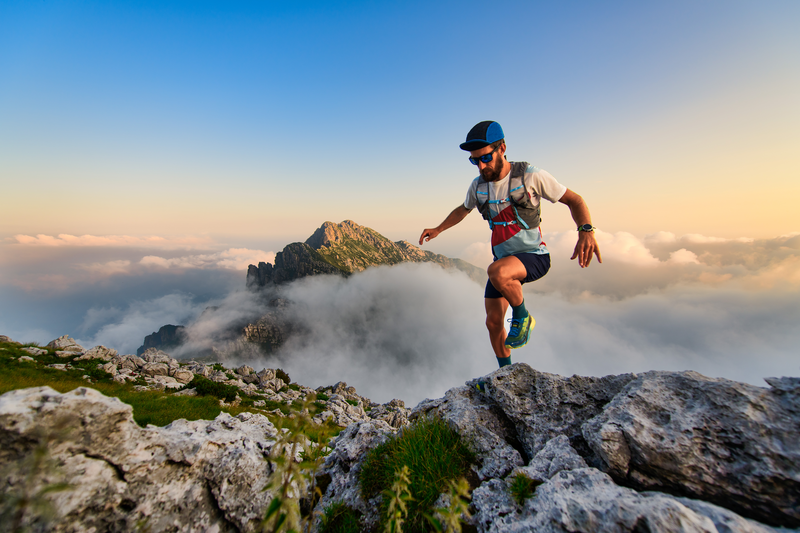
94% of researchers rate our articles as excellent or good
Learn more about the work of our research integrity team to safeguard the quality of each article we publish.
Find out more
REVIEW article
Front. Nutr.
Sec. Nutritional Immunology
Volume 12 - 2025 | doi: 10.3389/fnut.2025.1561119
This article is part of the Research Topic Dietary Bioactive Compounds as Coadjuvants in Antiviral Preventive and Curative Strategies View all 3 articles
The final, formatted version of the article will be published soon.
You have multiple emails registered with Frontiers:
Please enter your email address:
If you already have an account, please login
You don't have a Frontiers account ? You can register here
Marine polysaccharides, particularly those derived from red, brown, and green algae, have shown promising antiviral activity. Among them, sulfated polysaccharides are particularly notable due to their broad-spectrum antiviral properties. These include direct viral destruction, inhibition of virus adsorption, disruption of viral transcription and replication, and the stimulation of the host's antiviral immunity. With low toxicity, minimal drug resistance, and excellent biocompatibility, these polysaccharides represent promising candidates for the development of antiviral medications. For instance, carrageenan, a polysaccharide from red algae, and fucoidan, a polymer from brown algae, have both been proven to effectively inhibit viral infections. Sulfated polysaccharides from green algae, such as those found in Ulva species, also exhibit antiviral properties, including activity against the Japanese encephalitis virus. These polysaccharides function by blocking the attachment of viruses to host cells or interfering with various stages of the viral life cycle. Moreover, marine polysaccharides have been shown to enhance host immune responses, thereby aiding in viral clearance. Although these findings highlight the antiviral potential of marine polysaccharides, most studies have been conducted in vitro or in animal models. Further clinical trials are necessary to validate their effectiveness and safety for therapeutic use.
Keywords: Marine polysaccharides1, Sulfated polysaccharides2, virus3, Antiviral mechanisms4, algae
Received: 16 Jan 2025; Accepted: 12 Mar 2025.
Copyright: © 2025 Dong, Qiu, Jia, Wu, Nie, Wen, Zhao and Zhai. This is an open-access article distributed under the terms of the Creative Commons Attribution License (CC BY). The use, distribution or reproduction in other forums is permitted, provided the original author(s) or licensor are credited and that the original publication in this journal is cited, in accordance with accepted academic practice. No use, distribution or reproduction is permitted which does not comply with these terms.
* Correspondence:
Chao Zhao, Fujian Agriculture and Forestry University, Fuzhou, China
Yongzhen Zhai, China Medical University, Shenyang, 110122, Liaoning Province, China
Disclaimer: All claims expressed in this article are solely those of the authors and do not necessarily represent those of their affiliated organizations, or those of the publisher, the editors and the reviewers. Any product that may be evaluated in this article or claim that may be made by its manufacturer is not guaranteed or endorsed by the publisher.
Research integrity at Frontiers
Learn more about the work of our research integrity team to safeguard the quality of each article we publish.