- 1The Pathophysiology Department, School of Basic Medical Sciences, College of Medicine, Zhengzhou University, Zhengzhou, China
- 2China-US (Henan) Hormel Cancer Institute, Zhengzhou, China
- 3State Key Laboratory of Esophageal Cancer Prevention and Treatment, Zhengzhou, China
- 4Basic Medicine Sciences Research Center, Zhengzhou University, Zhengzhou, China
- 5Provincial Cooperative Innovation Center for Cancer Chemoprevention, Zhengzhou University, Zhengzhou, China
- 6Cancer Chemoprevention International Collaboration Laboratory, Zhengzhou, China
Gastrointestinal cancer represents a public health concern that seriously endangers human health. The emerging single-cell sequencing (SCS) technologies are different from the large-scale sequencing technologies which provide inaccurate data. SCS is a powerful tool for deciphering the single-cell resolutions of cellular and molecular landscapes, revealing the features of single-cell genomes, transcriptomes, and epigenomes. Recently, SCS has been applied in the field of gastrointestinal cancer research for clarifying the origin and heterogeneity of gastrointestinal cancer, acquiring micro-environmental information, and improving diagnostic and treatment methods. This review outlines the applications of SCS in gastrointestinal cancer research and summarizes the most recent advances in the field.
Introduction
Single-cell sequencing (SCS) has rapidly developed in recent years. The first single-cell mRNA sequencing experiment was performed in 2009, then the first single-cell DNA sequencing experiment in human cancer cells was performed two years later, and the first single-cell exon sequencing experiment was performed in 2012. In 2013, Picelli et al. made some improvements on the smart-seq technology, an SCS protocol (1). This new technology is called smart-seq2. In 2017, 10xGenomics and the Fred Hutchinson Cancer Research Center developed a new single-cell RNA sequencing (scRNA-seq) method, and since then, thousands of immune cells had been analyzed (2, 3). Single-cell sequencing involves the isolation of the cell group in the tissue or body fluid to a single cell level, then the expansion of the extracted nucleic acid (DNA or RNA) to the lowest detection level, followed by sequencing of the genome and transcriptome, and finally correction and analysis of the data. The SCS procedure is shown in Figure 1.
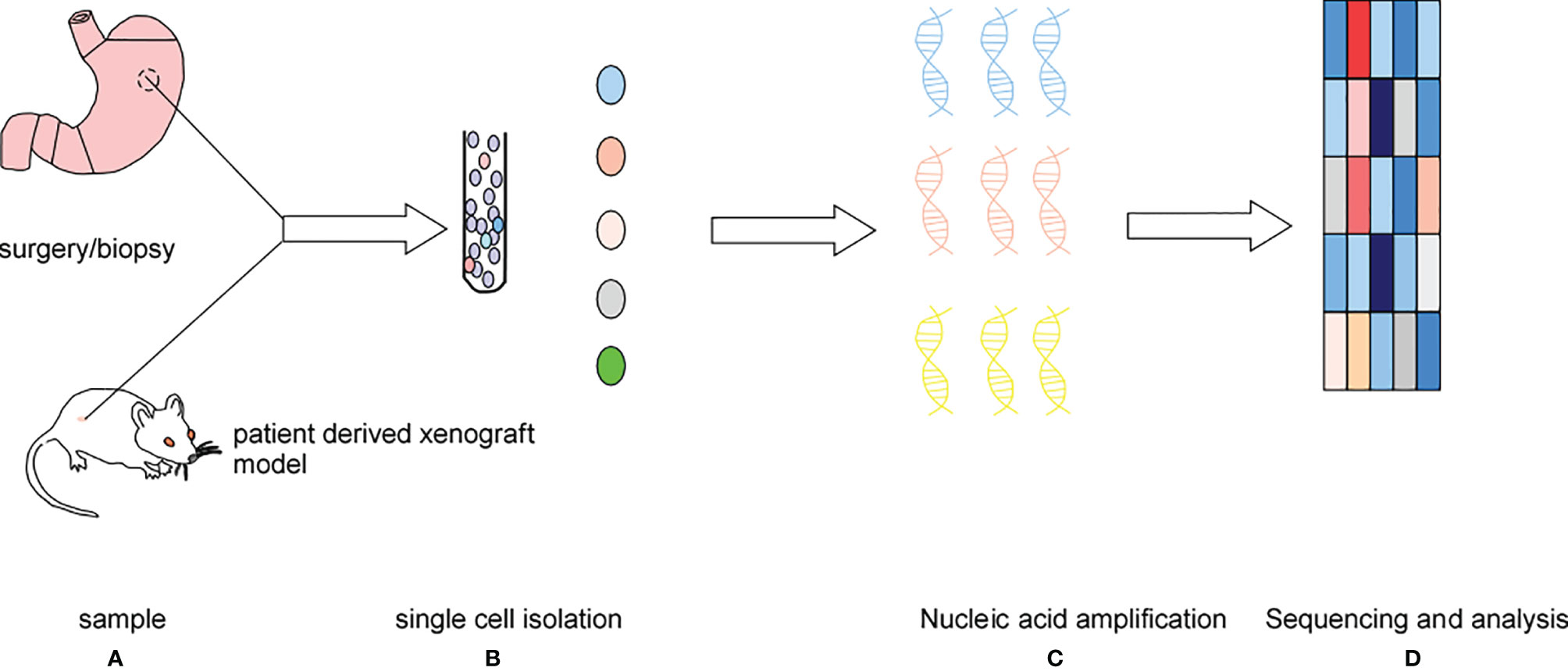
Figure 1 The main processes: (A) sample acquisition, (B) cell isolation, (C) single-cell DNA or RNA amplification, (D) high-throughput sequencing and single-cell sequencing data correction and analysis.
Gastrointestinal (GI) cancers are one of the most common malignant tumors and comprise gastric cancer (GC), esophageal cancer (EC), colorectal cancer (CRC), pancreatic cancer, etc. They are characterized by high morbidity, mortality, malignancy rates, and rapid development (4). GC has a high global prevalence. It accounted for more than 1 million new cases and an estimated 769,000 deaths in 2020, ranking fifth among the causes of global cancer morbidity and fourth among those of cancer mortality (5). Due to the lack of obvious early symptoms, the mortality rate of GC was still one of the highest among malignant tumors. Intriguingly, the incidence of EC was ranked seventh (604,000 cases) for new cases and sixth in the overall mortality rate (544,000 deaths) in 2020. Moreover, CRC (including anal cancer), which accounted for over 1.9 million new cases and 935,000 deaths, ranked third in terms of incidence, but second in terms of mortality among all cancers in 2020. GI cancer is characterized by complex heterogeneity (6) and a specific tumor microenvironment and is extremely suitable for promoting tumor progression and metastasis (7).
SCS plays a significant role in cancer research. Bulk sequencing does not perform well with intratumoral heterogeneity, as it misses rare mutations. For example, in cancer cells, mutations are diluted or lost during averaging of the bulk sequencing (8). In contrast, SCS can be used for the molecular profiling of individual cells and helps in obtaining more precise information about the tumor (Figure 2). Therefore, SCS is a potential superior alternative to traditional sequencing methods. Moreover, although the combination of new resistant chemotherapy, molecular targeted therapy, and immunotherapy techniques has shown promising anti-tumor effects against advanced GI tumors, these techniques have several limitations. SCS can better help researchers investigate problems in tumor heterogeneity, microenvironment, diagnosis and treatment. Based on these advantages, many researchers have made important achievements in cancer research by using this technique. This review focuses on SCS and its applications and achievements in GI cancer studies.
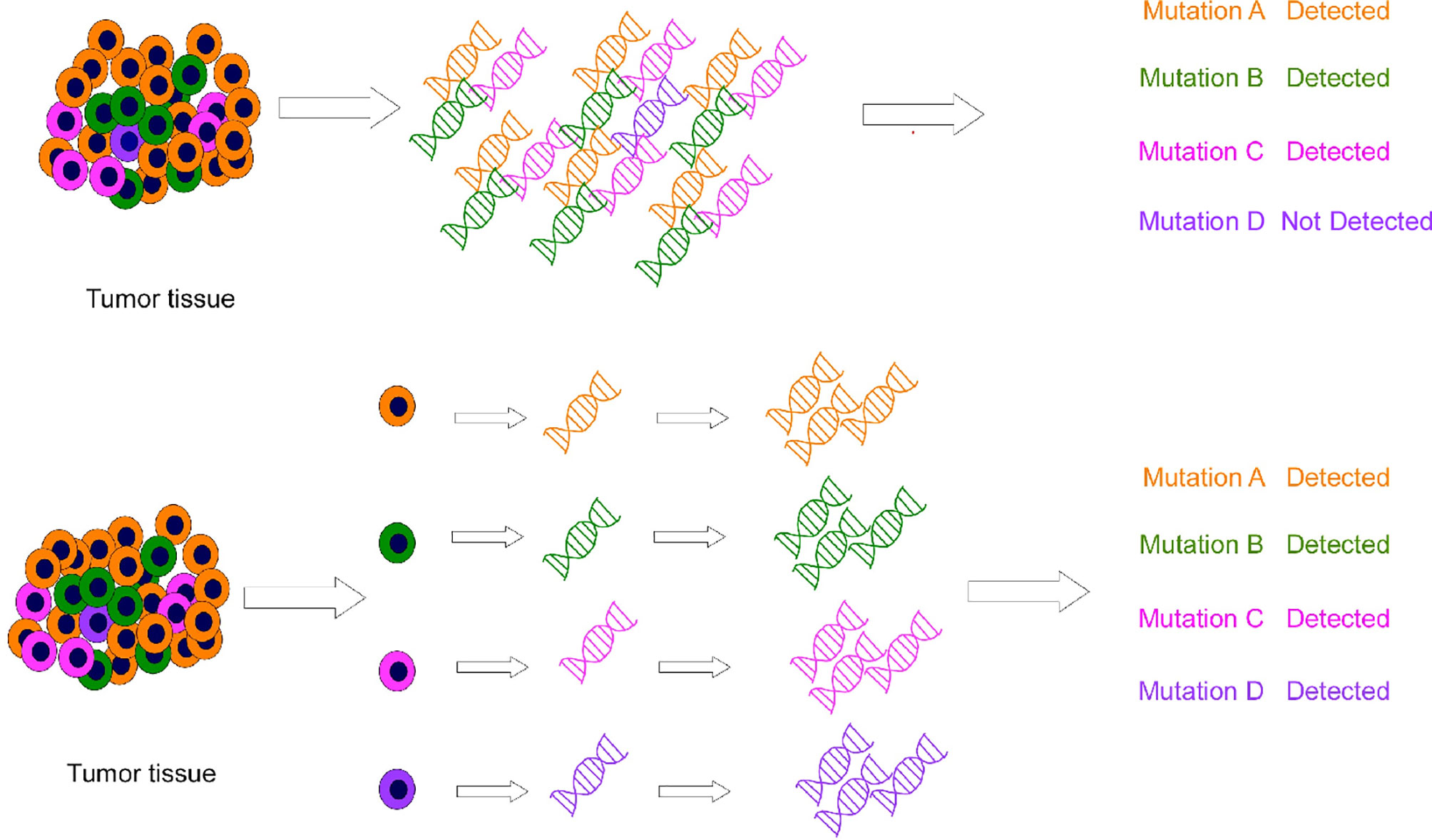
Figure 2 Conventional sequencing (methods above) results in the neglect of some low-abundance information, and single-cell sequencing (methods below) combines cell heterogeneity.
SCS Applications and Achievements in GC
SCS Reveals the Origin and Heterogeneity of GC
Tumor heterogeneity exists due to cell groups with different genotypes in tumor cells during the process of growth, this different cell groups lead to phenotypic inconsistencies. Intratumoral heterogeneity which is observed in various cancers is also one of the main clinical and pathological characteristics of GC. Cellular heterogeneity is significant genotypic differences within the same phenotype and leads to the differences in the growth, invasion, metastasis and drug sensitivity of GC cells (9, 10). Many researchers have used SCS to investigate the origin and heterogeneity of GC (Table 1). Andor et al. characterized cell diversity in nine GC cell lines and assessed gastric cancer heterogeneity and detected scarce clones more efficiently by the high resolution of SCS (11). Peng et al. identified 201 individual cell single nucleotide variations, including 117 non-synonymous mutations, in GC cells by using SCS. They also revealed that there were 24 significant mutant genes, such as CTAGE5, REC8, SORD, and PTCH2 genes, in single cells, wherein the change in single amino acids affected protein conformation. This study firstly showed the mutation pattern of GC cells at the intratumoral level and provided more important information for understanding individualized targeted therapy and the heterogeneity of GC cancer (12). Based on the SCS results of three primary and paired metastatic lymph node cancers in GC patients, Wang et al. identified different tumor characteristics and different patients with different microenvironment subsets; moreover, their clustering data revealed that KIF5B, NOTCH2, NOTCH2NL and ERBB4 were highly expressed in primary carcinomas, whereas ERBB2, CDK12, and CLDN11 were highly expressed in metastatic carcinomas (13). Similarly, in Zhang’s experiment, they classified a subclass of tumor-specific epithelial cells as “GC type 1”, and a subclass consisting of epithelial cells and normal tissues of GC as “GC type 2”. The expression of the intestinal mucosal markers MUC13, TFF3, SPINK4, FABP1, and REG4 were increased in the GC type 1 subclass, while, the expression of the previously identified gastric cancer marker gene KRT7 was increased significantly in the GC type 2 subclass (14). A previous study used SCS and GC tumor cell clusters (C1-C5) to investigate that REG4, CLDN4, TFF3, and CLDN7 were upregulated in the malignant epithelium as compared with that in the non-malignant epithelium. In addition, PGC, MUC5AC, LIPF, and GKN1 were highly expressed in the non-malignant epithelium (15). In a recent study, Wang et al. clarified the relationship between tumor cell lineage/state composition and intratumoral heterogeneity at the transcriptional, genotypic, molecular, and phenotypic levels by using SCS. The study demonstrated the diversity of tumor cell lineage/state components in peritoneal carcinomatosis (PC) samples. The relationship was defined as the key factor responsible for intratumoral heterogeneity (16).
SCS Enables the Discovery of the Features of GC Microenvironment
Tumor cells and their microenvironments are interactive and coevolutionary. The tumor immune microenvironment comprises various tumor-infiltrating immune cells (such as B lymphocytes, T lymphocytes, mast cells, natural killer cells, and myeloid suppressor cells) (17, 18). Tumor cells are also surrounded by the stroma, which is divided into cellular and acellular parts. These compartments are composed of complex tumor microenvironments that interact with cancer cells. SCS helps to clarify the molecular level mechanism of the immune cells in the tumor microenvironment during tumor cell generation, development, metastasis, drug resistance and immune escape. It contributes to a more accurate clinical diagnosis, treatment, and prognosis of solid tumors (19, 20). In the past few years, several researchers have made significant advances in microenvironmental research by using SCS to analyze GC cells (Table 2). For example, Eum et al. used SCS and found that macrophages which were recovered from malignant ascites of GC patients have non-inflammatory characteristics and the anti-inflammatory properties of tumor-associated macrophages (TAMs) were controlled by tumor cells. These findings helped the researchers to continuously improve the treatment strategies for patients with GC (21). Another study by Meyer et al. demonstrated that the expression of several secretory factors, including IL4, IL5, IL9, IL13, and ARG, were involved in the function of L635-treated ILC2 (type 2 innate lymphoid) cells (22). Fu et al. found that the expression of the transcription factor IRF8 in CD8+ tumor-infiltrating lymphocytes in GC tissue was downregulated in the late stage of GC the disease by using SCS. These findings provided a further rationale for targeted immunotherapy in GC (23). Through SCS analysis, researchers confirmed that tumor-specific macrophages existed in a continuum of stimulus-dependent functional states and were regulated by a specific set of genes. They even found that the increase of the abundance of regulatory cells (Tregs) in the gastric tumor microenvironment was related to immunosuppression (14). Kwon et al. found that the dynamic tumor evolution was more related to the collapse of mutant structures in treatment response. Different T-cell receptor lineages were found to be related with a longer progression-free survival with pembrolizumab treatment by combining whole-exome sequencing (WES) with scRNA-seq. In addition, the increase in the number of PD-1+CD8+ T cells was associated with lasting clinical benefits (24).
SCS Facilitates the Diagnosis and Treatment of GC
ScRNA-seq approaches can identify optimal combination therapies that efficiently target heterogeneous cell populations. Furthermore, scRNA-seq can identify the alterations associated with treatment resistance in different cell clusters to support individualized cancer therapy (25). The optimization of existing chemotherapeutic agents and the development of targeted therapies have provided more options for the treatment of advanced gastric cancer and further prolonged the survival expectations of the patients. In addition, global efforts, including the employment of SCS, have been made to identify new specific, predictive, sensitive, and prognostic biomarkers and to establish innovative molecular classifications based on gene expression profiles (26). With the use of SCS, the researchers have made great progress in the diagnosis and treatment of gastric cancer (Table 3). For example, Zhang et al. used SCS to construct a single-cell network based on the cellular and molecular characteristics of gastric epithelial cells with different lesions and establish OR51E1 as a unique endocrine cell marker in early malignant lesions. They also suggested that HES6 may mark goblet precell clusters and these findings helped to identify metaplasia in the early stage. Zhang et al. also determined the specific characteristics which is clinically significant for its accurate diagnosis in early GC (27). SCS can also help to identify markers related to tumor diagnosis and personalized therapy (33, 34). Wang et al. performed SCS to classify PC samples into two subtypes that were predicted independent of clinical variables, obtained and verified the prognostic markers of 12 genes (TPM2, FCGBP, CDK6, NCBP2, CLCX3, PIGR, BTF3, CKB, VPS28, TM4SF1, EIF3E, GPX4) in multiple large-scale gastric adenocarcinoma (GAC) cohorts (16). Another study by Bockerstett et al. established that in Spasmolytic polypeptide-expressing metaplasia (SPEM) and cervical cell proliferation hypertrophy, the expression of SPEM-related transcripts were similar, and the mechanism of drug-mediated parietal cell ablation was similar to that of SPEM induced by chronic inflammation (28). Chen performed single-cell DNA sequencing of 50 target circulating tumor cells (CTCs) and discovered that large multiploid CTCs (LCTCsmulti) and small CTCs with trisomy 8 (SCTCstri) had different gene variations. Moreover, mutations in the KRAS and Rap1 pathways were abundant in SCTCstri, while several unique mutations in the MET/PI3K/AKT pathway and SMARCB1 genes were found in LCTCsmulti. These findings highlighted the different mechanisms of drug resistance for modulating target therapy and could help in preventing the poor prognosis of patients (29). Based on the data from scRNA-seq, some researchers analyzed GC samples to classify them into three GC differentiation-related genes molecular subtypes. They found that molecular typing based on cell differentiation could successfully predict the overall survival of the patient, immune checkpoint gene expression, clinicopathological features. This study emphasized the significance of GC cell differentiation in predicting the clinical outcomes and potential immunotherapy responses of patients (30). Furthermore, by transplanting two GC cell lines into mice and performing single-cell transcriptome sequencing of the transplanted tumors, Nagaoka confirmed that interleukin-17 (IL-17) could be a potential target for enhanced programmed cell death 1, anti-PD-1 (programmed cell death protein 1) mAb treatment for GC (31). Bockerstett et al. sequenced the transcriptome of gastric mucosal epithelial cells and found that gastrin 3 mRNA was a tumor-specific marker of the gastric epithelium of intestinal metaplasia (32). Analysis of the SCS data from mice with hereditary diffuse gastric cancer (HDGC) revealed that inactivation of Cdh1 led to metastasis along the squamous cell differentiation trajectory associated with aberrant expression of GI epithelial differentiation center genes. Cytokeratin 7 encoded by the differentiation-dependent gene Krt7, was a specific marker of early neoplastic lesions in CDH1 carriers (20). In conclusion, SCS is valuable in identifying prognostic tumor markers for predicting potential clinical outcomes and immune responses, as well as, for individualized therapy.
SCS Applications and Achievements in EC
SCS Reveals the Origin and Heterogeneity of EC
SCS plays an important role in studying the origin and heterogeneity of esophageal cancer. Many researchers have used this technique to achieve important results in revealing the esophageal cancer heterogeneity (Table 4). For example, Zhang et al. screened the ESCC cells into 38 subsets, and found that there were 24 subsets with more than 75% of the cells coming from an individual patient. These 24 subsets were defined as the cluster 1, and the remained 14 subsets were defined as the cluster 2. Cluster 1 showed increased pathway activity in cell proliferation and EMT, while cluster 2 showed activation of immune-related pathways. This study showed high heterogeneity in ESCC (35). Wu et al. found that NOTCH signaling was not activated in esophageal adenocarcinoma (EAC) and only activated in ESCC. ESCC tumors with higher NOTCH activity were associated with significantly worse survival. Furthermore, levels of DLL1, JAG1 and NOTCH2 were higher in ESCC and EAC tumors than in normal tissues, while products of active signature genes (SNAI2 and TNFSF10) were only detected in ESCC tissues. This study revealed differences in cellular transcriptomic profiles of ESCC and EAC, and a wide range of intratumoral cellular heterogeneity. This founding had important implications for future therapeutic strategies and drug development (36). Chen et al. reclustered malignant cells from five ESCC tumor samples and identified five subsets. Each subset was corresponded to a patient. They revealed high-risk genes in different patients, and different patients showed different expression patterns for different high-level genes. Furthermore, the SCS and copy number variations (CNVs) analysis data showed relative changes in the CNV profiles of all tumor cells compared to non-malignant epithelial cells. The tumor cells in each subpopulation exhibited different CNV status. These findings demonstrated the high heterogeneity of ESCC tumor cells in terms of gene expression and CNV status (37). Another study explained the high degree of heterogeneity in the ESCC microenvironment. Macrophages were clustered into five subsets. Among the five macrophage subsets, Mac_1, Mac_2 and Mac_3 expressed higher anti-inflammatory “M2” -related genes in Mac_C, with Mac_5 expressing M2-like genes (38).
SCS Enables the Discovery of the Features of EC Microenvironment
SCS plays an important role in determining the cellular characteristics of the EC microenvironment. To date, various studies have utilized SCS techniques to explore the problems in the microenvironment of EC (Table 5). For example, some researchers discovered that TAMs expressed not only genes related to immunosuppression (TGFB1 and COX2) but also genes related to angiogenesis (VEGFA, CXCL8, MMP9, and MMP12), and found that VEGFA was upregulated in monocytes, while MMPs were mainly expressed in TAMs. These results revealed the immunosuppressive status of the esophageal squamous cell carcinoma (ESCC) tumor microenvironment and improved our understanding of ESCC (35). Similarly, several characteristics of CD4+ T cells had been identified by using SCS. Three classes of CD4+ T cells were identified via SCS, and it was found that CD4_1 upregulates TIGIT expression in tumors, CD4_2 expresses PD-1 (encoded by PDCD1) exclusively in tumors, and CD4_3 showed tumor-specific TIGIT and CD96 expression. In addition, both CD4_1 and CD4_2 were found to express high levels of CTLA-4 in tumors (38). Wu et al. recently showed that cell cycle signaling was associated with high cancer stemness of EAC, such as E2F3, CHEK1, CDC20, SMC3, and TFDP1. In addition, they identified a novel cancer stem cell-associated gene, poly (ADP-ribose) polymerase 4, and they validated its association with survival in a cohort study of 121 ESCC patients (39). Another study discovered a strong correlation between FGF2 and SPRY1 expression in EC using SCS. In the fibroblasts in EC tissues, a high FGF2 expression was found to be associated with low overall survival, and the mouse tumor model confirmed that FGF2 overexpression in fibroblasts significantly upregulated SPRY1 expression in the depleted T cells, weakened the cytotoxic activity of T cells, and promoted tumor growth (40). Together, several studies have identified the features of different cells in the EC microenvironment and found some exclusively expressed genes using SCS. These researches has considerably improved our understanding of esophageal carcinogenesis.
SCS Facilitates the Diagnosis and Treatment of EC
SCS also plays an important role in the diagnosis and treatment of EC by facilitating the identification of diagnostic markers and development of new treatment. Many investigators have applied SCS to make great progress in the diagnosis and treatment of EC (Table 6). For example, using scRNA-seq and real-time quantitative PCR, Zhang et al. verified that RAD51AP1, KIF2C, KIF20A,NUF2,PBK, and DEPDC1 are all potential biomarkers for the diagnosis and prognosis of ESCC and may be potential therapeutic targets for ESCC (41). Furthermore, researchers found that CD4+ Tregs expressed the highest levels of IL-32 and had relatively low expression in proliferating natural killer cells and suggested that IL-32 may be a target for immunosuppressive therapy in EC (42). Using scRNA-seq analysis of KYSE-30 cells and paclitaxel-resistant KYSE-30 cells (paclitaxel-R), Wu et al. showed that the two subsets based on KRT19 expression levels had different paclitaxel sensitivity, suggesting there were intrinsic paclitaxel resistance in KYSE-30 cells. They also found that the proteasome inhibitor carfilzomib could attenuate resistance to paclitaxel-R cancer cells by activating HIF-1 signaling, suggesting that the combination of carfilzomib and paclitaxel could be used as a novel cancer treatment (43). Yang et al. carried out scRNA-seq of KYSE-180 cells and found radioresistance in KYSE-180 cells after fractionated irradiation (FIR) treatment. These foundings provided an important reference for developing radiotherapy strategies (44). Another study involved the identification of 42 recurrent radioresponsive genes (sensitive and resistant), including GAS2L2, NOTCH1, OBSCN, MAML3, NFE2L2,TP53 and CDKN2A. This finding provided a reference for the diagnosis and treatment of EC (45).
SCS Applications and Achievements in CRC
SCS Reveals the Origin and Heterogeneity of CRC
SCS is important in revealing the origin and heterogeneity of CRC. With the help of SCS, many investigators have further revealed the origin and heterogeneity of CRC (Table 7). Some researchers performed single-cell analysis of colon cancer samples using high-throughput SCS based on multiple displacement amplification. They focused on a mutant gene, SLC12A5, and found that SLC12A5 activation could promote cell proliferation and inhibit apoptosis, thus potentially promoting oncogenesis and demonstrating the biclonal origin of CRC cases (46). In addition, two normal or adenomatous polyps in CRC patients were studied via single-cell whole-exome sequencing and matched bulk WES. The results indicated that accumulation of non-random somatic gene mutations were involved in the GPCR, PI3K-Akt, and FGFR signaling pathways were also observed. These new driver mutations in OR1B1 (GPCR signaling), LAMA1 (PI3K-Akt signal in CRC evolution), and ADCY3 (FGFR signaling) of adenoma evolution and cancer evolution, confirming that both colorectal adenomas and CRC were of monoclonal origin (47). Davel analyzed scRNA-seq data of 2824 cells from CRC cancer tissues, dividing different cells of tumor tissues into five clusters according to specific genes, further analyzing the cluster data, gene ontology terms, KEGG pathways and trajectory maps.The study found that cluster 1 was characterized by a unique set of genes, such as IGLC7, IGLC2, IGLC3, cluster 2 has unique HLA-DRA, IGHM, IGHG2 other genes, and the remaining three clusters also defined themselves by unique genes. A high degree of specificity between the different clusters was found (48). These results showed that SCS was a powerful tool for studying tumor cell heterogeneity. Owing to the unique high resolution of SCS, scTRIO-SEQ (a type of single-cell triple sequencing) can simultaneously assess somatic copy number changes, DNA methylation, and transcriptomic information and facilitate single-cell heterogeneity research. The high-throughput and high-resolution characteristics of scRNA-seq are also beneficial for the detection of tumor samples (49). Some studies have shown that mutations in ATM and GNAS, as well as deletions in the tumor suppressor gene PTEN, likely led to tumorigenesis because these genes were potential cancer driver genes. Besides, it had been suggested that mutations in TP53, ERBB2, and APC may play an important role in tumorigenesis and may serve as drug targets (50). Markers for two different subtypes of cancer-associated fibroblasts (CAF) were identified using SCS studies. CAF-B cells expressed markers of myofibroblasts such as TAGLN, ACTA2, and PDGFA, and CAF-A cells expressed DCN, MMP2, and COL1A2. Only the CAF-A cells expressed the FAP (fibroblast activa tion protein α). Thus, this indicated that the heterogeneity of CAF may constitute a potential barrier to FAP-directed therapy (51).
SCS Enables the Discovery of the Features of Tumor Microenvironment in CRC
SCS facilitates the discovery of cellular features in the CRC microenvironment (Table 8). SCS data revealed that the proportion of somatic copy number alteration (SCNA) in cancer tissues was much higher than that in adjacent normal tissues (11.1% v.s.10.6%), and five genes (BGN, RCN3, TAGLN, MYL9, and TPM2) were identified as fibroblast-specific biomarkers of poor CRC prognosis. Thus CRC successfully confirmed the extensive genomic alteration in cells in CRC tumor microenvironment (52). Studies of the CRC tumor microenvironment using SCS revealed cancer type-specific T cell subsets and developmental patterns, as well as detailed molecular characterization of tumor immune-related T cell clusters. The cellular and molecular mechanisms underlying the tumor immune microenvironment composition, heterogeneity, and formation were revealed (53). Comprehensive analysis of the non-epithelial scRNA-seq data derived from precancerous lesions and CRC revealed that the proportion of CD8+ T cells, natural killer cells, and γδT cells (labeled cytotoxic cells) was significantly increased in serrated polyps compared to that in adenomas (54). Another study examined TAMs in CRC and found that Bcl9 deficiency caused macrophage polarization inhibition from M0 to M2 and altered the CRC tumor microenvironment to further interfere with the inflammation of M0 and M1, the cell type balance and transcription differences in TAMs regulated by BCL9-driven Wnt signaling affected immune surveillance and inflammation in cancer (55). Together, with the support of new technologies, SCS has greatly promoted a thorough understanding of the tumor microenvironment.
SCS Facilitates the Diagnosis and Treatment of CRC
Many researchers have used SCS to make great achievements in the diagnosis and treatment of CRC (Table 9). Some studies demonstrated that primary tumor cells evolved for a long time and acquired many mutations such as in KRAS, NRAS, APC, and TP53, which spread to distant sites and organs by using high-throughput single-cell DNA sequencing to study the advanced transmission of model metastatic CRC. This transmission model could be extended to many human cancers with important clinical significance (56). Lei et al. conducted scRNA-seq analysis on immune and stromal populations from CRC patients and identified specific macrophage and conventional dendritic cell subsets as key mediators of cellular cross-talk in the tumor microenvironment. Besides, they determined that anti-CSF1R treatment preferentially depleted macrophages with inflammatory features, and CD40 agonist antibody treatment preferentially activated the conventional dendritic cell population (57).
Summary and Prospects
The incidence and mortality rate of malignant tumors in China are the highest among the world, and the overall situation of GI cancer prevention and treatment are very grim. SCS has become an important technique for studying GI cancer. Currently, with its development and integration with other technologies, further improvements and advances in SCS technologies will improve its applicability in clinical settings. However, the technique has some limitations. For example, biological noise will lead to the change of single cell sequencing data and affect the results of data (36). Another limitation is RNA leakage. It may occur during reverse transcription and then may introduce substantial bias (58). SCS also has some shortcomings. Single-cell sequencing is very sensitive to samples and therefore not suitable for analysis of preserved or poorly processed clinical samples.So it is difficult to translate the results from sequencing studies into the clinic (59). The high of SCS cost limits the ability to analyze a large number of tumors, and often only a few to dozens of samples are analyzed per study.
With the development of single-cell multiplexed technologies and the miniaturization and automation of SCS instruments, these limitations and shortcomings may be solved gradually and SCS will have more vast applications in GI cancer research. What’s more, with continuous innovation and optimization of methods, the SCS technology will continue to promote the development of biomedicine and the accurate treatment of GI cancer and may likely aid in achieving high-quality long-term survival for patients with GI cancer.
Author Contributions
YJ and KL participated in the design, conception. ZX, JL, PH, YZ and JY wrote the review. ZX, JL, YJ and KL revised the review. All authors contributed to the article and approved the submitted version.
Funding
This work was supported by the National Natural Science Foundations of China (No. 81872335), National Natural Science Youth Foundation (No. 81902486), Technology Innovation Leading Talents (No. 224200510015), The Central Plains Science and Technology Innovation Leading Talents, the Science and Technology Project of Henan Province (No. 212102310187). The National Undergraduate Innovation Experiment Program of Zhengzhou university. This work is supported by The Pathophysiology Department, School of Basic Medical Sciences, College of Medicine, Zhengzhou University.
Conflict of Interest
The authors declare that the research was conducted in the absence of any commercial or financial relationships that could be construed as a potential conflict of interest.
Publisher’s Note
All claims expressed in this article are solely those of the authors and do not necessarily represent those of their affiliated organizations, or those of the publisher, the editors and the reviewers. Any product that may be evaluated in this article, or claim that may be made by its manufacturer, is not guaranteed or endorsed by the publisher.
Acknowledgments
We thank Dr. Jiang Yanam for his guidance and corrections.
Abbreviations
CAF, cancer-associated fibroblasts; CNVs, copy number variations; CRC, colorectal cancer; CTC, circulating tumor cells; EC, esophagealcancer; EAC, esophageal adenocarcinoma; FIR, fractionated irradiation; GAC, gastric adenocarcinoma; GC, gastric cancer ; GI, Gastrointestinal; HDGC, hereditary diffuse gastric cancer; IL-17, interleukin-17; LCTCsmulti, large multiploid CTCs; paclitaxel-R, paclitaxel-resistant KYSE-30 cells; PC, pancreatic cancer; PD-1, Programmed cell death protein 1; PC, peritoneal carcinomatosis; scRNA-seq, single-cell RNA sequencing; SCS, single-cell sequencing; SCTCstri, small CTCs with trisomy 8; SPEM, Spasmolytic polypeptide-expressing metaplasia; TAMS, tumor-associated macrophages; Tregs, regulatory cells; WES, whole-exome sequencing.
References
1. Picelli S, Björklund ÅK, Faridani OR, Sagasser S, Winberg G, Sandberg R. Smart-Seq2 for Sensitive Full-Length Transcriptome Profiling in Single Cells. Nat Methods (2013) 10(11):1096–8. doi: 10.1038/nmeth.2639
2. Tang F, Barbacioru C, Wang Y, Nordman E, Lee C, Xu N, et al. mRNA-Seq Whole-Transcriptome Analysis of a Single Cell. Nat Methods (2009) 6(5):377–82. doi: 10.1038/nmeth.1315
3. Navin N, Kendall J, Troge J, Andrews P, Rodgers L, McIndoo J, et al. Tumour Evolution Inferred by Single-Cell Sequencing. Nature (2011) 472(7341):90–4. doi: 10.1038/nature09807
4. Zitvogel L, Ma Y, Raoult D, Kroemer G, Gajewski TF. The Microbiome in Cancer Immunotherapy: Diagnostic Tools and Therapeutic Strategies. Science (2018) 359(6382):1366–70. doi: 10.1126/science.aar6918
5. Sung H, Ferlay J, Siegel RL, Laversanne M, Soerjomataram I, Jemal A, et al. Global Cancer Statistics 2020: GLOBOCAN Estimates of Incidence and Mortality Worldwide for 36 Cancers in 185 Countries. CA Cancer J Clin (2021) 71(3):209–49. doi: 10.3322/caac.21660
6. Figueiredo C, Camargo MC, Leite M, Fuentes-Pananá EM, Rabkin CS, Machado JC, et al. Pathogenesis of Gastric Cancer: Genetics and Molecular Classification. Curr Top Microbiol Immunol (2017) 400:277–304. doi: 10.1007/978-3-319-50520-6_12
7. Rojas A, Araya P, Gonzalez I, Morales E. Gastric Tumor Microenvironment. Adv Exp Med Biol (2020) 1226:23–35. doi: 10.1007/978-3-030-36214-0_2
8. Shirai M, Taniguchi T, Kambara H. Emerging Applications of Single-Cell Diagnostics. Top Curr Chem (2014) 336:99–116. doi: 10.1007/128_2012_327
9. Andor N, Graham TA, Jansen M, Xia LC, Aktipis CA, Petritsch C, et al. Pan-Cancer Analysis of the Extent and Consequences of Intratumor Heterogeneity. Nat Med (2016) 22(1):105–13. doi: 10.1038/nm.3984
10. Saunders NA, Simpson F, Thompson EW, Hill MM, Endo-Munoz L, Leggatt G, et al. Role of Intratumoural Heterogeneity in Cancer Drug Resistance: Molecular and Clinical Per-Spectives. EMBO Mol Med (2012) 4(8):675–84. doi: 10.1002/emmm.201101131
11. Andor N, Lau BT, Catalanotti C, Sathe A, Kubit M, Chen J, et al. Joint Single Cell DNA-Seq and RNA-Seq of Gastric Cancer Cell Lines Reveals Rules OfIn Vitroevolution. NAR Genom Bioinform (2020) 2(2):lqaa016. doi: 10.1093/nargab/lqaa016
12. Peng LH, Xing R, Liu DB, Bao L, Cheng WX, Wang HY, et al. Characterization and Validation of Somatic Mutation Spectrum to Reveal Heterogeneity in Gastric Cancer by Single Cell Sequencing. Sci Bull (2019) 64:236–44. doi: 10.1016/j.scib.2018.12.014
13. Wang B, Zhang Y, Qing T, Xing K, Li J, Zhen T, et al. Comprehensive Analysis of Metastatic Gastric Cancer Tumour Cells Using Single-Cell RNA-Seq. Sci Rep (2021) 11(1):1141. doi: 10.1038/s41598-020-80881-2
14. Sathe A, Grimes SM, Lau BT, Chen J, Suarez C, Huang RJ, et al. Single Cell Genomic Characterization Reveals the Cellular Reprogramming of the Gastric Tumor Microenvironment. Clin Cancer Res (2020) 26(11):2640–53. doi: 10.1158/1078-0432.CCR-19-3231
15. Zhang M, Hu S, Min M, Ni Y, Lu Z, Sun X, et al. Dissecting Transcriptional Heterogeneity in Primary Gastric Adenocarcinoma by Single Cell RNA Sequencing. Gut (2021) 70(3):464–75. doi: 10.1136/gutjnl-2019-320368
16. Wang R, Dang M, Harada K, Han G, Wang F, Pool Pizzi M. Single-Cell Dissection of Intratumoral Heterogeneity and Lineage Diversity in Metastatic Gastric Adenocarcinoma. Nat Med (2021) 27(1):141–51. doi: 10.1038/s41591-020-1125-8
17. Stankovic B, Bjørhovde HAK, Skarshaug R, Aamodt H, Frafjord A, Müller E, et al. Immune Cell Composition in Human Non-Small Cell Lung Cancer. Front Immunol (2019) 9:3101. doi: 10.3389/fimmu.2018.03101
18. Hadler-Olsen E, Wirsing AM. Tissue-Infiltrating Immune Cells as Prognostic Markers in Oral Squamous Cell Carcinoma: A Systematic Review and Meta-Analysis. Br J Cancer (2019) 120:714–27. doi: 10.1038/s41416-019-0409-6
19. Bremnes RM, Dønnem T, Al-Saad S, Al-Shibli K, Andersen S, Sirera R, et al. The Role of Tumor Stroma in Cancer Progression and Prognosis: Emphasis on Carcinoma-Asso-Ciated Fibroblasts and non-Small Cell Lung Cancer. J Thorac Oncol (2011) 6(1):209–17. doi: 10.1097/JTO.0b013e3181f8a1bd
20. Dixon K, Brew T, Farnell D, Godwin TD, Cheung S, Chow C, et al. Modelling Hereditary Diffuse Gastric Cancer Initiation Using Transgenic Mouse-Derived Gastric Organoids and Single-Cell Sequencing. J Pathol (2021) 254(3):254–64. doi: 10.1002/path.5675
21. Eum HH, Kwon M, Ryu D, Jo A, Chung W, Kim N, et al. Tumor-Promoting Macrophages Prevail in Malignant Ascites of Advanced Gastric Cancer. Exp Mol Med (2020) 52(12):1976–88. doi: 10.1038/s12276-020-00538-y
22. Meyer AR, Engevik AC, Madorsky T, Belmont E, Stier MT, Norlander AE, et al. Group 2 Innate Lymphoid Cells Coordinate Damage Response in the Stomach. Gastroenterology (2020) 159(6):2077–91.e8. doi: 10.1053/j.gastro.2020.08.051
23. Fu K, Hui B, Wang Q, Lu C, Shi W, Zhang Z, et al. Single-Cell RNA Sequencing of Immune Cells in Gastric Cancer Patients. Aging (Albany NY) (2020) 12(3):2747–63. doi: 10.18632/aging.102774
24. Kwon M, An M, Klempner SJ, Lee H, Kim KM, Sa JK, et al. Determinants of Response and Intrinsic Resistance to PD-1 Blockade in Microsatellite Instability-High Gastric Cancer. Cancer Discov (2021) 11(9):2168–85. doi: 10.1158/2159-8290.CD-21-0219
25. Ding S, Chen X, Shen K. Single-Cell RNA Sequencing in Breast Cancer: Understanding Tumor Heterogeneity and Paving Roads to Individualized Therapy. Cancer Commun (Lond). (2020) 40(8):329–44. doi: 10.1002/cac2.12078
26. Pellino A, Riello E, Nappo F, Brignola S, Murgioni S, Djaballah SA, et al. Targeted Therapies in Metastatic Gastric Cancer: Current Knowledge and Future Perspectives. World J Gastroenterol (2019) 25(38):5773–88. doi: 10.3748/wjg.v25.i38.5773
27. Zhang P, Yang M, Zhang Y, Xiao S, Lai X, Tan A, et al. Dissecting the Single-Cell Transcriptome Network Underlying Gastric Premalignant Lesions and Early Gastric Cancer. Cell Rep (2019) 27(6):1934–47.e5. doi: 10.1016/j.celrep.2019.04.052
28. Bockerstett KA, Lewis SA, Wolf KJ, Noto CN, Jackson NM, Ford EL, et al. Single- Cell Transcriptional Analyses of Spasmolytic Polypeptide- Expressing Metaplasia Arising From Acute Drug Injury and Chronic Inflammation in the Stomach. Gut (2020) 69(6):1027–38. doi: 10.1136/gutjnl-2019-318930
29. Chen Y, Li Y, Qi C, Zhang C, Liu D, Deng Y, et al. Dysregulated KRAS Gene-Signaling Axis and Abnormal Chromatin Remodeling Drive Therapeutic Resistance in Heterogeneous-Sized Circulating Tumor Cells in Gastric Cancer Patients. Cancer Lett (2021) 517:78–87. doi: 10.1016/j.canlet.2021.06.002
30. Xiang R, Rong Y, Ge Y, Song W, Ren J, Fu T. Cell Differentiation Trajectory Predicts Patient Potential Immunotherapy Response and Prognosis in Gastric Cancer. Aging (Albany NY) (2021) 13(4):5928–45. doi: 10.18632/aging.202515
31. Nagaoka K, Shirai M, Taniguchi K, Hosoi A, Sun C, Kobayashi Y, et al. Deep Immunophenotyping at the Single-Cell Level Identifies a Combination of Anti-IL-17 and Checkpoint Blockade as an Effective Treatment in a Preclinical Model of Data-Guided Personalized Immunotherapy. J Immunother Cancer. (2020) 8(2):e001358. doi: 10.1136/jitc-2020-001358
32. Bockerstett KA, Lewis SA, Noto CN, Ford EL, Saenz JB, Jackson NM, et al. Single-Cell Transcriptional Analyses Identify Lineage-Specific Epithelial Responses to Inflammation and Metaplastic Development in the Gastric Corpus. Gastroenterology (2020) 159( 6):2116–29. doi: 10.1053/j.gastro.2020.08.027
33. Mäbert K, Cojoc M, Peitzsch C, Kurth I, Souchelnytskyi S, Dubrovska A. Cancer Biomarker Discovery: Current Status and Future Perspectives. Int J Radiat Biol (2014) 90(8):659–77. doi: 10.3109/09553002.2014.892229
34. Normanno N, Rachiglio AM, Roma C, Fenizia F, Esposito C, Pasquale R, et al. Molecular Diagnostics and Personalized Medicine in Oncology: Challenges and Opportunities. J Cell Biochem (2013) 114(3):514⁃524. doi: 10.1002/jcb.24401
35. Zhang X, Peng L, Luo Y, Zhang S, Pu Y, Chen Y, et al. Dissecting Esophageal Squamous-Cell Carcinoma Ecosystem by Single-Cell Transcriptomic Analysis. Nat Commun (2021) 12(1):5291. doi: 10.1038/s41467-021-25539-x
36. Wu H, Yu J, Li Y, Hou Q, Zhou R, Zhang N, et al. Single-Cell RNA Sequencing Reveals Diverse Intratumoral Heterogeneities and Gene Signatures of Two Types of Esophageal Cancers. Cancer Lett (2018) 438:133–43. doi: 10.1016/j.canlet.2018.09.017
37. Chen Z, Zhao M, Liang J, Hu Z, Huang Y, Li M, et al. Dissecting the Single-Cell Transcriptome Network Underlying Esophagus non-Malignant Tissues and Esophageal Squamous Cell Carcinoma. EBioMedicine (2021) 69:103459. doi: 10.1016/j.ebiom.2021.103459
38. Dinh HQ, Pan F, Wang G, Huang QF, Olingy CE, Wu ZY, et al. Integrated Single-Cell Transcriptome Analysis Reveals Heterogeneity of Esophageal Squamous Cell Carcinoma Microenvironment. Nat Commun (2021) 12(1):7335. doi: 10.1038/s41467-021-27599-5
39. Wu H, Li Y, Hou Q, Zhou R, Li Z, Wu S, et al. Single-cell Intratumoral Stemness Analysis Reveals the Involvement of Cell Cycle and DNA Damage Repair in Two Different Types of Esophageal Cancer. Oncol Rep (2019) 41(6):3201–8. doi: 10.3892/or.2019.7117
40. Chen QY, Li YN, Wang XY, Zhang X, Hu Y, Li L, et al. Tumor Fibroblast-Derived FGF2 Regulates Expression of SPRY1 in Esophageal Tumor-Infiltrating T Cells and Plays a Role in T-Cell Exhaustion. Cancer Res (2020) 80(24):5583–96. doi: 10.1158/0008-5472.CAN-20-1542
41. Zheng L, Li L, Xie J, Jin H, Zhu N. Six Novel Biomarkers for Diagnosis and Prognosis of Esophageal Squamous Cell Carcinoma: Validated by scRNA-Seq and qPCR. J Cancer. (2021) 12(3):899–911. doi: 10.7150/jca.50443
42. Han L, Chen S, Chen Z, Zhou B, Zheng Y, Shen L. Interleukin 32 Promotes Foxp3+ Treg Cell Development and CD8+ T Cell Function in Human Esophageal Squamous Cell Carcinoma Microenvironment. Front Cell Dev Biol (2021) 9:704853. doi: 10.3389/fcell.2021.704853
43. Wu H, Chen S, Yu J, Li Y, Zhang XY, Yang L, et al. Single-Cell Transcriptome Analyses Reveal Molecular Signals to Intrinsic and Acquired Paclitaxel Resistance in Esophageal Squamous Cancer Cells. Cancer Lett (2018) 420:156–67. doi: 10.1016/j.canlet.2018.01.059
44. Yang L, Zhang X, Hou Q, Huang M, Zhang H, Jiang Z, et al. Single-Cell RNA-Seq of Esophageal Squamous Cell Carcinoma Cell Line With Fractionated Irradiation Reveals Radioresistant Gene Expression Patterns. BMC Genomics (2019) 20(1):611. doi: 10.1186/s12864-019-5970-0
45. Yang L, Zhang X, MacKay M, Foox J, Hou Q, Zheng X, et al. Identification of Radioresponsive Genes in Esophageal Cancer From Longitudinal and Single Cell Exome Sequencing. Int J Radiat Oncol Biol Phys (2020) 108(4):1103–14. doi: 10.1016/j.ijrobp.2020.06.015
46. Yu C, Yu J, Yao X, Wu WKK, Lu Y, Tang S, et al. Discovery of Biclonal Origin and a Novel Oncogene SLC12A5 in Colon Cancer by Single-Cell Sequencing. Cell Res (2014) 24(6):701–12. doi: 10.1038/cr.2014.43
47. Wu H, Zhang X-Y, Hu Z, Hou Q, Zhang H, Li Y, et al. Evolution and Heterogeneity of non-Hereditary Colorectal Cancer Revealed by Single-Cell Exome Sequencing. Oncogene (2017) 36(20):2857–67. doi: 10.1038/onc.2016.438
48. Dai W, Zhou F, Tang D, Lin L, Zou C, Tan W, et al. Single-Cell Transcriptional Profiling Reveals the Heterogenicity in Colorectal Cancer. Med (Baltimore) (2019) 98(34):e16916. doi: 10.1097/MD.0000000000016916
49. Bian S, Hou Yu, Zhou X, Li X, Yong J, Wang Y, et al. Single-Cell Multiomics Sequencing and Analyses of Human Colorectal Cancer. Science (2018) 362(6418):1060–3. doi: 10.1126/science.aao3791
50. Liu M, Liu Y, Di J, Su Z, Yang H, Jiang B, et al. Multi-Region and Single-Cell Sequencing Reveal Variable Genomic Heterogeneity in Rectal Cancer. BMC Cancer (2017) 17(1):787. doi: 10.1186/s12885-017-3777-4
51. Li H, Courtois ET, Sengupta D, Tan Y, Chen KH, Goh JJL, et al. Reference Component Analysis of Single-Cell Transcriptomes Elucidates Cellular Heterogeneity in Human Colorectal Tumors. Nat Genet (2017) 49(5):708–18. doi: 10.1038/ng.3818
52. Zhou Y, Bian S, Zhou X, Cui Y, Wang W, Wen Lu. Single-Cell Multiomics Sequencing Reveals Prevalent Genomic Alterations in Tumor Stromal Cells of Human Colorectal Cancer. Cancer Cell (2020) 38(6):818–28.e5. doi: 10.1016/j.ccell.2020.09.015
53. Ren X, Zhang Z. Understanding Tumor-Infiltrating Lymphocytes by Single Cell RNA Sequencing. Adv Immunol (2019) 144:217–45. doi: 10.1016/bs.ai.2019.08.004
54. Chen B, Scurrah CR, McKinley ET, Simmons AJ, Ramirez-Solano MA, Zhu X, et al. Differential Pre-Malignant Programs and Microenvironment Chart Distinct Paths to Malignancy in Human Colorectal Polyps. Cell (2021) 184(26):6262–80.e26. doi: 10.1016/j.cell.2021.11.031
55. Wei Z, Yang M, Feng M, Wu Z, Rosin-Arbesfeld R, Dong J, et al. Inhibition of BCL9 Modulates the Cellular Landscape of Tumor-Associated Macrophages in the Tumor Immune Microenvironment of Colorectal Cancer. Front Pharmacol (2021) 12:713331. doi: 10.3389/fphar.2021.713331
56. Leung ML, Davis A, Gao R, Casasent A, Wang Y, Sei E, et al. Single-Cell DNA Sequencing Reveals a Late-Dissemination Model in Metastatic Colorectal Cancer. Genome Res (2017) 27(8):1287–99. doi: 10.1101/gr.209973.116
57. Zhang L, Li Z, Skrzypczynska KM, Fang Q, Zhang W, O'Brien SA. Single-Cell Analyses Inform Mechanisms of Myeloid Targeted Therapies in Colon Cancer. Cell (2020) 181(2):442–59.e29. doi: 10.1016/j.cell.2020.03.048
58. Rosati D, Giordano A. Single-Cell RNA Sequencing and Bioinformatics as Tools to Decipher Cancer Heterogenicity and Mechanisms of Drug Resistance. Biochem Pharmacol (2022) 195:114811. doi: 10.1016/j.bcp.2021.114811
Keywords: single-cell sequencing, gastrointestinal cancer, gastric cancer, esophageal cancer, colon cancer
Citation: Xie Z, Li J, Huang P, Zhang Y, Yang J, Liu K and Jiang Y (2022) Applications and Achievements of Single-Cell Sequencing in Gastrointestinal Cancer. Front. Oncol. 12:905571. doi: 10.3389/fonc.2022.905571
Received: 27 March 2022; Accepted: 13 May 2022;
Published: 16 June 2022.
Edited by:
Dunfa Peng, University of Miami, United StatesReviewed by:
Kannappan Sriramajayam, University of Miami Health System, United StatesLei Chen, Capital Medical Univerisity, China
Copyright © 2022 Xie, Li, Huang, Zhang, Yang, Liu and Jiang. This is an open-access article distributed under the terms of the Creative Commons Attribution License (CC BY). The use, distribution or reproduction in other forums is permitted, provided the original author(s) and the copyright owner(s) are credited and that the original publication in this journal is cited, in accordance with accepted academic practice. No use, distribution or reproduction is permitted which does not comply with these terms.
*Correspondence: Yanan Jiang, eWFuYW5qaWFuZ0B6enUuZWR1LmNu; Kangdong Liu, a2RsaXVAenp1LmVkdS5jbg==
†These authors have contributed equally to this work and share first authorship