- 1AZTI, Marine Research, Basque Research and Technology Alliance (BRTA), Pasaia, Gipuzkoa, Spain
- 2Dipartimento di Scienze e Tecnologie Biologiche e Ambientali (DiSTeBA), Università del Salento, Lecce, Italy
- 3Consorzio Nazionale Interuniversitario per le Scienze del Mare (CoNISMa), Rome, Italy
- 4National Biodiversity Future Center (NBFC), Palermo, Italy
- 5Centre for Environment, Fisheries and Aquaculture Science (CEFAS), Lowestoft Laboratory, Lowestoft, United Kingdom
- 6Department of Marine Sciences, University of the Aegean, Mytilene, Greece
- 7DTU Aqua, Centre for Gelatinous Plankton Ecology & Evolution, Technical University of Denmark, Lyngby, Denmark
- 8Laboratory of Ecology, Department of Earth and Marine Science (DiSTeM), University of Palermo, Palermo, Italy
- 9Italian Institute for Environmental Protection and Research (ISPRA), Rome, Italy
- 10International Estuarine & Coastal Specialists (IECS) Ltd., Leven, United Kingdom
- 11Consiglio Nazionale delle Ricerche (CNR), Istituto di Scienze delle Produzioni Alimentari, Lecce, Italy
- 12Marine Conservation Research Group, University of Plymouth, Plymouth, United Kingdom
- 13School of Biological, Earth, and Environmental Sciences, University College Cork, Cork, Ireland
- 14Science Foundation Ireland Research Centre for Energy, Climate, and Marine Environmental Research Centre, University College Cork, Cork, Ireland
Jellyfish and gelatinous zooplankton (GZ) in general, fulfill important ecological roles with significant impacts, although they are often oversimplified or misunderstood. This paper reviews the impacts, pressures, monitoring methods and current management strategies for various GZ groups. It also introduces potentially applicable indicators for their assessment in ecosystem-based management approaches, such as the European Marine Strategy Framework Directive (MSFD). This multi-faceted review is primarily envisioned to serve as a state-of-the-art document for scientists and policymakers to foster a holistic assessment and management of GZ across European regional seas. The systematic review on global impacts of GZ shows a notable increase in the number of studies since the early 2000s. Stings were the main cause of human health impacts. Mechanisms that impact biodiversity included direct predation, modification of trophic flows or competition for resources. Several GZ taxa may be beneficial to biodiversity acting as biological regulators and provide societal ecosystem services such as food provision or medical applications. The systematic review on monitoring techniques outlined a variety of methods, such as nets (the most common technique), continuous plankton recorder (CPR), polyp and jelly-fall monitoring, acoustic methods, remote aerial and underwater imaging, molecular methods, and citizen science. Furthermore, several currently employed management strategies were enumerated, including the use of anti-jelly nets, bubble curtains, chemical compounds, or the introduction of GZ predators. This study highlights the pressing need for enhanced GZ-dedicated monitoring, assessment, and anticipatory management of GZ populations to address future GZ crises more effectively and cost-efficiently. Moreover, exploring GZ ecosystem services unveils opportunities to harness marine resources while mitigating adverse effects, thereby supporting sustainable blue economies.
1 Introduction
The term “jellyfish” collectively encompasses gelatinous zooplankton (GZ) from diverse metazoan taxonomic groups, including Cnidaria (cnidarian jellyfish or medusozoans: the planktonic life stages of Hydrozoa, Scyphozoa and Cubozoa), Ctenophora (comb jellyfish), and pelagic Tunicata (e.g., larvaceans, salps, and doliolids) (Boero, 2013; Jaspers et al., 2023). GZ can exhibit complex life cycles. Several medusozoan species frequently comprise an alternation or coexistence of asexual (often benthic) polyp and sexual medusa stages (Russell, 1953, 1970). Non-metazoan gelatinous organisms are not considered here.
Jellyfish have long been associated with stinging risks to bathers and adverse impacts on diverse socioeconomic activities at sea (Bosch-Belmar et al., 2021b; Lee et al., 2023). In recent years, there seems to be a public perception of a global increase of jellyfish outbreaks, which is often attributed to climate change. This perception is enhanced by media reports portraying historically documented jellyfish outbreaks as novel phenomena (e.g., mass fish kills in the UK and Ireland caused by Pelagia noctiluca outbreaks). However, there are few long-term data and insufficient reference baselines to substantiate this claim (Condon et al., 2012) and the available long-term data document large fluctuations without understanding the underlying causes (see Decker et al., 2023).
It is now scientifically acknowledged that gelatinous zooplankton play a vital role in marine ecosystems and may provide a range of benefits to humans (Doyle et al., 2014; Graham et al., 2014; Culhane et al., 2019; Jaspers et al., 2023). However, despite their importance, the ecological roles of GZ are often grossly oversimplified or misunderstood, and GZ taxa remain poorly monitored compared to other zooplankton groups (Templeman et al., 2021). Managers limit resources for monitoring GZ based on the assumption of their unpredictable nature (Aubert et al., 2018) and some monitoring difficulties, such as sample damages during collection, preservation difficulties, or scarcity of taxonomic expertise on surveys.
Currently, jellyfish management is mainly focused on responsive control and mitigation of local impacts (Dong, 2019). Nevertheless, as marine ecosystems continue to be altered by climate change and human activities, and the abundance and frequency of some GZ species increases in some coastal waters (Brotz and Pauly, 2012; Lee et al., 2023), approaches such as ecosystem-based management strategies can help anticipate GZ outbreaks, rather than simply respond to emergencies, which have greater costs and societal impacts (Brodeur et al., 2016).
In Europe, the Marine Strategy Framework Directive (MSFD, European Commission, 2008), marked a significant milestone in adopting an ecosystem-based management approach for sustainable supply of marine goods and services across Europe. The initial MSFD's objective was to achieve Good Environmental Status (GES) in European seas by 2020 (now, by 2026) (European Commission, 2020). Implemented through a 6-year adaptive management cycle, the MSFD includes assessing the status of the marine environment and its essential features, analyzing their predominant pressures and impacts, and considering economic and social aspects of sea use (Art. 8 MSFD, European Commission, 2008). For assessing the status of European Seas, determining GES (Art. 9 MSFD), environmental targets and associated indicators (Art. 10 MSFD), leads to the development of monitoring programs (Art. 11 MSFD), and programs of measures (Art. 13 MSFD) to maintain or restore GES (Palialexis et al., 2021).
In 2010, the Joint Research Centre - MSFD Task Group 4 on Food Webs recommended assessing the abundance and distribution of key taxa with fast turnover rates, such as jellyfish. These taxa can serve as early warning indicators of food web functioning in response to environmental changes (Rogers et al., 2010). Despite this recommendation, GZ were almost absent in the 2012 and 2018 assessment reporting cycles (Tornero Alvarez et al., 2023). Nonetheless, past and ongoing initiatives continue to propose “cost-effective” monitoring and assessment strategies and tools to include GZ information in MSFD assessments (Aubert et al., 2018; Magliozzi et al., 2021, 2023).
This study reviews the main impacts, pressures, and management options described in the literature, as well as current and upcoming monitoring methods and indicators applicable to assess GZ. This paper aims to serve as a practical state-of-the-art document for scientists and policymakers to foster the assessment and management of GZ across European regional seas and contribute to the achievement of GES.
2 Methods
This work includes one traditional literature review based on comprehensive, critical, and objective analysis of the current knowledge for pressures, indicators, and management sections, and three systematic literature reviews for the sections on impacts and monitoring techniques. The systematic reviews followed the PRISMA (Preferred Reporting Items for Systematic Reviews and Meta-Analyses) guidelines (Moher et al., 2009) and the detailed search criteria were the following ones:
For the systematic review on impacts, the search string used, combining keywords, Boolean operators and wildcards, was: (“gelatinous *plankton” OR jellyfish OR cnidaria* OR scyphozoa* OR hydrozoa* OR cubozoa* OR medusozoa OR medusa* OR ctenophor* OR salp* OR tunicat* OR thaliacea* OR appendicularia* OR doliolid* OR urochordat* OR siphonophor*) AND (impact* OR effect* OR consequence* OR damag* OR loss OR sting OR econom*) AND (bloom* OR outbreak* OR swarm* OR proliferation* OR aggregation* OR accumulation* OR “mass occurrence”). The search was conducted on the 5th of April 2023, it was limited to the title, abstract, and keywords, and was not restricted by publication year. The initial search yielded 2,382 and 1,378 articles from Scopus and Web of Science online databases, respectively (Supplementary Figure S1). Screening of additional publications identified by experts or within the references of assessed articles or reviews was carried out (n = 151 articles). Following the removal of duplicate entries, 2,596 articles remained for the initial screening stage. Four reviewers assessed the articles for eligibility and inclusion in the second-stage full-text screening, based on the titles and abstracts. Inclusion criteria was based on a GZ relative population level being assessed for potential negative impacts on biodiversity, ecosystem services or human health at global scale, without any geographical restriction. Exclusion criteria included the manuscript's language (only English, Spanish, Italian, Portuguese, Greek, or French were considered), and the publication type encompassing only published research documents with primary evidence of GZ impacts. Review studies that did not provide primary knowledge on impacts were removed to prevent the inclusion of duplicate records of impacts. It is important to note that all relevant review articles underwent full-text screening, and additional relevant references missing in the literature search were added for screening. To ensure inter-rater reliability, the reviewers independently evaluated a randomly selected sample of 50 retrieved articles, subsequently discussing any discrepancies. This validation process involved the four participant reviewers of the first stage screening. The first stage screening of title and abstract for eligibility against the inclusion criteria resulted in 306 articles selected for the second stage full-text screening (the full process can be tracked in PRISMA flow diagram Supplementary Figure S1). In this subsequent phase, eight reviewers were engaged in examining the full text of retrieved articles to determine their eligibility and extract pertinent information from the included studies. Finally, 212 articles were included for data extraction and 94 articles were excluded as they did not meet the above-mentioned inclusion criteria.
The relevant information extracted from the selected articles included: (1) year of publication; (2) marine realm and province (based on Spalding et al., 2007); (3) species identified as having an impact; (4) type of evidence classified into six categories (Katsanevakis et al., 2014): manipulative or natural experiments, experiments, direct observation of impacts, modeling, non-experimental based correlations, and expert judgment (definitions of each type of evidence category are provided in Supplementary Table S1), (5) mechanisms of impacts on biodiversity, ecosystem services, and human health, (6) magnitude of the impact on biodiversity categorized as minimal, minor, moderate, major, or massive according to Blackburn et al. (2014) (definitions of each magnitude category are provided in Supplementary Table S2), and (7) any indication of benefits from GZ.
For the systematic review on monitoring methods, the search string used was: (“gelatinous *plankton” OR jellyfish* OR cnidaria* OR scyphozoa* OR hydrozoa* OR cubozoa* OR medusozoa OR medusa* OR ctenophor* OR salp* OR tunicat* OR thaliacea* OR appendicularia* OR doliolid* OR urochordat* OR siphonophor*) AND (monitor* OR survey* OR sampl* OR detect*) AND (bloom* OR outbreak* OR swarm* OR proliferation* OR aggregation* OR accumulation* OR “mass occurrence”). The search was implemented on Scopus and Web of Science online databases, covering peer-reviewed literature from 2008 (year of the MSFD publication) to 20th April 2023. The initial search yielded 1,113 and 665 articles from Scopus and Web of Science online databases, respectively (Supplementary Figure S2). Screening of additional publications identified by experts was carried out (n = 8 articles). Following the removal of duplicate entries, 1,171 articles remained for the initial screening stage. Three reviewers assessed the articles for eligibility to be included in the second-stage screening, based on title and abstract. The inclusion criteria applied was based on (1) the mention of GZ identification techniques; and (2) the use or development of monitoring tools. Exclusion criteria considered (1) the specific language of the manuscripts (only English, Spanish, Italian, Portuguese, Greek, or French were considered), (2) publication type and (3) if monitoring method referred to modeling approach to forecast GZ occurrence, not to a direct and currently applied technique for monitoring. Each reviewer independently evaluated a third of the total articles, and subsequently the other two reviewers checked for agreement/disagreement with the original decision and discussed any discrepancies. This validation process occurred during various virtual meetings involving the three reviewers. After the first screening regarding the appropriateness of each article to be included as a monitoring technique paper, 282 articles were selected for the second-stage screening. In this subsequent phase, eleven reviewers were engaged in examining the full text of retrieved articles to determine their eligibility and extract pertinent information from the included studies. Ultimately, 212 articles were included for data extraction.
An additional systematic search was performed specifically for monitoring on medusozoan polyps. In this case the search string was: (polyp* OR scyphopolyp* OR cubopolyp* OR scyphistoma*) AND (monitor* OR survey* OR sampl* OR detect*) AND (“gelatinous *plankton” OR jellyfish* OR cnidaria* OR scyphozoa* OR hydrozoa* OR cubozoa* OR medusozoa OR medusa*). The initial inventory of 291 (Scopus) and 181 (Web of Science) papers published from 2008 to 19th June 2023 was reduced to 302 after removing duplicates (Supplementary Figure S3). These papers were consecutively screened by title, abstract and full text by three reviewers, resulting in 73 articles that mentioned marine polyp identification techniques (excluding freshwater species and benthic hydrozoan), the use or development of monitoring tools, and were written in English, Spanish, Italian, Portuguese, Greek, or French. Out of these, 72 articles were selected for the second-stage screening. In this subsequent phase, four reviewers were engaged in examining the full text of retrieved articles to determine their eligibility and extract pertinent information from the included studies. Ultimately, 19 articles were included for data extraction.
From both sets of selected articles on monitoring techniques, relevant information was retrieved, including: (1) year of publication, (2) survey temporal coverage (year/month), (3) survey spatial coverage (country, site name, geographical coordinates, and marine realm and province, based on Spalding et al., 2007), (4) monitoring methodology used, (5) GZ species considered, (6) monitoring objectives, and (7) results related to stressors present in the area of GZ proliferation, predictions, geographical or phenological changes, abundance/biomass (and units used), outbreak periodicity, and shifts in species composition.
The list of articles obtained in the three searches, as well as the row data extracted are publicly available as Supplementary material (see Data availability statement). The figures were created using the open-source software R 3.6.0 (R Core Team, 2020) and the “ggplot2” package (Wickham, 2016).
3 Results
3.1 Impacts caused by gelatinous zooplankton
Most adverse impacts caused by GZ on maritime activities and ecosystems result from mass occurrences that can range from small, localized spots to large patches detectable for kilometers. GZ aggregations can form due to passive drift by wind or currents, or because of active swimming, by which they form swarms, often in association with sudden population increases or “outbreaks” (Alldredge, 1982; Hamner and Dawson, 2009). The high reproductive and growth potential for outbreaks is observed in a limited number of Cnidaria genera, belonging to the Rhizostomeae and Semaeostomae scyphozoans, in hydrozoans (Hamner and Dawson, 2009; Fernández-Alías et al., 2021; Leoni, 2022), in some ctenophore species (Jaspers et al., 2018a; Shiganova et al., 2019), and in most pelagic Tunicata (Jaspers et al., 2023). In cases of highly venomous species (e.g., Chironex fleckeri, Physalia physalis), only a few individuals may adversely impact human health (e.g., Lippmann et al., 2011; Cegolon et al., 2013).
The systematic review on global impacts of GZ revealed that most of the studies were conducted in the temperate Northern Atlantic (57%) (Figure 1A). The number of studies on negative GZ's impacts notably increased after the early 2000s (Figure 1B). Most of these studies focused on GZ's impacts on biodiversity, food provision, or human health, and few addressed impacts on recreation and tourism, ocean nourishment and water storage (Figure 1C). The term “biodiversity” is used hereby in accordance with the definition of “biological diversity” proposed by the Convention on Biological Diversity (CBD, 1992) and taken up in the MSFD - Task Group 1 report (Cochrane et al., 2010).
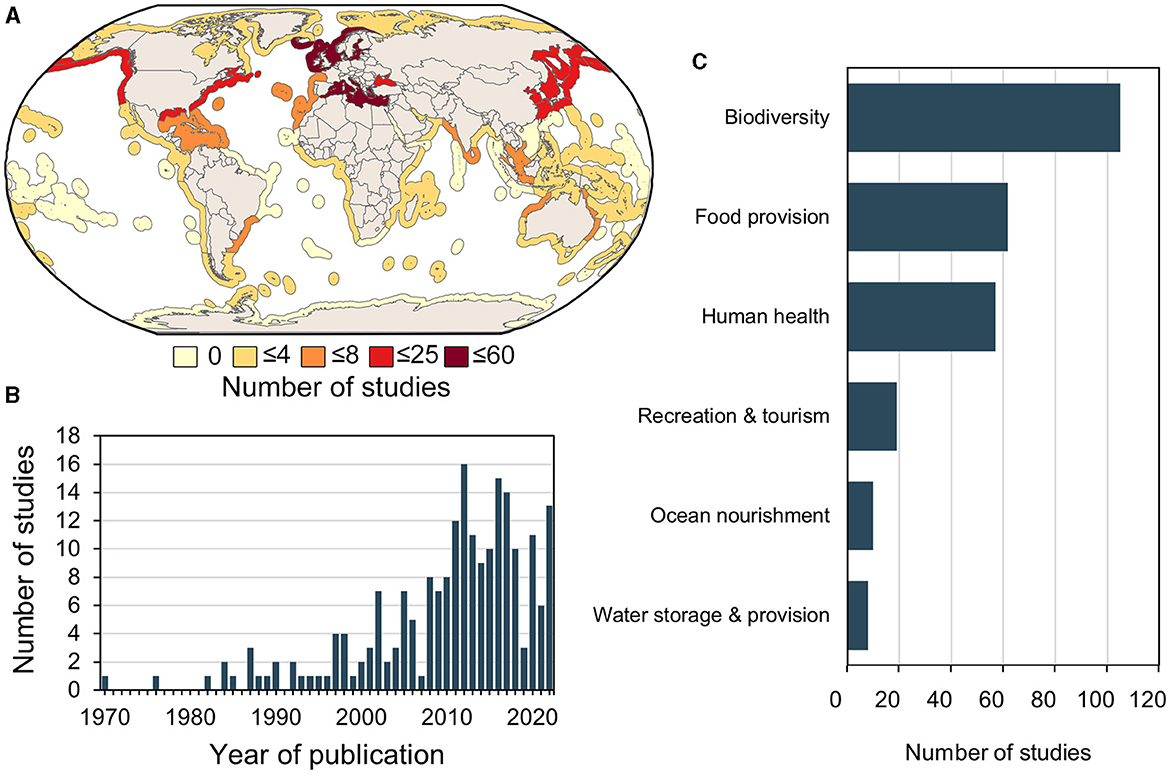
Figure 1. (A) Spatial distribution of studies investigating negative impacts of gelatinous zooplankton (GZ) on biodiversity, ecosystem services and human health, (B) number of studies published per year investigating GZ impacts, and (C) number of studies investigating GZ impacts on biodiversity, ecosystem services categories (food provision, recreation and tourism, water storage and provision, and ocean nourishment) and human health.
Impacts on biodiversity and ecosystem services were investigated mostly in the northern European Seas and the Mediterranean Sea (Figures 2A, B). GZ were frequently reported to impact aquaculture facilities through both stings (Baxter et al., 2011a; Bosch-Belmar et al., 2016a,b, 2017; Marcos-López et al., 2016; Powell et al., 2018; Mitchell et al., 2021) and disease transmission to farmed fish (Ferguson et al., 2010; Delannoy et al., 2011; Clinton et al., 2021). Impacts on human health were less frequently reported and mostly found along temperate and tropical coasts. Stings were the primary cause of human health impacts, and only three articles identified GZ as potential vectors of pathogens (Basso et al., 2019; Stabili et al., 2020, 2022) (Figure 2C).
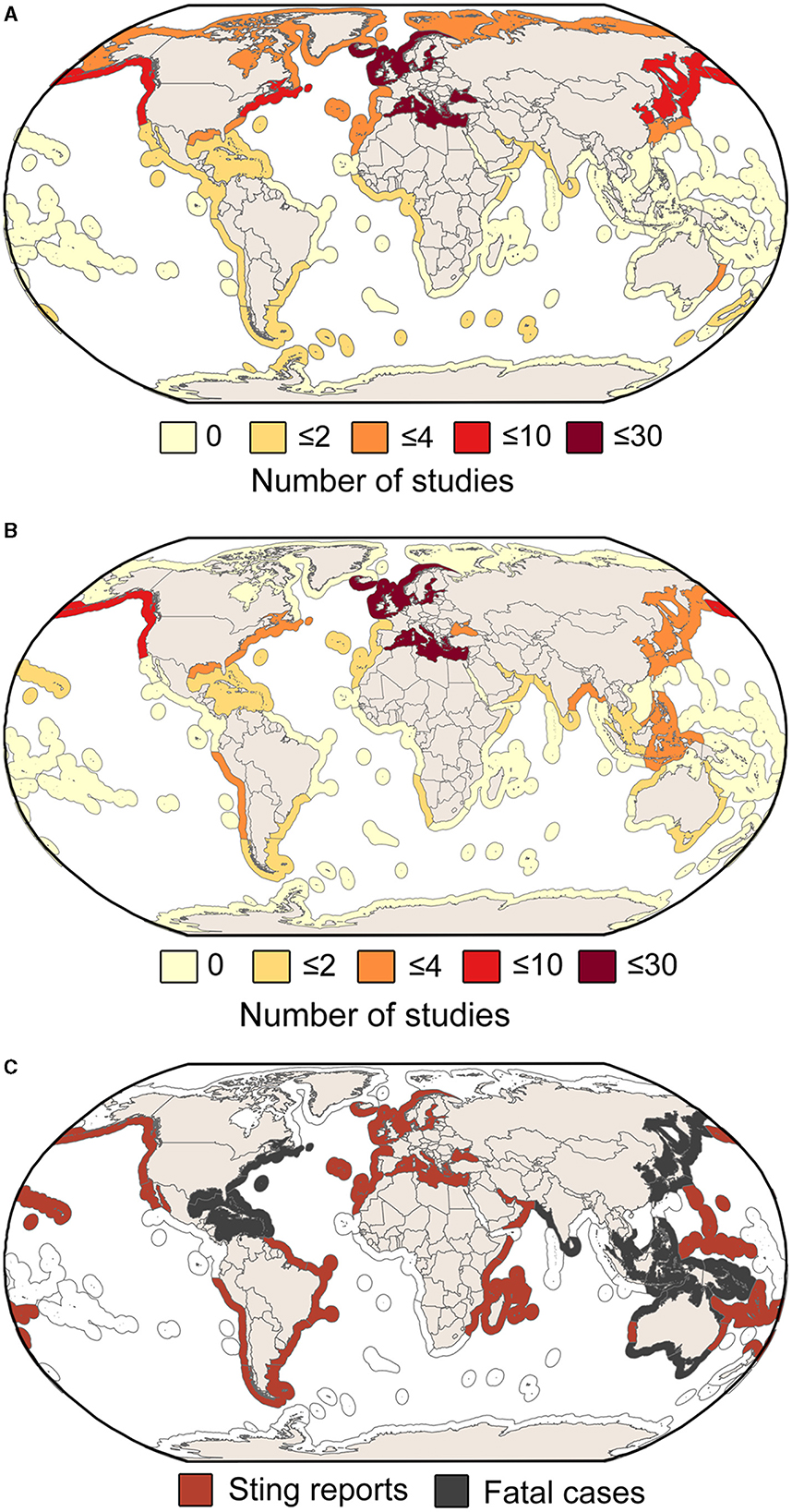
Figure 2. Spatial distribution of studies investigating gelatinous zooplankton impacts on (A) biodiversity and (B) ecosystem services. (C) Marine provinces where stinging events were reported from the retrieved studies and fatal cases.
Among the species frequently cited for negative impacts on biodiversity, Mnemiopsis leidyi (n = 28 articles), Aurelia aurita (n = 19), and P. noctiluca (n = 10) were the most prominent. For impacts on ecosystem services, the most cited species were also P. noctiluca (n = 15), A. aurita (n = 15), and M. leidyi (n = 6). In contrast, impacts on human health were mainly associated with P. physalis (n = 11), P. noctiluca (n = 9), Carukia spp. (n = 9), C. fleckeri (n = 6), and Rhopilema nomadica (n = 4). Fatal cases involved mostly box jellyfish, such as C. fleckeri (Currie and Jacups, 2005), but also scyphozoans, such as Nemopilema nomurai (Fenner and Williamson, 1996; Kim et al., 2018). A detailed enumeration of the reported species associated with different adverse impacts is included in Supplementary Table S3. It is important to note that some GZ taxa are formed by a complex of cryptic species, often misidentified and typically referred to as a single, most popular species (e.g., the moon jellyfish A. aurita; see Scorrano et al., 2017; Lawley et al., 2021; Moura et al., 2023).
Impacts on biodiversity were suggested to be caused through various mechanisms, such as direct predation (Yilmaz, 2015; Wang et al., 2020; Báez et al., 2022; Vineetha et al., 2022), modification of trophic flows (West et al., 2009b; Dinasquet et al., 2012b), competition for resources (Lynam et al., 2005; Báez et al., 2022), transmission of pathogens (Basso et al., 2019; Stabili et al., 2020, 2022), reduction of light penetration (Zaitsev, 1992; Stoner et al., 2014), behavioral changes of species in order to avoid GZ (Carr and Pitt, 2008; Chittenden et al., 2018), and envenomation (Helmholz et al., 2010) (Figure 3A). “Modification of trophic flows” (Dinasquet et al., 2012a; Tiselius and Møller, 2017) may occur through indirect predation (Schneider and Behrends, 1998; Dinasquet et al., 2012a; West et al., 2009a), accumulation of decaying organic matter (i.e., jelly-falls) (Tinta et al., 2012; Chelsky et al., 2016: Dunlop et al., 2018; Lebrato et al., 2019), and mucus and nutrient excretion altering abiotic parameters such as nutrients and oxygen concentrations (West et al., 2009b; Condon et al., 2011; Dinasquet et al., 2012b; Manzari et al., 2015; Marques et al., 2021).
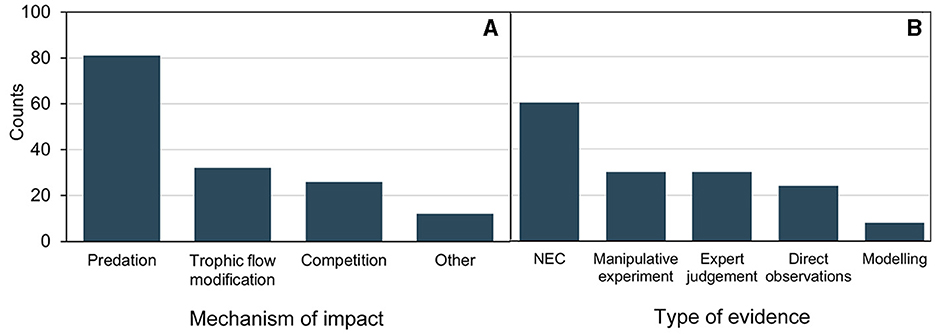
Figure 3. (A) Counts of the reported mechanisms of impacts of gelatinous zooplankton (GZ) on biodiversity. “Other” includes reduction of light penetration, disease transmission, envenomation, and behavioral changes to other species. (B) Counts of types of evidence for the reported impacts of GZ on biodiversity. “NEC” stands for non-experimental based correlations.
Most studies on GZ impacts on biodiversity drew conclusions primarily from non-experimental correlations (39%), followed by manipulative experiments and expert judgment (both 20%), direct observations (16%), and modeling (5%) (Figure 3B). Hence, the strength of evidence was low for a substantial portion of the reported impacts.
Stronger evidence provided by manipulative experiments demonstrated impacts on biodiversity through predation or modification of trophic flows (Sullivan and Gifford, 2004; West et al., 2009a; Dinasquet et al., 2012a,b; Pereira et al., 2014; Zoccarato et al., 2016; Wang et al., 2020). Aurelia aurita and M. leidyi were the most frequently studied species through manipulative experiments (7 and 6 studies respectively). Other mechanisms of impacts on biodiversity, such as competition were primarily reported through non-experimental correlations, modeling and expert judgment.
Concerning GZ effects on ecosystem services, manipulative experimental studies revealed impacts on farmed fish (Baxter et al., 2011b; Bosch-Belmar et al., 2016a,b; Powell et al., 2018) and alterations in nutrient cycling and carbon flow (Chelsky et al., 2016; Sweetman et al., 2016; Tinta et al., 2016). Additionally, studies based on direct observations addressed GZ impacts on fishing (Kim et al., 2012; Mianzan et al., 2014; Conley and Sutherland, 2015; Diciotti et al., 2016; Mghili et al., 2022), aquaculture (Ferguson et al., 2010; Marcos-López et al., 2016; Mitchell et al., 2021), and desalination and coastal power plants through clogging or ingress (Mianzan et al., 2014; Graham et al., 2014; Angel et al., 2016; Kumar et al., 2017).
According to the categories of the magnitude of the impact on biodiversity sensu Blackburn et al. (2014) (see Supplementary Table S2), “moderate” impacts inducing population declines in other species (e.g., though predation on copepods, pteropods, rotifers, cladocerans, chaetognaths, hydromedusae, or fish larvae and eggs) were prevalent (n = 77 reports) and followed by “minor” impacts (n = 43). “Major” impacts resulting in the local extinction of at least one prey species were less abundant (n = 21) and “massive” impacts have not been documented for GZ species. “Minimal” impacts were infrequently reported in the literature (n = 11), although this category may be under-represented, as non-significant results are less likely to be published (Jennions and Møller, 2002).
Probably one of the most notable examples of a “major” impact is the invasion of M. leidyi in the Black Sea in the mid-1980s, causing significant reductions in zooplankton abundances, primarily by predation (Shiganova, 1998), as documented for other invaded areas as well (e.g., Riisgård et al., 2012). This intense predation caused changes in the community structure of the Black Sea, with some species becoming virtually absent (Zaitsev, 1992; Shiganova, 2005). However, in the late 1990s, a new non-indigenous species (NIS) of ctenophore, Beroe ovata, was introduced in the Black Sea. This species preyed exclusively on GZ including M. leidyi, leading to reductions of M. leidyi population densities (Finenko et al., 2003). Since then, impacted species have been recovering (Finenko et al., 2003; Shiganova, 2005). The reduction of other ecosystem pressures, such as eutrophication and fishing pressure, along with changes in large-scale atmospheric conditions, also contributed to the changes observed in the Black Sea food web (Bilio and Niermann, 2004).
Therefore, GZ can have positive impacts on biodiversity: they are an important food source for various top predators and threatened species like sea turtles, birds and fish (Cardona et al., 2012; Jarman et al., 2013; Mianzan et al., 2014; Sato et al., 2015; Smith et al., 2016; Hays et al., 2018; Thiebot and McInnes, 2020; Jaspers et al., 2023) and some species can act as biological regulators of invasive species, such as the arrival of B. ovata in the Black Sea (Shiganova et al., 2001; Finenko et al., 2003; Bilio and Niermann, 2004). Additionally, certain GZ species, especially scyphomedusae provide shelter and trophic resources to juvenile fish, thereby improving their survival rates (Lynam and Brierley, 2007; Masuda et al., 2008; Mianzan et al., 2014; D'Ambra et al., 2015; Tilves et al., 2018). In some quite exceptional cases, GZ may also play a role in maintaining water quality and preventing dystrophic crises through a top-down control process (Pérez-Ruzafa et al., 2002, 2019; Fernández-Alías et al., 2022).
Furthermore, GZ can provide several societal ecosystem services (Doyle et al., 2014; Leone et al., 2015). Up to 35 species of jellyfish have been reported to be consumed by humans (Brotz et al., 2017). For millennia, GZ (mostly scyphomedusae) have been consumed in Asia, where they are considered delicacies (Omori and Nakano, 2001; Brotz and Pauly, 2017; Syazwan et al., 2020) and recently, there has been growing interest in Western countries considering jellyfish as a sustainable food resource (Brotz et al., 2017; Pedersen and Vilgis, 2019; Ramires et al., 2022a,b). Since the 1970s−80s there have been attempts to establish Stomolophus meleagris fisheries in the United States (Page, 2015), and in Mexico (Cruz-Colín et al., 2021), mainly where commercial fishing crises occurred due to overfishing of finfish and shrimps. However, the product was primarily exported to Asia, due to low consumer acceptance and neophobia in western countries (Torri et al., 2020), as well as its health- and cost-effective processing (Brotz, 2016; Raposo et al., 2022). In Europe, several species have the potential to support jellyfish fisheries (Brotz, 2016; Brotz et al., 2017; Bleve et al., 2019; Leone et al., 2019; Youssef et al., 2019; Edelist et al., 2021; Duarte et al., 2022; Raposo et al., 2022). The target species are particularly large-sized Rhizostomeae, with low stinging potential and recurring annual blooms, such as Rhizostoma pulmo and Rhizostoma octopus. In the absence of significant consumption, jellyfish have been labeled as “novel foods” under the current European regulation (European Union Regulation 2015/2283) and some innovative processing tests have already yielded new patented jellyfish-based foods that overcome the limitations of traditional Asian processes (Bleve et al., 2019, 2021; Leone et al., 2021; Ramires et al., 2022a,b). Jellyfish harvesting from by-catch of finfish fisheries may also offer novel resources to diverse industries and economic activities, as organic fertilizers (Hussein et al., 2015; Emadodin et al., 2020; Borchert et al., 2021), insecticides (Yu et al., 2005, 2014, 2016, 2021), animal feed for terrestrial or aquaculture farming (Miyajima et al., 2011; Wakabayashi et al., 2012; Liu et al., 2015; Duarte et al., 2022), or bait for fishermen (Mianzan et al., 2014).
GZ may also provide important biomaterial for medical applications and research (Ahn et al., 2018; Rastian et al., 2018; Widdowson et al., 2018; Felician et al., 2019). In the early 1900s, Charles Richet won the Nobel Prize in Medicine for his groundbreaking research on anaphylaxis, uncovered by studying P. physalis. From the serendipitous discovery of green fluorescent protein (GFP) in the hydromedusa Aequorea victoria (Shimomura et al., 1962), the biotechnological potential of cnidarians started to attract the attention of researchers for their well-documented ability to produce venoms (Turk and Kem, 2009). Other bioactive compounds obtained from various GZ species have been examined for their antioxidant, anticancer, antihypertensive, and antimicrobial properties, suggesting potential use in the pharmaceutical sector (Leone et al., 2013, 2015; Amreen Nisa et al., 2021; De Rinaldis et al., 2021; Ranasinghe et al., 2022; De Domenico et al., 2019, 2023). Furthermore, in the fields of biotechnology and biomedicine, GZ biomass has been explored for designing cell-scaffold devices to address non-healing skin wounds (Nudelman et al., 2019; Fernández-Cervantes et al., 2020).
Other GZ provisional services, include the contribution to the aquarium trade (Duarte et al., 2022), and more recently GZ material was proposed as a potential alternative for replacing fossil-based plastics (Steinberger et al., 2019). Moreover, it has been proposed that cnidarian GZ mucus might be used as bio-flocculation material for trapping and sequestrating plastic micro- and nanoparticles from contaminated waters of factories (Patwa et al., 2015; Lengar et al., 2021) and wastewater treatment plant effluents (Ben-David et al., 2023).
Further, pelagic tunicates, especially salps (Décima et al., 2023) and larvaceans (Jaspers et al., 2023) as well as cnidarian jelly-falls (Lebrato et al., 2019) have a significant capacity to fuel carbon sequestration, highlighting a crucial role amidst the ongoing climate crisis.
3.2 Potential pressures causing gelatinous zooplankton outbreaks and aggregations
Attributing GZ outbreaks to specific causes, whether natural or anthropogenic, is often challenging and accompanied by uncertainty (Lee et al., 2023). Increases in GZ populations appear often to be influenced by a combination of human activities, which might interact synergistically to trigger outbreaks of certain species (Richardson et al., 2009). A critical review by Pitt et al. (2018) contended that there was weak evidence that anthropogenic stressors trigger GZ outbreaks, because such claims were mostly based on two highly invasive (and often cryptic) taxa (A. aurita and M. leidyi) and relied on correlative investigations or circumstantial evidence that cannot establish causation (e.g., Duarte et al., 2015). However, the increase of human activities and uses of the marine ecosystems suggests, that regardless of “natural” global increases of GZ populations, their interactions with human activities are expected to increase, particularly in coastal waters (Gibbons and Richardson, 2013).
Several GZ have specific functional attributes that enable them to thrive in disturbed marine ecosystems and capitalize on ecological opportunities presented by anthropogenic activities. These include a broad diet (Purcell, 1992; Lilley et al., 2009; Fleming et al., 2015; Nagata et al., 2015), rapid growth rates (Marques et al., 2015; Jaspers et al., 2023), tolerance of harsh conditions (Purcell, 2012), or the ability to shrink and channel body carbon into reproduction during food-shortage to keeping up high reproduction rates (Lilley et al., 2014; Jaspers et al., 2015).
Various human-related causes of GZ outbreaks have been reported in the literature (Supplementary Table S4):
• Overfishing. The removal and decline of fish populations, which compete with certain carnivorous GZ for prey or predate on them, has allowed certain GZ taxa to exploit available resources more effectively and has led to abnormally large and long-lasting outbreaks of some jellyfish in certain areas (Lynam et al., 2006; Richardson et al., 2009; Boero, 2013). These declines may be reversible if new ecological components control these outbreaks or if the fishing pressure is reduced (Daskalov et al., 2007).
• Eutrophication. It has been hypothesized that excessive nutrients from fertilizer runoff and sewage into coastal waters, rich in nitrogen and phosphorus but poor in silica, promotes the dominance of non-siliceous phytoplankton, such as flagellates, and can create conditions that are more suitable for certain GZ than for fish to thrive. Various reasons have been suggested for this. Some GZ have the ability to feed on these protists directly (e.g., Parsons and Lalli, 2002; Richardson et al., 2009); further, GZ are reported to be more competitive than other metazoans including finfish to thrive under hypoxic conditions, that often occur in eutrophic environments (e.g., Purcell et al., 2001; Breitburg et al., 2003; Purcell, 2012).
• Climate change, with its associated sea surface warming, altered water column stratification, and increased climate variability, can also influence GZ population dynamics (Boero et al., 2016; Jaspers et al., 2023; Lee et al., 2023). Increased sea surface temperatures can create more favorable conditions for GZ by favoring their prey abundances (Jaspers et al., 2023) and/or accelerating their growth (Purcell, 2005). Fernández-Alías et al. (2021) showed that large species living in temperate, shallow waters appear to have a high outbreak potential, with temperature appearing to be the main environmental factor regulating the onset of population outbreaks, and food availability, enhanced by bottom-up eutrophication, being key to maintaining large biomass. Furthermore, the expansion of venomous tropical jellyfish species to subtropical and temperate latitudes due to warming, poses potential threats to the colonized ecosystems and local economies.
• Species translocation. The human-assisted movement of species in new marine regions through ballast water exchange, fouling on ship hulls, aquaculture, and the opening of corridors connecting previously isolated seas (such as the Suez Canal) has translocated many outbreak forming GZ species such as the hydrozoans Blackfordia virginica (Marques et al., 2017), Maeotias marginata, Nemopsis bachei (Nowaczyk et al., 2016), or Gonionemus vertens (Marchessaux et al., 2017) which can reach remarkably high abundances and cause significant ecosystem impacts on invaded habitats. Although we are still far from understanding the true number of NIS of gelatinous zooplankton, especially small sized hydrozoan jellyfish species, examples of non-indigenous GZ in European waters include R. nomadica (Galil et al., 1990; Spanier and Galil, 1991; Deidun et al., 2011) and Cassiopea andromeda (Mammone et al., 2021; Cillari et al., 2022) in the Mediterranean Sea, G. vertens in the northern European coasts (Marchessaux et al., 2017) as well as multiple independent invasions of M. leidyi into other European seas (Jaspers et al., 2018b, 2021).
• Habitat modification, such as an increase in suitable benthic habitat, either natural or artificial (ocean sprawl), could contribute to the proliferation of jellyfish polyps by providing additional substrates for polyp attachment and growth (Duarte et al., 2013).
3.3 Management measures and strategies
Despite the numerous and heterogeneous known impacts of GZ outbreaks and the studies dedicated to identifying their triggering causes, current GZ management strategies are based on reactive strategies that primarily focus on controlling and mitigating the adverse impacts caused by cnidarian jellyfish populations at local scales (Lucas et al., 2014; Dong, 2019). Their main objective is to reduce health risks and loss of revenue for the affected coastal and marine activities (Ghermandi et al., 2015). Some of the control methods that are used nowadays are enumerated below.
Jellyfish cutters are used in Japan and Korea to remove aggregated jellyfish like Nemopilema spp. or Aurelia spp. (Kim et al., 2013; Lucas et al., 2014). However, cutting and/or grinding jellyfish do not affect their distribution at sub-surface depths and do not consider the powerful regenerative property of cnidarians such as Aurelia coerulea, able to produce new polyps even by few cell debris (He et al., 2015). Jellyfish-excluding devices for towed fishing gears (Matsushita and Honda, 2006) have been developed to prevent GZ from entering nets, reducing their bycatch and adverse impacts on fisheries. First adopted in Australia to protect beachgoers against envenomation from lethal cubozoans, anti-jellyfish nets are nowadays broadly used throughout the Mediterranean Sea (Piraino et al., 2016) to create enclosed areas for safe swimming and maintain tourism appeal (Ruiz-Frau, 2023). The effectiveness of these nets demands specific design, material and installation procedures (mostly standardized by patents) as well as monitoring and maintenance services, even requiring rapid intervention to remove the nets when required. Other solutions like protective covers, mesh screens, and bubble screens are used in the aquaculture industry to safeguard fish production, and in power stations and desalination plants to protect the cooling and pumping systems from GZ infestations (Verner, 1984; Ratcliff, 2004; Lucas et al., 2014; Haberlin et al., 2021).
Chemical compounds are also used for antifouling ship paints to inhibit polyp settlement and attachment in aquaculture facilities and other artificial structures (Guenther et al., 2009, 2010; Feng et al., 2017, 2022). Introducing natural polyp predators, like nudibranchs, to habitat areas can also help control some GZ populations with a benthic life-stage such as most cnidarian jellyfish species (Hernroth and Gröndahl, 1985; Hoover et al., 2012). Here, recruitment is reduced and thereby the frequency and intensity of population outbreaks.
Operational early warning systems (EWS) are being developed to inform coastal users about potential GZ presence or predict the outbreak probability or intensity for certain noxious species. EWS may be based on hydrodynamic models, real-time observations, remote sensing techniques and/or unmanned aerial vehicles (UAVs) allied with high resolution imagery and effective image analysis algorithms or deep learning technology (Aznar et al., 2017; Mcilwaine and Casado, 2021; Edelist et al., 2022; Han et al., 2022; Zhang et al., 2023). EWS may also benefit from recent scientific advancements on environmental DNA (eDNA) for detecting rare but life-threatening species (Bolte et al., 2021). EWS are currently employed to detect outbreaks of GZ species such as N. nomurai (Uye, 2008; Lucas et al., 2014) Cyanea purpurea, R. pulmo, Phacellophora camtschatica, Agalma okeni, A. aurita, Phyllorhiza punctata, and Rhopilema esculentum (Gao et al., 2023).
Public education and awareness (i.e., ocean literacy) on different GZ groups and their associated risks can also play a significant role in mitigating the impacts of GZ outbreaks on public health and tourism (Gershwin et al., 2010; Lucas et al., 2014). In addition, collaborative citizen science approaches (Boero, 2013) involving trained personnel, volunteers, social networks, and media may contribute to gather data on GZ and enhance public engagement (e.g., Pikesley et al., 2014; Gatt et al., 2018; Marambio et al., 2021; Dobson et al., 2023; Levy et al., 2024).
Ecosystem-based strategies including different GZ taxa are uncommon, even though integrating the multifaceted linkages between GZ, human activities and other ecosystem components may help to prevent or reduce outbreaks of harmful GZ species (Lynam et al., 2006; Richardson et al., 2009; Brodeur et al., 2016; Bastardie et al., 2021; Edelist et al., 2021). This is evidenced by the example of the Benguela upwelling region, where historically similar ecosystems (in terms of their structure, species communities, and functions) followed different trajectories after the collapse of small pelagic fish stocks in the 1960/70s. In the northern Benguela system (Namibia), the prolonged absence of fishing regulations after the collapse of stocks, combined with changing environmental conditions, led to the domination of GZ in the mid-trophic level and the depletion of small pelagic fish abundance. In contrast, in the southern Benguela system (South Africa), catch limits and management measures for small pelagic fishes prevented overfishing and succeeded to maintain their ecosystem dominance (Roux et al., 2013).
3.4 Indicators to include GZ in the MSFD's assessments
The MSFD is an ecosystem-based management approach adopted by the European Commission to attain GES across European Seas. In practice, GES is assessed through eleven thematic descriptors and associated criteria related to different ecosystem components (state) and pressures (European Commission, 2017). European Union (EU) member states or Regional Sea Conventions are required to determine indicators and associated thresholds consistent with GES achievement in their marine reporting units. Moreover, the 6-yearly reports must assess the cumulative effects of pressures and social and economic costs of environmental degradation (Tornero Alvarez et al., 2023).
GZ have been considered minimally in the 2012 and 2018 reporting cycles, even though GZ are frequently regarded as sentinels of marine ecosystem health (Schrope, 2012; Lee et al., 2023), offering diagnostic insights to interpret changes across the food web, including higher and lower trophic levels (Bedford et al., 2018). To introduce GZ component in MSFD, an initial assessment would be required to (i) define appropriate indicators and associated thresholds for different GZ taxa and areas, (ii) differentiate between anthropogenic and natural factors driving GZ outbreaks and aggregations (“pressures”), (iii) identify impacts and services of GZ in the ecosystem, and (iv) devise relevant management actions to mitigate/prevent their harmful effects where practical. Such analysis is pivotal for designing and implementing effective monitoring programs that aid GZ-related assessments and establish a robust scientific foundation for crafting efficient management strategies to attain GES. In this holistic approach, various MSFD descriptors (D) and criteria may be of relevance to assess GZ state, their pressures and impacts (see Supplementary Table S5).
The MSFD indicators should include, as a minimum, a measure of the ecological state of an ecosystem component to evaluate change over time (e.g., abundance or biomass of different GZ groups, or the frequency of occurrence of their aggregations). In addition, to understand the changes on GZ populations in the ecosystem, indicators related to the relevant natural and anthropogenic pressures are required (Ndah et al., 2022). Many pressures are already captured in MSFD and regional biodiversity assessments, including temperature increase due to climate change, fishing effort, seabed and hydrological changes, nutrient and contaminant levels and change in the base of the food web through primary production metrics. However, indicators of direct pressure(s) favoring GZ (e.g., provision of artificial settling habitat for polyp stages of cnidarian jellyfish; Duarte et al., 2013) should be included to develop efficient mitigation measures and identify risk of expansion (Foster et al., 2016). Similarly, a measure of GZ as a pressure and their impacts on the ecosystem (e.g., losses in fisheries, aquaculture, or energy generation) would be useful to inform managers of the scale of their effects (Abdul Azis et al., 2000; Doyle et al., 2008; Uye, 2008; Quiñones et al., 2013; Ghermandi et al., 2015; Kennerley et al., 2022).
A few indicators including GZ have been considered in current European biodiversity assessments: the Oslo/Paris Convention's Intermediate Assessment (OSPAR, 2017; Holland et al., 2023a), the Helsinki Commission's coreset of indicators (HELCOM, 2018) and the Black Sea Commission's report on the “State of Gelatinous Plankton” (BSC, 2019) were delivered to assist contracting parties when reporting to the MSFD. While OSPAR, BSC and HELCOM have each developed indicators for NIS, only the BSC mentions GZ explicitly. However, HELCOM acknowledged that GZ were an important group missing from the indicator: “Trends in arrival of new non-indigenous species” (HELCOM, 2018).
Recently, specific metrics for GZ have been proposed by OSPAR as part of the indicator “Changes in Phytoplankton and Zooplankton Communities” (Holland et al., 2023a; Magliozzi et al., 2023). This indicator is used within MSFD descriptors on pelagic habitats (for D1) and food webs (D4) and adopts the Phytoplankton Community Index approach of Tett et al. (2008). This indicator relies on the concept of “lifeforms” or multiple unrelated taxa that are considered to share a similar functional role within their ecosystem (e.g., primary producers, grazers or carnivores). Once the abundance or biomass of the lifeform groupings are determined from sample data, the ratios of specific pairs of lifeforms are used to evaluate the energy or mass flow through trophic pathways in marine food webs (McQuatters-Gollop et al., 2019; Holland et al., 2023a,b). For GZ, two lifeform pairs indices are considered: (1) GZ vs. fish larvae/eggs and (2) Crustaceans vs. GZ (Supplementary Figure S5). In each case, GZ are considered as a predator (of crustacean plankton and of fish eggs and larvae) directing energy away from fish populations. However, GZ outbreaks may also result from (rather than cause) ecosystem degradation, and metrics of GZ abundance have been proposed as a potential indicator of ecosystem instability (Lynam et al., 2011).
Unfortunately, these assessments are still supported by very little monitoring data on GZ, with all species of Cnidaria and Ctenophora currently grouped together as “Gelatinous zooplankton.” Data for GZ were available to OSPAR (2017) from a single sampling site within the western Channel (“L4,” Atkinson et al., 2021) and was improved for OSPARs Quality Status Report 2023 (Holland et al., 2023a), with an additional station off north-western Scotland (Loch Ewe) (see Supplementary Figure S5), a station off eastern Scotland (Stonehaven), and Swedish data for the Kattegat and Norwegian Trench in the eastern North Sea. However, these GZ data were insufficient to support additional analyses to determine key environmental pressures (Holland et al., 2023a,b).
In light of the aforementioned considerations and in anticipation of forthcoming assessments, we consider the following to be potentially useful indicators related to GZ:
Pressure indicators driving change in GZ:
• Indicators of water-mass dynamics (e.g., Ndah et al., 2022).
• Provision of artificial habitat (e.g., Duarte et al., 2013; Foster et al., 2016).
• Sea surface temperature and eutrophication (in shallow coastal waters) (Fernández-Alías et al., 2021).
• Potential change of state indicators for GZ:
• Estimation of episodic and/or seasonal GZ outbreaks and aggregations as an early warning indicator of climate effects on the marine environment (Van Walraven et al., 2013, 2015).
• Frequency of occurrence of GZ in stomach contents samples of predators (e.g., Smith et al., 2016).
• Polyp presence and abundance in coastal habitats (lagoons, marinas) (e.g., Van Walraven et al., 2016).
Impact indicators due to GZ outbreaks:
• Frequency of occurrence of GZ supporting foraging grounds of dependent predators like leatherback turtles (Houghton et al., 2006).
• Economic losses in fisheries (e.g., Uye, 2008; Quiñones et al., 2013), aquaculture (e.g., Doyle et al., 2008), and coastal desalination or energy installations (e.g., Abdul Azis et al., 2000).
• Social impact indicators, such as number of bathers requiring medical attention due to jellyfish stings (De Donno et al., 2014), or beach closures and loss of tourism (Ghermandi et al., 2015; Kennerley et al., 2022).
The precise selection and definition of GZ-related indicators including their specific metrics, variables and threshold values, remains a pending and challenging exercise which must consider practical aspects, such as feasible/required sampling and analysis capabilities, temporal, spatial, and taxonomic resolutions of underlying data, capacity to reflect pressures-state-impact linkages, inter-indicator connections (Dale and Beyeler, 2001; Niemeijer and De Groot, 2008; Marques et al., 2009; Magliozzi et al., 2023), accumulated uncertainties (Racault et al., 2014), and the potential for pan-European intercomparison and harmonization (European Commission, 2017; Magliozzi et al., 2023). These requisites will heavily rely on the monitoring programs and techniques to be implemented to support the assessment process.
3.5 Current monitoring programs and techniques and new alternatives
Presently there is a growing demand for cost-effective and innovative monitoring approaches to improve research on GZ and integrate them into the MSFD assessment and management framework (Magliozzi et al., 2021, 2023). Technological advancements have introduced new techniques for monitoring GZ outbreaks and aggregations, including sampling approaches more suitable to study these fragile animals.
The findings of our systematic review on monitoring programs and methodologies for GZ are described hereafter.
• Nets: WP2 and Bongo nets are the most widely used GZ monitoring tools. They are particularly suited for small, abundant hydromedusae, scyphozoan ephyra and calycophoran siphonophores. Different sampling gears provide complementary insights in GZ populations studies (Hosia et al., 2008; Purcell, 2009). Fish trawl nets are also employed, mainly for sampling larger and more robust gelatinous species (Purcell, 2009). In Europe, night-time ichthyoplankton work conducted during fisheries trawl surveys have been proposed as a cost-saving approach to support GZ monitoring (Aubert et al., 2018). Here, ichthyoplankton sampling gear such as MIK-nets can quantitatively assess the gelatinous macrozooplankton community (Aubert et al., 2018; Køhler et al., 2022). However, these depth integrated nets have the disadvantage of underestimating fragile gelatinous organisms that may break during collection. In addition, true abundances may be underestimated if species are present at very low abundances and low water volumes are processed. Other nets such as MOCNESS or MultiNets may be alternatives for sampling discrete depth strata where GZ are known to accumulate (e.g., Haraldsson et al., 2013).
• The Continuous Plankton Recorder (CPR) is a continuous surface monitoring method (Lynam et al., 2011) that can detect outbreaks of both meroplanktonic and holoplanktonic hydrozoans and scyphozoans. For instance, outbreaks of P. noctiluca, recorded by the CPR off Ireland in October 2007, were confirmed by net tows and visual examination (Baxter et al., 2010; Licandro et al., 2010), suggesting that CPR can provide reliable information for identifying regions and periods favorable for GZ outbreaks. The main limitations of this technique are the inability to sample complete specimens of GZ larger than the aperture of the CPR which is only a few centimeters in size, and the difficulties to preserve the GZ morphology, except for rigid calycophoran siphonophores (Gibbons and Richardson, 2009), impairing their taxonomic identification at species level. However, preserved samples can be used for re-analysis and genetic studies (Kirby et al., 2006; Licandro et al., 2010). CPR devices can be mounted on ships of opportunity, enabling periodic surveys covering extensive spatial and temporal scales.
• Polyp monitoring: Polyp monitoring ranks as the third most frequently reported monitoring method. Despite their crucial role in cnidarians outbreaks, polyps remain the least known stage in the cnidarians' life cycle, and field investigations of this stage have only recently gained attention. Among Scyphozoa and Cubozoa species (the most conspicuous GZ), 5% are holopelagic, 32% have a benthic stage, whilst the life cycle of the remaining 63% is unknown (estimated from Jarms and Morandini, 2019).
• We observed that polyp species detection in nature is not in accordance with increasing sampling efforts. In only 18.6% of species with benthic stage, polyps have been observed in the natural environment (i.e., 16 of 86 species), with the genus Aurelia and Chrysaora accounting for almost the half (44%) of these observations (Cargo and Schultz, 1966; Hartwick, 1991; Kikinger, 1992; Dawson et al., 2001 and references in Supplementary Table S6 for reports after 2008). Polyp monitoring and research efforts encompass density estimations, ephyrae production, and the identification of suitable substrates (e.g., Miyake et al., 2002; Van Walraven et al., 2016). In the few occasions when polyps have been observed, monitoring of this benthic stage is usually carried out through visual surveys by SCUBA divers or by employing underwater cameras for recording (Supplementary Table S6).
• Visual counts: Although GZ monitoring based on visual observations from ships ranks as the fourth most frequently reported method in the review, this approach is inherently biased toward species of detectable size and relatively straightforward taxonomic identification present on the surface during daytime. Monthly visual surveys from boats have been successfully implemented to monitor five jellyfish species for several years in Irish/UK waters, showing where aggregations tend to occur (Bastian et al., 2011; Purcell, 2012). Additionally, for certain remarkable (dangerous or visually striking) species, visual counts from ferries (Yoon et al., 2018), or boats used for beach surveillance, cleanup, or touristic activities (such as whale watching, birdwatching, and coastal tours) can serve as early warning systems to manage bathing and/or fishing areas and support educational initiatives respectively. In recent years, visual counts have gradually been substituted by aerial and underwater imagery and videos. Visual counts are also sometimes used to ground truth other data collected through other monitoring techniques.
• Acoustic methods: Underwater acoustic devices like single-beam and multibeam echosounders, scanning sonars and, hydrophones, have been used in several studies for detecting GZ presence, tracking their movements and vertical diel migrations, and estimating their abundance in the water column (e.g., Han and Uye, 2009). In the past, the use of acoustic systems to detect GZ was disregarded because of their high-water content, resulting in a very low-density contrast at the water–body interface. However, several studies have demonstrated that different species of gelatinous plankton can generate significant levels of sound scattering (Brierley et al., 2005) even at low sound frequencies (38–50 kHz; Colombo et al., 2003). These methods enable faster and broader coverage surveys (including the water column and nighttime), providing continuous count data along transects and accompanying environmental data. Moreover, the acoustic characterization of GZ aggregations from previous recorded acoustic cruises for fish abundance assessment have proved to be valuable in identifying and reconstructing historical scenarios of their abundance and their potential impact on ecosystems (Colombo et al., 2003). However, implementing these methods requires substantial efforts to ground truth species identifications and density estimates, staff training, labor-intensive data processing as well as equipment investment. Acoustic equipment can be mounted on fixed mooring platforms (e.g., for monitoring pumping facilities) on board scientific vessels, or in UAVs and remotely operated vehicles (ROVs).
• Aerial remote images: Satellite imagery, aerial photography, and video recording from piloted aircraft or drones are increasingly employed for GZ monitoring. Whereas drones, UAVs, and ROVs may include optical sensors with sufficient resolution for GZ detection, aerial and satellite platforms should be equipped with very high resolution or hyperspectral sensors to support GZ monitoring. However, even though this seems to be a straightforward approach, sea state, cloud cover and data processing are major obstacles. Moreover, customized signal processing algorithms must be developed to enable the detection and/or counting of GZ aggregations from the acquired imagery (Raoult and Gaston, 2018; Schaub et al., 2018), e.g., JellyX and JellyNet (Mcilwaine and Casado, 2021). Satellite data from multispectral and infrared sensors are often used to provide environmental data that can be incorporated into GZ prediction models, habitat suitability maps, and early warning systems. An example of this approach can be seen in the multi-platform study of the extreme outbreak of the barrel jellyfish R. pulmo in the Gulf of Trieste in April 2021 (Reyes Suárez et al., 2022).
• UAVs and drone platforms allow the collection of larger datasets in less time than those acquired during boat-based surveys and can also monitor species that are delicate to sample with nets. However, this method requires a sound knowledge of the species present from net-based surveys, as images are often not good enough to allow for species identification. Also, UAVs access is limited, and deployments are costly. Drones could facilitate high spatial coverage (Hamel et al., 2021), however, their usage is constrained by factors such as flight duration, local flight operation regulations, and environmental conditions such as rain and wind speed (Mcilwaine et al., 2022). In addition, remote image quality can degrade due to foggy conditions, sun-glint, or high-water turbidity (Hamel et al., 2021).
• Other remote sensing methods such as airborne LiDAR have been used to describe the vertical distribution of GZ in the water column (Churnside et al., 2016). However, it is important to keep in mind that some GZ actively modify their position within the water column. For the ctenophore M. leidyi it has been shown that sea state and turbulence conditions impact their vertical position, where animals avoid surface waters during high wind speeds (Jaspers et al., 2018b). Furthermore, electronic tags have shown that the large scyphozoan jellyfish R. octopus spent < 10% of their time at the surface (Hays et al., 2012). This data was then used by Elliott et al. (2017) to apply a correction factor to their aerial surface estimates of R. octopus abundance.
• Citizen science: Active participation from the public in collecting GZ data offers a valuable opportunity to cover larger coastal areas that would be costly or impractical to cover through scientific projects (Marambio et al., 2021; Edelist et al., 2022; Gueroun et al., 2022). However, this information must be verified by experts or requires prior training of the participating volunteers to ensure the quality required for scientific studies, putting a high maintenance demand on this kind of data generation. In addition, data collection often suffers from spatial and temporal bias, as more data is obtained from popular sites during high season for easily detectable species. Therefore, it is advisable to include local communities such as schools, diving or sailing clubs into data acquisition programs and to not restrict these programs to summer seasons and short-term projects.
• Underwater images and automatic count systems: Underwater photography and video recording systems (Cillari et al., 2022) acquired by scuba divers (Gibbons et al., 2021) or by underwater platforms like ROVs, AUVs or underwater video profilers (UVPs) (Biard et al., 2016), can facilitate quantitative evaluations from long-lasting and spatially extensive surveys. However, they can bias local fauna's behavior by causing species to escape. In contrast, static systems that are quickly accepted by resident fauna can collect information over longer periods, albeit with lower spatial coverage than mobile systems. Utilizing camera systems in conjunction with computer vision algorithms enables real-time detection and counting of GZ, reducing observer bias and enhancing monitoring efficiency (Gao et al., 2023). Furthermore, specialized bathyphotometer cameras have been employed for the observation and analysis of bioluminescence signals in salps (Melnik et al., 2022).
• Molecular genetic methods: The advancement of DNA barcoding, mitochondrial and nuclear DNA and RNA facilitate species detection, including GZ (Créach et al., 2022). Quantitative real-time PCR (qPCR) has also been used to identify GZ by tracing eDNA (Bayha and Graham, 2009; Marques et al., 2019). This emerging method enables the analysis of water and sediment samples to detect extracellular DNA or associated to dead cells (Torti et al., 2015; Minamoto et al., 2017; Ogata et al., 2021). Molecular techniques are limited to detecting the presence or absence of taxa and cannot estimate abundance variability. However, they increase the likelihood of species detection and, in some cases, reduce the time and costs compared to other monitoring and sampling methods. Moreover, marine eDNA is preserved for only 1 day in water, whereas it can persist for at least 1 year in sediments and could therefore be useful to reconstruct past occurrences (Ogata et al., 2021). Furthermore, these techniques can be applied to analyze the gut content of potential GZ predators, contributing to food web characterization (Smith et al., 2016).
• Jelly-falls monitoring: Elevated gelatinous biomass may translate into increased transfer of this organic material to the seafloor, providing a food supply to benthic fauna. Monitoring the presence and fate of GZ carcasses has been conducted using various techniques, including sediment traps, photography and video systems, and trawling nets (e.g., Lalande and Fortier, 2011; Sweetman and Chapman, 2011, 2015; Lebrato et al., 2012; Dunlop et al., 2018).
Most GZ monitoring publications were found in Europe, Asia, and the United States (Supplementary Figure S6). Nets were the predominant monitoring method in all Marine Realms (Figure 4), except for the Eastern Indo-Pacific, where citizen science was the only method recorded. Most studies focused on cnidarians and used nets, underwater images, acoustics, and visual counts. Remote images, molecular methods, and jelly-falls have been implemented only for Cnidaria and Tunicata (Figure 5). The number of studies monitoring both the GZ pelagic and benthic stages increased from 2008 to 2022. There was a rise in the number of citizen science publications in 2021–2022 (Supplementary Figure S7).
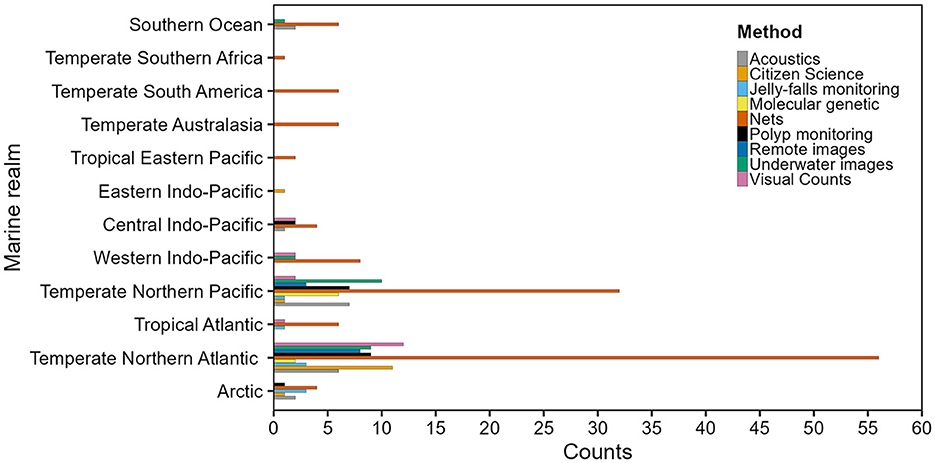
Figure 4. Types of monitoring methods used to monitor gelatinous zooplankton outbreaks and polyps (benthic stage of Scyphozoa and Cubozoa) across each Marine Realm, published between 2008–2023.
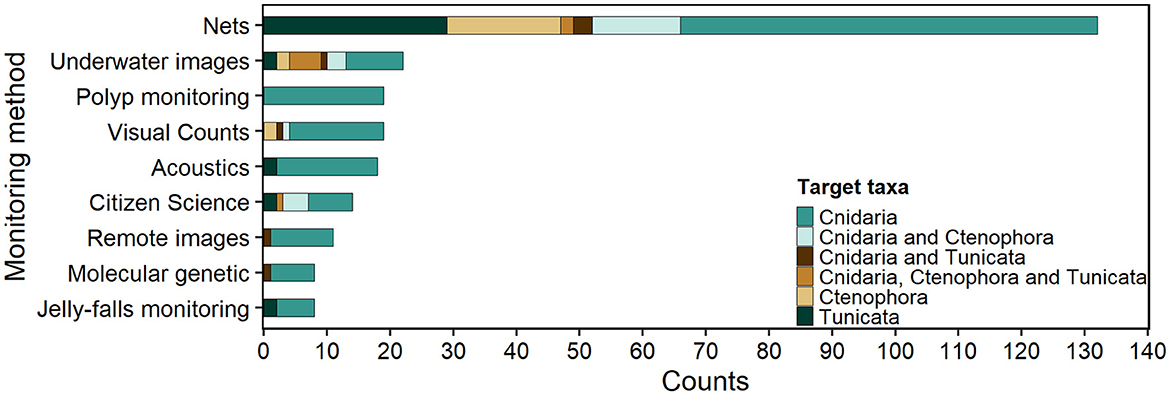
Figure 5. Gelatinous zooplankton and polyp (benthic stage of Scyphozoa and Cubozoa) monitoring studies sorted by target taxa based on the methodology used, published between 2008–2023.
Articles focusing on polyp monitoring are relatively scarce (n = 19). The methods used are summarized in Supplementary Table S6. Among the species considered, 64% belonged to the genus Aurelia (e.g., Aurelia sp., A. aurita, A. coerulea, and A. labiata), 9% to the genus Chrysaora (C. pacifica and C. hysoscella), and the remaining 27% included four Scyphozoa species (Atolla sp., Atorella sibogae, Cyanea lamarkii, Nausithoe cf. rubra, and N. nomurai) and one Cubozoa (Copula sivicksi). Polyps were found in both natural substrates (shells of clams Spisula subtruncata and Mactra stultorum, shells of dead clams, hollows of stones, under-surfaces of oysters growing on port pillars, biogenic reefs formed by polychaeta, hidden within the coral substratum, or on barnacles, bivalves, tunicates, sponges, and bryozoan) and artificial substrates (undersides of floating piers, PVC, synthetic rubber, iron oxide, wood, granite, glass, floating docks, and plastic debris).
In several publications, data from direct GZ monitoring techniques (e.g., counting methods and citizen science) have been combined with environmental information (e.g., currents direction, temperature, and salinity) to develop ecological models, EWS, or forecast of GZ trajectories (Ferrer et al., 2015; Ferrer and González, 2021). Ecological modeling approaches used to predict outbreaks and spatial occurrence patterns include trait-based models, mixed models, and ecosystem-functional models (Lamb et al., 2019; Rahi et al., 2020; Ramondenc et al., 2020; Bosch-Belmar et al., 2021a).
4 Discussion: recommendations to move forward
Several key recommendations stem from the present multifaceted review.
4.1 Data representativeness and knowledge bias
The results of the systematic reviews demonstrate a pronounced prevalence of studies conducted in northern Atlantic regions, focusing on Cnidaria, and employing nets as primary monitoring technique. This highlights the need to diversify GZ studies, to publish additional research on non-cnidarian GZ taxa such as ctenophores and tunicates, to diversify monitoring methods, and to include underrepresented marine regions in the Southern Hemisphere.
4.2 Further dedicated and sustained monitoring of GZ is required to support research and management
Despite their important ecological and socio-economic roles in marine ecosystems, monitoring programs focused on GZ are scarce. Currently, there are no coordinated European efforts to monitor GZ, unlike the ICES fishery surveys (e.g., coordinated trawl and beam trawl surveys), or GZ defined monitoring requirements under other EU Directives (MSFD, CFP). Many GZ surveys are dependent on researchers “piggy backing” onboard during fisheries surveys to record “jellyfish bycatch”. Certain GZ surveys rely on temporary national or EU funding (such as INTERREG or H2020), which limits their usefulness for assessing changes in GZ populations and distribution. Other monitoring efforts and funding are focused on harmful or noxious GZ species (e.g., P. noctiluca research in the Mediterranean in the 1980s) and dry up once abundances return to normal (Boero, 2013). As such, the abundance and distribution of GZ tends to be monitored haphazardly, using an uncoordinated approach.
Often, the implementation of new marine monitoring programs is hindered by their high costs. However, when considering the total costs of environmental management, from monitoring to management programs, monitoring costs constitute only a small proportion that becomes even smaller when factoring in the benefits achieved through efficient management (Nygård et al., 2016). Therefore, one of the most salient recommendations from this exercise is that the GZ research community across Europe needs to work together to design a European-wide monitoring framework supported by European bodies, funding mechanisms and institutes.
In this context, the coordination and GZ-dedicated extensions of existing monitoring programs supporting the Fisheries Data Policy, the Bathing Water Directive, the Water Framework Directive, the Integrated Coastal Zone Management (ICZM) and other monitoring facilities like long term ecological research (LTER) sites or the monitoring of pipelines and aquaculture sites, could help to enhance the coverage and resolution of data and rationalize costs. However, it is important not to forget that to accurately assess GZ, dedicated surveys would require adapted spatial and temporal extensions and resolutions, and adequate sampling gears and analysis methods.
4.3 Understanding the onset of GZ outbreaks and the role of the polyp phase
The limited understanding of the interplay between the diversity of pressures that can lead to different GZ species outbreaks, and their complex interaction mechanisms (i.e., additive, synergistic, or antagonistic), highlights the need for further research to comprehend the connections between human-induced stressors with GZ outbreaks.
This entails studying a range of different GZ functional groups, conducting experiments, considering ecosystem dynamics and employing multidisciplinary approaches to detect and quantify factors driving GZ outbreaks: traditional net-based methods and complementary technologies such as automated underwater imaging systems, artificial intelligence-based species detection and calibrated multibeam acoustics can be useful for species detection, abundance estimations, and inference of their interactions and spatial heterogeneity. Molecular techniques can assist in the early detection of potential NIS and problematic species. Remote sensing and modeling activities can cover large spatial scales and upscale local results to a European and global perspective. Standardized global monitoring through citizen science can enhance the availability of in-situ observations and increase public awareness of GZ-related issues.
Furthermore, the study of cnidarians' early phases, such as polyps and the first pelagic stages (i.e., ephyrae), is crucial to understand their recruitment and populations dynamics (Kingsford et al., 2000). Many factors determine the timing and magnitude of scypho- and cubomedusae recruitment, such as the polyps' abundance, budding and strobilation rates, and ephyrae survival rates (Pitt and Kingsford, 2003). However, polyps are elusive and efforts to monitor these pivotal stages are still scarce (19 peer-reviewed articles found in the last 16 years). Establishing in-situ sampling programs to identify the locations, abundance, and spatial extent of polyps, as well as estimating asexual reproduction, mortality, and growth rates, both in-situ and in laboratory experiments, would provide insight into the environmental factors that regulate jellyfish outbreak dynamics.
4.4 Integration of GZ in the MSFD framework
Integrating GZ into the MSFD framework involves starting to define baselines, indicators and thresholds for status, pressures and impacts of relevant GZ taxa across the different European marine and coastal areas. In this challenging context, coordination and complementation of experts, data, methods, and funding are key to achieving consistency and governance efficiency.
GZ can be considered in different descriptors, especially D1 (biodiversity), D2 (NIS), D4 (food-webs), and D5 (eutrophication). Defining relevant indicators and clear thresholds will help to identify when GZ populations exceed natural variability and become a concern for ecosystem health and marine ecosystem services. Moreover, differentiating between anthropogenic and natural factors driving outbreaks is crucial for ensuring that interventions address the manageable causes of GZ outbreaks. Due to the unpredictable and dynamic nature of GZ outbreaks and the current knowledge limitations, it is recommended to implement adaptive management through reassessment of the effectiveness of monitoring and management measures.
4.5 Public awareness, education and engagement
By informing the public about GZ species, risks, and safety/mitigating measures, safer behaviors and proactive preparations can be encouraged, leading to a more supportive and informed community. Therefore, ocean literacy and citizen science can be powerful tools to reduce the impact of GZ outbreaks on public health, tourism, fisheries, and marine facilities. These initiatives not only provide valuable insights for scientists and managers but also foster a sense of stewardship among the public, making them active participants in observing, reporting, and managing GZ populations and marine health.
4.6 GZ and sustainable blue economy
Finally, GZ provides numerous ecosystem services and potentially new valuable marine resources. However, the exploitation of certain GZ taxa -especially NIS- should undergo scientific safety assessments, and address sustainability issues for massive commercial harvesting. In addition, potential impacts on pelagic food webs, including outbreaks of other non-target GZ or harvesting in sensitive ecological areas such as the leatherback turtle hotspots (Houghton et al., 2006) should be carefully considered before providing incentives for such fisheries (Gibbons et al., 2016; Hays et al., 2018).
To develop a successful blue economy, it is essential to align a healthy and resilient blue natural capital with secure investment and marine uses. This requires the best science, data, and technology, especially to address topics where important fallouts coexist with knowledge gaps, as is the case for GZ.
Data availability statement
The original contributions presented in the study are included in the article/Supplementary material. Specifically, the list of articles detected and examined during the PRISMA systematic reviews are provided as in two excel files added as Supplementary Tables 7 and 8. Further inquiries can be directed to the corresponding author.
Author contributions
YS: Conceptualization, Investigation, Methodology, Visualization, Writing – original draft, Writing – review & editing. SP: Conceptualization, Investigation, Methodology, Writing – original draft, Writing – review & editing. CL: Conceptualization, Investigation, Methodology, Writing – original draft, Writing – review & editing. VL: Conceptualization, Formal analysis, Investigation, Methodology, Visualization, Writing – original draft, Writing – review & editing. AN: Conceptualization, Formal analysis, Investigation, Methodology, Visualization, Writing – original draft, Writing – review & editing. CJ: Conceptualization, Formal analysis, Investigation, Methodology, Writing – original draft, Writing – review & editing. MB-B: Conceptualization, Formal analysis, Investigation, Methodology, Writing – original draft, Writing – review & editing. LF: Formal analysis, Investigation, Methodology, Writing – review & editing. ÁB: Conceptualization, Funding acquisition, Investigation, Methodology, Supervision, Writing – review & editing. ES: Writing – review & editing. EA: Writing – review & editing. PB: Writing – review & editing. RA: Writing – review & editing. AL: Writing – review & editing. NM: Visualization, Writing – review & editing. LF: Writing – review & editing. MH: Visualization, Writing – review & editing. TD: Writing – review & editing. GT: Writing – review & editing. SK: Conceptualization, Funding acquisition, Investigation, Methodology, Supervision, Writing – original draft, Writing – review & editing.
Funding
The author(s) declare financial support was received for the research, authorship, and/or publication of this article. This manuscript is a result of the joint activity of two projects funded by the European Union, under the Horizon Europe program: GES4SEAS (“Achieving Good Environmental Status for maintaining ecosystem services, by assessing integrated impacts of cumulative pressures,” grant agreement no. 101059877, www.ges4seas.eu), and ACTNOW (“Advancing understanding of cumulative impacts on European marine biodiversity, ecosystem functions and services for human wellbeing”, grant agreement No. 101060072). EA (and IECS Ltd participation in GES4SEAS) was supported by UK Research and Innovation (grant no. 10050522), MH was supported by Defra (UK) through the marine arm of their Natural Capital and Ecosystem Assessment (NCEA) program (NC34 Pelagic program- “PelCap”), and CJ by Villum Fonden Denmark (grant no. 25512).
Acknowledgments
We would like to thank Science Crunchers for their artwork on the graphical abstract representing an overview of the contents of this article (Supplementary Figure S4). This is contribution number 1236 from AZTI's Marine Research, Basque Research and Technology Alliance (BRTA).
Conflict of interest
EA was employed by International Estuarine & Coastal Specialists (IECS) Ltd.
The remaining authors declare that the research was conducted in the absence of any commercial or financial relationships that could be construed as a potential conflict of interest.
The author(s) declared that they were an editorial board member of Frontiers, at the time of submission. This had no impact on the peer review process and the final decision.
Publisher's note
All claims expressed in this article are solely those of the authors and do not necessarily represent those of their affiliated organizations, or those of the publisher, the editors and the reviewers. Any product that may be evaluated in this article, or claim that may be made by its manufacturer, is not guaranteed or endorsed by the publisher.
Supplementary material
The Supplementary Material for this article can be found online at: https://www.frontiersin.org/articles/10.3389/focsu.2024.1449190/full#supplementary-material
Supplementary Figure S1 | Schematic diagram of the screening steps for gelatinous zooplankton (GZ) impacts article selection. Reason 1 = out of scope (e.g., no negative impacts reported or not about GZ species), Reason 2 = language, Reason 3 = publication type (e.g., review; note that all retrieved review articles were screened for citation identification and relevant articles were added for screening as “Other sources”).
Supplementary Figure S2 | Schematic diagram of the screening steps undergone for global gelatinous zooplankton (GZ) monitoring articles selection. Reason 1 = not GZ monitoring, Reason 2 = language, Reason 3 = publication type, Reason 4 = modeling.
Supplementary Figure S3 | Schematic diagram of article selection on polyp (Scyphozoa and Cubozoa) monitoring through the screening steps. Reason 1 = not polyp monitoring, Reason 2 = Hydrozoans, Reason 3 = freshwater species, Reason 4 = publication type.
Supplementary Figure S4 | Overview of the different aspects related to gelatinous zooplankton covered by this study. The box sizes of the different impacts and monitoring categories are proportional to the number of articles addressing them within the systematic reviews performed.
Supplementary Figure S5 | (A) Location of Loch Ewe Site from the Scottish Coastal Observatory. Paired monthly observations of lifeform abundances: (B) gelatinous zooplankton versus fish larvae/eggs, and (C) gelatinous zooplankton vs. crustacean zooplankton. (D) Interannual decreases of gelatinous zooplankton (Marine Scotland Science, 2018; Wells et al., 2022).
Supplementary Figure S6 | Spatial distribution of studies on gelatinous zooplankton outbreaks and aggregations (n = 200) and polyp monitoring (n = 19) published between 2008–2023.
Supplementary Figure S7 | Counts of reports of monitoring methods used for gelatinous zooplankton assessment and polyp (benthic stage of Scyphozoa and Cubozoa) monitoring by year, published between 2008–2023 (*articles published in 2023 are not representative as the literature research was performed until June of that year).
Supplementary Table S1 | Definitions of type of evidence categories considered sensu Katsanevakis et al. (2014).
Supplementary Table S2 | Magnitude of impacts categories sensu Blackburn et al. (2014).
Supplementary Table S3 | Adverse impacts, and the gelatinous zooplankton (GZ) species causing them, identified in this study. Abbreviations for the European regions: GN, Greater North Sea; C, Celtic Sea; B, Baltic Sea; SE, South European Shelf Seas (including Bay of Biscay and the Iberian Coast), MED, Mediterranean Sea.
Supplementary Table S4 | Potential causes (pressures) of gelatinous zooplankton (GZ) outbreaks reported in the literature.
Supplementary Table S5 | Marine Strategy Framework Directive (MSFD) descriptors and gelatinous zooplankton (GZ) relevance.
Supplementary Table S6 | Methods used for Scyphozoa and Cubozoa polyp stage monitoring, published from 2008 to 2023 (n = 19) and the target species.
References
Abdul Azis, P. K., Al-Tisan, I., Al-Daili, M., Green, T. N., Dalvi, A. G. I., and Javeed, M. A. (2000). Effects of environment on source water for desalination plants on the eastern coast of Saudi Arabia. Desalination 132, 29–40. doi: 10.1016/S0011-9164(00)00132-6
Ahn, E.-Y., Hwang, S. J., Choi, M.-J., Cho, S., Lee, H.-J., and Park, Y. (2018). Upcycling of jellyfish (Nemopilema nomurai) sea wastes as highly valuable reducing agents for green synthesis of gold nanoparticles and their antitumor and anti-inflammatory activity. Artif. Cells Nanomed. Biotechnol. 46, 1127–1136. doi: 10.1080/21691401.2018.1480490
Alldredge, A. L. (1982). Aggregation of spawning appendicularians in surface windrows. Bull. Mar. Sci. 32, 250–254.
Amreen Nisa, S., Vinu, D., Krupakar, P., Govindaraju, K., Sharma, D., and Vivek, R. (2021). Jellyfish venom proteins and their pharmacological potentials: a review. Int. J. Biol. Macromol. 176, 424–436. doi: 10.1016/j.ijbiomac.2021.02.074
Angel, D. L., Edelist, D., and Freeman, S. (2016). Local perspectives on regional challenges: jellyfish proliferation and fish stock management along the Israeli Mediterranean coast. Reg. Environ. Change 16, 315–323. doi: 10.1007/s10113-014-0613-0
Atkinson, A., Lilley, M. K. S., Hirst, A. G., McEvoy, A. J., Tarran, G. A., Widdicombe, C., et al. (2021). Increasing nutrient stress reduces the efficiency of energy transfer through planktonic size spectra. Limnol. Oceanogr. 66, 422–437. doi: 10.1002/lno.11613
Aubert, A., Antajan, E., Lynam, C., Pitois, S., Pliru, A., Vaz, S., et al. (2018). No more reason for ignoring gelatinous zooplankton in ecosystem assessment and marine management: concrete cost-effective methodology during routine fishery trawl surveys. Mar. Policy 89, 100–108. doi: 10.1016/j.marpol.2017.12.010
Aznar, F., Pujol, M., and Rizo, R. (2017). A swarm behaviour for jellyfish bloom detection. Ocean Eng. 134, 24–34. doi: 10.1016/j.oceaneng.2017.02.009
Báez, J. C., Pennino, M. G., Albo-Puigserver, M., Coll, M., Giraldez, A., and Bellido, J. M. (2022). Effects of environmental conditions and jellyfish blooms on small pelagic fish and fisheries from the Western Mediterranean Sea. Estuar. Coast. Shelf Sci. 264:107699. doi: 10.1016/j.ecss.2021.107699
Basso, L., Rizzo, L., Marzano, M., Intranuovo, M., Fosso, B., Pesole, G., et al. (2019). Jellyfish summer outbreaks as bacterial vectors and potential hazards for marine animals and humans health? The case of Rhizostoma pulmo (Scyphozoa, Cnidaria). Sci. Total Environ. 692, 305–318. doi: 10.1016/j.scitotenv.2019.07.155
Bastardie, F., Brown, E. J., Andonegi, E., Arthur, R., Beukhof, E., Depestele, J., et al. (2021). A review characterizing 25 ecosystem challenges to be addressed by an ecosystem approach to fisheries management in Europe. Front. Mar. Sci. 7:629186. doi: 10.3389/fmars.2020.629186
Bastian, T., Haberlin, D., Purcell, J. E., Hays, G. C., Davenport, J., McAllen, R., et al. (2011). Large-scale sampling reveals the spatio-temporal distributions of the jellyfish Aurelia aurita and Cyanea capillata in the Irish Sea. Mar. Biol. 158, 2639–2652. doi: 10.1007/s00227-011-1762-z
Baxter, E., Rodger, H., McAllen, R., and Doyle, T. (2011a). Gill disorders in marine-farmed salmon: investigating the role of hydrozoan jellyfish. Aquac. Environ. Inter. 1, 245–257. doi: 10.3354/aei00024
Baxter, E. J., Sturt, M. M., Ruane, N. M., Doyle, T. K., McAllen, R., Harman, L., et al. (2011b). Gill damage to Atlantic salmon (Salmo salar) caused by the common jellyfish (Aurelia aurita) under experimental challenge. PLoS ONE 6:e18529. doi: 10.1371/journal.pone.0018529
Baxter, E. J., Walne, A. W., Purcell, J. E., McAllen, R., and Doyle, T. K. (2010). “Identification of jellyfish from Continuous Plankton Recorder samples,” in Jellyfish Blooms: New Problems and Solutions Developments in Hydrobiology 212, eds. J. E. Purcell and D. L. Angel (Dordrecht: Springer Netherlands), 193–201. doi: 10.1007/978-90-481-9541-1_15
Bayha, K. M., and Graham, W. M. (2009). A new Taqman© PCR-based method for the detection and identification of scyphozoan jellyfish polyps. Hydrobiologia 616, 217–228. doi: 10.1007/s10750-008-9590-y
Bedford, J., Johns, D., Greenstreet, S., and McQuatters-Gollop, A. (2018). Plankton as prevailing conditions: a surveillance role for plankton indicators within the Marine Strategy Framework Directive. Marine Policy 89, 109–115. doi: 10.1016/j.marpol.2017.12.021
Behrends, G., and Schneider, G. (1995). Impact of Aurelia aurita medusae (Cnidaria, Scyphozoa) on the standing stock and community composition of mesozooplankton in the Kiel Bight (western Baltic Sea). Mar. Ecol. Prog. Ser. 127, 39–45. doi: 10.3354/meps127039
Ben-David, E., Habibi, M., Hadad, E., Hasanin, M., Sammar, M., Angel, D. L., et al. (2023). Mechanism of nanoplastics capture by jellyfish mucin and its potential as a sustainable water treatment technology. Sci. Total Environ. 869:161824. doi: 10.1016/j.scitotenv.2023.161824
Biard, T., Stemmann, L., Picheral, M., Mayot, N., Vandromme, P., Hauss, H., et al. (2016). In situ imaging reveals the biomass of giant protists in the global ocean. Nature 532, 504–507. doi: 10.1038/nature17652
Bilio, M., and Niermann, U. (2004). Is the comb jelly really to blame for it all? Mnemiopsis leidyi and the ecological concerns about the Caspian Sea. Mar. Ecol. Prog. Ser. 269, 173–183. doi: 10.3354/meps269173
Blackburn, T. M., Essl, F., Evans, T., Hulme, P. E., Jeschke, J. M., Kühn, I., et al. (2014). A unified classification of alien species based on the magnitude of their environmental impacts. PLoS Biol. 12:e1001850. doi: 10.1371/journal.pbio.1001850
Bleve, G., Ramires, F. A., De Domenico, S., and Leone, A. (2021). An alum-free jellyfish treatment for food applications. Front. Nutr. 8:718798. doi: 10.3389/fnut.2021.718798
Bleve, G., Ramires, F. A., Gallo, A., and Leone, A. (2019). Identification of safety and quality parameters for preparation of jellyfish based novel food products. Foods 8, 263. doi: 10.3390/foods8070263
Boero, F. (2013). Review of jellyfish blooms in the Mediterranean and Black Sea. General Fisheries Commission for the Mediterranean. Studies and Reviews 92, 63. Available at: https://www.fao.org/3/i3169e/i3169e.pdf (accessed September 6, 2023).
Boero, F., Brotz, L., Gibbons, M., Piraino, S., and Zampardi, S. (2016). “3.10 - Impacts and effects of ocean warming on jellyfish,” in Explaining Ocean warming: causes, scale, effects, and consequences. Full report. ed. D. Laffoley and J.M. Baxter (Gland, Switzerland: IUCN), 213–237.
Bolte, B., Goldsbury, J., Huerlimann, R., Jerry, D., and Kingsford, M. (2021). Validation of eDNA as a viable method of detection for dangerous cubozoan jellyfish. Environ. DNA 3, 769–779. doi: 10.1002/edn3.181
Borchert, F., Emadodin, I., Kluss, C., Rotter, A., and Reinsch, T. (2021). Grass growth and N2O emissions from soil after application of jellyfish in coastal areas. Front. Mar. Sci. 8:711601. doi: 10.3389/fmars.2021.711601
Bosch-Belmar, M., Giomi, C., Milisenda, G., Abbruzzo, A., and Sarà, G. (2021a). Integrating functional traits into correlative species distribution models to investigate the vulnerability of marine human activities to climate change. Sci. Total Environ. 799:149351. doi: 10.1016/j.scitotenv.2021.149351
Bosch-Belmar, M., Giomi, F., Rinaldi, A., Mandich, A., Fuentes, V., Mirto, S., et al. (2016a). Concurrent environmental stressors and jellyfish stings impair caged European sea bass (Dicentrarchus labrax) physiological performances. Sci. Rep. 6:27929. doi: 10.1038/srep27929
Bosch-Belmar, M., Milisenda, G., Basso, L., Doyle, T. K., Leone, A., and Piraino, S. (2021b). Jellyfish impacts on marine aquaculture and fisheries. Rev. Fisheries Sci. Aquac. 29, 242–259. doi: 10.1080/23308249.2020.1806201
Bosch-Belmar, M., Milisenda, G., Girons, A., Taurisano, V., Accoroni, S., Totti, C., et al. (2017). Consequences of stinging plankton blooms on finfish mariculture in the Mediterranean Sea. Front. Mar. Sci. 4:240. doi: 10.3389/fmars.2017.00240
Bosch-Belmar, M., M'Rabet, C., Dhaouadi, R., Chalghaf, M., Daly Yahia, M. N., Fuentes, V., et al. (2016b). Jellyfish stings trigger gill disorders and increased mortality in farmed Sparus aurata (Linnaeus, 1758) in the Mediterranean Sea. PLoS ONE 11:e0154239. doi: 10.1371/journal.pone.0154239
Breitburg, D. L., Adamack, A., Rose, K. A., Kolesar, S. E., Decker, B., Purcell, J. E., et al. (2003). The pattern and influence of low dissolved oxygen in the Patuxent River, a seasonally hypoxic estuary. Estuaries 26, 280–297. doi: 10.1007/BF02695967
Brierley, A. S., Boyer, D. S., Axelsen, B. E., Lynam, C. P., Sparks, C. A. J., Boyer, H., et al. (2005). Towards the acoustic estimation of jellyfish abundance. Mar. Ecol. Prog. Ser. 295, 105–111. doi: 10.3354/meps295105
Brodeur, R. D., Link, J. S., Smith, B. E., Ford, M. D., Kobayashi, D. R., and Jones, T. T. (2016). Ecological and economic consequences of ignoring jellyfish: a plea for increased monitoring of ecosystems. Fisheries 41, 630–637. doi: 10.1080/03632415.2016.1232964
Brotz, L. (2016). Jellyfish fisheries of the world. PhD Thesis, University of British Columbia, Vancouver, BC, Canada, 180.
Brotz, L., and Pauly, D. (2012). Jellyfish populations in the Mediterranean Sea. Acta Adri 53, 211–230.
Brotz, L., and Pauly, D. (2017). “Studying jellyfish fisheries: toward accurate national catch reports and appropriate methods for stock assessments,” in Jellyfish: Ecology, Distribution Patterns and Human Interactions Fish, fishing, and fisheries, ed. G. L. Mariottini (Hauppauge, New York: Nova Science Publishers), 313–329.
Brotz, L., Schiariti, A., López-Martínez, J., Álvarez-Tello, J., Hsieh, Y.-H. P., Jones, R. P., et al. (2017). Jellyfish fisheries in the Americas: origin, state of the art, and perspectives on new fishing grounds. Rev. Fish Biol. Fish 27, 1–29. doi: 10.1007/s11160-016-9445-y
BSC (2019). State of the Environment of the Black Sea (2009-2014/5). Publications of the Commission on the Protection of the Black Sea Against Pollution, Istanbul, Turkey, 811. Available at: http://www.blacksea-commission.org/SoE2009-2014/SoE2009-2014.pdf (accessed September 6, 2023).
Budiša, A., Paliaga, P., Juretić, T., Lučić, D., Supić, N., Pasarić, Z., et al. (2021). Distribution, diet and relationships of the invasive ctenophore Mnemiopsis leidyi with anchovies and zooplankton, in the northeastern Adriatic Sea. Mediter. Marine Sci. 22, 827–842. doi: 10.12681/mms.23305
Burrell, V. G., and Van Engel, W. A. (1976). Predation by and distribution of a ctenophore, Mnemiopsis leidyi A. Agassiz, in the York River estuary. Estuar. Coastal Marine Sci. 4, 235–242. doi: 10.1016/0302-3524(76)90057-8
Cardona, L., Álvarez De Quevedo, I., Borrell, A., and Aguilar, A. (2012). Massive consumption of gelatinous plankton by Mediterranean apex predators. PLoS ONE 7:e31329. doi: 10.1371/journal.pone.0031329
Cargo, D. G., and Schultz, L. P. (1966). Notes on the biology of the sea nettle, Chrysaora quinquecirrha, in Chesapeake Bay. Chesapeake Sci. 7, 95–100. doi: 10.2307/1351129
Carr, E. F., and Pitt, K. A. (2008). Behavioural responses of zooplankton to the presence of predatory jellyfish. J. Exp. Mar. Biol. Ecol. 354, 101–110. doi: 10.1016/j.jembe.2007.10.012
CBD (1992). Convention on Biological Diversity: Text and Annexes. Secretariat of the Convention on Biological Diversity, Montreal, 30. Available at: https://www.cbd.int/doc/legal/cbd-en.pdf (accessed September 6, 2023).
Cegolon, L., Heymann, W., Lange, J., and Mastrangelo, G. (2013). Jellyfish stings and their management: a review. Mar. Drugs 11, 523–550. doi: 10.3390/md11020523
Chelsky, A., Pitt, K. A., Ferguson, A. J. P., Bennett, W. W., Teasdale, P. R., and Welsh, D. T. (2016). Decomposition of jellyfish carrion in situ: Short-term impacts on infauna, benthic nutrient fluxes and sediment redox conditions. Sci. Total Environ. 566, 929–937. doi: 10.1016/j.scitotenv.2016.05.011
Chen, N., and Hong, H. (2012). Integrated management of nutrients from the watershed to coast in the subtropical region. Curr. Opin. Environ. Sustain. 4, 233–242. doi: 10.1016/j.cosust.2012.03.007
Chittenden, C. M., Sweeting, R., Neville, C. M., Young, K., Galbraith, M., Carmack, E., et al. (2018). Estuarine and marine diets of out-migrating Chinook Salmon smolts in relation to local zooplankton populations, including harmful blooms. Estuar. Coast. Shelf Sci. 200, 335–348. doi: 10.1016/j.ecss.2017.11.021
Churnside, J. H., Marchbanks, R. D., Donaghay, P. L., Sullivan, J. M., Graham, W. M., and Wells, R. J. D. (2016). Hollow aggregations of moon jellyfish (Aurelia spp.). J. Plankton Res. 38, 122–130. doi: 10.1093/plankt/fbv092
Cillari, T., Allegra, A., Berto, D., Bosch-Belmar, M., Falautano, M., Maggio, T., et al. (2022). Snapshot of the distribution and biology of alien jellyfish Cassiopea andromeda (Forsskål, 1775) in a Mediterranean touristic harbour. Biology 11:319. doi: 10.3390/biology11020319
Clinton, M., Ferrier, D. E., Martin, S. A., and Brierley, A. S. (2021). Impacts of jellyfish on marine cage aquaculture: an overview of existing knowledge and the challenges to finfish health. ICES J. Mar. Sci. 78, 1557–1573. doi: 10.1093/icesjms/fsaa254
Cochrane, S. K. J., Connor, D. W., Nilsson, P., Mitchell, I., Reker, J., Franco, J., et al. (2010). Marine Strategy Framework Directive—Task Group 1 Report Biological Diversity. EUR 24337 EN-Joint Research Centre. Office for Official Publications of the European Communities, Luxembourg, 110. Available at: https://publications.jrc.ec.europa.eu/repository/handle/JRC58101 (accessed February 20, 2024).
Colombo, G. A., Mianzan, H., and Madirolas, A. (2003). Acoustic characterization of gelatinous plankton aggregations: four case studies from the Argentine continental shelf. ICES J. Mar. Sci. 60, 650–657. doi: 10.1016/S1054-3139(03)00051-1
Condon, R. H., Graham, W. M., Duarte, C. M., Pitt, K. A., Lucas, C. H., Haddock, S. H. D., et al. (2012). Questioning the rise of gelatinous zooplankton in the world's oceans. Bioscience 62, 160–169. doi: 10.1525/bio.2012.62.2.9
Condon, R. H., Steinberg, D. K., Del Giorgio, P. A., Bouvier, T. C., Bronk, D. A., Graham, W. M., et al. (2011). Jellyfish blooms result in a major microbial respiratory sink of carbon in marine systems. Proc. Natl Acad. Sci. USA 108, 10225–10230. doi: 10.1073/pnas.1015782108
Conley, K. R., and Sutherland, K. R. (2015). Commercial fishers' perceptions of jellyfish interference in the Northern California Current. ICES J. Mar. Sci. 72, 1565–1575. doi: 10.1093/icesjms/fsv007
Créach, V., Derveaux, S., Owen, K. R., Pitois, S., and Antajan, E. (2022). Use of environmental DNA in early detection of Mnemiopsis leidyi in UK coastal waters. Biol. Invasions 24, 415–424. doi: 10.1007/s10530-021-02650-0
Cruz-Colín, M. E., Cisneros-Mata, M. A., Montaño-Moctezuma, G., Espejel, I., Cisneros-Montemayor, A. M., and Malpica-Cruz, L. (2021). Analysis of the Gulf of California cannonball jellyfish fishery as a complex system. Ocean Coastal Manag. 207:105610. doi: 10.1016/j.ocecoaman.2021.105610
Culhane, F., Frid, C., Gelabert, E. R., and Robinson, L. (2019). EU policy-based assessment of the capacity of marine ecosystems to supply ecosystem services. European Topic Centre on Inland, Coastal and Marine Waters. ETC/ICM Technical Report 2/2019, 263 pp. Available at: https://www.eionet.europa.eu/ (accessed February 20, 2024).
Currie, B. J., and Jacups, S. P. (2005). Prospective study of Chironex fleckeri and other box jellyfish stings in the “Top End” of Australia's Northern Territory. Med. J. Austr. 183, 631–636. doi: 10.5694/j.1326-5377.2005.tb00062.x
Dale, V. H., and Beyeler, S. C. (2001). Challenges in the development and use of ecological indicators. Ecol. Indic. 1, 3–10. doi: 10.1016/S1470-160X(01)00003-6
D'Ambra, I., Graham, W. M., Carmichael, R. H., and Hernandez, F. J. (2015). Fish rely on scyphozoan hosts as a primary food source: evidence from stable isotope analysis. Mar. Biol. 162, 247–252. doi: 10.1007/s00227-014-2569-5
Daskalov, G. M., Grishin, A. N., Rodionov, S., and Mihneva, V. (2007). Trophic cascades triggered by overfishing reveal possible mechanisms of ecosystem regime shifts. Proc. Natl. Acad. Sci. USA 104, 10518–10523. doi: 10.1073/pnas.0701100104
Dawson, M. N., Martin, L. E., and Penland, L. K. (2001). Jellyfish swarms, tourists, and the Christ-child. Hydrobiologia 451, 131–144. doi: 10.1023/A:1011868925383
De Domenico, S., De Rinaldis, G., Mammone, M., Bosch-Belmar, M., Piraino, S., and Leone, A. (2023). The zooxanthellate jellyfish holobiont Cassiopea andromeda, a source of soluble bioactive compounds. Mar. Drugs 21:272. doi: 10.3390/md21050272
De Domenico, S., De Rinaldis, G., Paulmery, M., Piraino, S., and Leone, A. (2019). Barrel jellyfish (Rhizostoma pulmo) as source of antioxidant peptides. Mar. Drugs 17:134. doi: 10.3390/md17020134
De Donno, A., Idolo, A., Bagordo, F., Grassi, T., Leomanni, A., Serio, F., et al. (2014). Impact of stinging jellyfish proliferations along south Italian coasts: Human health hazards, treatment and social costs. Int. J. Environ. Res. Public Health 11, 2488–2503. doi: 10.3390/ijerph110302488
De Rinaldis, G., Leone, A., De Domenico, S., Bosch-Belmar, M., Slizyte, R., Milisenda, G., et al. (2021). Biochemical characterization of Cassiopea andromeda (Forsskål, 1775), another red sea jellyfish in the Western Mediterranean Sea. Mar. Drugs 19:498. doi: 10.3390/md19090498
Décima, M., Stukel, M. R., Nodder, S. D., Gutiérrez-Rodríguez, A., Selph, K. E., Lopes Dos Santos, A., et al. (2023). Salp blooms drive strong increases in passive carbon export in the Southern Ocean. Nat. Commun. 14:425. doi: 10.1038/s41467-022-35204-6
Decker, M. B., Brodeur, R. D., Ciannelli, L., Britt, L. L., Bond, N. A., DiFiore, B. P., et al. (2023). Cyclic variability of eastern Bering Sea jellyfish relates to regional physical conditions. Prog. Oceanogr. 210:102923. doi: 10.1016/j.pocean.2022.102923
Deidun, A., Arrigo, S., and Piraino, S. (2011). The westernmost record of Rhopilema nomadica (Galil, 1990) in the Mediterranean – off the Maltese Islands. Aquatic Invasions 6, S99–S103. doi: 10.3391/ai.2011.6.S1.023
Delannoy, C. M. J., Houghton, J. D., Fleming, N. E., and Ferguson, H. W. (2011). Mauve Stingers (Pelagia noctiluca) as carriers of the bacterial fish pathogen Tenacibaculum maritimum. Aquaculture 311, 255–257. doi: 10.1016/j.aquaculture.2010.11.033
Di Camillo, C. G., Betti, F., Bo, M., Martinelli, M., Puce, S., and Bavestrello, G. (2010). Contribution to the understanding of seasonal cycle of Aurelia aurita (Cnidaria: Scyphozoa) scyphopolyps in the northern Adriatic Sea. J. Mar. Biol. Assoc. United Kingdom 90, 1105–1110. doi: 10.1017/S0025315409000848
Diciotti, R., Culurgioni, J., Serra, S., Trentadue, M., Chessa, G., Satta, C. T., et al. (2016). First detection of Mnemiopsis leidyi (Ctenophora, Bolinopsidae) in Sardinia (S'Ena Arrubia Lagoon, Western Mediterranean): a threat for local fishery and species recruitment. Mediterranean Mar. Sci. 17, 714–719. doi: 10.12681/mms.1719
Dinasquet, J., Granhag, L., and Riemann, L. (2012a). Stimulated bacterioplankton growth and selection for certain bacterial taxa in the vicinity of the ctenophore Mnemiopsis leidyi. Front. Microbiol. 3:302. doi: 10.3389/fmicb.2012.00302
Dinasquet, J., Titelman, J., Møller, L., Setälä, O., Granhag, L., Andersen, T., et al. (2012b). Cascading effects of the ctenophore Mnemiopsis leidyi on the planktonic food web in a nutrient-limited estuarine system. Mar. Ecol. Prog. Ser. 460, 49–61. doi: 10.3354/meps09770
Dobson, J. Y., Fonfría, E. S., Palacios, R., Blasco, E., and Bordehore, C. (2023). Citizen science effectively monitors biogeographical and phenological patterns of jellyfish. Ocean Coastal Manag. 242:106668. doi: 10.1016/j.ocecoaman.2023.106668
Dong, Z. (2019). “Chapter 8- Blooms of the Moon Jellyfish Aurelia: Causes, Consequences and Controls,” in World Seas: An Environmental Evaluation, Volume III: Ecological Issues and Environmental Impacts, ed. C. Sheppard (New York: Academic Press), 163–171. doi: 10.1016/B978-0-12-805052-1.00008-5
Dong, Z., Liu, D., and Keesing, J. K. (2010). Jellyfish blooms in China: Dominant species, causes and consequences. Mar. Pollut. Bull. 60, 954–963. doi: 10.1016/j.marpolbul.2010.04.022
Dong, Z., Wang, L., Sun, T., Liu, Q., and Sun, Y. (2018). Artificial reefs for sea cucumber aquaculture confirmed as settlement substrates of the moon jellyfish Aurelia coerulea. Hydrobiologia 818, 223–234. doi: 10.1007/s10750-018-3615-y
Doyle, T. K., De Haas, H., Cotton, D., Dorschel, B., Cummins, V., Houghton, J. D. R., et al. (2008). Widespread occurrence of the jellyfish Pelagia noctiluca in Irish coastal and shelf waters. J. Plankton Res. 30, 963–968. doi: 10.1093/plankt/fbn052
Doyle, T. K., Hays, G. C., Harrod, C., and Houghton, J. D. R. (2014). “Ecological and Societal Benefits of Jellyfish,” in Jellyfish Blooms, eds. K. A. Pitt and C. H. Lucas (Dordrecht: Springer Netherlands), 105–127. doi: 10.1007/978-94-007-7015-7_5
Dror, H., and Angel, D. (2023). Rising seawater temperatures affect the fitness of Rhopilema nomadica polyps and podocysts and the expansion of this medusa into the western Mediterranean. Mar. Ecol. Progr. Series 728, 123–143. doi: 10.3354/meps14224
Duarte, C. M., Fulweiler, R. W., Lovelock, C. E., Martinetto, P., Saunders, M. I., Pandolfi, J. M., et al. (2015). Reconsidering ocean calamities. Bioscience 65, 130–139. doi: 10.1093/biosci/biu198
Duarte, C. M., Pitt, K. A., Lucas, C. H., Purcell, J. E., Uye, S., Robinson, K., et al. (2013). Is global ocean sprawl a cause of jellyfish blooms? Front. Ecol. Environ. 11, 91–97. doi: 10.1890/110246
Duarte, I. M., Marques, S. C., Leandro, S. M., and Calado, R. (2022). An overview of jellyfish aquaculture: for food, feed, pharma and fun. Rev. Aquacult. 14, 265–287. doi: 10.1111/raq.12597
Dunlop, K. M., Jones, D. O. B., and Sweetman, A. K. (2018). Scavenging processes on jellyfish carcasses across a fjord depth gradient. Limnol. Oceanogr. 63, 1146–1155. doi: 10.1002/lno.10760
Edelist, D., Angel, D. L., Canning-Clode, J., Gueroun, S. K. M., Aberle, N., Javidpour, J., et al. (2021). Jellyfishing in Europe: current status, knowledge gaps, and future directions towards a sustainable practice. Sustainability 13:12445. doi: 10.3390/su132212445
Edelist, D., Guy-Haim, T., Kuplik, Z., Zuckerman, N., Nemoy, P., and Angel, D. L. (2020). Phenological shift in swarming patterns of Rhopilema nomadica in the Eastern Mediterranean Sea. J. Plankton Res. 42, 211–219. doi: 10.1093/plankt/fbaa008
Edelist, D., Knutsen, Ø., Ellingsen, I., Majaneva, S., Aberle, N., Dror, H., et al. (2022). Tracking jellyfish swarm origins using a combined oceanographic-genetic-citizen science approach. Front. Mar. Sci. 9:869619. doi: 10.3389/fmars.2022.869619
Elliott, A., Hobson, V., and Tang, K. W. (2017). Balancing fishery and conservation: a case study of the barrel jellyfish Rhizostoma octopus in South Wales. ICES J. Mar. Sci. 74, 234–241. doi: 10.1093/icesjms/fsw157
Emadodin, I., Reinsch, T., Rotter, A., Orlando-Bonaca, M., Taube, F., and Javidpour, J. (2020). A perspective on the potential of using marine organic fertilizers for the sustainable management of coastal ecosystem services. Environ. Sustain. 3, 105–115. doi: 10.1007/s42398-020-00097-y
European Commission (2008). Directive 2008/56/EC of the European Parliament and the Council of 17 June 2008 establishing a framework for community action in the field of marine environmental policy. Offic. J. Eur. Union L 164, 19–40.
European Commission (2017). Commission Decision (EU) 2017/848 of 17 May 2017 laying down criteria and methodological standards on good environmental status of marine waters and specifications and standardised methods for monitoring and assessment, and repealing Decision 2010/477/EU. Offic. J. Eur. Commun. L 125, 43–74.
European Commission (2020). Report from the Commission to the European Parliament and the Council on the implementation of the Marine Strategy Framework Directive (Directive 2008/56/EC- {SWD(2020) 60 final} – {SWD(2020) 61 final} – {SWD(2020) 62 final}. COM(2020) 259 final, 31.
Felician, F. F., Yu, R.-H., Li, M.-Z., Li, C.-J., Chen, H.-Q., Jiang, Y., et al. (2019). The wound healing potential of collagen peptides derived from the jellyfish Rhopilema esculentum. Chin. J. Traumatol. 22, 12–20. doi: 10.1016/j.cjtee.2018.10.004
Feng, S., Lin, J., Sun, S., and Zhang, F. (2017). Artificial substrates preference for proliferation and immigration in Aurelia aurita (s. l.) polyps. Chinese J. Oceanology and Limnology 35, 153–162. doi: 10.1007/s00343-016-5230-y
Feng, S., Sun, S., Li, C., and Zhang, F. (2022). Controls of Aurelia coerulea and Nemopilema nomurai (Cnidaria: Scyphozoa) blooms in the coastal sea of China: Strategies and measures. Front. Mar. Sci. 9:946830. doi: 10.3389/fmars.2022.946830
Feng, S., Zhang, G.-T., Sun, S., Zhang, F., Wang, S.-W., and Liu, M.-T. (2015). Effects of temperature regime and food supply on asexual reproduction in Cyanea nozakii and Nemopilema nomurai. Hydrobiologia 754, 201–214. doi: 10.1007/s10750-015-2279-0
Fenner, P. J., and Williamson, J. A. (1996). Worldwide deaths and severe envenomation from jellyfish stings. Med. J. Austr. 165, 658–661. doi: 10.5694/j.1326-5377.1996.tb138679.x
Ferguson, H. W., Christian, M. D., Hay, S., Nicolson, J., Sutherland, D., and Crumlish, M. (2010). Jellyfish as vectors of bacterial disease for farmed salmon (Salmo salar). J. Veter. Diagn. Invest. 22, 376–382. doi: 10.1177/104063871002200305
Fernández-Alías, A., Marcos, C., and Pérez-Ruzafa, A. (2021). Larger scyphozoan species dwelling in temperate, shallow waters show higher blooming potential. Mar. Pollut. Bull. 173:113100. doi: 10.1016/j.marpolbul.2021.113100
Fernández-Alías, A., Montaño-Barroso, T., Conde-Caño, M.-R., Manchado-Pérez, S., López-Galindo, C., Quispe-Becerra, J.-I., et al. (2022). Nutrient overload promotes the transition from top-down to bottom-up control and triggers dystrophic crises in a Mediterranean coastal lagoon. Sci. Total Environ. 846:157388. doi: 10.1016/j.scitotenv.2022.157388
Fernández-Cervantes, I., Rodríguez-Fuentes, N., León-Deniz, L. V., Alcántara Quintana, L. E., Cervantes-Uc, J. M., Herrera Kao, W. A., et al. (2020). Cell-free scaffold from jellyfish Cassiopea andromeda (Cnidaria; Scyphozoa) for skin tissue engineering. Mater. Sci. Eng. 111:110748. doi: 10.1016/j.msec.2020.110748
Ferrer, L., and González, M. (2021). Relationship between dimorphism and drift in the Portuguese man-of-war. Cont. Shelf Res. 212:104269. doi: 10.1016/j.csr.2020.104269
Ferrer, L., Zaldua-Mendizabal, N., Del Campo, A., Franco, J., Mader, J., Cotano, U., et al. (2015). Operational protocol for the sighting and tracking of Portuguese man-of-war in the southeastern Bay of Biscay: observations and modeling. Cont. Shelf Res. 95, 39–53. doi: 10.1016/j.csr.2014.12.011
Finenko, G. A., Romanova, Z. A., Abolsmasova, G. I., Anninsky, B. E., Svetlichny, L. S., Hubareva, E. S., et al. (2003). Population dynamics, ingestion, growth and reproduction rates of the invader Beroe ovata and its impact on plankton community in Sevastopol Bay, the Black Sea. J. Plankton Res. 25, 539–549. doi: 10.1093/plankt/25.5.539
Fleming, N. E. C., Harrod, C., Newton, J., and Houghton, J. D. R. (2015). Not all jellyfish are equal: isotopic evidence for inter- and intraspecific variation in jellyfish trophic ecology. PeerJ 3:e1110. doi: 10.7717/peerj.1110
Foster, V., Giesler, R. J., Wilson, A. M. W., Nall, C. R., and Cook, E. J. (2016). Identifying the physical features of marina infrastructure associated with the presence of non-native species in the UK. Mar. Biol. 163:173. doi: 10.1007/s00227-016-2941-8
Galil, B. S., Spanier, E., and Ferguson, W. W. (1990). The scyphomedusae of the Mediterranean coast of Israel including two Lessepsian migrants new to the Mediterranean. Zoologische Mededelingen 64, 95–106.
Gao, M., Li, S., Wang, K., Bai, Y., Ding, Y., Zhang, B., et al. (2023). Real-time jellyfish classification and detection algorithm based on improved YOLOv4-tiny and improved underwater image enhancement algorithm. Sci. Rep.13:12989. doi: 10.1038/s41598-023-39851-7
Gatt, P. M., Deidun, A., Galea, A., and Gauci, A. (2018). Is citizen science a valid tool to monitor the occurrence of jellyfish? The Spot the Jellyfish case study from the Maltese Islands. J. Coastal Res. 85, 316–320. doi: 10.2112/SI85-064.1
Gershwin, L.-A., De Nardi, M., Winkel, K. D., and Fenner, P. J. (2010). Marine Stingers: Review of an Under-Recognized Global Coastal Management Issue. Coast. Manag. 38, 22–41. doi: 10.1080/08920750903345031
Ghermandi, A., Galil, B., Gowdy, J., and Nunes, P. A. L. D. (2015). Jellyfish outbreak impacts on recreation in the Mediterranean Sea: welfare estimates from a socioeconomic pilot survey in Israel. Ecosyst. Serv. 11, 140–147. doi: 10.1016/j.ecoser.2014.12.004
Gibbons, M. J., Boero, F., and Brotz, L. (2016). We should not assume that fishing jellyfish will solve our jellyfish problem. ICES J. Mar. Sci. 73, 1012–1018. doi: 10.1093/icesjms/fsv255
Gibbons, M. J., Haddock, S. H. D., Matsumoto, G. I., and Foster, C. (2021). Records of ctenophores from South Africa. PeerJ 9:e10697. doi: 10.7717/peerj.10697
Gibbons, M. J., and Richardson, A. J. (2009). Patterns of jellyfish abundance in the North Atlantic. Hydrobiologia 616, 51–65. doi: 10.1007/s10750-008-9593-8
Gibbons, M. J., and Richardson, A. J. (2013). Beyond the jellyfish joyride and global oscillations: advancing jellyfish research. J. Plankton Res. 35, 929–938. doi: 10.1093/plankt/fbt063
Graham, W. M., and Bayha, K. M. (2007). “Biological invasions by marine jellyfish,” in Biological Invasions, ed. W. Nentwig (Berlin, Heidelberg: Springer Berlin Heidelberg), 193, 239–255. doi: 10.1007/978-3-540-36920-2_14
Graham, W. M., Gelcich, S., Robinson, K. L., Duarte, C. M., Brotz, L., Purcell, J. E., et al. (2014). Linking human well-being and jellyfish: ecosystem services, impacts, and societal responses. Front. Ecol. Environ. 12, 515–523. doi: 10.1890/130298
Guenther, J., Carl, C., and Sunde, L. M. (2009). The effects of colour and copper on the settlement of the hydroid Ectopleura larynx on aquaculture nets in Norway. Aquaculture 292, 252–255. doi: 10.1016/j.aquaculture.2009.04.018
Guenther, J., Misimi, E., and Sunde, L. M. (2010). The development of biofouling, particularly the hydroid Ectopleura larynx, on commercial salmon cage nets in Mid-Norway. Aquaculture 300, 120–127. doi: 10.1016/j.aquaculture.2010.01.005
Gueroun, S. K. M., Piraino, S., Yahia, O. K.-D., and Yahia, M. N. D. (2022). Jellyfish diversity, trends and patterns in Southwestern Mediterranean Sea: a citizen science and field monitoring alliance. J. Plankton Res. 44, 819–837. doi: 10.1093/plankt/fbac057
Haberlin, D., McAllen, R., and Doyle, T. K. (2021). Field and flume tank experiments investigating the efficacy of a bubble curtain to keep harmful jellyfish out of finfish pens. Aquaculture 531, 735915. doi: 10.1016/j.aquaculture.2020.735915
Hamel, H., Lhoumeau, S., Wahlberg, M., and Javidpour, J. (2021). Using drones to measure jellyfish density in shallow estuaries. J. Mar. Sci. Eng. 9, 659. doi: 10.3390/jmse9060659
Hamner, W. M., and Dawson, M. N. (2009). A review and synthesis on the systematics and evolution of jellyfish blooms: advantageous aggregations and adaptive assemblages. Hydrobiologia 616, 161–191. doi: 10.1007/s10750-008-9620-9
Han, C.-H., and Uye, S.-I. (2009). Quantification of the abundance and distribution of the common jellyfish Aurelia aurita s.l. with a Dual-frequency IDentification SONar (DIDSON). J. Plankton Research 31, 805–814. doi: 10.1093/plankt/fbp029
Han, Y., Chang, Q., Ding, S., Gao, M., Zhang, B., and Li, S. (2022). Research on multiple jellyfish classification and detection based on deep learning. Multimed. Tools Appl. 81, 19429–19444. doi: 10.1007/s11042-021-11307-y
Haraldsson, M., Jaspers, C., Tiselius, P., Aksnes, D. L., Andersen, T., and Titelman, J. (2013). Environmental constraints of the invasive Mnemiopsis leidyi in Scandinavian waters. Limnol. Oceanogr. 58, 37–48. doi: 10.4319/lo.2013.58.1.0037
Hartwick, R. F. (1991). Distributional ecology and behaviour of the early life stages of the box-jellyfish Chironex fleckeri. Hydrobiologia 216, 181–188. doi: 10.1007/BF00026460
Hays, G. C., Bastian, T., Doyle, T. K., Fossette, S., Gleiss, A. C., Gravenor, M. B., et al. (2012). High activity and Lévy searches: jellyfish can search the water column like fish. Proc. R. Soc. B 279, 465–473. doi: 10.1098/rspb.2011.0978
Hays, G. C., Doyle, T. K., and Houghton, J. D. R. (2018). A paradigm shift in the trophic importance of jellyfish? Trends Ecol. Evol. 33, 874–884. doi: 10.1016/j.tree.2018.09.001
He, J., Zheng, L., Zhang, W., and Lin, Y. (2015). Life cycle reversal in Aurelia sp.1 (Cnidaria, Scyphozoa). PLoS ONE 10:e0145314. doi: 10.1371/journal.pone.0145314
HELCOM (2018). Trend in arrival of new non-indigenous species. HELCOM core indicator report, 20 pp. ISSN 2343-2543. Available at: https://helcom.fi/wp-content/uploads/2019/08/Trends-in-arrival-of-new-non-indigenous-species-HELCOM-core-indicator-2018.pdf (accessed September 6, 2023).
Helmholz, H., Johnston, B. D., Ruhnau, C., and Prange, A. (2010). Gill cell toxicity of northern boreal scyphomedusae Cyanea capillata and Aurelia aurita measured by an in vitro cell assay. Hydrobiologia 645, 223–234. doi: 10.1007/s10750-010-0216-9
Hernroth, L., and Gröndahl, F. (1985). On the biology of Aurelia aurita (L.) 3. Predation by Coryphella verrucosa (gastropoda, opisthobranchia), a major factor regulating the development of Aurelia populations in the Gullmar Fjord, Western Sweden. Ophelia 24, 37–45. doi: 10.1080/00785236.1985.10426617
Holland, M., Louchart, A., Artigas, L. F., and McQuatters-Gollop, A. (2023a). Changes in phytoplankton and zooplankton communities. OSPAR, 2023: The 2023 Quality Status Report for the Northeast Atlantic, OSPAR Commission, London. Available at: https://oap.ospar.org/en/ospar-assessments/quality-status-reports/qsr-2023/indicator-assessments/changes-plankton-communities/ (accessed February 2, 2024).
Holland, M. M., Louchart, A., Artigas, L. F., Ostle, C., Atkinson, A., Rombouts, I., et al. (2023b). Major declines in NE Atlantic plankton contrast with more stable populations in the rapidly warming North Sea. Sci. Total Environ. 898:165505. doi: 10.1016/j.scitotenv.2023.165505
Hoover, R. A., Armour, R., Dow, I., and Purcell, J. E. (2012). Nudibranch predation and dietary preference for the polyps of Aurelia labiata (Cnidaria: Scyphozoa). Hydrobiologia 690, 199–213. doi: 10.1007/s10750-012-1044-x
Hosia, A., Stemmann, L., and Youngbluth, M. (2008). Distribution of net-collected planktonic cnidarians along the northern Mid-Atlantic Ridge and their associations with the main water masses. Deep Sea Res. Part II. 55, 106–118. doi: 10.1016/j.dsr2.2007.09.007
Houghton, J. D. R., Doyle, T. K., Wilson, M. W., Davenport, J., and Hays, G. C. (2006). Jellyfish aggregations and leatherback turtle foraging patterns in a temperate coastal environment. Ecology 87, 1967–1972. doi: 10.1890/0012-9658(2006)87[1967:JAALTF]2.0.CO;2
Hussein, O., Sayed, R., and Saleh, O. (2015). Uses of jellyfish in pre sowing seeds treatment and pest control. Am. J. Exper. Agric. 5, 60–69. doi: 10.9734/AJEA/2015/12196
Janßen, H., Augustin, C. B., Hinrichsen, H. H., and Kube, S. (2013). Impact of secondary hard substrate on the distribution and abundance of Aurelia aurita in the western Baltic Sea. Mar. Pollut. Bull. 75, 224–234. doi: 10.1016/j.marpolbul.2013.07.027
Jarman, S. N., McInnes, J. C., Faux, C., Polanowski, A. M., Marthick, J., Deagle, B. E., et al. (2013). Adélie penguin population diet monitoring by analysis of food DNA in scats. PLoS ONE 8:e82227. doi: 10.1371/journal.pone.0082227
Jarms, G., and Morandini, A. C. (2019). “World atlas of jellyfish: Scyphozoa except Stauromedusae,” in Abhandlungen des Naturwissenschaftlichen Vereins, ed. N. F. Hamburg, and A. C. Morandini (Hamburg: Dölling und Galitz Verlag), 816. ISBN 9783862180820.
Jaspers, C., Costello, J., Sutherland, K., Gemmell, B., Lucas, K., Tackett, J., et al. (2018b). Resilience in moving water: Effects of turbulence on the predatory impact of the lobate ctenophore Mnemiopsis leidyi. Limnol. Oceanogr. 63, 445–458. doi: 10.1002/lno.10642
Jaspers, C., Ehrlich, M., Pujolar, J. M., Künzel, S., Bayer, T., Limborg, M. T., et al. (2021). Invasion genomics uncover contrasting scenarios of genetic diversity in a widespread marine invader. Proc. Nat. Acad. Sci. U.S.A. 118:e2116211118. doi: 10.1073/pnas.2116211118
Jaspers, C., Hopcroft, R. R., Kiørboe, T., Lombard, F., López-Urrutia, Á., Everett, J. D., et al. (2023). Gelatinous larvacean zooplankton can enhance trophic transfer and carbon sequestration. Trends Ecol. Evolut. 38, 980–993. doi: 10.1016/j.tree.2023.05.005
Jaspers, C., Huwer, B., Antajan, E., Hosia, A., Hinrichsen, H.-H., Biastoch, A., et al. (2018a). Ocean current connectivity propelling the secondary spread of a marine invasive comb jelly across western Eurasia. Global Ecol. Biogeogr. 27, 814–827. doi: 10.1111/geb.12742
Jaspers, C., Møller, L. F., and Kiørboe, T. (2015). Reproduction rates under variable food conditions and starvation in Mnemiopsis leidyi: significance for the invasion success of a ctenophore. J. Plankton Res. 37, 1011–1018. doi: 10.1093/plankt/fbv017
Jennions, M. D., and Møller, A. P. (2002). Publication bias in ecology and evolution: an empirical assessment using the ‘trim and fill' method. Biol. Rev. Camb. Philos. Soc. 77, 211–222. doi: 10.1017/S1464793101005875
Katsanevakis, S., Wallentinus, I., Zenetos, A., Leppäkoski, E., Çinar, M. E., Oztürk, B., et al. (2014). Impacts of invasive alien marine species on ecosystem services and biodiversity: a pan-European review. Aquatic Invasions 9, 391–423. doi: 10.3391/ai.2014.9.4.01
Kennerley, A., Wood, L. E., Luisetti, T., Ferrini, S., and Lorenzoni, I. (2022). Economic impacts of jellyfish blooms on coastal recreation in a UK coastal town and potential management options. Ocean Coastal Manag. 227:106284. doi: 10.1016/j.ocecoaman.2022.106284
Kikinger, R. (1992). Cotylorhiza tuberculata (Cnidaria: Scyphozoa) - Life history of a stationary population. Marine Ecol. 13, 333–362. doi: 10.1111/j.1439-0485.1992.tb00359.x
Kim, D., Shin, J., Kim, H., Lee, D., Lee, S.-M., and Myung, H. (2013). “Experimental Tests of Autonomous Jellyfish Removal Robot System JEROS,” in Robot Intelligence Technology and Applications 2012. Advances in Intelligent Systems and Computing, eds. J. H. Kim, E. Matson, H. Myung, and P. Xu (Berlin, Heidelberg: Springer Berlin Heidelberg), 395–403. doi: 10.1007/978-3-642-37374-9_38
Kim, D. H., Seo, J. N., Yoon, W. D., and Suh, Y. S. (2012). Estimating the economic damage caused by jellyfish to fisheries in Korea. Fisher. Sci. 78, 1147–1152. doi: 10.1007/s12562-012-0533-1
Kim, J. H., Han, S. B., and Durey, A. (2018). Fatal pulmonary edema in a child after jellyfish stings in Korea. Wild. Environ. Med. 29, 527–530. doi: 10.1016/j.wem.2018.07.002
Kingsford, M., Pitt, K. A., and Gillanders, B. M. (2000). Management of jellyfish fisheries, with special reference to the Order Rhizostomeae. Oceanogr. Mar. Biol. 38, 85–156.
Kirby, R. R., Johns, G. G., and Lindley, J. A. (2006). Fathers in hot water: rising sea temperatures and a Northeastern Atlantic pipefish baby boom. Biol. Lett. 2, 2597–2600. doi: 10.1098/rsbl.2006.0530
Køhler, L. G., Huwer, B., Pujolar, J. M., Werner, M., Wikström, K., Wernbo, A., et al. (2022). Gelatinous macrozooplankton diversity and distribution dataset for the North Sea and Skagerrak/Kattegat during January-February 2021. Data Brief 44:108493. doi: 10.1016/j.dib.2022.108493
Kumar, S. B., Mohanty, A. K., Das, N. P. I., Satpathy, K. K., and Sarkar, S. K. (2017). Impingement of marine organisms in a tropical atomic power plant cooling water system. Mar. Pollut. Bull. 124, 555–562. doi: 10.1016/j.marpolbul.2017.07.067
Lalande, C., and Fortier, L. (2011). Downward particulate organic carbon export and jellyfish blooms in southeastern Hudson Bay. J. Mar. Syst. 88, 446–450. doi: 10.1016/j.jmarsys.2010.12.005
Lamb, P. D., Hunter, E., Pinnegar, J. K., Doyle, T. K., Creer, S., and Taylor, M. I. (2019). Inclusion of jellyfish in 30+ years of Ecopath with Ecosim models. ICES J. Mar. Sci. 76, 1941–1950. doi: 10.1093/icesjms/fsz165
Larson, R. J. (1987). A note on the feeding, growth, and reproduction of the epipelagic scyphomedusa Pelagia noctiluca (Forskål). Biol. Oceanogr. 4, 447–454.
Lawley, J. W., Gamero-Mora, E., Maronna, M. M., Chiaverano, L. M., Stampar, S. N., Hopcroft, R. R., et al. (2021). The importance of molecular characters when morphological variability hinders diagnosability: systematics of the moon jellyfish genus Aurelia (Cnidaria: Scyphozoa). PeerJ 9:e11954. doi: 10.7717/peerj.11954
Lebrato, M., Pahlow, M., Frost, J. R., Küter, M., De Jesus Mendes, P., Molinero, J., et al. (2019). Sinking of gelatinous zooplankton biomass increases deep carbon transfer efficiency globally. Global Biogeochem. Cycles 33, 1764–1783. doi: 10.1029/2019GB006265
Lebrato, M., Pitt, K. A., Sweetman, A. K., Jones, D. O. B., Cartes, J. E., Oschlies, A., et al. (2012). Jelly-falls historic and recent observations: a review to drive future research directions. Hydrobiologia 690, 227–245. doi: 10.1007/s10750-012-1046-8
Lee, S.-H., Tseng, L.-C., Ho Yoon, Y., Ramirez-Romero, E., Hwang, J.-S., and Molinero, J. C. (2023). The global spread of jellyfish hazards mirrors the pace of human imprint in the marine environment. Environ. Int. 171:107699. doi: 10.1016/j.envint.2022.107699
Lengar, Ž., Klun, K., Dogsa, I., Rotter, A., and Stopar, D. (2021). Sequestration of polystyrene microplastics by jellyfish mucus. Front. Mar. Sci. 8:690749. doi: 10.3389/fmars.2021.690749
Leone, A., Bleve, G., Gallo, A., Ramires, F. A., De Domenico, S., and Perbellini, E. (2021). Method for the treatment of jellyfish intended for human consumption without the use of aluminum salts and products/ingredients obtained by this process. EP20185250.6. Available at: https://worldwide.espacenet.com/publicationDetails/biblio?CC=ITandNR=201900011472A1andKC=A1andFT=DandND=anddate=20210111andDB=andlocale= (accessed September 6, 2023).
Leone, A., Lecci, R., Durante, M., Meli, F., and Piraino, S. (2015). The bright side of gelatinous blooms: nutraceutical value and antioxidant properties of three Mediterranean jellyfish (Scyphozoa). Mar. Drugs 13, 4654–4681. doi: 10.3390/md13084654
Leone, A., Lecci, R., Durante, M., and Piraino, S. (2013). Extract from the Zooxanthellate Jellyfish Cotylorhiza tuberculata modulates gap junction intercellular communication in human cell cultures. Mar. Drugs 11, 1728–1762. doi: 10.3390/md11051728
Leone, A., Lecci, R. M., Milisenda, G., and Piraino, S. (2019). Mediterranean jellyfish as novel food: effects of thermal processing on antioxidant, phenolic, and protein contents. Eur. Food Res. Technol. 245, 1611–1627. doi: 10.1007/s00217-019-03248-6
Leoni, V. (2022). Ecology of Rhizostoma pulmo in the southern European seas: From biogeography to population dynamics. PhD Thesis, Université de Montpellier, France 203.
Levy, T., Ghermandi, A., Lehahn, Y., Edelist, D., and Angel, D. L. (2024). Monitoring jellyfish outbreaks along Israel's Mediterranean coast using digital footprints. Sci. Total Environ. 922:171275. doi: 10.1016/j.scitotenv.2024.171275
Licandro, P., Conway, D. V. P., Daly Yahia, M. N., Fernandez De Puelles, M. L., Gasparini, S., Hecq, J. H., et al. (2010). A blooming jellyfish in the northeast Atlantic and Mediterranean. Biol. Lett. 6, 688–691. doi: 10.1098/rsbl.2010.0150
Lilley, M. K. S., Elineau, A., Ferraris, M., Thiery, A., Stemmann, L., Gorsky, G., et al. (2014). Individual shrinking to enhance population survival: quantifying the reproductive and metabolic expenditures of a starving jellyfish, Pelagia noctiluca. J. Plankton Res. 36, 1585–1597. doi: 10.1093/plankt/fbu079
Lilley, M. K. S., Houghton, J. D. R., and Hays, G. C. (2009). Distribution, extent of inter-annual variability and diet of the bloom-forming jellyfish Rhizostoma in European waters. J. Mar. Biol. Assoc. United Kingdom 89, 39–48. doi: 10.1017/S0025315408002439
Lippmann, J. M., Fenner, P. J., Winkel, K., and Gershwin, L. (2011). Fatal and severe box jellyfish stings, including Irukandji stings, in Malaysia, 2000–2010. J. Travel Med.18, 275–281. doi: 10.1111/j.1708-8305.2011.00531.x
Liu, C. S., Chen, S. Q., Zhuang, Z. M., Yan, J. P., Liu, C. L., and Cui, H. T. (2015). Potential of utilizing jellyfish as food in culturing Pampus argenteus juveniles. Hydrobiologia 754, 189–200. doi: 10.1007/s10750-014-1869-6
Lo, W.-T., Purcell, J. E., Hung, J.-J., Su, H.-M., and Hsu, P.-K. (2008). Enhancement of jellyfish (Aurelia aurita) populations by extensive aquaculture rafts in a coastal lagoon in Taiwan. ICES J. Mar. Sci. 65, 453–461. doi: 10.1093/icesjms/fsm185
Lucas, C. H., Gelcich, S., and Uye, S.-I. (2014). “Living with jellyfish: management and adaptation strategies,” in Jellyfish Blooms, eds. K. A. Pitt and C. H. Lucas (Dordrecht: Springer Netherlands), 129–150. doi: 10.1007/978-94-007-7015-7_6
Lynam, C. P., and Brierley, A. S. (2007). Enhanced survival of 0-group gadoid fish under jellyfish umbrellas. Mar. Biol. 150, 1397–1401. doi: 10.1007/s00227-006-0429-7
Lynam, C. P., Gibbons, M. J., Axelsen, B. E., Sparks, C. A. J., Coetzee, J., Heywood, B. G., et al. (2006). Jellyfish overtake fish in a heavily fished ecosystem. Curr. Biol. 16, R492–R493. doi: 10.1016/j.cub.2006.09.012
Lynam, C. P., Heath, M. R., Hay, S. J., and Brierley, A. S. (2005). Evidence for impacts by jellyfish on North Sea herring recruitment. Mar. Ecol. Prog. Ser. 298, 157–167. doi: 10.3354/meps298157
Lynam, C. P., Lilley, M. K. S., Bastian, T., Doyle, T. K., Beggs, S. E., and Hays, G. C. (2011). Have jellyfish in the Irish Sea benefited from climate change and overfishing? Glob. Chang. Biol. 17, 767–782. doi: 10.1111/j.1365-2486.2010.02352.x
Magliozzi, C., Druon, J.-N., Palialexis, A., Aguzzi, L., Alexande, B., Antoniadis, K., et al. (2021). Pelagic habitats under the MSFD D1: scientific advice of policy relevance: recommendations to frame problems and solutions for the pelagic habitats' assessment. EUR 30671 EN, Publications Office of the European Union, Luxembourg, JRC124882, 50.
Magliozzi, C., Palma, M., Druon, J.-N., Palialexis, A., McQuatters-Gollop, A., Varkitzi, I., et al. (2023). Status of pelagic habitats within the EU-Marine Strategy Framework Directive: proposals for improving consistency and representativeness of the assessment. Marine Policy 148:105467. doi: 10.1016/j.marpol.2022.105467
Makabe, R., Furukawa, R., Takao, M., and Uye, S. (2014). Marine artificial structures as amplifiers of Aurelia aurita s.l. blooms: a case study of a newly installed floating pier. J. Oceanogr. 70, 447–455. doi: 10.1007/s10872-014-0249-1
Mammone, M., Ferrier-Pagés, C., Lavorano, S., Rizzo, L., Piraino, S., and Rossi, S. (2021). High photosynthetic plasticity may reinforce invasiveness of upside-down zooxanthellate jellyfish in Mediterranean coastal waters. PLoS ONE 16:e0248814. doi: 10.1371/journal.pone.0248814
Manzari, C., Fosso, B., Marzano, M., Annese, A., Caprioli, R., D'Erchia, A. M., et al. (2015). The influence of invasive jellyfish blooms on the aquatic microbiome in a coastal lagoon (Varano, SE Italy) detected by an Illumina-based deep sequencing strategy. Biol. Invasions 17, 923–940. doi: 10.1007/s10530-014-0810-2
Marambio, M., Canepa, A., Lòpez, L., Gauci, A. A., Gueroun, S. K. M., Zampardi, S., et al. (2021). Unfolding jellyfish bloom dynamics along the Mediterranean basin by transnational citizen science initiatives. Diversity 13:274. doi: 10.3390/d13060274
Marchessaux, G., Gadreaud, J., Martin-Garin, B., Thiéry, A., Ourgaud, M., Belloni, B., et al. (2017). First report of the invasive jellyfish Gonionemus vertens (A. Agassiz, 1862) in the Berre Lagoon, southeast France. Bioinvasions Records. Available at: https://www.reabic.net/journals/bir/2017/Issue4.aspx (accessed January 25, 2024). doi: 10.3391/bir.2017.6.4.06
Marcos-López, M., Mitchell, S. O., and Rodger, H. D. (2016). Pathology and mortality associated with the mauve stinger jellyfish Pelagia noctiluca in farmed Atlantic salmon Salmo salar L. J. Fish Dis. 39, 111–115. doi: 10.1111/jfd.12267
Marine Scotland Science (2018). Scottish Coastal Observatory - Loch Ewe Site: Loch Ewe: LTM Loch Ewe. Scottish Coastal Observatory - Loch Ewe site data. Available at: https://data.marine.gov.scot/dataset/scottish-coastal-observatory-loch-ewe-site (accessed January 25, 2024).
Marques, F., Angélico, M. M., Costa, J. L., Teodósio, M. A., Presado, P., Fernandes, A., et al. (2017). Ecological aspects and potential impacts of the non-native hydromedusa Blackfordia virginica in a temperate estuary. Estuar. Coast. Shelf Sci. 197, 69–79. doi: 10.1016/j.ecss.2017.08.015
Marques, J. C., Salas, F., Patricio, J., Teixeira, H., and Neto, J. (2009). Ecological Indicators for Coastal and Estuarine Environmental Assessment: a User Guide. Southampton, Boston: WIT Press, 183.
Marques, R., Albouy-Boyer, S., Delpy, F., Carr,é, C., Le Floc'h, É., Roques, C., et al. (2015). Pelagic population dynamics of Aurelia sp. in French Mediterranean lagoons. J. Plankton Res. 37, 1019–1035. doi: 10.1093/plankt/fbv059
Marques, R., Darnaude, A. M., Crochemore, S., Bouvier, C., and Bonnet, D. (2019). Molecular approach indicates consumption of jellyfish by commercially important fish species in a coastal Mediterranean lagoon. Mar. Environ. Res. 152:104787. doi: 10.1016/j.marenvres.2019.104787
Marques, R., Rufino, M., Darnaude, A. M., Carcaillet, F., Meffre, M., and Bonnet, D. (2021). Jellyfish degradation in a shallow coastal Mediterranean lagoon. Estuar. Coast. Shelf Sci. 261:107527. doi: 10.1016/j.ecss.2021.107527
Masuda, R., Yamashita, Y., and Matsuyama, M. (2008). Jack mackerel Trachurus japonicus juveniles use jellyfish for predator avoidance and as a prey collector. Fisher. Sci. 74, 276–284. doi: 10.1111/j.1444-2906.2008.01522.x
Matsushita, Y., and Honda, N. (2006). Method of designing and manufacturing JET (Jellyfish Excluder for Towed fishing gear for various towed fishing gears. Bull. Fisheries Res. Agency 16, 19–27.
Mcilwaine, B., and Casado, M. R. (2021). JellyNet: The convolutional neural network jellyfish bloom detector. Int. J. Appl. Earth Observ. Geoinf. 97:102279. doi: 10.1016/j.jag.2020.102279
Mcilwaine, B., Casado, M. R., and Waine, T. (2022). Investigating optimal unmanned aircraft systems flight plans for the detection of marine ingress. Int. J. Appl. Earth Observ. Geoinf. 108:102729. doi: 10.1016/j.jag.2022.102729
McQuatters-Gollop, A., Atkinson, A., Aubert, A., Bedford, J., Best, M., Bresnan, E., et al. (2019). Plankton lifeforms as a biodiversity indicator for regional-scale assessment of pelagic habitats for policy. Ecol. Indic. 101, 913–925. doi: 10.1016/j.ecolind.2019.02.010
Melnik, A. V., Melnik, L. A., Mashukova, O. V., and Chudinovskikh, E.lena.. (2022). Field studies of bioluminescence in Bransfield Strait in 2022. Luminescence 37, 1906–1913. doi: 10.1002/bio.4372
Mghili, B., Analla, M., and Aksissou, M. (2022). Medusae (Scyphozoa and hydrozoa) from the Moroccan Mediterranean coast: abundance and spatiotemporal dynamics and their economic impact. Aquatic Ecol. 56, 213–226. doi: 10.1007/s10452-021-09910-0
Mianzan, H., Quiñones, J., Palma, S., Schiariti, A., Acha, E. M., Robinson, K. L., et al. (2014). “Chrysaora plocamia: a poorly understood jellyfish from South American Waters,” in Jellyfish Blooms, eds. K. A. Pitt and C. H. Lucas (Dordrecht: Springer Netherlands), 219–236. doi: 10.1007/978-94-007-7015-7_10
Mills, C. E. (2001). Jellyfish blooms: are populations increasing globally in response to changing ocean conditions? Hydrobiologia 451, 55–68. doi: 10.1007/978-94-010-0722-1_6
Minamoto, T., Fukuda, M., Katsuhara, K. R., Fujiwara, A., Hidaka, S., Yamamoto, S., et al. (2017). Environmental DNA reflects spatial and temporal jellyfish distribution. PLoS ONE 12:e0173073. doi: 10.1371/journal.pone.0173073
Mitchell, S. O., Bresnihan, S., and Scholz, F. (2021). Mortality and skin pathology of farmed Atlantic salmon (Salmo salar) caused by exposure to the jellyfish Physalia physalis in Ireland. J. Fish Dis. 44, 1861–1864. doi: 10.1111/jfd.13499
Miyajima, Y., Masuda, R., Kurihara, A., Kamata, R., Yamashita, Y., and Takeuchi, T. (2011). Juveniles of threadsail filefish, Stephanolepis cirrhifer, can survive and grow by feeding on moon jellyfish Aurelia aurita. Fisher. Sci. 77, 41–48. doi: 10.1007/s12562-010-0305-8
Miyake, H., Terazaki, M., and Kakinuma, Y. (2002). On the polyps of the common jellyfish Aurelia aurita in Kagoshima Bay. J. Oceanogr. 58, 451–459. doi: 10.1023/A:1021628314041
Moher, D., Liberati, A., Tetzlaff, J., and Altman, D. G. (2009). Preferred reporting items for systematic reviews and meta-analyses: the PRISMA statement. Ann. Internal Med. 151:264. doi: 10.7326/0003-4819-151-4-200908180-00135
Møller, L. F., and Riisgård, H. U. (2007). Population dynamics, growth and predation impact of the common jellyfish Aurelia aurita and two hydromedusae, Sarsia tubulosa, and Aequorea vitrina in Limfjorden (Denmark). Mar. Ecol. Prog. Ser. 346, 153–165. doi: 10.3354/meps06960
Moura, C. J., Magalhães, B. I., and Gonçalves, J. M. (2023). DNA Barcoding of Moon jellyfish (Cnidaria, Scyphozoa, Ulmaridae, Aurelia): Two cryptic species from the Azores (NE Atlantic, Macaronesia), and evaluation of the non-Indigenous species (NIS). Diversity 15:323. doi: 10.3390/d15030323
Nagata, R. M., Moreira, M. Z., Pimentel, C. R., and Morandini, A. C. (2015). Food web characterization based on δ15N and δ13C reveals isotopic niche partitioning between fish and jellyfish in a relatively pristine ecosystem. Mar. Ecol. Prog. Ser. 519, 13–27. doi: 10.3354/meps11071
Ndah, A. B., Meunier, C. L., Kirstein, I. V., Göbel, J., Rönn, L., and Boersma, M. (2022). A systematic study of zooplankton-based indices of marine ecological change and water quality: application to the European marine strategy framework Directive (MSFD). Ecol. Indic. 135:108587. doi: 10.1016/j.ecolind.2022.108587
Niemeijer, D., and De Groot, R. S. (2008). A conceptual framework for selecting environmental indicator sets. Ecol. Indic. 8, 14–25. doi: 10.1016/j.ecolind.2006.11.012
Nowaczyk, A., David, V., Lepage, M., Goarant, A., De Oliveira, É., and Sautour, B. (2016). Spatial and temporal patterns of occurrence of three alien hydromedusae, Blackfordia virginica (Mayer, 1910), Nemopsis bachei (Agassiz, 1849) and Maeotias marginata (Modeer, 1791), in the Gironde Estuary (France). Aquatic Invasions 11, 397–409. doi: 10.3391/ai.2016.11.4.05
Nudelman, R., Alhmoud, H., Delalat, B., Fleicher, S., Fine, E., Guliakhmedova, T., et al. (2019). Jellyfish-based smart wound dressing devices containing in situ synthesized antibacterial nanoparticles. Adv. Funct. Mater. 29:1902783. doi: 10.1002/adfm.201902783
Nygård, H., Oinonen, S., Hällfors, H. A., Lehtiniemi, M., Rantajärvi, E., and Uusitalo, L. (2016). Price vs. value of marine monitoring. Front. Mar. Sci. 3:205. doi: 10.3389/fmars.2016.00205
Ogata, M., Masuda, R., Harino, H., Sakata, M. K., Hatakeyama, M., Yokoyama, K., et al. (2021). Environmental DNA preserved in marine sediment for detecting jellyfish blooms after a tsunami. Sci. Rep. 11:16830. doi: 10.1038/s41598-021-94286-2
Omori, M., and Nakano, E. (2001). Jellyfish fisheries in southeast Asia. Hydrobiologia 451, 19–26. doi: 10.1023/A:1011879821323
OSPAR (2017). “PH1/FW5: Changes in phytoplankton and zooplankton communities,” in OSPAR Intermediate Assessment OSPAR, 2017, ed. OSPAR (London UK). Available at: https://oap.ospar.org/en/ospar-assessments/intermediate-assessment-2017/biodiversity-status/habitats/changes-phytoplankton-and-zooplankton-communities/ (accessed September 6, 2023).
Page, J. W. (2015). Characterization of bycatch in the cannonball jellyfish fishery in the coastal waters off Georgia. Mar. Coastal Fisher. 7, 190–199. doi: 10.1080/19425120.2015.1032456
Palialexis, A., Kousteni, V., Boicenco, L., Enserink, L., Pagou, K., Zweifel, U. L., et al. (2021). Monitoring biodiversity for the EU marine strategy framework directive: lessons learnt from evaluating the official reports. Marine Policy 128:104473. doi: 10.1016/j.marpol.2021.104473
Parsons, T. R., and Lalli, C. M. (2002). Jellyfish population explosions: revisiting a hypothesis of possible causes. La mer 40, 111–121.
Patwa, A., Thiéry, A., Lombard, F., Lilley, M. K. S., Boisset, C., Bramard, J.-F., et al. (2015). Accumulation of nanoparticles in “jellyfish” mucus: a bio-inspired route to decontamination of nano-waste. Sci. Rep. 5:11387. doi: 10.1038/srep11387
Pedersen, M. T., and Vilgis, T. A. (2019). Soft matter physics meets the culinary arts: From polymers to jellyfish. Int. J. Gastr. Food Sci. 16:100135, doi: 10.1016/j.ijgfs.2019.100135
Pereira, R., Teodósio, M. A., and Garrido, S. (2014). An experimental study of Aurelia aurita feeding behaviour: inference of the potential predation impact on a temperate estuarine nursery area. Estuar. Coast. Shelf Sci. 146, 102–110. doi: 10.1016/j.ecss.2014.05.026
Pérez-Ruzafa, A., Campillo, S., Fernández-Palacios, J. M., García-Lacunza, A., García-Oliva, M., Ibañez, H., et al. (2019). Long-term dynamic in nutrients, chlorophyll a, and water quality parameters in a coastal lagoon during a process of eutrophication for decades, a sudden break and a relatively rapid recovery. Front. Mar. Sci. 6:26. doi: 10.3389/fmars.2019.00026
Pérez-Ruzafa, A., Gilabert, J., Gutiérrez, J. M., Fernández, A. I., Marcos, C., and Sabah, S. (2002). “Evidence of a planktonic food web response to changes in nutrient input dynamics in the Mar Menor coastal lagoon, Spain,” in Nutrients and Eutrophication in Estuaries and Coastal Waters, eds. E. Orive, M. Elliott, and V. N. De Jonge (Dordrecht: Springer Netherlands), 359–369. doi: 10.1007/978-94-017-2464-7_26
Pikesley, S. K., Godley, B. J., Ranger, S., Richardson, P. B., and Witt, M. J. (2014). Cnidaria in UK coastal waters: description of spatio-temporal patterns and inter-annual variability. J. Mar. Biol. Assoc. UK 94, 1401–1408. doi: 10.1017/S0025315414000137
Piraino, S., Deidun, A., Fuentes, V., Daly Yahia, M. N., Daly Yahia, O., Marambio, M., et al. (2016). “Are anti-jellyfish nets a useful mitigation tool for coastal tourism? Hindsight from the MED-JELLYRISK experience,” in 5th International Jellyfish Bloom Symposium in Barcelona, May 30-June 3, 16. Available at: https://digital.csic.es/bitstream/10261/162327/1/Piraino_et_al_2016.pdf (accessed February 20, 2024).
Pitt, K. A., and Kingsford, M. J. (2003). Temporal and spatial variation in recruitment and growth of medusae of the jellyfish, Catostylus mosaicus (Scyphozoa: Rhizostomeae). Mar. Freshwater Res. 54, 117–125. doi: 10.1071/MF02110
Pitt, K. A., Lucas, C. H., Condon, R. H., Duarte, C. M., and Stewart-Koster, B. (2018). Claims that anthropogenic stressors facilitate jellyfish blooms have been amplified beyond the available evidence: a systematic review. Front. Mar. Sci. 5:451. doi: 10.3389/fmars.2018.00451
Powell, M. D., Åtland, Å., and Dale, T. (2018). Acute lion's mane jellyfish, Cyanea capillata (Cnideria: Scyphozoa), exposure to Atlantic salmon (Salmo salar L.). J. Fish Dis. 41, 751–759. doi: 10.1111/jfd.12771
Purcell, J. E. (1992). Effects of predation by the scyphomedusan Chrysaora quinquecirrha on zooplankton populations in Chesapeake Bay, USA. Mar. Ecol. Prog. Ser. 87, 65–76. doi: 10.3354/meps087065
Purcell, J. E. (2005). Climate effects on formation of jellyfish and ctenophore blooms: a review. J. Mar. Biol. Assoc. UK 85, 461–476. doi: 10.1017/S0025315405011409
Purcell, J. E. (2009). Extension of methods for jellyfish and ctenophore trophic ecology to large-scale research. Hydrobiologia 616, 23–50. doi: 10.1007/s10750-008-9585-8
Purcell, J. E. (2012). Jellyfish and ctenophore blooms coincide with human proliferations and environmental perturbations. Ann. Rev. Mar. Sci. 4, 209–235. doi: 10.1146/annurev-marine-120709-142751
Purcell, J. E., and Arai, M. N. (2001). Interactions of pelagic cnidarians and ctenophores with fish: a review. Hydrobiologia 45, 27–44. doi: 10.1023/A:1011883905394
Purcell, J. E., Breitburg, D. L., Decker, M. B., Graham, W. M., Youngbluth, M. J., and Raskoff, K. A. (2001). “Pelagic cnidarians and ctenophores in low dissolved oxygen environments: A review,” in Coastal hypoxia: consequences for living resources and ecosystems, eds. N. N. Rabalais and R. E. Turner (Washington, DC: American Geophysical Union), 77–100. doi: 10.1029/CE058p0077
Purcell, J. E., and Grover, J. J. (1990). Predation and food limitation as causes of mortality in larval herring at a spawning ground in British Columbia. Mar. Ecol. Prog. Ser. 59, 55–61. doi: 10.3354/meps059055
Purcell, J. E., Hoover, R. A., and Schwarck, N. T. (2009). Interannual variation of strobilation by the scyphozoan Aurelia labiata in relation to polyp density, temperature, salinity, and light conditions in situ. Mar. Ecol. Prog. Ser. 375, 139–149. doi: 10.3354/meps07785
Purcell, J. E., Uye, S., and Lo, W.-T. (2007). Anthropogenic causes of jellyfish blooms and their direct consequences for humans: a review. Mar. Ecol. Prog. Ser. 350, 153–174. doi: 10.3354/meps07093
Quiñones, J., Monroy, A., Acha, E. M., and Mianzan, H. (2013). Jellyfish bycatch diminishes profit in an anchovy fishery off Peru. Fish. Res. 139, 47–50. doi: 10.1016/j.fishres.2012.04.014
R Core Team (2020). R: A language and environment for statistical computing. R Foundation for Statistical Computing. Available at: https://www.R-project.org (accessed December, 2022).
Racault, M.-F., Sathyendranath, S., and Platt, T. (2014). Impact of missing data on the estimation of ecological indicators from satellite ocean-colour time-series. Remote Sens. Environ. 152, 15–28. doi: 10.1016/j.rse.2014.05.016
Rahi, J. E., Weeber, M. P., and Serafy, G. E. (2020). Modelling the effect of behavior on the distribution of the jellyfish Mauve stinger (Pelagia noctiluca) in the Balearic Sea using an individual-based model. Ecol. Modell. 433:109230. doi: 10.1016/j.ecolmodel.2020.109230
Ramires, F. A., Bleve, G., De Domenico, S., and Leone, A. (2022a). Combination of solid state and submerged fermentation strategies to produce a new jellyfish-based food. Foods 11:3974. doi: 10.3390/foods11243974
Ramires, F. A., De Domenico, S., Migoni, D., Fanizzi, F. P., Angel, D. L., Slizyte, R., et al. (2022b). Optimization of a calcium-based treatment method for jellyfish to design food for the future. Foods 11:2697. doi: 10.3390/foods11172697
Ramondenc, S., Eveillard, D., Guidi, L., Lombard, F., and Delahaye, B. (2020). Probabilistic modeling to estimate jellyfish ecophysiological properties and size distributions. Sci. Rep. 10:6074. doi: 10.1038/s41598-020-62357-5
Ranasinghe, R. A. S. N., Wijesekara, W. L. I., Perera, P. R. D., Senanayake, S. A., Pathmalal, M. M., and Marapana, R. A. U. J. (2022). Nutritional value and potential applications of jellyfish. J. Aquat. Food Product Technol. 31, 445–482. doi: 10.1080/10498850.2022.2060717
Raoult, V., and Gaston, T. F. (2018). Rapid biomass and size-frequency estimates of edible jellyfish populations using drones. Fish. Res. 207, 160–164. doi: 10.1016/j.fishres.2018.06.010
Raposo, A., Alasqah, I., Alfheeaid, H. A., Alsharari, Z. D., Alturki, H. A., and Raheem, D. (2022). Jellyfish as food: a narrative review. Foods 11:2773. doi: 10.3390/foods11182773
Rastian, Z., Pütz, S., Wang, Y., Kumar, S., Fleissner, F., Weidner, T., et al. (2018). Type I collagen from jellyfish Catostylus mosaicus for biomaterial applications. ACS Biomater. Sci. Eng. 4, 2115–2125. doi: 10.1021/acsbiomaterials.7b00979
Ratcliff, J. (2004). Mulroy Bay plankton monitoring, May to September 2004. Marine Harvest Ireland Report. Kindrum, Co, Donegal, Ireland, 12.
Rekstad, M. E., Majaneva, S., Borgersen, Å. L., and Aberle, N. (2021). Occurrence and habitat characteristics of Aurelia sp. polyps in a high-latitude fjord. Front. Mar. Sci. 8:684634. doi: 10.3389/fmars.2021.684634
Reyes Suárez, N. C., Tirelli, V., Ursella, L., Ličer, M., Celio, M., and Cardin, V. (2022). Multi-platform study of the extreme bloom of the barrel jellyfish Rhizostoma pulmo (Cnidaria: Scyphozoa) in the northernmost gulf of the Mediterranean Sea (Gulf of Trieste) in April 2021. Ocean Sci. 18, 1321–1337. doi: 10.5194/os-18-1321-2022
Richardson, A. J., Bakun, A., Hays, G. C., and Gibbons, M. J. (2009). The jellyfish joyride: causes, consequences and management responses to a more gelatinous future. Trends Ecol. Evol. 24, 312–322. doi: 10.1016/j.tree.2009.01.010
Riisgård, H. U., Jaspers, C., Serre, S., and Lundgreen, K. (2012). Occurrence, inter-annual variability and zooplankton-predation impact of the invasive ctenophore Mnemiopsis leidyi and the native jellyfish Aurelia aurita in Limfjorden (Denmark) in 2010 and 2011. Bioinvasions Rec. 1, 145–159. doi: 10.3391/bir.2012.1.3.01
Rogers, S., Casini, M., Cury, P., Heath, M., Irigoien, X., Kuosa, H., et al. (2010). Marine Strategy Framework Directive: Task Group 4 Report: food webs. Office for Official Publications of the European Communities, EUR 24343 EN – Joint Research Centre, Luxembourg, 63. Available at: https://publications.jrc.ec.europa.eu/repository/handle/JRC58110 (accessed February 4, 2024).
Roux, J.-P., Van Der Lingen, C. D., Gibbons, M. J., Moroff, N. E., Shannon, L. J., Smith, A. D., et al. (2013). Jellyfication of marine ecosystems as a likely consequence of overfishing small pelagic fishes: Lessons from the Benguela. Bull. Mar. Sci. 89, 249–284. doi: 10.5343/bms.2011.1145
Ruiz-Frau, A. (2023). Impacts of jellyfish presence on tourists' holiday destination choices and their willingness to pay for mitigation measures. J. Environ. Plan. Manag. 66, 2107–2125. doi: 10.1080/09640568.2022.2061926
Russell, F. S. (1953). The Medusae of the British Isles Vol. I: Anthomedusae, Leptomedusae, Limnomedusae, Trachymedusae, and Narcomedusae. E. T. Browne Monograph of the Marine Biological Association of the United Kingdom. New York: Cambridge Univ. Press, 530.
Russell, F. S. (1970). The Medusae of the British Isles volume II: Pelagic Scyphozoa, with a supplement to the first volume of Hydromedusae. New York: Cambridge Univ. Press, 284.
Sato, N. N., Kokubun, N., Yamamoto, T., Watanuki, Y., Kitaysky, A. S., and Takahashi, A. (2015). The jellyfish buffet: jellyfish enhance seabird foraging opportunities by concentrating prey. Biol. Lett. 11:20150358. doi: 10.1098/rsbl.2015.0358
Schaub, J., Hunt, B., Pakhomov, E., Holmes, K., Lu, Y., and Quayle, L. (2018). Using unmanned aerial vehicles (UAVs) to measure jellyfish aggregations. Mar. Ecol. Prog. Ser. 591, 29–36. doi: 10.3354/meps12414
Schneider, G., and Behrends, G. (1998). Top-down control in a neritic plankton system by Aurelia aurita medusae—a summary. Ophelia 48, 71–82. doi: 10.1080/00785236.1998.10428677
Scorrano, S., Aglieri, G., Boero, F., Dawson, M. N., and Piraino, S. (2017). Unmasking Aurelia species in the Mediterranean Sea: an integrative morphometric and molecular approach. Zool. J. Linn. Soc. 180, 243–267. doi: 10.1111/zoj.12494
Seo, Y., Muhammad, B. L., Chae, J., and Ki, J.-S. (2021). Population genetic structures of the jellyfish Aurelia coerulea polyps along Korean coasts and implications as revealed by mitochondrial COI. Zool. Stud. 60:63. doi: 10.6620/ZS.2021.60-63
Shiganova, T. (2005). Changes in appendicularian Oikopleura dioica abundance caused by invasion of alien ctenophores in the Black Sea. J. Mar. Biol. Assoc. UK 85, 477–494. doi: 10.1017/S0025315405011410
Shiganova, T., Mirzoyan, Z., Studenikina, E., Volovik, S., Siokou-Frangou, I., Zervoudaki, S., et al. (2001). Population development of the invader ctenophore Mnemiopsis leidyi, in the Black Sea and in other seas of the Mediterranean basin. Mar. Biol. 139, 431–445. doi: 10.1007/s002270100554
Shiganova, T. A. (1998). Invasion of the Black Sea by the ctenophore Mnemiopsis leidyi and recent changes in pelagic community structure. Fisheries Oceanogr. 7, 305–310. doi: 10.1046/j.1365-2419.1998.00080.x
Shiganova, T. A., Mikaelyan, A. S., Moncheva, S., Stefanova, K., Chasovnikov, V. K., Mosharov, S. A., et al. (2019). Effect of invasive ctenophores Mnemiopsis leidyi and Beroe ovata on low trophic webs of the Black Sea ecosystem. Mar. Pollut. Bull. 141, 434–447. doi: 10.1016/j.marpolbul.2019.02.049
Shimomura, O., Johnson, F. H., and Saiga, Y. (1962). Extraction, purification and pProperties of aequorin, a bioluminescent protein from the luminous hydromedusan, Aequorea. J. Cell. Comp. Physiol. 59, 223–239. doi: 10.1002/jcp.1030590302
Shoji, J., Kudoh, T., Takatsuji, H., Kawaguchi, O., and Kasai, A. (2010). Distribution of Moon jellyfish Aurelia aurita in relation to summer hypoxia in Hiroshima Bay, Seto Inland Sea. Estuar. Coast. Shelf Sci. 86, 485–490. doi: 10.1016/j.ecss.2009.03.001
Smith, B. E., Ford, M. D., and Link, J. S. (2016). Bloom or bust: synchrony in jellyfish abundance, fish consumption, benthic scavenger abundance, and environmental drivers across a continental shelf. Fisher. Oceanogr. 25, 500–514. doi: 10.1111/fog.12168
Song, X., Lyu, M., Zhang, X., Ruthensteiner, B., Ahn, I.-Y., Pastorino, G., et al. (2021). Large plastic debris dumps: New biodiversity hot spots emerging on the deep-sea floor. Environ. Sci. Technol. Lett. 8, 148–154. doi: 10.1021/acs.estlett.0c00967
Spalding, M. D., Fox, H. E., Allen, G. R., Davidson, N., Ferdaña, Z. A., Finlayson, M., et al. (2007). Marine ecoregions of the World: A bioregionalization of coastal and shelf areas. Bioscience 57, 573–583. doi: 10.1641/B570707
Spanier, E., and Galil, B. S. (1991). Lessepsian migration: a continuous biogeographical process. Endeavour 15, 102–106. doi: 10.1016/0160-9327(91)90152-2
Stabili, L., Rizzo, L., Basso, L., Marzano, M., Fosso, B., Pesole, G., et al. (2020). The Microbial community associated with Rhizostoma pulmo: Ecological significance and potential consequences for marine organisms and human health. Mar. Drugs 18:437. doi: 10.3390/md18090437
Stabili, L., Rizzo, L., Caprioli, R., Alabiso, G., and Piraino, S. (2022). How much recurrent outbreaks of the Moon jellyfish may impact the dynamics of bacterial assemblages in coastal lagoons? Water 14:3908. doi: 10.3390/w14233908
Steinberger, L. R., Gulakhmedova, T., Barkay, Z., Gozin, M., and Richter, S. (2019). Jellyfish-based plastic. Adv. Sustain. Syst. 3:1900016. doi: 10.1002/adsu.201900016
Stoner, E. W., Yeager, L. A., Sweatman, J. L., Sebilian, S. S., and Layman, C. A. (2014). Modification of a seagrass community by benthic jellyfish blooms and nutrient enrichment. J. Exp. Mar. Biol. Ecol. 461, 185–192. doi: 10.1016/j.jembe.2014.08.005
Sullivan, L. J., and Gifford, D. J. (2004). Diet of the larval ctenophore Mnemiopsis leidyi A. Agassiz (Ctenophora, Lobata). J. Plankton Research 26, 417–431. doi: 10.1093/plankt/fbh033
Sweetman, A., and Chapman, A. (2011). First observations of jelly-falls at the seafloor in a deep-sea fjord. Deep Sea Res. Part I 58, 1206–1211. doi: 10.1016/j.dsr.2011.08.006
Sweetman, A., and Chapman, A. (2015). First assessment of flux rates of jellyfish carcasses (jelly-falls) to the benthos reveals the importance of gelatinous material for biological C-cycling in jellyfish-dominated ecosystems. Front. Mar. Sci. 2:47. doi: 10.3389/fmars.2015.00047
Sweetman, A. K., Chelsky, A., Pitt, K. A., Andrade, H., van Oevelen, D., and Renaud, P. E. (2016). Jellyfish decomposition at the seafloor rapidly alters biogeochemical cycling and carbon flow through benthic food-webs. Limnol. Oceanogr. 61, 1449–1461. doi: 10.1002/lno.10310
Syazwan, W. M., Rizman-Idid, M., Low, L. B., Then, A. Y.-H., and Chong, V. C. (2020). Assessment of scyphozoan diversity, distribution and blooms: Implications of jellyfish outbreaks to the environment and human welfare in Malaysia. Regional Studies in Mar. Sci. 39:101444. doi: 10.1016/j.rsma.2020.101444
Templeman, M. A., McKenzie, M. R., and Kingsford, M. J. (2021). The utility of jellyfish as marine biomonitors. Mar. Pollut. Bull. 173:113056. doi: 10.1016/j.marpolbul.2021.113056
Tett, P., Carreira, C., Mills, D. K., Van Leeuwen, S., Foden, J., Bresnan, E., et al. (2008). Use of a Phytoplankton Community Index to assess the health of coastal waters. ICES J. Mar. Sci. 65, 1475–1482. doi: 10.1093/icesjms/fsn161
Thiebot, J. B., and McInnes, J. C. (2020). Why do marine endotherms eat gelatinous prey? ICES J. Mar. Sci. 77, 58–71. doi: 10.1093/icesjms/fsz208
Tilves, U., Purcell, J. E., Fuentes, V. L., Torrents, A., Pascual, M., Raya, V., et al. (2016). Natural diet and predation impacts of Pelagia noctiluca on fish eggs and larvae in the NW Mediterranean. J. Plankton Res. 38, 1243–1254. doi: 10.1093/plankt/fbw059
Tilves, U., Sabatés, A., Blázquez, M., Raya, V., and Fuentes, V. L. (2018). Associations between fish and jellyfish in the NW Mediterranean. Mar. Biol. 165:127. doi: 10.1007/s00227-018-3381-4
Tinta, T., Kogovšek, T., Malej, A., and Turk, V. (2012). Jellyfish modulate bacterial dynamic and community structure. PLoS ONE 7:e39274. doi: 10.1371/journal.pone.0039274
Tinta, T., Kogovšek, T., Turk, V., Shiganova, T. A., Mikaelyan, A. S., and Malej, A. (2016). Microbial transformation of jellyfish organic matter affects the nitrogen cycle in the marine water column—A Black Sea case study. J. Exp. Mar. Biol. Ecol. 475, 19–30. doi: 10.1016/j.jembe.2015.10.018
Tiselius, P., and Møller, L. F. (2017). Community cascades in a marine pelagic food web controlled by the non-visual apex predator Mnemiopsis leidyi. J. Plankton Res. 39, 271–279. doi: 10.1093/plankt/fbw096
Tornero Alvarez, M. V., Palma, M., Boschetti, S., Cardoso, A. C., Druon, J., Kotta, M., et al. (2023). Marine Strategy Framework Directive: review and analysis of EU Member States' 2020 reports on monitoring programmes: MSFD Article 11. EUR 31181 EN, Publications Office of the European Union, Luxembourg, 282. Available at: https://data.europa.eu/doi/10.2760/8457 (accessed February 4, 2024).
Torri, L., Tuccillo, F., Bonelli, S., Piraino, S., and Leone, A. (2020). The attitudes of Italian consumers towards jellyfish as novel food. Food Qual. Prefer. 79:103782. doi: 10.1016/j.foodqual.2019.103782
Torti, A., Lever, M. A., and Jørgensen, B. B. (2015). Origin, dynamics, and implications of extracellular DNA pools in marine sediments. Mar. Genomics 24, 185–196. doi: 10.1016/j.margen.2015.08.007
Toyokawa, M. (2011). First record of wild polyps of Chrysaora pacifica (Goette, 1886) (Scyphozoa, Cnidaria). Plankton Benthos Res. 6, 175–177. doi: 10.3800/pbr.6.175
Toyokawa, M., Aoki, K., Yamada, S., Yasuda, A., Murata, Y., and Kikuchi, T. (2011). Distribution of ephyrae and polyps of jellyfish Aurelia aurita (Linnaeus 1758) sensu lato in Mikawa Bay, Japan. J. Oceanogr. 67, 209–218. doi: 10.1007/s10872-011-0021-8
Tsirintanis, K., Azzurro, E., Crocetta, F., Dimiza, M., Froglia, C., Gerovasileiou, V., et al. (2022). Bioinvasion impacts on biodiversity, ecosystem services, and human health in the Mediterranean Sea. Aquatic Invasions 17, 308–352. doi: 10.3391/ai.2022.17.3.01
Turk, T., and Kem, W. R. (2009). The phylum Cnidaria and investigations of its toxins and venoms until 1990. Toxicon 54, 1031–1037. doi: 10.1016/j.toxicon.2009.06.031
Uye, S. (2008). Blooms of the giant jellyfish Nemopilema nomurai: a threat to the fisheries sustainability of the East Asian Marginal Seas. Plankton Benthos Res. 3, 125–131. doi: 10.3800/pbr.3.125
Van Walraven, L., Daan, R., Langenberg, V., and Van Der Veer, H. (2017). Species composition and predation pressure of the gelatinous zooplankton community in the western Dutch Wadden Sea before and after the invasion of the ctenophore Mnemiopsis leidyi A. Agassiz, 1865. Aquatic Invasions 12, 5–21. doi: 10.3391/ai.2017.12.1.02
Van Walraven, L., Driessen, F., Van Bleijswijk, J., Bol, A., Luttikhuizen, P. C., Coolen, J. W. P., et al. (2016). Where are the polyps? Molecular identification, distribution and population differentiation of Aurelia aurita jellyfish polyps in the southern North Sea area. Mar. Biol. 163:172. doi: 10.1007/s00227-016-2945-4
Van Walraven, L., Langenberg, V. T., Dapper, R., Witte, J., Zuur, A. F., et al. (2015). Long-term patterns in 50 years of scyphomedusae catches in the western Dutch Wadden Sea in relation to climate change and eutrophication. J. Plankton Res. 37, 151–167. doi: 10.1093/plankt/fbu088
Van Walraven, L., Langenberg, V. T., and Van Der Veer, H. W. (2013). Seasonal occurrence of the invasive ctenophore Mnemiopsis leidyi in the western Dutch Wadden Sea. J. Sea Res. 82, 86–92. doi: 10.1016/j.seares.2013.02.003
Van Walraven, L., Van Bleijswijk, J., and Van Der Veer, H. W. (2020). Here are the polyps: in situ observations of jellyfish polyps and podocysts on bivalve shells. PeerJ 8:e9260. doi: 10.7717/peerj.9260
Verner, B. (1984). “Jellyfish flotation by means of bubble barriers to prevent blockage of cooling water supply and a proposal for a semi-mechanical barrier to protect bathing beaches from jellyfish,” in Proceedings Workshop jellyfish blooms (Athens, Greece), 1–10.
Vineetha, G., Kripa, V., Karati, K. K., Madhu, N. V., Anil, P., and Vishnu Nair, M. S. (2022). Surge in the jellyfish population of a tropical monsoonal estuary: a boon or bane to its plankton community dynamics? Mar. Pollut. Bull. 182:113951. doi: 10.1016/j.marpolbul.2022.113951
Vodopivec, M., Mandeljc, R., Makovec, T., Malej, A., and Kristan, M. (2018). Towards automated scyphistoma census in underwater imagery: a useful research and monitoring tool. J. Sea Res. 142, 147–156. doi: 10.1016/j.seares.2018.09.014
Wakabayashi, K., Sato, R., Ishii, H., Akiba, T., Nogata, Y., and Tanaka, Y. (2012). Culture of phyllosomas of Ibacus novemdentatus (Decapoda: Scyllaridae) in a closed recirculating system using jellyfish as food. Aquaculture 330, 162–166. doi: 10.1016/j.aquaculture.2011.12.005
Wang, P., Zhang, F., Sun, S., Wang, W., Wan, A., and Li, C. (2020). Experimental clearance rates of Aurelia coerulea ephyrae and medusae, and the predation impact on zooplankton in Jiaozhou Bay. J. Oceanol. Limnol. 38, 1256–1269. doi: 10.1007/s00343-020-0024-7
Wells, S. R., Bresnan, E., Cook, K., Eerkes-Medrano, D., Machairopoulou, M., Mayor, D. J., et al. (2022). Environmental drivers of a decline in a coastal zooplankton community. ICES J. Mar. Sci. 79, 844–854. doi: 10.1093/icesjms/fsab177
West, E. J., Pitt, K. A., Welsh, D. T., Koop, K., and Rissik, D. (2009a). Top-down and bottom-up influences of jellyfish on primary productivity and planktonic assemblages. Limnol. Oceanogr. 54, 2058–2071. doi: 10.4319/lo.2009.54.6.2058
West, E. J., Welsh, D. T., and Pitt, K. A. (2009b). Influence of decomposing jellyfish on the sediment oxygen demand and nutrient dynamics. Hydrobiologia 616, 151–160. doi: 10.1007/s10750-008-9586-7
Wickham, H. (2016). ggplot2: Elegant Graphics for Data Analysis. New York: Springer-Verlag. doi: 10.1007/978-3-319-24277-4
Widdowson, J. P., Picton, A. J., Vince, V., Wright, C. J., and Mearns-Spragg, A. (2018). In vivo comparison of jellyfish and bovine collagen sponges as prototype medical devices. J. Biomed. Mater. Res. Part B 106B, 1524–1533. doi: 10.1002/jbm.b.33959
Witt, M., Broderick, A., Johns, D., Martin, C., Penrose, R., Hoogmoed, M., et al. (2007). Prey landscapes help identify potential foraging habitats for leatherback turtles in the NE Atlantic. Mar. Ecol. Prog. Ser. 337, 231–243. doi: 10.3354/meps337231
Xian, W., Kang, B., and Liu, R. (2005). Jellyfish blooms in the Yangtze estuary. Science 307:41. doi: 10.1126/science.307.5706.41c
Yilmaz, I. N. (2015). Collapse of zooplankton stocks during Liriope tetraphylla (Hydromedusa) blooms and dense mucilaginous aggregations in a thermohaline stratified basin. Marine Ecol. 36, 595–610. doi: 10.1111/maec.12166
Yoon, W., Chae, J., Koh, B.-S., and Han, C. (2018). Polyp removal of a bloom forming jellyfish, Aurelia coerulea, in Korean waters and its value evaluation. Ocean Sci. J. 53, 499–507. doi: 10.1007/s12601-018-0015-1
Youssef, J., Keller, S., and Spence, C. (2019). Making sustainable foods (such as jellyfish) delicious. Int. J. Gastr. Food Sci. 16:100141. doi: 10.1016/j.ijgfs.2019.100141
Yu, H., Li, R., Dong, X., Xing, R., Liu, S., and Li, P. (2014). Efficacy of venom from tentacle of jellyfish Stomolophus meleagris (Nemopilema nomurai) against the Cotton Bollworm Helicoverpa armigera. Biomed. Res. Int. 2014:315853. doi: 10.1155/2014/315853
Yu, H., Li, R., Wang, X., Yue, Y., Liu, S., Xing, R., et al. (2021). Field experiment effect on citrus spider mite Panonychus citri of venom from jellyfish Nemopilema nomurai: the potential use of jellyfish in agriculture. Toxins 13:411. doi: 10.3390/toxins13060411
Yu, H., Liu, X., Dong, X., Li, C., Xing, R., Liu, S., et al. (2005). Insecticidal activity of proteinous venom from tentacle of jellyfish Rhopilema esculentum Kishinouye. Bioorg. Med. Chem. Lett. 15, 4949–4952. doi: 10.1016/j.bmcl.2005.08.015
Yu, H., Yue, Y., Dong, X., Li, R., and Li, P. (2016). The acaricidal activity of venom from the jellyfish Nemopilema nomurai against the carmine spider mite Tetranychus cinnabarinus. Toxins 8 :179. doi: 10.3390/toxins8060179
Yue, M., Mi, T., Li, Y., Gong, X., and Zhen, Y. (2023). Molecular monitoring plankton community during jellyfish blooms in the water near the Liaoning Hongyan River Nuclear Power Plant. J. Oceanol. Limnol. 41, 1781–1794. doi: 10.1007/s00343-022-1450-5
Zaitsev, Y. P. (1992). Recent changes in the trophic structure of the Black Sea. Fisher. Oceanogr. 1, 180–189. doi: 10.1111/j.1365-2419.1992.tb00036.x
Zeman, S. M., Corrales-Ugalde, M., Brodeur, R. D., and Sutherland, K. R. (2018). Trophic ecology of the neustonic cnidarian Velella velella in the northern California Current during an extensive bloom year: insights from gut contents and stable isotope analysis. Mar. Biol. 165, 1–13. doi: 10.1007/s00227-018-3404-1
Zhang, W., Rui, F., Xiao, C., Li, H., and Li, Y. (2023). JF-YOLO: the jellyfish bloom detector based on deep learning. Multim. Tools Applic. 83, 7097–7117. doi: 10.1007/s11042-023-15465-z
Zhulay, I., Iken, K., Renaud, P. E., and Bluhm, B. A. (2019). Epifaunal communities across marine landscapes of the deep Chukchi Borderland (Pacific Arctic). Deep Sea Res. Part I 151:103065. doi: 10.1016/j.dsr.2019.06.011
Keywords: Cnidaria, Ctenophora, pelagic tunicates, impacts, monitoring, ecosystem based management approach (EBMA)
Citation: Sagarminaga Y, Piraino S, Lynam CP, Leoni V, Nikolaou A, Jaspers C, Bosch-Belmar M, Fumarola LM, Borja, Spada E, Amorim E, Borrello P, de Angelis R, Leone A, Montero N, Ferrer L, Holland MM, Doyle TK, Tsirtsis G and Katsanevakis S (2024) Management of jellyfish outbreaks to achieve good environmental status. Front. Ocean Sustain. 2:1449190. doi: 10.3389/focsu.2024.1449190
Received: 14 June 2024; Accepted: 02 September 2024;
Published: 25 September 2024.
Edited by:
Periklis Kleitou, Marine and Environmental Research Lab (MER), CyprusReviewed by:
Dror L. Angel, University of Haifa, IsraelVictoria Dominguez Almela, University of Southampton, United Kingdom
Copyright © 2024 Sagarminaga, Piraino, Lynam, Leoni, Nikolaou, Jaspers, Bosch-Belmar, Fumarola, Borja, Spada, Amorim, Borrello, de Angelis, Leone, Montero, Ferrer, Holland, Doyle, Tsirtsis and Katsanevakis. This is an open-access article distributed under the terms of the Creative Commons Attribution License (CC BY). The use, distribution or reproduction in other forums is permitted, provided the original author(s) and the copyright owner(s) are credited and that the original publication in this journal is cited, in accordance with accepted academic practice. No use, distribution or reproduction is permitted which does not comply with these terms.
*Correspondence: Yolanda Sagarminaga, ysagarminaga@azti.es