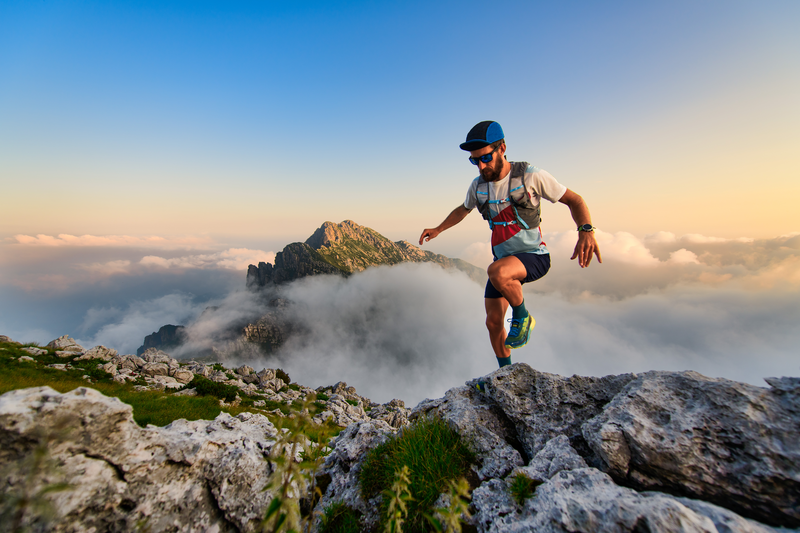
95% of researchers rate our articles as excellent or good
Learn more about the work of our research integrity team to safeguard the quality of each article we publish.
Find out more
PERSPECTIVE article
Front. Ocean Sustain. , 20 October 2023
Sec. Marine Pollution Assessments and Solutions
Volume 1 - 2023 | https://doi.org/10.3389/focsu.2023.1281484
Human activities have significantly impacted our natural environments, resulting in extensive degradation, with chemical contamination emerging as a critical concern. This type of pollution has far-reaching implications, affecting ecological health and socioeconomic dimensions. The concept of “contaminants of emerging concern” encompasses newly recognized environmental pollutants, highlighting their diverse and often understudied effects. This category includes microplastics, antibiotics, pharmaceuticals, and metals, among others, many of which lack adequate regulations and understanding of their ecological and human impacts. A subset of these pollutants, termed Technology-Critical Elements (TCEs), holds significance in high-tech industries but also poses environmental challenges due to increased extraction, manufacturing, and disposal. Aquatic environments, the ultimate chemical contaminant sinks, require heightened attention with regard to TCE dynamics and effects. Despite increasing research on TCEs in various aquatic taxa, a lack of comprehensive data hinders further knowledge assessments. In this scenario, fish, key environmental health indicators and links to human contamination, demand focused studies. Cartilaginous fish, specifically, are often overlooked, although ecologically and economically significant, warranting research into TCE effects. This type of research displays the potential to shed light on TCE dynamics, ecological outcomes, and guide conservation strategies for these species and their habitat, also aligning with the One Health concept, acknowledging the interconnectedness of humans, animals, and the environment and contributing to SDG goals, underpinning marine ecosystem conservation.
The Anthropocene has led to the massive degradation of our natural environments in many ways. Chemical contamination stands out as one of the main environmental issues of our times, as millions of harmful compounds are now widespread throughout all environmental compartments, i.e., air, water, soil, and biota, underscoring the magnitude of this problem. This matter has far-reaching implications, from many unforeseen health consequences to living organisms to direct reflections on socioeconomic and public-health dimensions.
Within the chemical contamination scenario, the term “contaminants of emerging concern” is often used interchangeably with the term “emerging contaminants,” usually applied to new synthetic molecules which have been recently identified as environmental pollutants (Khan et al., 2022). This expression, however, may also be applied in an alternative context, referring, instead, to recent concerns due to new and emerging uses of well-known compounds, their detections at higher-than-expected concentrations and the deleterious effects and risks they may pose to both environmental and human health (OECD, 2018; Batley and Campbell, 2022).
Contaminants of emerging concern include many different chemical classes, such as microplastics, antibiotics, drugs, Pharmaceuticals and Personal Care Products (PPCPs), Per- and Polyfluoroalkyl Substances (PFAS), pesticides, and metals (Kroon et al., 2020). Although these compounds are increasingly being detected in different environments, they are mostly not monitored or regulated, safe exposure limits are still lacking for many of them (Miller et al., 2020), and their potential ecological and human effects and interactions and still understudied and, often, unknown. Metals, in particular, are of significant concern in this scenario, as they are environmentally persistent and may present highly toxic effects at even very low concentrations (Singh and Kalamdhad, 2011).
Several metals have been recently deemed of emerging concern, such as nickel and cobalt, increasingly used in the emerging nickel-cobalt extraction industry in tropical and subtropical regions of the globe (Reichelt-Brushett and Hudspith, 2016). Some, in addition to being classified as contaminants of emerging concern, are also categorized as Technology-Critical Elements (TCEs), including titanium, rubidium, Rare Earth Elements (REEs), Platinum Group Metals (PGMs, including platinum, palladium, and rhodium) lithium, cobalt, graphite, indium, tantalum, gallium, and antimony, among others (Romero-Freire et al., 2019; European Commission, 2020). This classification encompasses the growing demands for previously underutilized elements but that now serve as a robust foundation for current industries, generating a diverse array of products and applications used in everyday life and modern technologies (Cobelo-García et al., 2015). Technology-Critical Elements, therefore, now play a crucial role in high-tech industries and in emerging technologies on a global scale (Romero-Freire et al., 2019). The increasing use of TCE has led to dramatic alterations in the cycling of these elements in environmentally relevant systems, resulting in increased attention to their potential environmental impacts (European Commission, 2020; Batley and Campbell, 2022), especially during the last five or so years.
Notably, the increasing development of technology-driven industries has resulted in heightened demands for TCEs. Several TCEs, however, exhibit limited supplies and availability, with many geopolitical complexities linked to their production and distribution (Nuss and Blengini, 2018). Accordingly, efforts are currently underway to broaden TCE exploration from different sources, enhance recycling and recovery technologies and develop alternative materials to reduce societal reliance on these elements, ensuring the continued development of cutting-edge technologies (Krishna et al., 2023). This however, will, in turn and indubitably, lead to heightened environmental contamination by these elements, as a result of increased mining and extraction and manufacturing processes, product use and disposal, combustion emissions, atmospheric deposition, runoff and leaching and wastewater discharges, among others.
Aquatic environments are of significant interest in the TCE context, as they comprise the ultimate sink for most environmental contaminants (Bashir et al., 2020). These ecosystems also play a role in cycling many elements, including TCE, back to land, directly or indirectly through the hydrological cycle, as well as through seafood consumption, affecting both environmental and human health (Kite-Powell et al., 2008; Herman and Gellasch, 2021). In this regard, a significant knowledge gap is noted for the dynamics and effects of many TCEs in aquatic ecosystems, especially as these elements do not exhibit uniform chemical properties, as some, like rare earth elements, are metals, while others, such as tellurium and gallium, are non-metals. This gap extends, but is not limited to, TCE environmental concentrations, potential speciation processes and consequent bioavailability, as well as mechanisms of action and bioaccumulation and biomagnification potential throughout aquatic food webs.
Numerous studies examining TCEs have been conducted across various aquatic taxonomic groups, encompassing algae, mollusks, crustaceans, and fish, and some valuable reviews have been recently conducted detailing their specific properties and sources, their fate and distribution in the marine environment and their interactions with marine biota (for example, Abdou et al., 2023). Data, however, is still very disperse in the scientific literature, hindering adequate specific knowledge gap assessments and further evaluations on the topic.
Assessments concerning TCEs in fish, in particular, are paramount, as these organisms are excellent environmental health indicators, indicative of ecosystem resilience and allow for the early detection of contaminants or pollution trends (Hook et al., 2014; Impellitteri et al., 2023). They are also the main link between environmental and human contamination. However, most of the available studies on TCEs, in both fish and in other organisms, simply present determined TCEs concentrations, without delving into metabolic or physiological implications. While these investigations certainly contribute valuable data on TCEs concentrations in aquatic biota, they fall short in elucidating potential TCEs effects. Therefore, the need to integrate physiological investigations and aquatic ecotoxicology focusing on TCEs becomes clear and, indeed, paramount.
Significantly, most fish studies concentrate exclusively on teleosts, while research on cartilaginous fish (sharks, rays, and chimeras), remains notably scarce. In fact, a huge delay in the scientific literature is noted concerning pollution assessments in elasmobranchs, as, historically, most studies assessments for this groups centered around ecology, overfishing, and bycatch issues. This will be elaborated upon in the following section.
As noted by Alves et al. (2020), despite the ecological and economic significance associated with elasmobranchs, their management is still a low priority compared to other marine predators. Particularly, chemical pollution has only recently been considered a priority for these animals (Consales and Marsili, 2021; Cooke et al., 2021). This has led to a paradigm shift, as a growing recognition of their vulnerability and ecological significance and, consequently, their role as valuable ecosystem service providers (Serrat et al., 2023), has become more widely known. These include their role in trophic regulation, preventing the abundance of certain prey species and maintaining healthy prey populations by preying on weak prey, carbon sequestration and nutrient cycling, among others. This has led to a surge of attention directed toward pollution effects in elasmobranchs in recent years aiming at their conservation and management, by identifying pollution hotspots, assessing exposure levels, and evaluating health effects, aiming at guiding mitigation strategies, informing policy decisions, raising public awareness and education, and contributing to global conservation initiatives. Studies on TCEs, however, are still notably lacking.
Concerning TCEs, some findings in bony fish have indicated developmental, genotoxicity, mutagenicity, and reproductive effects for some TCEs, such as titanium and rubidium and some REE, such as lanthanum and praseodymium, as well as potential trophic web biomagnification, directly affecting not only individual health but also ecosystem dynamics (Campbell et al., 2005; Dubé et al., 2019; Zhao et al., 2021; Santos et al., 2023). Extrapolating these findings to cartilaginous fish, significant physiological and ecological effects of TCEs in this highly vulnerable taxonomic group are probable, due to certain specific life-history traits, such as slow maturation rates, low fecundity, long lifespans, and mostly meso- and top predator positions (Ward-Paige et al., 2012).
Furthermore, cartilaginous fish hold significant economic importance, as they are highly consumed worldwide and comprise a cheap source of protein for millions of people around the globe (Giovos et al., 2022), particularly for communities who may suffer from limited alternatives in terms of protein sources, such as fishers and traditional peoples, as well as vulnerable populations, like children and marginalized communities. The previously noted lack of studies in this regard for cartilaginous fish, however, has also hindered human health risk assessments, as most TCEs have not yet been evaluated concerning safe exposure limits. Certain studies in this field suggest both primary and secondary human health effects due to the consumption of fish contaminated with TCE (Gwenzi et al., 2018; Bu-Olayan and Thomas, 2020), but, as cited previously, no standards or guidelines, as well as comprehensive assessments addressing this issue, have been established to date.
The One Health concept is key in this setting. This framework recognizes the interconnection between people, animals, plants, and their shared environments, encompassing a collaborative, multisectoral and transdisciplinary approach at local, regional, national, and global levels (FAO, 2021). It emphasizes that the health and wellbeing of these components are intricately linked, and that actions affecting one can have cascading effects on the others, requiring collaboration among various disciplines, including medicine, veterinary science, ecology, environmental science, and public health. Technology-Critical Elements assessments in marine organisms are, therefore, crucial in this sense, due to their paramount and irreplaceable role in maintaining marine ecosystem health and stability through ecosystem services, including roles in biodiversity maintenance, nutrient cycling, food web dynamics, climate regulation, pollution control, and finally, socio-economic value (Holmlund and Hammer, 1999; Worm et al., 2006).
Although studies applying the One Health approach for elasmobranchs are still in their infancy, the interconnectedness of these animals and humans, including as important ecological roles, subsidizing important ecosystem services, among others, should be considered in a broader context, as declines in elasmobranch populations can have far-reaching consequences for both ecological and human health.
Furthermore, pursuing these aims by carrying out TCEs pollution assessments in elasmobranchs also aligns with and significantly contributes to the achievement of multiple dimensions of the 2030 Agenda, a global initiative to address a wide range of social, economic, and environmental challenges now faced worldwide. For example, assessing TCE pollution in elasmobranchs directly aligns with SDG 3—Human Health and WellBeing, as TCE contamination in elasmobranchs can lead to human health risks due to bioaccumulation of toxic compounds in their edible tissues, particularly resonating with SDG 3–9, which aims to significantly diminish the toll of deaths and illnesses stemming from hazardous chemicals and environmental pollution, in this case, the potential hazards of TCEs present in elasmobranch meat. Monitoring TCE pollution in elasmobranchs also offers insight on the overall health of marine ecosystems, aligned with SDG 14 (Life Below Water, which focuses on conserving and promoting the sustainable utilization of oceans, seas, and marine resources.), and contributes to the preservation of biodiversity, in line with SDG 15 (Life on Land and Life Below Water). In addition, this type of assessment can also lead to sustainable practices in fisheries and aquaculture, encouraging responsible consumption and production patterns (SDG 12, Responsible Consumption and Production) by ensuring that seafood is safe for human consumption and harvested sustainably. Finally, Some TCEs, like rare earth elements, are essential for clean energy technologies, i.e., wind turbines and electric vehicles. Thus, assessing their potential impacts in elasmobranchs supports the development of sustainable technologies, aligning with SDG 13 (Climate Action).
Comprehensive investigations into the potential effects of TCEs on elasmobranchs, along with their broader ecological implications, will, therefore, comprise a pivotal step in understanding the dynamics of these contaminants of emerging concern in aquatic ecosystems, contributing to their sustainable preservation and the intricate relationships between contaminants, ecosystems, and human health. Thus, increased and extensive research on TCEs in elasmobranchs worldwide should be implemented, directing a novel field of research. Specifically, species inhabiting varied habitats such as coastal zones, deep ocean waters, and coral reef ecosystems, and occupying different ecological niches should be pursued for analysis, as distinct diets, mobility, and life history traits probably display different metabolism and physiology responses to TCEs. This may, in turn, shed light on how TCEs enter and become distributed throughout different food webs, as well as how they may impact the health and functionality of aquatic organisms and, ultimately, provide valuable insights in their ecological outcomes, guiding informed conservation strategies and sustainable management practices for elasmobranchs and their habitats.
The original contributions presented in the study are included in the article/supplementary material, further inquiries can be directed to the corresponding author.
RH-D: Conceptualization, Data curation, Formal analysis, Funding acquisition, Investigation, Methodology, Project administration, Resources, Software, Supervision, Validation, Visualization, Writing—original draft, Writing—review and editing.
The author(s) declare financial support was received for the research, authorship, and/or publication of this article. This research was funded by the Carlos Chagas Filho Foundation for Research Support of the State of Rio de Janeiro (FAPERJ) (RAHD), through a Jovem Cientista do Nosso Estado 2021–2024 grant (process number E-26/201.270/2021), Jovem Pesquisador Fluminense com Vínculo em ICTS do Estado do Rio des Janeiro grant (process number E-26/2010.300/2022), and the Brazilian National Council of Scientific and Technological Development (CNPq), through a productivity grant (process number 308811/2021-6). The implementation of the Projeto Pesquisa Marinha e Pesqueira is a compensatory measure established by the Conduct Adjustment Agreement under the responsibility of the PRIO company, conducted by the Federal Public Ministry-MPF/RJ.
The author declares that the research was conducted in the absence of any commercial or financial relationships that could be construed as a potential conflict of interest.
The author(s) declared that they were an editorial board member of Frontiers, at the time of submission. This had no impact on the peer review process and the final decision.
All claims expressed in this article are solely those of the authors and do not necessarily represent those of their affiliated organizations, or those of the publisher, the editors and the reviewers. Any product that may be evaluated in this article, or claim that may be made by its manufacturer, is not guaranteed or endorsed by the publisher.
Abdou, M., Cobelo-Garcia, M., Santos-Echeandía, J., and Schäfer, J. (2023). “Technology-critical elements,” in Contaminants of Emerging Concern in the Marine Environment, eds. V. M. León and J. Bellas (New York, NY: Elsevier), 401–438. doi: 10.1016/B978-0-323-90297-7.00007-X
Alves, L. M. F., Correia, J. P. S., Lemos, M. F. L., Novais, S. C., and Cabral, H. (2020). Assessment of trends in the Portuguese elasmobranch commercial landings over three decades. Fish. Res. 230:105648. doi: 10.1016/j.fishres.2020.105648
Bashir, I., Lone, F. A., Bhat, R. A., Mir, S. A., Dar, Z. A., and Dar, S. A. (2020). “Concerns and threats of contamination on aquatic ecosystems,” in Bioremediation and Biotechnology, eds. K. Hakeem, R. Bhat, H. Qadri (Cham: Springer), 1–26. doi: 10.1007/978-3-030-35691-0_1
Batley, G. E., and Campbell, P. G. C. (2022). Metal contaminants of emerging concern in aquatic systems. Environ. Chem. 19, 23–40. doi: 10.1071/EN22030
Bu-Olayan, A. H., and Thomas, B. V. (2020). Bourgeoning impact of the technology critical elements in the marine environment. Environ. Pollut. 265(Pt A):115064. doi: 10.1016/j.envpol.2020.115064
Campbell, L. M., Fisk, A. T., Wang, X., Köck, G., and Derek, C. G. (2005). Evidence for biomagnification of rubidium in freshwater and marine food webs. Canad. J. Fisher. Aquat. Sci. 62, 1161–1167. doi: 10.1139/f05-027
Cobelo-García, A., Filella, M., Croot, P., Frazzoli, C., Du Laing, G., Ospina-Alvarez, N., et al. (2015). COST action TD1407: network on technology-critical elements (NOTICE)–from environmental processes to human health threats. Environ. Sci. Pollut. Res. Int. 22, 15188–15194. doi: 10.1007/s11356-015-5221-0
Consales, G., and Marsili, L. (2021). Assessment of the conservation status of Chondrichthyans: Underestimation of the pollution threat. Eur. Zool. J. 88, 165–180. doi: 10.1080/24750263.2020.1858981
Cooke, S. J., Bergman, J. N., Madliger, C. L., Cramp, R. L., Beardall, J., Burness, G., et al. (2021). One hundred research questions in conservation physiology for generating actionable evidence to inform conservation policy and practice. Conserv. Physiol. 9, coab009. doi: 10.1093/conphys/coab009
Dubé, M., Auclair, J., Hanana, H., Turcotte, P., Gagnon, C., and Gagn,é, F. (2019). Gene expression changes and toxicity of selected rare earth elements in rainbow trout juveniles. Compar. Biochem. Physiol. Part C. 223, 88–95. doi: 10.1016/j.cbpc.2019.05.009
European Commission (2020). Critical materials for strategic technologies and sectors in the EU - a foresight study. Available online at: https://rmis.jrc.ec.europa.eu/uploads/CRMs_for_Strategic_Technologies_and_Sectors_in_the_EU_2020.pdf (accessed August 15, 2023).
FAO (2021). One Health. Available at: https://www.fao.org/family-farming/detail/en/c/1614268/ (accessed August 15, 2023).
Giovos, I., Brundo, M. V., Doumpas, N., Kazlari, Z., Loukovitis, D., Moutopoulos, D. K., et al. (2022). Trace elements in edible tissues of elasmobranchs from the North Aegean Sea (Eastern Mediterranean) and potential risks from consumption. Mar. Pollut. Bull. 184, 114129. doi: 10.1016/j.marpolbul.2022.114129
Gwenzi, W., Mangori, L., Danha, C., Chaukura, N., Dunjana, N., and Sanganyado, E. (2018). Sources, behaviour, and environmental and human health risks of high-technology rare earth elements as emerging contaminants. Sci. Total Environ. 636, 299–313. doi: 10.1016/j.scitotenv.2018.04.235
Herman, J. S., and Gellasch, C. A. (2021). “Geohydrology: Hydrogeochemistry,” in Encyclopedia of Geology, eds. S. A. Elias and D. H. M. Alderton (New York, NY: Elsevier), 416–425. doi: 10.1016/B978-0-12-409548-9.12504-7
Holmlund, C. M., and Hammer, M. C. (1999). Ecosystem services generated by fish populations. Ecol. Econ. 29, 253–268. doi: 10.1016/S0921-8009(99)00015-4
Hook, S. E., Gallagher, E. P., and Batley, G. E. (2014). The role of biomarkers in the assessment of aquatic ecosystem health. Integr. Environ. Assess. Manage. 10, 327–341. doi: 10.1002/ieam.1530
Impellitteri, F., Multisanti, C. R., Rusanova, P., Piccione, G., Falco, F., and Faggio, C. (2023). Exploring the impact of contaminants of emerging concern on fish and invertebrates physiology in the mediterranean sea. Biology 12, 767. doi: 10.3390/biology12060767
Khan, S., Naushad, M., Govarthanan, M., Iqbal, J., and Alfadul, S. M. (2022). Emerging contaminants of high concern for the environment: Current trends and future research. Environ. Res. 207, 112609. doi: 10.1016/j.envres.2021.112609
Kite-Powell, H. L., Fleming, L. E., Backer, L. C., Faustman, E. M., Hoagland, P., Tsuchiya, A., et al. (2008). Linking the oceans to public health: current efforts and future directions. Environ. Health 7, S6. doi: 10.1186/1476-069X-7-S2-S6
Krishna, R., Dhass, A. D., Arya, A., Prasad, R., and Colak, I. (2023). An assessment of the strategies for the energy-critical elements necessary for the development of sustainable energy sources. Environ. Sci. Pollut. Res. Int. 30, 1–22. doi: 10.1007/s11356-023-28046-2
Kroon, F. J., Berry, K. L. E., Brinkman, D. L., Kookana, R., Leusch, F. D., Melvin, S. D., et al. (2020). Sources, presence and potential effects of contaminants of emerging concern in the marine environments of the Great Barrier Reef and Torres Strait, Australia. Sci. Total Environ. 719:135140. doi: 10.1016/j.scitotenv.2019.135140
Miller, E., Mendez, M., Shimabuku, I., Buzby, N., and Sutton, R. (2020). Contaminants of Emerging Concern in San Francisco Bay: a Strategy for Future Investigations 2020 Update. SFEI Contribution No. 1007. Richmond, CA: San Francisco Estuary Institute.
Nuss, P., and Blengini, G. A. (2018). Towards better monitoring of technology critical elements in Europe: Coupling of natural and anthropogenic cycles. Sci. Total Environ. 613, 569–578. doi: 10.1016/j.scitotenv.2017.09.117
OECD (2018). OECD workshop on Managing Contaminants of Emerging Concern in Surface Waters: Scientific developments and cost-effective policy responses. Available online at: https://www.oecd.org/water/Summary%20Note%20-%20OECD%20Workshop%20on%20CECs.pdf (accessed February 5, 2018).
Reichelt-Brushett, A., and Hudspith, M. (2016). The effects of metals of emerging concern on the fertilization success of gametes of the tropical scleractinian coral Platygyra daedalea. Chemosphere 150, 398–406. doi: 10.1016/j.chemosphere.2016.02.048
Romero-Freire, A., Santos-Echeandía, J., Neira, P., and Cobelo-García, A. (2019). Less-studied technology-critical elements (Nb, Ta, Ga, In, Ge, Te) in the marine environment: review on their concentrations in water and organisms. Front. Marine Sci. 6, 532. doi: 10.3389/fmars.2019.00532
Santos, A. C. S. S., Souza, L. A., Araujo, T. G., de Rezende, C. E., and Hatje, V. (2023). Fate and trophic transfer of rare earth elements in a tropical estuarine food web. Environ. Sci. Technol. 57, 2404–2414. doi: 10.1021/acs.est.2c07726
Serrat, A., Farriols, M. T., Ramírez-Amaro, S., Ordines, F., Guijarro, B., Ferragut-Perello, F., et al. (2023). Conservation status assessment of demersal elasmobranchs in the Balearic Islands (Western Mediterranean) over the last two decades. Fishes 8, 230. doi: 10.3390/fishes8050230
Singh, J., and Kalamdhad, A. S. (2011). Effects of heavy metals on soil, plants, human health and aquatic. Int. J. Res. Chem. Environ. 1, 15–21.
Ward-Paige, C. A., Keith, D. M., Worm, B., and Lotze, H. K. (2012). Recovery potential and conservation options for elasmobranchs. J. Fish Biol. 80, 1844–1869. doi: 10.1111/j.1095-8649.2012.03246.x
Worm, B., Barbier, E. B., Beaumont, N., Duffy, J. E., Folke, C., Halpern, B. S., et al. (2006). Impacts of biodiversity loss on ocean ecosystem services. Science. 314, 787–790. doi: 10.1126/science.1132294
Keywords: contaminants of emerging concern, metals and metalloids, ecotoxicology, fish, chondrichthyes
Citation: Hauser-Davis RA (2023) This seems fishy: Technology-Critical Elements as a growing concern to aquatic biota and elasmobranchs in particular. Front. Ocean Sustain. 1:1281484. doi: 10.3389/focsu.2023.1281484
Received: 22 August 2023; Accepted: 03 October 2023;
Published: 20 October 2023.
Edited by:
Ilia Rodushkin, Luleå University of Technology, SwedenReviewed by:
Patrícia Pereira, University of Aveiro, PortugalCopyright © 2023 Hauser-Davis. This is an open-access article distributed under the terms of the Creative Commons Attribution License (CC BY). The use, distribution or reproduction in other forums is permitted, provided the original author(s) and the copyright owner(s) are credited and that the original publication in this journal is cited, in accordance with accepted academic practice. No use, distribution or reproduction is permitted which does not comply with these terms.
*Correspondence: Rachel Ann Hauser-Davis, cmFjaGVsLmhhdXNlci5kYXZpc0BnbWFpbC5jb20=
Disclaimer: All claims expressed in this article are solely those of the authors and do not necessarily represent those of their affiliated organizations, or those of the publisher, the editors and the reviewers. Any product that may be evaluated in this article or claim that may be made by its manufacturer is not guaranteed or endorsed by the publisher.
Research integrity at Frontiers
Learn more about the work of our research integrity team to safeguard the quality of each article we publish.