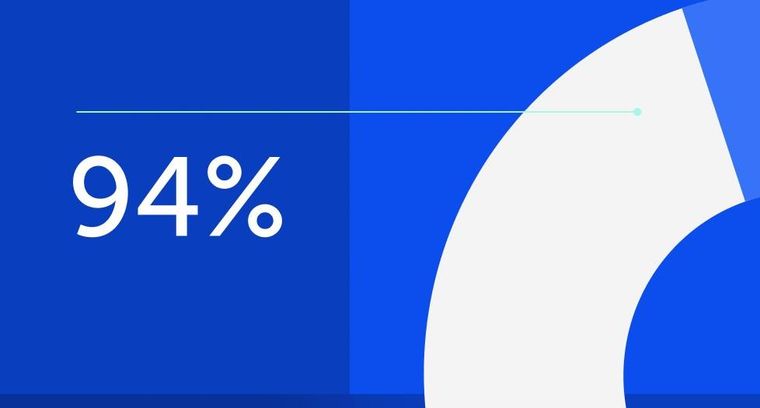
94% of researchers rate our articles as excellent or good
Learn more about the work of our research integrity team to safeguard the quality of each article we publish.
Find out more
ORIGINAL RESEARCH article
Front. Nutr., 25 March 2025
Sec. Nutritional Immunology
Volume 12 - 2025 | https://doi.org/10.3389/fnut.2025.1566505
This article is part of the Research TopicDietary Bioactive Compounds as Coadjuvants in Antiviral Preventive and Curative StrategiesView all 3 articles
Introduction: Zinc plays an important role in the functioning of the immune system. Zinc deficiency leads to increased susceptibility to inflammatory and infectious diseases. There are few studies investigating the role of zinc in the development and progression of COVID-19 in children, and their findings remain inconsistent. This study aimed to determine the zinc levels in children with COVID-19 and assess their association with symptoms, inflammation markers, and disease progression.
Methods: A prospective cohort study included hospitalized patients under 18 years who had a confirmed diagnosis of SARS-CoV-2 infection. Serum zinc concentrations were measured using a colorimetric method. Based on zinc levels, the children were divided into two groups: the first group had concentrations below 10.7 μmol/L, indicating zinc deficiency, while the second group had levels above 10.7 μmol/L, which was considered within the optimal range.
Results: In total, 140 hospitalized patients with COVID-19 were examined. Zinc deficiency was identified in 40 children (28.6%), while optimal levels were found in 100 children (71.4%). Zinc status did not depend on the age of the children. Among the symptoms of acute SARS-CoV-2 infection, children with zinc deficiency showed a trend toward more frequent fever occurrences (p = 0.0654). No significant impact of zinc status was observed on the severity of COVID-19 or the duration of hospitalization. Children with zinc deficiency had higher median values of the neutrophil-to-lymphocyte ratio (NLR) (1.84 vs. 1.09, p = 0.0010), C-reactive protein (CRP) levels (9.65 vs. 3.96 mg/L, p = 0.0053), and fibrinogen levels (2.88 vs. 2.07 g/L, p = 0.0057) compared to those with adequate zinc levels. Additionally, the percentage of patients with a NLR greater than 4, elevated CRP, and fibrinogen levels was higher in the zinc-deficient group (p = 0.0017, p = 0.0107, p = 0.0338, respectively).
Conclusion: Zinc deficiency was observed in 28.6% of children with COVID-19 and was not dependent on age. Children with hypozincemia had higher levels of inflammation markers, including the neutrophil-to-lymphocyte ratio and CRP.
The coronavirus disease 2019 (COVID-19) pandemic, caused by the severe acute respiratory syndrome coronavirus 2 (SARS-CoV-2), is the most significant global health crisis of the past century (1, 2). Since 2020, it has led to over 776 million reported cases and more than 7 million deaths worldwide (3).
In children, SARS-CoV-2 infection is usually mild, although more severe manifestations of the disease can sometimes develop (4, 5). The progression of COVID-19 involves complex interactions between many pathophysiological mechanisms, including the immune system’s innate and adaptive responses (6, 7). Disruption of the adaptive immune response, coupled with excessive activation of the innate immune system (inflammatory macrophages and neutrophils), contributes to severe disease outcomes (8, 9). Clinical data indicate that the severity of COVID-19 correlates with increased levels of the pro-inflammatory cytokine interleukin-6 (IL-6), elevated neutrophil-to-lymphocyte ratio (NLR), and lymphopenia. Hyperinflammation, driven by these immune changes, plays a central role in the development of acute respiratory distress syndrome and tissue damage, leading to airway collapse, multi-organ failure, and, ultimately, death in severe cases (10, 11).
Clinical or demographic risk factors for severe SARS-CoV-2 infection include older age, male sex, chronic diseases such as diabetes, cardiovascular diseases, immunosuppression, and obesity (12, 13), as well as suboptimal vitamin and micronutrient status (14–16). Key vitamins A, C, and D, and micronutrients such as selenium, copper, and zinc, which are essential for proper immune function, have been widely discussed regarding their impact on susceptibility to COVID-19 and disease progression (15, 17–20). Zinc (Zn2+) is an essential micronutrient necessary for supporting a variety of fundamental biological processes due to its roles as a cofactor, signaling molecule, and structural component (21, 22). Specifically, it is involved in growth development, neuro-sensory functions, deoxyribonucleic acid (DNA) synthesis, and gene expression (23). One of the most important roles of zinc in the human body is its broad impact on the immune system (21, 22), as zinc levels affect both adaptive and innate immunity. In adaptive immunity, zinc influences T-lymphocyte maturation, differentiation, and cytokine production. B-cell activation and plasma cell differentiation also depend on zinc signaling (22, 24). In innate immunity, zinc plays an anti-inflammatory role (25). Specifically, zinc deficiency is associated with higher levels of interleukin-1 (IL-1) beta and tumor necrosis factor alpha (TNF-alpha) (26), as well as altered monocyte, neutrophil, and natural killer (NK) cell activity (27). Accordingly, zinc deficiency leads to increased susceptibility to inflammatory and infectious diseases (22).
Several studies have illustrated a connection between upper respiratory tract infections, the duration of symptoms, and serum zinc levels (28, 29). The current literature on the effect of zinc on the course of COVID-19 is limited, with most of the information focusing on the adult population. For example, Vogel-González et al. (30) observed a significant association between prolonged clinical recovery, increased intensive care unit (ICU) stay, mortality, and lower zinc levels in the serum of adult patients. Chen et al. (31) conducted a retrospective review of patients with the Omicron variant of COVID-19 and found that zinc deficiency was connected with acute and persistent inflammation. However, other studies show contradictory results. Yao et al. (32) did not demonstrate a causal relationship between zinc levels and improved prognosis or survival in acute SARS-CoV-2 infection. However, studies examining the role of zinc in the development and course of COVID-19 in children are few, and their findings are inconsistent (14, 33, 34). The aim of our study was to determine the zinc levels in children with COVID-19 and assess their association with symptoms, inflammation markers, and disease progression.
A prospective cohort study included hospitalized pediatric patients with a confirmed diagnosis of SARS-CoV-2 infection through polymerase chain reaction (PCR), rapid tests, or serological methods (detection of IgM), which were used interchangeably.
The study was conducted from September 2022 to March 2024 in the pediatric infectious diseases department of Municipal City Hospital №2 in Ternopil, Ukraine.
The study involved hospitalized patients under 18 years who had a confirmed diagnosis of SARS-CoV-2 infection.
The inclusion criteria for the study were: age under 18 years, confirmed cases of SARS-CoV-2 infection, informed consent from parents for participation, and the ability to measure serum zinc levels during hospitalization. Exclusion criteria included the refusal of parents to consent to the study and unconfirmed cases of COVID-19.
Throughout the study, we adhered to all recommendations of the Helsinki Declaration of 1975 (as revised in 2000). The study was approved by the I. Horbachevsky Ternopil National Medical University Ethics Committee (Minutes № 70 from August 1, 2022). Upon admission, all parents or children over the age of 16 signed an informed, voluntary consent form for participation in the study, as well as for the use of diagnostic and treatment results in scientific publications.
We carefully collected baseline and clinical data from patients upon hospital admission. Baseline characteristics included age and sex, while clinical data included comorbidities, disease severity, and duration of hospitalization. Laboratory tests upon admission included an expanded complete blood count, biochemical markers, and the determination of C-reactive protein (CRP) and ferritin levels.
COVID-19 severity was determined according to the World Health Organization (WHO) definition (35). Based on this, patients were divided into four groups: mild, moderate, severe, and critical.
Blood samples for determining serum zinc levels were collected from patients in the morning or afternoon of the same or the following day if the SARS-CoV-2 test result was positive. The samples were left to clot, then centrifuged at 4000 rpm for 10 min to separate the serum. Zinc concentration in the serum was measured using a Multiskan FC-357 microplate photometer (Thermo Fisher Scientific). A colorimetric zinc (Zn) assay kit (Elabscience E-BC-K137-M, USA) was used for the analysis. The coefficients of variation (CV) for zinc content analysis in the samples were: inter-assay CV – 0.04%, and intra-assay CV – 2.7%.
Patients with confirmed COVID-19 were divided into two groups based on whether they had low or normal zinc levels in their serum. Currently, there is no universally accepted threshold for low serum zinc concentrations. In our study, we used 10.7 μmol/L as the cutoff for low zinc concentration in serum. Сlinical zinc deficiency was defined as a concentration of <70 μg/dL (10.7 μmol/L) (36).
Statistical analysis of the results was performed using STATISTICA 12 software. The median and interquartile range (IQR) were used for non-normal distribution of data and categorical variables were expressed as frequency (percentage). Categorical variables were compared using the Chi-square test. A p-value of less than 0.05 was considered statistically significant and is highlighted in bold in the tables.
In total, 140 hospitalized patients were included in the study. Clinical and laboratory characteristics of the children with COVID-19 are presented in Table 1. The average age of the hospitalized patients was 3.56 ± 4.55 years, ranging from 1 month to 18 years. The male sex predominated over the female sex in the study population (56.4% vs. 43.6%).
Table 1. Clinical characteristics of the patients with COVID-19 and their dependence on serum zinc levels.
Comorbid conditions were identified in more than half of the patients (50.7%). The most common comorbidities were allergic conditions (28.6%) and nutritional disorders (22.1%). Diseases of the nervous, digestive, urinary, and cardiovascular systems observed less frequently.
Mild COVID-19 was diagnosed in 90.7% of the studied children. Only 5 patients (3.6%) had a moderate course, 6 children (4.3%) had a severe course, and 2 children (1.4%) had a critical course. No deaths were observed in our sample. The average duration of hospitalization was 4.6 ± 3.4 days, ranging from 1 to 20 days.
Zinc deficiency was identified in 40 children (28.6%), while optimal zinc levels were found in 100 children (71.4%) with COVID-19. The number of children with zinc deficiency did not depend on the age of the patients (Figure 1a). In patients older than 6 years, optimal zinc levels were more commonly observed, though the difference was not statistically significant (78.8% vs. 69.2%, p = 0.3790). Conversely, 30.8% of children under 6 years demonstrated zinc deficiency.
Figure 1. Dependence of zinc levels on age in children with COVID-19: (a) Comparison of median zinc concentration in deficiency and optimal levels in children under 6 years and over 6 years, p = 0.9576; (b) Comparison of zinc levels between children under 6 years and over 6 years, p = 0.0983.
Zinc levels were independent of the patients’ age. The median zinc concentration in children under 6 years was 12.0 μmol/L (IQR: 10.4; 14.3), while in children older than 6 years, it was 13.2 μmol/L (IQR: 11.6; 15.7), p = 0.0983 (Figure 1b).
The comparison of clinical characteristics of COVID-19 patients based on zinc levels is presented in Table 1.
Among the symptoms of acute SARS-CoV-2 infection, fever was present in 100.0% of children with zinc deficiency, while it was observed in 92.0% of those with normal zinc levels (p = 0.0654). The next most frequent symptoms were respiratory signs, followed by fatigue and loss of appetite, although the frequency of these symptoms did not depend on zinc status (p = 0.8502, p = 0.3633, and p = 0.2704, respectively) (Figure 2).
Comorbidities were observed in both groups with similar frequency (42.5% vs. 54.0%, p = 0.2189). Malnutrition and diseases of the nervous and urinary systems were more frequently found in children with low serum zinc levels, but these differences were not statistically significant (p = 0.1966, p = 0.5329, and p = 0.5646, respectively).
The severity of COVID-19 and the duration of hospitalization were not dependent on zinc status.
Levels of leukocytes, neutrophils, lymphocytes were not significantly different between the two cohorts of patients. However, the NLR was found to be higher in children with zinc deficiency than in those with normal levels (p = 0.0010). The percentage of patients with a ratio greater than 4 was four times higher in the group with reduced zinc levels, and the difference was statistically significant (p = 0.0060).
The median CRP level was significantly higher in children with hypozincemia (p = 0.0053). Elevated CRP levels were also more frequently observed in the group with reduced zinc levels.
The median platelet count and the frequency of thrombocytopenia did not differ statistically. A tendency toward a higher frequency of thrombocytosis was observed in patients with low zinc levels (p = 0.0692). The mean values of prothrombin time, activated partial thromboplastin time (aPTT), and D-dimer were not dependent on serum zinc status. However, a trend toward more frequent increases in aPTT and D-dimer was observed in children with hypozincemia (p = 0.0674 and p = 0.0923, respectively). The median fibrinogen level was higher and hyperfibrinogenemia was significantly more common in children with zinc deficiency (p = 0.0057, p = 0.0338, respectively), although the percentage of its reduced levels was higher in children with optimal zinc values (p = 0.0290).
Analysis of creatinine and total protein levels did not show any significant differences based on zinc status.
In our study, the frequency of hypozincemia among children with acute SARS-CoV-2 infection was 28.6%. However, findings from other studies on the prevalence of zinc deficiency in children with COVID-19 have been variable, likely due to differences in study design, patient populations, and methods of zinc level assessment.
Doğan et al. (10) found that zinc deficiency occurred in 23.9% of outpatient children diagnosed with COVID-19, which aligns with our findings, although our cohort consisted of hospitalized patients. In contrast, Ekemen Keleş et al. (33) reported a lower prevalence of hypozincemia (11.0%) among children visiting outpatient clinics due to COVID-19, with a significantly higher average zinc concentration compared to our cohort. On the other hand, another study (37) identified zinc deficiency in 57.4% of children with COVID-19.
Several factors beyond SARS-CoV-2 infection can influence zinc levels. These include older age, male sex, and certain comorbidities, such as diabetes, overweight, and obesity (38–41). In our study population, zinc status did not significantly depend on either the age or sex of the patients. Interestingly, we did not observe zinc deficiency in children with overweight, while 11% of those with an optimal zinc level had excessive weight (p = 0.0289). Some studies report a negative correlation between body mass index and zinc levels (42–44), while others have observed a positive impact of zinc supplementation on weight reduction (45, 46). However, other researchers dispute this hypothesis, indicating no significant relationship between zinc status and obesity (47, 48). We did not study zinc intake from food sources or the impact of other factors on zinc status, which could have also influenced the results of our study.
We did not find any difference in the frequency of hypozincemia depending on the severity of COVID-19 or the duration of hospitalization. The conclusions on this issue are contradictory. Ekemen Keleş et al. (33) reported that patients with low serum zinc levels did not have longer hospital stays compared to those with normal zinc levels, which is consistent with our results. In contrast, Yasui et al. (49) demonstrated a connection between zinc deficiency and the severity of COVID-19 in adult patients. Fujita et al. (50) showed that individuals requiring oxygen therapy during the acute phase of SARS-CoV-2 infection had a higher prevalence of zinc deficiency, and the presence of hypozincemia at the time of COVID-19 diagnosis was an independent risk factor for severe disease.
Some studies demonstrate the impact of zinc deficiency on mortality from COVID-19, especially among the adult population (51, 52). Razeghi Jahromi et al. (51) found that patients with zinc deficiency had higher rates of hospitalization, acute respiratory distress syndrome, and mortality. Maares et al. (52) revealed that the overall serum zinc level was significantly lower in patients with COVID-19 compared to the control group, with the lowest levels observed in those who died from the coronavirus disease.
Laboratory analysis showed that the levels of lymphocytes and neutrophils were not dependent on zinc status. In contrast, the NLR was significantly higher in patients with reduced serum zinc levels (p = 0.0010). Similar results were observed in the study by Ekemen Keleş (33), which also found no statistically significant differences between zinc levels and the number of leukocytes, neutrophils, or lymphocytes in the blood. Another study showed that the median lymphocyte count was lower in patients with hypozincemia, although the difference was not statistically significant (30). Research involving older adults found that, compared to normal zinc levels, hypozincemia was associated with biomarkers of severe COVID-19, including a higher NLR and lymphopenia (p < 0.001) (53).
The association between zinc deficiency and a higher NLR may be explained by zinc’s crucial role in immune system regulation (22). Zinc is essential for lymphocyte proliferation, differentiation, and function, particularly in maintaining T-cell homeostasis and adaptive immunity (24). A deficiency in zinc leads to lymphocyte dysfunction and increased apoptosis, contributing to lymphopenia. At the same time, zinc plays an anti-inflammatory role by regulating neutrophil activity and reducing excessive inflammatory responses. In a state of zinc deficiency, neutrophil activation becomes dysregulated, leading to an increased neutrophil count and a higher NLR, which is considered a marker of systemic inflammation and disease severity in infections such as COVID-19 (9, 54–56). This imbalance between neutrophils and lymphocytes in patients with hypozincemia may contribute to the exacerbation of inflammatory responses and worse clinical outcomes. The limited antiviral response in COVID-19 in the presence of reduced zinc levels may enhance neutrophil infiltration, leading to severe inflammation (57).
The study of inflammation markers during acute viral infection with SARS-CoV-2 is of significant importance, as they can serve as prognostic factors for severe disease progression, mortality, or the development of long-term consequences. In our study, the median CRP was significantly higher in children with COVID-19 and zinc deficiency (p = 0.0053). Elevated CRP levels were observed in 68.4% of patients with hypozincemia, and the difference was statistically significant (p = 0.0120). Vogel-González et al. (30) also noted that the median CRP was more than twice as high in adult patients with zinc deficiency, and this difference was statistically significant. Razeghi Jahromi et al. (51) also showed a statistically significant negative correlation between zinc levels and CRP using Spearman’s correlation analysis. Almasaud et al. (58) demonstrated a significant negative correlation between serum zinc and inflammation markers, such as leukocytes, CRP, procalcitonin, lactate dehydrogenase, and ferritin. Another study also showed that high CRP levels were significantly associated with hypozincemia, as well as the median ferritin, which was significantly higher in adults with decreased serum zinc levels (53).
Zinc plays an important role in modulating the inflammatory response. Zinc functions as an anti-inflammatory and immunoregulatory micronutrient, influencing various signaling pathways involved in immune activation. It inhibits the NF-κB signaling pathway, a key regulator of pro-inflammatory cytokine production, including IL-6, a major inducer of CRP synthesis in the liver (59, 60). When zinc levels are insufficient, this inhibitory effect is weakened, leading to an increased release of pro-inflammatory cytokines and subsequently higher CRP levels (61).
Additionally, zinc is essential for maintaining the integrity of cell membranes and reducing oxidative stress. A deficiency in zinc results in increased production of reactive oxygen species (ROS) and promotes systemic inflammation, further contributing to elevated CRP levels (20, 62). This mechanism may explain why children with hypozincemia exhibit a more pronounced inflammatory response during SARS-CoV-2 infection.
It is known that zinc acts as an effector of coagulation, anticoagulation, and fibrinolysis, and has properties that regulate hemostasis and thrombosis (63). When analyzing all coagulation markers, we found that the fibrinogen level was significantly higher in patients with zinc deficiency (p = 0.0057), and there was also a trend toward thrombocytosis, increased aPTT, and D-dimer levels. Another of our studies showed the age-related characteristics of coagulation markers in children with COVID-19 (64). Vogel-González et al. (30) noted that the average D-dimer level was significantly higher in patients with decreased serum zinc levels. Another study demonstrated that the median platelet count was significantly lower in children with hypozincemia, although D-dimer was higher in the cohort with optimal zinc levels (33).
When analyzing biochemical indicators, we did not observe a statistically significant difference between creatinine and total protein levels. Jothimani et al. (37) also noted that the median creatinine level was not significantly related to zinc levels, unlike lactate dehydrogenase, which was significantly higher in children with zinc deficiency.
This study provides valuable information about the role of zinc in children with the SARS-CoV-2 viral infection, an area where data remains limited. Most studies focus on adults, which makes this work a significant contribution to understanding the symptoms and course of COVID-19 in pediatric patients. The study features a prospective cohort design with careful monitoring, allowing for a detailed analysis of clinical and laboratory characteristics of COVID-19 based on zinc status.
However, the study has several limitations. The sample size is relatively small, which may affect the generalizability of the results. The study population was selected from a tertiary pediatric hospital, meaning the patients may differ from those in other pediatric hospitals. Additionally, the study did not include a control group consisting of healthy children who did not have COVID-19. Such a group would have provided comparative data on zinc levels in uninfected populations. Another limitation is that serum zinc concentrations were measured after the children were infected. Inflammation can direct zinc to tissues, which can cause a significant decrease in serum or plasma zinc concentrations, regardless of zinc nutriture (65, 66). This phenomenon is typically reversible after recovery. Since infections are known to decrease serum zinc levels without affecting overall body zinc stores, it is unclear whether the observed lower zinc levels are related to a true deficiency or simply part of the normal acute-phase response. In future studies, it would be important to correct for acute-phase response effects, and including CRP as a covariate in zinc analyses could help address this limitation. Although we did not find a correlation between zinc levels and CRP levels, which could suggest that the observed decrease in serum zinc levels may not be solely due to inflammation or the acute-phase response. In future studies, it would be important to correct for acute-phase response effects, and including CRP as a covariate in zinc analyses could help address this limitation.
Zinc deficiency was found in 28.6% of children with COVID-19 and did not depend on age. In children with hypozincemia, higher levels of inflammation markers were observed, including the neutrophil-to-lymphocyte ratio and CRP. No effect of zinc status on other clinical symptoms of COVID-19 or disease severity was detected. Further studies into the impact of zinc status on the course of COVID-19 and other viral infections require the determination of serum concentrations of the trace element in children before illness and immediately after recovery to retrospectively assess whether low values on admission were transient and likely caused by acute inflammation or whether zinc deficiency is still present. This may improve our understanding of the pathogenesis, symptoms, course, and outcomes of acute infection and predict the likelihood of developing complications.
The raw data supporting the conclusions of this article will be made available by the authors, without undue reservation.
The studies involving humans were approved by I. Horbachevsky Ternopil National Medical University Ethics Committee (Minutes № 70 from August 1, 2022). The studies were conducted in accordance with the local legislation and institutional requirements. Written informed consent for participation in this study was provided by the participants’ legal guardians/next of kin.
VP: Conceptualization, Data curation, Formal analysis, Methodology, Resources, Software, Validation, Visualization, Writing – original draft, Writing – review & editing. TK: Data curation, Formal analysis, Funding acquisition, Investigation, Methodology, Resources, Validation, Writing – original draft, Writing – review & editing. LV: Data curation, Formal analysis, Methodology, Resources, Validation, Writing – original draft, Writing – review & editing. OB: Conceptualization, Data curation, Formal analysis, Funding acquisition, Investigation, Methodology, Project administration, Resources, Software, Supervision, Validation, Visualization, Writing – original draft, Writing – review & editing.
The author(s) declare that financial support was received for the research and/or publication of this article. The study was financed by the Ministry of Health of Ukraine with funds from the state budget, project title “Assessment of the quality of life and psychological state of children with long COVID-19 in time of war,” state registration number 0123 U100301, implementation period - 2023-2025.
We sincerely thank the medical staff of the Pediatric Infectious Disease Department of Ternopil Municipal City Hospital №2 for their invaluable assistance in recruiting patients for our study. We are also deeply grateful to the patients and their parents who agreed to participate in the research and diligently adhered to all the requirements and recommendations.
The authors declare that the research was conducted in the absence of any commercial or financial relationships that could be construed as a potential conflict of interest.
The authors declare that no Gen AI was used in the creation of this manuscript.
All claims expressed in this article are solely those of the authors and do not necessarily represent those of their affiliated organizations, or those of the publisher, the editors and the reviewers. Any product that may be evaluated in this article, or claim that may be made by its manufacturer, is not guaranteed or endorsed by the publisher.
1. Docea, AO, Tsatsakis, A, Albulescu, D, Cristea, O, Zlatian, O, Vinceti, M, et al. A new threat from an old enemy: re-emergence of coronavirus (review). Int J Mol Med. (2020) 45:1631–43. doi: 10.3892/ijmm.2020.4555
2. Ellul, MA, Benjamin, L, Singh, B, Lant, S, Michael, BD, Easton, A, et al. Neurological associations of COVID-19. Lancet Neurol. (2020) 19:767–83. doi: 10.1016/S1474-4422(20)30221-0
3. World Health Organization. COVID-19 dashboard. World Health Organization. (2024). Available online at: https://data.who.int/dashboards/covid19/cases (Accessed July 22, 2023)
4. Huang, C, Wang, Y, Li, X, Ren, L, Zhao, J, Hu, Y, et al. Clinical features of patients infected with 2019 novel coronavirus in Wuhan, China. Lancet. (2020) 395:497–506. doi: 10.1016/S0140-6736(20)30183-5
5. Gasmi, A, Peana, M, Pivina, L, Srinath, S, Gasmi Benahmed, A, Semenova, Y, et al. Interrelations between COVID-19 and other disorders. Clin Immunol. (2021) 224:108651. doi: 10.1016/j.clim.2020.108651
6. Ahmad, T, Chaudhuri, R, Joshi, MC, Almatroudi, A, Rahmani, AH, and Ali, SM. COVID-19: the emerging Immunopathological determinants for recovery or death. Front Microbiol. (2020) 11:588409. doi: 10.3389/fmicb.2020.588409
7. Wu, Z, and McGoogan, JM. Characteristics of and important lessons from the coronavirus disease 2019 (COVID-19) outbreak in China: summary of a report of 72 314 cases from the Chinese Center for Disease Control and Prevention. JAMA. (2020) 323:1239–42. doi: 10.1001/jama.2020.2648
8. Liu, T, Zhang, J, Yang, Y, Ma, H, Li, Z, Zhang, J, et al. The role of interleukin-6 in monitoring severe case of coronavirus disease 2019. EMBO Mol Med. (2020) 12:e12421. doi: 10.15252/emmm.202012421
9. Tan, L, Wang, Q, Zhang, D, Ding, J, Huang, Q, Tang, YQ, et al. Lymphopenia predicts disease severity of COVID-19: a descriptive and predictive study. Signal Transduct Target Ther. (2020) 5:33. doi: 10.1038/s41392-020-0148-4
10. Gibson, PG, Qin, L, and Puah, SH. COVID-19 acute respiratory distress syndrome (ARDS): clinical features and differences from typical pre-COVID-19 ARDS. Med J Aust. (2020) 213:54–56.e1. doi: 10.5694/mja2.50674
11. Chen, Z, and John, WE. T cell responses in patients with COVID-19. Nat Rev Immunol. (2020) 20:529–36. doi: 10.1038/s41577-020-0402-6
12. Berlin, DA, Gulick, RM, and Martinez, FJ. Severe Covid-19. N Engl J Med. (2020) 383:2451–60. doi: 10.1056/NEJMcp2009575
13. Bechmann, N, Barthel, A, Schedl, A, Herzig, S, Varga, Z, Gebhard, C, et al. Sexual dimorphism in COVID-19: potential clinical and public health implications. Lancet Diabetes Endocrinol. (2022) 10:221–30. doi: 10.1016/S2213-8587(21)00346-6
14. Doğan, A, Dumanoğlu Doğan, İ, Uyanık, M, Köle, MT, and Pişmişoğlu, K. The clinical significance of vitamin D and zinc levels with respect to immune response in COVID-19 positive children. J Trop Pediatr. (2022) 68:fmac072. doi: 10.1093/tropej/fmac072
15. Galmés, S, Serra, F, and Palou, A. Current state of evidence: influence of nutritional and nutrigenetic factors on immunity in the COVID-19 pandemic framework. Nutrients. (2020) 12:2738. doi: 10.3390/nu12092738
16. Du Laing, G, Petrovic, M, Lachat, C, De Boevre, M, Klingenberg, GJ, Sun, Q, et al. Course and survival of COVID-19 patients with comorbidities in relation to the trace element status at hospital admission. Nutrients. (2021) 13:3304. doi: 10.3390/nu13103304
17. Sinopoli, A, Sciurti, A, Isonne, C, Santoro, MM, and Baccolini, V. The efficacy of multivitamin, vitamin a, vitamin B, vitamin C, and vitamin D supplements in the prevention and management of COVID-19 and Long-COVID: an updated systematic review and Meta-analysis of randomized clinical trials. Nutrients. (2024) 16:1345. doi: 10.3390/nu16091345
18. Notz, Q, Herrmann, J, Schlesinger, T, Helmer, P, Sudowe, S, Sun, Q, et al. Clinical significance of micronutrient supplementation in critically ill COVID-19 patients with severe ARDS. Nutrients. (2021) 13:2113. doi: 10.3390/nu13062113
19. Alexander, J, Tinkov, A, Strand, TA, Alehagen, U, Skalny, A, and Aaseth, J. Early nutritional interventions with zinc, selenium and vitamin D for raising anti-viral resistance against progressive COVID-19. Nutrients. (2020) 12:2358. doi: 10.3390/nu12082358
20. Wessels, I, Rolles, B, and Rink, L. The potential impact of zinc supplementation on COVID-19 pathogenesis. Front Immunol. (2020) 11:1712. doi: 10.3389/fimmu.2020.01712
21. Kinash, M, Boyarchuk, O, and Dobrovolska, L. Zinc: its impact on immune function in children. Pediatria Polska. (2021) 96:263–9. doi: 10.5114/polp.2021.112401
22. Gammoh, NZ, and Rink, L. Zinc in infection and inflammation. Nutrients. (2017) 9:624. doi: 10.3390/nu9060624
23. Wessells, KR, and Brown, KH. Estimating the global prevalence of zinc deficiency: results based on zinc availability in national food supplies and the prevalence of stunting. PLoS One. (2012) 7:e50568. doi: 10.1371/journal.pone.0050568
24. Beck, FW, Prasad, AS, Kaplan, J, Fitzgerald, JT, and Brewer, GJ. Changes in cytokine production and T cell subpopulations in experimentally induced zinc-deficient humans. Am J Phys. (1997) 272:E1002–7. doi: 10.1152/ajpendo.1997.272.6.E1002
25. Jarosz, M, Olbert, M, Wyszogrodzka, G, Młyniec, K, and Librowski, T. Antioxidant and anti-inflammatory effects of zinc Zinc-dependent NF-κB signaling. Inflammopharmacology. (2017) 25:11–24. doi: 10.1007/s10787-017-0309-4
26. Wessels, I, Haase, H, Engelhardt, G, Rink, L, and Uciechowski, P. Zinc deficiency induces production of the proinflammatory cytokines IL-1β and TNFα in promyeloid cells via epigenetic and redox-dependent mechanisms. J Nutr Biochem. (2013) 24:289–97. doi: 10.1016/j.jnutbio.2012.06.007
27. Hasan, R, Rink, L, and Haase, H. Chelation of free Zn2+ impairs chemotaxis, phagocytosis, oxidative burst, degranulation, and cytokine production by neutrophil granulocytes. Biol Trace Elem Res. (2016) 171:79–88. doi: 10.1007/s12011-015-0515-0
28. Singh, M, and Das, RR. WITHDRAWN: zinc for the common cold. Cochrane Database Syst Rev. (2015) 2015:CD001364. doi: 10.1002/14651858.CD001364.pub5
29. Kartasurya, MI, Ahmed, F, Subagio, HW, Rahfiludin, MZ, and Marks, GC. Zinc combined with vitamin a reduces upper respiratory tract infection morbidity in a randomised trial in preschool children in Indonesia. Br J Nutr. (2012) 108:2251–60. doi: 10.1017/S0007114512000499
30. Vogel-González, M, Talló-Parra, M, Herrera-Fernández, V, Pérez-Vilaró, G, Chillón, M, Nogués, X, et al. Low zinc levels at admission associates with poor clinical outcomes in SARS-CoV-2 infection. Nutrients. (2021) 13:562. doi: 10.3390/nu13020562
31. Chen, KY, Lin, CK, and Chen, NH. Effects of vitamin D and zinc deficiency in acute and long COVID syndrome. J Trace Elem Med Biol. (2023) 80:127278. doi: 10.1016/j.jtemb.2023.127278
32. Yao, JS, Paguio, JA, Dee, EC, Tan, HC, Moulick, A, Milazzo, C, et al. The minimal effect of zinc on the survival of hospitalized patients with Covid-19: an observational study. Chest. (2020) 159:108–11. doi: 10.1016/j.chest.2020.06.082
33. Ekemen Keleş, Y, Yılmaz Çiftdoğan, D, Çolak, A, Kara Aksay, A, Üstündag, G, Şahin, A, et al. Serum zinc levels in pediatric patients with COVID-19. Eur J Pediatr. (2022) 181:1575–84. doi: 10.1007/s00431-021-04348-w
34. Ibrahim Alhajjaji, G, Alotaibi, N, Abutaleb, N, Alotaibi, MM, Alhajjaji, A, Alotaibi, AS, et al. Effect of zinc supplementation on symptom reduction and length of hospital stay among pediatric patients with coronavirus disease 2019 (COVID-19). Saudi Pharm J. (2023) 31:585–91. doi: 10.1016/j.jsps.2023.02.011
35. World Health Organization. Clinical management of COVID-19: interim guidance (2020). Available online at: https://iris.who.int/handle/10665/332196 (Accessed May 27, 2020).
36. Suter, PM, and Russell, RM. Vitamin and trace mineral deficiency and excess In: J Jameson, AS Fauci, DL Kasper, SL Hauser, DL Longo, and J Loscalzo, editors. Harrison’s principles of internal medicine, 20e. New York: McGraw-Hill Education (2018)
37. Jothimani, D, Kailasam, E, Danielraj, S, Nallathambi, B, Ramachandran, H, Sekar, P, et al. COVID-19: poor outcomes in patients with zinc deficiency. Int J Infect Dis. (2020) 100:343–9. doi: 10.1016/j.ijid.2020.09.014
38. Kim, L, Whitaker, M, O’Halloran, A, Kambhampati, A, and Chai, SJ. COVID-NET surveillance team, et al. hospitalization rates and characteristics of children aged <18 years hospitalized with laboratory-confirmed COVID-19 - COVID-NET, 14 states, march 1-July 25, 2020. MMWR Morb Mortal Wkly Rep. (2020) 69:1081–8. doi: 10.15585/mmwr.mm6932e3
39. Kvamme, JM, Grønli, O, Jacobsen, BK, and Florholmen, J. Risk of malnutrition and zinc deficiency in community-living elderly men and women: the Tromsø study. Public Health Nutr. (2015) 18:1907–13. doi: 10.1017/S1368980014002420
40. Sanjeevi, N, Freeland-Graves, J, Beretvas, SN, and Sachdev, PK. Trace element status in type 2 diabetes: a meta-analysis. J Clin Diagn Res. (2018) 12:OE01–8. doi: 10.7860/JCDR/2018/35026.11541
41. Astrup, A, and Bügel, S. Overfed but undernourished: recognizing nutritional inadequacies/deficiencies in patients with overweight or obesity. Int J Obes. (2019) 43:219–32. doi: 10.1038/s41366-018-0143-9
42. Hernández-Mendoza, H, Martínez-Navarro, I, Hernández-Ochoa, E, Espinoza-Ruiz, M, Lugo-Trampe, A, Trujillo-Murillo, KDC, et al. Serum zinc levels are associated with obesity and low-density lipoprotein cholesterol in Mexican adults. J Trace Elem Med Biol. (2022) 73:127002. doi: 10.1016/j.jtemb.2022.127002
43. Rios-Lugo, MJ, Madrigal-Arellano, C, Gaytán-Hernández, D, Hernández-Mendoza, H, and Romero-Guzmán, ET. Association of Serum Zinc Levels in overweight and obesity. Biol Trace Elem Res. (2020) 198:51–7. doi: 10.1007/s12011-020-02060-8
44. Syed, M, Siddiqui, S, Ahmad, S, and Syed, K. Assessing the link between zinc levels and obesity in young adults: zinc levels and obesity in young adults. Med J South Punjab. (2023) 4:76–82. doi: 10.61581/MJSP.VOL04/01/09
45. Payahoo, L, Ostadrahimi, A, Mobasseri, M, Khaje Bishak, Y, Farrin, N, Asghari Jafarabadi, M, et al. Effects of zinc supplementation on the anthropometric measurements, lipid profiles and fasting blood glucose in the healthy obese adults. Adv Pharm Bull. (2013) 3:161–5. doi: 10.5681/apb.2013.027
46. Gunanti, IR, Al-Mamun, A, Schubert, L, and Long, KZ. The effect of zinc supplementation on body composition and hormone levels related to adiposity among children: a systematic review. Public Health Nutr. (2016) 19:2924–39. doi: 10.1017/S1368980016001154
47. Zaky, D, Sultan, EA, Salim, MF, and Dawod, RS. Zinc level and obesity. Egypt J Internal Med. (2013) 25:209–12. doi: 10.4103/1110-7782.124985
48. Ennes Dourado Ferro, F, de Sousa Lima, VB, Mello Soares, NR, Franciscato Cozzolino, SM, and do Nascimento Marreiro, D. Biomarkers of metabolic syndrome and its relationship with the zinc nutritional status in obese women. Nutr Hosp. (2011) 26:650–4. doi: 10.1590/S0212-16112011000300032
49. Yasui, Y, Yasui, H, Suzuki, K, Saitou, T, Yamamoto, Y, Ishizaka, T, et al. Analysis of the predictive factors for a critical illness of COVID-19 during treatment - relationship between serum zinc level and critical illness of COVID-19. Int J Infect Dis. (2020) 100:230–6. doi: 10.1016/j.ijid.2020.09.008
50. Fujita, K, Ocho, K, Kadowaki, T, Yorifuji, T, Hagiya, H, and Otsuka, F. Zinc deficiency is a potential risk factor for COVID-19 progression to pneumonia requiring oxygen therapy. J Infect Chemother. (2024) 30:978–82. doi: 10.1016/j.jiac.2024.03.007
51. Razeghi Jahromi, S, Moradi Tabriz, H, Togha, M, Ariyanfar, S, Ghorbani, Z, Naeeni, S, et al. The correlation between serum selenium, zinc, and COVID-19 severity: an observational study. BMC Infect Dis. (2021) 21:899. doi: 10.1186/s12879-021-06617-3
52. Maares, M, Hackler, J, Haupt, A, Heller, RA, Bachmann, M, Diegmann, J, et al. Free zinc as a predictive marker for COVID-19 mortality risk. Nutrients. (2022) 14:1407. doi: 10.3390/nu14071407
53. Fromonot, J, Gette, M, Ben Lassoued, A, Guéant, JL, Guéant-Rodriguez, RM, and Guieu, R. Hypozincemia in the early stage of COVID-19 is associated with an increased risk of severe COVID-19. Clin Nutr. (2022) 41:3115–9. doi: 10.1016/j.clnu.2021.04.042
54. Zhou, S, Zhang, F, Chen, F, Li, P, He, Y, Wu, J, et al. Micronutrient level is negatively correlated with the neutrophil-lymphocyte ratio in patients with severe COVID-19. Int J Clin Pract. (2022) 2022:6498794–8. doi: 10.1155/2022/6498794
55. Wang, J, Jiang, M, Chen, X, and Montaner, LJ. Cytokine storm and leukocyte changes in mild versus severe SARS-CoV-2 infection: review of 3939 COVID-19 patients in China and emerging pathogenesis and therapy concepts. J Leukoc Biol. (2020) 108:17–41. doi: 10.1002/JLB.3COVR0520-272R
56. Singh, K, Mittal, S, Gollapudi, S, Butzmann, A, Kumar, J, and Ohgami, RS. A meta-analysis of SARS-CoV-2 patients identifies the combinatorial significance of D-dimer, C-reactive protein, lymphocyte, and neutrophil values as a predictor of disease severity. Int J Lab Hematol. (2021) 43:324–8. doi: 10.1111/ijlh.13354
57. McElvaney, O, McEvoy, N, McElvaney, O, Carroll, T, Murphy, M, Dunlea, D, et al. Characterization of the inflammatory response to severe COVID-19 illness. Am J Respir Crit Care Med. (2020) 202:812–21. doi: 10.1164/rccm.202005-1583OC
58. Almasaud, AS, Chalabi, J, Arfaj, AA, Qarni, AA, Alkroud, A, Nagoor, Z, et al. Association of Serum Zinc and Inflammatory Markers with the severity of COVID-19 infection in adult patients. Nutrients. (2023) 15:340. doi: 10.3390/nu15020340
59. Prasad, AS. Zinc in human health: effect of zinc on immune cells. Mol Med. (2008) 14:353–7. doi: 10.2119/2008-00033.Prasad
60. Read, SA, Obeid, S, Ahlenstiel, C, and Ahlenstiel, G. The role of zinc in antiviral immunity. Adv Nutr. (2019) 10:696–710. doi: 10.1093/advances/nmz013
61. Bao, B, Prasad, AS, Beck, FW, and Godmere, M. Zinc modulates mRNA levels of cytokines. Am J Physiol Endocrinol Metab. (2003) 285:E1095–102. doi: 10.1152/ajpendo.00545.2002
62. Haase, H, and Rink, L. The immune system and the impact of zinc during aging. Immun Ageing. (2009) 6:9. doi: 10.1186/1742-4933-6-9
63. Vu, TT, Fredenburgh, JC, and Weitz, JI. Zinc: an important cofactor in haemostasis and thrombosis. Thromb Haemost. (2013) 109:421–30. doi: 10.1160/TH12-07-0465
64. Boyarchuk, O, Perestiuk, V, Kosovska, T, and Volianska, L. Coagulation profile in hospitalized children with COVID-19: pediatric age dependency and its impact on long COVID development. Front Immunol. (2024) 15:1363410. doi: 10.3389/fimmu.2024.1363410
65. Hedegaard, CV, Soerensen, MD, Jørgensen, LH, and Schaffalitzky de Muckadell, OB. Investigating hypozincemia and validity of plasma zinc measurements in infected patients. Scand J Clin Lab Invest. (2022) 82:371–7. doi: 10.1080/00365513.2022.2114935
66. McDonald, CM, Suchdev, PS, Krebs, NF, Hess, SY, Wessells, KR, Ismaily, S, et al. Adjusting plasma or serum zinc concentrations for inflammation: biomarkers reflecting inflammation and nutritional determinants of Anemia (BRINDA) project. Am J Clin Nutr. (2020) 111:927–37. doi: 10.1093/ajcn/nqz304
Keywords: COVID-19, SARS-CoV-2 infection, zinc, children, adolescents, CRP, inflammation
Citation: Perestiuk V, Kosovska T, Volianska L and Boyarchuk O (2025) Association of zinc deficiency and clinical symptoms, inflammatory markers, severity of COVID-19 in hospitalized children. Front. Nutr. 12:1566505. doi: 10.3389/fnut.2025.1566505
Received: 24 January 2025; Accepted: 11 March 2025;
Published: 25 March 2025.
Edited by:
Miguel Angel Prieto Lage, University of Vigo, SpainReviewed by:
Pauline Donn, University of Yaounde I, CameroonCopyright © 2025 Perestiuk, Kosovska, Volianska and Boyarchuk. This is an open-access article distributed under the terms of the Creative Commons Attribution License (CC BY). The use, distribution or reproduction in other forums is permitted, provided the original author(s) and the copyright owner(s) are credited and that the original publication in this journal is cited, in accordance with accepted academic practice. No use, distribution or reproduction is permitted which does not comply with these terms.
*Correspondence: Vita Perestiuk, cGVyZXN0aXVrX3ZvQHRkbXUuZWR1LnVh
Disclaimer: All claims expressed in this article are solely those of the authors and do not necessarily represent those of their affiliated organizations, or those of the publisher, the editors and the reviewers. Any product that may be evaluated in this article or claim that may be made by its manufacturer is not guaranteed or endorsed by the publisher.
Research integrity at Frontiers
Learn more about the work of our research integrity team to safeguard the quality of each article we publish.