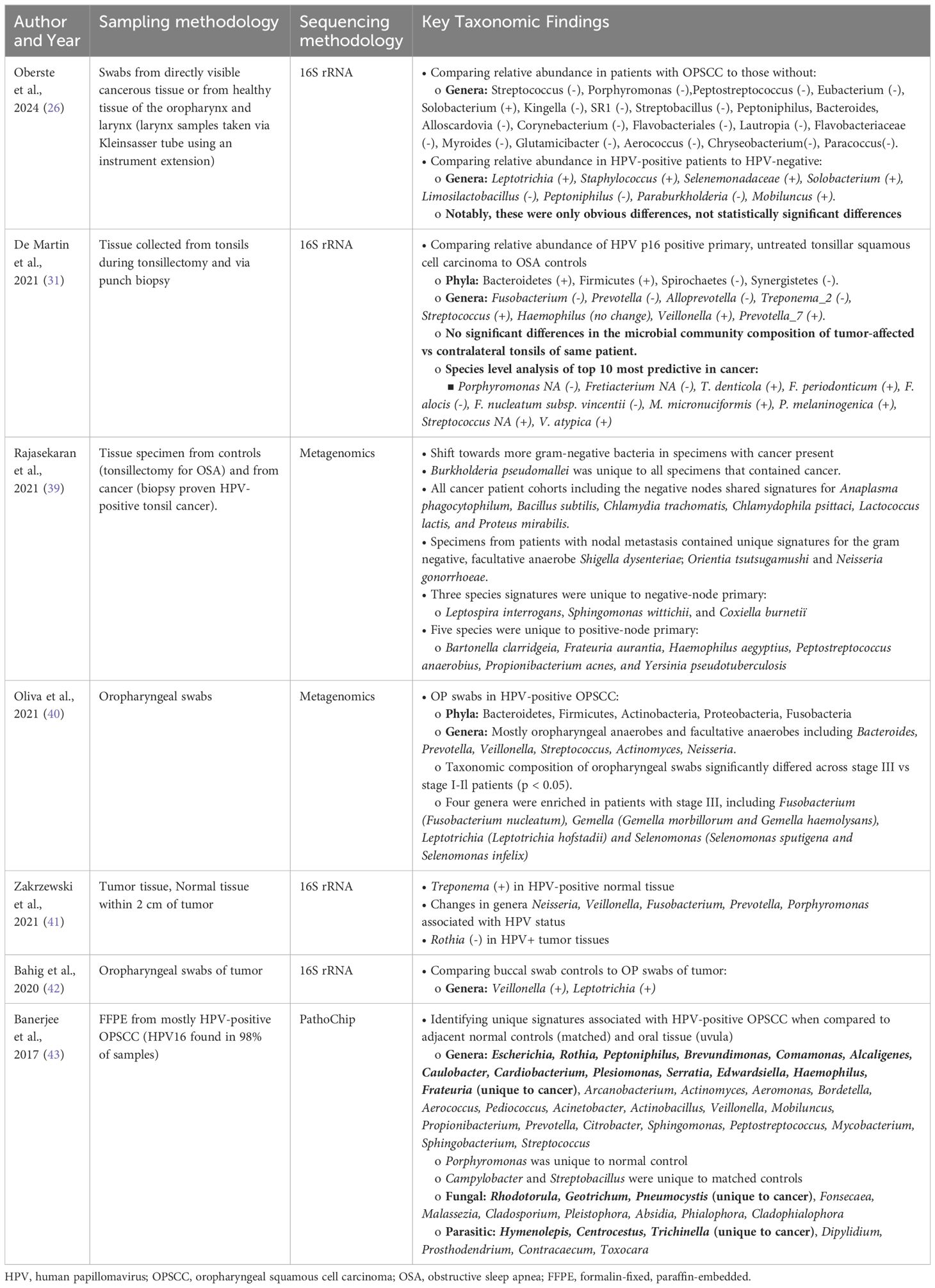
Table 2. Summary of the key taxonomic findings in the HPV-positive OPSCC microbiome based on sampling methods specific to the oropharynx.
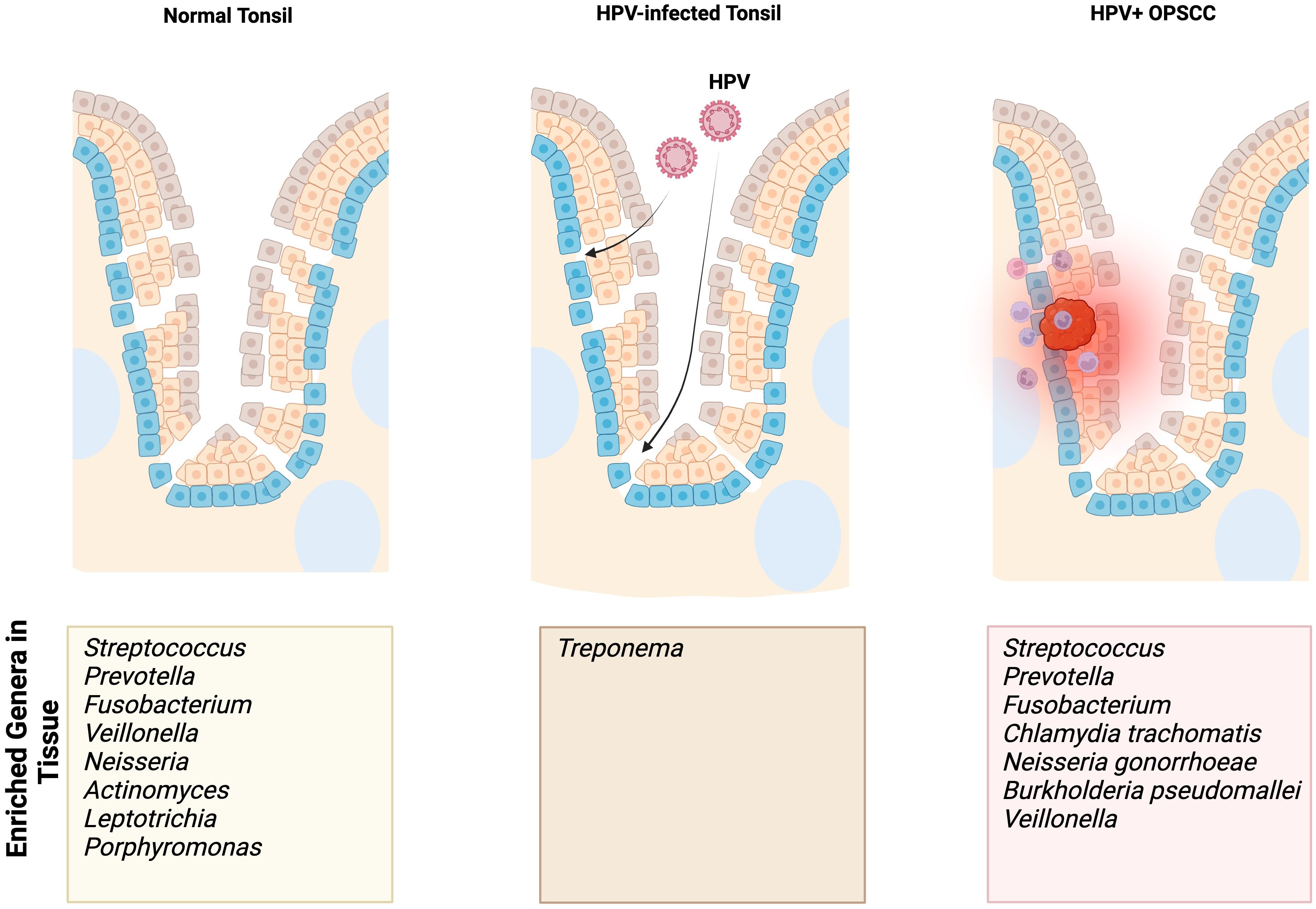
Figure 1. Summary findings of enriched genera/species identified in normal, HPV-infected premalignant, and HPV-positive OPSCC tonsils based on our focused review of the literature. The enriched genera/species outlined in this figure result from studies using sampling methods specific to the tonsils.
When comparing the relative abundances of the tonsillar microbiota in patients with OPSCC and OSA controls, De Martin et al. found a relative increase in Firmicutes and Bacteroidetes at the phylum level (48). At the genus level, there was a relatively decreased abundance of Fusobacterium and a relatively increased abundance of Streptococcus (48). One study found enriched presence of Chlamydia trachomatis in HPV-positive tonsil SCC and patients with more advanced disease were colonized with Neisseria gonorrhoeae (47).
Crosstalk between HPV and the oropharyngeal microbiome
Persistent HPV infection in the oropharynx is a known risk factor for the development of OPSCC. While 4% of adults have oral high-risk HPV DNA present, not all develop persistent infection or OPSCC (4, 51–53). Patients with detectable oral HPV (vs non-detectable HPV) and patients with HPV-positive OPSCC (vs HPV-negative OPSCC) have significant shifts in oral microbiome (54–57). Other studies have similarly identified compositional shifts in the vaginal microbiota of pre-malignant lesions and cervical cancer, with mechanistic evidence of HPV-microbial crosstalk contributing to neoplastic progression (58–62). Therefore, HPV-microbial crosstalk may contribute to neoplastic progression in the oropharynx.
After our review of the literature, no study has independently validated the interplay between specific microbes and HPV in OPSCC. Some have reported the oral bacterial signatures of HPV-positive OPSCC, but these are primarily descriptive (9, 17, 63–65). In the subsequent sections, we propose potential mechanisms of crosstalk between HPV and specific microbes identified from our literature search in promoting HPV-related carcinogenesis.
HPV-Neisseria/Chlamydia: overview
HPV-positive OPSCC patients exhibit tumor microbiome enriched in Neisseria, particularly in regionally metastatic disease (Table 2) (45–47). Furthermore, one study identified Neisseria gonorrhoeae (NG) as the only signature present in patients with nodal disease and Chlamydia trachomatis (CT) as a shared signature amongst all HPV-positive OPSCC patient cohorts (Table 2) (47). These findings contribute to the growing evidence linking Neisseria and Chlamydia to persistent HPV infection and HPV-positive cancers of the cervix and oropharynx (66–68).
The carcinogenic potential of the crosstalk between HPV-NG and HPV-CT in the oropharynx may stem from their abilities to enable persistent HPV infection within tissues. Cervicitis is associated with prolonged duration of HPV infection as well as an increased likelihood of high-grade cervical dysplasia (69, 70). Furthermore, high-risk HPV (HR-HPV) and NG co-infection showed an increased risk of atypical squamous cells of undetermined significance (≥ ASC-US) and high-grade squamous intraepithelial lesions (HSIL) (66). HPV-CT co-infection resulted in 5 times higher risk of both ≥ ASC-US and HSIL. A meta-analysis of 22 studies confirmed that HPV-CT co-infection conferred a higher risk of cervical cancer than either alone (71). As NG and CT are primarily GU pathogens, they are thought to be transplanted to the oropharynx during oral sex (59) and subsequently cause chronic subclinical infections, with a predilection to tonsillar tissues where they are difficult to eradicate (72, 73).
HPV-NG crosstalk: proposed mechanisms
Although both NG and CT contribute to persistence of HPV infection in the oropharynx, their underlying mechanisms may differ. NG disrupts the mucosal barrier by inhibiting epithelial cell renewal and exfoliation. More specifically, NG can engage human carcinoembryonic antigen-related cell adhesion molecules (CEACAMs) present within pharyngeal and urogenital mucosal surfaces by using outer membrane adhesins (74–76). Once engaged, NG blocks detachment of infected epithelial cells from the extracellular matrix (ECM) by triggering the full-length expression of CD105. CD105 is sufficient to inhibit infection-induced detachment, suggesting a mechanism by which NG can disrupt the innate defense mechanism of epithelial exfoliation (77).
In vitro studies have demonstrated that NG can also induce DNA damage. Normal vaginal mucosa and cervical adenocarcinoma cell lines infected with NG displayed significantly more DNA strand breaks compared to uninfected controls (78). NG was also shown to induce the cell cycle inhibitors, p21 and p27 (79). Interestingly, NG infected cells can evade DNA damage-induced apoptosis via downregulation of tumor suppressor p53 (78). Through expression of Tfp/pilT, NG can activate the extracellular signal-regulated kinase (ERK) prosurvival pathway resulting in downregulation of proapoptotic proteins Bim and Bad (80). By inhibiting apoptosis in host cells, NG can delay the immune response, triggering a “carrier state” and/or intracellular survival. In HPV-infected epithelium, such a survival mechanism may confer greater immortality in combination with well described oncoproteins HPV E6/E7.
HPV-CT crosstalk: proposed mechanisms
At a molecular level, CT can also traumatize the epithelium of susceptible mucosal tissues, creating opportunities for microabrasions that promote HPV entry (81). Persistent CT infection in epithelial cells increases the secretion of proinflammatory cytokines IL-8, GROɑ, GM-CSF, and IL-6, which can cause oxidative stress (82). Moreover, the epithelial cells continue to release IL-1ɑ after CT infection, triggering neighboring non-infected epithelial cells to produce additional cytokines, sustaining the inflammatory response and ultimately leading to epithelial tissue damage (82). Of note, HPV-CT co-infected cervical lesions demonstrate significantly upregulated expression of NF-kB compared to non-CT infected cervical lesions. NF-kB is a family of transcription factors that play critical roles in intracellular regulation of immune response and inflammation (83, 84). The upregulated NF-kB pathway caused by HPV-CT co-infection can exacerbate IL-8 mediated epithelial tissue damage, facilitating HPV virion penetrance (85). Furthermore, CT infection has been shown to disrupt N-cadherin-dependent cell-cell junctions in human cervical epithelial cells, resulting in an increase in epithelial paracellular permeability (86). This may explain how CT can increase the risk of HPV acquisition and persistence and how HPV-CT co-infection can increase risk of carcinogenesis (87, 88).
Beyond epithelial disruption, CT can also disturb the immune response necessary to clear HPV, leading to persistent infection. CT can cause a shift in the immune landscape from a cell-mediated response to a humoral response due to its chronic infection in mucosal tissues (83, 89, 90). As cell-mediated immune responses are critical for clearance of HPV infections, a shift to a humoral response creates an environment where HPV infection can thrive (91). Indeed, a cohort study of Swedish women showed that a self-reported history of CT infection was the most significant risk factor for presence of HPV DNA in the blood (92). Furthermore, the CT outer membrane pore protein OmpA can inhibit apoptosis in infected host cells by targeting the pro-apoptotic proteins Bax and Bak. Similar to HPV-NG crosstalk, inhibition of apoptosis may compound the anti-apoptotic effect of HPV E6/E7 and is a hallmark of carcinogenesis (93).
Chronic CT infection can also promote carcinogenesis via DNA damage caused by reactive oxygen species (94). One study used an HPV16 infected ectocervix organoid and CT co-culture system to model HPV-CT crosstalk (61). Using this model, they found that CT impedes HPV-induced mechanisms that maintain genome integrity, including mismatch repair, creating a genotoxic effect in host cells. Furthermore, HPV16 E6/E7 integration in the host genome slows the CT life cycle and induces persistent infection. This study demonstrates the potential complexity of this bidirectional relationship between CT and HPV in cancer development and progression (61).
Summary of HPV-NG/CT crosstalk
The crosstalk between HPV-NG and HPV-CT may contribute to carcinogenesis in the oropharynx by disrupting the normal mucosal barrier and facilitating entry of HPV virions, delaying immune response, and promoting genotoxicity. Additional research is required to evaluate the impact of HPV-NG and HPV-CT co-infection in oropharyngeal models.
HPV-Fusobacterium: overview and proposed mechanisms
Fusobacterium spp are well established periodontal pathogens (95). Of these, Fusobacterium nucleatum (FN) is well known for its role in OSCC development (96–98). FN colonization increases risk of OSCC by augmenting IL-6-STAT3 signaling pathways, promoting tumor growth and invasiveness (15, 99). However, studies on the oropharyngeal microbiome suggest the contrary: HPV-positive OPSCC exhibits a reduction of Fusobacterium spp compared to the normal oropharynx (48). As with NG and CT, such differences can be partially explained by differences in experimental methods: lack of stratification of HPV-positive to HPV-negative, sampling methods, and sequencing methodology (100).
Although less prominent in HPV-positive OPSCC compared to otherwise healthy adults, progressive over-representation of FN has been characterized with stage III HPV-positive OPSCC patients, showing a significantly higher relative abundance of FN compared to earlier stages. This correlation between FN representation and stage is consistent with that of OSCC and gastrointestinal squamous cell carcinoma studies (46, 98, 101–103). Furthermore, some have proposed that patients with stage III HPV-positive OPSCC are at a higher risk of recurrence despite definitive concurrent chemoradiation due to differences in FN enrichment (104). Ultimately, the etiology explaining these associations remain unclear. HPV-FN co-infection in the tonsillar tissues of susceptible patients can be a contributing factor.
The potential mechanisms by which FN contributes to cancerous growth have been studied in the colon and oral cavity. FadA, a virulence factor expressed by FN, is a cell surface adhesion protein involved in the attachment and invasion of epithelial cells. It can interact with E-cadherin of epithelial cells to stimulate the Wnt/β-catenin pathway (105). As a result, β-catenin can activate the expression of cyclin D1, c-Myc, and Wnt signaling genes, which promote cell proliferation and tumor growth. HR-HPV oncoproteins also activate the canonical Wnt/β-catenin pathway, which further contribute to the onset, progression, and maintenance of transformed cells (106). Studies in cervical cancer have highlighted the role of Wnt signaling as the “second hit,” responsible for transforming HPV-immortalized cells (107). Therefore, HPV-FN crosstalk may result in a multiplicative effect on the growth of HPV-positive cancers (Figure 2).
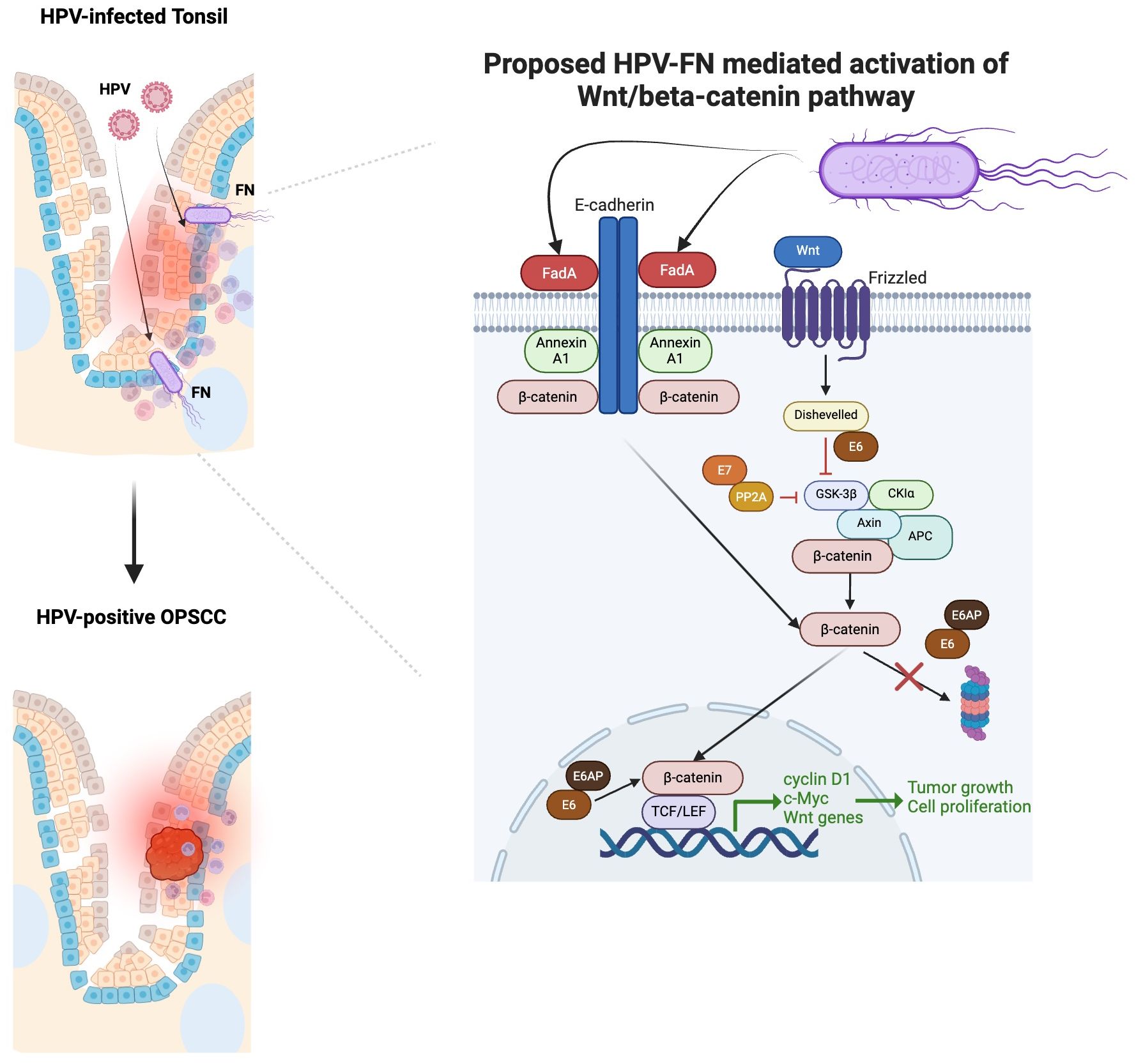
Figure 2. Proposed multiplicative effect HPV-Fusobacterium nucleatum (FN) crosstalk in contributing to HPV-positive OPSCC based on our focused review of the literature. FadA binding enhances when Annexin A1 level increases (105). Annexin A1 is significantly elevated in the margins of HPV-positive OPSCC compared to HPV-negative OPSCC (108). FadA can interact with E-cadherin of epithelial cells to stimulate the Wnt/β-catenin pathway (105). As a result, β-catenin can activate the expression of cyclin D1, c-Myc, and Wnt signaling genes, which promote cell proliferation and tumor growth. HR-HPV oncoproteins E6/E7 can also activate the canonical Wnt/β-catenin pathway, which further contribute to the onset, progression, and maintenance of transformed cells (106). E7 binds to PP2A in the structural and catalytic domain to contribute to the inhibition of GSK3β activation (106). The E6/E6AP complex can stabilize β-catenin, avoiding its proteasomal degradation and promoting its nuclear translocation. The binding of E6 to Dishevelled can disrupt the β-catenin degradation complex.
HPV-FN crosstalk may also alter mucosal integrity, predisposing to HPV infection and OPSCC progression. FN produces matrix metalloproteinases, which are capable of degrading components of the extracellular matrix (ECM) (109). FN can also promote the epithelial-mesenchymal transition (EMT), predisposing cells to malignant transformation. One study in oral epithelial cells demonstrated that FN infection can trigger EMT in both normal and cancerous cells via the lncRNA MIR4435–2HG/miR-296–5p/Akt2/SNAIl signaling pathway, a process that also downregulates E-cadherin (110), thereby breaking down the epithelial barrier and allowing HPV infection and malignant transformation (111). Additional research must explore the mechanisms by which HPV-FN crosstalk can foster tumor growth, progression, and clinical outcomes.
HPV-Streptococcus: overview and proposed mechanism
Oropharyngeal microbiome studies have also revealed that HPV-positive OPSCC patients demonstrate enriched signatures of the genus Streptococcus (38, 46, 48). Streptococcus has been heavily associated with both periodontal inflammation as well as OSCC, and is one of the most common microbes in the oral cavity and oropharynx (11, 15, 16, 112). Furthermore, one study of salivary samples from patients with OPSCC revealed that the relative abundance of Streptococcus could be used to differentiate tumors from control samples (17). As Streptococcus spp are commensal to the oral cavity and oropharynx, environmental insults that trigger dysbiosis may allow for opportunistic organisms to colonize (113). Streptococcus mutans (SM), a viridans group streptococcus (VGS), represents one of these species. SM has been shown to induce IL-6 expression in human and mouse oral cancer cell lines, representing a specific mechanism for its potential carcinogenicity (114). As with the other microbes mentioned, it is uncertain whether the changes observed in streptococci are the cause or consequence of cancer.
One study demonstrated that Streptococcus may promote HPV16 entry into basal keratinocytes by supplying a furin-like peptidase (115). HPV16 infection is initiated by binding to receptor heparan sulfate proteoglycans (HSPGs) on the exposed basement membrane (81, 115, 116). Following binding to HSPGs, the L2 cleavage site is exposed and subsequently cleaved by furin, facilitating HPV16 entry into tissues (116). Unlike HSPGs, the expression of furin varies based on tissues (117). In the human oropharyngeal epithelium, furin expression is low in the basal layers where HPV16 typically initiates infections (118). One study revealed that PepO, the furin-like peptidase of S. gordonii, promoted HPV16 infection (119). Altogether, this suggests that HPV-Streptococcus crosstalk may allow for better penetration of HPV into the basal keratinocytes, where it can proliferate.
Conclusions and future directions
The intricate composition of the oropharyngeal microbiome is only beginning to be understood. Previous studies have focused on saliva due to ease of access (16, 65, 120). Although these findings have been used as a mirror of the oropharyngeal microbiome, targeted collection methods have shown unique signatures of bacteria and other microbes in this region (37, 47, 48). Traditional periodontal pathogens found in OSCC (Porphyromonas gingivalis and Treponema denticolum) did not present as key players (19, 121). Instead, FN and SM provide pro-inflammatory environments, in conjunction with chronic HPV infection, that may increase propensity for the development of cancer (46, 48). High quality mechanistic studies have yet to provide a comprehensive landscape of these interactions critical for the development of novel therapy.
We propose that HPV-microbial crosstalk impacts patient outcomes at multiple levels. First, it facilitates HPV virion entry to the basal keratinocytes and fosters an immune environment that also allows for HPV persistence. Furthermore, as demonstrated in other solid tumors, direct and secreted microbial features may confer treatment resistance in advanced stages of HPV-positive OPSCC (101, 103). Key microbial signatures of HPV-positive OPSCC may provide useful biomarkers for deintensification of patients.
Novel reductionist approaches utilizing tissue-microbe co-culture may be one way to model microbial effects on HPV-associated carcinogenesis (61). These studies would also benefit from including fungi, proteus, and bacteriophages, which occupy their own niche roles in the microbiome. In cervical cancer, specific fungal communities (Candida, Malassezia, and Sporidiobolaceae) were identified as significantly associated with HR-HPV and premalignant cervical lesions (122). We believe similar associations occur in the oropharynx, triggering carcinogenesis in the HPV-infected tonsil. As the incidence of HPV-positive OPSCC begins to exceed that of cervical cancer, it is necessary to understand the functional relationships between microbes and HPV to characterize oropharyngeal premalignant lesions and enhance our understanding of HPV-related carcinogenesis (123).
Author contributions
RC: Conceptualization, Data curation, Investigation, Visualization, Writing – original draft, Writing – review & editing. SW: Conceptualization, Investigation, Supervision, Validation, Writing – original draft, Writing – review & editing. DL: Conceptualization, Investigation, Supervision, Writing – original draft, Writing – review & editing. NK: Conceptualization, Investigation, Supervision, Writing – original draft, Writing – review & editing. US: Conceptualization, Investigation, Supervision, Writing – original draft, Writing – review & editing. AH: Conceptualization, Investigation, Supervision, Validation, Visualization, Writing – original draft, Writing – review & editing.
Funding
The author(s) declare that no financial support was received for the research, authorship, and/or publication of this article.
Conflict of interest
The authors declare that the research was conducted in the absence of any commercial or financial relationships that could be construed as a potential conflict of interest.
Publisher’s note
All claims expressed in this article are solely those of the authors and do not necessarily represent those of their affiliated organizations, or those of the publisher, the editors and the reviewers. Any product that may be evaluated in this article, or claim that may be made by its manufacturer, is not guaranteed or endorsed by the publisher.
References
1. Mourad M, Jetmore T, Jategaonkar AA, Moubayed S, Moshier E, Urken ML. Epidemiological trends of head and neck cancer in the United States: A SEER population study. J Oral Maxillofac Surg. (2017) 75:2562–72. doi: 10.1016/j.joms.2017.05.008
2. Global Burden of Disease Cancer Collaboration. Global, regional, and national cancer incidence, mortality, years of life lost, years lived with disability, and disability-adjusted life-years for 29 cancer groups, 1990 to 2017: A systematic analysis for the global burden of disease study. JAMA Oncol. (2019) 5:1749–68. doi: 10.1001/jamaoncol.2019.2996
3. HPV and Cancer - NCI. (2019). Available online at: https://www.cancer.gov/about-cancer/causes-prevention/risk/infectious-agents/hpv-and-cancer.
4. Bodily J, Laimins LA. Persistence of human papillomavirus infections: keys to Malignant progression. Trends Microbiol. (2011) 19:33–9. doi: 10.1016/j.tim.2010.10.002
5. McKeon M, Gallant JN, Kim Y, Das S. It takes two to tango: A review of oncogenic virus and host microbiome associated inflammation in head and neck cancer. Cancers. (2022) 14:3120. doi: 10.3390/cancers14133120
6. Turnbaugh PJ, Ley RE, Hamady M, Fraser-Liggett CM, Knight R, Gordon JI, et al. The human microbiome project. Nature. (2007) 449:804–10. doi: 10.1038/nature06244
7. Nejman D, Livyatan I, Fuks G, Gavert N, Zwang Y, Geller LT, et al. The human tumor microbiome is composed of tumor type-specific intracellular bacteria. Science. (2020) 368:973–80. doi: 10.1126/science.aay9189
8. Xian P, Xuedong Z, Xin X, Yuqing L, Yan L, Jiyao L, et al. The oral microbiome bank of China. Int J Oral Sci. (2018) 10:1–9. doi: 10.1038/s41368-018-0018-x
9. Peng X, Cheng L, You Y, Tang C, Ren B, Li Y, et al. Oral microbiota in human systematic diseases. Int J Oral Sci. (2022) 14:1–11. doi: 10.1038/s41368-022-00163-7
10. Caselli E, Fabbri C, D’Accolti M, Soffritti I, Bassi C, Mazzacane S, et al. Defining the oral microbiome by whole-genome sequencing and resistome analysis: the complexity of the healthy picture. BMC Microbiol. (2020) 20:120. doi: 10.1186/s12866-020-01801-y
11. Hsiao JR, Chang CC, Lee WT, Huang CC, Ou CY, Tsai ST, et al. The interplay between oral microbiome, lifestyle factors and genetic polymorphisms in the risk of oral squamous cell carcinoma. Carcinogenesis. (2018) 39:778–87. doi: 10.1093/carcin/bgy053
12. Lax AJ, Thomas W. How bacteria could cause cancer: one step at a time. Trends Microbiol. (2002) 10:293–9. doi: 10.1016/S0966-842X(02)02360-0
13. Vogelmann R, Amieva MR. The role of bacterial pathogens in cancer. Curr Opin Microbiol. (2007) 10:76–81. doi: 10.1016/j.mib.2006.12.004
14. Frank DN, Qiu Y, Cao Y, Zhang S, Lu L, Kofonow JM, et al. A dysbiotic microbiome promotes head and neck squamous cell carcinoma. Oncogene. (2022) 41:1269–80. doi: 10.1038/s41388-021-02137-1
15. Ganly I, Yang L, Giese RA, Hao Y, Nossa CW, Morris LGT, et al. Periodontal pathogens are a risk factor of oral cavity squamous cell carcinoma, independent of tobacco and alcohol and human papillomavirus. Int J Cancer. (2019) 145:775–84. doi: 10.1002/ijc.32152
16. Lee WH, Chen HM, Yang SF, Liang C, Peng CY, Lin FM, et al. Bacterial alterations in salivary microbiota and their association in oral cancer. Sci Rep. (2017) 7:16540. doi: 10.1038/s41598-017-16418-x
17. Guerrero-Preston R, Godoy-Vitorino F, Jedlicka A, Rodríguez-Hilario A, González H, Bondy J, et al. 16S rRNA amplicon sequencing identifies microbiota associated with oral cancer, human papilloma virus infection and surgical treatment. Oncotarget. (2016) 7:51320–34. doi: 10.18632/oncotarget.v7i32
18. Nagy KN, Sonkodi I, Szöke I, Nagy E, Newman HN. The microflora associated with human oral carcinomas. Oral Oncol. (1998) 34:304–8. doi: 10.1016/S1368-8375(98)80012-2
19. Kylmä AK, Jouhi L, Listyarifah D, Mohamed H, Mäkitie A, Remes SM, et al. Treponema denticola chymotrypsin-like protease as associated with HPV-negative oropharyngeal squamous cell carcinoma. Br J Cancer. (2018) 119:89–95. doi: 10.1038/s41416-018-0143-5
20. Katz J, Onate MD, Pauley KM, Bhattacharyya I, Cha S. Presence of Porphyromonas gingivalis in gingival squamous cell carcinoma. Int J Oral Sci. (2011) 3:209–15. doi: 10.4248/IJOS11075
21. Chowdhry R, Singh N, Sahu DK, Tripathi RK, Mishra A, Singh A, et al. Dysbiosis and variation in predicted functions of the granulation tissue microbiome in HPV positive and negative severe chronic periodontitis. BioMed Res Int. (2019) 2019:8163591. doi: 10.1155/2019/8163591
22. Nouri Z, Choi SW, Choi IJ, Ryu KW, Woo SM, Park SJ, et al. Exploring connections between oral microbiota, short-chain fatty acids, and specific cancer types: A study of oral cancer, head and neck cancer, pancreatic cancer, and gastric cancer. Cancers. (2023) 15:2898. doi: 10.3390/cancers15112898
23. Lechner M, Liu J, Masterson L, Fenton TR. HPV-associated oropharyngeal cancer: epidemiology, molecular biology and clinical management. Nat Rev Clin Oncol. (2022) 19:306–27. doi: 10.1038/s41571-022-00603-7
24. Siasios P, Giosi E, Ouranos K, Christoforidi M, Dimopoulou I, Leshi E, et al. Oropharyngeal Microbiome Analysis in Patients with Varying SARS-CoV-2 Infection Severity: A Prospective Cohort Study. J Pers Med. (2024) 14:369. doi: 10.3390/jpm14040369
25. Cui G, Sun Y, Zou Y, Sun R, Gao Y, Liu X, et al. Dynamic changes of Bacterial Microbiomes in Oropharynx during Infection and Recovery of COVID-19 Omicron Variant. PloS Pathog. (2024) 20:e1012075. doi: 10.1371/journal.ppat.1012075
26. Oberste M, Böse BE, Dos Anjos Borges LG, Junca H, Plumeier I, Kahl S, et al. Effects of squamous cell carcinoma and smoking status on oropharyngeal and laryngeal microbial communities. Head Neck. (2024) 46:145–60. doi: 10.1002/hed.27562
27. Chung A, Chung Y-T, Liang Y-W, Chung Y-L. Waldeyer ring microbiome in relation to chemoradiation-induced oral mucositis in patients with nasopharyngeal carcinoma. Head Neck. (2023) 45:2047–57. doi: 10.1002/hed.27431
28. Lu S, Zhou Y, Hu Y, Wang J, Li H, Lin Y, et al. Metatranscriptomic analysis revealed Prevotella as a potential biomarker of oropharyngeal microbiomes in SARS-CoV-2 infection. Front Cell Infect Microbiol. (2023) 13:1161763. doi: 10.3389/fcimb.2023.1161763
29. Wu J, Liu W, Zhu L, Li N, Luo G, Gu M, et al. Dysbiosis of oropharyngeal microbiome and antibiotic resistance in hospitalized COVID-19 patients. J Med Virol. (2023) 95:e28727. doi: 10.1002/jmv.28727
30. Xu X, Ding F, Hu X, Yang F, Zhang T, Dong J, et al. Upper respiratory tract mycobiome alterations in different kinds of pulmonary disease. Front Microbiol. (2023) 14:1117779. doi: 10.3389/fmicb.2023.1117779
31. De Martin A, Lütge M, Stanossek Y, Engetschwiler C, Cupovic J, Brown K, et al. Distinct microbial communities colonize tonsillar squamous cell carcinoma. OncoImmunology. (2021) 10:1945202. doi: 10.1080/2162402X.2021.1945202
32. Gao M, Wang H, Luo H, Sun Y, Wang L, Ding S, et al. Characterization of the Human Oropharyngeal Microbiomes in SARS-CoV-2 Infection and Recovery Patients. Adv Sci. (2021) 8:2102785. doi: 10.1002/advs.202102785
33. Bach LL, Ram A, Ijaz UZ, Evans TJ, Lindström J. A longitudinal study of the human oropharynx microbiota over time reveals a common core and significant variations with self-reported disease. . Front Microbiol. (2021) 11:573969. doi: 10.3389/fmicb.2020.573969
34. Yang W, Shao L, Heizhati M, Wu T, Yao X, Wang Y, et al. Oropharyngeal Microbiome in Obstructive Sleep Apnea: Decreased Diversity and Abundance. J Clin Sleep Med. (2019) 15:1777–88. doi: 10.5664/jcsm.8084
35. Jensen A, Fagö-Olsen H, Sørensen CH, Kilian M. Molecular mapping to species level of the tonsillar crypt microbiota associated with health and recurrent tonsillitis. PloS One. (2013) 8:e56418. doi: 10.1371/journal.pone.0056418
36. Segata N, Haake SK, Mannon P, Lemon KP, Waldron L, Gevers D, et al. Composition of the adult digestive tract bacterial microbiome based on seven mouth surfaces, tonsils, throat and stool samples. Genome Biol. (2012) 13:R42. doi: 10.1186/gb-2012-13-6-r42
37. Segata N, Haake SK, Mannon P, Lemon KP, Waldron L, Gevers D, et al. Composition of the adult digestive tract bacterial microbiome based on seven mouth surfaces, tonsils, throat and stool samples. Genome Biol. (2012) 13:R42. doi: 10.1186/gb-2012-13-6-r42
38. Banerjee S, Tian T, Wei Z, Peck KN, Shih N, Chalian AA, et al. Microbial signatures associated with oropharyngeal and oral squamous cell carcinomas. Sci Rep. (2017) 7:4036. doi: 10.1038/s41598-017-03466-6
39. Rajasekaran K, Carey RM, Lin X, Seckar TD, Wei Z, Chorath K, et al. The microbiome of HPV-positive tonsil squamous cell carcinoma and neck metastasis. Oral Oncol. (2021) 117:105305. doi: 10.1016/j.oraloncology.2021.105305
40. Oliva M, Schneeberger PHH, Rey V, Cho M, Taylor R, Hansen AR, et al. Transitions in oral and gut microbiome of HPV+ oropharyngeal squamous cell carcinoma following definitive chemoradiotherapy (ROMA LA-OPSCC study). Br J Cancer. (2021) 124:1543–51. doi: 10.1038/s41416-020-01253-1
41. Zakrzewski M, Gannon OM, Panizza BJ, Saunders NA, Antonsson A. Human papillomavirus infection and tumor microenvironment are associated with the microbiota in patients with oropharyngeal cancers—pilot study. Head Neck. (2021) 43:3324–30. doi: 10.1002/hed.26821
42. Bahig H, Fuller CD, Mitra A, Yoshida-Court K, Solley T, Ping Ng S, et al. Longitudinal characterization of the tumoral microbiome during radiotherapy in HPV-associated oropharynx cancer. Clin Transl Radiat Oncol. (2021) 26:98–103. doi: 10.1016/j.ctro.2020.11.007
43. Banerjee S, Tian T, Wei Z, Peck KN, Shih N, Chalian AA, et al. Microbial Signatures Associated with Oropharyngeal and Oral Squamous Cell Carcinomas. Sci Rep. (2017) 7:4036. doi: 10.1038/s41598-017-03466-6
44. Bahig H, Fuller CD, Mitra A, Yoshida-Court K, Solley T, Ping Ng S, et al. Longitudinal characterization of the tumoral microbiome during radiotherapy in HPV-associated oropharynx cancer. Clin Transl Radiat Oncol. (2021) 26:98–103. doi: 10.1016/j.ctro.2020.11.007
45. Zakrzewski M, Gannon OM, Panizza BJ, Saunders NA, Antonsson A. Human papillomavirus infection and tumor microenvironment are associated with the microbiota in patients with oropharyngeal cancers—pilot study. Head Neck. (2021) 43:3324–30. doi: 10.1002/hed.26821
46. Oliva M, Schneeberger PHH, Rey V, Cho M, Taylor R, Hansen AR, et al. Transitions in oral and gut microbiome of HPV+ oropharyngeal squamous cell carcinoma following definitive chemoradiotherapy (ROMA LA-OPSCC study). Br J Cancer. (2021) 124:1543–51. doi: 10.1038/s41416-020-01253-1
47. Rajasekaran K, Carey RM, Lin X, Seckar TD, Wei Z, Chorath K, et al. The microbiome of HPV-positive tonsil squamous cell carcinoma and neck metastasis. Oral Oncol. (2021) 117:105305. doi: 10.1016/j.oraloncology.2021.105305
48. De Martin A, Lütge M, Stanossek Y, Engetschwiler C, Cupovic J, Brown K, et al. Distinct microbial communities colonize tonsillar squamous cell carcinoma. OncoImmunology. (2021) 10:1945202. doi: 10.1080/2162402X.2021.1945202
49. Oberste M, Böse BE, Dos Anjos Borges LG, Junca H, Plumeier I, Kahl S, et al. Effects of squamous cell carcinoma and smoking status on oropharyngeal and laryngeal microbial communities. Head Neck. (2024) 46:145–60. doi: 10.1002/hed.27562
50. Gopinath D, Menon RK, Wie CC, Banerjee M, Panda S, Mandal D, et al. Differences in the bacteriome of swab, saliva, and tissue biopsies in oral cancer. Sci Rep. (2021) 11:1181. doi: 10.1038/s41598-020-80859-0
51. Rieth KKS, Gill SR, Lott-Limbach AA, Merkley MA, Botero N, Allen PD, et al. Prevalence of high-risk human papillomavirus in tonsil tissue in healthy adults and colocalization in biofilm of tonsillar crypts. JAMA Otolaryngol Neck Surg. (2018) 144:231–7. doi: 10.1001/jamaoto.2017.2916
52. Berman TA, Schiller JT. Human papillomavirus in cervical cancer and oropharyngeal cancer: One cause, two diseases. Cancer. (2017) 123:2219–29. doi: 10.1002/cncr.30588
53. McQuillan G, Kruszon-Moran D, Markowitz LE, Unger ER, Paulose-Ram R. Prevalence of HPV in adults aged 18–69: United States, 2011–2014. NCHS Data Brief. (2017) 280):1–8.
54. Zhang Y, D’Souza G, Fakhry C, Bigelow EO, Usyk M, Burk RD, et al. Oral human papillomavirus associated with differences in oral microbiota beta diversity and microbiota abundance. J Infect Dis. (2022) 226:1098–108. doi: 10.1093/infdis/jiac010
55. Dahlstrom KR, Sikora AG, Liu Y, Chang CC, Wei P, Sturgis EM, et al. Characterization of the oral microbiota among middle-aged men with and without human papillomavirus infection. Oral Oncol. (2023) 142:106401. doi: 10.1016/j.oraloncology.2023.106401
56. Börnigen D, Ren B, Pickard R, Li J, Ozer E, Hartmann EM, et al. Alterations in oral bacterial communities are associated with risk factors for oral and oropharyngeal cancer. Sci Rep. (2017) 7:17686. doi: 10.1038/s41598-017-17795-z
57. Mougeot JLC, Beckman MF, Langdon HC, Lalla RV, Brennan MT, Bahrani Mougeot FK. Haemophilus pittmaniae and Leptotrichia spp. Constitute a Multi-Marker Signature in a Cohort of Human Papillomavirus-Positive Head and Neck Cancer Patients. Front Microbiol. (2022) 12:794546/full. doi: 10.3389/fmicb.2021.794546/full
58. Gardella B, Pasquali MF, La Verde M, Cianci S, Torella M, Dominoni M. The complex interplay between vaginal microbiota, HPV infection, and immunological microenvironment in cervical intraepithelial neoplasia: A literature review. Int J Mol Sci. (2022) 23:7174. doi: 10.3390/ijms23137174
59. Lin D, Kouzy R, Jaoude JA, Noticewala SS, Medrano AYD, Klopp AH, et al. Microbiome factors in HPV-driven carcinogenesis and cancers. PloS Pathog. (2020) 16:e1008524. doi: 10.1371/journal.ppat.1008524
60. Mitra A, MacIntyre DA, Marchesi JR, Lee YS, Bennett PR, Kyrgiou M. The vaginal microbiota, human papillomavirus infection and cervical intraepithelial neoplasia: what do we know and where are we going next? Microbiome. (2016) 4:58. doi: 10.1186/s40168-016-0203-0
61. Koster S, Gurumurthy RK, Kumar N, Prakash PG, Dhanraj J, Bayer S, et al. Modelling Chlamydia and HPV co-infection in patient-derived ectocervix organoids reveals distinct cellular reprogramming. Nat Commun. (2022) 13:1030. doi: 10.1038/s41467-022-28569-1
62. Lebeau A, Bruyere D, Roncarati P, Peixoto P, Hervouet E, Cobraiville G, et al. HPV infection alters vaginal microbiome through down-regulating host mucosal innate peptides used by Lactobacilli as amino acid sources. Nat Commun. (2022) 13:1076. doi: 10.1038/s41467-022-28724-8
63. Dorobisz K, Dorobisz T, Zatoński T. The microbiome’s influence on head and neck cancers. Curr Oncol Rep. (2023) 25:163–71. doi: 10.1007/s11912-022-01352-7
64. Metsäniitty M, Hasnat S, Salo T, Salem A. Oral microbiota—A new frontier in the pathogenesis and management of head and neck cancers. Cancers. (2021) 14:46. doi: 10.3390/cancers14010046
65. Wolf A, Moissl-Eichinger C, Perras A, Koskinen K, Tomazic PV, Thurnher D. The salivary microbiome as an indicator of carcinogenesis in patients with oropharyngeal squamous cell carcinoma: A pilot study. Sci Rep. (2017) 7:5867. doi: 10.1038/s41598-017-06361-2
66. de Abreu AL, Malaguti N, Souza RP, Uchimura NS, Ferreira ÉC, Pereira MW, et al. Association of human papillomavirus, Neisseria gonorrhoeae and Chlamydia trachomatis co-infections on the risk of high-grade squamous intraepithelial cervical lesion. Am J Cancer Res. (2016) 6:1371–83.
67. Tamarelle J, Thiébaut ACM, de Barbeyrac B, Bébéar C, Ravel J, Delarocque-Astagneau E. The vaginal microbiota and its association with human papillomavirus, Chlamydia trachomatis, Neisseria gonorrhoeae and Mycoplasma genitalium infections: a systematic review and meta-analysis. Clin Microbiol Infect. (2019) 25:35–47. doi: 10.1016/j.cmi.2018.04.019
68. Lu Z, Zhao P, Lu H, Xiao M. Analyses of human papillomavirus, Chlamydia trachomatis, Ureaplasma urealyticum, Neisseria gonorrhoeae, and co-infections in a gynecology outpatient clinic in Haikou area, China. BMC Womens Health. (2023) 23:117. doi: 10.1186/s12905-023-02259-6
69. Shew ML, Fortenberry JD, Tu W, Juliar BE, Batteiger BE, Qadadri B, et al. Association of condom use, sexual behaviors, and sexually transmitted infections with the duration of genital human papillomavirus infection among adolescent women. Arch Pediatr Adolesc Med. (2006) 160:151–6. doi: 10.1001/archpedi.160.2.151
70. Castle PE, Hillier SL, Rabe LK, Hildesheim A, Herrero R, Bratti MC, et al. An association of cervical inflammation with high-grade cervical neoplasia in women infected with oncogenic human papillomavirus (HPV). Cancer Epidemiol biomark Prev Publ Am Assoc Cancer Res Cosponsored Am Soc Prev Oncol. (2001) 10:1021–7.
71. Zhu H, Shen Z, Luo H, Zhang W, Zhu X. Chlamydia trachomatis infection-associated risk of cervical cancer: A meta-analysis. Med (Baltimore). (2016) 95:e3077. doi: 10.1097/MD.0000000000003077
72. Wiesner PJ, Tronca E, Bonin P, Pedersen AHB, Holmes KK. Clinical spectrum of pharyngeal gonococcal infection. N Engl J Med. (1973) 288:181–5. doi: 10.1056/NEJM197301252880404
73. Ogawa H, Yamazaki Y, Hashiguchi K. Chlamydia trachomatis: a currently recognized pathogen of tonsillitis. Acta Otolaryngol (Stockh). (1988) 105:197–201. doi: 10.3109/00016488809125026
74. Voges M, Bachmann V, Kammerer R, Gophna U, Hauck CR. CEACAM1 recognition by bacterial pathogens is species-specific. BMC Microbiol. (2010) 10:117. doi: 10.1186/1471-2180-10-117
75. Virji M. Pathogenic neisseriae: surface modulation, pathogenesis and infection control. Nat Rev Microbiol. (2009) 7:274–86. doi: 10.1038/nrmicro2097
76. Hauck CR, Meyer TF. Small” talk: Opa proteins as mediators of Neisseria-host-cell communication. Curr Opin Microbiol. (2003) 6:43–9. doi: 10.1016/S1369-5274(03)00004-3
77. Muenzner P, Rohde M, Kneitz S, Hauck CR. CEACAM engagement by human pathogens enhances cell adhesion and counteracts bacteria-induced detachment of epithelial cells. J Cell Biol. (2005) 170:825–36. doi: 10.1083/jcb.200412151
78. Vielfort K, Söderholm N, Weyler L, Vare D, Löfmark S, Aro H, et al. Neisseria gonorrhoeae infection causes DNA damage and affects the expression of p21, p27 and p53 in non-tumor epithelial cells. J Cell Sci. (2013) 126:339–47.
79. Rodrŕiguez-Vilarrupla A, Dí́az C, Canela N, Rahn H-P, Bachs O, Agell N. Identification of the nuclear localization signal of p21(cip1) and consequences of its mutation on cell proliferation. FEBS Lett. (2002) 531:319–23. doi: 10.1016/S0014-5793(02)03549-4
80. Howie HL, Shiflett SL, So M. Extracellular signal-regulated kinase activation by neisseria gonorrhoeae downregulates epithelial cell proapoptotic proteins bad and bim. Infect Immun. (2008) 76:2715–21. doi: 10.1128/IAI.00153-08
81. Horvath CA, Boulet GA, Renoux VM, Delvenne PO, Bogers J-PJ. Mechanisms of cell entry by human papillomaviruses: an overview. Virol J. (2010) 7:11. 10.1186/1743-422X-7-11
82. Rasmussen SJ, Eckmann L, Quayle AJ, Shen L, Zhang YX, Anderson DJ, et al. Secretion of proinflammatory cytokines by epithelial cells in response to Chlamydia infection suggests a central role for epithelial cells in chlamydial pathogenesis. J Clin Invest. (1997) 99:77–87. doi: 10.1172/JCI119136
83. Paba P, Bonifacio D, Di Bonito L, Ombres D, Favalli C, Syrjänen K, et al. Co-expression of HSV2 and chlamydia trachomatis in HPV-positive cervical cancer and cervical intraepithelial neoplasia lesions is associated with aberrations in key intracellular pathways. Intervirology. (2008) 51:230–4. doi: 10.1159/000156481
84. Branca M, Giorgi C, Santini D, Di Bonito L, Ciotti M, Benedetto A, et al. Aberrant expression of VEGF-C is related to grade of cervical intraepithelial neoplasia (CIN) and high risk HPV, but does not predict virus clearance after treatment of CIN or prognosis of cervical cancer. J Clin Pathol. (2006) 59:40–7. doi: 10.1136/jcp.2005.026922
85. Buchholz KR, Stephens RS. Activation of the host cell proinflammatory interleukin-8 response by Chlamydia trachomatis. Cell Microbiol. (2006) 8:1768–79. doi: 10.1111/j.1462-5822.2006.00747.x
86. Prozialeck WC, Fay MJ, Lamar PC, Pearson CA, Sigar I, Ramsey KH. Chlamydia trachomatis disrupts N-cadherin-dependent cell-cell junctions and sequesters beta-catenin in human cervical epithelial cells. Infect Immun. (2002) 70:2605–13. doi: 10.1128/IAI.70.5.2605-2613.2002
87. Safaeian M, Quint K, Schiffman M, Rodriguez AC, Wacholder S, Herrero R, et al. Chlamydia trachomatis and risk of prevalent and incident cervical premalignancy in a population-based cohort. JNCI J Natl Cancer Inst. (2010) 102:1794–804. doi: 10.1093/jnci/djq436
88. Jensen KE, Thomsen LT, Schmiedel S, Frederiksen K, Norrild B, Brule van den Iftner A T, et al. Chlamydia trachomatis and risk of cervical intraepithelial neoplasia grade 3 or worse in women with persistent human papillomavirus infection: a cohort study. JNCI J Natl Cancer Inst. (2010) 102:1794–804. doi: 10.1093/jnci/djq436
89. Verteramo R, Pierangeli A, Mancini E, Calzolari E, Bucci M, Osborn J, et al. Human Papillomaviruses and genital co-infections in gynaecological outpatients. BMC Infect Dis. (2009) 9:1–7. doi: 10.1186/1471-2334-9-16
90. Simonetti AC, Humberto de Lima Melo J, Eleutério de Souza PR, Bruneska D, Luiz de Lima Filho J. Immunological’s host profile for HPV and Chlamydia trachomatis, a cervical cancer cofactor. Microbes Infect. (2009) 11:435–42. doi: 10.1016/j.micinf.2009.01.004
91. Verteramo R, Pierangeli A, Mancini E, Calzolari E, Bucci M, Osborn J, et al. Cell-mediated immune response to human papillomavirus infection. Clin Diagn Lab Immunol. (2001) 8:209–20. doi: 10.1128/CDLI.8.2.209-220.2001
92. Silins I, Ryd W, Strand A, Wadell G, Törnberg S, Hansson BG, et al. Chlamydia trachomatis infection and persistence of human papillomavirus. Int J Cancer. (2005) 116:110–5. doi: 10.1002/ijc.20970
93. Hanahan D, Weinberg RA. The hallmarks of cancer. Cell. (2000) 100:57–70. doi: 10.1016/S0092-8674(00)81683-9
94. Smith JS, Bosetti C, Muñoz N, Herrero R, Bosch FX, Eluf-Neto J, et al. Chlamydia trachomatis and invasive cervical cancer: A pooled analysis of the IARC multicentric case-control study. Int J Cancer. (2004) 111:431–9. doi: 10.1002/ijc.20257
95. Lourenço TGB, Heller D, Silva-Boghossian CM, Cotton SL, Paster BJ, Colombo APV. Microbial signature profiles of periodontally healthy and diseased patients. J Clin Periodontol. (2014) 41:1027–36. doi: 10.1111/jcpe.12302
96. Bronzato JD, Bomfim RA, Edwards DH, Crouch D, Hector MP, Gomes BPFA. Detection of Fusobacterium in oral and head and neck cancer samples: A systematic review and meta-analysis. Arch Oral Biol. (2020) 112:104669. doi: 10.1016/j.archoralbio.2020.104669
97. McIlvanna E, Linden GJ, Craig SG, Lundy FT, James JA. Fusobacterium nucleatum and oral cancer: a critical review. BMC Cancer. (2021) 21:1212. doi: 10.1186/s12885-021-08903-4
98. Yang CY, Yeh YM, Yu HY, Chin CY, Hsu CW, Liu H, et al. Oral microbiota community dynamics associated with oral squamous cell carcinoma staging. Front Microbiol. (2018) 9:862. doi: 10.3389/fmicb.2018.00862
99. Gallimidi AB, Fischman S, Revach B, Bulvik R, Maliutina A, Rubinstein AM, et al. Periodontal pathogens Porphyromonas gingivalis and Fusobacterium nucleatum promote tumor progression in an oral-specific chemical carcinogenesis model. Oncotarget. (2015) 6:22613–23. doi: 10.18632/oncotarget.4209
100. Brzychczy-Sroka B, Talaga-Ćwiertnia K, Sroka-Oleksiak A, Gurgul A, Zarzecka-Francica E, Ostrowski W, et al. Standardization of the protocol for oral cavity examination and collecting of the biological samples for microbiome research using the next-generation sequencing (NGS): own experience with the COVID-19 patients. Sci Rep. (2024) 14:3717. doi: 10.1038/s41598-024-53992-3
101. Yamamura K, Baba Y, Nakagawa S, Mima K, Miyake K, Nakamura K, et al. Human microbiome fusobacterium nucleatum in esophageal cancer tissue is associated with prognosis. Clin Cancer Res. (2016) 22:5574–81. doi: 10.1158/1078-0432.CCR-16-1786
102. Shao D, Vogtmann E, Liu A, Qin J, Chen W, Abnet CC, et al. Microbial characterization of esophageal squamous cell carcinoma and gastric cardia adenocarcinoma from a high-risk region of China. Cancer. (2019) 125:3993–4002. doi: 10.1002/cncr.32403
103. Yamamura K, Izumi D, Kandimalla R, Sonohara F, Baba Y, Yoshida N, et al. Intratumoral fusobacterium nucleatum levels predict therapeutic response to neoadjuvant chemotherapy in esophageal squamous cell carcinoma. Clin Cancer Res. (2019) 25:6170–9. doi: 10.1158/1078-0432.CCR-19-0318
104. Oliva M, Huang SH, Xu W, Su J, Hansen AR, Bratman SV, et al. Impact of cisplatin dose and smoking pack-years in human papillomavirus–positive oropharyngeal squamous cell carcinoma treated with chemoradiotherapy. Eur J Cancer. (2019) 118:112–20. doi: 10.1016/j.ejca.2019.06.019
105. Rubinstein MR, Wang X, Liu W, Hao Y, Cai G, Han YW. Fusobacterium nucleatum Promotes Colorectal Carcinogenesis by Modulating E-Cadherin/β-Catenin Signaling via its FadA Adhesin. Cell Host Microbe. (2013) 14:195–206. doi: 10.1016/j.chom.2013.07.012
106. Muñoz Bello JO, Olmedo Nieva L, Contreras Paredes A, Fuentes Gonzalez AM, Rocha Zavaleta L, Lizano M. Regulation of the wnt/β-catenin signaling pathway by human papillomavirus E6 and E7 oncoproteins. Viruses. (2015) 7:4734–55. doi: 10.3390/v7082842
107. Üren A, Fallen S, Yuan H, Usubütün A, Küçükali T, Schlegel R, et al. Activation of the canonical wnt pathway during genital keratinocyte transformation: A model for cervical cancer progression. Cancer Res. (2005) 65:6199–206. doi: 10.1158/0008-5472.CAN-05-0455
108. Queiroz CJ dos S, Nakata CM de AG, Solito E, Damazo AS. Relationship between HPV and the biomarkers annexin A1 and p53 in oropharyngeal cancer. Infect Agent Cancer. (2014) 9:13. doi: 10.1186/1750-9378-9-13
109. Uitto VJ, Baillie D, Wu Q, Gendron R, Grenier D, Putnins EE, et al. Fusobacterium nucleatum increases collagenase 3 production and migration of epithelial cells. Infect Immun. (2005) 73:1171–9. doi: 10.1128/IAI.73.2.1171-1179.2005
110. Zhang S, Li C, Liu J, Geng F, Shi X, Li Q, et al. Fusobacterium nucleatum promotes epithelial-mesenchymal transiton through regulation of the lncRNA MIR4435–2HG/miR-296–5p/Akt2/SNAI1 signaling pathway. FEBS J. (2020) 287:4032–47. doi: 10.1111/febs.15233
111. Lefevre M, Rousseau A, Rayon T, Dalstein V, Clavel C, Beby-Defaux A, et al. Epithelial to mesenchymal transition and HPV infection in squamous cell oropharyngeal carcinomas: the papillophar study. Br J Cancer. (2017) 116:362–9. doi: 10.1038/bjc.2016.434
112. Alanazi SAS, Alduaiji KTA, Shetty B, Alrashedi HA, Acharya BLG, Vellappally S, et al. Pathogenic features of Streptococcus mutans isolated from dental prosthesis patients and diagnosed cancer patients with dental prosthesis. Microb Pathog. (2018) 116:356–61. doi: 10.1016/j.micpath.2018.01.037
113. Senthil Kumar S, Johnson MDL, Wilson JE. Insights into the enigma of oral streptococci in carcinogenesis. Microbiol Mol Biol Rev. () 0:e00095–23. doi: 10.1128/mmbr.00095-23
114. Tsai MS, Chen YY, Chen WC, Chen MF. Streptococcus mutans promotes tumor progression in oral squamous cell carcinoma. J Cancer. (2022) 13:3358–67. doi: 10.7150/jca.73310
115. Tao L, Pavlova SI, Gasparovich SR, Jin L, Schwartz J. Alcohol metabolism by oral streptococci and interaction with human papillomavirus leads to Malignant transformation of oral keratinocytes. In: Vasiliou V, Zakhari S, Seitz HK, Hoek JB, editors. Biological basis of alcohol-induced cancer. Springer International Publishing, Cham (2015). p. 239–64.
116. Sapp M, Bienkowska-Haba M. Viral entry mechanisms: human papillomavirus and a long journey from extracellular matrix to the nucleus. FEBS J. (2009) 276:7206–16. doi: 10.1111/j.1742-4658.2009.07400.x
117. Bronnimann MP, Calton CM, Chiquette SF, Li S, Lu M, Chapman JA, et al. Furin cleavage of L2 during papillomavirus infection: minimal dependence on cyclophilins. J Virol. (2016) 90:6224–34. doi: 10.1128/JVI.00038-16
118. Lopez de Cicco R, Bassi DE, Page R, Klein-Szanto AJ. Furin expression in squamous cell carcinomas of the oral cavity and other sites evaluated by tissue microarray technology. Acta Odontol Latinoam AOL. (2002) 15:29–37.
119. Pavlova SI, Wilkening RV, Federle MJ, Lu Y, Schwartz J, Tao L. Streptococcus endopeptidases promote HPV infection in vitro. MicrobiologyOpen. (2019) 8:e00628. doi: 10.1002/mbo3.628
120. Lu H, Zou P, Zhang Y, Zhang Q, Chen Z, Chen F. The sampling strategy of oral microbiome. iMeta. (2022) 1:e23. doi: 10.1002/imt2.23
121. Shigeishi H, Sugiyama M, Ohta K. Relationship between the prevalence of oral human papillomavirus DNA and periodontal disease (Review). BioMed Rep. (2021) 14:40. doi: 10.3892/br.2021.1416
122. Godoy-Vitorino F, Romaguera J, Zhao C, Vargas-Robles D, Ortiz-Morales G, Vázquez-Sánchez F, et al. Cervicovaginal fungi and bacteria associated with cervical intraepithelial neoplasia and high-risk human papillomavirus infections in a hispanic population. Front Microbiol. (2018) 9:2533. doi: 10.3389/fmicb.2018.02533
Keywords: oropharyngeal cancer, HPV, microbiome, crosstalk, carcinogenesis
Citation: Chung RS, Wong S, Lin D, Kokot NC, Sinha UK and Han AY (2024) Mechanisms of crosstalk between the oropharyngeal microbiome and human papillomavirus in oropharyngeal carcinogenesis: a mini review. Front. Oncol. 14:1425545. doi: 10.3389/fonc.2024.1425545
Received: 30 April 2024; Accepted: 24 June 2024;
Published: 15 August 2024.
Edited by:
Anastasios Maniakas, University of Texas MD Anderson Cancer Center, United StatesReviewed by:
Bhaskar Roy, Chinese Academy of Sciences, ChinaTheresa Guo, University of California, San Diego, United States
Copyright © 2024 Chung, Wong, Lin, Kokot, Sinha and Han. This is an open-access article distributed under the terms of the Creative Commons Attribution License (CC BY). The use, distribution or reproduction in other forums is permitted, provided the original author(s) and the copyright owner(s) are credited and that the original publication in this journal is cited, in accordance with accepted academic practice. No use, distribution or reproduction is permitted which does not comply with these terms.
*Correspondence: Albert Y. Han, albert.han@med.usc.edu