- 1Cancer Research Institute, Key Laboratory of Cancer Cellular and Molecular Pathology in Hunan, Hengyang Medical School, University of South China, Hengyang, Hunan, China
- 2Department of Gastroenterology, The First Affiliated Hospital of University of South China, Hengyang Medical School, University of South China, Hengyang, Hunan, China
Levels of the Wnt pathway components are abnormally altered in gastric cancer cells, leading to malignant cell proliferation, invasion and metastasis, poor prognosis and chemoresistance. Therefore, it is important to understand the mechanism of Wnt signaling pathway in gastric cancer. We systematically reviewed the molecular mechanisms of the Wnt pathway in gastric cancer development; and summarize the progression and the challenges of research on molecular agents of the Wnt pathway.
1 Introduction
Gastric cancer(GC) has the fifth highest incidence and fourth highest mortality rate in the worldwide (1). Activation of Wnt1 was first identified more than 40 years ago as causing breast hyperplasia and breast tumors, thus linking the Wnt pathway to cancer (2). Recent studies have identified frequent dysregulation of Wnt pathway activity in GC, and the phenomenon is associated with proliferation and metastasis, tumor microenvironment regulation, stemness maintenance, treatment resistance, and prognosis of gastric cancer (3–6). Inhibitors acting on different components of the Wnt pathway have been continuously developed, and some of them have shown good anticancer potential (7).
2 The Wnt signaling pathway
Wnt signaling pathways include the classical Wnt/β-catenin pathway, the non-classical Wnt/PCP pathway and the Wnt/Ca2+ pathway (Figure 1). Wnt ligands bind to the transmembrane receptor Frizzled (Fzd) to activate the Wnt pathway (8). The classical Wnt pathway is mainly involved in cell proliferation, while nonclassical Wnt pathways participate in cell polarity and migration (9).
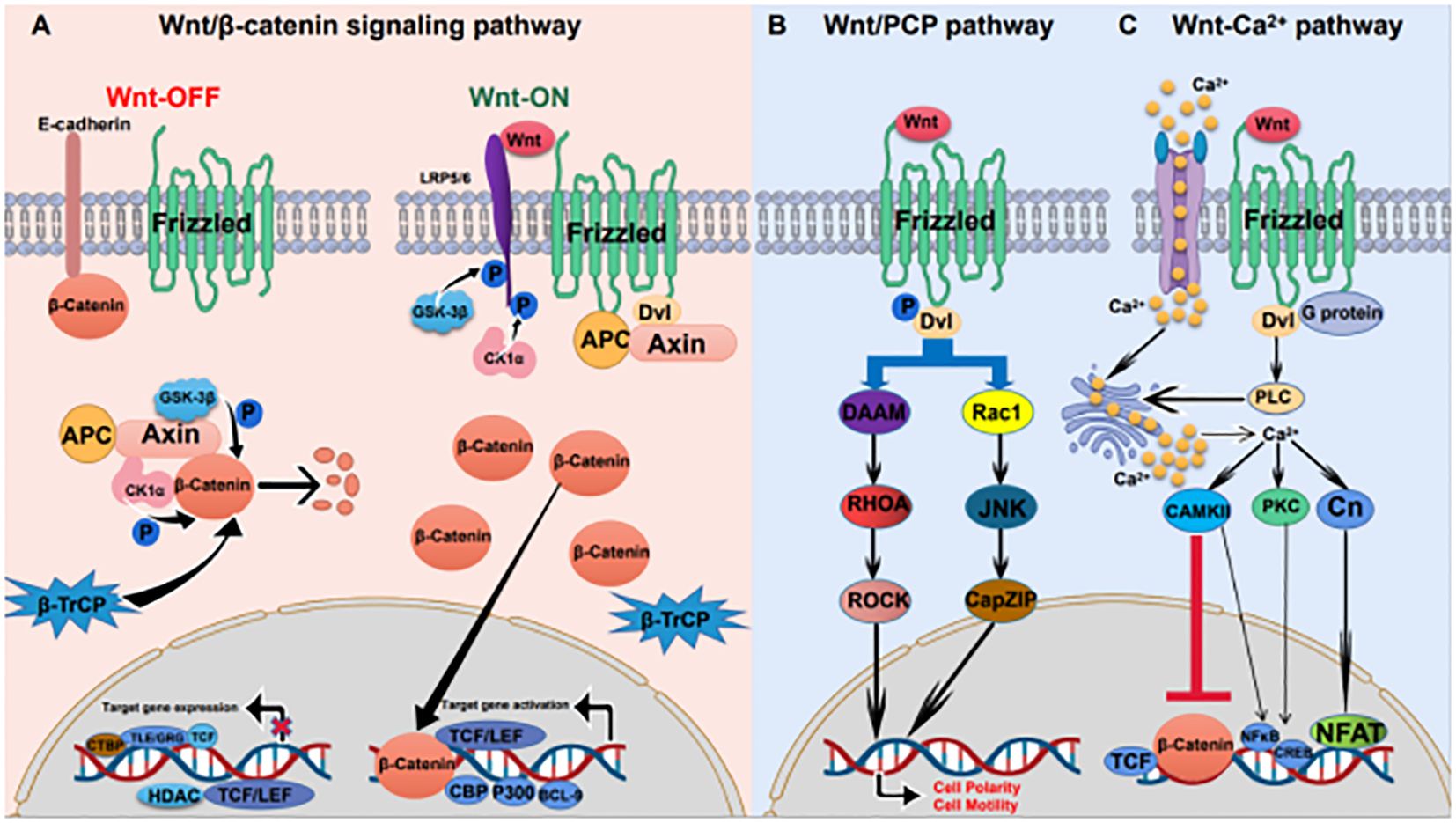
Figure 1 Wnt signaling pathway in gastric cancer (A) Wnt/β-catenin signaling pathway: in the absence of a Wnt ligand, β-catenin is degraded by the destruction complex; in the presence of a Wnt ligand, Wnt ligand binds to the receptor, and β-catenin separates from the destruction complex and is transported to the nucleus, resulting in the expression of target genes. (B) Wnt/PCP pathway: Wnt ligand binds to the Fzd receptor and phosphorylates Dvl. Phosphorylated Dvl activates RHOA and Rac1, causing downstream cascade reactions. (C) Wnt/Ca2+ pathway: Increased intracytoplasmic Ca2+ activates the protein phosphatase Cn, PKC, and CAMKII. CaMKII and PKC activate NF-κB and CREB. Cn activates nuclear factor of T cells (NFAT).
3 Altered Wnt signaling in GC Cells
Wnt ligand expression levels, β-catenin cytoplasmic and nuclear content, and intracellular cytoplasmic Ca2+ concentration were all altered. More than half of all gastric cancer patients have significant dysregulation of the Wnt/β-catenin pathway (5). The number of patients with gastric carcinogenesis with only nonclassical Wnt pathway activation is much higher than that of patients with only classical Wnt pathway activation (10).
3.1 Wnt ligand
Wnt ligands constitute of 19 members with functions that are largely dependent on posttranscriptional modifications, including glycosylation, palmitoylation and acylation. Among these modifications, acylation is necessary for extracellular transport of Wnt ligands and receptor/coreceptor recognition and binding (11), and Wnt ligands are essential for activating the Wnt pathway signaling cascade, making these ligands attractive therapeutic targets in gastric cancer. The vast majority of Wnt ligands show altered expression levels in gastric cancer cells, which affects the growth phenotype these cells and the efficacy of chemotherapy (11).
3.1.1 WNT1
In 2022, Zheng et al. found that high expression of WNT1 ligands in GC not only lead to malignant proliferation, invasion, and migration of gastric cancer cells but also accelerates the self-renewal and proliferation of GC stem cells (GCSCs) (12). LINC00665 activates WNT1 by binding to the WNT1 promoter, leading to M2 polarization of tumor-associated macrophages and promoting GC progression. It has been suggested that WNT1 is involved in gastric cancer progression and the induction of immune tolerance (13).
3.1.2 WNT2
Immunohistochemistry assays have confirmed that WNT2 was positively expressed only in gastric cancer tissues (14). The high expression of WNT2 was related to β- Catenin entering the nucleus. Collagen type X alpha 1 (COL10A1) activates the WNT2-mediated Wnt pathway and promotes the development of gastric cancer (15), and the vast majority of patients with high expression of WNT2 have cancer in TNM Stage III or IV with lymph node metastasis, it may be a marker for patients with advanced GC (14). WNT2B is a paralog of WNT2 (16). In 2022, Zhang et al. found that WNT2B is an oncogene in GC and upregulated in both GC tissues and GC cell lines (17). The mRNA of WNT2B carries a binding site for microRNA- 376c-3p and that overexpression of microRNA-376c-3p reduces the expression of WNT2B, inhibiting the growth and promoting the apoptosis of gastric cancer cells (17).
3.1.3 WNT3
An immunohistochemical positive assay indicated that the expression of WNT3 reached 87.50% of the cells in the poorly differentiated carcinoma group, which was higher than that in the precancerous lesion group (61.11%) and the chronic superficial gastritis group (20%) (18). Interference with WNT3 expression suppresses the malignant phenotype and promotes apoptosis in gastric cancer cells (19). WNT3A is a homolog of WNT3, with an amino acid homology as high as 84.2% (20). As a procarcinogenic gene in gastric cancer tissues, WNT3A expression is nearly twice that in adjacent noncancerous tissues (21), not only promoting malignant proliferation of gastric cancer cells and inhibiting apoptosis but is also patient chemoresistance and gastric cancer neuroinvasion (21, 22). It promotes gastric cancer cell proliferation by activating the downstream target high mobility Group A type 1 protein (HMGA1) (23). High expression of WNT3A is closely associated with neural infiltration in gastric cancer patients and may be caused by activation of matrix metalloproteinases (MMPs) downstream of the Wnt/β-catenin pathway (21). Therefore, WNT3A may be a potential prognostic indicator, although a large amount of clinical data are needed to validate its usefulness as a biomarker.
3.1.4 WNT4
Using a database for gene expression analysis of gastric cancer, in 2013, Volkomorov et al. (24) found that one-half of gastric cancer patients presented with a greater than twofold reduction in WNT4 expression compared to the level in normal gastric epithelial tissues. The main active metabolite, indole-3-methanol, 3,3′-diindolylmethane (DIM), prevents and treats cancers by inhibiting cell proliferation and inducing apoptosis; however, low-dose DIM induced WNT4 secretion and promoted gastric cancer progression (25).
3.1.5 WNT5A
The immunohistochemical assay showed that the WNT5A expression was evident in approximately 77.6% of gastric cancer tissues, and WNT5A-positive gastric cancer patients showed a higher infiltration of late tumor cells and more lymph node metastases than patients who did not express WNT5A. These differences might be related to the epithelial-mesenchymal transformation(EMT) of gastric cancer cells induced by WNT5A. Notably, the five-year survival rate was lower for WNT5A-positive patients in WNT5A-negative patients (26). WNT5A activates the nonclassical Wnt/Ca2+ pathway by binding to the FZD2 and promotes the invasion and migration of gastric cancer cells (27). In 2019, Xu et al. (28) reported that inhibition of zinc-finger transcription factor 1 (ZEB1) reduced the level of WNT5A in GC, induced apoptosis and inhibited the proliferation and migration of GC cells. Current research on WNT5B is not very clear, although it can predict the sensitivity of the epigenetic regulator BET inhibitor iBET-151 to inhibit the growth of gastric cancer, especially when combined with paclitaxel (29). Therefore, it may be possible to predict the effect of chemotherapy in gastric cancer by WNT5B.
3.1.6 WNT7、WNT8
The expression of WNT7A in gastric cancer is controversial. One study showed that its expression was decreased in gastric cancer tissues and gastric cancer cell lines, and the immunohistochemistry positivity rate of gastric cancer tissues was only 51.3%, whereas the positivity rate of normal gastric mucosal tissues was as high as 86.7% (30), and its low expression often suggests that the prognosis of gastric cancer patients is poor; another study showed that WNT7A elevated expression, which promotes migration and invasion of gastric cancer cells. miRNA-127 can target the 3′-UTR region of WNT7A mRNA, reduce its expression, and inhibit the invasion and migration of gastric cancer cells (31). WNT7B shares 77.1% amino acid homology with WNT7A (32). Moreover, Serum levels of WNT7B are higher in gastric cancer patients with lymph node metastasis than in gastric cancer patients without lymph node metastasis (33), and the level of serum WNT7B was positively correlated with the progression and metastasis of GC. It has been suggested that the effect of treatment can be observed and the prognosis of patients can be evaluated by measuring serum WNT7B levels.
The total amino acid homology of the WNT8A and WNT8B genes was 60.0%, and human WNT8A mRNA was expressed in NT2 cells with neural differentiation potential, whereas human WNT8B mRNA was expressed in diffuse gastric cancer (34).WNT8B was significantly up-regulated in gastric cancer cell lines.WNT8B may play a critical role in gastric cancer by activating the β-catenin-TCF signaling pathway (35).
3.1.7 WNT10
The expression of WNT10B was up-regulated. Down-regulation of Wnt10B expression using shRNA inhibited EMT in cancer cells. stem cell marker Oct4 was positively correlated with the expression level of WNT10B in gastric cancer tissues, and Wnt10B may also be involved in the generation and maintenance of gastric cancer stem cells (36).
3.1.8 WNT11, WNT16
WNT11, an important ligand in the nonclassical Wnt pathway, can promote tumorigenesis (37). In 2016, Mori et al. (38) found that hypoxia and hypoxia-inducible Factor 1α induced WNT11 expression, increased the activity levels of matrix metalloproteinase (MMP)-2 and 9, and promoted the proliferation, migration and invasion of gastric cancer cells.
As a Wnt family members, WNT16 can lead to gastric cancer chemoresistance through paracrine secretion, and the polyphenolic flavonoid compound quercetin (QC) can decrease the expression of WNT16. In 2018, Fang et al. (39) developed a hyaluronic acid (HA)-modified silica nanoparticle (HA-SiLN/QD) that reversed chemoresistance and remodeled tumor microenvironments by recognizing the overexpression of CD44 in gastric cancer cells and specifically targeting the delivery of quercetin and the gastric cancer chemotherapeutic agent adriamycin (DOX).
3.2 Wnt receptors and coreceptors
Aberrant expression of Wnt family transmembrane receptors and their coreceptors leads to overactivation of the Wnt pathway and affects gastric cancer development. In this section, we outline the roles of the Fzd receptor, coreceptors LRP and ROR1/2 and related molecules affecting the expression of these receptors and coreceptors in gastric cancer.
3.2.1 FZD
FZD proteins are seven-transmembrane coiled-coil receptors of which 10 (FZD1-FZD10) have been identified in humans. The cysteine-rich structural domain (CRD) of the extracellular N segment binds to Wnt ligands, and the intracellular C-terminus binds to Dvl to activate the downstream cascade reaction. FZD exerts both tumor-promoting and tumor-suppressing effects on gastric cancer cells. With the exceptions of FZD3 and FZD6, which are expressed at low levels in gastric cancer, all FZD isoforms are highly expressed in gastric cancer cells (40–46). FZD1 and FZD8 as oncogenes promote the proliferation, invasion, and migration of gastric cancer cells (40), and high expression of FZD8 suggests that a patients has a poor prognosis (41). FZD2 and FZD7 can be used as markers to assess the treatment and prognosis of gastric cancer patients and guide individualized treatment of patients with GC. The FZD2 level can be used to predict median overall survival (OS), the degree of immune cell infiltration, and the immune response of gastric cancer patients and to assess the effect of immunotherapy, with FZD7 expression suggesting a higher gastric cancer incidence and lymph node metastasis (42, 43). Moreover, knockdown of FZD7 decreased the expression of the stem cell genes Nanog and Oct-4, the multidrug resistance transporter protein gene ABCG2, and the tumor stem cell-associated surface antigens CD133, CD44, and CD24, indicating that FZD7 possibly involved in the regulation of gastric cancer stem cell function and drug resistance (46). In contrast to other isoforms, FZD5 and FZD6 play anti-oncogenic roles in gastric cancer. FZD5 maintains the epithelioid phenotype of gastric cancer cells, inhibits EMT of gastric cancer, and reduce its metastatic potential (44). FZD6 inhibits the proliferation and migration of gastric cancer cells (45).
Wnt pathway often engages in crosstalk with other pathways to affect the development of GC: it engages in crosstalk with the TGF-β pathway to inhibit the— apoptosis of gastric cancer cells. The transcription factor Smad family member 4 (SMAD4), a core factor in the TGF-β signaling pathway, increases the transcriptional activity of FZD4 and activates the Wnt pathway (47); FZD10 promotes the proliferation of gastric cancer cells. Moreover, the expression of FZD10 is increased in plasma exosomes, FZD10 can be used as a plasma biomarker (48).
3.2.2 LRP5/6, LGR5
LRP5/6 function as important coreceptors in the Wnt pathway. LRP5 is highly expressed; its high expression suggests late clinical stage and poor prognosis of patients with gastric cancer (49). In 2022, Zheng et al. (50) found that LRP6 interacted with capillary morphology genesis gene 2 (CMG2) to maintain the stemness of gastric cancer stem cells and accelerate gastric cancer progression.
The leucine-rich repeat sequence G protein-coupled receptor 5 (LGR5), a seven times transmembrane receptor. It’s a marker for gastric cancer stem cells, and its expression is higher in intestinal-type gastric cancer than in diffuse-type GC. In 2022, Nakazawa et al. (51) demonstrated that LGR5 expression in intestinal-type gastric cancer indicated poor overall survival (OS) (52).
3.3 β-Catenin
β-Catenin is a key component of the Wnt pathway, and immunohistochemistry assays that showed high β-Catenin levels in the group with poorly differentiated gastric adenocarcinomas was as high as 90.63%, which was much higher than that in the chronic superficial gastritis group and the precancerous group (18). In 2020, Ye et al. (53) found that the expression of myosin heavy chain 9 (MYH9) was increased in metastatic gastric cancers and was correlated with a poor prognosis; MYH9 provides gastric cancer cells with the ability to resist apoptosis and promote metastasis by inducing the transcription of CTNNB1.The expression of the destruction complex member APC is downregulated in GC, leading to lymph node metastasis and distant metastasis of GC (54). The role of RAS-related C3 botulinum toxin substrate 1 (Rac1) is mediation of β-catenin nuclear localization, and its activation promotes the proliferation, invasion, and drug resistance of gastric cancer cells (55).
Ca2+ is a key component of the nonclassical Wnt/Ca2+ pathway, and an elevated intracellular Ca2+ concentration can activate or inhibit β-catenin action through different pathways. Plasma membrane Na+/Ca2+ exchanger 1 (NCX1) and transient receptor potential canonical (TRPC1) play a key role in maintaining cytosolic free Ca2+ homeostasis. Elevation of Ca2+ levels induced by the coupling of these two proteins increases β-catenin phosphorylation (Ser675), and phosphorylated β-catenin enters the nucleus to activate downstream effector molecules and promote H. pylori-associated GC cell proliferation, migration and invasion (56). However, in 2016, Wan et al. (57) used nucleotide UTP to activate the P2Y6 receptor, and the elevation of cytoplasmic Ca2+ concentration in gastric cancer cells induced by store-operated calcium entry (SOCE) inhibited β-catenin, thus inhibiting the proliferation of gastric cancer cells. Moreover, this inhibitory effect did not alter the proliferation of normal gastric cells, making it a potential strategy for preventing or treating GC (58).
Wnt ligand, FZD receptor, and β-catenin are core components of the Wnt pathway, and their expression is altered to varying degrees in gastric cancer and affects the progression and prognosis of gastric cancer patients. The development of specific drugs targeting all three of them may achieve unexpected efficacy in the treatment of gastric cancer patients.
4 Wnt signaling in the microenvironment of gastric cancer
For the last few years, the emergence of immune checkpoint inhibitors (ICIs) has brought new hope to gastric cancer patients. Understanding the mechanism of tumor microenvironment (TME)-induced immune tolerance in gastric cancer will help to overcome the therapeutic inefficacy for patients with advanced gastric cancer. Gastric cancer cells establish a complex TME, in which immune cells, the extracellular matrix (ECM), and cell-secreted cytokines are important components (59). The Wnt/β-catenin pathway can inhibit T-cell infiltration and reduce the sensitivity of gastric cancer cells to anti-PD-1 antibodies (60). In 2020, CCL28, a mucosal-associated epithelial chemokine, is the key factor in the activation of β-catenin/TCF directly targeted genes, which can increase the infiltration of regulatory T (Treg) cells and lead to immunosuppression in the gastric cancer microenvironment (61). During the progression of gastric cancer, increased ECM deposition disrupts the interaction between E-cadherin and β-catenin and promotes gastric cancer cell proliferation, invasion and metastasis (62). WNT ligands (e.g., WNT2, WNT5A) from tumor cells and cancer-associated fibroblasts (CAFs) in the TME activate the Wnt pathway through the proliferation of CSCs and resurrection of dormant CSCs, causing treatment resistance and cancer recurrence (63). In 2020, Maeda et al. (64) found that H3K27me3 deletion in CAFs promoted the secretion of WNT5A, which may have enhanced the invasiveness of GCSCs. In 2016, Tumor-associated macrophages (TAMs) in TMEs secrete the proinflammatory factor TNF-α to activate the Wnt pathway and thus promote gastric cancer development (57). In 2016, Bone marrow-derived mesenchymal stem cells (BM-MSCs), important components of the TME, have been shown to promote the proliferation of gastric cancer cells through Wnt5a-Ror2 pathway (65). The evidence presented suggests that the Wnt pathway is related to immunosuppression in GC, and combining Wnt inhibitors and immunotherapy may lead to increased therapeutic effects (3).
5 Wnt signaling regulates stemness and drug resistance in gastric cancer cells
GCSCs, key players in gastric cancer with self-renewal and tumorigenic potential, are involved in both chemoresistance and cancer metastasis and gastric cancer recurrence. WNT1 is involved in the maintenance and proliferation of GCSCs through the classical Wnt pathway (66), and WNT5A is involved in the initiation of the EMT and gastric cancer stem cell proliferation through the nonclassical Wnt pathway (67, 68). Overexpression of human epidermal growth factor receptor-2 (HER2) in GC activates Wnt/β-catenin pathway in GCSCs, leading to enhanced invasiveness and treatment resistance of gastric cancer cells (69). In 2021, Wen et al.found that The retinoic acid-associated orphan receptor β (RORβ) reduces the activity of the Wnt/β-catenin pathway in GCSCs to inhibit their tumor-forming ability (70). The homology cassette transcription factor NANOG is essential for embryonic stem cell renewal, and the related pseudogene NANOGP8 is a major contributor to NANOG-induced effects on gastric cancer. NANOGP8 has been associated with almost all malignant phenotypes of GC: it has been associated with the upregulation of GCSC markers and EMT-related genes, accumulation of β-catenin in the nucleus, enhancement of Wnt signaling, and increased gastric cancer cell drug resistance; NANOGP8 is a potential therapeutic target for gastric cancer (71).
Aberrant activation of glutathione peroxidase 4 (GPX4), a key antioxidant enzyme, confers resistance to radiation and chemotherapy-induced iron death to cancer cells (72). The β-catenin/TCF4 transcriptional complex in the Wnt pathway directly binds to and induces the expression of GPX4, which attenuates the production of cytosolic lipid ROS, leading to iron death resistance (29). H. pylori infection upregulates GPX4 expression and activity through TCF4, leading to a high antioxidant state of cancer cells and inhibition of iron-induced death; thus, GPX4 may be a potential target to enhance chemosensitivity in patients with advanced GC (29).
Both multidrug resistance (MDR)-related proteins and Wnt/β-catenin pathway markers were upregulated in gastric cancer drug-resistant cells, overexpression of the novel tumor suppressor basic leucine zipper ATF-like transcription factor 2 (BATF2) inhibited the Wnt/β-catenin pathway to improve drug sensitivity and attenuate gastric cancer drug resistance (73). TRIM14 is a member of the triple structural domain protein (TRIM) family and plays an E3 ubiquitinating enzyme role. In 2023, Chen et al. (74) demonstrated in vitro and in vivo that circ_0091741 increased the expression of TRIM14 by blocking the binding of miR-330–3p to TRIM14, which activated Wnt/β-catenin by stabilizing the Dvl2 pathway to promote chemoresistance in gastric cancer cells.
The above evidence suggests that targeting the Wnt pathway may be one of the breakthroughs in alleviating drug resistance in gastric cancer patients.
6 Wnt signaling regulates EMT and metastasis in gastric cancer
The EMT is a reversible biological process that plays a crucial role in cancer cell metastasis. Ligands such as Wnt2, WNT5A, and WNT10B are involved in the EMT in gastric cancer (36, 75, 76). Chaperonin-containing T-complex protein 1ϵ subunit (CCT5) binds to E-cadherin and abrogates the interaction between E-cadherin and β-catenin, releasing β-catenin into the nucleus, leading to the EMT in gastric cancer cells and facilitating gastric cancer progression and lymphatic metastasis (77). Hsp90ab1, an isoform of heat shock protein 90, inhibits ubiquitin-mediated degradation of LRP5, leading to the upregulation of multiple targets of Wnt/β-catenin, activation of the Wnt pathway, and promotion of the EMT in gastric cancer cells (78). High mobility histone A2 (HMGA2) promotes the EMT, exacerbating GC metastasis through the Wnt/β-catenin pathway (79). Procalcitonin GA9 (PCDHGA9) may be a potential novel biomarker in GC and is closely related to the prognosis of GC patients. PCDHGA9 directly interacts with β-catenin and prevents the dissociation of β-catenin, thereby antagonizing the Wnt/β-catenin pathway and inhibiting EMT, which in turn inhibits invasive metastasis (9).The above evidence proves that it is feasible to delay the disease progression and improve the survival of gastric cancer patients by targeting the Wnt pathway.
7 Molecular agents targeting the Wnt pathway
In recent years, numerous inhibitors against the Wnt pathway have been developed, including small-molecule inhibitors (SMIs), monoclonal antibodies (mAbs), and peptide mimics, which can target Wnt ligands/receptors, β-catenin, and downstream target genes, respectively.
7.1 Targeting Wnt ligands/receptors
Hanaki et al. (80) showed that the anti-WNT5A polyclonal antibody pAb5a-5 prevented metastasis of gastric cancer cells by targeting WNT5A to inhibit the activation of Rac1 and the expression of laminin γ2. Dickkopf proteins (DKKs), which are endogenous Wnt ligand antagonists, showed hypermethylation and loss of inhibitory effects on Wnt proteins in gastric cancer. In 2021, Yang et al. (81) developed a chimeric 5/35 adenovirus-delivered Dickkopf-1 (Ad5/35-DKK1), which introduced DKK1 into CD44+ gastric cancer cells to promote the infection of GCSCs with the virus vector and the reduction in GCSC tumorigenicity. Porcupine (PORCN), for example, is a key molecule in inducing Wnt ligand secretion, and its inhibitors show great anticancer potential. The porcupine inhibitors (PORCNis) CGX1321 (NCT: 03507998), CGX1321 (with pembrolizumab) (NCT. 03507998) are all currently being evaluated in gastric cancer clinical trials to determine the maximum tolerated dose in patients with advanced gastrointestinal tumors (82). IWP (inhibitor of Wnt production) is a PORCNi that inhibits the growth of gastric cancer cells by downregulating the Wnt/β-catenin pathway (83). R-Spondin (RSPO) scavenges membrane Znrf3/Rnf43 and promotes activation of the Wnt pathway. The monoclonal antibody OMP131-R10 (rosmantuzumab) exhibits excellent safety and efficacy in patients with advanced solid tumor cancers (84). Hence, RSPO may be a potential therapeutic target. A recent study showed that PORCNi and anti-RSPO antibodies showed great potential as therapeutic targets for gastric cancer (85). Foxy-5, a peptide mimic of WNT5A, increases the expression level of WNT5A and prevents tumor metastasis in the breast and prostate cancer contexts (38).
MicroRNAs (miRNAs), as noncoding RNAs, can inhibit gene expression by binding to complementary mRNAs; thus, microRNAs are effective inhibitors. miRNAs targeting Wnt ligands are constantly being discovered. miR-132–3p and miR-140–5p can reduce the expression of WNT1 and inhibit gastric cancer cell proliferation and invasion (86, 87); miR-491–5p can target and silence Wnt3a, inhibit the proliferation of gastric cancer cells and induce apoptosis (21). miR-876–5p and miR-26a-5p can reduce the expression of WNT5A, inhibit the proliferation and migration of gastric cancer cells and induce apoptosis (15).
FZD proteins, as receptors of Wnt ligands, are essential for the activation of the Wnt pathway. Therefore, researchers have inhibited the activated Wnt pathway in gastric cancer by FZD inhibitors. Fz7–21, an inhibitor of FZD7, inhibits the growth of gastric adenomas and is currently being evaluated in preclinical trials for patients with gastrointestinal tumors (88). In addition, knockdown of FZD7 or treatment with vantictumab inhibited the growth of gastric adenomas. Vantictumab has been used in phase Ib clinical trials for advanced pancreatic, lung and breast cancers, a finding that demonstrates that it will be effective in treating patients with gastric cancer (89). The recombinant fusion protein ipafricept (OMP-54F28) mainly inhibits the binding of FZD8 to the Wnt ligand and has been evaluated for its ability to inhibit tumor growth in Phase I clinical trials for patients with advanced solid tumors (90). The monoclonal antibody OMP-18R5 (Vantictumab) (NCT:01345201) targets the FZD receptor and reduces the clone-forming ability of gastric cancer cells (88). Salinomycin, an inhibitor of LRP5/6, inhibits the growth of GC by inhibiting Wnt signaling in CSCs, and salinomycin targeting Wnt/β-catenin pathway may have important clinical therapeutic value in gastric cancer (69). As an FDA-approved drug with relatively low toxicity, clonidine can simultaneously target multiple Wnt pathway targets, namely FZD1, Dvl2, and LRP6, and it has demonstrated greater inhibition of colorectal and breast cancers; studies with clonidine have also provided insights for research on the application of Wnt inhibitors in the treatment of gastric cancer (9).
7.2 Targeting beta-catenin and the beta-catenin/TCF transcription complex
M435–1279 is a novel ubiquitin-conjugating enzyme E2T (UBE2T) inhibitor that inhibits UBE2T-induced β-catenin accumulation in the nucleus, blocks the overactivation of the Wnt signaling pathway and effectively inhibits GC progression. More importantly, M435–1279 induces low cytotoxicity in normal gastric mucosal cells at a concentration that is effective against gastric cancer cells. Although the mechanism underlying M435–1279 action is clear, the results of clinical trials have not validated its effects (91). β-Catenin responsive transcription inhibitor 3/5 (iCRT3/5), which mainly blocks β-catenin-TCF4 interactions, is in the preclinical stage of gastric cancer treatment, and it can kill gastric cancer malignant cells and inhibit gastric cancer development (88). LF3 is a 4-thioureido-benzenesulfonamide derivative that profoundly inhibits the interaction between β-catenin and TCF4 and reduces the expression of GPX4, inducing iron-induced death in gastric cancer cells (29). In 2018, Wang et al. Found that XAV939, a small-molecule inhibitor (SMI), increases degradation of β-catenin by stabilizing Axin and inhibits gastric cancer invasion and metastasis (92).
7.3 Targeting β-catenin downstream target genes
In 2020, Chang et al. found that 2,4-Diaminoquinazoline (2,4-DAQ), a selective inhibitor of LEF1, inhibits the expression of AXIN2, MYC and LGR5 and suppresses the proliferation, migration and invasion of gastric cancer cells (93). The oral Compound E7386 selectively inhibited the interaction of β-catenin with the β-catenin transcriptional coactivator CREB-binding protein (CBP) and exerted high antitumor activity in a human gastric cancer xenograft tumor model (94).
In 2016, Feng et al. found that Pantoprazole, a proton pump inhibitor, inhibited the proliferation of gastric cancer cells and increased the therapeutic sensitivity of GC to 5-FU by inhibiting the EMT/β-catenin pathway (95). Ibuprofen, a commonly used antipyretic and analgesic, inhibited the Wnt/β-catenin pathway in gastric cancer stem cells to reduce their proliferation (96). Fiber-modified hexose chimeric recombinant lysosomal adenovirus targeting CAFs in TAMs specifically killed CAFs and inhibited the growth of gastric cancer cells (97). In summary, various molecular agents target the Wnt pathway, and monoclonal antibodies have received much attention from researchers because of their high specificity, which is in line with a precision therapy strategy (98).
Many molecular agents have been developed to target the Wnt pathway, but none of the FDA-approved drugs have been used in the clinical treatment of GC to date; the primary reason for this is the side-effects they produce, as they are, mainly toxic to bone. PORCN inhibitors cause loss of bone density and volume (99), and OMPs targeting FZD cause even more severe bone damage, which was the main reason that clinical trials to evaluate OMPs were discontinued. Monoclonal antibodies targeting FZD cause abdominal pain, diarrhea, and constipation (100), limiting their clinical applications. Second, the molecular structures of SMIs, mAbs and peptide mimics can mitigate the therapeutic effect. SMI show higher permeability and are suitable for oral administration, but they show low targeting specificity. mAbs have large molecular weights and a long half-life; however, they burden a patient’s liver and kidneys and are poorly tolerated. Although the emergence of peptide mimics solved the problems of permeability and tolerance of SMIs and mABs, due to their short half-life, larger doses are required to maintain therapeutic concentrations (38). In 2023, isoproterenol (2,6-diisopropylphenol), a commonly used intravenous anesthetic in clinical practice, significantly reduced the likelihood of cancer recurrence when used as an anesthetic for breast cancer surgery. Kaishuai Zhan et al. demonstrated that it can activate the Wnt/β-catenin pathway and inhibit the proliferation of gastric cancer cells (101).
Due to the complexity of the Wnt pathway, it is difficult to find targets that can be reached with high specificity and efficacy. The nonclassical Wnt pathway has been increasingly studied, but there are few drugs targeting the nonclassical Wnt pathway. Therefore, development of drugs targeting the nonclassical Wnt pathway is an emerging research direction.
8 Discussion
Abnormal activation of Wnt signaling plays important roles in the occurrence, development and chemotherapy resistance of gastric cancer. Targeting the Wnt pathway is a great strategy for the treatment of GC (Figure 2). An increasing number of small molecules and biological agents targeting the Wnt pathway have been entered into clinical trials for patients with GC. In view of the unique role of the non-classical Wnt pathway in gastric cancer, more specific inhibitors can be developed. Whether Wnt pathway inhibitors can be used in the clinical treatment of GC in the future depends on how the problems with targeting, efficacy and patient tolerance by Wnt pathway inhibitors are solved.
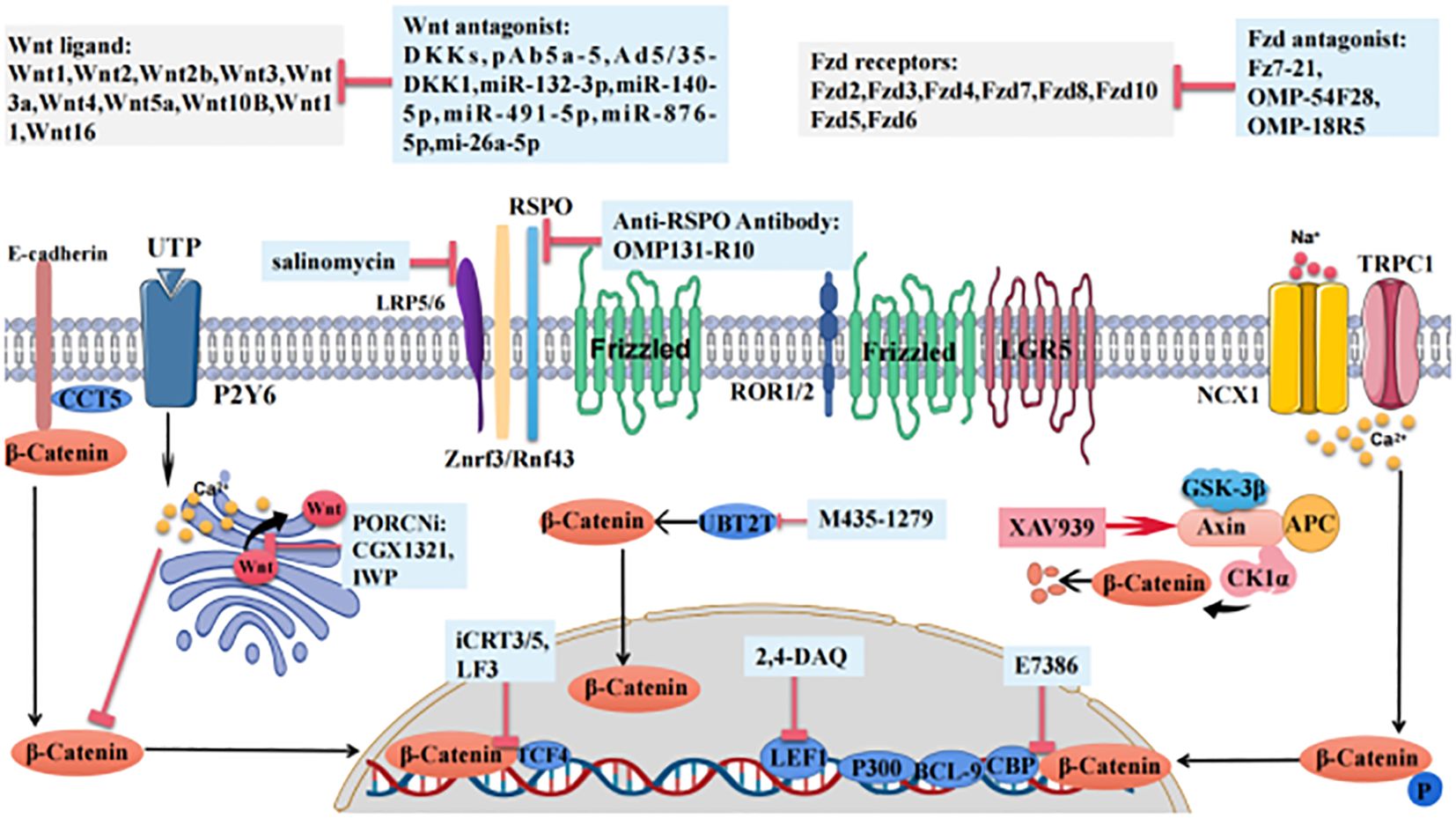
Figure 2 The roles of Wnt pathway members and Wnt-targeted agents in gastric cancer. Wnt ligands and FZD receptors are altered in gastric cancer. The background colors of the following various Wnt-targeted agents are shown in light blue boxes. DKKs and PORCNi are Wnt ligand antagonists, pAb5a-5 targets and inhibits WNT5A, Ad5/35-DKK1 delivers DKK1, miR-132–3p and miR-140–5p inhibit WNT1, miR-491–5p inhibits Wnt3a, and miR-876–5p and mi-26a-5p inhibit WNT5A. OMP-18R5 targets and inhibits the FZD receptor, Fz7–21 inhibits FZD7. OMP-54F28 inhibits FZD8. CCT5 inhibits β-catenin adhesion to E-cadherin, salinomycin inhibits LRP5/6, and OMP131-R10 inhibits the Znrf3-/Rnf43-scavenging effect of RSPO. M435–1279 inhibits UBT2T-induces β-catenin accumulation in the nucleus. XAV939 promotes β-catenin degradation. iCRT3/5 and LF3 inhibit the interaction of β-catenin and TCF4. 2,4-DAQ inhibits LEF1, and E7386 inhibits the interaction between β-catenin and CBP.
Author contributions
RH: Writing – original draft. JY: Writing – review & editing. YZ: Supervision, Writing – review & editing. RG: Supervision, Writing – review & editing.
Funding
The author(s) declare financial support was received for the research, authorship, and/or publication of this article. This work was supported by the Natural Science Foundation of Hunan Province (No. 2021JJ30590, 2021JJ40502, 2021JJ30611), the Project of Hunan Provincial Health Commission (No. 202103030223 and 202307019501), and the Scientific Research Fund Project of Hunan Provincial Health Commission (No. 20201921).
Conflict of interest
The authors declare that the research was conducted in the absence of any commercial or financial relationships that could be construed as a potential conflict of interest.
Publisher’s note
All claims expressed in this article are solely those of the authors and do not necessarily represent those of their affiliated organizations, or those of the publisher, the editors and the reviewers. Any product that may be evaluated in this article, or claim that may be made by its manufacturer, is not guaranteed or endorsed by the publisher.
References
1. Sung H, Ferlay J, Siegel RL, Laversanne M, Soerjomataram I, Jemal A, et al. Global cancer statistics 2020: GLOBOCAN estimates of incidence and mortality worldwide for 36 cancers in 185 countries. CA Cancer J Clin. (2021) 71:209–49. doi: 10.3322/caac.21660
2. Nüsslein-Volhard C, Wieschaus E. Mutations affecting segment number and polarity in Drosophila. Nature. (1980) 287:795–801. doi: 10.1038/287795a0
3. Lei Z-N, Teng Q-X, Tian Q, Chen W, Xie Y, Wu K, et al. Signaling pathways and therapeutic interventions in gastric cancer. Signal Transduction Targeted Ther. (2022) 7:358. doi: 10.1038/s41392-022-01190-w
4. Wang L, Wang H, Duan X, Dai P, Li J. Comprehensive analysis of the canonical and non-canonical wnt signaling pathways in gastric cancer. Dig Dis Sci. (2019) 64:2830–42. doi: 10.1007/s10620-019-05606-6
5. Wang Y, Zheng L, Shang W, Yang Z, Li T, Liu F, et al. Wnt/beta-catenin signaling confers ferroptosis resistance by targeting GPX4 in gastric cancer. Cell Death Differ. (2022) 29:2190–202. doi: 10.1038/s41418-022-01008-w
6. Koushyar S, Powell AG, Vincan E, Phesse TJ. Targeting wnt signaling for the treatment of gastric cancer. Int J Mol Sci. (2020) 21(11):3927. doi: 10.3390/ijms21113927
7. Lu B, Green BA, Farr JM, Lopes FCM, Van Raay TJ. Wnt drug discovery: weaving through the screens, patents and clinical trials. Cancers. (2016) 8(9):82. doi: 10.3390/cancers8090082
8. Sun Y, Wang W, Zhao C. Frizzled receptors in tumors, focusing on signaling, roles, modulation mechanisms, and targeted therapies. Oncol Res. (2021) 28:661–74. doi: 10.3727/096504020X16014648664459
9. Liu J, Xiao Q, Xiao J, Niu C, Li Y, Zhang X, et al. Wnt/β-catenin signalling: function, biological mechanisms, and therapeutic opportunities. Signal Transduct Target Ther. (2022) 7:3. doi: 10.1038/s41392-021-00762-6
10. Chen Y, Chen Z, Tang Y, Xiao Q. The involvement of noncanonical Wnt signaling in cancers. Biomedicine Pharmacotherapy. (2021) 133:110946. doi: 10.1016/j.biopha.2020.110946
11. Torres VI, Godoy JA, Inestrosa NC. Modulating Wnt signaling at the root: Porcupine and Wnt acylation. Pharmacol Ther. (2019) 198:34–45. doi: 10.1016/j.pharmthera.2019.02.009
12. Zheng X, Peng B, Wu X, Ye J, Zhao H, Li Y, et al. Male-specific long non-coding RNA testis-specific transcript, Y-linked 1 5 promotes gastric cancer cell growth by regulating Wnt family member 1/β-catenin signaling by sponging microRNA let-7a-5p. Bioengineered. (2022) 13:8605–16. doi: 10.1080/21655979.2022.2053814
13. Yang B, Su K, Sha G, Bai Q, Sun G, Chen H, et al. LINC00665 interacts with BACH1 to activate Wnt1 and mediates the M2 polarization of tumor-associated macrophages in GC. Mol Immunol. (2022) 146:1–8. doi: 10.1016/j.molimm.2022.03.120
14. Zhang Z, Wang J, Dong X. Wnt2 contributes to the progression of gastric cancer by promoting cell migration and invasion. Oncol Lett. (2018) 16:2857–64. doi: 10.3892/ol
15. Zhang M, Jin M, Gao Z, Yu W, Zhang W. High COL10A1 expression potentially contributes to poor outcomes in gastric cancer with the help of LEF1 and Wnt2. J Clin Lab Anal. (2022) 36:e24612. doi: 10.1002/jcla.24612
16. Katoh M. WNT2 and human gastrointestinal cancer (review). Int J Mol Med. (2003) 12:811–6. doi: 10.3892/ijmm
17. Zhang L, Liu F, Meng Z, Luo Q, Pan D, Qian Y. Inhibited HDAC3 promotes microRNA-376c-3p to suppress Malignant phenotypes of gastric cancer cells by reducing WNT2b. Genomics. (2021) 113:3512–22. doi: 10.1016/j.ygeno.2021.07.018
18. Zhou Z, Ye G, Peng J, He B, Xu S, Fan W, et al. Expression of Wnt3, β-catenin and MMP-7 in gastric cancer and precancerous lesions and their correlations with Helicobacter pylori infection. Zhong Nan Da Xue Bao Yi Xue Ban. (2021) 46:575–82. doi: 10.11817/j.issn.1672-7347.2021.200733
19. Wang HS, Nie X, Wu RB, Yuan HW, Ma YH, Liu XL, et al. Downregulation of human Wnt3 in gastric cancer suppresses cell proliferation and induces apoptosis. Onco Targets Ther. (2016) 9:3849–60. doi: 10.2147/OTT
20. Yu F, Yu C, Li F, Zuo Y, Wang Y, Yao L, et al. Wnt/β-catenin signaling in cancers and targeted therapies. Signal Transduction Targeted Ther. (2021) 6:307. doi: 10.1038/s41392-021-00701-5
21. Sun R, Liu Z, Tong D, Yang Y, Guo B, Wang X, et al. miR-491–5p, mediated by Foxi1, functions as a tumor suppressor by targeting Wnt3a/β-catenin signaling in the development of gastric cancer. Cell Death Dis. (2017) 8:e2714. doi: 10.1038/cddis.2017.134
22. Yan C, Song X, Wang S, Wang J, Li L. Knockdown of PDIA6 inhibits cell proliferation and enhances the chemosensitivity in gastric cancer cells. Cancer Manag Res. (2020) 12:11051–62. doi: 10.2147/CMAR.S267711
23. Akaboshi S, Watanabe S, Hino Y, Sekita Y, Xi Y, Araki K, et al. HMGA1 is induced by Wnt/beta-catenin pathway and maintains cell proliferation in gastric cancer. Am J Pathol. (2009) 175:1675–85. doi: 10.2353/ajpath.2009.090069
24. Volkomorov V, Grigoryeva E, Krasnov G, Litviakov N, Tsyganov M, Karbyshev M, et al. Search for potential gastric cancer markers using miRNA databases and gene expression analysis. Exp Oncol. (2013) 35:2–7.
25. Zhu Y, Zhang B, Gong A, Fu H, Zhang X, Shi H, et al. Anti-cancer drug 3,3’-diindolylmethane activates Wnt4 signaling to enhance gastric cancer cell stemness and tumorigenesis. Oncotarget. (2016) 7:16311–24. doi: 10.18632/oncotarget.v7i13
26. Nam S, Chung JW, Yang JY. WNT5A correlates with clinicopathological characteristics in gastric cancer: a meta-analysis. Cell Physiol Biochem. (2017) 41:33–40. doi: 10.1159/000455934
27. Kurayoshi M, Oue N, Yamamoto H, Kishida M, Inoue A, Asahara T, et al. Expression of Wnt-5a is correlated with aggressiveness of gastric cancer by stimulating cell migration and invasion. Cancer Res. (2006) 66:10439–48. doi: 10.1158/0008-5472.CAN-06-2359
28. Xue Y, Zhang L, Zhu Y, Ke X, Wang Q, Min H. Regulation of proliferation and epithelial-to-mesenchymal transition (EMT) of gastric cancer by ZEB1 via modulating wnt5a and related mechanisms. Med Sci Monit. (2019) 25:1663–70. doi: 10.12659/MSM.912338
29. Kang SK, Bae HJ, Kwon WS, Kim TS, Kim KH, Park S, et al. Inhibition of the bromodomain and extra-terminal family of epigenetic regulators as a promising therapeutic approach for gastric cancer. Cell Oncol (Dordr). (2021) 44:1387–403. doi: 10.1007/s13402-021-00647-4
30. Liu Y, Qiao Y, Zhang H, Li W, Zheng J. Wnt7a, frequently silenced by CpG methylation, inhibits tumor growth and metastasis via suppressing epithelial-mesenchymal transition in gastric cancer. J Cell Biochem. (2019) 120:18142–51. doi: 10.1002/jcb.29118
31. Wang L, Wang X, Jiang X. miR-127 suppresses gastric cancer cell migration and invasion via targeting Wnt7a. Oncol Lett. (2019) 17:3219–26. doi: 10.3892/ol
32. Kirikoshi H, Sekihara H, Katoh M. Molecular cloning and characterization of human WNT7B. Int J Oncol. (2001) 19:779–83. doi: 10.3892/ijo
33. Gao Q, Yang L, Shen A, Li Y, Li Y, Hu S, et al. A WNT7B-m(6)A-TCF7L2 positive feedback loop promotes gastric cancer progression and metastasis. Signal Transduct Target Ther. (2021) 6:43. doi: 10.1038/s41392-020-00397-z
34. The Cancer Genome Atlas Research Network. Comprehensive molecular characterization of gastric adenocarcinoma. Nature. (2014) 513(7517):202–9. doi: 10.1038/nature13480
35. Saitoh T, Mine T, Katoh M. Up-regulation of WNT8B mRNA in human gastric cancer. Int J Oncol. (2002) 20:343–8. doi: 10.3892/ijo
36. Wu XD, Bie QL, Zhang B, Yan ZH, Han ZJ. Wnt10B is critical for the progression of gastric cancer. Oncol Lett. (2017) 13:4231–7. doi: 10.3892/ol.2017.5992
37. Rodriguez-Hernandez I, Maiques O, Kohlhammer L, Cantelli G, Perdrix-Rosell A, Monger J, et al. WNT11-FZD7-DAAM1 signalling supports tumour initiating abilities and melanoma amoeboid invasion. Nat Commun. (2020) 11:5315. doi: 10.1038/s41467-020-18951-2
38. Mori H, Yao Y, Learman BS, Kurozumi K, Ishida J, Ramakrishnan SK, et al. Induction of WNT11 by hypoxia and hypoxia-inducible factor-1α regulates cell proliferation, migration and invasion. Sci Rep. (2016) 6:21520. doi: 10.1038/srep21520
39. Fang J, Zhang S, Xue X, Zhu X, Song S, Wang B, et al. Quercetin and doxorubicin co-delivery using mesoporous silica nanoparticles enhance the efficacy of gastric carcinoma chemotherapy. Int J Nanomedicine. (2018) 13:5113–26. doi: 10.2147/IJN
40. Sun JG, Li XB, Yin RH, Li XF. lncRNA VIM−AS1 promotes cell proliferation, metastasis and epithelial−mesenchymal transition by activating the Wnt/β−catenin pathway in gastric cancer. Mol Med Rep. (2020) 22:4567–78. doi: 10.3892/mmr
41. Chen W, Liu Z, Mai W, Xiao Y, You X, Qin L. FZD8 indicates a poor prognosis and promotes gastric cancer invasion and metastasis via B-catenin signaling pathway. Ann Clin Lab Sci. (2020) 50:13–23.
42. Dai S, Liu T, Liu XQ, Li XY, Xu K, Ren T, et al. Identification of an immune-related signature predicting survival risk and immune microenvironment in gastric cancer. Front Cell Dev Biol. (2021) 9:687473. doi: 10.3389/fcell.2021.687473
43. Freiin Grote A, Halske C, Behrens HM, Krüger S, Wilhelm F, Egberts JH, et al. Expression of LGR5, FZD7, TROY, and MIST1 in perioperatively treated gastric carcinomas and correlation with therapy response. Dis Markers 2019. (2019) p:8154926. doi: 10.1155/2019/8154926
44. Dong D, Na L, Zhou K, Wang Z, Sun Y, Zheng Q, et al. FZD5 prevents epithelial-mesenchymal transition in gastric cancer. Cell Commun Signal. (2021) 19:21. doi: 10.1186/s12964-021-00708-z
45. Yan J, Liu T, Zhou X, Dang Y, Yin C, Zhang G. FZD6, targeted by miR-21, represses gastric cancer cell proliferation and migration via activating non-canonical wnt pathway. Am J Transl Res. (2016) 8:2354–64.
46. Li G, Su Q, Liu H, Wang D, Zhang W, Lu Z, et al. Frizzled7 promotes epithelial-to-mesenchymal transition and stemness via activating canonical wnt/β-catenin pathway in gastric cancer. Int J Biol Sci. (2018) 14:280–93. doi: 10.7150/ijbs.23756
47. Du X, Li Q, Yang L, Liu L, Cao Q, Li Q. SMAD4 activates Wnt signaling pathway to inhibit granulosa cell apoptosis. Cell Death Dis. (2020) 11:373. doi: 10.1038/s41419-020-2578-x
48. Scavo MP, Rizzi F, Depalo N, Armentano R, Coletta S, Serino G, et al. Exosome released FZD10 increases ki-67 expression via phospho-ERK1/2 in colorectal and gastric cancer. Front Oncol. (2021) 11:730093. doi: 10.3389/fonc.2021.730093
49. Nie X, Wang H, Wei X, Li L, Xue T, Fan L, et al. LRP5 promotes gastric cancer via activating canonical wnt/β-catenin and glycolysis pathways. Am J Pathol. (2022) 192:503–17. doi: 10.1016/j.ajpath.2021.11.007
50. Zheng J, Li C, Zhu Z, Yang F, Wang X, Jiang P, et al. A 5`-tRNA derived fragment namedtiRNA-val-CAC-001 works as a suppressor in gastric cancer. Cancer Manag Res. (2022) 14:2323–37. doi: 10.2147/CMAR.S363629
51. Nakazawa N, Sohda M, Ide M, Shimoda Y, Tateno K, Watanabe T, et al. PROX1 was associated with LGR5 and wnt signaling and contributed to poor prognosis in gastric cancer. Oncology. (2022) 100:569–75. doi: 10.1159/000526734
52. Wang H, Lu Y, Wang M, Wu Y, Wang X, Li Y. Roles of E3 ubiquitin ligases in gastric cancer carcinogenesis and their effects on cisplatin resistance. J Mol Med (Berl). (2021) 99:193–212. doi: 10.1007/s00109-020-02015-5
53. Ye G, Yang Q, Lei X, Zhu X, Li F, He J, et al. Nuclear MYH9-induced CTNNB1 transcription, targeted by staurosporin, promotes gastric cancer cell anoikis resistance and metastasis. Theranostics. (2020) 10:7545–60. doi: 10.7150/thno.46001
54. Deng S, Zhang X, Qin Y, Chen W, Fan H, Feng X, et al. miRNA-192 and -215 activate Wnt/β-catenin signaling pathway in gastric cancer via APC. J Cell Physiol. (2020) 235:6218–29. doi: 10.1002/jcp.29550
56. Wan H, Gao N, Lu W, Lu C, Chen J, Wang Y, et al. NCX1 coupled with TRPC1 to promote gastric cancer via Ca(2+)/AKT/β-catenin pathway. Oncogene. (2022) 41:4169–82. doi: 10.1038/s41388-022-02412-9
57. Oishi S, Takano R, Tamura S, Tani S, Iwaizumi M, Hamaya Y, et al. M2 polarization of murine peritoneal macrophages induces regulatory cytokine production and suppresses T-cell proliferation. Immunology. (2016) 149:320–8. doi: 10.1111/imm.12647
58. Wan H, Xie R, Xu J, He J, Tang B, Liu Q, et al. Anti-proliferative effects of nucleotides on gastric cancer via a novel P2Y6/SOCE/ca(2+)/β-catenin pathway. Sci Rep. (2017) 7:2459. doi: 10.1038/s41598-017-02562-x
59. Cai WY, Dong ZN, Fu XT, Lin LY, Wang L, Ye GD, et al. Identification of a tumor microenvironment-relevant gene set-based prognostic signature and related therapy targets in gastric cancer. Theranostics. (2020) 10:8633–47. doi: 10.7150/thno.47938
60. Li J, Li J, Zhang H, Bei S, Zhang X, Li H, Ye L, et al. Disruption of wnt/β-catenin pathway elevates the sensitivity of gastric cancer cells to PD-1 antibody. Curr Mol Pharmacol. (2022) 15:557–69. doi: 10.2174/1874467214666210617163821
61. Ji L, Qian W, Gui L, Ji Z, Yin P, Lin GN, et al. Blockade of β-catenin-induced CCL28 suppresses gastric cancer progression via inhibition of treg cell infiltration. Cancer Res. (2020) 80:2004–16. doi: 10.1158/0008-5472.CAN-19-3074
62. Jang M, Koh I, Lee JE, Lim JY, Cheong JH, Kim P. Increased extracellular matrix density disrupts E-cadherin/β-catenin complex in gastric cancer cells. Biomater Sci. (2018) 6:2704–13. doi: 10.1039/C8BM00843D
63. Katoh M, Katoh M. WNT signaling and cancer stemness. Essays Biochem. (2022) 66:319–31. doi: 10.1042/EBC20220016
64. Maeda M, Takeshima H, Iida N, Hattori N, Yamashita S, Moro H, et al. Cancer cell niche factors secreted from cancer-associated fibroblast by loss of H3K27me3. Gut. (2020) 69:243–51. doi: 10.1136/gutjnl-2018-317645
65. Takiguchi G, Nishita M, Kurita K, Kakeji Y, Minami Y. Wnt5a-Ror2 signaling in mesenchymal stem cells promotes proliferation of gastric cancer cells by activating CXCL16-CXCR6 axis. Cancer Sci. (2016) 107:290–7. doi: 10.1111/cas.12871
66. Mao J, Fan S, Ma W, Fan P, Wang B, Zhang J, et al. Roles of Wnt/β-catenin signaling in the gastric cancer stem cells proliferation and salinomycin treatment. Cell Death Dis. (2014) 5:e1039. doi: 10.1038/cddis.2013.515
67. Song Y, Wang Y, Tong C, Xi H, Zhao X, Wang Y, et al. A unified model of the hierarchical and stochastic theories of gastric cancer. Br J Cancer. (2017) 116:973–89. doi: 10.1038/bjc.2017.54
68. Kanzawa M, Semba S, Hara S, Itoh T, Yokozaki H. WNT5A is a key regulator of the epithelial-mesenchymal transition and cancer stem cell properties in human gastric carcinoma cells. Pathobiology. (2013) 80:235–44. doi: 10.1159/000346843
69. Jung DH, Bae YJ, Kim JH, Shin YK, Jeung HC. HER2 regulates cancer stem cell activities via the wnt signaling pathway in gastric cancer cells. Oncology. (2019) 97:311–8. doi: 10.1159/000502845
70. Wen Z, Chen M, Guo W, Guo K, Du P, Fang Y, et al. RORβ suppresses the stemness of gastric cancer cells by downregulating the activity of the Wnt signaling pathway. Oncol Rep. (2021) 46(2):180. doi: 10.3892/or.2021.8131
71. Ma X, Wang B, Wang X, Luo Y, Fan W. NANOGP8 is the key regulator of stemness, EMT, Wnt pathway, chemoresistance, and other Malignant phenotypes in gastric cancer cells. PloS One. (2018) 13:e0192436. doi: 10.1371/journal.pone.0192436
72. Zhang H, Deng T, Liu R, Ning T, Yang H, Liu D, et al. CAF secreted miR-522 suppresses ferroptosis and promotes acquired chemo-resistance in gastric cancer. Mol Cancer. (2020) 19:43. doi: 10.1186/s12943-020-01168-8
73. Yang W, Wu B, Ma N, Wang Y, Guo J, Zhu J, et al. BATF2 reverses multidrug resistance of human gastric cancer cells by suppressing Wnt/β-catenin signaling. In Vitro Cell Dev Biol Anim. (2019) 55:445–52. doi: 10.1007/s11626-019-00360-5
74. Chen Y, Liu H, Zou J, Cao G, Li Y, Xing C, et al. Exosomal circ_0091741 promotes gastric cancer cell autophagy and chemoresistance via the miR-330–3p/TRIM14/Dvl2/Wnt/β-catenin axis. Hum Cell. (2023) 36:258–75. doi: 10.1007/s13577-022-00790-6
75. Kang SK, Bae HJ, Kwon WS, Che J, Kim TS, Chung HC, et al. Transcriptome analysis of iBET-151, a BET inhibitor alone and in combination with paclitaxel in gastric cancer cells. Genomics Inform. (2020) 18:e37. doi: 10.5808/GI.2020.18.4.e37
76. Tian S, Peng P, Li J, Deng H, Zhan N, Zeng Z, et al. SERPINH1 regulates EMT and gastric cancer metastasis via the Wnt/β-catenin signaling pathway. Aging (Albany NY). (2020) 12:3574–93. doi: 10.18632/aging.v12i4
77. Li Y, Liu C, Zhang X, Huang X, Liang S, Xing F, et al. CCT5 induces epithelial-mesenchymal transition to promote gastric cancer lymph node metastasis by activating the Wnt/β-catenin signalling pathway. Br J Cancer. (2022) 126:1684–94. doi: 10.1038/s41416-022-01747-0
78. Wang H, Deng G, Ai M, Xu Z, Mou T, Yu J, et al. Hsp90ab1 stabilizes LRP5 to promote epithelial-mesenchymal transition via activating of AKT and Wnt/β-catenin signaling pathways in gastric cancer progression. Oncogene. (2019) 38:1489–507. doi: 10.1038/s41388-018-0532-5
79. Dong J, Wang R, Ren G, Li X, Wang J, Sun Y, et al. HMGA2-FOXL2 axis regulates metastases and epithelial-to-mesenchymal transition of chemoresistant gastric cancer. Clin Cancer Res. (2017) 23:3461–73. doi: 10.1158/1078-0432.CCR-16-2180
80. Hanaki H, Yamamoto H, Sakane H, Matsumoto S, Ohdan H, Sato A, et al. An anti-Wnt5a antibody suppresses metastasis of gastric cancer cells in vivo by inhibiting receptor-mediated endocytosis. Mol Cancer Ther. (2012) 11:298–307. doi: 10.1158/1535-7163.MCT-11-0682
81. Yang Y, Meng WJ, Wang ZQ. Cancer stem cells and the tumor microenvironment in gastric cancer. Front Oncol. (2021) 11:803974.doi: 10.3389/fonc.2021.803974
82. Wang X, Wang X, Liu Y, Dong Y, Wang Y, Kassab MA, et al. LGR5 regulates gastric adenocarcinoma cell proliferation and invasion via activating Wnt signaling pathway. Oncogenesis. (2018) 7:57. doi: 10.1038/s41389-018-0071-5
83. Mo ML, Li MR, Chen Z, Liu XW, Sheng Q, Zhou HM. Inhibition of the Wnt palmitoyltransferase porcupine suppresses cell growth and downregulates the Wnt/β-catenin pathway in gastric cancer. Oncol Lett. (2013) 5:1719–23. doi: 10.3892/ol.2013.1256
84. Morgan RG, Mortensson E, Williams AC. Targeting LGR5 in Colorectal Cancer: therapeutic gold or too plastic? Br J Cancer. (2018) 118:1410–8. doi: 10.1038/s41416-018-0118-6
85. Ter Steege EJ, Bakker ERM. The role of R-spondin proteins in cancer biology. Oncogene. (2021) 40:6469–78. doi: 10.1038/s41388-021-02059-y
86. Liu B, Qiang L, Guan B, Ji Z. Targeting kinesin family member 21B by miR-132–3p represses cell proliferation, migration and invasion in gastric cancer. Bioengineered. (2022) 13:9006–18. doi: 10.1080/21655979.2022.2054755
87. Cha Y, He Y, Ouyang K, Xiong H, Li J, Yuan X. MicroRNA-140–5p suppresses cell proliferation and invasion in gastric cancer by targeting WNT1 in the WNT/β-catenin signaling pathway. Oncol Lett. (2018) 16:6369–76. doi: 10.3892/ol
88. Zhang Y, Wang X. Targeting the Wnt/β-catenin signaling pathway in cancer. J Hematol Oncol. (2020) 13:165. doi: 10.1186/s13045-020-00990-3
89. Flanagan DJ, Barker N, Costanzo NSD, Mason EA, Gurney A, Meniel VS, et al. Frizzled-7 is required for wnt signaling in gastric tumors with and without apc mutations. Cancer Res. (2019) 79:970–81. doi: 10.1158/0008-5472.CAN-18-2095
90. Jimeno A, Gordon M, Chugh R, Messersmith W, Mendelson D, Dupont J, et al. A first-in-human phase I study of the anticancer stem cell agent ipafricept (OMP-54F28), a decoy receptor for wnt ligands, in patients with advanced solid tumors. Clin Cancer Res. (2017) 23:7490–7. doi: 10.1158/1078-0432.CCR-17-2157
91. Yu Z, Jiang X, Qin L, Deng H, Wang J, Ren W, et al. A novel UBE2T inhibitor suppresses Wnt/β-catenin signaling hyperactivation and gastric cancer progression by blocking RACK1 ubiquitination. Oncogene. (2021) 40:1027–42. doi: 10.1038/s41388-020-01572-w
92. Wang J, Cai H, Xia Y, Wang S, Xing L, Chen C, et al. Bufalin inhibits gastric cancer invasion and metastasis by down-regulating Wnt/ASCL2 expression. Oncotarget. (2018) 9:23320–33. doi: 10.18632/oncotarget.v9i34
93. Chang TS, Lu CK, Hsieh YY, Wei KL, Chen WM, Tung SY, et al. 2,4-diamino-quinazoline, a wnt signaling inhibitor, suppresses gastric cancer progression and metastasis. Int J Mol Sci. (2020) 21(16):5901. doi: 10.3390/ijms21165901
94. Yamada K, Hori Y, Inoue S, Yamamoto Y, Iso K, Kamiyama H, et al. Selective inhibitor of the interaction between β-catenin and CBP, exerts antitumor activity in tumor models with activated canonical wnt signaling. Cancer Res. (2021) 81:1052–1062.E7386. doi: 10.1158/0008-5472.CAN-20-0782
95. Feng S, Zheng Z, Feng L, Yang L, Chen Z, Lin Y, et al. Proton pump inhibitor pantoprazole inhibits the proliferation, self−renewal and chemoresistance of gastric cancer stem cells via the EMT/β−catenin pathways. Oncol Rep. (2016) 36:3207–14. doi: 10.3892/or.2016.5154
96. Akrami H, Moradi B, Borzabadi Farahani D, Mehdizadeh K. Ibuprofen reduces cell proliferation through inhibiting Wnt/β catenin signaling pathway in gastric cancer stem cells. Cell Biol Int. (2018) 42:949–58. doi: 10.1002/cbin.10959
97. Pang T, Wang X, Gao J, Chen W, Shen XJ, Nie MM, et al. Fiber-modified hexon-chimeric oncolytic adenovirus targeting cancer associated fibroblasts inhibits tumor growth in gastric carcinoma. Oncotarget. (2017) 8:76468–78. doi: 10.18632/oncotarget.v8i44
98. Suthon S, Perkins RS, Bryja V, Miranda-Carboni GA, Krum SA. WNT5B in physiology and disease. Front Cell Dev Biol. (2021) 9:667581.doi: 10.3389/fcell.2021.667581
99. Madan B, McDonald MJ, Foxa GE, Diegel CR, Williams BO, Virshup DM. Bone loss from Wnt inhibition mitigated by concurrent alendronate therapy. Bone Res. (2018) 6:17. doi: 10.1038/s41413-018-0017-8
100. Fischer MM, Cancilla B, Yeung VP, Cattaruzza F, Chartier C, Murriel CL, et al. WNT antagonists exhibit unique combinatorial antitumor activity with taxanes by potentiating mitotic cell death. Sci Adv. (2017) 3:e1700090. doi: 10.1126/sciadv.1700090
Keywords: gastric cancer, Wnt, EMT, tumor microenvironment, molecular agents
Citation: Han R, Yang J, Zhu Y and Gan R (2024) Wnt signaling in gastric cancer: current progress and future prospects. Front. Oncol. 14:1410513. doi: 10.3389/fonc.2024.1410513
Received: 01 April 2024; Accepted: 13 May 2024;
Published: 07 June 2024.
Edited by:
Anurag Kumar Singh, Martin Luther University of Halle-Wittenberg, GermanyReviewed by:
Khyati Maulik Kariya, Harvard Medical School, United StatesRuchi Gupta, George Washington University, United States
Copyright © 2024 Han, Yang, Zhu and Gan. This is an open-access article distributed under the terms of the Creative Commons Attribution License (CC BY). The use, distribution or reproduction in other forums is permitted, provided the original author(s) and the copyright owner(s) are credited and that the original publication in this journal is cited, in accordance with accepted academic practice. No use, distribution or reproduction is permitted which does not comply with these terms.
*Correspondence: Runliang Gan, Z2FucnVubGlhbmdAMTYzLmNvbQ==